- 1Department of Agronomy, College of Agriculture, Chaudhary Charan Singh Haryana Agricultural University, Hisar, Haryana, India
- 2Regional Research Station, Chaudhary Charan Singh Haryana Agricultural University, Bawal, Haryana, India
- 3Regional Research Station, Chaudhary Charan Singh Haryana Agricultural University, Karnal, Haryana, India
- 4Department of Microbiology, College of Basic Sciences, Chaudhary Charan Singh Haryana Agricultural University, Hisar, Haryana, India
- 5Department of Plant Pathology, College of Agriculture, Chaudhary Charan Singh Haryana Agricultural University, Hisar, Haryana, India
In response to the degraded soil health and lack of improvement in the yield of rice–wheat cropping systems in South Asia’s Indo-Gangetic Plains, an experiment was formulated in a split-plot design. Four rice residue management practices were the primary factor, alongside two nitrogen levels (150 and 180 kg/ha) and two nitrogen split levels (two and three splits) as sub-treatments. The findings revealed a notable increase in soil organic carbon (SOC), microbial count, and enzymatic activity in plots subjected to conservation tillage and residue treatment compared to those in plots subjected to partial residue (anchored stubbles) and conventional methods (residue incorporated with chopping). The collective analysis demonstrated a significant influence of rice residue management practices and nitrogen application levels on wheat yield attributes and productivity. Specifically, zero tillage with full residue (unchopped) in wheat exhibited a 5.23% increase in grain yield compared to conventional tillage with full residue (chopped), concurrently boosting the soil microbial count by 19.80–25%, the diazotrophic count by 29.43–31.6%, and the actinomycete count by 20.15–32.99% compared with conventional tillage. Moreover, applying nitrogen in three splits (at sowing, before the 1st irrigation, and after the 1st irrigation) led to a 6.25% increase in grain yield than that in two splits (at sowing and after the 1st irrigation), significantly impacting wheat productivity in the soil. Furthermore, the zero tillage-happy seeder with full residue elevated dehydrogenase activity from 77.94 to 88.32 μg TPF/g soil/24 h during the study year, surpassing that in the conventional plot. This increase in enzymatic activity was paralleled by a robust positive correlation between the microbial population and enzymatic activity across various residue retention practices. In conclusion, the results underscore the efficacy of crop residue retention following conservation tillage, in tandem with nitrogen optimization and scheduling, in enhancing wheat yield within the rice–wheat cropping system.
1 Introduction
The rice–wheat cropping system (RWCS) constitutes a dominant agricultural paradigm in Asia, particularly in southeastern Asia, encompassing an expanse of approximately 24 mha (Nawaz et al., 2019). It occupies approximately 13.5 mha across South Asia’s Indo-Gangetic Plains (Padre et al., 2016). This cropping system holds paramount importance for the maintenance of livelihoods, food security, employment, and income generation for millions across Asia. Approximately 33 and 42% of the total acreage devoted to these crops are rice and wheat, respectively. In addition, it contributes significantly, at 25 and 33%, to aggregate rice–wheat production (Gupta et al., 2024). In northwestern India, the RWCS prevails as the principal cropping system, encompassing an area of approximately 12 mha (Bhatt et al., 2016).
On average, paddy cultivation yields approximately 5–6 tons of straw per hectare. It is estimated that Southeast Asian countries collectively generate 150 million metric tons of rice residues annually (Singh et al., 2021). The window between paddy harvesting and subsequent sowing of wheat, following field cleansing or paddy straw management, is markedly short, typically spanning 2–3 weeks (Meena et al., 2020), compelling farmers to resort to paddy straw burning. Paddy residue is assimilated through tillage post-partial incorporation/removal, facilitating expedited field preparation before seeding operations for the succeeding crop in the sequence (DOACFW, 2019). However, the RWCS faces numerous secondary challenges, including declining groundwater tables, deteriorating soil health, and diminished total factor productivity. One prospective strategy involves in situ management of paddy straw, encompassing direct drilling of wheat under no-till circumstances utilizing zero-tillage (ZT) machinery or a happy seeder (retention of residues on the surface). In addition, the incorporation of paddy straw into the soil can be achieved through conventional tillage (CT) machinery, such as harrows and rotavators, or straw chopping followed by mixing into the soil. Tillage is an energy-intensive farm operation which contributes to ~30% of the total energy use in crop production (Yadav et al., 2018). Retaining paddy straw as mulch on the surface of soil preserves soil moisture, moderates thermal regimes, enriches soil nutrient content, suppresses weed growth, and augments soil health, thereby fostering improved crop yields. Nitrogen (N) dynamics may undergo alteration due to ZT and surface residue retention, thereby necessitating nuanced N management strategies vis-à-vis residue burning and conventional tillage (Singh et al., 2015). The influx of crop residue-derived N is intricately linked with soil N management (Zhang et al., 2021). However, ZT combined with residue retention holds promise for augmenting long-term N availability to crops by elevating soil nitrogen levels and the labile nitrogen pool within upper soil strata (Sun et al., 2015). The implementation of farming systems that conserves soil and water through minimal soil disturbances and residue retention cover is one of the best management practices and improving the fertility status and microscopic population in degraded lands (Babu et al., 2023). In the last 60 years, the consumption of nitrogen fertilizer in India has grown rapidly. Emerging trends have resulted in escalating losses of nitrogen, posing significant threats to the quality of air, soil, and freshwater resources and consequently jeopardizing climate, ecosystems, and human health (Móring et al., 2021). Conservation agriculture increases available soil water, saves irrigation water, reduces heat and drought stresses, reduces GHG emissions, captures carbon, and improves soil health in the long term (Dhillon and Sohu, 2024).
Crop residues serve as nutrient-rich sources, liberating essential plant nutrients upon microbial decomposition in the soil. Consequently, reintegrating crop residues into soil, instead of incinerating them, serves to enhance several soil quality parameters. To withstand soil health within the RWCS in northwestern India, it is imperative to manage rice residue in a manner that is economically viable, environmentally sustainable, and logistically feasible. Generally, crop residues comprise approximately 40 to 45% of the carbon content, the restitution of which to the soil facilitates its utilization by soil microbes, thereby bolstering the soil organic matter (OM) content and mitigating organic carbon (OC) loss (Bin et al., 2021). Soil organic carbon (SOC) plays a pivotal role in shaping biological soil properties (Varatharajan et al., 2022). Microbial populations exert a profound influence on nutrient accessibility and contribute to the growth of various soil health indices. Consequently, understanding soil biological attributes is highly important for sustainability (Harish et al., 2022; Gopinath et al., 2022). Parameters such as total microbial count (TMC), actinomycetes, diazotrophs, and enzymatic activities, including dehydrogenase, alkaline phosphatase, and urease, serve as pivotal indicators of soil eminence (Doran and Zeiss, 2000). Soil-inhabiting microbes are central to nutrient release and soil purification. The inherent characteristics of soil, encompassing various soil classes, exert control over the soil microbiota, which in turn plays a pivotal role in modulating soil nutrient contents and rendering them bioavailable (Majumdar et al., 2024). The decomposition and biochemical transformation processes are expedited by soil enzymes, facilitating the release of nutrients from plants (Meena et al., 2022). Soil bacteria play a pivotal role in converting OM from its organic to accessible inorganic form, thereby facilitating the breakdown of OM (Six et al., 2004). Soil microorganisms are sensitive to changes in soil moisture as it affects the physiological state of microorganisms and plants which may lead to changes in their population in the soil (Gangmei et al., 2024). Recycling crop residues is imperative for reintegrating organic matter into the soil (Rajanna et al., 2022). Reduced tillage practices coupled with stubble retention promote the proliferation of diazotrophs, which is ascribed to reduced soil composition, fostering an optimal soil pore network conducive to interactions between stubble decomposers and nitrogen-fixing organisms (Gupta et al., 2019; Li et al., 2021). In the short term, applying cereal residues may require higher levels of fertilizer N to account for N immobilization, compared to not using residues (Sharma et al., 2021). However, over the long term, returning crop residues can lead to a net build-up of readily mineralized soil organic N, potentially reducing crops’ fertilizer N requirements (Jat et al., 2019). The amount of N loss due to volatilization increases when the food–water pH and temperature are favorable (Hayashi et al., 2008). Broadcast application of PU results in higher food–water NH4 + -N compared to deep placement of N (Huda et al., 2016; Islam et al., 2016; Islam et al., 2018; Liu et al., 2015), which increases N loss through NH3 volatilization. Excessive use of N fertilizer through broadcast application has negative environmental consequences, including N2O and NO emissions, nitrate pollution of groundwater, and eutrophication (Savant and Stangel, 1990).
The initial yellowing of upper wheat leaves observed under mulched conditions is associated with a reduction in soil temperature (minimum) during the late January period of 2020 and 2021, which is attributable to the insulating effect of mulch on the soil (Chaudhary et al., 2023). Consequently, a standard shift in agronomic practices concerning rice straw management is warranted to enhance resource utilization efficiency and system productivity. We hypothesized that combined application of N@150 kg/ha in 3 splits at basal, before, and after 1st irrigation and residue incorporation improve productivity of wheat. Hence, the present study aimed to explore the timing, number of splits, and nitrogen application rates of wheat crops to achieve improved productivity, microbial dynamics, and enzymatic activities and optimal nitrogen management in the northwestern Indian Plains. Given the circumstances within the RWCS, there is an urgent need for systematic research endeavors aimed at evaluating diverse nitrogen dosages and scheduling regimes to optimize productivity.
2 Materials and methods
2.1 Experimental site description
The experiment was conducted at CCS Haryana Agricultural University’s Regional Research Station in Karnal, India [290 43′ 41″ North and 760 58′ 50″ East]. The soil was sandy loam with proportions of sand, silt, and clay (Table 1). Prior to starting the study, soil was sampled from the entire experimental field at five locations at 0 to 15 cm depth, the samples were mixed thoroughly, the bulk was reduced to approximately 1 kg by quartering, and then the samples were analyzed. The initial pH of the soil was correlated with the electrical conductivity (EC), bulk density, soil organic carbon (SOC), KMnO4 oxidizable N, NaHCO3 extractable phosphorus (P), and 1.0 N NH4OAc exchangeable potassium (K) and is shown in Table 1.
2.2 Experimental details and field management
In 2019–2020 and 2020–2021, field experiments comprised of four main-plot treatments, viz., Zero tillage wheat-Happy Seeder (ZTW-HS) with full residue (chopped), ZTW-HS with full residue (unchopped), ZTW-HS with partial residues (anchored stubbles), and conventional tillage wheat-drill sown (CTW-DS) with full residue (chopped), and six subplots with two N levels, viz., 150 and 180 kg/ha, applied into 2 (at sowing and after 1st irrigation) and 3 splits, that is, at sowing, before 1st irrigation, after 1st irrigation and at sowing, after 1st irrigation, after 2nd irrigation (Table 2). The hypothesis behind the two nitrogen levels was that N@150 kg/ha is the state-recommended practice for conventional sown wheat, and a higher dose of N@180 kg/ha is used because there is yellowing of plants during the initial days in zero-tillage wheat because of the nitrogen used by microbes for the decomposition of rice residues, which leads to nitrogen immobilization. In zero tillage, sowing was performed by a Happy Seeder, which is a machine towed on a tractor that cuts paddy stubble, lifts it, sows wheat seeds into the soil, and covers the sown area with straw as mulch. The individual plot size was 6 m × 2.2 m, totaling 13.2 m2, and the treatment details are summarized in Table 2. To remove the residue (M3), loose straw was manually collected, and the remaining anchored stubble was left in the field. Full residue loose straw was uniformly spread and chopped using a chopper-cum-spreader machine before wheat crop planting (M1). In the M4 treatment, loose straw from the full residue was uniformly spread and chopped using a chopper-cum-spreader machine, and then, a rotavator was used to mix the chopped residue in the soil. Presowing irrigation at a depth of 6 cm through an irrigation channel preceded wheat sowing. In ZT plots with full residue (chopped or unchopped), seeding was performed using a happy seeder, and the seeds were directly sown into chopped or loose stubble residues (M1, M2) and seed-cum-fertilizer drill machine was used for sowing conventional plots. All the operations were conducted 1–2 days prior to sowing. Rice variety HKR-47 was transplanted on 1st July and harvested on 25th October and 22nd October throughout the Kharif seasons of 2019 and 2020, respectively. Cultivation practices followed the university’s package of practices. Wheat sowing in the ZT plots utilized a happy seeder, while the plants in the CT plots with residues were drill-sown. Wheat variety HD-2967 was sown 2019 and 12th November 2020, and the seed rate was 100 kg/ha. Recommended fertilizer doses of phosphorus (60 kg P2O5/ha) and nitrogen (150 and 180 kg N/ha) were applied in both growing seasons, that is, 2019 and 2020, using urea and DAP as nitrogen and phosphorus sources, respectively. The herbicide mesosulfuron (12 g/ha) + iodosulfuron (2.4 g/ha) was applied 35 days after sowing (DAS) for weed control in 500 L/ha of water via a knapsack sprayer with a flat fan nozzle.
