- 1Dipartimento di Agraria, Università degli Studi di Sassari, Sassari, Italy
- 2Department of Environment and Natural Resources, Lebanese University, Beirut, Lebanon
In the last years, olive pomace, a by-product of olive oil extraction, has shown great interest. The work aims to valorize the olive pomace of two Sardinian olive cultivars, Bosana and Semidana, by incorporating them into the formulation of functional baked products to improve their nutritional value. The freeze-dried pomace of the two varieties has been used to substitute the type 00 flour in percentages of 1, 2, and 3% (w/w). The olive pomace was characterized by macro-composition analysis, while the bread samples were characterized for their proximate, physical, technological, sensory characteristics and shelf life. The specific volume of fortified samples decreased significantly compared to the control, while antioxidant activity, and nutritional parameters were significantly improved using olive pomace. Moreover, fortified samples showed a reduction of browning and whiteness indices with respect to control. Textural profile analysis showed a firmer product, compared to the control, with higher levels of olive pomace which also positively affected the cell size distribution in the crumb. Sensorially, consumers were mostly appealed by the 1% levels of substitution of olive pomace, in particular that of the Bosana. Hardness of all samples increased significantly along 7 days of storage thus resulting in a shelf life of less than 3 days. Therefore, it can be concluded that the incorporation of olive pomace, especially at low levels (1%), into white bread significantly improves the nutritional and sensorial quality of bread without significantly affecting its technological properties.
1 Introduction
The demand for bakery products is increasing annually at a rate of 10.07% (Tripathi et al., 2018). These products fall among the most consumed food commodities being low-cost and well-accepted worldwide (Gažarová et al., 2018; Monteiro et al., 2018). Among these products, bread is the most daily consumed staple food worldwide. It was first produced by Egyptians, and it falls among the oldest industries in history present in all civilizations (Elkatry et al., 2022). The common ingredients in bread are water and flour. Bread may contain other ingredients, depending on its type, like leavening agents, salt, sugar, butter, etc. (Mondal and Datta, 2008; Toumi et al., 2022). Bread is subjected to a whole load of physical changes during the baking like water evaporation, protein denaturation, crust generation, volume expansion, starch gelatinization among others (Mondal and Datta, 2008).
In addition to being nutritionally poor, bread has several health drawbacks that the consumer started being aware of. The health drawbacks consist mainly of cardiovascular diseases, celiac disease, and obesity (Hadnađev et al., 2011; Moreira-Rosário et al., 2016; Gažarová et al., 2018). Bakery products’ consumption is also associated with an increased glycemic index increasing the risk of type 2 diabetes mellitus and biliary tract cancer (Ferrer-Mairal et al., 2012; Monteiro et al., 2018). Shewry and Hey (2015) conducted a study to compare “the healthiness” of old wheat varieties (Einkorn, Emmer, Spelt and Khorasan wheat) to that of modern wheat varieties (durum and bread wheat) and it was found out that modern wheat varieties are significantly richer in carotenoids; however, neither can be claimed healthier. Similarly, Laus et al. (2015) compared the health benefits of old and modern durum wheat varieties based on the phenolic content. No significant differences were detected between the two varieties, indicating that the health potential related to phenolic compounds of durum wheat is preserved.
To overcome these drawbacks, the trend is shifting towards the consumption of healthier, and higher-quality products. This fact is causing a challenge to the baking industry, which is experiencing the difficulty of producing bakery products with improved sensory and nutritional qualities (Mariotti et al., 2013).
Bread fortification with by-products from the agro-food industry is a good way to produce a functional food containing bioactive compounds such as fibres, antioxidants, vitamins, and minerals while at the same time it could be of great help for environmental sustainability (Di Nunzio et al., 2020; Elkatry et al., 2022).
Olive pomace (OP) is the main by-product resulting from olive oil extraction. Around 35–40 g of OP are obtained from 100 g of olives (Yanık, 2017). The olive pomace, being considered a waste, is very expensive to manage. It mainly contains an important amount of insoluble fibers especially lignin, cellulose, and hemicellulose, in addition to minerals, oils, and relatively high amounts of polyphenols. This richness in polyphenols exerts a negative impact on the environment, but a positive impact on human health, along with fibers and other components (Albahari et al., 2018). The OP may be obtained from both two-phase and three-phase decanters, the latter being usually more environmentally harmful than the two-phase one. This is due to greater amounts of wastewater in the three-phase extraction, in addition to its acidic pH, high salinity, and presence of lipids. On the other hand, these elements give the OP important antioxidant and antimicrobial properties. OP, which constitutes around 80% of the olive’s fruit, is characterized by 2.3–3.4% fat, and 54–62% moisture, in addition to a significant number of biological products (Cardoso et al., 2005; Cequier et al., 2019). To reduce waste, OP has been used as a combustion agent for renewable energy, animal feed, fertilizer by being composted, cosmetics, pharmaceuticals, and the food industry (Lammi et al., 2018; Cequier et al., 2019).
OP is a rich source of minerals and other bioactive compounds such as fibers, proteins and polyphenols, and its incorporation into other food products can contribute to increasing their mineral content and improve their nutritional value (Lin et al., 2017; Di Nunzio et al., 2020; Azadfar et al., 2023; Al-Juhaimi et al., 2024; Cardinali et al., 2024; Dahdah et al., 2024). However, the incorporation of OP into bread may decrease consumer acceptance because it alters the sensory attributes and technological characteristics of bread, which requires major carefulness in selecting the level of substitution. Unfortunately, this may limit the quantity of by-products used (Cedola et al., 2020). Selim et al. (2020) stated that approximately 89% of phenolic compounds from olive fruit are preserved in the olive pomace, bringing up similarity in the functional properties of olive oil and olive pomace. The phenolic profile of olive pomace varies due to several factors such as fruit ripeness, climate, cultivar., origin, and extraction method. The authors reported in their article that some studies identified oleuropein and its derivatives as the primary compounds, while others highlighted hydroxytyrosol (Selim et al., 2020). Olive pomace contains dietary fiber, protein, lipids, pigments, and polyphenolic compounds. Incorporating fiber into food formulations improves the food product characteristics (Selim et al., 2020; Azadfar et al., 2023).
Although there has been extensive research dealing with the fortification of wheat bread with bioactive compounds, the potential of incorporating OP as a practical and nutrient-dense fortifying agent in toast bread is currently less studied. Recently, Dahdah et al. (2024) carried out the characterization of olive pomace obtained by two typical varieties of Sardinia island (Italy) and studied the rheological characteristics of dough obtained by partial substitution of wheat flour with freeze-dried olive pomace at different levels of fortification. The authors observed beneficial effects of partial substitution, such as a decrease in development time, improved dough stability and storage, and a superior loss modulus and gas retention capacity compared to the control. At the same time negative effects, such as a decrease in dough development height, gas production, gas retention, pasting profile, stickiness, elasticity, and an increase in dough resistance, were registered. Also, Azadfar et al. (2023) explored the incorporation of an Iranian OP dietary fiber into Barbari flat bread at levels ranging between 5 and 15%. With increasing OP dietary fiber, both the water absorption of the dough and its hardness increased, while the bread’s specific volume decreased with increasing fortification levels.
The aim of this study was to improve bread quality attributes, especially the content of dietary fiber, total polyphenols, and antioxidant activity using the olive pomace, a by-product of the olive industry. To this aim, technological, nutritional, and sensory properties of bread fortified with OP of two typical Sardinian cultivars Bosana and Semidana at three levels of substitution (1, 2, and 3%), each, were assessed.
2 Materials and methods
2.1 Bread ingredients
OP samples of two typical Sardinian cultivars Bosana and Semidana were provided from Accademia Olearia (Alghero, Italy) from the 2021–2022 crop season. Olives were milled through a two-phase centrifugal extractor Leopard DMF (Pieralisi) which produced an OP made up of wet pulp without stones, also known as Patè. OP samples were immediately stored at −20°C until the analysis. Subsequently, OP samples were freeze-dried, ground to a particle size <500 μm using a domestic grinder (Moulinex A320R1, 700 W, Paris, France), and stored under vacuum conditions in the dark until analysis. The characterization of raw OP (ROP) and freeze-dried OP (FD-OP) was previously described in Dahdah et al. (2024).
A commercial common type 00 wheat flour (Triticum aestivum) was provided by SIMEC Spa (Santa Giusta, Italy). It had the following proximate composition: 14% moisture, 11.6% protein, 3.8% fiber, 1.5% lipids, and 0.1% ash. Fresh compressed yeast (Conad S.C., Bologna, Italy) and chloride sodium were purchased from a local supermarket.
2.2 Bread formulation and preparation
The control dough (CTRL) was made of type 00 wheat flour, 2% (w/w) of yeast, 1.8% (w/w) of salt, and 54% (w/w) of water at a temperature of 30°C. The mixture was kneaded for 8 min in a mixer (Kitchen Aid Professional, Model 5KSM7990, St. Joseph, MI, United States) equipped with a stainless-steel hook at speed 3 at room temperature. The OP doughs were made with the same base formulation of the CTRL dough with the exception of water content, which varied according to the percentage of pomace olive addition and the type of cultivar., as previously reported (Dahdah et al., 2024): Bosana 1% (water: 54.0%), Bosana 2% (53.5%), Bosana 3% (53.0%), Semidana 1% (54.5%), Semidana 2% (54.2%), and Semidana 3% (53.5%). Bread sample codes were named according to cultivar and percentage of olive pomace supplementation, as follows: Bos 1%; Bos 2%, Bos 3%, Sem 1%, Sem 2%, and Sem 3%.
First, the flour and OP were mixed at room temperature with a mixer equipped with a stainless-steel dough hook (Kitchen Aid Professional, model 5KSM7990, St. Joseph, MI, United States) set to speed 3. Yeast and salt were added, respectively, after being dissolved in the required amount of water at a temperature of 30°C.
The first mass leavening took place in a climate chamber (Tecnomac Lev2+, Castel MAC S.r.l., Castelfranco Veneto, Italy) at 30°C and 85% relative humidity (RH) for 30 min. Fermented doughs were divided into 250 g pieces, hand molded and sheeted, and put into rectangular bread molds (length: 11.5 cm, width: 7.5 cm, height: 6.5 cm) in the same conditions to reach up the double the volume. The bread was baked at 210°C for 40 min in a preheated static electric oven (E4318PA, Europa S.r.l., Molina di Malo, Italy). Samples were left to cool for 2 h before analysis. For the shelf-life assessment, each sample was put in a polyethylene plastic bag and stored at 20°C for 7 days to evaluate the changes of the qualitative parameters of the samples.
The pH of all dough samples was also controlled before and after the first bulk leavening by pH meter (Knick 911, Knick, Berlin, Germany), equipped with a InLab® Solids electrode (Mettler Toledo, Ohio, United States).
A total of two baking trials for each sample were performed. Each trial gave rise to 3 bread loaves per replicate.
2.3 Bread measurements
2.3.1 Specific volume
The bread’s specific volume was estimated by dividing the bread volume (mL) obtained following the rapeseed displacement method over bread weight (g) following the AACC International Method 10-05.01 (AACC. American Association of Cereal Chemist, 2001).
2.3.2 Moisture content
The moisture content of the bread loaves was determined by weight difference through a thermo-balance set at 105°C (DAB 100-3, Kern & Sohn GmbH, Balingen, Germany) after having dried the loaves for 48 h and ground by a domestic grinder (Moulinex A320R1, 700 W, Paris, France) according to the Official Standard Method (AACC. American Association of Cereal Chemist, 2005).
2.3.3 Water activity content
Water activity (aw) was measured in ground bread samples by an electronic hygrometer (Aw-Win, Rotronic, equipped with a Karl-Fast probe, Bassersdorf, Switzerland) calibrated with known solutions of LiCl in the range of 0.1–0.95 (ISO International Organization for Standardization, 2017).
2.3.4 Lipid content
Lipid content in bread was determined using a solvent extractor (SER 158, Velp Scientific, Deer Park, NY, United States), with petroleum ether (Carlo Erba Reagents, Milano, Italy) used as a solvent (AOAC Association of Official Analytical Chemists, 2006a). Fat content was then quantified gravimetrically.
2.3.5 Ash content
A portion of 5 g of ground bread was weighed into a capsule that was heated, cooled, and weighed at room temperature. The samples were incinerated in a muffle furnace at 550°C. After cooling the capsules in a desiccator, they were weighed at room temperature (AOAC Association of Official Analytical Chemists, 2006b).
