- 1Food & Agriculture, The Breakthrough Institute, Berkeley, CA, United States
- 2School of Business, Universidad Torcuato Di Tella, Buenos Aires, Argentina
Alternative meat (AltMeat), which includes plant-based and cultured meats, has the potential to improve the global food supply chain on numerous environmental and societal dimensions. Some of these gains, derived from lower animal meat consumption, could disrupt the supply chain of crops that are used as animal feed. This study evaluates the potential impact of animal meat displacement on major corn and soybean-producing regions, globally or in developed regions. We combine trading patterns and regional cost structures with potential crop demand reductions caused by animal meat displacement, to occur by 2032 on top of an Organisation for Economic Co-operation and Development and the Food and Agriculture Organization of the United Nations (OECD-FAO) baseline projection without AltMeat. We find that potential animal meat displacement in the US and the European Union (EU) would have a minor effect on crop markets. Worldwide displacement, however, would lead to significantly lower corn and soybean prices relative to the baseline OECD-FAO projection without AltMeat. We explore quantitatively the heterogeneous impact of such developments in crop-producing regions. Our findings shed light on a trade-off associated with the significant benefits of more sustainable meat production.
1 Introduction
Alternative meat (AltMeat), a class of human food that includes plant-based meat (PBM) and cultured meats, is a recent radical innovation with the potential, if scaled up, to bring significant environmental, dietary, and economic benefits (Post et al., 2020; Mazac et al., 2022; Dueñas-Ocampo et al., 2023; Mylan et al., 2023). AltMeat potentially reduces the need for animal meat and for its very large animal feed requirements. Organisation for Economic Co-operation and Development and the Food and Agriculture Organization of the United Nations (OECD-FAO) projects that in 2032, 57% of global corn and 70% of global soybean production (as meals) will be used as animal feed, in line with feed utilization ratios for 2020–2022. Baldi and Merener (2023) estimated that under a potential 3% global animal meat displacement by 2032, mean feed crop prices would be 10–18% lower than under existing OECD-FAO projections without AltMeat. In this study, we explore the potential impact of such events on major corn and soybean producing regions. We address these questions through a first-order quantitative analysis that brings together a crop demand reduction defined by the displacement of animal meat under current feed utilization rates and global supply that aggregates regional producers with heterogeneous trading and productivity structures. We neither model AltMeat growth nor assume a specific ratio of AltMeat growth to animal meat displacement. Rather, we start from an assumed animal meat displacement, compute its associated reduction in corn and soybean demand, and derive the new global and regional equilibrium in crop output and prices. This event is uncertain given the complexity of the required transition (Dueñas-Ocampo et al., 2023), the very early stage of cultured meat marketing, recently stagnating sales of plant-based meat in the United States, challenges for further adoption in other geographies (Newton et al., 2024; Erfanian et al., 2024) and the unsure link between AltMeat adoption and effective animal meat substitution (Neuhofer and Lusk, 2022; Zhao et al., 2022; Taylor et al., 2023; Tonsor et al., 2023). However, motivated by its potential significance, we interpret our work as a meaningful risk assessment exercise. Our analysis contemplates the possibility of soybean usage as a direct input to plant-based meat, although its effect is small. AltMeat is represented as scenarios of animal meat displacement relative to the 2023 OECD-FAO Agricultural Outlook, which projects that animal meat and feed crop global output for 2032 will be 13% higher than 2020–2022 levels (OECD/FAO, 2023).
Newton and Blaustein-Rejto (2021) and Morais-da Silva et al. (2022) performed extensive interviews in the US and Brazil to assess the social and economic impacts on the transition from animal meat to plant-based meat. Farming disruptions caused by a decrease in demand for feed crops were identified as a potential risk. This study aims to contribute with an early quantitative assessment of the potential impact of AltMeat growth on the main animal feed-producing regions.
The literature has already presented quantitative measures for the impact of plant-based meat on agricultural output. Wirsenius et al. (2010) scenarios show that a 25% decrease in meat consumption per capita in developed regions and a 20% global substitution of ruminant meat consumption with pork and poultry would significantly decrease the total agricultural area required for food production. Stehfest et al. (2009) analyzed the substitution of animal proteins by those sourced from plants between 2010 and 2030 and found that the same amount of proteins would be obtained with much less land. Lusk et al. (2022) developed a model of the US beef market and found that a 10% plant-based meat price decrease would lead to a minor effect on US cattle output due to its low elasticity of supply and low cross elasticity of demand between plant-based meat and ground beef. Kozicka et al. (2023) analyzed large-scale shifts from animal-sourced foods to plant-based foods by 2050 and found major reductions in global greenhouse gas emissions from agriculture, land use, biodiversity loss, and both crop and animal-sourced food prices. This study focuses on output and price effects by 2032, conditional on regional or global displacements of beef, pork, and poultry meat that would be driven by growing access to AltMeat in the form of plant-based and cultured meats.
2 Materials and methods
2.1 A model for animal feed
We focus on the supply chain of corn and soybeans, two of the most important crops that are used to produce animal meat such as beef, pork, and poultry. We study the long-term permanent effects of crop demand reduced by the displacement of animal meat, therefore, we disregard storage that may smooth price variations in the short run but not on a 10-year horizon. In line with the evidence in the study by Merener (2015) we assume that the markets for corn or soybeans are largely globally integrated. Table 1 displays statistics for corn and soybeans projected in OECD/FAO (2023) for 2032. This is the horizon for the risk assessments of our study. Most of the global soybean output is crushed and transformed into soybean meal, at an approximate conversion ratio of 77% of bean weight and soy oil. Roughly 57% of corn and 70% of soybeans (in the form of soybean meal) are projected to be used as animal feed, or as input for feed. These utilization ratios are similar to those reported by the OECD-FAO for 2020–2022. Crucially, figures in Table 1 are projected by the OECD-FAO under the assumption of no significant AltMeat growth. Therefore, we will use these estimates as the baseline case over which we will impose crop demand reductions assumed to be induced by AltMeat growth. The starting point of our modeling work is a decomposition of the world in a collection of i = 1, ..., N regions in Table 1 that are either leading crop exporters or major crop importers.