2.3 Soil physicochemical properties
Soil was sampled from three pots in each plot at 0 to 15 cm soil depth after wheat crop harvesting in 2020–2021 to determine the impact of rice residue management. These samples were properly processed before the analysis, including all the processes, that is, drying, grinding, and sieving. SOC (%) (Walkley and Black, 1934), available N (Subbiah and Asija, 1956), available P (Olsen et al., 1954), available K (Jackson, 1967), and soil moisture content (Su et al., 2014) were determined 105°C until constant weight is obtained and dry weight of the sample is recorded.
2.4 Soil biological properties
2.4.1 Bacterial activity analysis
To assess the soil biological properties, soil samples were collected from three locations within each plot after last season crop harvesting. Microbial counts were determined on various agar-based media by using the standard serial dilution plate assay (Ben-David and Davidson, 2014). Serial dilutions were prepared from 10 g soil samples in 90 mL sterile water, followed by incubation of the Petri plates at 28 ± 2°C for 2 to 6 days in a BOD incubator. The colony-forming units (CFUs) were enumerated and are expressed as dry soil/gram (Wright et al., 1933).
2.4.2 Enzymatic activity analysis
For dehydrogenase enzyme activity, soil samples (1 g each) were incubated with 0.2 mL of 2,3,5-triphenyl tetrazolium chloride (TTC, 3% w/v) and 0.5 mL of glucose solution (1% w/v) at 28 ± 2°C for 24 h (Casida et al., 1964). The absorbance at 485 nm was measured after extraction with methanol and filtration. Enzyme activity was quantified using a TPF standard curve.
The Tabatabai and Bremner (1969) method was used to determine soil alkaline phosphatase activity. The yellow color formed by adding p-nitrophenyl phosphate solution was measured at 420 nm and is expressed as μg p-nitrophenol released/g/soil/h.
The Bremner and Douglas (1971) method was used to determine urease activity. Soil samples (5 g) were incubated with 5 mL of 2,000 ppm urea solution at 37°C for 7 h. After adding 2 M KCl-PMA, the solution was filtered and analyzed for urea content. Enzyme activity was calculated from a standard curve and expressed as μg urea/g dry soil/min.
2.5 Statistical analysis
The data were analyzed by using two-way ANOVA to assess the effects of different treatments. Significant differences between treatments were determined using Duncan’s multiple range test (DMRT) in conjunction with standard error of the mean (SEM ±) and least significant difference (LSD) computations (Gomez and Gomez, 1984).
3 Results
3.1 Impact of rice residue management practices and nutrient scheduling on soil physicochemical properties
The impact of rice residue management on soil chemical properties was investigated, with a particular focus on sowing methods and treatments with rice residues during the 2020–2021 cropping season, as depicted in Table 3. Significant levels of soil organic carbon (OC) were observed among the various treatments at the final wheat harvest stage. Notably, during the initial phase of the experiment, the effects were deemed non-significant. However, in 2020–2021, treatments such as M1 (0.41%) and M2 (0.42%) exhibited higher OC levels than did M3, which had an OC content of 0.37%. In addition, the OC content of M4 was notably lower at 0.32%. Intriguingly, the results were not significantly influenced by the nitrogen dose or scheduling treatments applied during either year.
According to the data, varying nitrogen levels did not exert a significant impact on the soil organic carbon content. However, it is noteworthy that a higher numerical value was observed when a high dose of nitrogen was administered.
3.2 Impact of rice residue management practices and nutrient scheduling on soil moisture content
The investigation revealed a discernible pattern of increasing soil moisture content as the cropping season progressed, as detailed in Table 4. Across both years of observation, treatments featuring M1 consistently exhibited SMC (%) at 75 days after sowing (DAS) and at maturity that was statistically equivalent to 13.91–15.25% in 2019–2020 and 17.88–20.34% in 2020–2021, respectively. These values were significantly greater than those in M3. Conversely, M4 yielded notably lower soil moisture contents (%), with values of 12.22 and 12.45% at 75 DAS and 16.34 and 18.67% at maturity in 2019–2020 and 2020–2021, respectively.
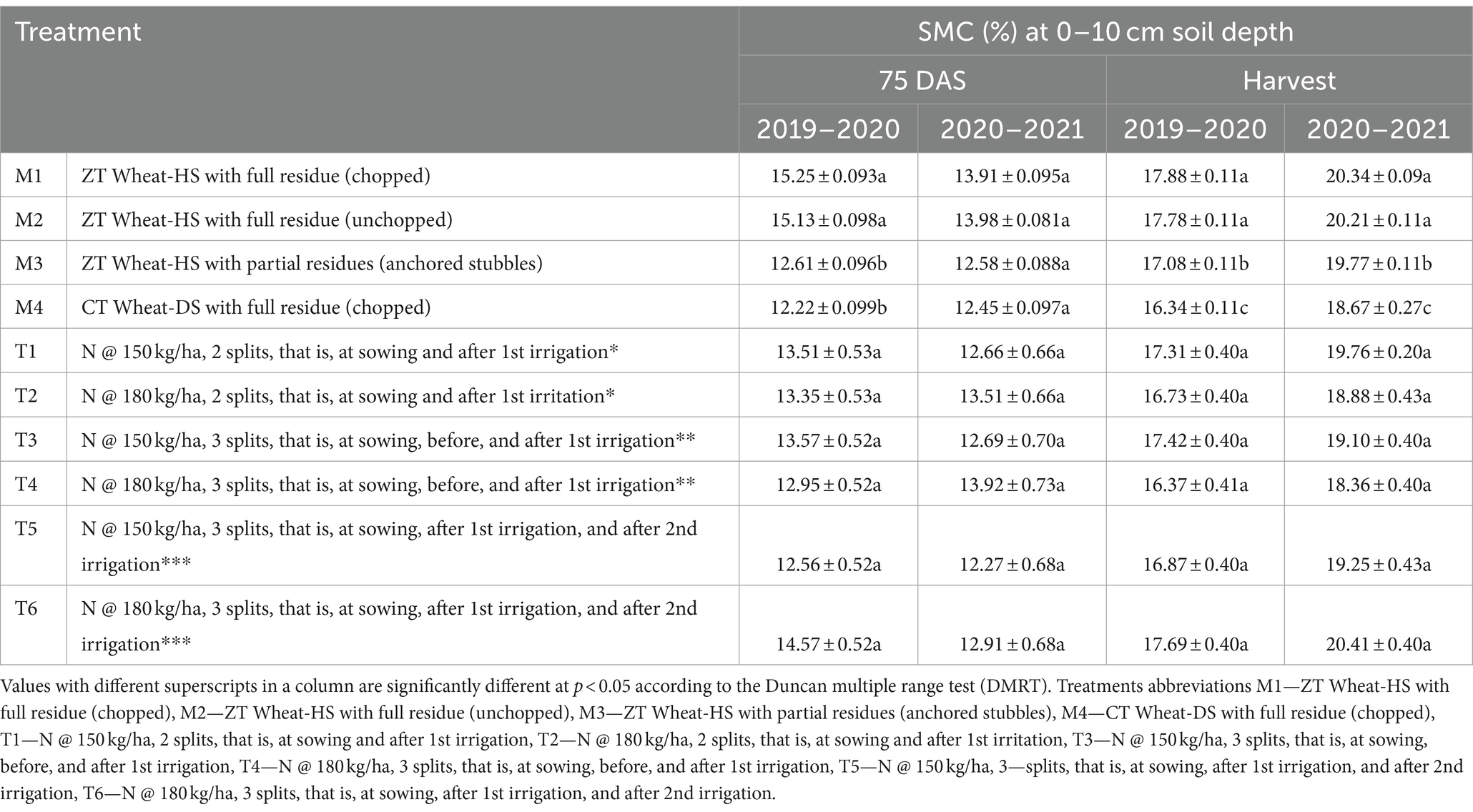
Table 4. Effect of rice residue practices, wheat crop establishment methods, and nutrient scheduling on soil moisture content (%) (SMC) in wheat crop under rice–wheat cropping system (2019–2020 and 2020–2021).
Furthermore, the subplot analysis indicated a non-significant difference in soil moisture content (%) across treatments, underscoring the consistent influence of rice residue practices on soil moisture dynamics throughout the cropping season.
3.3 Impact of rice residue management practices and nutrient scheduling on microbial count
Microbial activity serves as the primary catalyst driving decomposition processes within the soil matrix. Notably, the management of rice crop residues in wheat cultivation significantly influenced the soil microbial populations throughout the study, as shown in Table 5. Soil samples were meticulously analyzed at two critical crop growth stages: 75 days after sowing (DAS) and at harvest. Remarkably, the microbial count was markedly greater at 75 DAS than at harvest, underscoring the dynamic nature of microbial populations throughout the cropping season. In addition, the second year of the study, specifically 2020–2021, yielded higher microbial counts than did the preceding year. Across both years, M1 consistently had greater microbial counts: 93.42 at 75 DAS and 88.09 × 107 cfu/g dry soil at harvest in 2019–2020; 98.53 at 75 DAS and 88.52 × 107 cfu/g dry soil at harvest in 2020–2021. Conversely, M4 exhibited lower counts: 78.76 at 75 DAS and 66.06 × 107 cfu/g dry soil at harvest in 2019–2020; 66.06 at 75 DAS and 70.99 × 107 cfu/g dry soil at harvest in 2020–2021. Moreover, within subplots defined by nitrogen dose and scheduling, no significant differences were observed in the total microbial count. Notably, in the T4 treatment, the total microbial counts at 75 DAS (89.44 in 2019–2020 and 96.63 × 107 cfu/g dry soil in 2020–2021) and at harvest (79.73 in 2019–2020 and 83.06 × 107 cfu/g dry soil in 2020–2021) were greater than those in the T1 treatment (Table 5).
