- 1Department of Soil and Environment, Swedish University of Agricultural Sciences, Skara, Sweden
- 2Department of Crop Production Ecology, Swedish University of Agricultural Sciences, Uppsala, Sweden
- 3Department of Agroecology, Aarhus University, Tjele, Denmark
Introduction: Cattle slurry used as fertilizer in crop production is a mix of feces, urine, water, and bedding material from the housing system. Previous studies have shown that slurry nitrogen (N) availability to crops is dependent on C/N ratio. As the bedding material can contribute a significant part of total slurry carbon (C), its characteristics may affect the C/N ratio of the slurry. There is increasing interest in using the solid fraction from mechanical slurry separation as bedding material, and therefore this study investigated the potential effect of this fraction on slurry N availability, compared with more commonly used bedding materials such as straw and sawdust.
Methods: In two parallel 28-day laboratory incubations, net mineral N release and C mineralization from slurries applied to sandy loam soil were measured. The slurries comprised a liquid fraction (LF) from mechanical cattle slurry separation with a screw-press and different added bedding materials. Liquid fraction was mixed with two types of bedding material, solid slurry fraction (SF) and chopped straw, in different proportions, resulting in C/N ratios of 10, 12, and 14 in the slurry. In additional treatments, two other bedding materials, ground straw and sawdust, with slurry C/N ratio 12, were used.
Results: For SF and chopped straw, similar negative linear correlations were seen between slurry C/N ratio and net mineral N release after 28 days. Carbon mineralization, expressed as a percentage of total C added, was higher from the mixture containing SF than that containing straw, while no clear relationship with C/N ratio was found. At slurry C/N ratio 12, net release of mineral N was 28–39% of total N and decreased in the order: sawdust>chopped straw=SF=ground straw. Net C mineralization at the same slurry C/N ratio was 33–46% and decreased in the order: SF=ground straw>chopped straw>sawdust.
Discussion: For bedding materials with similar fiber composition (i.e., SF and straw), differences in C availability due to particle size or degree of degradation by microorganisms did not influence slurry N availability measurably. For sawdust, with high lignin content, the results indicate that limited C availability may lead to lower slurry N immobilization.
1 Introduction
Animal manure is an important source of nitrogen (N), a vital plant nutrient in crop production. However, only around 50% of the N in cattle slurry is present as easily available ammonium (NH), while the rest is in organic form (Webb et al., 2013). The fraction of slurry N available for plant uptake as NH or NO is influenced by several factors, including animal type (Lukehurst et al., 2010), feed (Sørensen et al., 2003; Powell et al., 2006), pre-treatments such as solid-liquid separation (Fangueiro et al., 2015), anaerobic digestion (Möller and Müller, 2012), or acidification (Fangueiro et al., 2013), and slurry storage (Sørensen, 1998). The 1st-year availability of slurry N is negatively correlated with its carbon/nitrogen (C/N) ratio (Sørensen et al., 2003; Delin et al., 2012). Organic bedding materials are rich in C and poor in N, and may hence increase the C/N ratio in slurry. However, organic bedding materials differ in e.g., lignin content, particle size, and degree of degradation of organic matter, which may influence C availability and thereby mineralization/immobilization of N. Commonly used bedding materials include sawdust, sand, woodchips, straw, and peat (Ferraz et al., 2020; Frondelius et al., 2020). With pressurized filtration of slurry using a screw-press separator, a fibrous solid fraction suitable as bedding material can be produced (Hjorth et al., 2010). There is interest in using this solid fraction (SF), also called recycled manure solids (RMS), as bedding material in dairy housing systems (Husfeldt et al., 2012; Lybæk and Kjær, 2019; Leach et al., 2022).
In Sweden, free stall barns with cubicle housing is the most common housing system in dairy production (Växa Sverige, 2024), with concrete passageways cleaned with automatic scrapers, transporting the slurry to storage tanks outside the building. Storage tanks for slurry require coverage, either with a roof or a naturally formed cover crust, to reduce ammonia emissions (Swedish Board of Agriculture, 2021). Swedish standard figures state that the slurry production per dairy cow is 26–29 tons per year (Andersson et al., 2023) and the amount of bedding material needed is 400 kg (Agriwise, 2023), which implies ~13–15 g bedding kg−1 slurry. Depending on the need for and use of the solid fraction, the amount of slurry that is separated may differ between farms with slurry separation. At the farm where the material for this study came from, untreated slurry collected from a pumping pit between the cow shed and the slurry separator had an average DM content of 8.5% which is close to the Swedish standard value of 9% (Andersson et al., 2023), while separated slurry collected from the storage tank had an average DM content of 5.3% (n = 7 over 3 years; Andersson and Delin, unpublished1). The C/N ratio of untreated and separated slurry was 10.7 and 6.4, respectively.
When SF is used as bedding material, partly degraded material in the form of animal feces is re-circulated in the system. With the most easily degradable C already degraded in the rumen, SF as bedding material could potentially reduce slurry N immobilization, due to a lower C availability than with other bedding materials, but knowledge in this area is limited. For slurry application in spring and summer, less immobilization could enhance crop N uptake and hence reduce the risk of N re-mineralization and nitrate leaching after harvest, since nitrate leaching is strongly dependent on whether there is a crop present to take up the nitrogen (Sørensen et al., 2023). Compared with untreated slurry, solid-liquid separation reduces ammonia emissions from field application of the liquid fraction, while the overall ammonia mitigation effect when including emissions from the solid fraction is more uncertain (Pedersen et al., 2022). Slurry separation can also reduce greenhouse gas emissions (Amon et al., 2006; Holly et al., 2017). Solid-liquid separation thus potentially has important environmental benefits, but a better understanding of the individual and overall effects is needed.