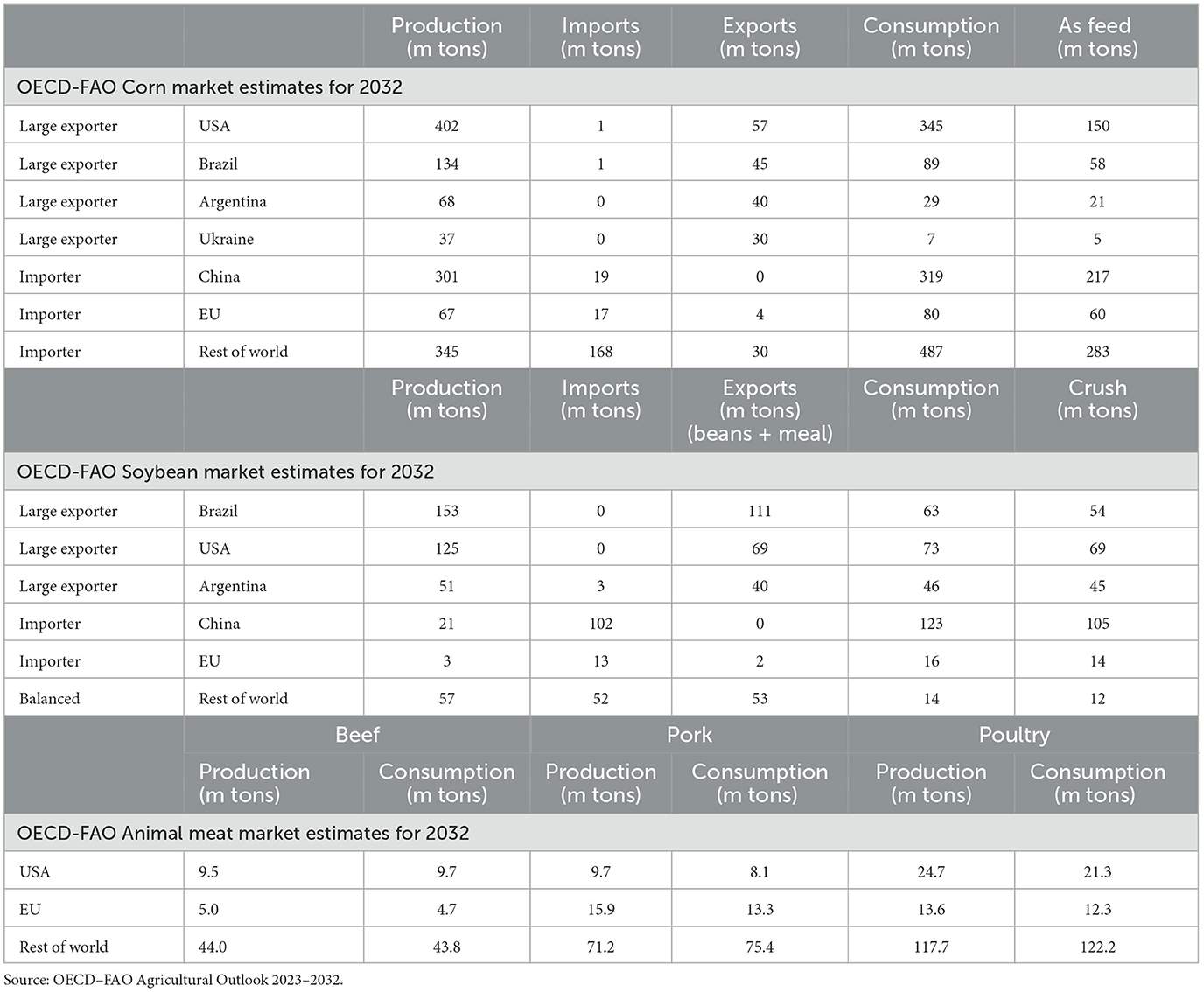
Table 1. Projected world production, trade and consumption statistics for feed crops and animal meat by 2032.
2.2 Feed demand
As in the simplest model considered in the study by Baldi and Merener (2023), we assume a global crop demand reduction defined in terms of the animal feed no longer needed after an animal meat consumption reduction of a certain magnitude has been induced by AltMeat. We do not model the link between AltMeat growth and animal meat displacement. Local demand reductions occur on top of a baseline demand and are driven by consumer choices. As such, they might occur in exporting and importing regions. In this study we focus on meat consumption in the United States and the European Union (EU), which, by their living standards and disposable income, are likely to lead to the adoption of environmentally friendly innovations (Pfeiffer and Mulder, 2013; Elgin et al., 2023), and the world as a whole. Animal meat market statistics projected by OECD-FAO for 2032 under the assumption of no significant AltMeat growth are reported in Table 1.
The crop demand reduction is a function of animal meat displacement and feed utilization parameters in Table 2. The tonnage of corn used to produce beef consumed in region i in the baseline scenario is Mbeef,iibeeffbeefcbeef. Expressions for other animal meats or crops are analogous. In the case of soybean, we adjust by a factor of 1/0.77 because we work with soybeans in our computations, but the actual feed mix usually contains soybean meal. The decrease in crop demand in region i is determined by exogenous animal meat replacement shares {dbeef,i, dpork,i, dpoultry,i}. While AltMeat growth could decrease corn and soybean consumption by lowering animal feed demand, it could also increase demand for crops that are used as a direct input to plant-based meat. This is currently the case for soybeans. We include this effect in our analysis through parameters rc,PBM and rs,PBM although its size is likely small. Corn and soybeans would not be required as inputs in cultured meat, therefore, the impact of a sustainability transition driven purely by this type of novel meat would be slightly larger than the estimates reported in this study. In net, the exogenous crop demand shift associated to meat displacement in region i is
An exogenous demand reduction caused by animal meat displacement would be expected to induce a price decrease and, therefore, lead to extra demand for other crop uses. For instance, increased biofuel production or higher human consumption. These effects are captured by the elasticity of demand, γ < 0. Let P0 and P be global crop prices under the baseline OECD projection without AltMeat and after the adoption of AltMeat, respectively. Baseline and post-AltMeat demand are D0 and D(P). Under a linear supply and demand model, appropriate for small changes in crop markets, global demand is
Table 3, summarized from Baldi and Merener (2023), displays approximate global estimates for animal meat production and feed crop utilization to implement Equations 1 in 2. Industrial methods are more prevalent in poultry and pork than in beef, where grass, forage or mixed systems are widely used. Poultry uses the smallest amount of feed per kg of meat, and beef is the least efficient in this regard. Estimates of the proportion of corn and soybean in the feed of ruminants, pork and poultry, show significant variation across animal species. Information on the composition of plant-based meat products is very scarce. Among some of the best-known products, Beyond Meat's burger uses proteins from peas and beans. Impossible Foods includes soy and potato protein, among other inputs. Approximately 50% of soybean mass is protein and the protein weight in a typical plant-based meat burger is about 20% of its total weight. Therefore, soybean mass per kilogram of plant-based meat ranges between zero and 0.40 kg. This is an order of magnitude smaller than the mass conversion ratios from animal feed to animal meat in Table 3.
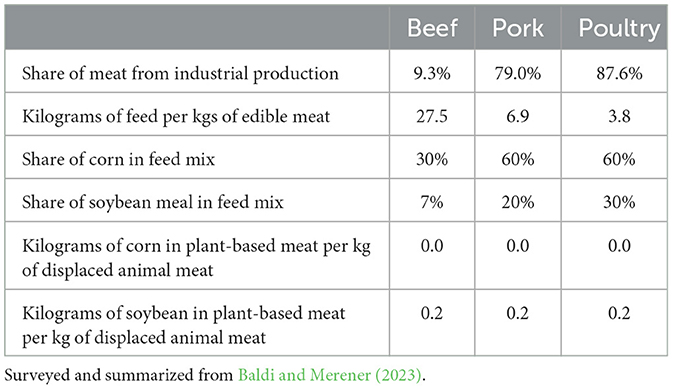
Table 3. Estimates for industrial animal meat production, feeding efficiency, feed mix composition and plant-based meat composition for beef, pork and poultry.
2.3 Regionally distributed feed supply
It is important to recognize that exporters and importers in Table 1 are likely to behave differently in response to a demand reduction. In particular, transportation costs from distant sources, and strategic and political considerations in the EU and China, two main corn and soybean importers, suggest that a decrease in the global demand for crop and soybeans may be absorbed first by a reduction in imports rather than a reduction in local production (Peters et al., 2009, Bellmann and Hepburn, 2017). To explore the potential implications of different regional adjustment policies on the impact of AltMeat, we consider three alternative crop supply structures. In all of them we use a global estimate for the elasticity of crop supply, β, from the literature. Crop supply structures differ on the regional attribution of global supply adjustments. Let be the supply in region i in the absence of AltMeat and Si(P), S(P) be the regional and global crop outputs, respectively, under AltMeat adoption.
