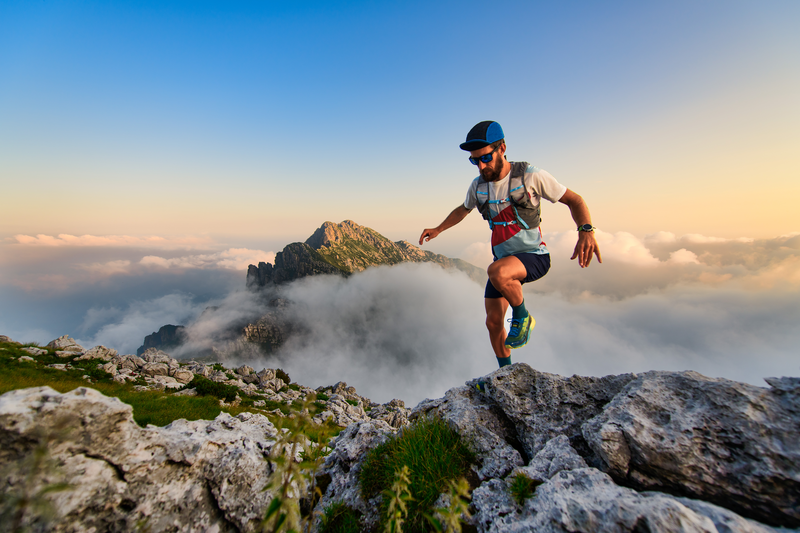
95% of researchers rate our articles as excellent or good
Learn more about the work of our research integrity team to safeguard the quality of each article we publish.
Find out more
ORIGINAL RESEARCH article
Front. Sustain. Food Syst. , 18 June 2024
Sec. Sustainable Food Processing
Volume 8 - 2024 | https://doi.org/10.3389/fsufs.2024.1399801
Cultivated meat refers to edible meat obtained by proliferating cells without killing livestock in a laboratory. The selection of donor animals is a crucial factor for efficient cell culture production. Hanwoo is a native Korean taurine cattle breed raised as livestock in Korea since before 2000 B.C. Cells isolated from Hanwoo, which has little genetic diversity, are expected to be advantageous in cell culture because of the existence of fewer individual differences. However, cells collected from Hanwoo are in a state where efficient culture conditions have not been established. Therefore, in this study, we investigated the effects of mimicking an in vivo environment on the proliferation and differentiation of Hanwoo muscle satellite cells. The culture conditions consisted of CON (37°C/20% O2), T1 (37°C/2% O2), T2 (39°C/20% O2), and T3 (39°C/2% O2). Cell numbers decreased and expression levels of PAX7 and MYF5 decreased at a temperature of 39°C (p < 0.05). Conversely, 2% oxygen increased the number of cells and expression levels of PAX7 and MYF5 (p < 0.05). A temperature of 39°C inhibited the proliferation of Hanwoo muscle satellite cells by reducing the expression of PAX7 and MYF5 (p < 0.05). Conversely, 2% oxygen promoted the proliferation of Hanwoo muscle satellite cells by enhancing the expression of PAX7 and MYF5 (p < 0.05). During differentiation, a temperature of 39°C improved the myotube area and fusion index (p < 0.05). The RT-qPCR and Western blotting results revealed that a culture temperature of 39°C increased expression levels of the MYH2 gene and DES and MYOG proteins (p < 0.05). Additionally, an interactive condition increased expression levels of MYOD1, DES, and MYOG genes (p < 0.05). These results indicated that a temperature of 39°C promoted the differentiation of Hanwoo muscle satellite cells by increasing DES and MYOG protein expression. Thus, the production of cultivated meat using Hanwoo muscle satellite cells is expected to be efficient under 2% oxygen for proliferation and 39°C for differentiation.
The consumption of meat has long been considered a vital source of essential nutrients in the human diet and an indicator of societal development (Ahmad et al., 2018; Van der Weele et al., 2019). The supply of foods such as meat needs to be increased from limited resources to meet the food requirements of the world’s growing population (Godfray et al., 2018; Chriki and Hocquette, 2020). Global meat demand has tripled over the past 50 years, reaching 340 million tons in 2018 (Chen et al., 2022). However, conventionally produced meat is one of the most intensive land uses per kilogram of protein and a major source of greenhouse gases (Ritchie et al., 2022). Therefore, efficient protein production methods are being developed. Cellular agriculture is a technology in which meat and other agricultural products are produced by culturing cells rather than rearing livestock (Mattick, 2018). Cultivated meat refers to edible meat obtained by proliferating cells without animal slaughter. It is also called cell-based meat, clean meat, or in vitro meat (Allan et al., 2019; Lee et al., 2021; Treich, 2021). During cultivated meat production, cells are harvested through a biopsy conducted on a host animal (cattle, pig, and sheep), followed by their proliferation, differentiation, and production into meat tissues (Humbird, 2021; Lanzoni et al., 2022). Various factors, such as age, gender, and breed of the donor, can affect the production quantity and quality of cells (Melzener et al., 2022). Therefore, the selection of donor animals is a crucial factor for efficient cell culture production (Martins et al., 2023). Hanwoo is a native Korean taurine cattle breed raised as livestock in Korea since before 2000 B.C. (Jo et al., 2012; Lee et al., 2014). Korea has four varieties of Hanwoo cattle: brown (Major Hanwoo), black face (Heukwoo), black (Jeju Heukwoo), and tiger color (Chickso). Among them, Brown Hanwoo is the most common type of cattle being raised (Jo et al., 2012). Hanwoo has a low milk production capacity and a slow growth rate. However, it has excellent reproductive ability and relatively good meat quality (Kim and Lee, 2000). Hanwoo is mainly isolated within the Korean Peninsula. Its lineage is managed through pure breeding. Therefore, its genetic diversity is lower than that of other species (Joo et al., 2017). Cells isolated from Hanwoo, which has little genetic diversity, are expected to be advantageous in cell culture because of the existence of fewer individual differences (Park et al., 2023). However, cells collected from Hanwoo are in a state where efficient culture conditions have not been established yet. In general, the core temperature of Hanwoo is 38.5°C, and physiological oxygen concentrations in its muscles are approximately 2.3 to 6.0% (Ast and Mootha, 2019; Kim et al., 2020). Therefore, in this study, we investigated the effects of culture conditions mimicking the in vivo environment of Hanwoo on the proliferation and differentiation of Hanwoo muscle satellite cells.