The aim of this study was to investigate if the type and properties of the bedding material added to cattle slurry affects the N and C turnover of the slurry within the 1st month after slurry application, and thus the short term potential of the slurry to supply N to a crop. The results could be used to improve advice to farmers about the 1st year fertilizer value they can expect from their slurry, so that they can adjust their crop fertilization rates accordingly. In all treatments, the liquid fraction from mechanical slurry separation (LF) was used, representing cattle slurry without bedding material. In a two-factor experiment with the factors bedding material and C/N ratio, chopped straw and SF was added in different amounts to obtain certain slurry C/N ratios, and the slurry mixtures were compared to determine whether the relationship between slurry C/N ratio and N availability differed between the two bedding materials. In additional treatments, aiming at the same slurry C/N ratio, addition of different bedding materials (chopped straw, ground straw, solid fraction, and sawdust) to LF was compared, in order to investigate their effect on slurry turnover rate and N availability. The hypotheses were that: (1) carbon dioxide (CO2) production increases with increasing chemical and physical C availability of the bedding material to microbial utilization, where C availability was assumed to be lowest for sawdust (due to high lignin content), followed by SF (where easily degradable compounds in the consumed feed has been digested in the rumen, increasing the proportion of recalcitrant compounds in the SF), higher for chopped straw with large particle size, and highest for ground straw with small particle size; (2) net mineral N release is related to the C availability of the bedding material, with lower N immobilization, and thereby higher net N mineralization, from slurries with a lower content of easily available C; and (3) in chopped straw and SF bedding materials, there is a negative linear relationship between slurry C/N ratio and net mineral N release and the slope of this relationship is steeper for chopped straw, which is a more easily available C source than solid fraction.
2 Materials and methods
2.1 Soil, manure, and bedding materials
The soil used in laboratory incubation experiments was a sandy loam with 17% clay, 61% sand, 3% organic matter, and pH (1:5 H2O) 6.7. It was collected from a field at SLU's experimental farm Götala close to Skara in western Sweden (58°22′N, 13°29′E). The crop rotation at the farm includes both 3–4 years grass/clover forage leys and annual crops, with frequent addition of slurry and farmyard manure from beef cattle. Top soil was collected from a plowed field after a cereal crop in late autumn, ~2 months before experiment start and stored in open containers at cool temperature (0–5°C) until use. Two separate incubation experiments, one on N turnover and one on C turnover, were performed. These started on different dates (February 8 and 22, 2022, respectively), so soil preparation was performed using two separate batches, one for each experiment. The procedure used was the same for both batches, and started 10 and 13 days before the start of the N and C turnover incubation, respectively. To reduce the water content, soil was air-dried at 16–18°C for 2 days, with gentle mixing twice a day. The soil was sieved through a coarse (7 mm) mesh sieve and water-holding capacity (WHC) was determined using the method described in Jansson (1958). Water content after drying was 56 and 51% of WHC, respectively, for the two batches. Both soil batches were pre-incubated at 15°C in a climate chamber (Termaks, KB 6395, Nordic Labtech AB, Fjärås, Sweden), for 8 and 11 days, respectively.
Liquid (LF) and solid (SF) fractions from screw-press separated cattle slurry were collected from an organic dairy cattle farm (Otterslättens Lantbruk, Hova, Västra Götaland County, Sweden) 2 weeks before the start of the first experiment. Both fractions were collected directly from the slurry separator (CRI-MAN SM 260/75 FA DM, CRI-MAN, Correggio, Italy). The fractions were stored at cool temperature (0–5°C) until the start of the experiment.
Winter wheat straw was collected from a conventional farm. To obtain chopped straw, the straw was cut with scissors into ~0.5–1.0 cm pieces. To obtain ground straw, pieces of straw were milled in a grain mill to maximum particle size 0.8 mm. Sawdust (spruce and pine in unknown proportions) was collected from a commercial sawmill and sieved through a 2 mm mesh sieve.
2.2 Analyses of manure, bedding material, and soil samples
Samples of LF from slurry separation and the bedding materials used in the experimental slurries were analyzed for dry matter content (DM), total C, total N, and ammonium-N at the Soil and Plant Laboratory, Swedish University of Agricultural Sciences (SLU), Uppsala, Sweden. Total C and organic N were analyzed by combustion of dried samples followed by elemental analysis [928 Series Macro Determinator (model CN928), LECO, St. Joseph, MI, USA]. Ammonium-N was extracted with 2M potassium chloride (KCl) and analyzed using a flow injection analyzer [FIAstar Analyzer (5000), FOSS, Hillerød, Denmark]. Total N was calculated as the sum of organic N and ammonium-N.
After the incubations, an additional analysis of fiber fractions [neutral detergent fiber (NDF), acid detergent fiber (ADF), and acid detergent lignin (ADL)] was performed on stored samples of the original bedding materials, at the laboratory of the Department of Applied Animal Science and Welfare, SLU, Uppsala, using the method described by Van Soest et al. (1991). Acid detergent lignin was analyzed by the Klason lignin method, using sulfuric acid (72%). Hemicellulose content was calculated as the difference between NDF and ADF, and cellulose as the difference between ADF and ADL. As shown in Table 1, the concentrations of fiber fractions (hemicellulose, cellulose, and lignin) were similar in solid fraction and straw, while sawdust had a higher content of both cellulose and lignin, and higher total NDF content.
Soil samples from the N incubation were analyzed for ammonium-N and nitrate-N at the Soil and Plant Laboratory, SLU, Uppsala. Milled, frozen soil samples were extracted with 2M KCl and then analyzed by colorimetry on a Seal AA3 auto-analyzer (SEAL Analytical Inc., Mequon, Wisconsin, USA).
2.3 Treatments
In all treatments, the bedding materials were added to the LF from slurry separation, which was assumed to represent “pure” cattle manure without any bedding material added. This allowed differences in slurry properties related to the degree of slurry separation to be analyzed. Using LF (rather than unseparated slurry with higher DM content) as the basis for the treatments also resulted in greater variation in C/N ratio in the mixtures, without reaching unrealistically high C/N ratios in relation to that on real farms. The mixtures of LF and bedding materials are referred to hereafter as “slurry” or “slurries.”
The amount of slurry added to the soil was based on supplying 50 kg total N ha−1 in all treatments, i.e., 26 tons ha−1 with LF, in this experimental setting with a soil depth of 50 mm corresponding to 102 mg kg−1 dry weight (DW) of soil. To obtain the different desired C/N ratios, the relative proportions of LF and bedding material were varied between the treatments. Straw and sawdust contributed very little N to the slurries, and in these treatments the proportion of bedding material was low and the amount of LF was more or less constant between C/N ratios. For SF, with higher ammonium N concentration, the amount of LF was reduced by at most 23%. Ammonium N content relative to total N in the slurries varied slightly between treatments and was on average 35% (Table 2). Due to the differences in N concentration, the amount of bedding material that needed to be added varied widely, between 12 and 127 g kg−1 LF, and there was also variation in the amount of total C applied to the soil (Table 2).