Supply structure 1. In this case, we assume that all producers respond to reduced demand in the same manner, regardless of their exporting/importing status. Under a linear model, we have
Supply structure 2. Here we assume that the adjustment of global supply falls entirely on large exporters, while importers and balanced regions maintain their output at their pre-AltMeat levels. For global output to be elastic to prices as β, exporters must be more responsive than in the supply structure 1. Linearizing we have
Supply structure 3. In our third supply specification, we focus on the fact that there is significant heterogeneity in profit margins across crop-producing regions. Supply from crop-exporting regions responds to changes in profitability induced by changes in global demand. The price per ton of crop perceived by local farmers in region i is Pi and may differ from the global market crop price P due to the existence of subsidies (as in the United States in certain scenarios adverse for farmers) or taxes (as currently in Argentina). We assume that this effect is captured by a factor Ii so that Pi = IiP. Production cost per ton in each region is the sum of a fixed and variable component. We aim to capture the notion that certain inputs, such as labor, fuel, and machinery, machinery are generally uncorrelated with crop prices, while fertilizer, seeds, and land rental are strongly and positively correlated with local crop prices (Schnitkey et al., 2021a). Hence the cost per ton in region i is
for positive ηi and ψi that represent the fixed and variable cost components. Farmer economic profit per ton is IiP − Ci(P). Under a local supply function that is linear in local profits, while holding the global elasticity of supply equal to β, exporter supply can be approximated as
In this specification we continue to assume that output in importing regions is unaffected by a relatively small reduction in global demand so that .
Baseline levels of crop output for producers are in Table 1 and are sufficient to implement supply structures 1 and 2 in Section 2. Supply structure 3 depends on local profitability, determined by the price received by farmers and their cost of production. Farmers' income depends on taxes, subsidies, and transportation costs to main destinations. In Table 4 we report regional revenue and costs in USD per ton for 2015–2019. It is important to note that this was a period of moderate commodity prices by historical standards, after the end of the supercycle in 2014 and prior to the run-up in prices after the COVID-19 pandemic. Average CME prices during this period were 145 and 340 USD/ton for corn and soybeans, respectively.
It is standard practice to differentiate between direct costs, operating costs, and overhead. Up to 90% of direct costs are fertilizers, seeds, and pesticides. A primary input for fertilizers is ammonia, which had a 74 % correlation with corn between 2008 and 2021 (Schnitkey et al., 2021b). Overall direct costs in Illinois had a 68% correlation with corn prices between 2000 and 2021 (Schnitkey et al., 2021a). Operating costs include labor, machinery, repairs, and fuel. These items are much less sensitive to crop prices than direct costs. Overhead costs include land, building depreciation, and insurance. Land costs can be expressed as a ratio of expected future farmer income, itself a function of current crop prices, and long-term interest rates. This standard theoretical construction diverged from market prices in recent years in the US when interest rates approached zero. Following widespread inflation and interest rate increases in the aftermath of the COVID-19 pandemic, land costs are once again in closer agreement with the theoretical prediction (Schnitkey et al., 2021a). Colussi et al. (2023) documented that croplands in Brazil increased 128 % on average from 2019 to 2022, as corn and soybean prices received by local farmers almost doubled in the same period. In sum, we will assume in our computations that direct costs and overhead are highly correlated with crop prices while operating costs are not. In some cases discussed in Table 4, net farmer profits are negative. However, as discussed in the studies by Saavoss et al. (2021) and Vaiknoras and Hubbs (2023), this has been often the case historically due to the inclusion of the opportunity cost of land and unpaid farmer labor in the computation of cost measures. Since farmers are often not paying rent for their land nor wages for their own labor, negative net profits do not imply that farms have negative accounting profits. We translate regional supply information provided in Table 4 to the parameters used in the supply function in Equation 6 through the following algebraic identities
Calibrated values for these parameters are in Table 5.
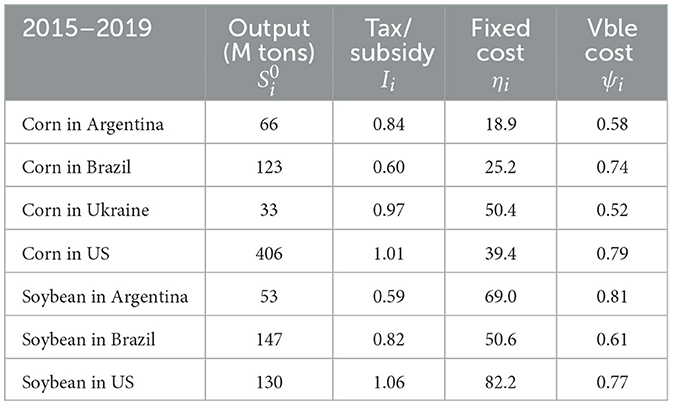
Table 5. Model parameters for supply structures calculated from empirical data in Table 4.
2.4 Equilibrium under animal meat displacement
For any supply structure specified above, the market impact of animal meat displacement is given by the change in crop price, ΔP = P − P0, that leads to the satisfaction of the market clearing condition
The collection of local supply functions Si(P) incorporates information on regional crop production as expressed in Equation 7. Exogenous demand reductions Zi combine in Equations 1, 2 the magnitude of the animal meat displacement induced by AltMeat. In addition, Equation 7 depends on the elasticities of supply, β, and demand, γ. For these, we rely on supply and demand elasticities for corn, soybeans, and calories, as discussed in the study by Roberts and Schlenker (2009, 2013). Using global data from 1960 to 2007, these studies combined yield variations, weather shocks and variations in inventories to obtain a wide number of elasticity estimates. Roberts and Schlenker (2013) aggregated corn and soybeans by their caloric content given their partial substitutability and complementarity as sources of animal feed. We take the sharpest estimates from the study by Roberts and Schlenker (2013) for calories, which are more strongly identified than those for individual crops, and apply them to corn and soybeans individually. We rely on the notion that very unequal price impacts on corn vs. soybeans would be limited by substitution in the short run and by planting decisions in the long run. The average values for the estimates provided in the studies by Roberts and Schlenker (2009, 2013) are β = 0.11 for the elasticity of supply and γ = −0.05 for the elasticity of demand. In our numerical experiments, we solve Equation 7 for crop price and regional output changes.
2.5 AltMeat growth and animal meat displacement scenarios
We follow the approach discussed in the study by Baldi and Merener (2023) and propose a plausible animal meat displacement scenario based on quantitative and qualitative information. Our results in this study, should be understood as risk assessments conditional on animal meat displacement scenarios rather than precise forecasts for which there is no sufficient information. Acceptance for plant-based meat grew strongly until 2021, and more so among those who are educated, younger, more liberal, from urban areas, and concerned about animal treatment (Slade, 2018; Godfray et al., 2018; Hartmann and Siegrist, 2019; Bryant et al., 2019; Van Loo et al., 2020; Szejda et al., 2021; Neuhofer and Lusk, 2022; Zhao et al., 2022; Taylor et al., 2023). This suggested a gradual, relative decrease in animal meat consumption led by aging and generational change. Forecasts published prior to the pandemic for 2030 plant-based meat market share were in the 2–10% range. This was consistent with a 2020 share in the 1–2% range and subsequent annual growth rate between 6 and 18%. In recent years, plant-based meat made inroads in becoming relatively more affordable, but it is still a premium product. However, plant-based meat sales have stagnated since 2021, as budget-conscious consumers have been reluctant to embrace it during the inflation peak that followed the COVID-19 pandemic1. Interest among investors and funding for plant-based startups rose dramatically between 2017 and 2021 and cooled off since 2022 in line with the general retrenchment of investment on risky disruptive technologies induced by higher interest rates2.