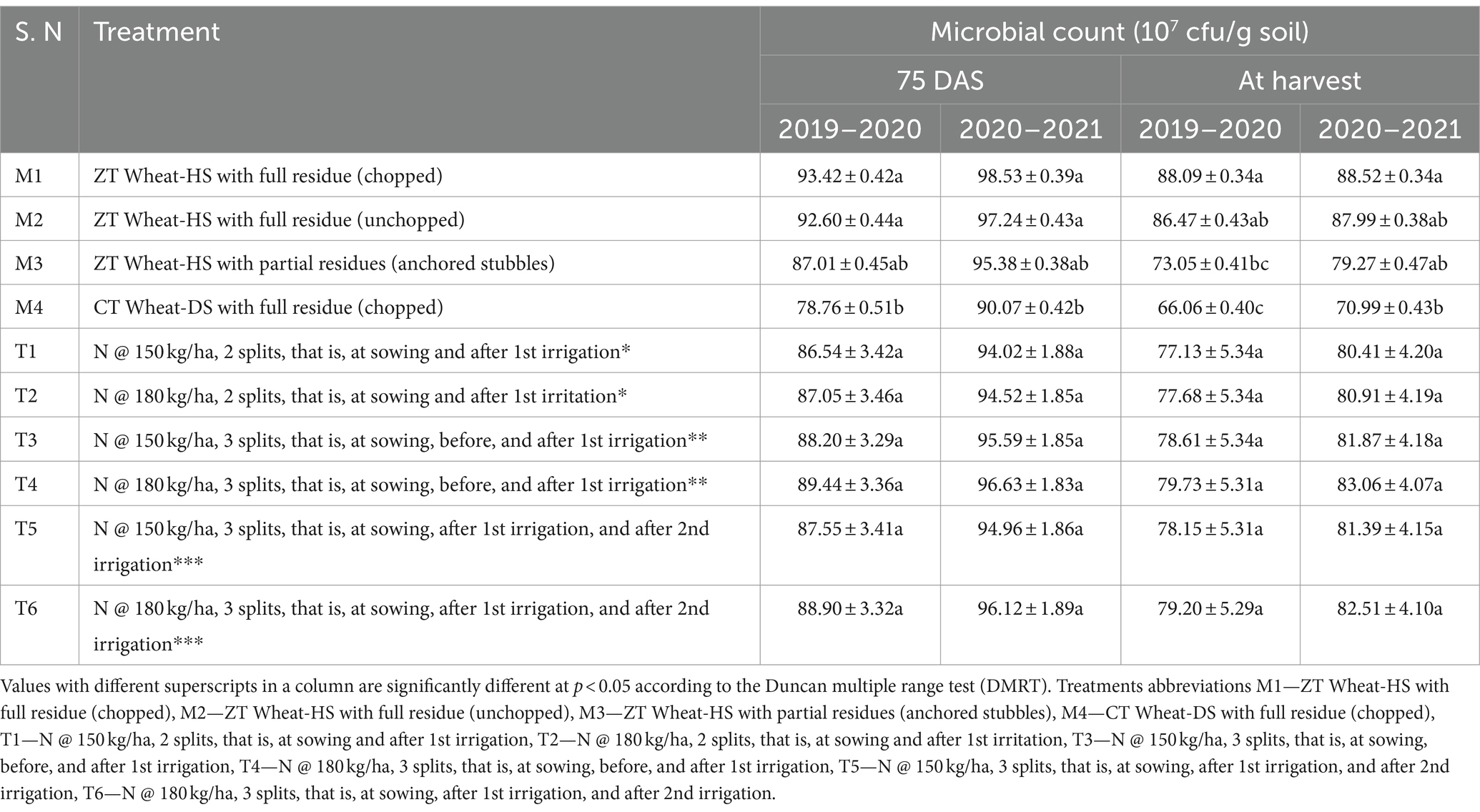
Table 5. Effect of crop establishment methods, rice residue practices, and nutrient scheduling on soil microbial count under rice–wheat cropping system (2019–2020 and 2020–2021).
Throughout both years of observation, treatments involving M1 consistently exhibited greater diazotrophic counts at 75 days after sowing (DAS) and at harvest, comparable to those of M2 and significantly greater than those of M3 (Table 6). Specifically, the diazotrophic counts for M1 were 64.49 in 2019–2020 and 88.52 × 104 cfu/g dry soil in 2020–2021 at 75 DAS and 62.87 in 2019–2020 and 71.49 × 104 cfu/g dry soil in 2020–2021 at harvest. Within subplots defined by nitrogen dose and scheduling, no significant differences were detected in the diazotrophic count. However, treatment T4 consistently yielded a greater diazotrophic count at both 75 DAS and harvest.
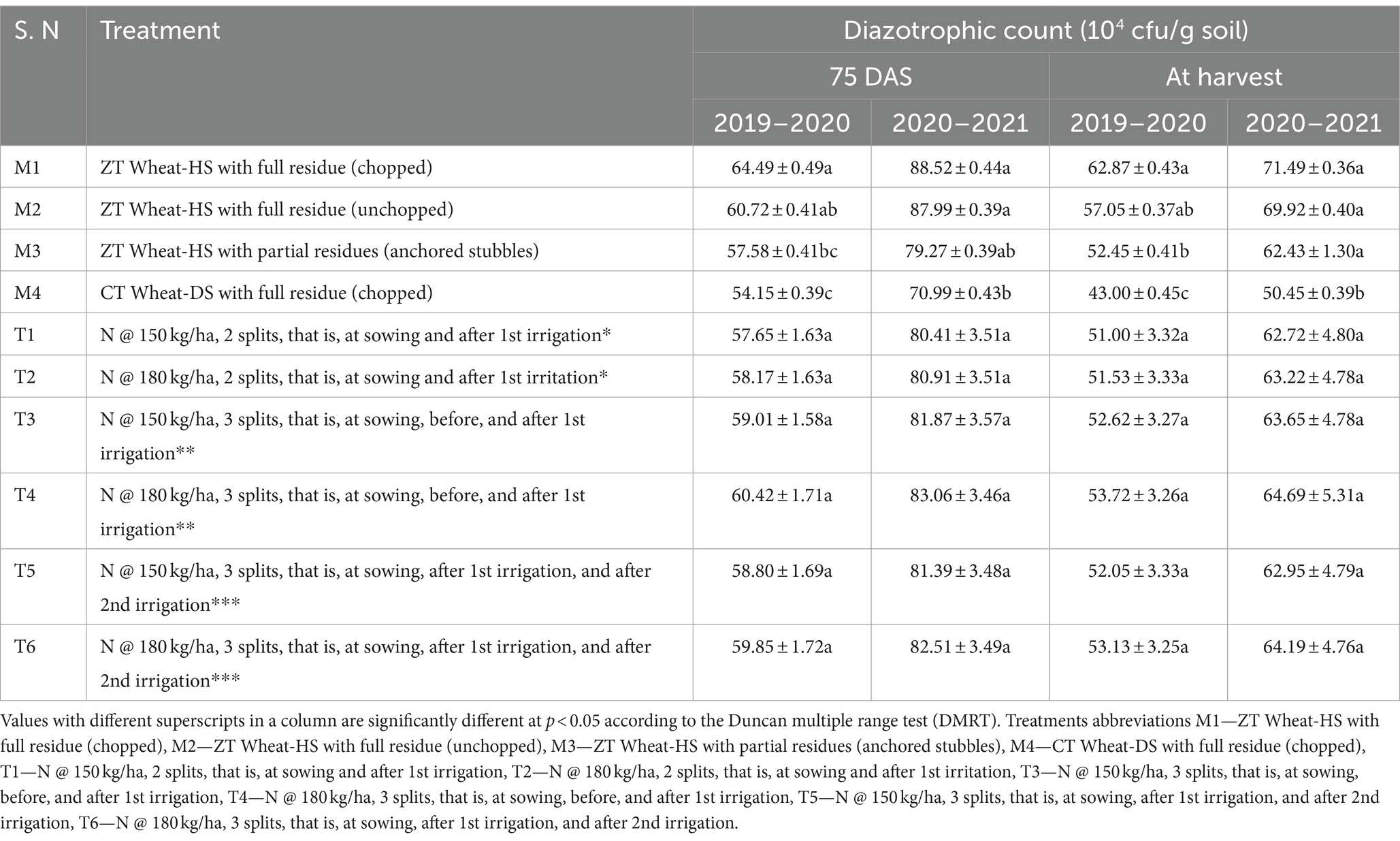
Table 6. Effect of crop establishment methods, rice residue practices, and nutrient scheduling on diazotrophic count under rice–wheat cropping system (2019–2020 and 2020–2021).
The data in Table 7 indicate that the actinomycete count in M1 was statistically similar to that in M2 at both 75 days after sowing (DAS) and at harvest across both years. Specifically, the counts were 67.51 at 75 DAS and 47.17 × 105 cfu/g dry soil at harvest in 2019–2020 and 63.09 at 75 DAS and 51.65 × 105 cfu/g dry soil at harvest in 2020–2021. These counts were significantly greater than those observed in M3 at both stages. Conversely, significantly lower actinomycete counts were recorded in M4 at both 75 DAS and harvest during both years. Subplot analysis revealed no significant differences in the actinomycete count. However, treatments involving nitrogen application at 180 kg/ha with three splits consistently resulted in higher counts at both 75 DAS and harvest. In comparison, a lower count was observed with nitrogen application at 150 kg/ha with two splits.
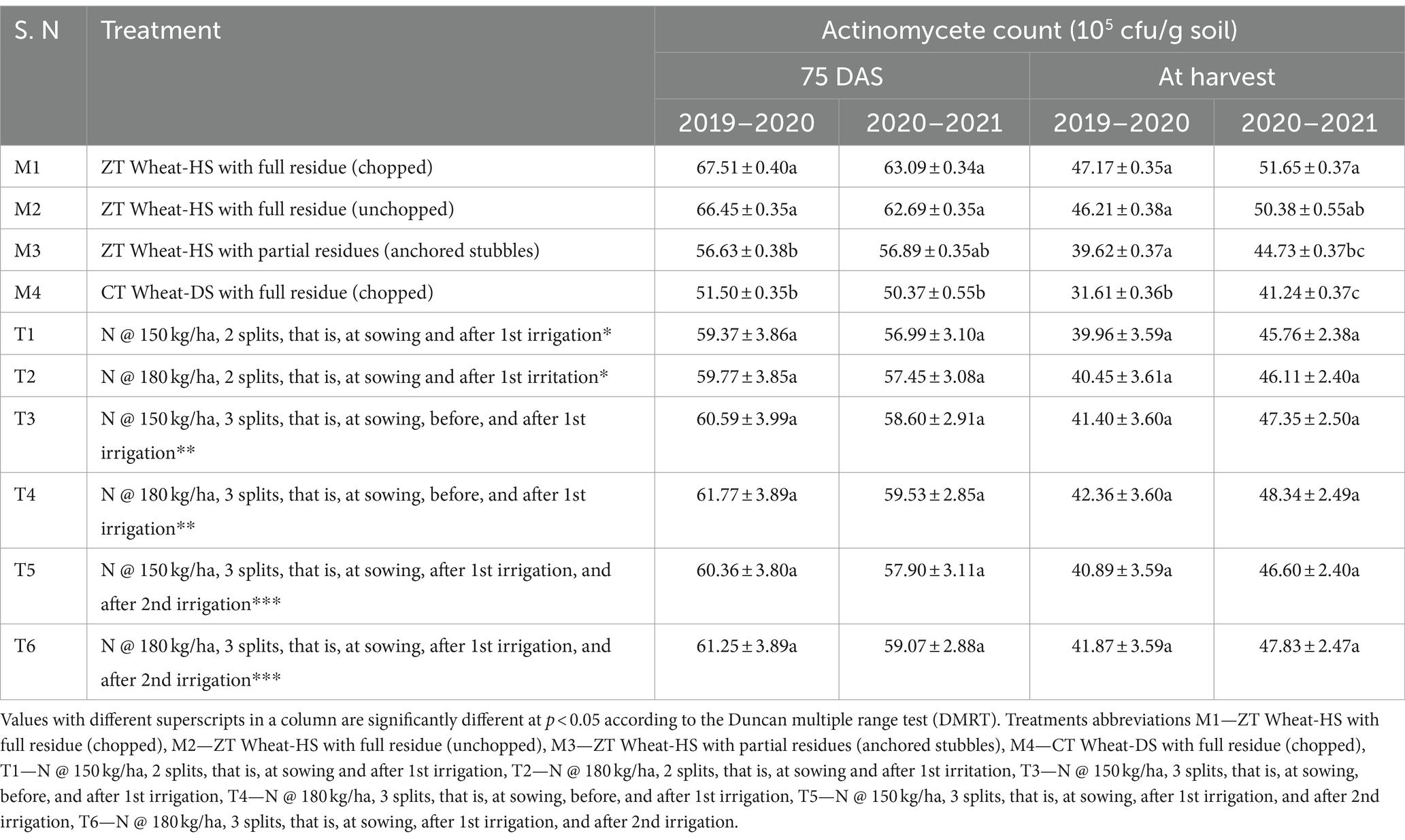
Table 7. Effect of rice residue practices, wheat crop establishment methods, and nutrient scheduling on actinomycete count of wheat under rice–wheat cropping system (2019–2020 and 2020–2021).
3.4 Impact of rice residue management practices and nutrient scheduling on yield
M2 exhibited the highest grain yield, with yields of 5,849 kg/ha in 2019–2020 and 5,874 kg/ha in 2020–2021, which were statistically similar to those of M3, which yielded 5,753 kg/ha in 2019–2020 and 5,636 kg/ha in 2020–2021. Compared to M4, M2 resulted in a 5.23% increase in grain yield. M2 increased the grain yield of wheat by 9.18% more than did M4. Three splits, that is, at sowing, before the 1st irrigation, and after the 1st irrigation, improved the grain yield of wheat by 8.08% compared with 2 splits, that is, at sowing and after the 1st irrigation. Within subplots, a significantly greater grain yield was observed under T4 (5,791–5,771 kg/ha during the study), which was statistically on par with T3 (5,724–5,712 kg/ha during the study), while T5 and T6 has lower grain yield. Applying nitrogen in three splits of wheat led to a 6.25% increase in grain yield than that in two splits (Table 8).