The gluteobiceps muscle tissues of a 25-month-old Hanwoo were collected by removing the surface fat tissue from the hip area and using a scalpel from Dong-A Food Co., Ltd., Heungdeok-gu, Cheongju-si, Chungcheongbuk-do, stored in an icebox, and transported to the laboratory, where connective and adipose tissues were removed. The extracellular matrix (ECM) in muscle tissues was decomposed using collagenase type II mix (Cat. # LS004176, Worthington Biochemical, Columbus, Ohio, USA) and centrifuged alternately at 70 g for 3 min and 800 g for 5 min to isolate the cells. The cell pellet was obtained through centrifugation and filtration through a 100-μm cell strainer (Cat. # 93100, Cytiva, Marlborough, MA, USA) and a 40-μm strainer (Cat. # 431750, Corning, Corning, NY, USA). Red blood cells were then lysed with ammonium chloride-potassium lysis buffer (Cat. # A1049201, Gibco, Waltham, MA, USA). The obtained Hanwoo muscle cells were stored in a freezing medium (Cat. # 12648010, Gibco) and then in liquid nitrogen until use for subsequent experiments. These cells were considered passage 0 (P0).
Fluorescence-activated cell sorting (FACS) was performed for isolating bovine muscle satellite cells according to the method of Melzener et al. (2022). The P0 cells were cultured in a collagen-coated flask and then transferred to a FACS buffer (1:100, BSA in PBS). These cells were then incubated with FITC anti-sheep CD31 (1:10, Cat. # MCA1097F, Bio-Rad, Hercules, CA, USA), FITC anti-sheep CD45 (1:10, Cat. # MCA2220F, Bio-Rad), APC anti-human CD29 antibody (1,10, Cat. # 303008, BioLegend, San Diego, CA, USA), and PE-Cy™7 anti-human CD56 (1,10, Cat. # 335826, Becton Dickinson, Franklin, NJ, USA) for 30 min at 4°C. CD31−, CD45−, CD29+, and CD56+ cells were sorted using the FACS Aria II Cell Sorter (Becton Dickinson). The obtained muscle satellite cells (Figure 1) were seeded into a growth medium (GM; Ham’s F-10 nutrient mix (Cat. # 11550043, Gibco) supplemented with 20% fetal bovine serum (FBS) (Cat. # 35-015-CV, Corning), 1% penicillin–streptomycin–amphotericin B (PSA) mixture (Cat. # 17-745E, Lonza, Basel, Swiss), and 5 ng/mL of human fibroblast growth factor-basic recombinant protein (bFGF, Cat. # 13256–029, Gibco)) and proliferated at 37°C in an incubator at 5% CO2. The obtained muscle satellite cells were stored in a freezing medium (Cat. # 12648010, Gibco) and liquid nitrogen until use for subsequent experiments.
The isolated Hanwoo muscle satellite cells were cultured with the GM in a collagen-coated flask. The cells were seeded at a density of 1,800 cells/cm2 and cultured in separate incubators under CON conditions (37°C, 20% O2), T1 conditions (37°C, 2% O2), T2 conditions (39°C, 20% O2), and T3 conditions (39°C, 2% O2) for 5 days. For differentiation, the cells were seeded at a density of 1,800 cells/cm2 into a flask coated with Matrigel solution (Cat. # 354234, Corning) and proliferated in a humidified incubator at 37°C with 5% CO2. When confluence was reached, the GM was replaced with a differentiation medium (DM; Dulbecco’s modified eagle’s medium (DMEM) (Cat. # 11995–065, Gibco) supplemented with 2% FBS (Corning) and 1% PSA mixture (Lonza)) and cultured in an incubator under each condition.
For cell counting, confluent cells were harvested using trypsin–EDTA (Cat. # 25300–062, Gibco). The number of cells was determined by staining with trypan blue (Cat. # T8154, Sigma-Aldrich, Saint Louis, MO, USA). The MTS assay was performed using an MTS reagent (Cat. # G3582, Promega, Madison, WI, USA) in a confluent cell flask. Absorbance was then measured at 490 nm.