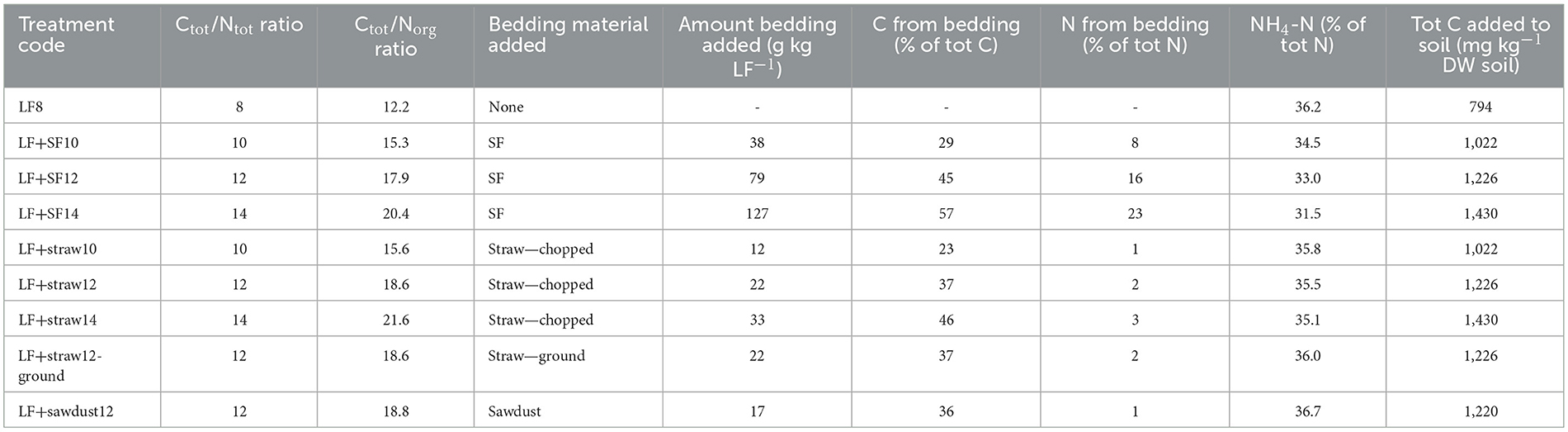
Table 2. Properties of the liquid fraction (LF) from separated slurry and of mixtures of LF and different bedding materials [solid fraction (SF), straw, and sawdust] used in experimental slurries.
2.4 Incubation for analysis of mineral N
Small plastic flowerpots (height 50 mm, volume ~98 cm3) were used as soil containers. On the day before experiment start, a filter paper was placed at the bottom of each pot to prevent soil loss through the drainage holes, and 70 g of pre-incubated soil were added and lightly packed down. The pots were placed in plastic boxes (36 x 24 x 13 cm), each containing pots for three replicates of one treatment and all sampling times, and placed in the same climate chamber where the soil was pre-incubated. The temperature was uniform in all parts of the chamber and was kept at 15 ± 0.3°C throughout the experiment. At the start of the experiment, one box at a time was removed from the climate chamber. The bedding material was added first to each pot and evenly distributed over the soil surface, and then LF was added. The separate weighing and addition of bedding material and LF was done to ensure that accurate C/N ratios were obtained. For ground straw and sawdust, the manure layer was stirred gently, taking care not to disturb the soil beneath, in order to create a slurry from the hydrophobic bedding material and the LF. Different amounts of water was added to obtain the same soil water content (70% of WHC) in all treatments and then another 45 g of pre-incubated soil were added to each pot, on top of the slurry, and gently packed down. The pots in their plastic box were then returned to the climate chamber. The reason for adding the experimental slurry as a layer in the middle, rather than thoroughly mixing it with the entire soil volume, was to mimic a real-life situation with animal slurry incorporated by plowing or injection, resulting in a more uneven distribution of slurry within the soil.
The lids of the plastic boxes were kept closed to prevent the soil from drying out. To ensure enough oxygen supply and avoid anaerobic conditions, the boxes were aerated at intervals of 3–4 days by opening the lid for a few minutes. On days 3, 7, 14, and 28, the three replicates of each treatment were destructively sampled for mineral N analysis. The entire soil volume from each replicate was placed in a plastic bag and frozen within 30 min for later analysis. To ensure that the water content remained constant during incubation, the boxes were weighed on each sampling occasion and, when necessary, deionized water was sprayed over the pots to replace the weight loss.
2.5 Incubation for analysis of CO2 production
The procedure for preparing the soil and adding LF and bedding materials in incubation for analysis of CO2 production was similar to that used in incubation for N analysis. However, the water content was kept slightly lower, at 65% of WHC, since the 70% used in the N incubation proved to be slightly too high, causing mold in some pots. Three replicates of each treatment were prepared. Each replicate was placed in a glass jar (outer diameter 120 mm, height 185 mm, volume 1,660 cm3) with metal screw-lid, together with a CO2 trap. The CO2 trap consisted of a 50 mL plastic scintillation vial with 30 mL of 0.3 M KOH for the first measurements and 0.2 M KOH from day 10, absorbing the CO2 produced in the jar. To ensure that all CO2 produced was captured, the jar lids were kept closed, except when the traps were changed and CO2 production measured. The KOH concentration was changed in order to permit the desired sampling frequency throughout the experiment, since the activity was expected to be lower during the later stage of incubation and a sufficiently high saturation level in the traps was needed to allow precise measurements. On days 2, 5, 10, 15, 21, and 28, the CO2 traps were changed and electrical conductivity (EC) in the KOH solution was measured using a conductivity meter (ProfiLine Cond 3310 Portable Conductivity Meter, WTW, Weilheim, Germany). At each sampling occasion, the jars were aerated for a few minutes, thereafter a new CO2 trap was placed in the jar, the lid was immediately closed and the jar was returned to the climate chamber. To correct for background CO2 in the air and for basic soil respiration, the set-up included one blank treatment with only a CO2 trap and one control treatment without any slurry added to the soil.
2.6 Calibrations for CO2 determination
For calibration of the conductivity meter, solutions with different ratios of KOH and potassium carbonate (K2CO3) were prepared in order to imitate absorption of different amounts of CO2, following the procedure described by Smirnova et al. (2014). Constant A, describing the theoretical maximum amount of CO2 absorbed, was calculated for the 0.3 M and 0.2 M KOH solutions as:
where C(t0) is conductance before any absorption of CO2, C(t1) is conductance at time t1 and A is empirically determined.