2.3.6 Fibers content
Total dietary fibers (TDF) were determined using the K-TDFR analytical kit from Megazyme Ltd., (Bray, Ireland), through enzymatic digestion, followed by a filtration using a filtration unit (CSF6, Velp Scientific, Deer Park, NY, United States) as described in the AACC 32-05.01 (AACC. American Association of Cereal Chemist, 2011) and AOAC 985.29 (AOAC Association of Official Analytical Chemists, 2007) methods.
2.3.7 Protein content (C, H, N analysis)
After having dried and ground all bread samples using a domestic grinder (Moulinex A320R1, 700 W, Paris, France), 100 mg of the powdered samples were accurately weighed using an analytical balance (BP210S, Sartorius, Goettingen, Germany), and then placed into a small tin capsule and sealed tightly.
The tin capsule was placed into the combustion chamber of the C, H, and N elemental analyzer as previously described in Dahdah et al. (2024) using a CHN628 carbon/nitrogen/hydrogen elemental analyzer (Leco Corporation, St. Joseph, MI, United States) following the AOAC official method 990.03 (AOAC Association of Official Analytical Chemists, 2006c). The protein content was calculated through nitrogen content with a conversion factor of 6.25.
2.3.8 Total carbohydrates and energy
Total carbohydrates content and energy were calculated according to the following formulae (Zarzycki et al., 2022):
Carbohydrates content in bread samples was expressed as a percentage based on dry matter (DM) basis.
Energy’s results were expressed as kcal per 100 g of bread on wet basis.
2.3.9 Mineral composition of bread
Macro elemental (Na, Mg, K, and Ca) analysis on bread samples was conducted by using AAS (Atomic Absorption Spectrometry) analyst 200 spectrometer, produced by Perkin Elmer (Milan, Italy), as previously described in Dahdah et al. (2024).
2.3.10 Antioxidant activity of bread samples
The organic extracts were obtained to assess the antioxidant activity as previously described by Collar et al. (2014). The ground bread samples (3 g) were added 10 mL of 50% methanol (Carlo Erba Reagents, Milano, Italy) (MeOH/H2O, 50:50, v/v, pH = 2), and left in magnetic stirring for one hour at room temperature. Afterwards, they were subjected to centrifugation at 2500 g for 10 min (Neya 16 R, Remi Elektrotechnki LTD, Vasai, India), and the resulting supernatant was collected. The same extraction procedure is repeated one more time with the solvent being 70% acetone (Carlo Erba Reagents, Milano, Italy) (acetone/water, 70:30, v/v). The supernatant was recovered and added to that of the first extraction.
The antioxidant activity of bread samples through ABTS assay was determined according to Cannas et al. (2023). Briefly, the stock solution was prepared by mixing a 7.4 mM ABTS solution (Sigma-Aldrich, Milan, Italy), and a 2.6 mM potassium persulfate solution (1:1, v/v) (Sigma-Aldrich, Milan, Italy). Both solutions were prepared with 0.1 M phosphate buffer saline (PBS, pH = 7.4) (Sigma-Aldrich, Milan, Italy). The stock solution was left in the dark, for 12 h at room temperature. The working solution (WS) was then prepared by diluting 1 mL of ABTS stock solution with 20 mL PBS, to reach an absorbance of 0.70 ± 0.02 at 734 nm. The absorbance of samples was read in a spectrophotometer (Agilent, model Cary 3,500, Cernusco, Milan, Italy) at 734 nm, using 2 mL of ABTS working solution and 40.8 μL of sample extract under constant stirring over a 6 min period. The analysis was done in duplicate and a Trolox standard curve was used (Sigma-Aldrich, Milan, Italy) Results of antioxidant activity were expressed as μmol Trolox equivalent (TE)/g of DM.
2.3.11 Color determinations
The crust and crumb color attributes were measured on the day of baking by using a colorimeter (Minolta CR-300, Konica Minolta Sensing, Osaka, Japan) equipped with a measuring head CR-300, using a D65 illuminant and a CIE 10° standard observer angle, calibrated against a white tile supplied with the instrument. The color of the crust was taken from the extremities and the middle parts of three loaves, while the color of the crumb was measured at three points of three central slices of three loaves. Parameters determined were L (L = 0 [black] and L = 100 [white]), a* (−a = greenness and +a = redness); b* (b (−b = blueness and +b = yellowness)), browning index BI (crust), and whiteness index WI (crumb) as previously described by Borsuk et al. (2012) and Conte et al. (2018).
2.3.12 Texture properties
Evaluation of the bread firmness was conducted using a TA-XT2 plus Texture Analyzer (Stable Micro Systems Ltd., Godalming, United Kingdom) fitted with a 30 kg load cell. Bread was divided into 2 cm slices obtained with manual slicer equipment (Sibread, Mod. S4/S5, Caltrano (VI), Veneto, Italy). A TPA test was carried out on three slices of three bread loaves per replicate by using a cylindrical probe (36 mm of diameter) that compressed the samples at a constant speed of 1 mm/s and with a 30 second gap between compressions. The force necessary to compress samples by 50% of their original width was determined from the force–time curve. From the resulting force–time curves, the studied parameters were the following: hardness, cohesiveness, springiness, resilience, chewiness, and adhesiveness. Texture Exponent Software TEE32, version 6.1.10.0 (Stable Micro System, Surrey, United Kingdom), was used for data processing.
2.3.13 Bread image analysis
On the day of baking, bread loaves were sliced into 2 mm thick slices using an electric slicer (Sibread, Mod. S4/S5, Caltrano (VI), Veneto, Italy). These slices were then scanned in color on one side using an EPSON Perfection V500 Photo (Epson, Suwa, Japan) flatbed scanner with a resolution of 300 DPI. The scanner settings were kept as default following the settings of EPSON scan software. The resulting images were saved in JPEG format. Then, using Image J 1.52a software (NIH, United States), a square of 30 cm × 30 cm was cropped from the center of each slice and converted to an 8 bit greyscale image. Each replicate consisted of 3 loaves, thus 3 slices which were evaluated for their porosity in size and distribution. Crumb grain was described by the following parameters: number of cells, cell-to-total area ratio, wall-to-total area ratio, and cells/cm2 (Collar et al., 2014). Cell area distribution and cell number distribution were acquired by four pre-selected dimensional categories: class 1 (<0.1 mm2), class 2 (0.1–0.99 mm2), class 3 (1–9.9 mm2), class 4 (10–210 mm2).
2.3.14 Sensory evaluation
Sensory analysis was performed to investigate the effect of incorporating OP from the two cultivars at different percentages on the sensory characteristics of bread. On day 0, a preference ranking test was conducted by a consumer panel. The participants were randomly selected from the students and staff of the University of Sassari, comprising both genders and aged between 18 and 65 years old. They were regular consumers of bread and did not have celiac disease or any food allergies to olives and wheat. The sensory analysis was performed in three separate sessions and data were collected by using the Smart Sensory Box 2.11.4 software (Smart Sensory Solutions S.r.l., Sassari. Italy). The first two sessions each involved 80 panelists who participated in a ranking preference test. This test enables judges to rank bread samples from the most preferred to the least preferred, allowing them to compare bread fortified at three different levels (1, 2, and 3%) for both the Bosana cultivar (session 1) and Semidana (session 2) according to the attribute preference. Successively, a paired comparison test with 150 panelists was performed to determine the difference between the two bread samples that were most preferred in the previous sensory evaluation sessions, based solely on the attribute preference. All assessors were students and staff of the University of Sassari of both genders, aged between 18 and 65 years old.
The 2 mm thick bread slices for each level of fortification were diced into four pieces and placed into sealed plastic bags. The samples were labelled with randomized 3-digit codes and provided at room temperature in a randomized and balanced order. Water drinking was required before and between samples to ensure effective palate cleansing. The same procedure was followed for each cultivar and the paired comparison test.
2.3.15 Shelf-life
The shelf-life of bread was studied by detecting the crumb firmness with a TPA analysis as reported in Section 2.3.12 as well as measuring moisture content, and water activity on days 0, 1, 2, 3, 4, 5, and 7 (T0, T1, T2, T3, T4, T5, T6, T7) at room temperature (RT).
2.4 Statistical analysis
The statistical analysis of data was done using Minitab® 17.1.0 software (Minitab, Inc.). For the pH of dough samples analysis before and after fermentation within the same formulation, a t-test was carried out to compare the significance of the differences. A one-way ANOVA was conducted to compare the pH levels among different dough formulations both before and after fermentation, as well as to compare the data of the resulting bread samples. To determine the variation between the means of each pair, Tukey’s Honestly Significant Difference test (HSD) was employed. Significance was defined with a p-value <0.05 (confidence level of 95%).
Sensory analysis results obtained from the ranking preference test were analyzed using Friedman’s test at a 5% significance level. If there was a significant difference between at least two samples, the LSD Fisher’s test (p-value <0.05) was used to determine significant differences among all samples. Data obtained from the paired-comparison test were analyzed using the statistical table for the directional difference test (two-tailed). The chosen significance level of the test was 5%.
3 Results and discussion
3.1 Bread measurements
3.1.1 pH of the bread dough samples before and after fermentation
The pH measurements of dough samples before and after fermentation are reported in Table 1.
Significant differences between samples were recorded at higher levels of substitution. As before the fermentation the lowest pH levels belonged to Bos 2%, Bos 3%, and Sem 3%. Whereas after fermentation, the lowest pH levels were recorded for Bos 3% and Sem 3%. This is due to the high acidity of the matrix used which is OP (Dahdah et al., 2024). According to Foti et al. (2022), OP is an acidic matrix having pH values ranging between 4.95 and 5.20. Therefore, its incorporation into a food matrix makes it acidic, which explains the decreasing pH of the bread doughs at higher OP substitution levels both before and after fermentation. The relatively low changes in pH are due to the low concentration of OP used to fortify the bread doughs.
All dough samples showed significant decreases in pH levels after fermentation. The drop in pH levels is due to the abundant production of organic acids by the yeast in the dough during fermentation (Jayaram et al., 2013). The same authors reported that the most prominent organic acid produced is succinic acid. The pH decrease can be down to 1 unit during fermentation (Jayaram et al., 2013).
3.1.2 Bread-specific volume and moisture content
Table 2 summarizes the moisture content and specific volume characteristics of the bread samples.
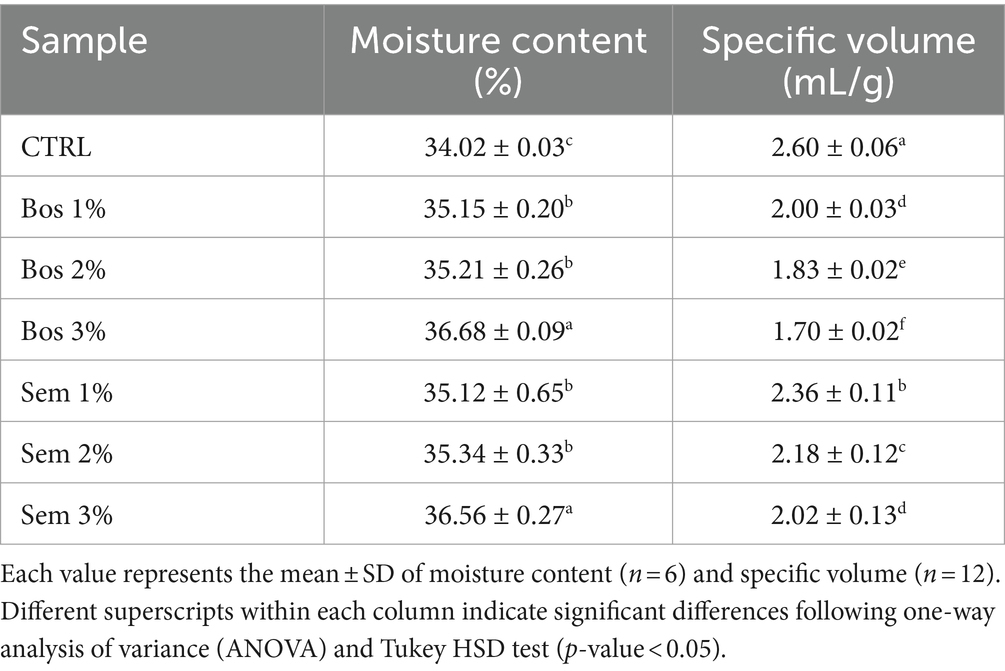
Table 2. Moisture content and specific volumes of the control bread and bread fortified with different percentages (1, 2, 3%) of olive pomace of cultivars Bosana and Semidana.