The Hanwoo muscle satellite cells were fixed with 4% paraformaldehyde (Cat. # SM-P01-050, GeneAll Biotechnology, Seoul, Korea) for 45 min at 37°C. The fixed cells were then permeabilized with 0.1% Triton X-100 (Cat. # T8787, Sigma-Aldrich) and blocked with 2% bovine serum albumin (Cat. # 10738328103, Roche, Basel, Switzerland). The cells were stained with myosin type II antibody (Cat. # M4276, Sigma-Aldrich), secondary antibody (Alexa flour 488; Cat. # A21121, Invitrogen), and Hoechst 33342 (Cat. # H3570, Invitrogen). The image of the stained cells was analyzed using the ImageJ program (NIH).
The cells were stained with myosin antibody (Cat. # M4276, Sigma-Aldrich), secondary antibody (Alexa flour 488; Cat. # A21121, Invitrogen), and Hoechst 33342 (Cat. # H3570, Invitrogen). The image of the stained cells was analyzed using the ImageJ program (NIH). RNA was extracted from the cells using a Total RNA Extraction Kit (Cat. # 17221, iNtRON Biotechnology, Seongnam, Korea) and converted to cDNA using a high-capacity cDNA Reverse Transcription Kit (Cat. # 4368814, Applied Biosystems, Waltham, MA, USA). Genes were amplified using a Fast qPCR 2xSYBR Green Master Mix (Cat. # A46012, Applied Biosystems). Proliferation target genes were PAX7, MYOD1, MYF5, and GAPDH. Differentiation target genes were MYH2, MYOD1, DES, MYOG, MB, and GAPDH. Table 1 presents primers used for the PCR analysis. Relative gene expression was calculated using the 2−ΔΔCt method (Livak and Schmittgen, 2001).
The cells were washed using PBS and lysed on ice using RIPA Lysis Buffer (Cat. # MB-030-0050, Rockland, Philadelphia, PA, USA). The extracted proteins were separated by SDS-PAGE (Cat. # 4561024, Bio-Rad) and transferred to polyvinylidene fluoride (PVDF) membranes (Cat. # 1620175, Bio-Rad). The membranes were blocked with a blocking buffer (Cat. # 120110020, Bio-Rad) and stained with primary antibodies against MYOD1, PAX7, DES, and ACTB. They were then stained with respective affinity-purified goat anti-mouse (H + L)-HRP-conjugated (Cat. # 1706516, Bio-Rad) or affinity-purified goat anti-rabbit IgG (H + L) HRP-conjugated (Cat. # SA002-500, GenDEPOT, Katy, TX, USA) secondary antibodies. The membranes were developed using a Clarity Western ECL Substrate (Cat. # 1705061, Bio-Rad) and visualized on an Amersham™ ImageQuant™ 800 (Cytiva).
All statistical analyses were performed using the SAS program (SAS Institute, Cary, NC, USA). Duncan’s multiple range test was used to compare significant differences (p < 0.05). A two-way analysis of variance (ANOVA) was used to determine the effects of temperature and oxygen concentration as well as the interaction of these two parameters.
To investigate the effects of temperature and oxygen concentration conditions on muscle cell proliferation, the Hanwoo muscle satellite cells were grown at different temperatures (37°C and 39°C) and oxygen levels (20 and 2%) for 5 days. Figure 2A presents the image of the Hanwoo muscle satellite cells on day 5 after being grown at different temperatures and oxygen levels. On the fifth day of proliferation, confluence was observed, and cell counting, as well as the MTS assay, was conducted. In addition, the relative mRNA expression levels of PAX7, MYOD1, and MYF5 as crucial muscle-related genes were measured by RT-qPCR. MYOD1 and PAX7 Protein expression levels were measured through Western blotting. The total and live cell numbers were significantly higher in CON and T1 at a temperature of 37°C than in T2 and T3 at a temperature of 39°C (p < 0.05) (Figure 2B). MTS absorbance was significantly higher in CON and T1 (p < 0.05). Figure 2C illustrates the influence of each condition and interaction through two-way-ANOVA statistics for all experimental results. The temperature and oxygen conditions affected the total cell number (p < 0.0001), live cell number (p < 0.0001), and MTS absorbance (p < 0.0001). The interactive condition affected the total cell number (p < 0.001) and live cell number (p < 0.001).
Figure 2. Proliferation of Hanwoo muscle satellite cells with different temperatures (37°C and 39°C) and oxygen levels (2 and 20%). Treatments: CON (37°C/20% O2); T1 (37°C/2% O2); T2 (39°C/20% O2); and T3 (39°C/2% O2). (A) Microscopic images of Hanwoo muscle satellite cells on day 5 of proliferation in a T25 flask under different conditions (x40). (B) A graph depicting the determination of live cells measured using trypan blue staining after 5 days of proliferation in a T25 flask under different conditions. After growing in a 96-well plate for 5 days, an MTS assay was performed, and absorbance was measured. The data are expressed as the means ± SD (n = 3 for total cell count and live cell count, n = 8 for MTS assay). a–dMeans with different superscriptions differ significantly (p < 0.05). (C) Two-way ANOVA statistical analysis p-value.