Cultured meat is produced from animal cells through sophisticated lab-based processes (Hocquette, 2016; Treich, 2021). While energy-intensive to produce (Lynch and Pierrehumbert, 2019), cultured meat is projected to have some environmental benefits (Mancini and Antonioli, 2022). Cultured meat is being evaluated in Europe (Lanzoni et al., 2024) and has already been approved for sale in the United States, Israel, and Singapore.
The alternative dairy market is a relevant medium term-growth benchmark for the AltMeat market. Animal milk consumption in the United States has been replaced in part by plant-based milk (Wolf et al., 2020). The American plant-based milk category accounts for about 14% of the dairy milk market. In our numerical experiments, we will consider a potential scenario in which AltMeat will induce the displacement of 3% of the animal meat consumption by 2032 relative to the current OECD-FAO projections, which are, in turn, 14% larger than the animal meat consumption in 2019–2021. We will also consider displacement scenarios for individual types of meat, and for specific regions. Based on cost and consumer preferences, it could be expected for AltMeat to be adopted first in highly developed regions as the United States and Europe, where the population may have more disposable income to allocate toward environmentally friendly products. We stress that this research study is not a forecast of AltMeat growth but an assessment of its potential impact on crop markets.
3 Results and discussion
In our animal meat displacement scenarios, crop demand is lower than the baseline demand projected for 2023 by OECD-FAO in Table 1. We condition on these demand shifts and use Equation 7 to calculate the equilibrium price change by year 2032 for corn and soybeans, and the regional distribution of changes in supply. Our results must be interpreted as price changes relative to a baseline case without AltMeat. We compare results for the displacement of specific animal meat types and in developed economies represented by the United States and EU vs. a worldwide transition. We present results for the global impact of a 3% animal meat displacement in Table 6. Since OECD-FAO projects a 14% growth in total animal meat output between 2019–2021 and 2032, our results still assume significant positive growth in animal meat output vs. 2021 levels. The results presented in Table 6 focus on the global crop market impact of alternative regional adjustments and therefore hold for any supply structure discussed in Section 2.
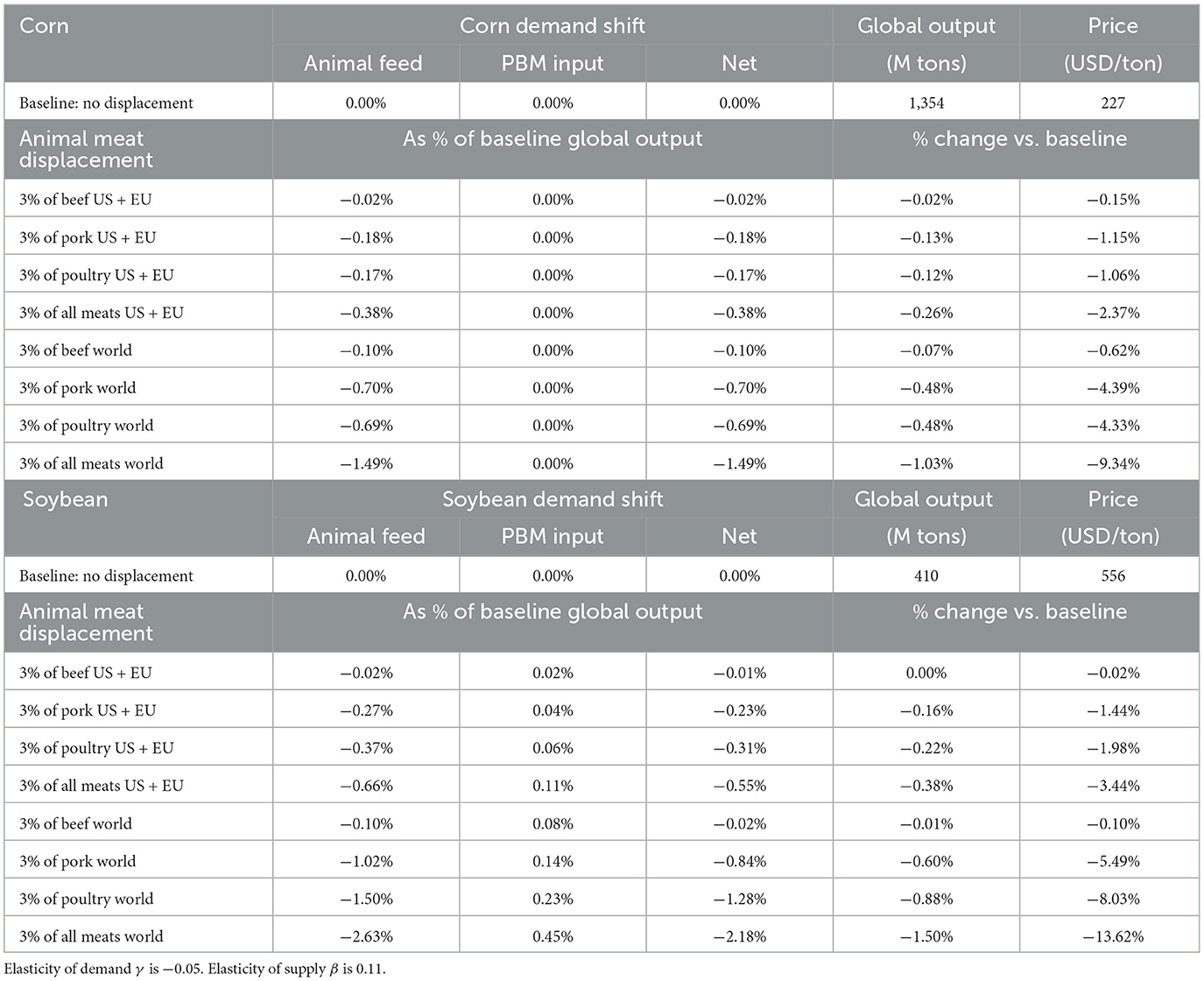
Table 6. Equilibrium shifts in the corn and soybean markets in 2032, in response to animal meat displacement scenarios induced by alternative meat.
Columns 2–4 in Table 6 display changes in crop demand relative to the OECD-FAO baseline. The changes for corn are entirely due to the reduced use of it as animal feed. Based on available information from plant-based meat producers, we assume that corn is not used as an input in their products. In soybeans, lower demand induced by smaller feed demand is partially compensated by the positive demand of this crop as plant-based meat input. This compensation would not occur if animal meat displacement is induced purely by cultured meat. The effect of increased demand due to plant-based meat is particularly strong in beef because <10% of this type of meat is produced industrially (Table 3). Hence, animal beef does not require significant amounts of soybean as feed and therefore this could be comparable to the amount of soybean used as direct input to plant-based meat.
The net crop output changes, as listed in column 5 of Table 6, are smaller in magnitude than demand reductions. The crop price shifts in column 6 of Table 6 are several times larger than the crop output changes for all scenarios—a consequence of the low elasticity of crop supply. Table 6 shows that animal beef displacement, in the United States + EU, or in the world as a whole, would have a very minor effect on crop prices. Results for poultry and pork are larger in soybeans than in corn because the latter is more largely used as a source of animal feed. Simultaneous displacement for all meat types in the United States and EU would lead to −2.1 and −3.0% lower prices in corn and soybeans, respectively, relative to the OECD-FAO baseline without AltMeat. Worldwide displacement induced by AltMeat adoption for pork, poultry, and all types of meat would lead to increasingly negative market impacts up to exceeding 10.0%. Our approach in Section 2 implies a linear relationship between the magnitude of the displacement and its market impact. Hence, the results shown in Table 6 for different meat types and regions can be simply scaled proportionally to gauge the impact of AltMeat induced shifts smaller or larger than 3%.