Temperature calibrations for the 0.3 M and 0.2 M KOH solutions at temperatures between 15 and 30°C revealed a negative linear correlation between conductivity and temperature. The resulting equations, obtained by fitting a straight line to the data points in Excel, were used for temperature correction in the conductivity calculations.
2.7 Data treatment
2.7.1 Carbon
As a first step, a temperature correction to 20°C was made for the measured conductivity data, using the temperature calibration described above. The equations used for correction (obtained from Excel) were: y = −0.261x + 69.181 for the 0.3 M KOH solution and y = −0.1774x + 47.142 for the 0.2 M solution, where x is temperature (°C) and y is conductance (mS cm−1). From the conductivity values obtained, CO2 production for each sampling period was calculated using Equation (1), with constant A being 346.7 for 30 mL 0.3 M KOH and 235.2 for 30 mL 0.2 M KOH. Checks for outliers revealed that in a few cases one of the three replicates had considerably higher CO2 production than the other two, indicating potential leaks between the glass jar and the lid. When the standard deviation within a treatment was more than 25% of the mean, the deviant value was omitted. This was the case for one of the blank samples on sampling days 2, 5, and 10.
For each sampling occasion, the average value of the blank samples was subtracted from all treatment values, to remove effects of background CO2. To correct for basic soil respiration, the average value of the control treatment replicates with unfertilized soil was subtracted from the CO2 values for the slurry treatments. As a last step, cumulative CO2 production and amount of CO2 produced per day, as a percentage of total added C, were calculated for each treatment. The amount of CO2 absorbed by the traps was in the range 0.2–0.6 mg day−1 for the blank samples and 1.5–2.4 mg day−1 for the control treatment with unfertilized soil, with no clear pattern over time. For the fertilized treatments, CO2 production (before correction for blank and control) decreased from 20.4–26.0 mg day−1 at the first sampling occasion to 3.6–6.2 mg day−1 after 28 days.
2.7.2 Nitrogen
The amount of mineral N supplied from slurry addition to soil is hereafter referred to as Net mineral N release, expressed as % of added total N. It gives a measure of potentially plant-available N from slurry, and was calculated according to Equation (2):
Net mineralization of slurry N over a given period (day 0-x), which describes the mineralization-immobilization processes, was calculated according to Equation (3):
where net N mineralization is expressed as a percentage of total N added. It was also recalculated as a percentage of mineral N and organic N added.
2.7.3 Statistical analyses
For statistical analyses, the software Minitab (Version 21.3.1, Minitab18, Ltd., Coventry, UK) was used. To test the main effects and potential interactions of the factors bedding material (levels chopped straw and SF) and C/N ratio (levels 10, 12, and 14) on net mineral N release and CO2 production, two-way ANOVA analyses were performed using the General Linear Model function. Response variables were mineral N content at day 3, 7, 14, and 28 and cumulative CO2 production at day 2, 5, 10, 15, 21, and 28. One-way ANOVA (using the General Linear Model) was used to test for effects of different bedding materials at C/N ratio 12. To test whether a transformation of data was needed, results from the Box-cox transformation options “No transformation” and “Optimal λ” in Minitab were compared. Since data transformation in none of the cases significantly affected the results, the ANOVA and regression analyses on non-transformed data are presented. Pairwise differences were tested with a Tukey test at significance level 5%. To test whether the slope of the regression between net mineral N release and slurry C/N ratio at days 14 and 28 differed between SF and chopped straw, regression models were fitted, with C/N ratio as a continuous variable and bedding material as a categorical variable. An interaction for C/N ratio×bedding material implies significant differences in the regression coefficient (describing the slope of the regressions for the two bedding materials).
3 Results
3.1 Effect of C/N ratio and differences between chopped straw and SF
3.1.1 Net mineral N release
The two-way ANOVA revealed increased net mineral N release with decreasing slurry C/N ratio, except at day 3 (Supplementary Table 1, Figure 1A). The analyses showed no interaction between the factors C/N ratio and bedding material, except at day 7, when net mineral N release from slurry with straw was higher at C/N ratio 14 than at C/N ratio 12 (Supplementary Table 2). Initial net N immobilization occurred at all C/N ratios, but from day 3 the net mineral N release patterns diverged between C/N ratios (Figure 1A). For C/N ratio 8 and 10, initial immobilization changed to net N mineralization from day 3, while for C/N ratio 12 the immobilization phase lasted somewhat longer. Slurry with C/N ratio 14 showed net N immobilization throughout the incubation, while the net mineralization declined for slurry C/N ratio 10 and 12 toward the end of the experiment (Figure 1A). Averaged over C/N ratios 10–14, chopped straw as bedding material had higher net mineral N release (i.e., less N immobilization) than SF at day 7 and 14, while there was no difference after 28 days (Supplementary Table 1, Figure 1B).
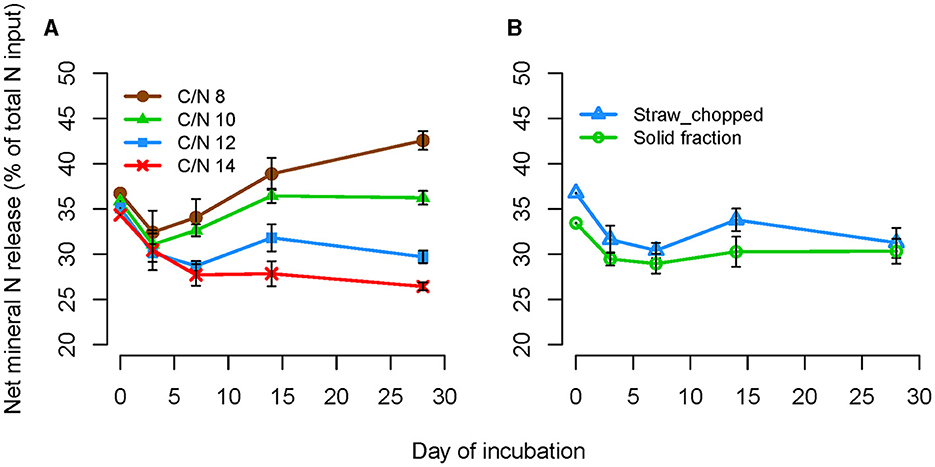
Figure 1. Effect of (A) C/N ratio and (B) bedding material type on net mineral N release from experimental slurries after 3, 7, 14, and 28 days of soil incubation. Values at day 0 are the calculated amounts added for each treatment. In (A), the values for C/N ratio 10, 12, and 14 are mean values for the bedding materials solid fraction and chopped straw (n = 6). In (B), the values for the bedding materials are mean values for C/N ratios 10, 12, and 14 (n = 9). C/N ratio 8 was not included in the two-way ANOVA, but is shown in the figure for comparison (n = 3). Error bars represent standard error of the mean (SEM). The two-way ANOVA showed a significant effect of C/N ratio (p < 0.05) for day 7, 14, and 28. For the factor bedding material, p < 0.05 at day 7 and 14. An interaction between the factors was seen at day 7 (p < 0.05).