The effects of temperature and oxygen concentration on differentiation were investigated. After the Hanwoo muscle satellite cells became confluent under the same temperature and oxygen conditions, they were differentiated for 2 days at different temperatures (37°C and 39°C) and oxygen levels (20 and 2%). Myotubes were fixed, subjected to immunofluorescence staining for myosin, and counterstained with DAPI (Figure 3A). The plates were imaged, and myogenesis was quantified by measuring the myotube area and fusion index. In addition, the relative mRNA expression levels of MYH2, MYOD1, DES, MYOG, and MB as vital muscle-related genes were measured through RT-qPCR. The DES and MYOG protein expression levels were measured through western blotting. Figure 3B presents the results of the myotube area and fusion index after the differentiation of the Hanwoo muscle satellite cells. The myotube area and fusion index were significantly higher in T2 (p < 0.05). The temperature condition affected the myotube area (p < 0.05) and the fusion index (p < 0.01) (Figure 3C). The oxygen condition affected the myotube area (p < 0.01) and the fusion index (p < 0.001). The interactive conditions affected the fusion index (p < 0.01).
Figure 3. Immunofluorescence staining of differentiated Hanwoo muscle satellite cells with different temperatures (37°C and 39°C) and oxygen levels (2 and 20%). Treatments: CON (37°C/20% O2); T1 (37°C/2% O2); T2 (39°C/20% O2); and T3 (39°C/2% O2). (A) Immunofluorescent staining image of Hanwoo muscle satellite cells (x100) with Myosin and Hoechst (for staining nuclei) on day 2 of differentiation after proliferation in a 96-well-plate under each condition. (B) After immunofluorescence staining, myotube area and fusion index were measured using the ImageJ program. The data are expressed as the means ± SD (n = 8). a,bMeans with different superscriptions differ significantly (p < 0.05). (C) Two-way ANOVA statistical analysis p-value.
Figure 4 presents the results of relative mRNA expression levels of PAX7, MYOD1, and MYF5 genes related to proliferation determined through RT-qPCR. The PAX7 and MYF5 expression levels were the highest in T1 and the lowest in T2 (p < 0.05). The significantly highest expression level of MYOD1 was observed in CON, and the lowest expression level was observed in T2 and T3 (p < 0.05). The treatment group with the same oxygen conditions and a temperature condition of 39°C exhibited significantly lower expression of all genes than the treatment group with a temperature condition of 37°C (p < 0.05). As shown in Figure 4B, the temperature and oxygen conditions significantly affected the expression levels of PAX7 (p < 0.0001), MYOD1 (p < 0.0001), and MYF5 (p < 0.0001). The interactive condition significantly affected PAX7 (p < 0.001), MYOD1 (p < 0.0001), and MYF5 (p < 0.0001). Figure 5 presents the results of relative mRNA expressions of MYH2, MYOD1, DES, MYOG, and MB related to differentiation by using RT-qPCR. As shown in Figure 5B, the expression of MYH2 showed the highest value in T2 (p < 0.05). In the case of MYOD1 and DES, a significantly higher value was obtained in T3 (p < 0.05). The expression of MYOG shows a significantly high value in CON and T3 (p < 0.05). The expression of MB shows the significantly lowest value in CON (p < 0.05) with no significant difference (p > 0.05) in T1, T2, and T3. Except for MYH2, other gene expressions indicated significantly higher values in T3. As shown in Figure 5C, the temperature conditions significantly affected the expressions of MYH2 (p < 0.05), MYOD1 (p < 0.0001), DES (p < 0.0001), and MB (p < 0.0001). Oxygen condition significantly affected the expressions of MYH2 (p < 0.0001), MYOD1 (p < 0.0001), DES (p < 0.0001), MYOG (p < 0.01), and MB (p < 0.0001). Interactive conditions significantly affected the expressions of MYH2 (p < 0.0001), MYOD1 (p < 0.0001), DES (p < 0.0001), MYOG (p < 0.0001), and MB (p < 0.0001).
Figure 4. Relative mRNA expression levels of proliferated Hanwoo muscle satellite cells with different temperatures (37°C and 39°C) and oxygen levels (2 and 20%). Treatments: CON (37°C/20% O2); T1 (37°C/2% O2); T2 (39°C/20% O2); and T3 (39°C/2% O2). (A) Relative mRNA expression levels of PAX7, MYOD1, and MYF5 (described as fold change) using RT-qPCR. The data are expressed as the means ± SD (n = 3). a–cMeans with different superscriptions differ significantly (p < 0.05). (B) Two-way ANOVA statistical analysis p-value.
Figure 5. Relative mRNA expression levels of differentiated Hanwoo muscle satellite cells with different temperatures (37°C and 39°C) and oxygen levels (2 and 20%). Treatments: CON (37°C/20% O2); T1 (37°C/2% O2); T2 (39°C/20% O2); and T3 (39°C/2% O2). (A) Image of day 2 differentiation when samples were collected for RT-qPCR and western blotting. (B) Relative mRNA expression levels of MYH2 (Myosin heavy chain 2), MYOD1, DES (desmin), MYOG (myogenin), and MB (myoglobin) (described as fold change) using RT-qPCR. The data are expressed as the means ± SD (n = 3). a–cMeans with different superscriptions differ significantly (p < 0.05). (C) Two-way ANOVA statistical analysis p-value.