The incorporation of OP into bread samples significantly decreased the specific volume, as reported in Table 2, as increasing levels of substitution with OP significantly decreased the specific volume within each cultivar, as well. However, Semidana-fortified bread loaves had a significantly higher specific volume as compared to the Bosana-fortified bread loaves at each level of substitution. The decrease in specific volume observed after the incorporation of OP can be attributed to the reduction in dough extensibility, as previously reported by Dahdah et al. (2024). Additionally, the weakening of the starch-gluten matrix, and the gluten network in particular, occurs as a result of the dilution of the gluten content with a non-gluten matrix containing fibers. These fibers compete for water absorption, leading to a decrease in maximum dough height (Hm), and consequently, the specific volume (Amoriello et al., 2020).
Bread belongs to the family of Intermediate Moisture Food (IMF). The moisture content of bread samples (Table 2) ranged between 34.02 and 36.68%, with the CTRL having significantly the lowest moisture content. Higher moisture content in fortified breads is due to the high-water content of the olive pomace, in addition to the presence of proteins and fibers which are known for their water-binding capacity (Adeleke and Odedeji, 2010; De Gennaro et al., 2022). These results were in major accordance with those reported by Das et al. (2012), who incorporated coriander leaf powder into bread, where moisture content was slightly higher in the fortified bread loaves. The increasing trend along with the increase of substitution levels was similar to the obtained trend in this study, but the moisture content reported was higher, ranging between 38 and 40% with respect to other studies that reported moisture contents of bread fortified with soy between of 34.1 and 35.6% (Olaoye et al., 2006), or with green tea reaching 29.6% (Abd El-Megeid et al., 2009). Moreover, bread fortified with moringa seeds ranged between 20.01 and 22.90% of moisture content (Bolarinwa et al., 2019).
3.1.3 Physicochemical analyses of bread samples
The proximate composition as fat content, ash content TDF content, and protein content of the CTRL bread samples, and those fortified with OP at all percentages is summarized in Table 3.
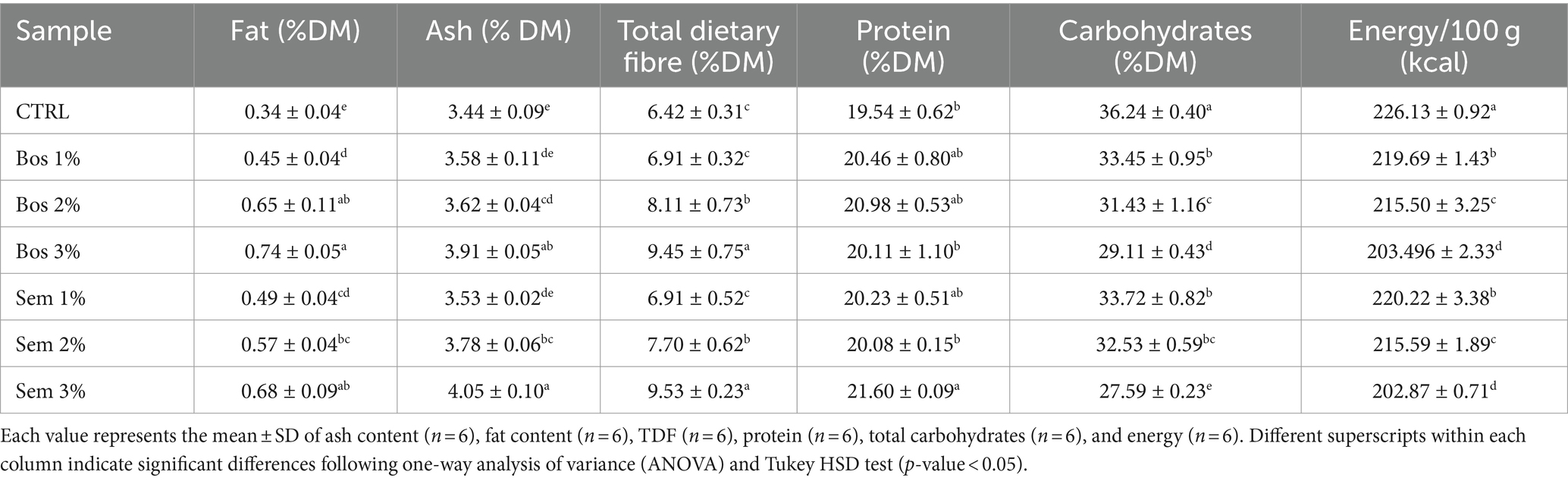
Table 3. Proximate analysis of the control bread and bread fortified with different percentages (1, 2, 3%) of olive pomace of cultivars Bosana and Semidana.
A significant increase was observed in the fat content of the OP-fortified bread samples at any percentage of fortification. The higher fat content in the bread is due to its fortification with an oily matrix. These results align with those reported by Al-Juhaimi et al. (2024), who fortified bread samples with varying levels of green olive pulp (5, 10, and 20%). The oil content of the samples ranged from 0.20% for the control to 0.83% for the bread fortified with 5%.
The ash content of the bread loaves increased significantly only with the incorporation of 2 and 3% OP for both cultivars. OP is a rich source of minerals, as evidenced by its high ash content (Bosana-OP: 4.29%, Semidana-OP: 5.29%) (Dahdah et al., 2024). The high ash content of OP resulted in higher ash content of fortified breads (Abiodun et al., 2012).
A product containing 3% or more of dietary fiber is claimed to be “rich in fibers” (Caponio et al., 2022). The total dietary fiber content of the bread samples was significantly higher in breads with 2 and 3% substitution levels of both cultivars as compared to CTRL, since the OP is rich in fibers (Bosana-OP: 61%, Semidana-OP: 66%). Bolarinwa et al. (2019) and Olaoye et al. (2006) reported fiber content of 0.03–0.62% for bread produced from fortified wheat flour with moringa seed powder and composite flour of wheat, plantain, and soybeans. Rainero et al. (2022) stated that a food product is considered high in fiber when it contains at least 6%, which corresponds to the TDF content of bread samples in this study. Such a high amount of fiber is an important help to reach the daily needs of fiber intake of 30 g per day for adults. Fiber intake is crucial for many vital body functions, especially in the digestive system such as rapid gastric emptying, decreased intestinal transit time, and increased fecal mass (Rainero et al., 2022).
Ingestion of proteins is crucial for the human body, as they serve as antibodies, and they are the primary source of amino acids needed for appropriate cellular functioning (Adeleke and Odedeji, 2010). The protein content varied significantly among with the highest being Sem 3% of 21.60% DM. These slight changes in the protein content agree with the results obtained by Caponio et al. (2022). The minor differences in the protein content between the control and most of the fortified bread are attributed to the fact that the protein from the OP replaced the protein of the wheat flour resulting in small changes (Rahman et al., 2021; Rainero et al., 2022). The protein content of the OP-fortified bread is in accordance with the values obtained by Gomes Natal et al. (2013), which ranged from 14 to 21% in potato bread fortified with varying levels of whole soy flour. Our bread samples contained a higher protein amount compared to Adeboye et al. (2018), which studied the effect of reduced-fat peanut flour on toast bread, and higher than bread produced with the fortification of lupin flour (8.7–12.2%) as reported by Plustea et al. (2022). The protein content usually depends on the dough formulation and the substitution level of the product fortification (Bolarinwa et al., 2019).
Carbohydrate content ranged from 27.59% for Sem 3% bread to 36.24% for CTRL bread. The latter had the highest value, and it was significantly different from all the other samples. A decreasing trend was, in general, observed along with increasing OP incorporation levels. This is due to the high content of complex carbohydrates such as fibers of the OP (61–66%) and thus a low content of simple sugars. These results are in accordance with those reported by Pauline et al. (2020), who reported a decrease in carbohydrate content as the level of wheat flour substitution with cereal bran increased. This trend was also similar to that observed by Mehder (2014), who found that substituting wheat flour with increasing percentages of pomegranate peels reduced total carbohydrate content from 81.43% DM to 77.27% DM. A lower carbohydrate content of breads fortified with OP is a promising nutritional improvement for preventing chronic non-communicable diseases, particularly type II diabetes (Pauline et al., 2020).
The CTRL bread had the significantly highest energy value of 226.13 kcal/100 g DM compared to all the other fortified samples. These results are comparable to those found by Pauline et al. (2020), who observed lower energy values for fortified breads with cereal brans compared to wheat bread.
3.1.4 Mineral composition of bread samples
Minerals play a crucial role in maintaining appropriate body functioning. Macro-minerals are needed in relatively large quantities, as compared to the other trace element-minerals.
No significant differences were observed for the levels of calcium, magnesium, and sodium among all bread samples (Table 4). On the other hand, potassium content varied significantly among all bread samples. These small changes are attributed to the low levels of substitution and the replacement of potassium in the wheat flour with that from OP.
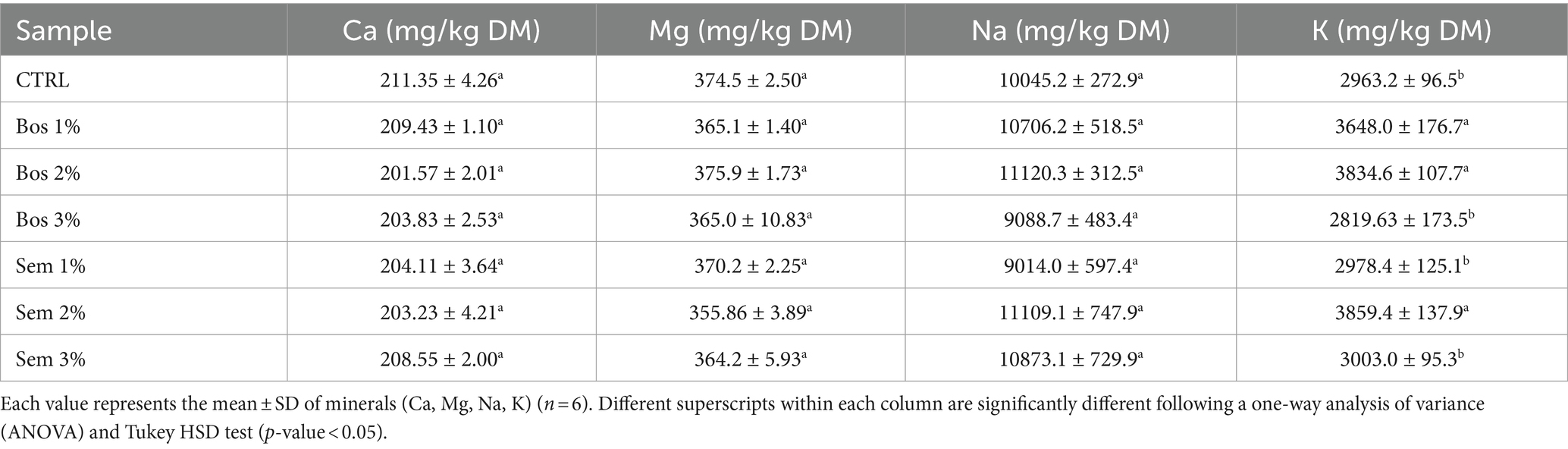
Table 4. Mineral composition of the control bread and bread fortified with different percentages (1, 2, 3%) of olive pomace of cultivars Bosana and Semidana.
Calcium did not significantly change its content among all bread samples. The amount of calcium in our bread was about 3-times higher than bread fortified with lupin flour (Plustea et al., 2022), and lower than bread formulated with the inclusion of moringa powder (Bolarinwa et al., 2019).
Magnesium did not show any significant differences in this study. However, the values were in accordance with the study conducted by Plustea et al. (2022), where bread was fortified with important percentages of 10, 20, and 30% of lupin flour. Sodium is the most abundant mineral detected. Mohajan et al. (2019) reported a significantly lower sodium content than the one obtained throughout this study for bread fortified with up to 40% buckwheat flour. The reported sodium content is much lower. These differences are probably due to the lower quantity of salt used in the composition of the dough which is 1% (Mohajan et al., 2019) compared to 1.8% in OP-fortified bread. Moreover, the sodium content in buckwheat is close to the sodium content of wheat flour (wheat flour: 147.8 mg/kg DM, buckwheat flour: 205.9 mg/kg DM), whereas olive pomace exhibited high sodium content of 458.6 and 432.4 mg/kg DM, for Bosana and Semidana (Dahdah et al., 2024). This can be behind the higher content of sodium in OP-fortified bread compared to buckwheat-fortified bread.