By the end of the experiment, average net mineral N release from the experimental slurries amounted to 43, 36, 30, and 26% of total N added for C/N ratio 8, 10, 12, and 14, respectively (Supplementary Table 2, Figure 1A). This corresponded to net immobilization of 13 and 21% of added mineral N (i.e., 5 and 7% of added total N) for C/N ratio 12 and 14, respectively. At C/N ratio 10, the amount of soil mineral N was similar at the start and end of the experiment, while LF without any bedding material added and C/N ratio 8 resulted in net N mineralization, corresponding to 10% of added organic N (i.e., 6% of added total N).
There was a negative linear correlation between slurry C/N ratio and net mineral N release from the slurries with straw or SF addition after both 14 and 28 days (Supplementary Table 1, Figure 2). On day 14, the correlation was stronger for SF than for straw (Figure 2A), and there was a tendency for a steeper slope of the regression for SF (p = 0.109). By day 28, there were no longer any differences between SF and straw (Supplementary Table 1, Figure 2B).
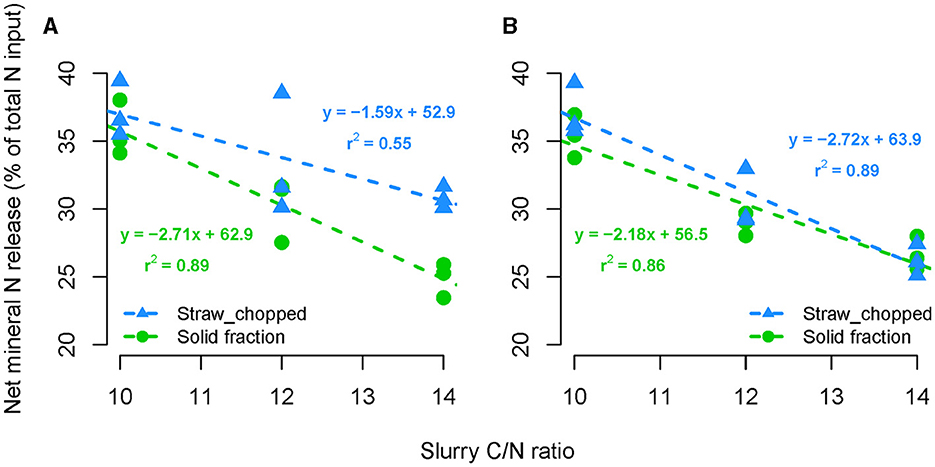
Figure 2. Relationship between C/N ratio and net mineral N release from experimental slurries with the bedding materials chopped straw and solid fraction after (A) 14 and (B) 28 days of incubation. Regression analysis showed a p-value < 0.001 for the factor C/N ratio at both day 14 and 28. For bedding material, the effect was non-significant (p > 0.05) both at day 14 and 28. For the interaction between C/N ratio and bedding material, the p-value was 0.109 at day 14 and 0.292 at day 28.
3.1.2 CO2 production
The two-way ANOVA for CO2 production showed interaction between the factors C/N ratio and bedding material at days 2, 5, and 10, and a tendency for interaction at days 15, 21, and 28 (Supplementary Table 3). The rate of CO2 production from both the SF and chopped straw slurries was highest during the 1st days, followed by considerably lower and slowly declining CO2 production during the rest of the incubation (Figure 3). The decline in CO2 production rate during the 2 last weeks of the experiment was most pronounced for C/N ratio 8 (Figure 3). Comparing C/N ratios, cumulative CO2 production at the end of the experiment was lowest for C/N ratio 14, while no difference was seen between the other C/N ratios (p = 0.002; Supplementary Table 4). Comparing the two bedding materials, cumulative net CO2 production by day 28 was higher for slurry with SF than with straw (p < 0.001), corresponding to 45 and 39% of total added C, respectively (Supplementary Table 4, Figure 3).
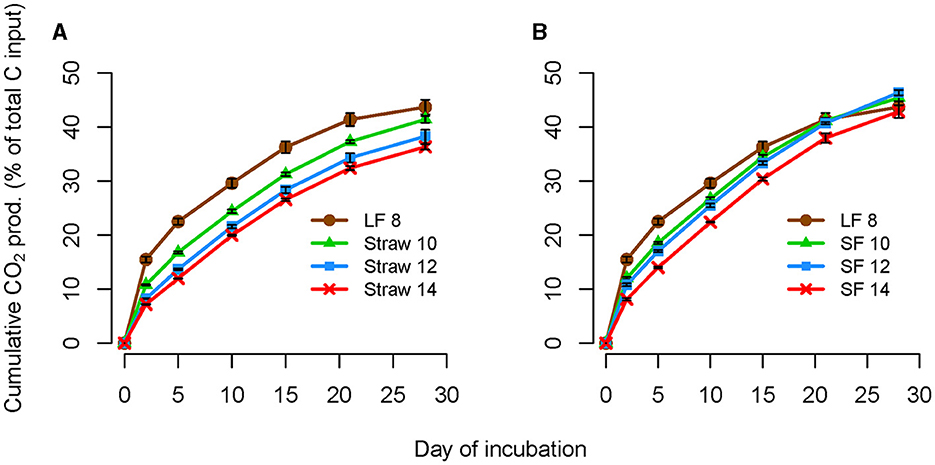
Figure 3. Cumulative net CO2 production from experimental slurries at different C/N ratios and with the bedding materials (A) straw and (B) solid fraction during soil incubation (n = 3). C/N ratio 8 (without bedding material) was not included in the two-way ANOVA analysis, but is shown in the figure for comparison (n = 3). Error bars represent standard error of the mean (SEM). The two-way ANOVA showed significant main effects (p < 0.05) for both C/N ratio and bedding material at all sampling occasions. The interaction between C/N ratio and bedding material had p-values < 0.05 for day 2, 5, and 10.