Figure 6A presents the results of fold change for CON by normalizing the fluorescence intensity with that of ACTB, the housekeeping gene. Figure 6B is an image of the fluorescence intensity measured by Western blotting for MYOD1, PAX7, DES, MYOG, and ACTB. The MYOD1 expression exhibited no significant difference (p > 0.05). The significantly highest PAX7 expression was observed in T1. Moreover, the treatment group with the same oxygen conditions and a temperature condition of 39°C exhibited significantly lower PAX7 expression than the treatment group with a temperature condition of 37°C (p < 0.05). The significantly lowest DES and MYOG expression was observed in T2 (p < 0.05), and no significant difference (p > 0.05) was observed in CON, T1, and T3. As shown in Figure 6C, temperature and interactive conditions significantly affected the expression of PAX7 (p < 0.0001), DES (p < 0.0001), and MYOG (p < 0.01). The oxygen condition significantly affected the expression of PAX7 (p < 0.0001), DES (p < 0.0001), and MYOG (p < 0.0001).
Figure 6. Relative protein expression levels of Hanwoo muscle satellite cells with different temperatures (37°C and 39°C) and oxygen levels (2 and 20%). Treatments: CON (37°C/20% O2); T1 (37°C/2% O2); T2 (39°C/20% O2); and T3 (39°C/2% O2). (A) Fold-change graphs of MYOD1, PAX7, DES (desmin), and MYOG (myogenin) expression levels compared to CON after normalization against ACTB (β-Actin). The data are expressed as the means ± SD (n = 3). a-c Means with different superscriptions differ significantly (p < 0.05). (B) Representative images of Western blotting against MYOD1, PAX7, DES, MYOG, and ACTB. (C) Two-way ANOVA statistical analysis p-value.
Our results revealed that temperature and oxygen conditions significantly affect cell numbers. The temperature condition of 39°C decreased the cell number and MTS absorbance of Hanwoo muscle satellite cells compared to the normal temperature and oxygen condition. Furthermore, the RT-qPCR and Western blot results revealed that a culture temperature of 39°C reduces the PAX7 and MYF5 gene and protein expression in the Hanwoo muscle satellite cells. Conversely, 2% oxygen increased the cell number as well as the expression of PAX7 and MYF5 genes and proteins in these cells. The PAX gene family defines a group of transcription factors that play crucial roles during organ organogenesis and tissue homeostasis (Seale et al., 2000; Olguín and Pisconti, 2012; González et al., 2016). Among them, PAX7 is responsible for developmental and adult regenerative muscle formation (Collins et al., 2009). Additionally, it is regarded as a key regulator of satellite cell growth and proliferation (Sincennes et al., 2016; Yang et al., 2016). The high expression levels of PAX7 factors increase cell proliferation and prevent premature muscle differentiation (Collins et al., 2009; Zhao et al., 2018). MYF5 is the first MRF expressed during myogenesis (Cai et al., 2020). It is expressed in activated satellite cells and proliferating myoblasts before differentiation and is involved in satellite cell activation and proliferation (Cooper et al., 1999; Gayraud-Morel et al., 2007; Averous et al., 2012). Ustanina reported (Ustanina et al., 2007) that proliferation is delayed in MYF5-deficient satellite cells. Consequently, cell proliferation is reduced because of decreased PAX7 and MYF5 gene and protein expression at 39°C. Conversely, 2% oxygen is considered to promote cell proliferation by enhancing the PAX7 and MYF5 gene and protein expression. Kim et al. (2023) reported that heat treatments at 38.5°C and 41°C enhanced cell proliferation and increased the expression of PAX7 and MYF5 in bovine muscle satellite cells. However, in our study, the culture temperature of 39°C decreased the expression of PAX7 and MYF5, resulting in decreased cell proliferation. These results may be due to differences in donor animals and heat treatment times, but further studies are needed. To summarize the above, we considered that a temperature of 39°C inhibited the proliferation of Hanwoo muscle satellite cells by decreasing the expression of PAX7 and MYF5, whereas 2% oxygen promoted the proliferation of these cells by increasing the expression of PAX7 and MYF5. During differentiation, a temperature of 39°C improved the myotube area and fusion index of Hanwoo muscle satellite cells. RT-qPCR and Western blot results revealed that a temperature of 39°C increases the MYH2 gene and DES and MYOG protein expression in the Hanwoo muscle satellite cells. The interactive condition increased the MYOD1, DES, and MYOG gene expression. MYOD1 determines the myogenic lineage during development and plays an important role in differentiation (Wei and Paterson, 2001; Langley et al., 2002). Cells progress through development by expressing and reducing various factors during proliferation and differentiation. In addition, proliferation and differentiation are completely different processes, and the expression of factors appears very different (Luo et al., 2021). Therefore, it is considered that the expression levels of the MYOD1 gene differed between proliferation and differentiation in this study. Desmin is a muscle-specific protein and the major subunit of the intermediate filaments of skeletal muscles (Paulin and Li, 2004). Additionally, it is among the myogenic markers with a functional role in muscle formation and differentiation (Li et al., 1994; Beier et al., 2011). The expression of structural proteins, such as desmin, increases when cells fuse with myotubes (Yablonka-Reuveni and Day, 2011). Myogenin is an essential factor in differentiation and is expressed when myoblasts differentiate into myotubes (Mastroyiannopoulos et al., 2012). Myogenin-deficient mice die shortly after birth because of skeletal muscle deficiency (Hasty et al., 1993). Myogenin is also used as an indicator of efficient differentiation (Mancini et al., 2011). Therefore, we considered T2 (39°C, 20% O2) to have improved myotube formation because DES and MYOG expression levels, myotube area, and fusion index were significantly higher. Based on these results, we concluded that a temperature of 39°C promoted the differentiation of Hanwoo muscle satellite cells by increasing DES and MYOG protein expression.