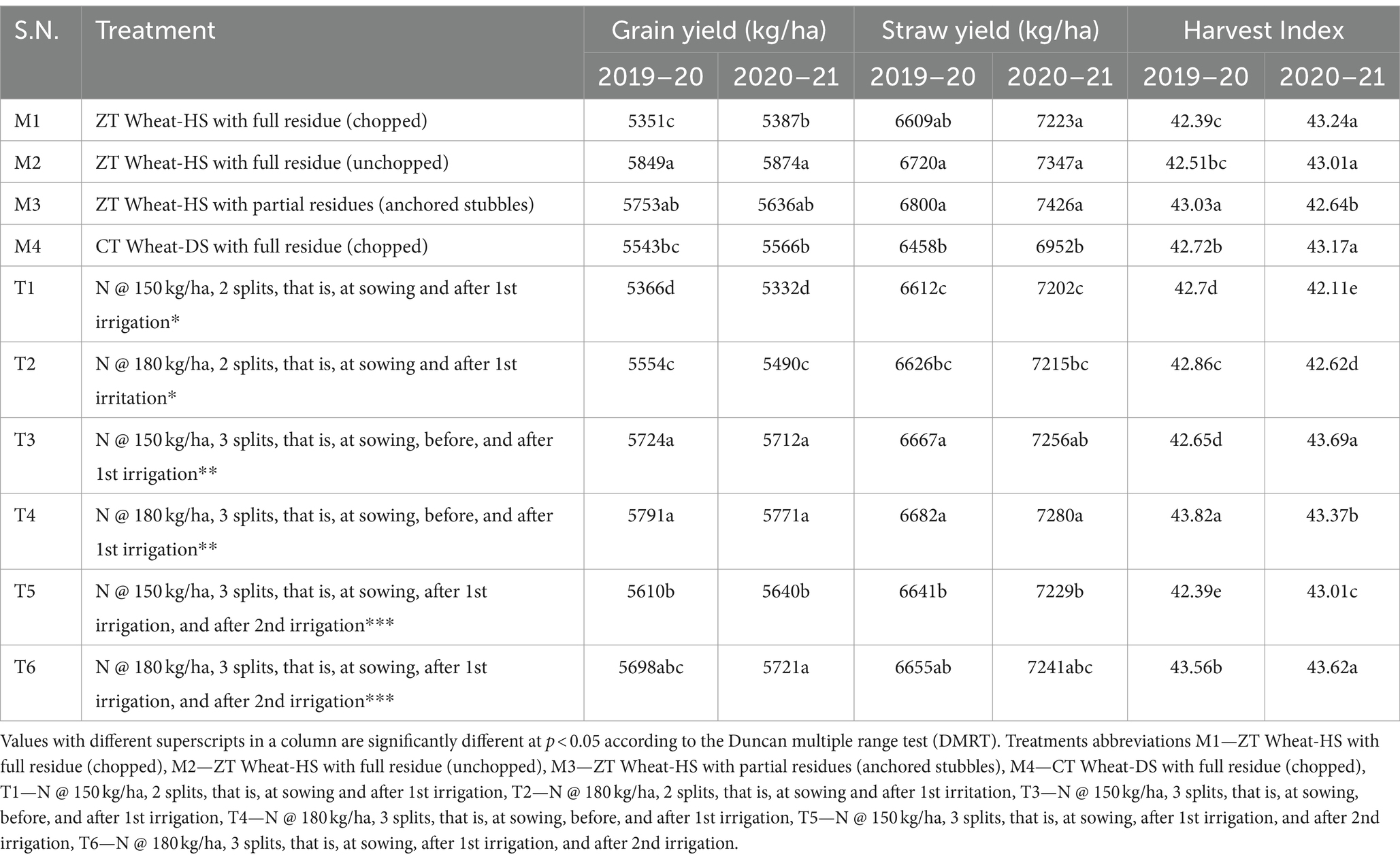
Table 8. Effect of rice residue practices, wheat crop establishment methods, and nutrient scheduling on yield of wheat under rice–wheat cropping system (2019–2020 and 2020–2021).
3.5 Impact of rice residue management practices and nutrient scheduling on enzymatic activity
Enzyme activity in soil, which is indicative of microbial processes, was significantly affected by rice residue practices during wheat cultivation. Notably, dehydrogenase, alkaline phosphatase, and urease activities were impacted during both cropping seasons, with higher levels observed at 75 days after sowing (DAS). For dehydrogenase activity, M1 displayed activity levels statistically similar to those of M2 but significantly greater than those of M3. The dehydrogenase activity ranged from 77.94 to 88.32 μg TPF/g soil/24 h and from 67.42 to 71.29 μg TPF/g soil/24 h at 75 DAS and harvest, respectively (Figure 1).
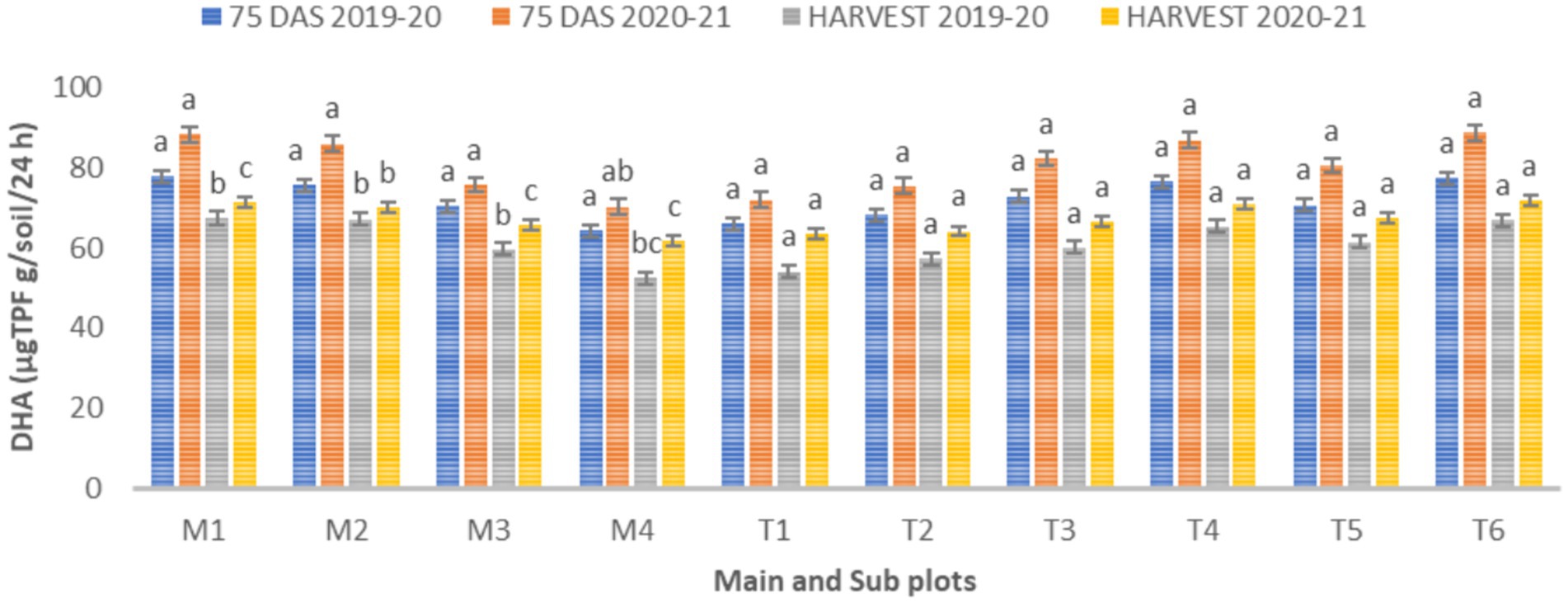
Figure 1. Effect of rice residue practices, wheat crop establishment methods. and nutrient scheduling on dehydrogenase activity in wheat under rice–wheat cropping system (2019–2020 and 2020–2021). The values within same color columns with different alphabetical letters differed significantly with each other. Treatments abbreviations: M1—ZT Wheat-HS with full residue (chopped), M2—ZT Wheat-HS with full residue (unchopped), M3—ZT Wheat-HS with partial residues (anchored stubbles), M4—CT Wheat-DS with full residue (chopped), T1—N @ 150 kg/ha, 2 splits, that is, at sowing and after 1st irrigation, T2—N @ 180 kg/ha, 2 splits, that is, at sowing and after 1st irritation, T3—N @ 150 kg/ha, 3 splits, that is, at sowing, before, and after 1st irrigation, T4—N @ 180 kg/ha, 3 splits, that is, at sowing, before, and after 1st irrigation, T5—N @ 150 kg/ha, 3 splits, that is, at sowing, after 1st irrigation, and after 2nd irrigation, T6—N @ 180 kg/ha, 3 splits, that is, at sowing, after 1st irrigation, and after 2nd irrigation.
Similarly, alkaline phosphatase activity was significantly influenced by rice residue management. M1 and M2 exhibited similar activity levels that were significantly greater than that of M3 (Figure 2). The urease activity at 75 DAS ranged from 95.21 to 98.51 μg TPF/g soil/24 h and that at harvest ranged from 82.77 to 88.43 μg TPF/g soil/24 h. Urease activity also significantly varied with residue management. M1 activity at 75 DAS ranged from 6.93 to 7.02 μg urea/g dry soil/min and at harvest from 4.73 to 4.82 μg urea/g dry soil/min; M2 had similar activity levels, which were significantly greater than those of M3. Subplot analysis revealed no significant differences in enzyme activity under different nitrogen doses or schedules. However, treatments involving nitrogen application at 180 kg/ha with three splits consistently exhibited greater enzyme activity than treatments involving two splits (Figure 3).
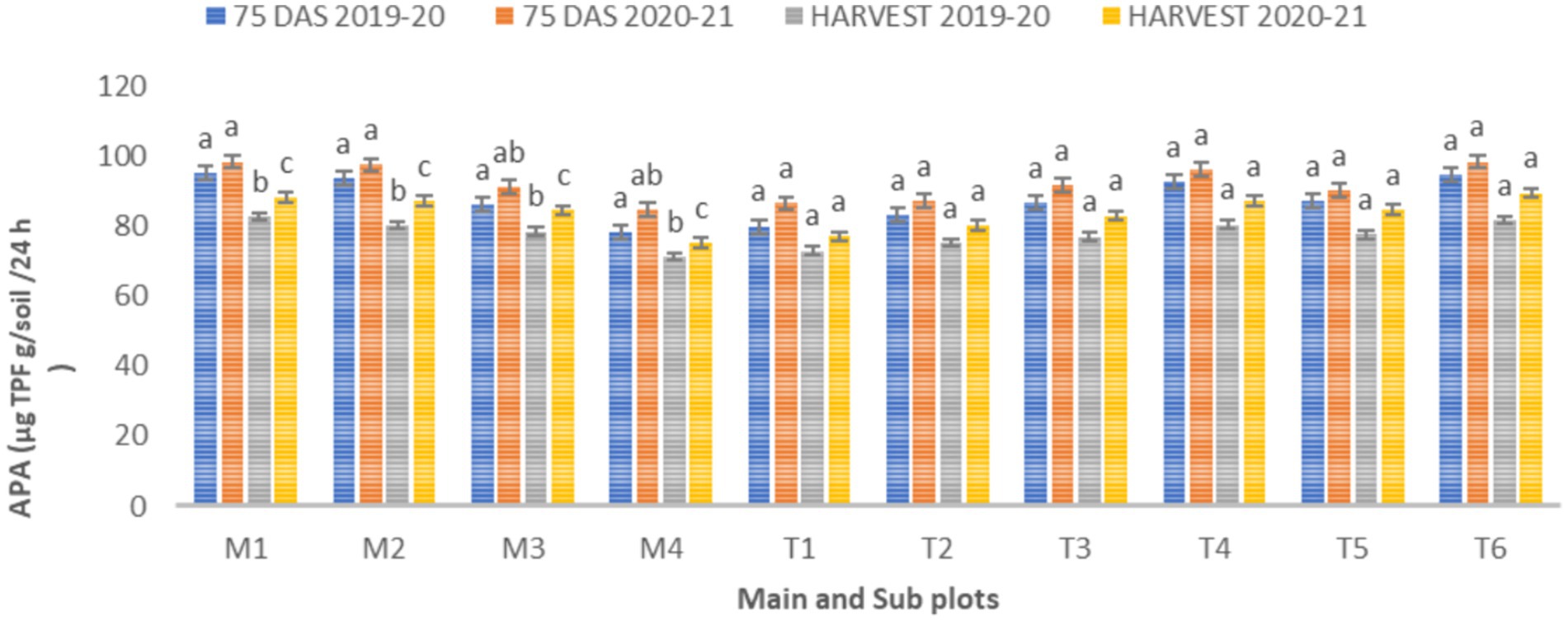
Figure 2. Effect of rice residue practices, wheat crop establishment methods, and nutrient scheduling on alkaline phosphatase activity in wheat under rice–wheat cropping system (2019–2020 and 2020–2021). The values within same color columns with different alphabetical letters differed significantly with each other. Treatments abbreviations: M1—ZT Wheat-HS with full residue (chopped), M2—ZT Wheat-HS with full residue (unchopped), M3—ZT Wheat-HS with partial residues (anchored stubbles), M4—CT Wheat-DS with full residue (chopped), T1—N @ 150 kg/ha, 2 splits, that is, at sowing and after 1st irrigation, T2—N @ 180 kg/ha, 2 splits, that is, at sowing and after 1st irritation, T3—N @ 150 kg/ha, 3 splits, that is, at sowing, before, and after 1st irrigation, T4—N @ 180 kg/ha, 3 splits, that is, at sowing, before, and after 1st irrigation, T5—N @ 150 kg/ha, 3 splits, that is, at sowing, after 1st irrigation, and after 2nd irrigation, T6—N @ 180 kg/ha, 3 splits, that is, at sowing, after 1st irrigation, and after 2nd irrigation.