Our results in Table 6 are obtained under elasticities from the literature. These estimates were successful in explaining the impact of the US government's ethanol mandate on corn, a permanent positive demand shock that lead to a permanent corn price increase in the vicinity of 30%. The feed crop market could respond differently to a negative demand shock. For instance, an initially significant crop price decrease could induce in the medium term novel uses for these crops. Similarly, in response to persistently lower prices, crop producers could improve production efficiency and be able to avoid a sharp production reduction. Therefore, for robustness, we compute market responses using elasticity estimates in an enlarged range. The results are in Table 7. Some reported cases have the same price impact because this is proportional to the multiplier 1/(−γ+β), and they all show that a −3% animal meat displacement would have a robust effect on feed crop markets. In sum, while the response to an uncertain permanent demand reduction induced by AltMeat is very difficult to project precisely, a large economic impact would occur under an ample range of elasticities, simply because of the very large utilization ratio of corn and soybeans as sources of animal feed.
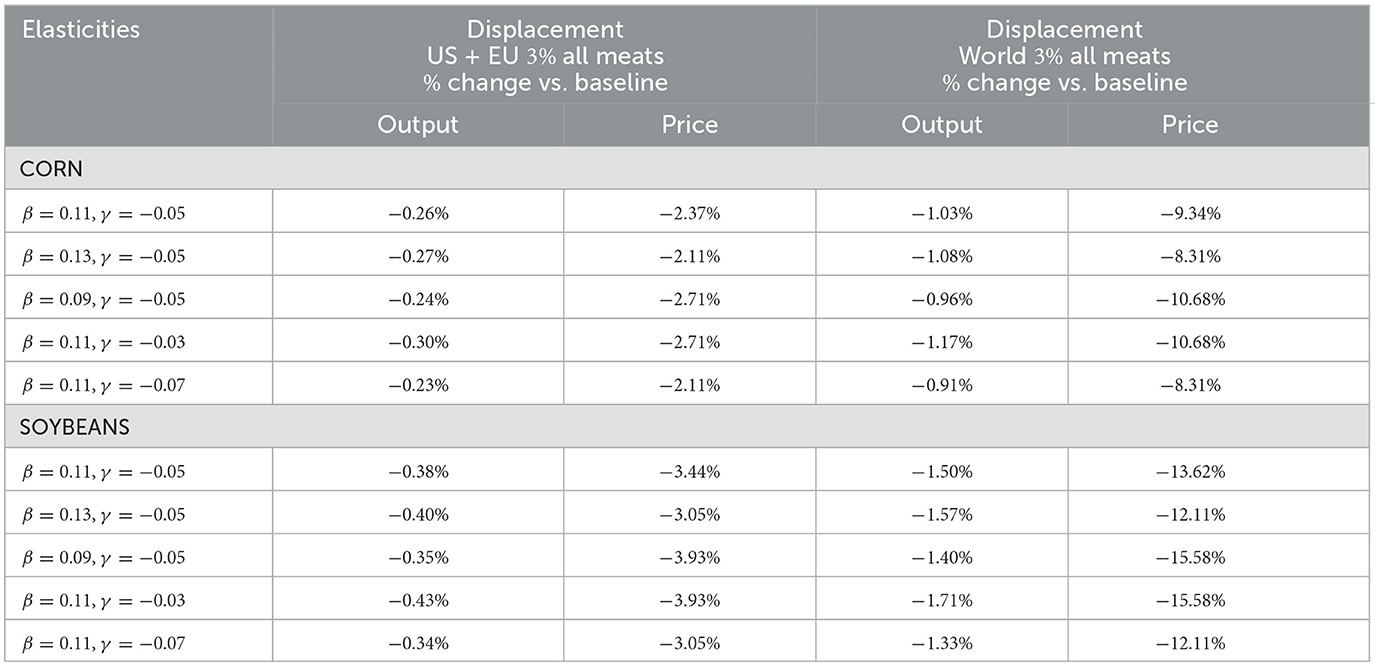
Table 7. Sensitivity of results in Table 6 with respect to changes in the elasticities of demand γ and supply β.
The impact of a 3.0% displacement for all types of animal meat demand globally, on regional crop supply, is presented in Tables 8, 9. The price impact reproduces that already reported in Table 6. Our focus is now about the regional allocation of the adjustment under the three supply structures proposed in Section 2. Supply structure 1 accommodates weaker demand equally across producers, regardless of their trading status. Corn and soybean net demand shifts are −1.5 and −2.2%, respectively. Their output would fall, in turn, by 1.0 and 1.5% (Tables 8, 9), and is shared equally by all producers under supply structure 1. The stronger decline in soybean output is due to its relatively higher usage as feed crop. Supply structure 2 builds on the notion that importers, such as the EU and China, would first react by cutting imports while protecting and maintaining local output unchanged. In this case, corn and soybean output by exporters fall by 2.2 and 1.9%. The impact on corn and soybean exporters inverts in importance relative to the first supply structure. This is due to the fact that major soybean exporters command a much larger market share of global soybean supply than it is the case in the corn market. The United States, Brazil, and Argentina produce roughly 80% of global soybean output. By contrast, the United States, Brazil, Argentina, and Ukraine produce only 47% of world corn. Therefore, relatively smaller corn exporters accommodating the full global adjustment show a more significant output decline. Finally, column 5 of Tables 8, 9 show results under supply structure 3, which incorporates significant heterogeneity and crop price dependence in the composition of income and costs across producers. Local output is assumed linear in local profits (Equation 6). Corn output from Argentina and Ukraine would experience the strongest declines at −3.3 and −4.4%, respectively. Combined with a simultaneous −9.3% decline in corn price, local revenues would be 10% lower than the OECD-FAO projections without AltMeat. This would have macroeconomic significance for these countries. In soybeans, as listed in Table 9, the most affected country would be Brazil, at −2.3% physical output decline and −16% in revenues. Under a 3% animal meat displacement, the United States' output does not fall beyond 3% in any crop or supply specification under consideration. Naturally, larger displacements would lead to larger revenue declines. For both crops, strong supply reductions are driven by low values in the variable cost coefficient ψ as shown in Table 5. The reduction in profits induced by a 3% animal meat displacement, through lower crop prices, lower revenues, and lower costs could imply negative profits in some regions, but we stress that our measures of net profit include the opportunity cost of land and unpaid farmer labor. Without these items, operating profits typically remain positive. Tables 8, 9 show the degree in which less flexible crop exporters might be more exposed to a permanent decrease in animal feed demand.