Potassium content results are in line with those obtained by Bolarinwa et al. (2019) who fortified bread with moringa seed powder. They reported potassium contents ranging between 2,240 and 3,270 mg/kg. On the other hand, Plustea et al. (2022) reported a much lower potassium content varying between 162.33 and 167.67 mg/kg in breads fortified with different levels of lupin flour. Mohajan et al. (2019), also obtained a lower potassium content ranging from 711.6 and 993.1 mg/kg upon the incorporation of buckwheat flour into bread.
3.1.5 Antioxidant activity
OP is a rich source of bioactive compounds, such as polyphenols and many others (Cardoso et al., 2005; Cequier et al., 2019). Its incorporation into bread provides beneficial health effects to the human body (Conte et al., 2021; Difonzo et al., 2021; Ribeiro et al., 2021). In addition, olive by-products, such as leaves and mill wastewater with its prominent antioxidant effect could be a good health-promoting alternative to wheat flour in the production of bakery products (Conte et al., 2021).
Figure 1 summarizes the antioxidant activity of bread samples in μmol TE/g DM as determined by the ABTS assay.
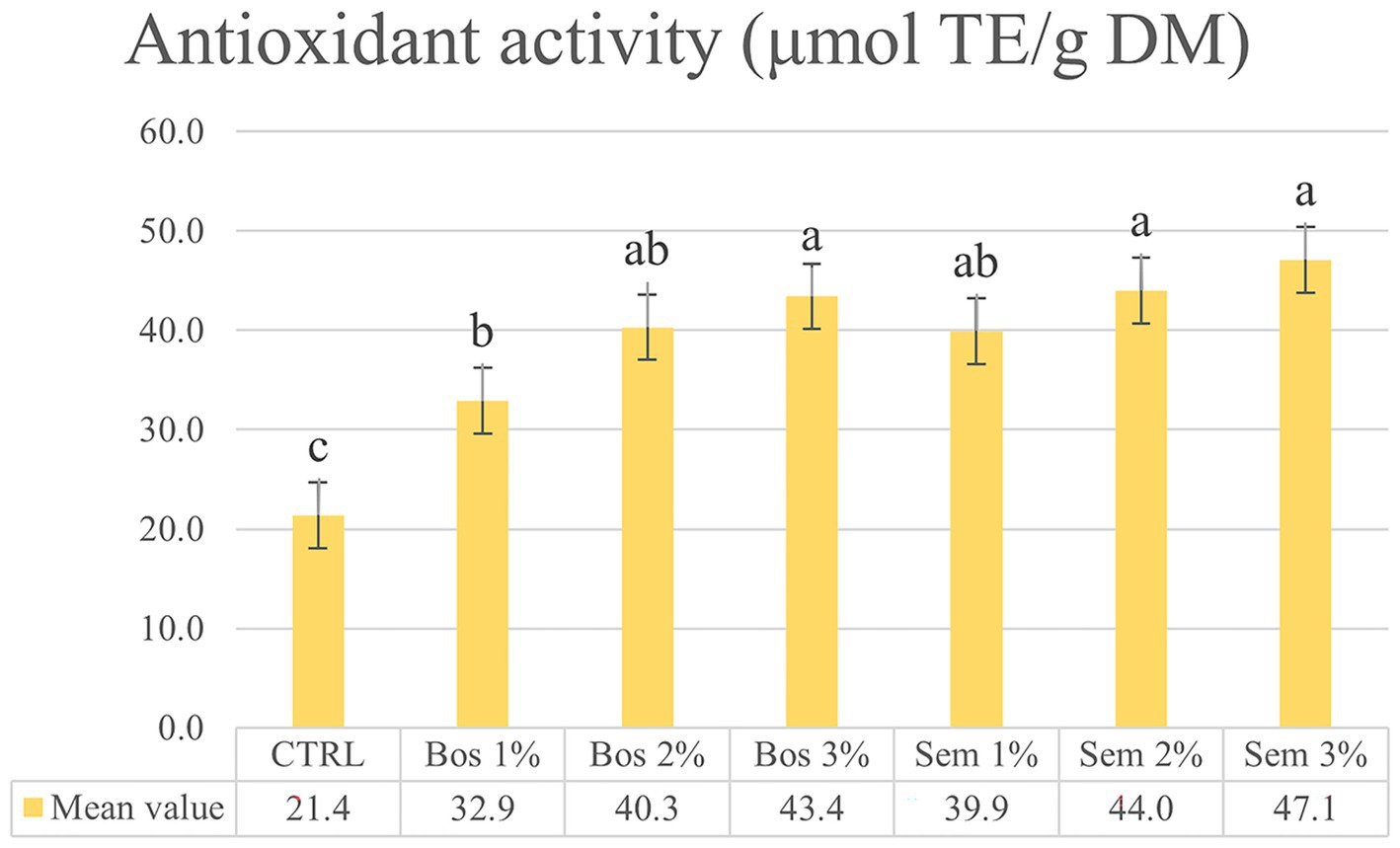
Figure 1. Antioxidant activity (μmol TE/g DM) of the control bread and bread fortified with different percentages (1, 2, 3%) of the OP of cultivars Bosana and Semidana using ABTS assay. Each bar represents mean values ± SD of antioxidant activity with ABTS assay (μmol TE/g DM) (n = 6). Different superscripts within bars indicate significant differences according to a one-way ANOVA, followed by Tukey HSD test (p-value <0.05).
The antioxidant activity through ABTS assays ranges from 21.4 to 47.1 μmol TE/g DM, with the lowest being attributed to the CTRL, which was significantly different from all other samples. Within each cultivar., an increase in OP substitution levels leads to a significant increase in antioxidant activity only between Bos 1% and Bos 3%.
Cedola et al. (2019, 2020) also reported an increase in the antioxidant activity with bread fortified with 10% olive pomace. However, our results were almost 23% higher than the ones reported by these authors because the OP of Bosana and Semidana were richer in polyphenols and had higher antioxidant activity than the cultivar Cellina di Nardò reported by Cedola and colleagues. It is well established that OP contains an important quantity of phenolic compounds, such as phenolic acids and flavonoids characterized by a very high ability to neutralize free radicals, and therefore could exhibit a strong antioxidant potential (Cedola et al., 2019). Also, two recently published studies conducted by Al-Juhaimi et al. (2024) and Cardinali et al. (2024) noted an increase in antioxidant activity with the augmentation of OP incorporation with a notable difference between the control and the levels of incorporation in OP-bread (5, 10, 15, 20%).
It is worth noting that Maillard browning synthesizes substances with antioxidant activity especially melanoidins which are formed at the end of the reaction, and has an antioxidant activity, as well. The antioxidant activity of bread samples is not only due to polyphenols incorporation. Polyphenol concentration decreases when subjected to a heat treatment. However, the antioxidant activity may still increase even in unfortified breads upon exposure to mechanical and thermal treatments during kneading and baking. This is due to the breakdown of several components particularly fibers, and consequently enhancing the susceptibility of bread to enzymatic digestion, thus allowing the release of fiber-associated polyphenols (Vogrinčič et al., 2010; Collar et al., 2014).
3.1.6 Crust and crumb color attributes
The effect of the incorporation of olive pomace into bread on the color parameters of the crust and crumb of bread loaves is presented in Table 5.
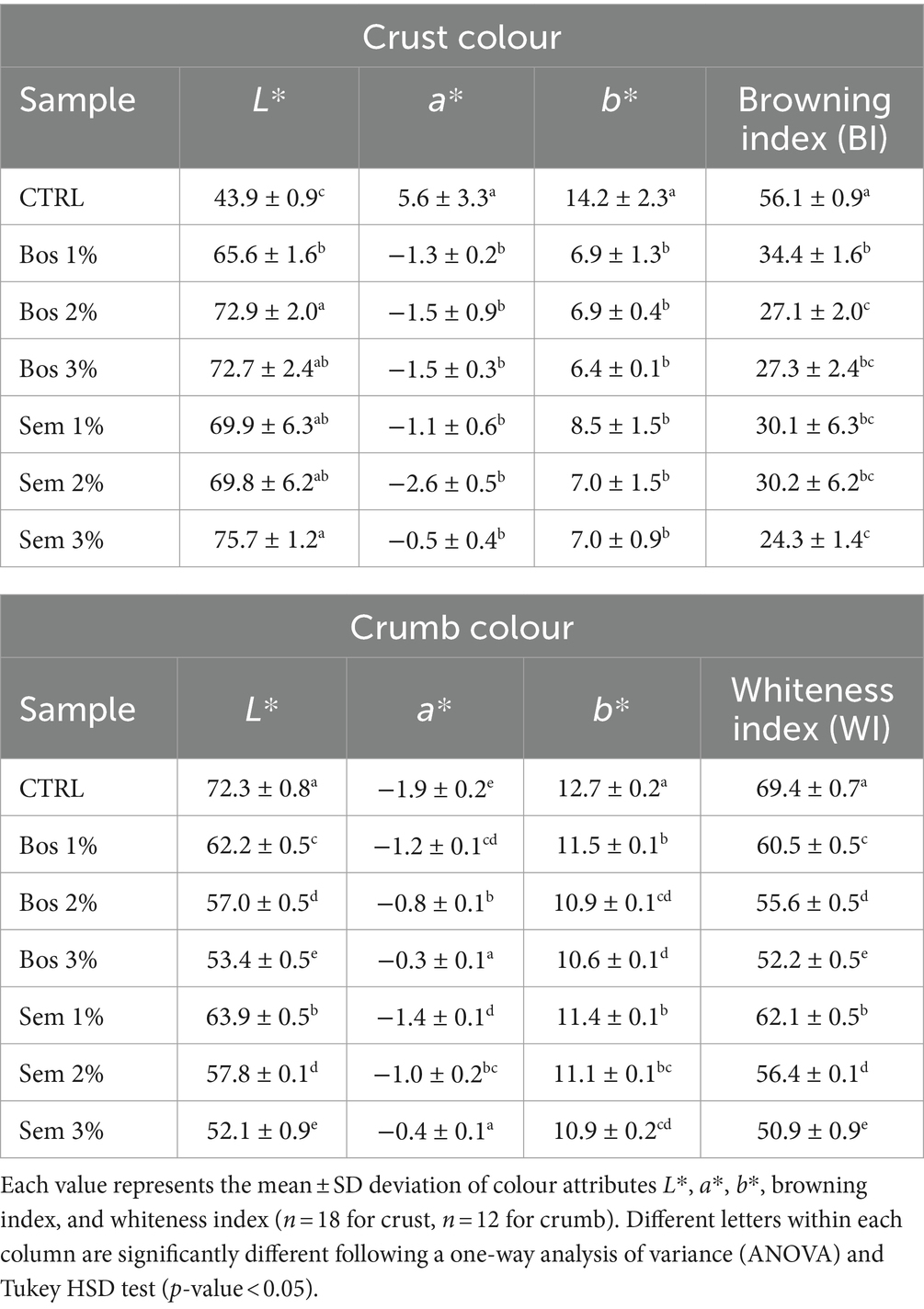
Table 5. Colour parameters of the CTRL and bread samples fortified with OP of cultivars Bosana and Semidana at 1, 2, and 3%.