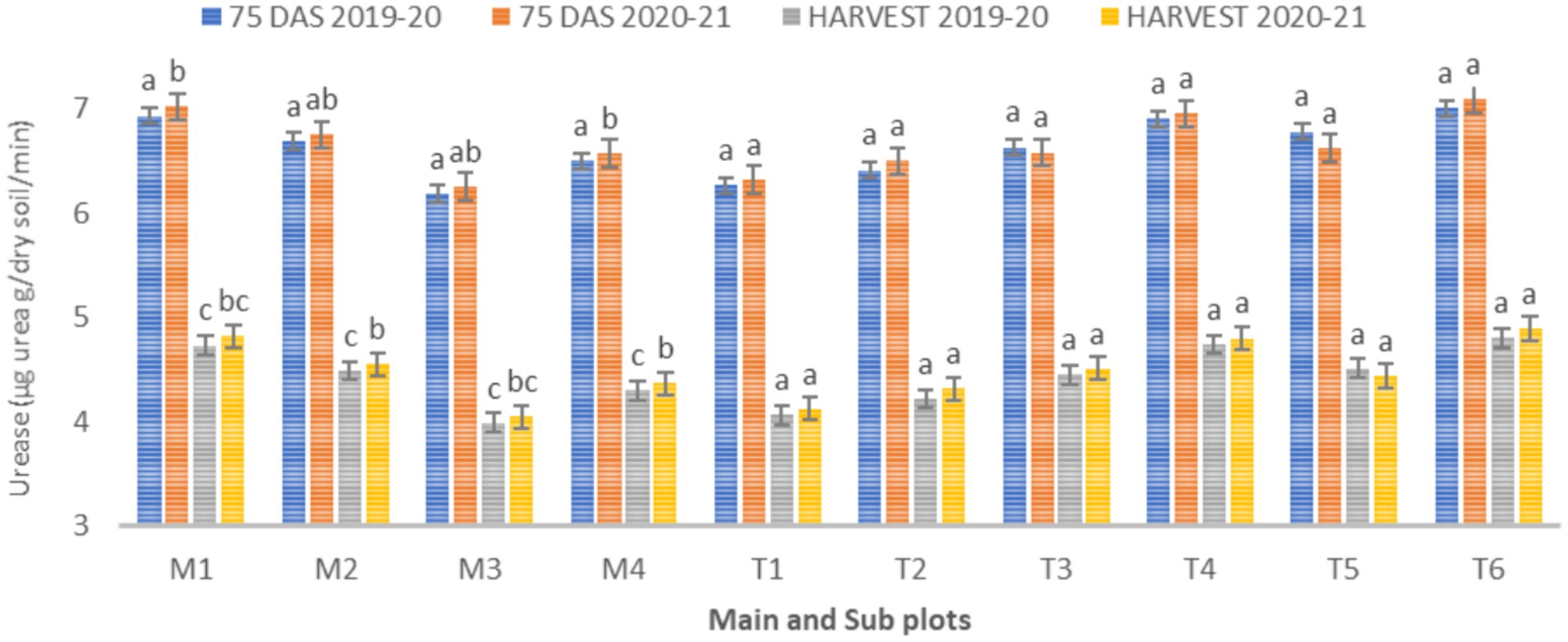
Figure 3. Effect of rice residue practices, wheat crop establishment methods, and nutrient scheduling on urease activity in wheat under rice–wheat cropping system (2019–2020 and 2020–2021). The values within same color columns with different alphabetical letters differed significantly with each other. Treatments abbreviations: M1—ZT Wheat-HS with full residue (chopped), M2—ZT Wheat-HS with full residue (unchopped), M3—ZT Wheat-HS with partial residues (anchored stubbles), M4—CT Wheat-DS with full residue (chopped), T1—N @ 150 kg/ha, 2 splits, that is, at sowing and after 1st irrigation, T2—N @ 180 kg/ha, 2 splits, that is, at sowing and after 1st irritation, T3—N @ 150 kg/ha, 3 splits, that is, at sowing, before, and after 1st irrigation, T4—N @ 180 kg/ha, 3 splits, that is, at sowing, before, and after 1st irrigation, T5—N @ 150 kg/ha, 3 splits, that is, at sowing, after 1st irrigation, and after 2nd irrigation, T6—N @ 180 kg/ha, 3 splits, that is, at sowing, after 1st irrigation, and after 2nd irrigation.
3.6 Correlations between microbial dynamics and enzymatic activity
The rice residue practice treatments demonstrated statistically significant correlations (p < 0.05) between microbial dynamics and enzymatic activities in wheat crops within the rice–wheat cropping system across both cropping seasons (Figures 4A–D). The increase in enzymatic activity was consistently paralleled by robust positive correlations between the microbial population and enzymatic activity across various residue retention practices.
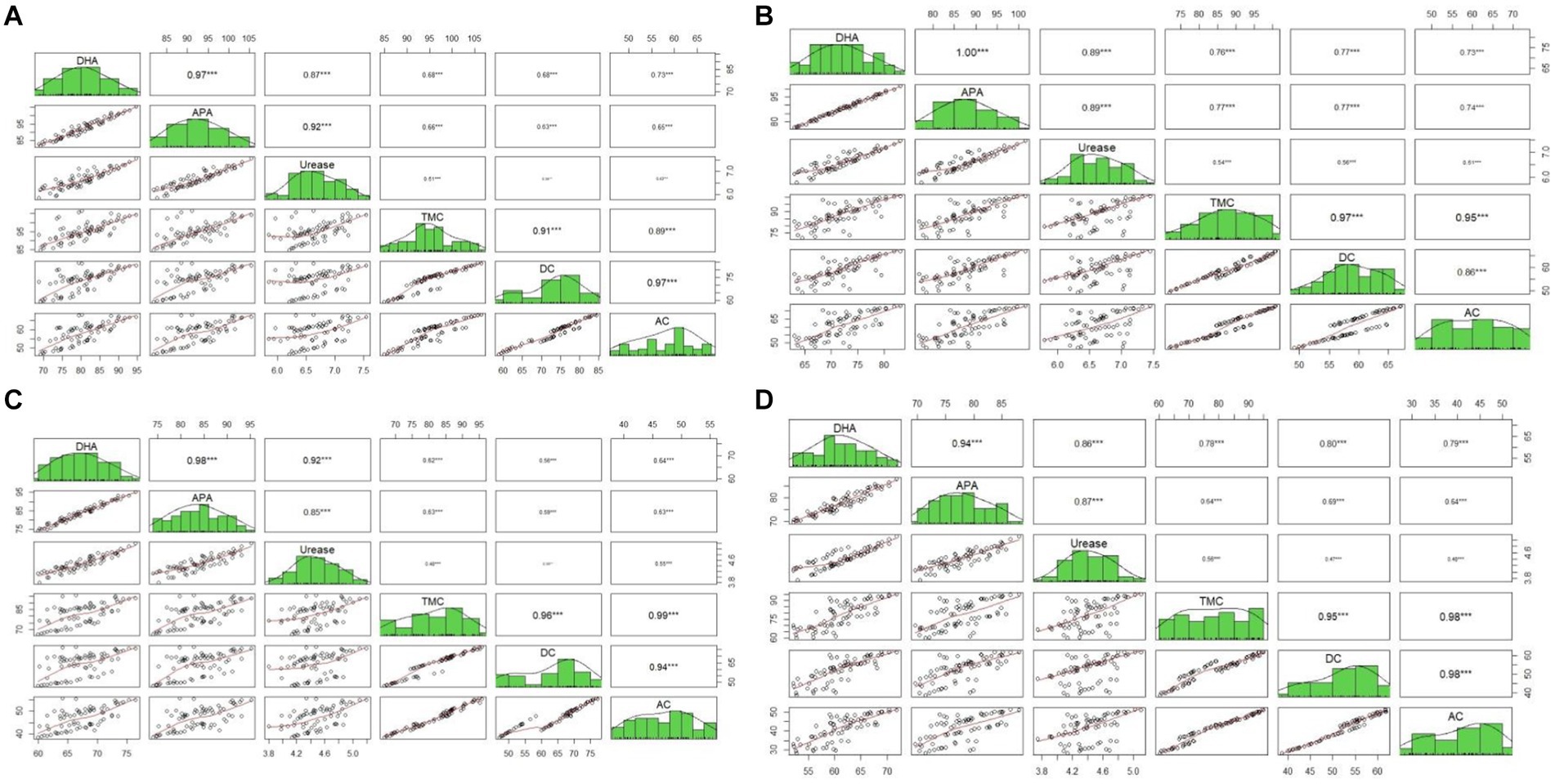
Figure 4. (A–D) Correlation analysis between microbial dynamics and enzymatic activities at 75 DAS and at maturity during 2019–2020 and 2020–2021, respectively.
Notably, TMC exhibited strong positive correlations with DHA at 75 DAS (r = 0.76) and maturity (r = 0.78) in 2019–2020 and at 75 DAS (r = 0.68) and maturity (r = 0.62) in 2020–2021, with APA at 75 DAS (r = 0.77) and maturity (r = 0.64) in 2019–2020 and at 75 DAS (r = 0.66) and maturity (r = 0.63) in 2020–2021, and with urease activity at 75 DAS (r = 0.54) and maturity (r = 0.64) in 2019–2020 and at 75 DAS (r = 0.66) and maturity (r = 0.63) in 2020–2021. Moreover, DC was significantly positively correlated with DHA at 75 DAS (r = 0.78) and maturity (r = 0.80) in 2019–2020 and at 75 DAS (r = 0.68) and maturity (r = 0.56) in 2020–2021, with APA at 75 DAS (r = 0.77) and maturity (r = 0.68) in 2019–2020 and at 75 DAS (r = 0.63) and maturity (r = 0.59) in 2020–2021, and with urease activity at 75 DAS (r = 0.54) and maturity (r = 0.48) in 2019–2020 and at 75 DAS (r = 0.36) and maturity (r = 0.38) in 2020–2021. Similarly, actinomycete activity was strongly positively correlated with DHA at 75 DAS (r = 0.73) and maturity (r = 0.79) in 2019–2020 and at 75 DAS (r = 0.73) and maturity (r = 0.64) in 2020–2021, with APA at 75 DAS (r = 0.74) and maturity (r = 0.64) in 2019–2020 and at 75 DAS (r = 0.65) and maturity (r = 0.63) in 2020–2021, and with urease activity at 75 DAS (r = 0.51) and maturity (r = 0.48) in 2019–2020 and at 75 DAS (r = 0.43) and maturity (r = 0.55) in 2020–2021 (Figures 4A–D).