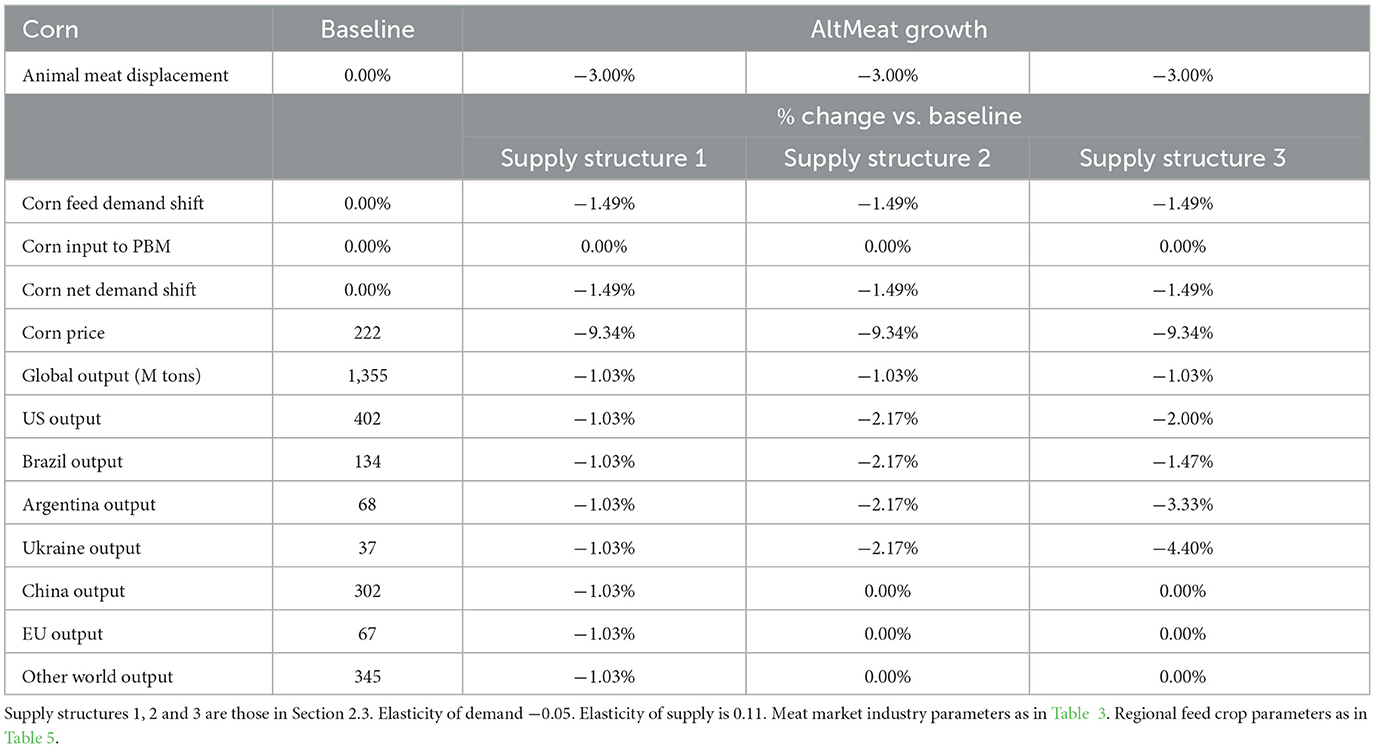
Table 8. Global and regional equilibrium shifts in the corn market in 2032, in response to a 3% displacement of all types of animal meat across the world.
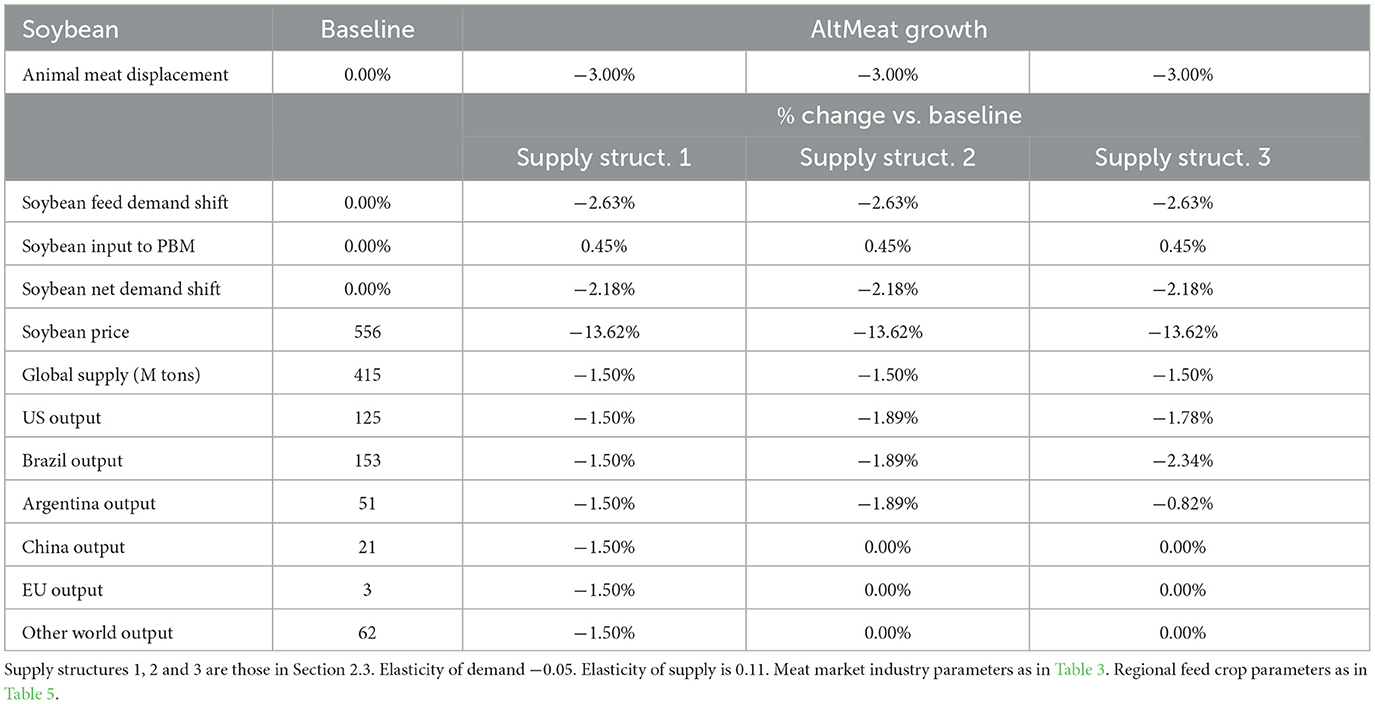
Table 9. Global and regional equilibrium shifts in the soybean market in 2032, in response to a 3% displacement of all types of animal meat across the world.
We have represented heterogeneity across countries but in reality there is also significant variation in productivity and costs across regions within a single country (Saavoss et al., 2021; Vaiknoras and Hubbs, 2023). This is likely to influence the finer spatial distribution of output adjustments in the event of AltMeat growth. Our calculations already include potential demand for soybeans as direct inputs to plant-based meat. In addition, although we have not modeled in our study, plant-based meat would demand a large increase in the global supply of peas and legumes used as ingredients (Newton and Blaustein-Rejto, 2021). Some of this growth could use available land in the United States, Brazil, Argentina, and Ukraine. The complement of the important reduction in farmer profitability would be lower food and biofuel costs faced by consumers all over the world. This impact would be particularly pronounced in low-income and developing countries, where a larger portion of the consumption basket is devoted to food staples than in developed countries. With the negative impact of lower corn and soybean prices being absorbed by a few major exporters, widespread adoption of AltMeat could improve overall welfare not only through the dietary benefits for its consumers but also through environmental and redistributive effects shared much more widely. For proper policy and global welfare analysis, our findings should not be evaluated in isolation but jointly with the extensive literature on the likely benefits associated with more sustainable meat production (Santo et al., 2020). In particular, lower demand for animal feed crops might slow, or even revert, land-use changes (Wright and Wimberly, 2013; Mazac et al., 2022). AltMeat growth could mitigate environmental stress imposed by a changing climate on agricultural supply chains (Desiderio et al., 2023; Lesk et al., 2021; Cornejo et al., 2024). Finally, AltMeat adoption might also strengthen food sustainability and alleviate consumer concerns about animal treatment by the traditional meat industry (De Boer and Aiking, 2011; Nelson et al., 2016; Godfray et al., 2018; El Bilali et al., 2019; Rubio et al., 2020).
Data availability statement
The original contributions presented in the study are included in the article/supplementary material, further inquiries can be directed to the corresponding author.
Author contributions
DB-R: Conceptualization, Funding acquisition, Writing – review & editing. NM: Conceptualization, Formal analysis, Investigation, Methodology, Writing – original draft. AS: Data curation, Investigation, Writing – review & editing.