The results of this study revealed that a temperature of 39°C decreased both the cell number and the expression of PAX7 and MYF5. Conversely, 2% oxygen had the opposite effect. Based on the aforementioned results, we concluded that a temperature of 39°C inhibits the proliferation of Hanwoo muscle satellite cells by reducing the expression of PAX7 and MYF5. Conversely, 2% oxygen promoted the proliferation of these cells by enhancing the expression of PAX7 and MYF5. During differentiation, a temperature of 39°C improved the myotube area and fusion index. The RT-qPCR and Western blot experiment results revealed that the culture temperature of 39°C increased the MYH2 gene and DES and MYOG protein expression. Additionally, the interactive condition increased the MYOD1, DES, and MYOG gene expression. Based on these results, we noted that a temperature of 39°C promoted the differentiation of Hanwoo muscle satellite cells by increasing the DES and MYOG protein expression. Thus, 2% O2 for proliferation and 39°C for differentiation are expected to be efficient for producing cultivated meat using Hanwoo muscle satellite cells (Figure 7). However, when these conditions are used for cultivated meat production, additional research is warranted because temperature- and oxygen-related economic aspects must also be considered.
Figure 7. Schematic figures show the effects of temperature and oxygen on proliferation and differentiation in culture conditions in Korean beef muscle satellite cells. Schematic diagram was created with BioRender.com.
The original contributions presented in the study are included in the article/supplementary material, further inquiries can be directed to the corresponding author.
Ethical approval was not required for the study involving animals in accordance with the local legislation and institutional requirements because cells were isolated from meat obtained from a commercial slaughterhouse.
GP: Data curation, Investigation, Project administration, Resources, Visualization, Writing – original draft. SP: Formal analysis, Methodology, Validation, Writing – review & editing. SO: Data curation, Investigation, Visualization, Writing – review & editing. S-HL: Formal analysis, Methodology, Validation, Writing – review & editing. JC: Conceptualization, Funding acquisition, Project administration, Supervision, Writing – review & editing.
The author declares financial support was received for the research, authorship, and/or publication of this article. This work was supported by the Korea Institute of Planning and Evaluation for Technology in Food, Agriculture, and Forestry (IPET) through the High Value-added Food Technology Development Project, funded by the Ministry of Agriculture, Food, and Rural Affairs (MAFRA) (321028-5). Additionally, this research was supported by the “Regional Innovation Strategy (RIS)” through the National Research Foundation of Korea (NRF), funded by the Ministry of Education (MOE) (2021RIS-001).
The authors declare that the research was conducted in the absence of any commercial or financial relationships that could be construed as a potential conflict of interest.
All claims expressed in this article are solely those of the authors and do not necessarily represent those of their affiliated organizations, or those of the publisher, the editors and the reviewers. Any product that may be evaluated in this article, or claim that may be made by its manufacturer, is not guaranteed or endorsed by the publisher.
Ahmad, R. S., Imran, A., and Hussain, M. B. (2018). Nutritional composition of meat. Meat Sci. Nutr. 61, 61–75. doi: 10.5772/intechopen.77045
Allan, S. J., De Bank, P. A., and Ellis, M. J. (2019). Bioprocess design considerations for cultured meat production with a focus on the expansion bioreactor. Front. Sustain. Food Syst. 3:44. doi: 10.3389/fsufs.2019.00044
Ast, T., and Mootha, V. K. (2019). Oxygen and mammalian cell culture: are we repeating the experiment of Dr. Ox? Nature Metabolism 1, 858–860. doi: 10.1038/s42255-019-0105-0
Averous, J., Gabillard, J.-C., Seiliez, I., and Dardevet, D. (2012). Leucine limitation regulates myf5 and myoD expression and inhibits myoblast differentiation. Exp. Cell Res. 318, 217–227. doi: 10.1016/j.yexcr.2011.10.015
Beier, J. P., Bitto, F. F., Lange, C., Klumpp, D., Arkudas, A., Bleiziffer, O., et al. (2011). Myogenic differentiation of mesenchymal stem cells co-cultured with primary myoblasts. Cell Biol. Int. 35, 397–406. doi: 10.1042/CBI20100417
Cai, S., Zhu, Q., Guo, C., Yuan, R., Zhang, X., Nie, Y., et al. (2020). MLL1 promotes myogenesis by epigenetically regulating Myf5. Cell Prolif. 53:e12744. doi: 10.1111/cpr.12744