3.2 Effect of bedding material particle size and C availability
3.2.1 Net mineral N release
All four slurries at C/N ratio 12, with different bedding materials, showed initial net immobilization, followed by different patterns for the net mineral N release (Figure 4). Sawdust as bedding material resulted in higher net mineral N release than from the other bedding materials from day 7 (Supplementary Table 5, Figure 4). On day 28, net mineral N release was 39% of total N added for sawdust, compared with 28–30% for the other bedding materials (Supplementary Table 5). Net N immobilization corresponded to 14% of added mineral N for SF, 16% for chopped straw, and 23% for ground straw, while slight net N mineralization occurred for sawdust (corresponding to 3% of added organic N).
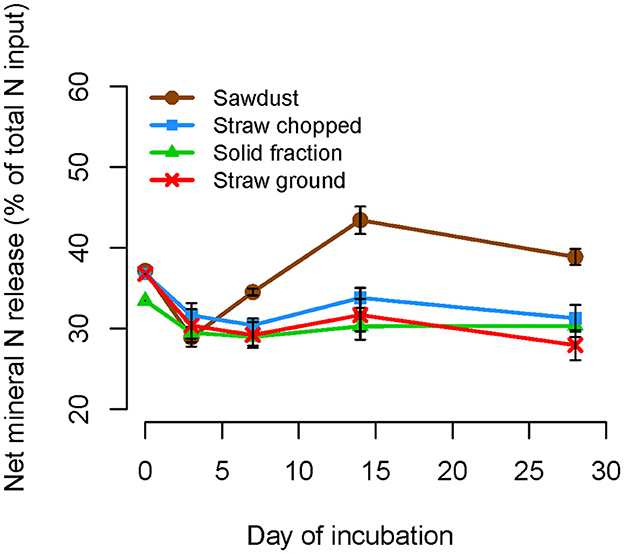
Figure 4. Net mineral N release during soil incubation from experimental slurries with different bedding materials, all with C/N ratio 12. Values at day 0 are the calculated amounts added for each treatment. Error bars represent standard error of the mean (SEM). The one-way ANOVA showed p-values < 0.05 for day 7, 14 and 28.
3.2.2 CO2 production
In the comparison between treatments with four different bedding materials, all with C/N ratio 12, CO2 production (% of C input) at day 2 was highest for SF, followed by ground straw and sawdust, and lowest for chopped straw (p < 0.001; Supplementary Table 6, Figure 5A). From day 5, SF and ground straw had, or tended to have, higher CO2 production per day compared with chopped straw and sawdust (Figure 5A). Accordingly, cumulative CO2 production at day 28 was highest for SF and ground straw (46% of added total C), followed by chopped straw (38%), and lowest for sawdust (33%; p < 0.001; Supplementary Table 7, Figure 5B).
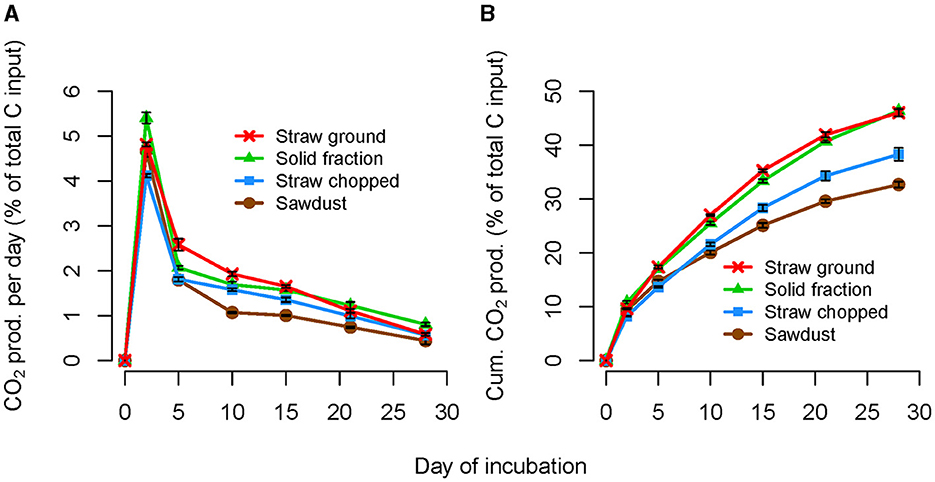
Figure 5. (A) Carbon dioxide production per day and (B) cumulative CO2 production from soil incubation of experimental slurries with different bedding materials, all with C/N ratio 12. Error bars represent standard error of the mean (SEM). In both one-way ANOVA analyses, the p-values were <0.05 for all sampling occasions. Note different scales on Y-axis.
4 Discussion
4.1 C and N turnover during the 1st days of incubation
The high initial CO2 production rate in all treatments was most likely an effect of breakdown of short-chain volatile fatty acids (VFA) from LF, as they are an easily available C source that is quickly consumed during the 1st days of incubation (Kirchmann and Lundvall, 1993). The higher initial CO2 production from slurries with lower C/N ratios (Supplementary Table 5, Figure 3, day 2) can be explained by the smaller amount of “extra” C added with bedding material in the slurry mixes at low C/N ratios (Table 2). Hence, those slurries contained a higher proportion of easily degradable C components, including VFAs.
Slurry with solid fraction had the highest initial CO2 production of the different bedding materials at C/N ratio 12 (Supplementary Table 6, Figure 5A), probably as a result of an already established micro fauna, due to its previous contact with animal feces. Chopped straw had the lowest initial CO2 production, probably owing to its larger particle size and thereby smaller specific surface area compared with sawdust and ground straw. Sawdust had higher initial CO2 production rate than chopped straw despite lower chemical C availability (more lignin), likely as a result of the smaller particle size making the most easily degradable C fractions accessible from the start (Sørensen et al., 1996).
Rapid initial N immobilization, similar to that in this study (Figures 1, 5), has been observed in a previous study which found a strong correlation between N immobilization and initial VFA content of slurries (Kirchmann and Lundvall, 1993). All treatments in the present study contained similar amounts of LF (the main source of VFAs) and showed similar initial N immobilization. This indicates that mineralization/immobilization processes during the 1st days after soil application were mainly driven by the properties of LF, while the added bedding materials had little influence.