4 Discussion
The impact of straw on crop yields is still under debate since field investigations across various pedoclimatic environments are indecisive, because of multiple and complex interaction of factors that affect the straw-derived N cycling under field conditions (Mu et al., 2016). Conservation tillage with residue retention (chopped and unchopped) significantly improved the physicochemical properties, microbial count, and soil enzymatic activities. Zero tillage with full residue (unchopped) in wheat exhibited a 5.23% increase in grain yield compared to conventional tillage with full residue (chopped), concurrently boosting the soil microbial count by 19.80–25%, the diazotrophic count by 29.43–31.6%, and the actinomycete count by 20.15–32.99% compared with conventional tillage.
Notably, there was a marked increase in SOC within the upper soil layer (0–15 cm) in ZTW-HS with full residue compared to that in CTW-DS (chopped) (Figure 4D). Numerous studies (Mondal et al., 2021; Zhang et al., 2021; Chen et al., 2021) have consistently reported significant increases in SOC under ZTW-HS compared to conventional practices. Throughout the study years, ZTW-HS and full residue load, both chopped and unchopped, exhibited higher soil moisture contents. Crop residues add a considerable amount of labile organic carbon to soils and boost enzyme activity due to the increased presence of microbial communities (Fang et al., 2018). Zero tillage (ZT) significantly enhances soil moisture availability by reducing soil compaction and ensuring a uniform distribution of soil micropores and macropores (Meena et al., 2015; Huang et al., 2012). Zero tillage with residue retention (ZTW) has become a vital practice for conserving soil organic carbon (SOC) and minimizing carbon mineralization. This practice boosts nutrient availability for crops, improves soil water-holding capacity, and promotes aeration through the formation of continuous soil pores in the root zone (Varatharajan et al., 2022; Biswakarma et al., 2022). In addition, retaining crop residues encourages a higher microbial population than residue removal in both ZT and conventional tillage (CT) systems (Govaerts et al., 2008). Over a 2-year crop cycle, ZTW-HS with full residue or stubble resulted in a significant increase in SOC within the upper 0–15 cm soil layer. This increase can be attributed to the disruption of soil macroaggregates under CT without residue, which promotes direct contact between straw and microorganisms, leading to increased carbon mineralization. In contrast, ZTW-HS with residue retention prevents direct microbial contact and provides fewer nutrients to microbes.
Soil microorganisms enhance soil quality, health, fertility, and overall microbial community. Among these parameters, microbial activity serves as a subtle indicator for assessing soil quality. Adding crop residues has been shown to stimulate the microbial activity of bacteria, fungi, and actinomycetes (Choudhary et al., 2018). Studies have demonstrated that rice straw returned to the soil, along with cow manure, significantly increases SOM, total N, and available P compared to those in scenarios without residue incorporation (Cheng et al., 2014; Gu et al., 2018; Moharana et al., 2012). Moreover, numerous reports have highlighted the positive impact of residue management, whether through incorporation or retention, on the soil organic carbon balance (Gangwar et al., 2006; Gupta et al., 2007). During the wheat season, rice straw returned to the soil promoted the growth and reproduction of soil microbes, which was conducive to the stability and promotion of microbial community structures. This accelerated the decomposition of straw and enhanced nutrient availability into the soil (Pathak et al., 2006; Tan et al., 2006; Qian et al., 2012). Straw incorporation enhances the metabolic activity of microorganisms, the relevant enzyme activity, and microbial population (Sharma et al., 2020a). The soil microbial population gradually increased up to 75 days after the sowing of wheat and decreased during later growth periods. Rice residue had a pronounced impact on the soil microbial community, particularly up to 75 DAS. However, at maturity, the increase in mineralization of the prevailing microbial community decreased, possibly due to the solubility of residues or relative microbial availability in the field (Lehmann and Kleber, 2015). The presence of an adequate amount of rice residue in the soil regulates soil temperature, facilitating better microbial reproduction up to 75 DAS and maturity. The TMC and diazotrophic count exceeded the actinomycete count under the various treatments, corroborating previous findings (Choudhary et al., 2018; Bhagat and Gosal, 2018; Stagnari et al., 2020). Microbial populations, including fungal, bacterial, and actinomycetes populations, were greater under ZT with surface residue retention than under ZT with incorporation or removal (Jat et al., 2019; Stagnari et al., 2020). These microbial communities likely have advanced to grow rapidly in response to easily mineralized OM (Whitman et al., 2016). Excessive nitrogen doses failed to enhance microbial quantity or enzyme activity. Studies suggest that high nitrogen levels can lead to the accretion of toxic substances, such as ammonia, which is detrimental to plant health and can impede the growth of certain microbial groups. In addition, excessive nitrogen can lower the soil pH, which is necessary for enzyme activity (Brzezińska and Włodarczyk, 2005).
Soil microbial activity and enzymatic activity are strongly linked to increased nutritional mineralization of native organic C after residue integration (Guenet et al., 2010; Kirkby et al., 2014; Liu et al., 2011). Enzymatic activity exhibits a strong relationship with the soil microbial population, with increased microbial populations correlating with enhanced enzymatic activities, such as DHA, APA, and urease activity. Residue management practices have demonstrated a considerable and favorable relationship between enzymatic activity and SMC during the study period. Similar findings were reported by Tang et al. (2020), who reported a positive correlation between diazotrophic and actinomycete counts and DHA and APA. These soil microbes are significantly improved under ZT conditions (Rajanna et al., 2022; Choudhary et al., 2018). ZTW-HS with full residue (unchopped) produced more grain, straw, and biological yields, similar to ZTW with partial residues (anchored stubbles). This finding aligns with previous research highlighting the significant effect of rice residues on wheat yield (Chandra, 2018; Dhar et al., 2014; Kesarwani et al., 2017; Sah et al., 2014; Singh et al., 2021; Pandiaraj et al., 2015). The higher wheat yield in straw-retained plots can be attributed to improved soil nutrient levels and microbial abundance following straw residue incorporation as mulch in the field (Chaudhary et al., 2023). ZT has emerged as the most competent tillage method for conserving resources and increasing wheat yield (Usman et al., 2013). Higher grain and straw yields of wheat were recorded with ZT-HS than with conventional methods (Nandan et al., 2018). The higher grain yield under the retention of residue/incorporation treatments can be ascribed to increased growth parameters, facilitated by improved SOM content, nutrient availability, and moderation of the soil’s hydrothermal regime (Gupta et al., 2016; Iqbal et al., 2017; Ram et al., 2013). Straw incorporation/retention enhances soil properties, leading to improved root growth, improved nutrient uptake, and ultimately enhanced plant growth and grain yield. Similar results have been reported by Gupta et al. (2016), Meena et al. (2015), and Sharma et al. (2019). The reason for the lowest yields under CTW when full residues are incorporated after a chopper and spreader could be that the N that bacteria utilize to break down incorporated residues causes the plants to turn yellow in the early days. T5 and T6 have lower yield due to more N losses as it was applied after 1st and 2nd irrigation.
Moreover, applying nitrogen in three splits (at sowing, before the 1st irrigation, and after the 1st irrigation) led to a 6.25% increase in grain yield than that in two splits (at sowing and after the 1st irrigation), significantly impacting wheat productivity in the soil. Optimal nitrogen management practices have been highlighted by Sidhu et al. (2007), who reported a greater grain yield of ZTW-HS in rice residues with fertilizer broadcasting at sowing and before the 1st irrigation. A study by Gill et al. (2019) determined that nitrogen management involving three equal splits applied at specific intervals was the most efficient practice for enhancing yield. Similarly, Singh et al. (2015) reported that specific nitrogen management practices significantly increased the mean wheat yield.
5 Conclusion
In summary, our study highlights the profound impact of various rice residue management techniques on the microbial characteristics of wheat, ultimately enhancing grain yield, SOC, and enzymatic activity. In particular, ZTW-HS (chopped) consistently demonstrated increased TMC and enzymatic activities, contrasting with the lowest count observed in CTW-DS (chopped), emphasizing the pivotal role of rice residue retention in fostering soil biological properties. Embracing zero tillage coupled with full residue retention, facilitated by machinery such as a happy seeder, has emerged as a crucial approach in rice–wheat cropping systems. This approach not only ensures the sustained productivity and income of farms but also enhances soil vigor and environmental quality. By adopting such innovations, the agricultural sector can improve rice residue management practices for long-term productivity and environmental sustainability, both regionally and globally. Moreover, optimizing nitrogenous fertilizer dosages for wheat crops alongside rice residue management approaches is imperative. Residue incorporation or retention significantly influences the chemical and biological properties of soil, reducing the need for nitrogenous fertilizers. In addition, our findings underscore the significant impact of nitrogen levels on wheat grain yield traits, with grain yield equivalent to the recommended N fertilizer dose (150 kg N/ha) with partial and full residue (unchopped) residue retention. Rice residue management in the rice–wheat cropping system is a multidisciplinary effort that combines technology advancements and sustainable agriculture techniques with economic considerations and legislative support. The agricultural sector may improve residue management techniques for long-term productivity and environmental stewardship by addressing these issues collectively in South Asia and globally in similar crop-growing regions. We must determine that the repercussions of harvesting crop residues for any purpose must be specified, and methods applied using site-specific technologies to ensure that productivity and agronomic resources are not jeopardized for future generations.
Data availability statement
The original contributions presented in the study are included in the article/supplementary material, further inquiries can be directed to the corresponding author.
Author contributions
CC: Formal analysis, Investigation, Writing – original draft, Writing – review & editing. DY: Conceptualization, Methodology, Resources, Supervision, Writing – review & editing. VH: Supervision, Writing – review & editing. AC: Methodology, Supervision, Writing – review & editing. JP: Methodology, Resources, Writing – review & editing. AK: Writing - review & editing, Formal analysis, Visualisation. RK: Writing – review& editing, Supervision. AY: Writing – review & editing.
Funding
The author(s) declare that no financial support was received for the research, authorship, and/or publication of this article.
Acknowledgments
The first author duly acknowledges CCSHAU for providing the fellowships and facilities to carry out the research.
Conflict of interest
The authors declare that the research was conducted in the absence of any commercial or financial relationships that could be construed as a potential conflict of interest.
Publisher’s note
All claims expressed in this article are solely those of the authors and do not necessarily represent those of their affiliated organizations, or those of the publisher, the editors and the reviewers. Any product that may be evaluated in this article, or claim that may be made by its manufacturer, is not guaranteed or endorsed by the publisher.
References
Babu, S., Singh, R., Avasthe, R. K., Kumar, S., Rathore, S. S., Singh, V. K., et al. (2023). Soil carbon dynamics under organic farming: Impact of tillage and cropping diversity : Ecolo. Indicators. 147:109940. doi: 10.1016/j.ecolind.2023.109940
Ben-David, A., and Davidson, C. E. (2014). Estimation method for serial dilution experiments. J. of Microbio. Methods 107, 214–221. doi: 10.1016/j.mimet.2014.08.023
Bhagat, P., and Gosal, S. K. (2018). Long-term application of rice straw and nitrogen fertilizer affects soil health and microbial communities. Chem. Sci. Rev. Lett. 7, 586–593. doi: 10.9734/CJAST/2018/39631
Bhatt, R., Kukal, S., Busari, M., Arora, S., and Yadav, M. (2016). Sustainability issues on rice–wheat cropping system. Int. Soil Water Conserv. Res. 4, 64–74. doi: 10.1016/j.iswcr.2015.12.001
Bin, F., Ling, C., Hongying, H., Ping, Q., and Zhenggui, W. (2021). Impacts of crop residues on soil health: A review. Environ. Pollut. Bio availab. 33, 164–173. doi: 10.1080/26395940.2021.1948354
Biswakarma, N., Pooniya, V., Zhiipao, R. R., Kumar, D., Shivay, Y. S., Meena, M. C., et al. (2022). Designing resource efficient integrated crop management modules for direct seeded rice -zero till wheat rotation of north western India: impacts on system productivity, energy-nutrient-carbon dynamics. Arch. Agron. Soil Sci. 69: 1236–1250. doi: 10.1080/03650340.2022.2079635
Bremner, J. M., and Douglas, L. A. (1971). Inhibition of urease activity in soils. Soil Biol. Biochem. 3, 297–307. doi: 10.1016/0038-0717(71)90039-3
Brzezińska, M., and Włodarczyk, T. (2005). Enzymes of intracellular redox transformations (oxidoreductases). Acta Agrophysica 3, 11–26.