The color of the crust is one of the major contributor to bread acceptability. Several factors influence the color of the crust including types and quantities of used ingredients in the formulation, baking temperature, and time (Bchir et al., 2014; De Gennaro et al., 2022). The incorporation of OP significantly affected the color attributes of the bread. The crust of all fortified breads was significantly lighter than the control. The whiter color of the crust for fortified samples was also proven by the BI with the CTRL having the significantly highest BI, followed by Bos 1%. These OP-fortified breads’ crusts exhibited a significant reduction in both a* and b* parameters increasing the intensities of the green and blue hues, respectively, compared to CTRL. The crust color is not only affected by the color of the fortifying compound, but rather by the Maillard and caramelization which are non-enzymatic reactions, and they are affected by pH, temperature, and water activity (Das et al., 2013; Bchir et al., 2014; Koletta et al., 2014; Oracz and Nebesny, 2019). These reactions take place upon the exposure of the products to high temperatures, and they contribute to the sensory attributes (i.e., color, taste, aroma, and texture of the end product) (De Gennaro et al., 2022). Maillard reactions occur between amine groups, mainly from proteins, and carbonyl compounds of reducing sugars such as fructose, glucose, maltose, or lactose. The condensation of sugars in the final stage of the reaction leads to the formation of melanoidins responsible for the brown color formation (De Gennaro et al., 2022). The crust of the fortified bread exhibited a paler color, with respect to the CTRL sample, due probably to the presence of polyphenols interfering with the Maillard reaction. Polyphenols do not only trap reactive oxygen, but also carbonyl compounds, Strecker aldehydes, and acrylamides. In addition, they interact with amino groups of amino acids and proteins to form adducts. Polyphenols also capture Amadori rearrangement products (ARPs) which constitute an important part of the Maillard’s reaction and act as browning precursors. All this inhibits the formation of several Maillard products responsible for browning including melanoidin (Cui et al., 2021; Han et al., 2022).
On the other hand, for the crumb color, the CTRL had the highest lightness parameter. Samples showing a decrease in L* values also showed a consequent darkening of the crumb. The a* parameter ranged from −1.9 to −0.4 increasing from the control to the substitution levels of the same cultivar. The b* parameter showed the higher value 12.7 ± 0.2 in CTRL. However, a significant decrease between the CTRL and all the other samples was detected, and within fortified samples. This means that the control has a yellower crumb color, whereas the others tend to decrease the yellow hue and increase the blue one. Consequently, a darker shade of green-blue can be attributed to the fortified bread with increasing OP substitution levels (Figure 2). This is also supported by the values of the whiteness index, as a darker-colored matrix results in a lower WI (Conte et al., 2018). Among the samples studied, the CTRL had the highest WI, while both 3% Bosana and Semidana OP fortified bread had the lowest. Similar results concerning the color attributes of the bread were reported by other authors (Bchir et al., 2014; Koletta et al., 2014; Yu and Smith, 2015; Conte et al., 2018; Pauline et al., 2020).

Figure 2. Images of a cross-sectional cut of control bread and bread fortified with different percentages (1, 2, 3%) of the OP of cultivars Bosana and Semidana.
3.1.7 Texture profile analysis
Table 6 shows the texture profile analysis (TPA) of the bread samples. The incorporation of OP of both cultivars significantly increased the hardness as compared to the control.
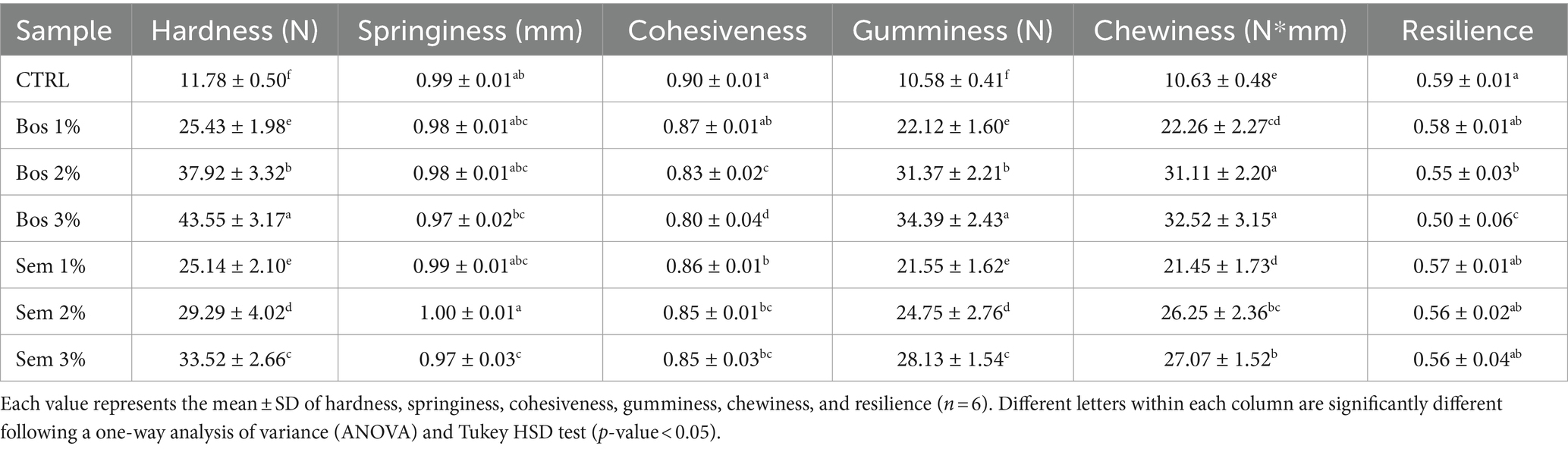
Table 6. TPA of bread samples with progressive incorporation of olive pomace of cultivars Bosana and Semidana.
Chewiness, defined as the energy required for food mastication, and in terms of texture analyzer is “the product of hardness, cohesiveness, and springiness” (Fik et al., 2012; Atta et al., 2023), exhibited almost a similar trend to the hardness and gumminess, and it kept increasing upon higher levels of fortification of both cultivars from being the lowest in the control at 10.63 to the highest in Bos 3% at 32.52%.
Springiness, defined as the distance at which the product can recover after the first compression and before the second one (Atta et al., 2023), exhibited very minor differences and it was the lowest (0.97 mm) at 3% level of fortification of both cultivars.
Cohesiveness which is the work area of the second compression divided by the first (Atta et al., 2023), kept decreasing upon the inclusion of OP, with the significantly highest values for the CTRL, Bos 1%, and Sem 1%. A lower cohesiveness corresponds to a higher ability of the product to break or crumble which may affect its acceptability to consumers (Atta et al., 2023).
Gumminess which is “the product of hardness and cohesiveness,” showed a very similar trend to hardness, while resilience, which is the elasticity of the sample or its ability to return to its original state after an applied force (Atta et al., 2023), decreased upon the incorporation of Bosana’s OP at 2 and 3%. Resilience decreased because OP is rich in fibers, and its incorporation disrupts the gluten network leading to a stiffer texture (Gül and Şen, 2017).
Hardness plays a major role in the acceptance of food products, especially bakery products. Consumers also showed a greater preference for samples with lower gumminess and chewiness values. As previously proven, substituting wheat flour with a fiber-rich food matrix results in a decrease in specific volume, leading to an increase in hardness and alveolar density (Sęczyk et al., 2017; Dziki et al., 2019; Atta et al., 2023). These results came in agreement with those reported previously by other authors (Sęczyk et al., 2017; Dziki et al., 2019; Zarzycki et al., 2022; Al-Juhaimi et al., 2024).
3.1.8 Crumb structure image acquisition analysis
Crumb structure and its morphology is another essential parameter in determining the quality of bread along with many others that include taste, color, etc. (Martins et al., 2017; Conte et al., 2018). Bos 1% had a significantly higher cell number and cell density as compared to the CTRL (Table 7).
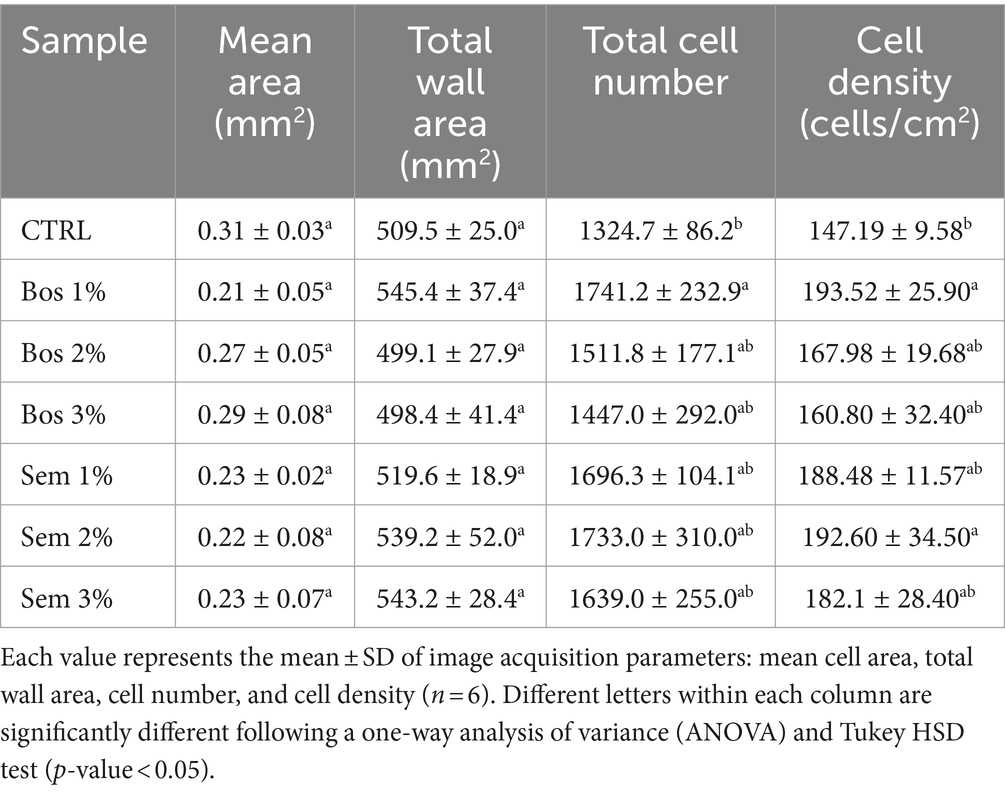
Table 7. Crumb image analysis parameters for unfortified bread, and bread with olive pomace of cultivars Bosana and Semidana at 1, 2, and 3%.
Figure 3 shows the histogram representing the percentage of size distribution of the different alveolar classes of all bread samples. Sem 3% had the greatest presence of alveoli with dimensions 1–9.9 mm2 compared to all the other percentages of both cultivars Bosana and Semidana, and the control. Moreover, bread loaves with Bos 3% showed an increase in the presence of large alveoli ranging between 10 and 210 mm2. Therefore, it can be concluded that the higher the percentage of freeze-dried OP incorporated, the larger the size of the alveoli will be, for both the Bosana and Semidana varieties. Similarly, Švec and Hrušková (2013), obtained similar results upon fortification of bread with barley and millet flours. Both types, although they had higher cell distribution, also decreased the specific volume of fortified bread.