4.2 Later C and N mineralization patterns
From day 5, the CO2 production rate followed a slowly declining pattern that continued during the rest of the incubation. During this phase, the most easily available C sources had been depleted and the processes controlling C turnover seemed to be dominated by the properties of the bedding materials added. This was reflected e.g., in sawdust giving the lowest CO2 production through having the highest lignin content, and in higher cumulative CO2 production from ground straw than chopped straw. Higher CO2 production from ground wheat straw compared with chopped straw during the 1st weeks after soil application was also observed by Angers and Recous (1997), and was hypothesized to be a result of higher N accessibility with finer particles. In studies with low C/N ratio amendments such as rye residue (Angers and Recous, 1997) and pea residue (Jensen, 1994), where N content in the residues was less limiting for decomposition, the opposite relationship was seen. This was attributed to more intimate association with soil mineral particles for the ground residues, leading to physical protection of C in microbial biomass and by-products. In the present study, CO2 production rate (averaged over C/N ratio 10–14) was consistently higher from SF compared with chopped straw, despite similar fiber composition, which was likely a combined effect of differences in particle size and degradation by microorganisms, as discussed in the section above for initial CO2 production. For nitrogen, the decline in net mineral N release at C/N ratios 8, 10, and 12 from day 14 to day 28 (Figure 1A) could potentially be explained by re-immobilization of mineralized N and/or gaseous N losses through denitrification (not measured).
4.3 Nitrogen turnover and slurry C/N ratio
The negative linear relationship between C/N ratio and net mineral N release (Figure 2) is in line with hypothesis 3. The slope of the relationship was anticipated to be steeper with chopped straw than SF, based on the assumption that the straw contained more un-degraded and easily available C, resulting in greater N immobilization. This was not the case, and analysis of the fiber fraction revealed very similar content of hemicellulose, cellulose, and lignin in SF and straw (Table 1). The tendency for a steeper slope of the relationship for SF at day 14 (Figure 2A) is likely because microorganisms were already integrated with that material, whereas the straw had to be colonized, slowing down the straw degradation process and hence the N demand and immobilization. In addition, chopped straw had larger particle size, making the C less easy to access, which likely also delayed the degradation process (Sørensen et al., 1996).
A negative relationship between C/N ratio and N availability has been reported previously, for different organic fertilizers by Delin et al. (2012) and for cattle slurry by Sørensen et al. (2003). Sørensen et al. (2003) found that N availability was positively correlated with ammonium N content of the slurry, but in the present study the ammonium N content was within a narrow range (Table 2) and the relationship could not be evaluated. Norris et al. (2019) found that prediction of N supply from slurries could be improved by considering the ratio of total C to water-extractable N (rather than total N), but that considering more labile C fractions rather than total C weakened the predictions. This suggests that the amount of bioavailable N in organic material has a stronger influence on N mineralization/immobilization processes than amount of bioavailable C.
4.4 Bedding material particle size
The hypothesis that higher C availability due to smaller particle size would increase N immobilization was neither supported nor refuted. Ground straw showed higher cumulative CO2 production than chopped straw (Figure 5) and a tendency for greater net N immobilization (Figure 4), but the difference in net mineral N release was not significant. However, on comparing ground and chopped barley residues added to soil, Ambus and Jensen (1997) found greater N immobilization with finer particle size after 2 weeks, and the difference remained throughout their 60-day incubation. Microbial growth was similar regardless of residue particle size in that study, leading to the conclusion that despite grinding, some C in the residues was still physically protected by lignin. In the present study, there were two different interacting sources of added C (LF and bedding material), which makes the results more difficult to interpret.
4.5 Relationship between C and N turnover
After 28 days, net mineral N release was similar for SF and chopped straw, despite differences in CO2 production. In a study by Jensen et al. (2005), net N mineralization from a wide variety of mostly low-lignified plant materials was most closely correlated with concentrations of total plant N and neutral-detergent soluble N. In the same study, C mineralization was most closely correlated with holocellulose (hemicellulose + cellulose) content, which is in line with our observation that sawdust had both the lowest cumulative CO2 production and the lowest holocellulose content. According to Chen et al. (2018), cellulose is rapidly degraded after soil incorporation of straw, while lignin is not broken down, but accumulates in the soil.
With regard to our three starting hypotheses, the results showed that: (1) C availability (as indicated by cumulative CO2 production) depended on a combination of bedding material particle size, fiber composition, and probably previous degradation by microorganisms; (2) for bedding materials with a relatively low degree of lignification (i.e., straw and solid fraction) there was no relationship between C and N availability, while for sawdust, with higher lignin content, high net mineral N release was associated with low CO2 production; and (3) for both SF and chopped straw, there was a negative linear relationship between slurry C/N ratio and net mineral N release, with similar slope for both bedding materials. The contrasting results for sawdust, with substantially higher net mineral N release than from the other bedding materials, show that in addition to slurry C/N ratio, bedding material fiber composition is an important factor affecting slurry N availability.
4.6 Practical and environmental implications
The results indicate that bedding materials with similar fiber composition (i.e., SF and straw) give slurries with similar short term fertilizer value, when applied in amounts resulting in the same slurry C/N ratio. Applied at farm scale, this means that since SF has a lower C/N ratio than straw (Table 1), addition of similar amounts of SF as with straw would result in a lower slurry C/N ratio and hence potentially in higher N availability. The results also indicate that sawdust as bedding material could result in higher N availability, provided that the amount used gives the same slurry C/N ratio as with other bedding materials. This study confirms the relationship between C/N ratio and N availability, suggesting that slurry C/N ratio can be used as a predictor for N use efficiency in crop production (Delin et al., 2012). The results indicate that a higher N use efficiency can be expected from slurry containing sawdust, compared with SF and straw.
The environmental effects of slurry separation are more complex and difficult to predict. For the liquid fraction, the lower DM content increases the infiltration rate and hence reduces ammonia emissions after slurry application (Pedersen et al., 2022). When applied in spring or summer, the lower C/N ratio and thereby increased N availability can also improve the synchronization between N supply and crop N uptake. This potentially reduces the risk of mineral N surplus in autumn and subsequent losses during winter, when crop N uptake is low or no crop is present to take up the nitrogen (Delin and Stenberg, 2021; Sørensen et al., 2023). Simultaneous application of easily available carbon and nitrogen, as with all animal slurries, may stimulate denitrification and increase the risk of nitrous oxide emissions (Senbayram et al., 2012). For soil application of the liquid fraction from separated slurries and digestates, studies have shown both similar (Holly et al., 2017) and higher (Fangueiro et al., 2008b; Meng et al., 2022) nitrous oxide emissions, compared with raw slurries.