Casida, L. E., Klein, D. A. K., and Santoro, T. (1964). Soil dehydrogenase activity. Soil Sci. 98, 371–376. doi: 10.1097/00010694-196412000-00004
Chandra, M. (2018). Effect of rice residue management on soil quality and productivity of wheat crop. M.Sc. Thesis, Indira Gandhi Krishi Vishwavidyalaya, Raipur.
Chaudhary, C., Yadav, D. B., Yadav, A., Chaudhary, A., and Hooda, V. S. (2023). Influence of crop residue and nitrogen management on nutrient uptake, yield, and economics of rice–wheat cropping system. J. Plant Nutr. 47, 376–391. doi: 10.1080/01904167.2023.2277399
Chen, A., Zhang, W., Sheng, R., Liu, Y., Hou, H., Liu, F., et al. (2021). Long-term partial replacement of mineral fertilizer with in situ crop residues ensures continued rice yields and soil fertility: A case study of a 27-year field experiment in subtropical China. Sci. Total Environ. 787:147523. doi: 10.1016/j.scitotenv.2021.147523
Cheng, Z. S., Yun, C. C., Jiang, L. K., Qiu, S. J., Wei, Z., and Ping, H. E. (2014). Effects of long-term straw return on soil fertility, nitrogen pool fractions and crop yields on a fluvo-aquic soil in North China. J. Plant Nutri. Fert. 20, 1441–1449.
Choudhary, M., Datta, A., Jat, H. S., Yadav, A. K., Gathala, M. K., Sapkota, T. B., et al. (2018). Changes in soil biology under conservation agriculture-based sustainable intensification of cereal systems in indo-Gangetic Plains. Geoderma 313, 193–204. doi: 10.1016/j.geoderma.2017.10.041
Dhar, D., Datta, A., Basak, N., Paul, N., Badole, S., and Thomas, T. (2014). Residual effect of crop residues on growth, yield attributes and soil properties of wheat under rice–wheat cropping system. Indian J. Agric Res. 48, 373–378. doi: 10.5958/0976-058X.2014.01317.1
Dhillon, B. S., and Sohu, V. S. (2024). Climate change shocks and crop production: the foodgrain bowl of India. Indian J. of Agron. 69, 1–10. doi: 10.59797/ija.v69i1.5474
DOACFW (2019). Review of the scheme "promotion of agricultural mechanization for in situ management of crop residue in states of Punjab, Haryana, Uttar Pradesh and NCT of Delhi", Ministry of Agriculture and farmers welfare. Krishi Bhawan, New Delhi: Deptt. of Agriculture, Cooperation and Farmers Welfare.
Doran, J. W., and Zeiss, M. R. (2000). Soil health and sustainability: managing the biotic component of soil quality. Appl. Soil Ecol. 15, 3–11. doi: 10.1016/S0929-1393(00)00067-6
Fang, Y., Singh, B. P., Collins, D., Li, B., Zhu, J., and Tavakkoli, E. (2018). Nutrient supply enhanced wheat residue-carbon mineralization, microbial growth, and microbial carbon-use efficiency when residues were supplied at high rate in contrasting soils. Soil Biol. Biochem. 126, 168–178. doi: 10.1016/j.soilbio.2018.09.003
Gangmei, T. P., Kaur, N., Thakur, A., Sahu, K. K., Kumar, A., Sandal, S. K., et al. (2024). Microbial population and yield of rice–wheat system under variable irrigation and nutrient management. Indian J. of Agron. 69, 17–22. doi: 10.59797/ija.v69i1.5476
Gangwar, K. S., Singh, K. K., Sharma, S. K., and Tomar, O. K. (2006). Alternative tillage and crop residue management in wheat after rice in sandy loam soils of indo-Gangetic plains. Soil Tillage Res. 88, 242–252. doi: 10.1016/j.still.2005.06.015
Gill, S. C., Sharma, R. K., Tripathi, S. C., Chhokar, R. S., Meena, R. P., and Jha, A. (2019). Nitrogen top dressing just before irrigation improves wheat growth, productivity and nitrogen use efficiency and profitability. J. Cereal Res. 11, 17–22. doi: 10.25174/2249-4065/2019/83006
Gomez, K. A., and Gomez, A. A. (1984). Statistical Procedures for Agricultural Research. 2nd Edn, New York: John Wiley and Sons, 680.
Gopinath, K. A., Rajanna, G. A., Venkatesh, G., Jayalakshmi, M., Kumari, V. V., Prabhakar, M., et al. (2022). Influence of crops and different production systems on soil carbon fractions and carbon sequestration in rainfed areas of semiarid tropics in India. Sustain. For. 14:4207. doi: 10.3390/su14074207
Govaerts, B., Mezzalama, M., Sayre, K. D., Crossa, J., Lichter, K., Troch, V., et al. (2008). Long-term consequences of tillage, residue management, and crop rotation on selected soil microflora groups in the subtropical highlands. Appl. Soil Ecol. 38, 197–210. doi: 10.1016/j.apsoil.2007.10.009
Gu, S., Guo, X., Cai, Y., Zhang, Z., Wu, S., Li, X., et al. (2018). Residue management alters microbial diversity and activity without affecting their community composition in black soil. Northeast China Peer J. 6:5754. doi: 10.7717/peerj.5754
Guenet, B., Neill, C., Bardoux, G., and Abbadie, L. (2010). Is there a linear relationship between priming effect intensity and the amount of organic matter input? Appl. Soil Ecol. 46, 436–442. doi: 10.1016/j.apsoil.2010.09.006
Gupta, V., Roper, M., and Thompson, J. (2019). Harnessing the benefits of soil biology in conservation agriculture. In Australian agriculture in 2020: from conservation to automation (eds. J. Pratley & Kirkegaard, J. ) 237–253 (Agronomy Australia and Charles Sturt University).
Gupta, R. K., Singh, Y., Ladha, J. K., Singh, B., Singh, J., Singh, G., et al. (2007). Yield and phosphorus transformations in a rice–wheat system with crop residue and phosphorus management. Soil Sci. Soc. Am. J. 71, 1500–1507. doi: 10.2136/sssaj2006.0325
Gupta, R. K., Sraw, P. K., Kang, J. S., Kaur, J., Sharma, V., Pathania, N., et al. (2024). Interactive effects of long term management of crop residue and phosphorus fertilization on wheat productivity and soil health in the rice–wheat. Sci. Rep. 14:1399. doi: 10.1038/s41598-024-51399-8
Gupta, S., Yadav, R., Gaikwad, K., Kushwah, A., Singh, A. M., Bainsla, N. K., et al. (2016). Genetic improvement trend analysis for end-use quality characteristics among wheat cultivars of North-Western India. Indian Jo. Genet. Plant Breeding 76, 137–143. doi: 10.5958/0975-6906.2016.00030.4
Harish, M. N., Choudhary, A. K., Bhupenchandra, I., Dass, A., Rajanna, G. A., Singh, V. K., et al. (2022). Double zero-tillage and foliar-P nutrition coupled with bioinoculants enhance physiological photosynthetic characteristics and resilience to nutritional and environmental stresses in maize–wheat rotation. Front. Plant Sci. 13:959541. doi: 10.3389/fpls.2022.959541
Hayashi, K., Nishimura, S., and Yagi, K. (2008). Ammonia volatilization from a paddy field following applications of urea: Rice plants are both an absorber and an emitter for atmospheric ammonia. Sci. Total Environ. 390, 485–494. doi: 10.1016/j.scitotenv.2007.10.037
Huda, A., Gaihre, Y. K., Islam, M. R., Singh, U., Islam, M. R., Sanabria, J., et al. (2016). Floodwater ammonium, nitrogen use efficiency and rice yields with fertilizer deep placement and alternate wetting and drying under triple rice cropping systems. Nutr. Cycl. Agroecosyst. 104, 53–66. doi: 10.1007/s10705-015-9758-6
Huang, P. M., Li, Y., and Sumner, M. P., (Eds). (2012) Handbook of Soil Sciences, Properties and Processes. 2nd Edn. Boca Raton: CRC Press.
Iqbal, M. F., Hussain, M., Faisal, N., Iqbal, J., Rehaman, A. U., Ahmad, M., et al. (2017). Happy seeder zero tillage equipment for sowing of wheat in standing rice stubbles. Int. J. Adv. Res. Biol. Sci. 4, 101–105. doi: 10.22192/ijarbs.2017.04.04.014
Islam, S. M. M., Gaihre, Y. K., Biswas, J. C., Jahan, M. S., Singh, U., Adhikay, S. K., et al. (2018). Different nitrogen rates and methods of application for dry season rice cultivation with alternate wetting and drying irrigation: fate of nitrogen and grain yield. Agric. Water Manag. 196, 144–153. doi: 10.1016/j.agwat.2017.11.002
Islam, S. M. M., Gaihre, Y. K., Shah, A. L., Singh, U., Sarkar, M. I. U., Satter, M. A., et al. (2016). Rice yields and nitrogen use efficiency with different fertilizers and water management under intensive lowland rice cropping systems in Bangladesh. Nutr. Cycl. Agroecosyst. 106, 143–156. doi: 10.1007/s10705-016-9795-9
Jackson, M. L. (1967). Soil chemical analysis. New Delhi, India: Prentice Hall of India, Private Limited, 234–246.
Jat, H. S., Kumar, P., Sutaliya, J. M., Kumar, S., Choudhary, M., Singh, Y., et al. (2019). Conservation agriculture based sustainable intensification of basmati rice–wheat system in north–West India. Arch. Agron. Soil Sci. 65, 1370–1386. doi: 10.1080/03650340.2019.1566708
Kesarwani, A., Shukla, R., Singh, V. P., Pandey, D. S., Pramanick, B., Yadav, S. K., et al. (2017). In situ Rice residue management under rice–wheat cropping system and their influence on wheat productivity. J. Pharmacognosy Phytochem. 6, 1422–1425.