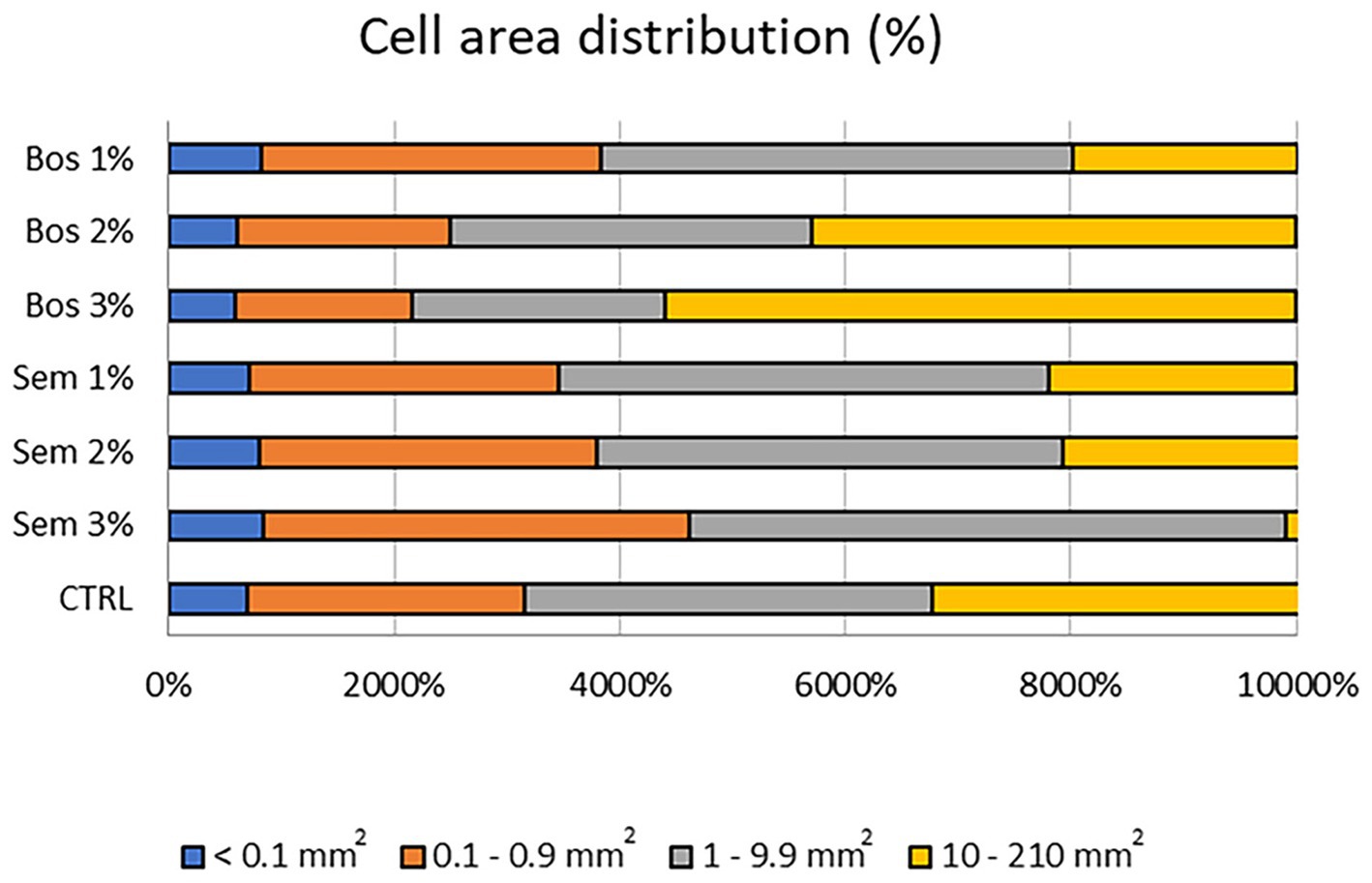
Figure 3. Histogram representing the percentage of size distribution of the different air cells of the control bread, and bread samples fortified with 1, 2, and 3% of OP of cultivars Bosana and Semidana.
Similar results were detected by Martins et al. (2017) upon fortification of bread with different by-products from the agri-food industry at different percentages. The decrease in the mean area also justifies the lowering of the specific bread volume reported previously, as cells did not expand to increase the volume, which also leads to an increase in the hardness of the bread. This decrease may also be associated with insufficient production or improper distribution of gas among alveoli or gas cells to support the structure. Furthermore, the gluten network disruption, caused by diluting wheat flour gluten with a gluten-free matrix like OP, contributes to denser bread (Iuga and Mironeasa, 2020). Moreover, the total wall area did not exhibit significant differences. All these results were supported by Švec and Hrušková (2013).
Conte et al. (2018) stated that an increase in the third class of gas cells which is 1–9.9 mm2 and a decrease in the air cells of an area above 10 mm2, is correlated to a better breadcrumb structure, in addition to having the highest cell density and number. This applies to bread samples fortified with Bos 1%, and all Semidana levels (Figure 3 and Table 7). The inverse effects of the incorporation of 2 and 3% of Bosana OP resulting in lower values of both cell number and cell density (Table 7), may be attributed to the fact that the number of gas cells in the breadcrumb is generated from the gas bubbles during kneading of the dough. However, their size would be affected by additional steps. Therefore, throughout the baking process, especially in the initial stages, an increase in CO2 production is detected leading to a rapid expansion of the size of alveoli, and consequently resulting in their coalescence and potential collapse (Conte et al., 2018).
3.1.9 Sensory evaluation of bread samples
Results of preference ranking tests for the breads fortified with all levels for both cultivars did not show any significant differences for this attribute (Table 8).
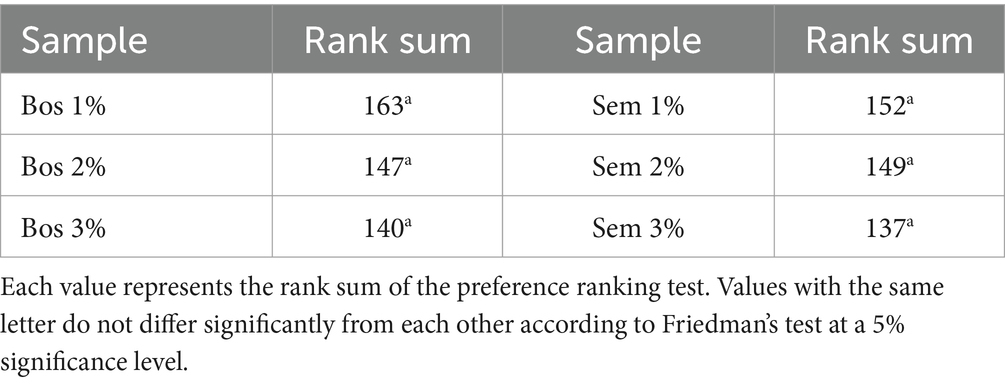
Table 8. Preference ranking tests of breads fortified with 1, 2, and 3% of both cultivars Bosana and Semidana.
However, there is a tendency to prefer Bos 1% bread, followed by Bos 2% and Bos 3%. Similarly, a tendency for the preference for Sem 1% bread was observed, followed by Sem 2 and 3%.
No significant difference was found in the analysis of the paired-comparison test data, for the bread samples that showed a tendency to be more preferred in the two previous ranking tests. Although the panel slightly preferred the Bosana fortified at 1% of OP (with a rank sum of 77 for Bos 1% vs. 63 for Sem 1%) this result was not statistically significant.
3.1.10 Bread quality during storage
The quality parameters of bread including moisture content, water activity, and texture profile analysis (TPA) were monitored over a storage period of 7 days (0, 1, 2, 3, 4, 5, 7) at RT. These parameters were plotted as a function of time for each sample, and they are represented in Table 9. Moisture content and water activity remained constant over time (Tables 9, 10). This was ensured by the plastic packaging system of bread inhibiting any water losses after baking (Secchi et al., 2018).
Concerning the hardness of the bread (Table 11A), all samples showed almost a linear increase in bread hardness throughout the 7 day storage period. It is worth noting that the control bread’s hardness was significantly lower than all other samples at all the measuring points. Progressive increment in the OP substitution levels resulted in a higher hardness of the bread within the same cultivar throughout the storage period. The hardness ranged between 26.21 N and 89.97 N after one day of baking, to reach a range of 83.09 N to 234.50 N on the seventh day after baking. A firmer bread structure in higher levels of OP inclusion would be attributed to the insoluble fiber content of the OP, which leads to a decrease in the bread’s specific volume, and compacts the slice (Dalgetty and Baik, 2006). The increase in hardness over time is a good indicator of bread staling. During staling, the starch-gluten matrix undergoes modifications, and water migrates from the crumb to the crust resulting in hardening the crumb and lessening the crust. The main contributor to bread staling is starch crystallization or retrogradation, mainly amylopectin. During kneading and baking, starch granules swell and gelatinize, and afterwards, with the start of cooling, retrogradation occurs. Amylose, containing fewer glucose molecules than amylopectin, first starts to crystallize as bread cools down, and therefore is responsible for the firming of the crust, and the initial structure of the crumb. Afterwards, during bread ageing, the retrogradation of amylopectin takes place, and this is when the crumb gets firmer over time (Piccinini et al., 2012; Secchi et al., 2018). Therefore, the complex phenomenon of bread staling is not due to moisture loss, and starch can re-gelatinize upon moderate heat application (Zlateva and Chochkov, 2019). The obtained results were in major accordance with the ones by Dalgetty and Baik (2006) and Secchi et al. (2018).
Cohesiveness and resilience had very similar trends, explaining the dependence of cohesiveness on resilience. For all samples, a dramatic decrease in both parameters was detected in the first 2 days after baking, to stabilize afterwards (Tables 11C,F). At the fourth day after baking, the resilience of the CTRL was detected to be a little bit lower than the other samples. This is due, first of all, to the decrease in gluten concentration and to the presence of fibers that interferes with gluten formation or disrupt the gluten network over time (Zlateva and Chochkov, 2019).
Chewiness and gumminess (Tables 11D,E) had similar increasing patterns and were due to the increase in hardness as it contributes majorly to their evolvement. Other factors like cohesiveness, and springiness (Table 11B), recorded almost constant patterns and therefore did not interfere with the increment recorded for chewiness and gumminess. These results agreed with Mamat et al. (2014) who studied the fortification of bread with seaweed composite. Similar results were also obtained by Fik et al. (2012), upon fortification of whole wheat bread with calcium, but bread staling occurred at a further stage than the one recorded in this study, due to their storage in modified atmosphere packaging (MAP).
Upon fortification with chestnut flour, hardness, cohesiveness, chewiness, and resilience were similar to our results as studied by Paciulli et al. (2016). Similarities, in fact, were due to fibers increasing hardness and thus induced bread staling, leading to an increased ability of bread to break and crumble, due to the dilution of wheat with gluten-free matrix, resulting in a higher water binding capacity and the possible hydrogen bonding with starch.
Bread’s shelf-life is usually short and estimated to be on average 3 days. Bread spoilage occurs after a few days due to visible mold or crumb firmness increase (Del Nobile et al., 2003).
Control bread with the lowest hardness values at all times reached 39.43 N on the third day after baking. Bread fortified with 2 and 3% of the OP of both cultivars had similar values on the day of baking. Whereas bread with a 1% level of substitution reached such hardness 24 h after baking, resulting in values of 49.54 N and 48.59 N for Sem 1% and Bos 1%, respectively.
Also, Sivam et al. (2010) claimed that bonds between starch and gluten delay and prevent bread staling, and this also came in accordance with our results, as OP-fortified bread, due to a decrease in the concentration of starch and gluten, underwent staling at a higher rate than the control.
4 Conclusion
In this study, different levels of OP powders were chosen for partial substitution of formula based on wheat for a functional bread-making process. The experiment results showed that bread with higher levels of OP had poor physical characteristics (lower specific volume, firmer texture) to control bread. Sensory evaluation showed that the inclusion of OP at higher levels (2 and 3%) affects the overall acceptability of bread, and an acceptable bread was obtained with 1% of OP levels of both cultivars. Upon fortification of bread samples with OP, image analysis resulted in a greater cell size distribution for fortified breads, compared to the control. The increment in OP levels in bread results in a decrease in the yellowness of the color of bread which appears darker, progressively decreasing both browning and whitening indices of the crust and crumb, respectively. The inclusion of OP notably enhanced the antioxidant activity of the fortified bread. It is worth noting that the 1% inclusion of Bosana’s and Semidana’s OP had similar quality parameters to the control, with a significant increase in functional compounds. To our knowledge, very limited sources have evaluated the physical, technological, and sensory parameters of the incorporation of OP into toast bread.
Therefore, it can, be concluded that OP has great potential as an important ingredient in food processing, particularly in bread-making. Based on the above analyses, this study demonstrated that the level of substitution of 1% OP powder, when using wheat flour, can improve the breadmaking process and the nutritional quality of the bread.
Data availability statement
The original contributions presented in the study are included in the article/supplementary material, further inquiries can be directed to the corresponding author.
Ethics statement
Ethical review and approval were waived for this study since the participation was voluntary. All data were anonymous. Informed consent was obtained from all subjects involved in the study.
Author contributions
PD: Data curation, Formal analysis, Investigation, Methodology, Writing – original draft. RC: Conceptualization, Data curation, Formal analysis, Investigation, Methodology, Supervision, Writing – original draft, Writing – review & editing. MF: Data curation, Formal analysis, Investigation, Methodology, Writing – original draft. CF: Conceptualization, Funding acquisition, Investigation, Supervision, Writing – original draft. AC: Data curation, Formal analysis, Investigation, Supervision, Writing – original draft, Writing – review & editing. LM: Writing – review & editing. GH: Writing – review & editing. AP: Conceptualization, Funding acquisition, Investigation, Supervision, Writing – original draft, Writing – review & editing.
Funding
The author(s) declare that financial support was received for the research, authorship, and/or publication of this article. This research was funded by the IDOLI-the Innovation in Dairy and OLive Industry project, Sardegna Ricerche, POR FESR Sardegna 2014–2020, Program of research and development, Area of specialization: Agroindustria.