Chen, L., Guttieres, D., Koenigsberg, A., Barone, P. W., Sinskey, A. J., and Springs, S. L. (2022). Large-scale cultured meat production: trends, challenges and promising biomanufacturing technologies. Biomaterials 280:121274. doi: 10.1016/j.biomaterials.2021.121274
Chriki, S., and Hocquette, J.-F. (2020). The myth of cultured meat: a review. Front. Nutr. 7:7. doi: 10.3389/fnut.2020.00007
Collins, C. A., Gnocchi, V. F., White, R. B., Boldrin, L., Perez-Ruiz, A., Relaix, F., et al. (2009). Integrated functions of Pax3 and Pax7 in the regulation of proliferation, cell size and myogenic differentiation. PLoS One 4:e4475. doi: 10.1371/journal.pone.0004475
Cooper, R., Tajbakhsh, S., Mouly, V., Cossu, G., Buckingham, M., and Butler-Browne, G. (1999). In vivo satellite cell activation via Myf5 and Myo D in regenerating mouse skeletal muscle. J. Cell Sci. 112, 2895–2901. doi: 10.1242/jcs.112.17.2895
Gayraud-Morel, B., Chrétien, F., Flamant, P., Gomès, D., Zammit, P. S., and Tajbakhsh, S. (2007). A role for the myogenic determination gene Myf5 in adult regenerative myogenesis. Dev. Biol. 312, 13–28. doi: 10.1016/j.ydbio.2007.08.059
Godfray, H. C. J., Aveyard, P., Garnett, T., Hall, J. W., Key, T. J., Lorimer, J., et al. (2018). Meat consumption, health, and the environment. Science 361:eaam5324. doi: 10.1126/science.aam5324
González, N., Moresco, J. J., Cabezas, F., de la Vega, E., Bustos, F., Yates, J. R. III, et al. (2016). Ck2-dependent phosphorylation is required to maintain Pax 7 protein levels in proliferating muscle progenitors. PLoS One 11:e0154919. doi: 10.1371/journal.pone.0154919
Hasty, P., Bradley, A., Morris, J. H., Edmondson, D. G., Venuti, J. M., Olson, E. N., et al. (1993). Muscle deficiency and neonatal death in mice with a targeted mutation in the myogenin gene. Nature 364, 501–506. doi: 10.1038/364501a0
Humbird, D. (2021). Scale-up economics for cultured meat. Biotechnol. Bioeng. 118, 3239–3250. doi: 10.1002/bit.27848
Jo, C., Cho, S., Chang, J., and Nam, K. (2012). Keys to production and processing of Hanwoo beef: a perspective of tradition and science. Anim. Front. 2, 32–38. doi: 10.2527/af.2012-0060
Joo, S.-T., Hwang, Y.-H., and Frank, D. (2017). Characteristics of Hanwoo cattle and health implications of consuming highly marbled Hanwoo beef. Meat Sci. 132, 45–51. doi: 10.1016/j.meatsci.2017.04.262
Kim, W. S., Daddam, J. R., Keng, B. H., Kim, J., and Kim, J. (2023). Heat shock protein 27 regulates myogenic and self-renewal potential of bovine satellite cells under heat stress. J. Anim. Sci. 101:skad303. doi: 10.1093/jas/skad303
Kim, J., and Lee, C. (2000). Historical look at the genetic improvement in Korean cattle-review. Asian Australas. J. Anim. Sci. 13, 1467–1481. doi: 10.5713/ajas.2000.1467
Kim, N. Y., Moon, S. H., Kim, S. J., Kim, E. K., Oh, M., Tang, Y., et al. (2020). Summer season temperature-humidity index threshold for infrared thermography in Hanwoo (Bos taurus coreanae) heifers. Asian Australas. J. Anim. Sci. 33, 1691–1698. doi: 10.5713/ajas.19.0762
Langley, B., Thomas, M., Bishop, A., Sharma, M., Gilmour, S., and Kambadur, R. (2002). Myostatin inhibits myoblast differentiation by down-regulating MyoD expression. J. Biol. Chem. 277, 49831–49840. doi: 10.1074/jbc.M204291200
Lanzoni, D., Bracco, F., Cheli, F., Colosimo, B. M., Moscatelli, D., Baldi, A., et al. (2022). Biotechnological and technical challenges related to cultured meat production. Appl. Sci. 12:6771. doi: 10.3390/app12136771
Lee, S. Y., Kang, H. J., Kang, J. H., Ramani, S., Park, S., and Hur, S. J. (2021). Principal protocols for the processing of cultured meat. J. Anim. Sci. Technol. 63, 673–680. doi: 10.5187/jast.2021.e40
Lee, S.-H., Park, B.-H., Sharma, A., Dang, C.-G., Lee, S.-S., Choi, T.-J., et al. (2014). Hanwoo cattle: origin, domestication, breeding strategies and genomic selection. J. Anim. Sci. Technol. 56, 1–8. doi: 10.1186/2055-0391-56-2
Li, H., Choudhary, S. K., Milner, D. J., Munir, M. I., Kuisk, I. R., and Capetanaki, Y. (1994). Inhibition of desmin expression blocks myoblast fusion and interferes with the myogenic regulators MyoD and myogenin. J. Cell Biol. 124, 827–841. doi: 10.1083/jcb.124.5.827
Livak, K. J., and Schmittgen, T. D. (2001). Analysis of relative gene expression data using real-time quantitative PCR and the 2− ΔΔCT method. Methods 25, 402–408. doi: 10.1006/meth.2001.1262
Luo, H., Lv, W., Tong, Q., Jin, J., Xu, Z., and Zuo, B. (2021). Functional non-coding RNA during embryonic myogenesis and postnatal muscle development and disease. Front. Cell Dev. Biol. 9:628339. doi: 10.3389/fcell.2021.628339
Mancini, A., Sirabella, D., Zhang, W., Yamazaki, H., Shirao, T., and Krauss, R. S. (2011). Regulation of myotube formation by the actin-binding factor drebrin. Skelet. Muscle 1, 1–13. doi: 10.1186/2044-5040-1-36