Kirkby, C. A., Richardson, A. E., Wade, L. J., Passioura, J. B., Batten, G. D., Blanchard, C., et al. (2014). Nutrient availability limits carbon sequestration in arable soils. Soil Biol. Biochem. 68, 402–409. doi: 10.1016/j.soilbio.2013.09.032
Lehmann, J., and Kleber, M. (2015). The contentious nature of soil organic matter. Nature 528, 60–68. doi: 10.1038/nature16069
Li, H., Wang, H., Jia, B., Li, D., Fang, Q., and Li, R. (2021). Irrigation has a higher impact on soil bacterial abundance, diversity and composition than nitrogen fertilization. Sci. Rep. 11:16901. doi: 10.1038/s41598-021-96234-6
Liu, T. Q., Fan, D. J., Zhang, X. X., Hen, J., Li, C. F., and Cao, C. G. (2015). Deep placement of nitrogen fertilizers reduces ammonia volatilization and increases nitrogen utilization efficiency in no-tillage paddy fields in Central China. Field Crop Res. 184, 80–90. doi: 10.1016/j.fcr.2015.09.011
Liu, C., Wang, K., Meng, S., Zheng, X., Zhou, Z., Han, S., et al. (2011). Effects of irrigation, fertilization and crop straw management on nitrous oxide and nitric oxide emissions from a wheat–maize rotation field in northern China. Agric. Ecosys. Environ. 140, 226–233. doi: 10.1016/j.agee.2010.12.009
Majumdar, A., Moulick, D., and Srivastava, S. (2024). Editorial: ‘save soil’ by managing soil nutrient losses, agronomic practices and crop-microbial interaction: world soil day 2022. Front. Sustain. Food Syst. 8:1360937. doi: 10.3389/fsufs.2024.1360937
Meena, H. N., Ajay, B. C., Rajanna, G. A., Yadav, R. S., Jain, N. K., and Meena, M. S. (2022). Polythene mulch and potassium application enhances peanut productivity and biochemical traits under sustained salinity stress condition. Agric. Water Manag. 273:107903. doi: 10.1016/j.agwat.2022.107903
Meena, J. R., Behera, U. K., Chakraborty, D., and Sharma, A. R. (2015). Tillage and residue management effect on soil properties, crop performance and energy relations in green gram (Vigna radiata L.) under maize-based cropping systems. Int. Soil Water Conserv. Res. 3, 261–272. doi: 10.1016/j.iswcr.2015.11.001
Meena, R. P., Venkatesh, K., Khobra, R., Tripathi, S. C., Prajapat, K., Sharma, R. K., et al. (2020). Effect of rice residue retention and foliar application of k on water productivity and profitability of wheat in north West India. Agronomy 10:434. doi: 10.3390/agronomy10030434
Moharana, P. C., Sharma, B. M., Biswas, D. R., Dwivedi, B. S., and Singh, R. V. (2012). Long-term effect of nutrient management on soil fertility and soil organic carbon pools under a 6-year-old pearl millet-wheat cropping system in an inceptisol of subtropical India. Field Crop Res. 136, 32–41. doi: 10.1016/j.fcr.2012.07.002
Mondal, S., Mishra, J. S., Poonia, S. P., Kumar, R., Dubey, R., Kumar, S., et al. (2021). Can yield, soil C and aggregation be improved under long-term conservation agriculture in the eastern indo-Gangetic plain of India? Eur. J. Soil Sci. 72, 1742–1761. doi: 10.1111/ejss.13092
Móring, A., Hooda, S., Raghuram, N., Adhya, T. K., Ahmad, A., Bandyopadhyay, S. K., et al. (2021). Nitrogen challenges and opportunities for agricultural and environmental science in India. Front. Sustain. Food Syst. 5:505347. doi: 10.3389/fsufs.2021.505347
Mu, X. Y., Zhao, Y. L., Liu, K., Ji, B. Y., Guo, H. B., Xue, Z. W., et al. (2016). Responses of soil properties, root growth and crop yield to tillage and crop residue management in a wheat–maize cropping system on the North China plain. Eur. J. Agron. 78, 32–43. doi: 10.1016/j.eja.2016.04.010
Nandan, R., Singh, V., Singh, S., Hazra, K., and Nath, P. C. (2018). Performance of crop residue management with different tillage and crop establishment practices on weed flora and crop productivity in rice–wheat cropping system of eastern indo-Gangetic plains. J. Crop Weed 14, 65–71. doi: 10.5555/20193025284
Nawaz, A., Farooq, M., Nadeem, F., Siddique, K. H. M., and Lal, R. (2019). Rice-wheat cropping systems in South Asia: issues, options and opportunities. Crop Pasture Sci. 70, 395–427. doi: 10.1071/CP18383
Olsen, S. R., Cole, C. V., Watanabe, F. S., and Dean, L. A. (1954). Estimation of available phosphorus in soil by extraction with sodium bicarbonate. USDA Circ. 939, 1–19.
Padre, A. T., Rai, M., Kumar, V., Gathala, M. K., Sharma, P. C., Sharma, S., et al. (2016). Quantifying changes to the global warming potential of rice–wheat systems with the adoption of conservation agriculture in northwestern India. Agric. Ecosyst. Environ. 219, 56–67. doi: 10.1016/j.agee.2015.12.020
Pandiaraj, T., Selvaraj, S., and Ramu, N. (2015). Effects of crop residue management and nitrogen fertilizer on soil nitrogen and carbon content and productivity of wheat (Triticum aestivum L.) in two cropping systems. J. Agric Sci. Tech. 17, 249–260.
Pathak, H., Singh, R., Bhatia, A., and Jain, N. (2006). Recycling of rice straw to improve wheat yield and soil fertility and reduce atmospheric pollution. Paddy Water Environ. 4, 111–117. doi: 10.1007/s10333-006-0038-6
Qian, H. Y., Yang, B. J., Huang, G. Q., Yan, Y. P., and Fang, Z. W. (2012). Effects of returning rice straw to fields with fertilizers and microorganism liquids on soil enzyme activities and microorganisms in paddy fields. Ecol. Environ. Sci. 21, 440–445.
Rajanna, G. A., Dass, A., Suman, A., Babu, S., Venkatesh, P., Singh, V. K., et al. (2022). Co-implementation of tillage, irrigation, and fertilizers in soybean: impact on crop productivity, soil moisture, and soil microbial dynamics. Field Crop Res. 288:108672. doi: 10.1016/j.fcr.2022.108672
Ram, H., Dadhwal, V., Vashist, K. K., and Kaur, H. (2013). Grain yield and water use efficiency of wheat (Triticum aestivum L.) in relation to irrigation levels and rice straw mulching in north West India. Agric. Water Manag. 128, 92–101. doi: 10.1016/j.agwat.2013.06.011
Sah, G., Shah, S. C., Sah, S. K., Thapa, R. B., McDonald, A., Sidhu, H. S., et al. (2014). Tillage, crop residue, and nitrogen level effects on soil properties and crop yields under rice–wheat system in the Terai region of Nepal. GJBAHS 3, 139–147. doi: 10.24925/turjaf.v8i3.610-615.3068
Savant, N. K., and Stangel, P. J. (1990). Deep placement of urea supergranules in transplanted rice: principles and practices. Fertil. Res. 25, 1–83. doi: 10.1007/BF01063765
Sharma, S., Singh, P., Choudhary, O. P., and Neemisha, P. (2021). Nitrogen and rice straw incorporation impact nitrogen use efficiency, soil nitrogen pools and enzyme activity in rice-wheat system in North-Western India. Field Crop Res. 266:108131. doi: 10.1016/j.fcr.2021.108131
Sharma, S., Singh, P., and Kumar, S. (2020a). Responses of soil carbon pools, enzymatic activity and crop yields to nitrogen and straw incorporation in a rice-wheat cropping system in North-Western India. Front. Sus. Food Sys. 4:532704. doi: 10.3389/fsufs.2020.532704
Sharma, S., Thind, H. S., Yadvinder-Singh, S. H. S., Sidhu, H. S., Jat, M. L., and Parihar, C. M. (2019). Effects of crop residue retention on soil carbon pools after 6 years of rice–wheat cropping system. Environ. Earth Sci. 78:296. doi: 10.1007/s12665-019-8305-1
Sidhu, H. S., Singh, M., Humphreys, E., Singh, Y., Singh, B., and Dhillon, S. S., et al. (2007). The happy Seeder enables direct drilling of wheat into rice stubble. Aust. J. Exp. Agric., 47, 844–854. doi: 10.1071/EA06225
Singh, Y., Singh, M., Sidhu, H. S., Humphreys, E., Thind, H. S., Jat, M. L., et al. (2015). Nitrogen management for zero till wheat with surface retention of rice residues in north–West India. Field Crop Res. 184, 183–191. doi: 10.1016/j.fcr.2015.03.025
Singh, P., Singh, G., Sodhi, G. P. S., and Sharma, S. (2021). Energy optimization in wheat establishment following rice residue management with happy Seeder technology for reduced carbon footprints in northwestern India. Energy 230:120680. doi: 10.1016/j.energy.2021.120680
Six, J., Ogle, S. M., Bredit, F. J., Conant, R. T., Mosier, A. R., and Paustian, K. (2004). The potential to mitigate global warming with no-tillage management is only realized when practiced in the long term. Glob. Change Biol. 10, 155–160. doi: 10.1111/j.1529-8817.2003.00730.x
Stagnari, F., Pagnani, G., Galieni, A., D’Egidio, S., Matteucci, F., and Pisante, M. (2020). Effects of conservation agriculture practices on soil quality indicators: A case study in a wheat-based cropping system of Mediterranean areas. Soil Sci. Plant Nutr. 66, 624–635. doi: 10.1080/00380768.2020.1779571
Su, S. L., Singh, D. N., and Baghini, M. S. (2014). A critical review of soil moisture measurement. Measurement 54, 92–105. doi: 10.1016/j.measurement.2014.04.007
Subbiah, B. V., and Asija, G. L. (1956). A rapid procedure for the estimation of available nitrogen in soils. Curr. Sci. 25, 259–260.
Sun, S. Q., Cai, H. Y., and Chang, S. (2015). Sample storage-induced changes in the quantity and quality of soil labile organic carbon. Sci. Rep. 51:7496. doi: 10.1038/srep17496
Tabatabai, M. A., and Bremner, J. M. (1969). Use of p-nitrophenyl phosphate for assay of soil phosphatase activity. Soil Biol. Biochem. 1, 301–307. doi: 10.1016/0038-0717(69)90012-1
Tan, Z. J., Li, Q., Chen, D. L., Zhou, Q. M., Xiao, Q. M., and Li, J. G. (2006). On the effect of rice straw returned to the field on microbes and enzyme activity in paddy soil. Acta Ecol. Sin. 26, 3385–3392.
Tang, W., Cerdán-García, E., Berthelot, H., Polyviou, D., Wang, S., Baylay, A., et al. (2020). New insights into the distributions of nitrogen fixation and diazotrophs revealed by high-resolution sensing and sampling methods. ISME J. 14, 2514–2526. doi: 10.1038/s41396-020-0703-6
Usman, K., Khan, E. A., Khan, N., Khan, M. A., Ghulam, S., Khan, S., et al. (2013). Effect of tillage and nitrogen on wheat production, economics, and soil fertility in rice–wheat cropping system. Am. J. Plant Sci. 4, 17–25. doi: 10.4236/ajps.2013.41004
Varatharajan, T., Dass, A., Choudhary, A. K., Sudhishri, S., Pooniya, V., Das, T. K., et al. (2022). Integrated management enhances crop physiology and final yield in maize intercropped with blackgram in semiarid South Asia. Front. Plant Sci. 13:975569. doi: 10.3389/fpls.2022.975569
Walkley, A., and Black, I. A. (1934). An examination of the degtjareff method for determining soil organic matter and a proposed modification of the chromic acid titration method. Soil Sci. 37, 29–38. doi: 10.1097/00010694-193401000-00003
Whitman, T., Peperanney, C., Enders, A., Koechli, C., Campbell, A., Buckley, D. H., et al. (2016). Dynamics of microbial community composition and soil organic carbon mineralization in soil following addition of pyrogenic and fresh organic matter. ISME J. 10, 2918–2930. doi: 10.1038/ismej.2016.68
Wright, S. J., Centonze, V. E., Stricker, S. A., DeVries, P. J., Paddock, S. W., and Schatten, G. (1993). Introduction to confocal microscopy and three-dimensional reconstruction. Methods Cell Biol., 4, 1–45. doi: 10.1016/S0091-679X(08)60998-X
Yadav, G. S., Das, A., Lal, R., Babu, S., Meena, R. S., Saha, P., et al. (2018). Energy budget and carbon footprint in a no-till and mulch-based rice–mustard cropping system. J. Cleaner Produ. 191, 144–157. doi: 10.1016/j.jclepro.2018.04.173
Keywords: rice residue, organic carbon, microbial dynamics, soil dynamics, wheat productivity
Citation: Chaudhary C, Yadav DB, Hooda VS, Chaudhary A, Parshad J, Kumar A, Khedwal RS and Yadav A (2024) Rice residue management alternatives and nitrogen optimization: impact on wheat productivity, microbial dynamics, and enzymatic activities. Front. Sustain. Food Syst. 8:1402803. doi: 10.3389/fsufs.2024.1402803
Edited by:
Johann G. Zaller, University of Natural Resources and Life Sciences Vienna, AustriaReviewed by:
Anchal Dass, Division of Agronomy, Indian Council of Agricultural Research (ICAR), IndiaParvender Sheoran, Central Soil Salinity Research Institute (ICAR), India
S. M. Mofijul Islam, Bangladesh Rice Research Institute, Bangladesh
Copyright © 2024 Chaudhary, Yadav, Hooda, Chaudhary, Parshad, Kumar, Khedwal and Yadav. This is an open-access article distributed under the terms of the Creative Commons Attribution License (CC BY). The use, distribution or reproduction in other forums is permitted, provided the original author(s) and the copyright owner(s) are credited and that the original publication in this journal is cited, in accordance with accepted academic practice. No use, distribution or reproduction is permitted which does not comply with these terms.
*Correspondence: Virender S. Hooda, dnNob29kYTc5QGdtYWlsLmNvbQ==