Funding
The author(s) declare financial support was received for the research, authorship, and/or publication of this article. This research was supported by The Food System Research Fund.
Conflict of interest
The authors declare that the research was conducted in the absence of any commercial or financial relationships that could be construed as a potential conflict of interest.
Publisher's note
All claims expressed in this article are solely those of the authors and do not necessarily represent those of their affiliated organizations, or those of the publisher, the editors and the reviewers. Any product that may be evaluated in this article, or claim that may be made by its manufacturer, is not guaranteed or endorsed by the publisher.
Footnotes
1. ^https://www.bloomberg.com/news/articles/2022-08-04/beyond-meat-falls-after-slashing-sales-outlook-as-demand-wanes
2. ^https://pitchbook.com/news/articles/vc-deals-fall-plant-based-protein-startups
References
Baldi, F., and Merener, N. (2023). Displacement Risk in Agricultural Commodity Markets: The Potential Impact of Alternative Meat. doi: 10.2139/ssrn.3940081
Bellmann, C., and Hepburn, J. (2017). The decline of commodity prices and global agricultural trade negotiations: a game changer? Int. Dev. Policy. doi: 10.4000/poldev.2384
Bryant, C. J., Szejda, K., Deshpande, V., Parekh, N., and Tse, B. (2019). A survey of consumer perceptions of plant-based and clean meat in the USA, India, and China. Front. Sustain. Food Syst. 3:11. doi: 10.3389/fsufs.2019.00011
Colussi, J., Schnitkey, G., Paulson, N., and Baltz, J. (2023). Farmland Prices in Brazil More Than Doubled in the Last Three Years, Vol. 13. Farmdoc Daily, Department of Agricultural and Consumer Economics, University of Illinois at Urbana-Champaign.
Cornejo, M., Merener, N., and Merovich, E. (2024). Extreme Dry Spells and Larger Storms in the US Midwest Raise Crop Prices. doi: 10.2139/ssrn.4514667
De Boer, J., and Aiking, H. (2011). On the merits of plant-based proteins for global food security: marrying macro and micro perspectives. Ecol. Econ. 70, 1259–1265. doi: 10.1016/j.ecolecon.2011.03.001
Desiderio, E., Shanmugam, K., and Östergren, K. (2023). Plant based meat alternative, from cradle to company-gate: a case study uncovering the environmental impact of the Swedish pea protein value chain. J. Clean. Prod. 418:138173. doi: 10.1016/j.jclepro.2023.138173
Dueñas-Ocampo, S., Eichhorst, W., and Newton, P. (2023). Plant-based and cultivated meat in the United States: a review and research agenda through the lens of socio-technical transitions. J. Clean. Prod. 405:136999. doi: 10.1016/j.jclepro.2023.136999
El Bilali, H., Callenius, C., Strassner, C., and Probst, L. (2019). Food and nutrition security and sustainability transitions in food systems. Food Energy Sec. 8:e00154. doi: 10.1002/fes3.154
Elgin, C., Özgür, G., and Cantekin, K. (2023). Measuring green technology adoption across countries. Sustain. Dev. 31, 1–11. doi: 10.1002/sd.2368
Erfanian, S., Qin, S., and Dayo, M. A. (2024). Cultivating a greener plate: understanding consumer choices in the plant-based meat revolution for sustainable diets. Front. Sustain. Food Syst. 7:1315448. doi: 10.3389/fsufs.2023.1315448
Godfray, H. C. J., Aveyard, P., Garnett, T., Hall, J., Key, T., Lorimer, J., et al. (2018). Meat consumption, health, and the environment. Science 361:eaam5324. doi: 10.1126/science.aam5324
Hartmann, C., and Siegrist, M. (2019). Impact of sustainability perception on consumption of organic meat and meat substitutes. Appetite 132, 196–202. doi: 10.1016/j.appet.2018.09.016
Hocquette, J.-F. (2016). Is in vitro meat the solution for the future? Meat Sci. 120, 167–176. doi: 10.1016/j.meatsci.2016.04.036
Kozicka, M., Havlík, P., Valin, H., Wollenberg, E., Deppermann, A., Leclère, D., et al. (2023). Feeding climate and biodiversity goals with novel plant-based meat and milk alternatives. Nat. Commun. 14:5316. doi: 10.1038/s41467-023-40899-2
Langemeier, M. (2021). International Benchmarks for Corn Production Vol. 11. Farmdoc Daily, Department of Agricultural and Consumer Economics, University of Illinois at Urbana-Champaign.
Langemeier, M., and Zhou, L. (2022). International Benchmarks For Soybean Production Vol. 12. Farmdoc Daily, Department of Agricultural and Consumer Economics, University of Illinois at Urbana-Champaign.
Lanzoni, D., Rebucci, R., Formici, G., Cheli, F., Ragone, G., Baldi, A., et al. (2024). Cultured meat in the European Union: legislative context and food safety issues. Curr. Res. Food Sci. 8:100722. doi: 10.1016/j.crfs.2024.100722
Lesk, C., Coffel, E., Winter, J., Ray, D., Zscheischler, J., Seneviratne, S. I., et al. (2021). Stronger temperature-moisture couplings exacerbate the impact of climate warming on global crop yields. Nat. Food 2, 683–691. doi: 10.1038/s43016-021-00341-6
Lusk, J. L., Blaustein-Rejto, D., Shah, S., and Tonsor, G. T. (2022). Impact of plant-based meat alternatives on cattle inventories and greenhouse gas emissions. Environ. Res. Lett. 17:024035. doi: 10.1088/1748-9326/ac4fda
Lynch, J., and Pierrehumbert, R. (2019). Climate impacts of cultured meat and beef cattle. Front. Sustain. Food Syst. 3:421491. doi: 10.3389/fsufs.2019.00005
Mancini, M. C., and Antonioli, F. (2022). “The future of cultured meat between sustainability expectations and socio-economic challenges,” in Future Foods: Global Trends, Opportunities, and Sustainability Challenges, ed. R. Bhat (London: Academic Press), 331–350. doi: 10.1016/B978-0-323-91001-9.00024-4
Mazac, R., Meinilä, J., Korkalo, L., Järviö, N., Jalava, M., and Tuomisto, H. L. (2022). Incorporation of novel foods in European diets can reduce global warming potential, water use and land use by over 80%. Nat. Food 3, 286–293. doi: 10.1038/s43016-022-00489-9
Merener, N. (2015). Globally distributed production and the pricing of CME commodity futures. J. Fut. Mark. 35, 1–30. doi: 10.1002/fut.21642
Morais-da Silva, R. L., Reis, G. G., Sanctorum, H., and Molento, C. F. M. (2022). The social impacts of a transition from conventional to cultivated and plant-based meats: evidence from Brazil. Food Policy 111:102337. doi: 10.1016/j.foodpol.2022.102337
Mylan, J., Andrews, J., and Maye, D. (2023). The big business of sustainable food production and consumption: exploring the transition to alternative proteins. Proc. Nat. Acad. Sci. U. S. A. 120:e2207782120. doi: 10.1073/pnas.2207782120
Nelson, M. E., Hamm, M. W., Hu, F. B., Abrams, S. A., and Griffin, T. S. (2016). Alignment of healthy dietary patterns and environmental sustainability: a systematic review. Adv. Nutr. 7, 1005–1025. doi: 10.3945/an.116.012567
Neuhofer, Z. T., and Lusk, J. L. (2022). Most plant-based meat alternative buyers also buy meat: an analysis of household demographics, habit formation, and buying behavior among meat alternative buyers. Sci. Rep. 12:13062. doi: 10.1038/s41598-022-16996-5