Acknowledgments
The authors thank Accademia Olearia Srl for their availability and support during sampling.
Conflict of interest
The authors declare that the research was conducted in the absence of any commercial or financial relationships that could be construed as a potential conflict of interest.
Publisher’s note
All claims expressed in this article are solely those of the authors and do not necessarily represent those of their affiliated organizations, or those of the publisher, the editors and the reviewers. Any product that may be evaluated in this article, or claim that may be made by its manufacturer, is not guaranteed or endorsed by the publisher.
References
AACC. American Association of Cereal Chemist (2001). 10-05.01 guidelines for measurement of volume by rapeseed displacement. 11th Edn. MN, USA: Cereals & Grains Association. St. Paul.
AACC. American Association of Cereal Chemist (2005). 44-15.02 moisture—Air-oven methods. 11th Edn. MN, USA: Cereals & Grains Association. St. Paul.
AACC. American Association of Cereal Chemist (2011). 32-05.01 insoluble, soluble, and total dietary fiber (codex definition) by an enzymatic-gravimetric method and liquid chromatography. Approved methods of analysis. 11th Edn. MN, USA: AACC Int: AACC. American Association of Cereal Chemists. St. Paul.
Abd El-Megeid, A. A., Abdallah, I. Z. A., Elsadek, M. F., and Abd El-Moneim, Y. F. (2009). The protective effect of the fortified bread with green tea against chronic renal failure induced by excessive dietary arginine in male albino rats. World J. Dairy Food Sci. 4, 107–117.
Abiodun, O. A., Adegbite, J. A., and Omolola, A. O. (2012). Chemical and physicochemical properties of Moringa flours and oil. Glob. J. Sci. Front. Res. 12, 13–17.
Adeboye, A. S., Fayemi, O. E., Bamgbose, A., Adewunmi, A., and Sobowale, S. S. (2018). Towards the development of peanut–wheat flour composite dough: influence of reduced-fat peanut flour on bread quality. J. Food Process. Preserv. 42, 1–9. doi: 10.1111/jfpp.13385
Adeleke, R. O., and Odedeji, J. O. (2010). Acceptability studies on bread fortified with Tilapia fish flour. Pakistan J. Nutr. 9, 531–534. doi: 10.3923/pjn.2010.531.534
Albahari, P., Jug, M., Radić, K., Jurmanović, S., Brnčić, M., Brnčić, S. R., et al. (2018). Characterization of olive pomace extract obtained by cyclodextrin-enhanced pulsed ultrasound assisted extraction. Lwt 92, 22–31. doi: 10.1016/j.lwt.2018.02.011
Al-Juhaimi, F., Mohamed Ahmed, I. A., Özcan, M. M., and Albakry, Z. (2024). Effect of enriching with fermented green olive pulp on bioactive compounds, antioxidant activities, phenolic compounds, fatty acids and sensory properties of wheat bread. Int. J. Food Sci. Technol. 59, 3860–3869. doi: 10.1111/ijfs.17133
Amoriello, T., Mellara, F., Galli, V., Amoriello, M., and Ciccoritti, R. (2020). Technological properties and consumer acceptability spent grains, 1–12.
AOAC Association of Official Analytical Chemists (2006a). No. 2003.06. Crude fat in feeds, cereal grains and forage (Randall/Soxtec/hexanes extraction-submersion method). Official methods of analysis. 18th Edn. Gaithersburg, MD, USA: AOAC.
AOAC Association of Official Analytical Chemists (2006b). No. 923.03. Ash of flour. Direct method. Official methods of analysis. 18th Edn. Gaithersburg, MD, USA: AOAC.
AOAC Association of Official Analytical Chemists (2006c). No. 990.03 protein (crude) in animal feed, combustion method. Official methods of analysis. 18th Edn. Gaithersburg, MD, USA: AOAC.
AOAC Association of Official Analytical Chemists (2007). No. 985.29. Total dietary Fiber in foods, enzymatic-gravimetric method. Official methods of analysis. 18th Edn. Gaithersburg, MD, USA: AOAC.
Atta, M. B., Salem, M. A., El-Sayed, M. E., and Elzoghby, A. A. S. (2023). Influence of sweet potato flour and okra mucilage on the rheological properties and water activity of rice bread. J. Sustain. Agric. Environ. Sci. 2, 66–76.
Azadfar, E., Elhami Rad, A. H., Sharifi, A., and Armin, M. (2023). Effect of olive pomace Fiber on the baking properties of wheat flour and flat bread (Barbari bread) quality. J. Food Process. Preserv. 2023, 1–9. doi: 10.1155/2023/1405758
Bchir, B., Rabetafika, H. N., Paquot, M., and Blecker, C. (2014). Effect of pear, apple and date fibres from cooked fruit by-products on dough performance and bread quality. Food Bioprocess Technol. 7, 1114–1127. doi: 10.1007/s11947-013-1148-y
Bolarinwa, I. F., Aruna, T. E., and Raji, A. O. (2019). Nutritive value and acceptability of bread fortified with moringa seed powder. J. Saudi Soc. Agric. Sci. 18, 195–200. doi: 10.1016/j.jssas.2017.05.002
Borsuk, Y., Arntfield, S., Lukow, O. M., Swallow, K., and Malcolmson, L. (2012). Incorporation of pulse flours of different particle size in relation to pita bread quality. J. Sci. Food Agric. 92, 2055–2061. doi: 10.1002/jsfa.5581
Cannas, M., Conte, P., Piga, A., and Del Caro, A. (2023). Green recovery optimization of phenolic compounds from “Spinoso sardo” globe artichoke by-products using response surface methodology. Front. Sustain. Food Syst. 7, 1–14. doi: 10.3389/fsufs.2023.1215809
Caponio, G. R., Difonzo, G., De Gennaro, G., Calasso, M., De Angelis, M., and Pasqualone, A. (2022). Nutritional improvement of gluten-free breadsticks by olive cake addition and sourdough fermentation: how texture, sensory, and aromatic profile were affected? Front. Nutr. 9, 1–15. doi: 10.3389/fnut.2022.830932
Cardinali, F., Belleggia, L., Reale, A., Cirlini, M., Boscaino, F., Renzo, T. Di, et al. (2024). Exploitation of black olive (Olea europaea L. cv. Piantone di Mogliano) pomace for the production of high-value bread. Food Secur. 13,:460, doi: 10.3390/foods13030460
Cardoso, S. M., Guyot, S., Marnet, N., Lopes-da-Silva, J. A., Renard, C. M. G. C., and Coimbra, M. A. (2005). Characterisation of phenolic extracts from olive pulp and olive pomace by electrospray mass spectrometry. J. Sci. Food Agric. 85, 21–32. doi: 10.1002/jsfa.1925
Cedola, A., Cardinali, A., D’Antuono, I., Conte, A., and Del Nobile, M. A. (2020). Cereal foods fortified with by-products from the olive oil industry. Food Biosci. 33:100490. doi: 10.1016/j.fbio.2019.100490
Cedola, A., Cardinali, A., Del Nobile, M. A., and Conte, A. (2019). Enrichment of bread with olive oil industrial by-product. J. Agric. Sci. Technol. B 9, 119–127. doi: 10.17265/2161-6264/2019.02.005
Cequier, E., Aguilera, J., Balcells, M., and Canela-Garayoa, R. (2019). Extraction and characterization of lignin from olive pomace: a comparison study among ionic liquid, sulfuric acid, and alkaline treatments. Biomass Convers. Biorefinery 9, 241–252. doi: 10.1007/s13399-019-00400-w
Collar, C., Jiménez, T., Conte, P., and Fadda, C. (2014). Impact of ancient cereals, pseudocereals and legumes on starch hydrolysis and antiradical activity of technologically viable blended breads. Carbohydr. Polym. 113, 149–158. doi: 10.1016/j.carbpol.2014.07.020
Conte, P., Del Caro, A., Balestra, F., Piga, A., and Fadda, C. (2018). Bee pollen as a functional ingredient in gluten-free bread: a physical-chemical, technological and sensory approach. LWT 90, 1–7. doi: 10.1016/j.lwt.2017.12.002
Conte, P., Pulina, S., Caro, A. Del, Fadda, C., Urgeghe, P. P., Bruno, A. De, et al. (2021). Gluten-free breadsticks fortified with phenolic-rich extracts. Food Secur. 10,:923, doi: 10.3390/foods10050923
Cui, H., Yu, J., Zhai, Y., Feng, L., Chen, P., Hayat, K., et al. (2021). Formation and fate of Amadori rearrangement products in Maillard reaction. Trends Food Sci. Technol. 115, 391–408. doi: 10.1016/j.tifs.2021.06.055
Dahdah, P., Cabizza, R., Farbo, M. G., Fadda, C., Mara, A., Hassoun, G., et al. (2024). Improving the rheological properties of dough obtained by partial substitution of wheat flour with freeze-dried olive pomace. Food Secur. 13:30478. doi: 10.3390/foods13030478
Dalgetty, D. D., and Baik, B. K. (2006). Fortification of bread with hulls and cotyledon fibers isolated from peas, lentils, and chickpeas. Cereal Chem. 83, 269–274. doi: 10.1094/CC-83-0269
Das, L., Raychaudhuri, U., and Chakraborty, R. (2012). Supplementation of common white bread by coriander leaf powder. Food Sci. Biotechnol. 21, 425–433. doi: 10.1007/s10068-012-0054-9
Das, L., Raychaudhuri, U., and Chakraborty, R. (2013). Herbal fortification of common bread by fennel seeds. Food Technol. Biotechnol. 51, 434–440.
De Gennaro, G., Difonzo, G., Summo, C., Pasqualone, A., and Caponio, F. (2022). Olive cake powder as functional ingredient to improve the quality of gluten-free breadsticks. Food Secur. 11:40552. doi: 10.3390/foods11040552
Del Nobile, M. A., Martoriello, T., Cavella, S., Giudici, P., and Masi, P. (2003). Shelf life extension of durum wheat bread. Ital. J. Food Sci. 15, 383–394.
Di Nunzio, M., Picone, G., Pasini, F., Chiarello, E., Caboni, M. F., Capozzi, F., et al. (2020). Olive oil by-product as functional ingredient in bakery products. Influence of processing and evaluation of biological effects. Food Res. Int. 131:108940. doi: 10.1016/j.foodres.2019.108940
Difonzo, G., Troilo, M., Squeo, G., Pasqualone, A., and Caponio, F. (2021). Functional compounds from olive pomace to obtain high-added value foods—a review. J. Sci. Food Agric. 101, 15–26. doi: 10.1002/jsfa.10478
Dziki, D., Cacak-Pietrzak, G., Gawlik-Dziki, U., Sułek, A., Kocira, S., and Biernacka, B. (2019). Effect of Moldavian dragonhead (Dracocephalum moldavica L.) leaves on the baking properties of wheat flour and quality of bread. CYTA—J. Food 17, 536–543. doi: 10.1080/19476337.2019.1609587
Elkatry, H. O., Ahmed, A. R., El-Beltagi, H. S., Mohamed, H. I., and Eshak, N. S. (2022). Biological activities of grape seed by-products and their potential use as natural sources of food additives in the production of Balady bread. Food Secur. 11:31948. doi: 10.3390/foods11131948
Ferrer-Mairal, A., Peñalva-Lapuente, C., Iglesia, I., Urtasun, L., De Miguel-Etayo, P., Remón, S., et al. (2012). In vitro and in vivo assessment of the glycemic index of bakery products: influence of the reformulation of ingredients. Eur. J. Nutr. 51, 947–954. doi: 10.1007/s00394-011-0272-6
Fik, M., Surówka, K., Maciejaszek, I., Macura, M., and Michalczyk, M. (2012). Quality and shelf life of calcium-enriched wholemeal bread stored in a modified atmosphere. J. Cereal Sci. 56, 418–424. doi: 10.1016/j.jcs.2012.06.006
Foti, P., Pino, A., Romeo, F. V., Vaccalluzzo, A., Caggia, C., and Randazzo, C. L. (2022). Olive pomace and Pâté olive cake as suitable ingredients for food and feed. Microorganisms 10, 1–12. doi: 10.3390/microorganisms10020237
Gažarová, M., Lenártová, P., Kopčeková, J., Mrázová, J., Holovičová, M., Chlebová, Z., et al. (2018). Consumption of different types of bakery products and its effect on visceral fat area in healthy population. Rocz. Panstw. Zakl. Hig. 69, 353–362. doi: 10.32394/rpzh.2018.0040
Gomes Natal, D. I., de Souza Dantas, M. I., Teixeira Ribeiro Vidigal, M. C., Machado Rocha Ribeiro, S., Ribeiro Silva, R., and Stampini Duarte Martino, H. (2013). Physical and sensorial properties of potato breads fortified with whole soybean flour. Rev. Chil. Nutr. 40, 62–70. doi: 10.4067/S0717-75182013000100010
Gül, H., and Şen, H. (2017). Effects of rosehip seed flour on the rheological properties of bread dough. Sci. Bull. Ser. F. Biotechnol. XXI 2017, 330–335.