Martins, B., Bister, A., Dohmen, R. G., Gouveia, M. A., Hueber, R., Melzener, L., et al. (2023). Advances and challenges in cell biology for cultured meat. Ann. Rev. Anim. Biosci. :12. doi: 10.1146/annurev-animal-021022-055132
Mastroyiannopoulos, N. P., Nicolaou, P., Anayasa, M., Uney, J. B., and Phylactou, L. A. (2012). Down-regulation of myogenin can reverse terminal muscle cell differentiation. PLoS One 7:e29896. doi: 10.1371/journal.pone.0029896
Mattick, C. S. (2018). Cellular agriculture: the coming revolution in food production. Bull. At. Sci. 74, 32–35. doi: 10.1080/00963402.2017.1413059
Melzener, L., Ding, S., Hueber, R., Messmer, T., Zhou, G., Post, M. J., et al. (2022). Comparative analysis of cattle breeds as satellite cells donors for cultured beef. bio Rxiv. doi: 10.1101/2022.01.14.476358
Olguín, H. C., and Pisconti, A. (2012). Marking the tempo for myogenesis: Pax 7 and the regulation of muscle stem cell fate decisions. J. Cell. Mol. Med. 16, 1013–1025. doi: 10.1111/j.1582-4934.2011.01348.x
Park, S., Park, G., Oh, S., Park, Y., Kim, Y., Kim, J., et al. (2023). Investigating proliferation and differentiation capacities of Hanwoo steer myosatellite cells at different passages for developing cell-cultured meat. Sci. Rep. 13:15614. doi: 10.1038/s41598-023-40800-7
Paulin, D., and Li, Z. (2004). Desmin: a major intermediate filament protein essential for the structural integrity and function of muscle. Exp. Cell Res. 301, 1–7. doi: 10.1016/j.yexcr.2004.08.004
Ritchie, H., Rosado, P., and Roser, M. (2022). Environmental impacts of food production. Our World Data.
Seale, P., Sabourin, L. A., Girgis-Gabardo, A., Mansouri, A., Gruss, P., and Rudnicki, M. A. (2000). Pax7 is required for the specification of myogenic satellite cells. Cell 102, 777–786
Sincennes, M.-C., Brun, C. E., and Rudnicki, M. A. (2016). Concise review: epigenetic regulation of myogenesis in health and disease. Stem Cells Transl. Med. 5, 282–290. doi: 10.5966/sctm.2015-0266
Treich, N. (2021). Cultured meat: promises and challenges. Environ. Resour. Econ. 79, 33–61. doi: 10.1007/s10640-021-00551-3
Ustanina, S., Carvajal, J., Rigby, P., and Braun, T. (2007). The myogenic factor Myf5 supports efficient skeletal muscle regeneration by enabling transient myoblast amplification. Stem Cells 25, 2006–2016. doi: 10.1634/stemcells.2006-0736
Van der Weele, C., Feindt, P., van der Goot, A. J., van Mierlo, B., and van Boekel, M. (2019). Meat alternatives: an integrative comparison. Trends Food Sci. Technol. 88, 505–512. doi: 10.1016/j.tifs.2019.04.018
Wei, Q., and Paterson, B. M. (2001). Regulation of MyoD function in the dividing myoblast. FEBS Lett. 490, 171–178. doi: 10.1016/S0014-5793(01)02120-2
Yablonka-Reuveni, Z., and Day, K. (2011). “Skeletal muscle stem cells in the spotlight: The satellite cell” in Regenerating the Heart: Stem Cells and the Cardiovascular System. eds. I. Cohen and G. Gaudette, 173–200. doi: 10.1007/978-1-61779-021-8_11
Yang, Q., Yu, J., Yu, B., Huang, Z., Zhang, K., Wu, D., et al. (2016). PAX3+ skeletal muscle satellite cells retain long-term self-renewal and proliferation. Muscle Nerve 54, 943–951. doi: 10.1002/mus.25117
Keywords: cultivated meat, culture condition, differentiation, Hanwoo, in vivo environment, muscle satellite cells, proliferation
Citation: Park G, Park S, Oh S, Lee S-H and Choi J (2024) Developmental ability of Hanwoo muscle satellite cells under culture conditions mimicking the in vivo environment. Front. Sustain. Food Syst. 8:1399801. doi: 10.3389/fsufs.2024.1399801
Received: 12 March 2024; Accepted: 29 May 2024;
Published: 18 June 2024.
Edited by:
Sara M. Oliveira, International Iberian Nanotechnology Laboratory (INL), PortugalReviewed by:
Claudia Terezia Socol, University of Oradea, RomaniaCopyright © 2024 Park, Park, Oh, Lee and Choi. This is an open-access article distributed under the terms of the Creative Commons Attribution License (CC BY). The use, distribution or reproduction in other forums is permitted, provided the original author(s) and the copyright owner(s) are credited and that the original publication in this journal is cited, in accordance with accepted academic practice. No use, distribution or reproduction is permitted which does not comply with these terms.
*Correspondence: Jungseok Choi, amNob2lAY2h1bmdidWsuYWMua3I=
Disclaimer: All claims expressed in this article are solely those of the authors and do not necessarily represent those of their affiliated organizations, or those of the publisher, the editors and the reviewers. Any product that may be evaluated in this article or claim that may be made by its manufacturer is not guaranteed or endorsed by the publisher.
Research integrity at Frontiers
Learn more about the work of our research integrity team to safeguard the quality of each article we publish.