Newton, P., and Blaustein-Rejto, D. (2021). Social and economic opportunities and challenges of plant-based and cultured meat for rural producers in the us. Front. Sustain. Food Syst. 5:624270. doi: 10.3389/fsufs.2021.624270
Newton, P., Eichhorst, W., Hegwood, M., Morais-da Silva, R. L., Heidemann, M. S., Hoffman, A., et al. (2024). Price above all else: an analysis of expert opinion on the priority actions to scale up production and consumption of plant-based meat in brazil. Front. Sustain. Food Syst. 8:1303448. doi: 10.3389/fsufs.2024.1303448
OECD/FAO (2023). OECD-FAO Agricultural Outlook 2023–2032. Paris: OECD Publishing. doi: 10.1787/08801ab7-en
Padilla, S., Ufer, D. J., Morgan, S., and Link, N. (2023). US Export Competitiveness in Select Crop Markets. U.S. Department of Agriculture, Economic Research Service. Available at: https://www.ers.usda.gov/webdocs/publications/106158/err-313.pdf?v=2606.7
Peters, M., Shane, M. D., and Torgerson, D. (2009). What the 2008/2009 World Economic Crisis Means for Global Agricultural Trade. Economic Research Service of the US Department of Agriculture. Available at: https://www.ers.usda.gov/webdocs/outlooks/40473/9377_wrs0905_1_.pdf?v=7708.2
Pfeiffer, B., and Mulder, P. (2013). Explaining the diffusion of renewable energy technology in developing countries. Energy Econ. 40, 285–296. doi: 10.1016/j.eneco.2013.07.005
Post, M. J., Levenberg, S., Kaplan, D. L., Genovese, N., Fu, J., Bryant, C. J., et al. (2020). Scientific, sustainability and regulatory challenges of cultured meat. Nat. Food 1, 403–415. doi: 10.1038/s43016-020-0112-z
Roberts, M., and Schlenker, W. (2009). World supply and demand of food commodity calories. Am. J. Agric. Econ. 91, 1235–1242. doi: 10.1111/j.1467-8276.2009.01290.x
Roberts, M., and Schlenker, W. (2013). Identifying supply and demand elasticities of agricultural commodities: implications for the us ethanol mandate. Am. Econ. Rev. 103, 2265–2295. doi: 10.1257/aer.103.6.2265
Rubio, N. R., Xiang, N., and Kaplan, D. L. (2020). Plant-based and cell-based approaches to meat production. Nat. Commun. 11:6276. doi: 10.1038/s41467-020-20061-y
Saavoss, M., Capehart, T., McBride, W. D., and Effland, A. (2021). Trends in Production Practices and Costs of the US Corn Sector. U.S. Department of Agriculture, Economic Research Service. Available at: https://www.ers.usda.gov/webdocs/publications/101722/err-294.pdf?v=6227.4
Santo, R. E., Kim, B. F., Goldman, S. E., Dutkiewicz, J., Biehl, E. M., Bloem, M. W., et al. (2020). Considering plant-based meat substitutes and cell-based meats: a public health and food systems perspective. Front. Sustain. Food Syst. 4:134. doi: 10.3389/fsufs.2020.00134
Schnitkey, G., Paulson, N., Zulauf, C., and Swanson, K. (2021a). Direct Costs and Prices on Grain Farms, Vol. 11. Farmdoc Daily, Department of Agricultural and Consumer Economics, University of Illinois at Urbana-Champaign.
Schnitkey, G., Zulauf, C., Swanson, K., and Paulson, N. (2021b). 2022 Planting Decisions, Nitrogen Fertilizer Prices, and Corn and Soybean Prices, Vol. 11. Farmdoc Daily, Department of Agricultural and Consumer Economics, University of Illinois at Urbana-Champaign.
Slade, P. (2018). If you build it, will they eat it? Consumer preferences for plant-based and cultured meat burgers. Appetite 125, 428–437. doi: 10.1016/j.appet.2018.02.030
Stehfest, E., Bouwman, L., Van Vuuren, D., Elzen, D., Michel, G., Eickhout, B., et al. (2009). Climate benefits of changing diet. Clim. Change 95, 83–102. doi: 10.1007/s10584-008-9534-6
Szejda, K., Stumpe, M., Raal, L., and Tapscott, C. E. (2021). South African consumer adoption of plant-based and cultivated meat: a segmentation study. Front. Sustain. Food Syst. 5:744199. doi: 10.3389/fsufs.2021.744199
Taylor, H., Tonsor, G. T., Lusk, J. L., and Schroeder, T. C. (2023). Benchmarking us consumption and perceptions of beef and plant-based proteins. Appl. Econ. Perspect. Policy 45, 22–43. doi: 10.1002/aepp.13287
Tonsor, G. T., Lusk, J. L., and Schroeder, T. C. (2023). Market potential of new plant-based protein alternatives: insights from four us consumer experiments. Appl. Econ. Perspect. Policy 45, 164–181. doi: 10.1002/aepp.13253
Treich, N. (2021). Cultured meat: promises and challenges. Environ. Resour. Econ. 79, 33–61. doi: 10.1007/s10640-021-00551-3
Vaiknoras, K., and Hubbs, T. (2023). Characteristics and Trends of US Soybean Production Practices, Costs, and Returns Since 2002 (Report No. ERR-316). U.S. Department of Agriculture, Economic Research Service. doi: 10.32747/2023.8023698.ers
Van Loo, E. J., Caputo, V., and Lusk, J. L. (2020). Consumer preferences for farm-raised meat, lab-grown meat, and plant-based meat alternatives: does information or brand matter? Food Policy 95:101931. doi: 10.1016/j.foodpol.2020.101931
Wirsenius, S., Azar, C., and Berndes, G. (2010). How much land is needed for global food production under scenarios of dietary changes and livestock productivity increases in 2030? Agric. Syst. 103, 621–638. doi: 10.1016/j.agsy.2010.07.005
Wolf, C. A., Malone, T., and McFadden, B. R. (2020). Beverage milk consumption patterns in the united states: who is substituting from dairy to plant-based beverages? J. Dairy Sci. 103, 11209–11217. doi: 10.3168/jds.2020-18741
Wright, C. K., and Wimberly, M. C. (2013). Recent land use change in the western corn belt threatens grasslands and wetlands. Proc. Nat. Acad. Sci. U. S. A. 110, 4134–4139. doi: 10.1073/pnas.1215404110
Keywords: alternative meat, plant-based meat, cultured meat, agriculture, sustainability, transitions, corn, soybeans
Citation: Blaustein-Rejto D, Merener N and Smith A (2024) The potential footprint of alternative meat adoption on corn and soybean producers. Front. Sustain. Food Syst. 8:1400515. doi: 10.3389/fsufs.2024.1400515
Received: 13 March 2024; Accepted: 30 September 2024;
Published: 22 October 2024.
Edited by:
Ashish Rawson, National Institute of Food Technology, Entrepreneurship and Management, Thanjavur (NIFTEM-T), IndiaReviewed by:
Hayley Hesseln, University of Saskatchewan, CanadaRussell Hedberg, Shippensburg University, United States
Copyright © 2024 Blaustein-Rejto, Merener and Smith. This is an open-access article distributed under the terms of the Creative Commons Attribution License (CC BY). The use, distribution or reproduction in other forums is permitted, provided the original author(s) and the copyright owner(s) are credited and that the original publication in this journal is cited, in accordance with accepted academic practice. No use, distribution or reproduction is permitted which does not comply with these terms.
*Correspondence: Nicolas Merener, bm1lcmVuZXImI3gwMDA0MDt1dGR0LmVkdQ==