Hadnađev, T. D., Torbica, A., and Hadnađev, M. (2011). Rheological properties of wheat flour substitutes/alternative crops assessed by Mixolab. Procedia Food Sci. 1, 328–334. doi: 10.1016/j.profoo.2011.09.051
Han, Z., Zhu, M., Wan, X., Zhai, X., Ho, C. T., and Zhang, L. (2022). Food polyphenols and Maillard reaction: regulation effect and chemical mechanism. Crit. Rev. Food Sci. Nutr. 64, 4904–4920. doi: 10.1080/10408398.2022.2146653
ISO International Organization for Standardization (2017). Foodstuffs—Determination of water activity. Geneva, Switzerland: International Organisation for Standardization.
Iuga, M., and Mironeasa, S. (2020). Potential of grape byproducts as functional ingredients in baked goods and pasta. Compr. Rev. Food Sci. Food Saf. 19, 2473–2505. doi: 10.1111/1541-4337.12597
Jayaram, V. B., Cuyvers, S., Lagrain, B., Verstrepen, K. J., Delcour, J. A., and Courtin, C. M. (2013). Mapping of Saccharomyces cerevisiae metabolites in fermenting wheat straight-dough reveals succinic acid as pH-determining factor. Food Chem. 136, 301–308. doi: 10.1016/j.foodchem.2012.08.039
Koletta, P., Irakli, M., Papageorgiou, M., and Skendi, A. (2014). Physicochemical and technological properties of highly enriched wheat breads with wholegrain non wheat flours. J. Cereal Sci. 60, 561–568. doi: 10.1016/j.jcs.2014.08.003
Lammi, S., Barakat, A., Mayer-Laigle, C., Djenane, D., Gontard, N., and Angellier-Coussy, H. (2018). Dry fractionation of olive pomace as a sustainable process to produce fillers for biocomposites. Powder Technol. 326, 44–53. doi: 10.1016/j.powtec.2017.11.060
Laus, M. N., Di Benedetto, N. A., Caporizzi, R., Tozzi, D., Soccio, M., Giuzio, L., et al. (2015). Evaluation of phenolic antioxidant capacity in grains of modern and old durum wheat genotypes by the novel QUENCHERABTS approach. Plant Foods Hum. Nutr. 70, 207–214. doi: 10.1007/s11130-015-0483-8
Lin, S., Chi, W., Hu, J., Pan, Q., Zheng, B., and Zeng, S. (2017). Sensory and nutritional properties of chinese olive pomace based high fibre biscuit. Emirates J. Food Agric. 29, 495–501. doi: 10.9755/ejfa.2016-12-1908
Mamat, H., Matanjun, P., Ibrahim, S., Siti, S. F., Abdul Hamid, M., and Rameli, A. S. (2014). The effect of seaweed composite flour on the textural properties of dough and bread. J. Appl. Phycol. 26, 1057–1062. doi: 10.1007/s10811-013-0082-8
Mariotti, M., Pagani, M. A., and Lucisano, M. (2013). The role of buckwheat and HPMC on the breadmaking properties of some commercial gluten-free bread mixtures. Food Hydrocoll. 30, 393–400. doi: 10.1016/j.foodhyd.2012.07.005
Martins, Z. E., Pinho, O., and Ferreira, I. M. P. L. V. O. (2017). Fortification of wheat bread with agroindustry by-products: statistical methods for sensory preference evaluation and correlation with color and crumb structure. J. Food Sci. 82, 2183–2191. doi: 10.1111/1750-3841.13837
Mehder, A. O. A. (2014). Pomegranate peels effectiveness in improving the nutritional, physical and sensory characteristics of pan bread. Available at: https://api.semanticscholar.org/CorpusID:171091039
Mohajan, S., Munna, M., Orchy, T., Hoque, M., and Farzana, T. (2019). Buckwheat flour fortified bread. Bangladesh J. Sci. Ind. Res. 54, 347–356. doi: 10.3329/bjsir.v54i4.44569
Mondal, A., and Datta, A. K. (2008). Bread baking—a review. J. Food Eng. 86, 465–474. doi: 10.1016/j.jfoodeng.2007.11.014
Monteiro, M. L. G., Mársico, E. T., Soares, M. S., Deliza, R., de Oliveira, D. C. R., and Conte-Junior, C. A. (2018). Tilapia-waste flour as a natural nutritional replacer for bread: a consumer perspective. PLoS One 13:e0196665. doi: 10.1371/journal.pone.0196665
Moreira-Rosário, A., Pinheiro, H., Calhau, C., and Azevedo, L. F. (2016). Can wheat germ have a beneficial effect on human health? A study protocol for a randomised crossover controlled trial to evaluate its health effects. BMJ Open 6, e013098–e013099. doi: 10.1136/bmjopen-2016-013098
Olaoye, O., Onilude, A., and Idowu, O. (2006). Quality characteristics of bread produced from composite flours of wheat, plantain and soybeans. African J. Biotechnol. 5, 1102–1106.
Oracz, J., and Nebesny, E. (2019). Effect of roasting parameters on the physicochemical characteristics of high-molecular-weight Maillard reaction products isolated from cocoa beans of different Theobroma cacao L. groups. Eur. Food Res. Technol. 245, 111–128. doi: 10.1007/s00217-018-3144-y
Paciulli, M., Rinaldi, M., Cirlini, M., Scazzina, F., and Chiavaro, E. (2016). Chestnut flour addition in commercial gluten-free bread: a shelf-life study. LWT 70, 88–95. doi: 10.1016/j.lwt.2016.02.034
Pauline, M., Roger, P., Sophie Natacha Nina, N. E., Arielle, T., Eugene, E. E., and Robert, N. (2020). Physico-chemical and nutritional characterization of cereals brans enriched breads. Sci Afr 7:e00251. doi: 10.1016/j.sciaf.2019.e00251
Piccinini, M., Fois, S., Secchi, N., Sanna, M., Roggio, T., and Catzeddu, P. (2012). The application of NIR FT-Raman spectroscopy to monitor starch Retrogradation and crumb firmness in semolina bread. Food Anal. Methods 5, 1145–1149. doi: 10.1007/s12161-011-9360-8
Plustea, L., Negrea, M., Cocan, I., Radulov, I., Tulcan, C., Berbecea, A., et al. (2022). Lupin (Lupinus spp.)-fortified bread: a sustainable, nutritionally, functionally, and technologically valuable solution for bakery. Food Secur. 11:42067. doi: 10.3390/foods11142067
Rahman, T., Akter, S., Sabuz, A. A., and Rana, R. (2021). Characterization of wheat flour bread fortified with Banana flour. Int. J. Food Sci. Agric. 5, 7–11. doi: 10.26855/ijfsa.2021.03.002
Rainero, G., Bianchi, F., Rizzi, C., Cervini, M., Giuberti, G., and Simonato, B. (2022). Breadstick fortification with red grape pomace: effect on nutritional, technological and sensory properties. J. Sci. Food Agric. 102, 2545–2552. doi: 10.1002/jsfa.11596
Ribeiro, T. B., Oliveira, A., Coelho, M., Veiga, M., Costa, E. M., Silva, S., et al. (2021). Are olive pomace powders a safe source of bioactives and nutrients? J. Sci. Food Agric. 101, 1963–1978. doi: 10.1002/jsfa.10812
Secchi, N., Fadda, C., Pinna, I., Del Caro, A., Conte, P., Piga, A., et al. (2018). Improving baking quality of weak gluten semolina using ovine whey powder. J. Food Qual. 2018, 1–10. doi: 10.1155/2018/7901901
Sęczyk, Ł., Świeca, M., Dziki, D., Anders, A., and Gawlik-Dziki, U. (2017). Antioxidant, nutritional and functional characteristics of wheat bread enriched with ground flaxseed hulls. Food Chem. 214, 32–38. doi: 10.1016/j.foodchem.2016.07.068
Selim, k., Badawy, W., and Smetanska, I. (2020). Utilization of olive pomace as a source of bioactive compounds in quality improving of toast bread. Egypt. J. Food Sci. 2020:1038. doi: 10.21608/ejfs.2020.22871.1038
Shewry, P. R., and Hey, S. (2015). Do “ancient” wheat species differ from modern bread wheat in their contents of bioactive components? J. Cereal Sci. 65, 236–243. doi: 10.1016/j.jcs.2015.07.014
Sivam, A. S., Sun-Waterhouse, D., Quek, S. Y., and Perera, C. O. (2010). Properties of bread dough with added fiber polysaccharides and phenolic antioxidants: a review. J. Food Sci. 75, R163–R174. doi: 10.1111/j.1750-3841.2010.01815.x
Švec, I., and Hrušková, M. (2013). Crumb evaluation of bread with hemp products addition by means of image analysis. Acta Univ. Agric. Silvic. Mendelianae Brun. 61, 1867–1872. doi: 10.11118/actaun201361061867
Toumi, O., Conte, P., Moreira, M. G., da Silva, A., João Barroca, M., and Fadda, C. (2022). Use of response surface methodology to investigate the effect of sodium chloride substitution with Salicornia ramosissima powder in common wheat dough and bread. J. Funct. Foods 99:105349. doi: 10.1016/j.jff.2022.105349
Tripathi, B., Tripathi, R., and Vaidya, M. (2018). Influence of orange bagasse addition on chemical composition of biscuits. Pharma Innov. J. 7, 338–341.
Vogrinčič, M., Timoracka, M., Melichacova, S., Vollmannova, A., and Kreft, I. (2010). Degradation of rutin and polyphenols during the preparation of tartary buckwheat bread. J. Agric. Food Chem. 58, 4883–4887. doi: 10.1021/jf9045733
Yanık, D. K. (2017). Alternative to traditional olive pomace oil extraction systems: microwave-assisted solvent extraction of oil from wet olive pomace. LWT 77, 45–51. doi: 10.1016/j.lwt.2016.11.020
Yu, J., and Smith, I. N. (2015). Nutritional and sensory quality of bread containing different quantities of grape pomace from different grape cultivars. E Cronicon Nutr., 291–301.
Zarzycki, P., Wirkijowska, A., Nawrocka, A., Kozłowicz, K., Krajewska, M., Kłosok, K., et al. (2022). Effect of Moldavian dragonhead seed residue on the baking properties of wheat flour and bread quality. LWT 155:112967. doi: 10.1016/j.lwt.2021.112967
Keywords: antioxidant activity, bread, by-products, functional food, olive pomace, physicochemical properties, sensory analysis, shelf-life
Citation: Dahdah P, Cabizza R, Farbo MG, Fadda C, Del Caro A, Montanari L, Hassoun G and Piga A (2024) Effect of partial substitution of wheat flour with freeze-dried olive pomace on the technological, nutritional, and sensory properties of bread. Front. Sustain. Food Syst. 8:1400339. doi: 10.3389/fsufs.2024.1400339
Edited by:
Monica Trif, Centre for Innovative Process Engineering, GermanyReviewed by:
Jagbir Rehal, Punjab Agricultural University, IndiaSeher Kumcuoglu, Ege University, Türkiye
Copyright © 2024 Dahdah, Cabizza, Farbo, Fadda, Del Caro, Montanari, Hassoun and Piga. This is an open-access article distributed under the terms of the Creative Commons Attribution License (CC BY). The use, distribution or reproduction in other forums is permitted, provided the original author(s) and the copyright owner(s) are credited and that the original publication in this journal is cited, in accordance with accepted academic practice. No use, distribution or reproduction is permitted which does not comply with these terms.
*Correspondence: Roberto Cabizza, cmNhYml6emFAdW5pc3MuaXQ=
†These authors have contributed equally to this work