With covered storage of the liquid fraction, reducing the ammonia emissions, the overall environmental effect of slurry separation is largely dependent on how the solid fraction is treated during storage. With composting of the solid fraction, the total ammonia emissions are increased, while greenhouse gas emissions are reduced (Amon et al., 2006; Fangueiro et al., 2008a). Storage of the solid fraction without adding oxygen by turning, could either increase (Holly et al., 2017) or reduce (Hansen et al., 2006) the emissions of nitrous oxide. A system where the solid fraction is used as bedding material is probably most comparable with composting, with good oxygen supply and hence increased ammonia emissions. However, an evaluation of that system would need to include a comparison of the in-house ammonia emissions with other types of bedding materials.
5 Conclusions
There was a negative linear relationship between slurry C/N ratio and net mineral N release after 28 days, with similar slope of the regression line for the bedding materials straw and solid fraction. Differences in C availability between these two bedding materials, as indicated by cumulative CO2 production, did not affect N availability. The particle size of straw used as bedding material also had no effect. However, the relationship for sawdust was different, with higher net mineral N release than from SF and straw, in combination with lower CO2 production, indicating limited C availability due to high lignin content. Therefore, if farmers use C/N ratio as an indicator of short term N availability in slurry, the same relationship can be used for calculation regardless of whether straw or SF is used as bedding material and regardless of particle size of the straw. If the same amount of bedding material is added, SF would result in a lower slurry C/N ratio, and hence potentially in higher N availability. If sawdust is used, N availability may be 24–39% higher at similar C/N ratio.
Data availability statement
The raw data supporting the conclusions of this article will be made available by the authors, without undue reservation.
Author contributions
KA: Conceptualization, Formal analysis, Investigation, Resources, Visualization, Writing – original draft, Writing – review & editing. AD: Formal analysis, Resources, Writing – review & editing. PS: Conceptualization, Writing – review & editing. SD: Conceptualization, Investigation, Resources, Writing – review & editing.
Funding
The author(s) declare that financial support was received for the research, authorship, and/or publication of this article. The work has been funded by Region Västra Götaland, Grant Number: RUN 2021-00020.
Acknowledgments
The authors thank Bo Stenberg for acquiring financial support for the project and for taking part in the conceptualization. Thanks also to Stina Larsson for practical assistance with the incubations.
Conflict of interest
The authors declare that the research was conducted in the absence of any commercial or financial relationships that could be construed as a potential conflict of interest.
Publisher's note
All claims expressed in this article are solely those of the authors and do not necessarily represent those of their affiliated organizations, or those of the publisher, the editors and the reviewers. Any product that may be evaluated in this article, or claim that may be made by its manufacturer, is not guaranteed or endorsed by the publisher.
Supplementary material
The Supplementary Material for this article can be found online at: https://www.frontiersin.org/articles/10.3389/fsufs.2024.1393674/full#supplementary-material
Footnotes
1. ^Andersson, K., and Delin, S. (unpublished). Nitrogen Efficiency of Cattle Slurry Depending on Slurry Treatment and Application Method in Different Crops.
References
Agriwise (2023). Agriwise-Smart kalkylering. Available online at: https://www.agriwise.se/web (accessed June 14, 2024).
Ambus, P., and Jensen, E. S. (1997). Nitrogen mineralization and denitrification as influenced by crop residue particle size. Plant Soil 197, 261–270. doi: 10.1023/A:1004276631914
Amon, B., Kryvoruchko, V., Amon, T., and Zechmeister-Boltenstern, S. (2006). Methane, nitrous oxide and ammonia emissions during storage and after application of dairy cattle slurry and influence of slurry treatment. Agricult. Ecosyst. Environ. 112, 153–162. doi: 10.1016/j.agee.2005.08.030
Andersson, E., Frostgård, G., Hjelm, E., Kvarmo, P., Stenberg, M., and Malgeryd, J. (2023). “Rekommendationer för gödsling och kalkning 2024,” in ed. E. Andersson (Jordbruksinformation 17–2023. Swedish Board of Agriculture, Jönköping). Available online at: https://webbutiken.jordbruksverket.se/sv/artiklar/jo219.html (accessed June 17, 2024).
Angers, D. A., and Recous, S. (1997). Decomposition of wheat straw and rye residues as affected by particle size. Plant Soil 189, 197–203. doi: 10.1023/A:1004207219678
Chen, X., Hu, Y., Feng, S., Rui, Y., Zhang, Z., He, H., et al. (2018). Lignin and cellulose dynamics with straw incorporation in two contrasting cropping soils. Sci. Rep. 8:1633. doi: 10.1038/s41598-018-20134-5
Delin, S., Stenberg, B., Nyberg, A., and Brohede, L. (2012). Potential methods for estimating nitrogen fertilizer value of organic residues. Soil Use Manag. 28, 283–291. doi: 10.1111/j.1475-2743.2012.00417.x
Delin, S., and Stenberg, M. (2021). Effects on nitrate leaching of the timing of cattle slurry application to leys. Soil Use Manag. 37, 436–448. doi: 10.1111/sum.12595
Fangueiro, D., Coutinho, J., Borges, L., and Vasconcelos, E. (2015). Recovery efficiency of nitrogen from liquid and solid fractions of pig slurry obtained using different separation technologies. J. Plant Nutr. Soil Sci. 178, 229–236. doi: 10.1002/jpln.201400261
Fangueiro, D., Coutinho, J., Chadwick, D., Moreira, N., and Trindade, H. (2008a). Effect of cattle slurry separation on greenhouse gas and ammonia emissions during storage. J. Environ. Qual. 37, 2322–2331. doi: 10.2134/jeq2007.0330
Fangueiro, D., Pereira, J., Chadwick, D., Coutinho, J., Moreira, N., and Trindade, H. (2008b). Laboratory assessment of the effect of cattle slurry pre-treatment on organic N degradation after soil application and N2O and N2 emissions. Nutr. Cycl. Agroecosyst. 80, 107–120. doi: 10.1007/s10705-007-9124-4
Fangueiro, D., Surgy, S., Coutinho, J., and Vasconcelos, E. (2013). Impact of cattle slurry acidification on carbon and nitrogen dynamics during storage and after soil incorporation. J. Plant Nutr. Soil Sci. 176, 540–550. doi: 10.1002/jpln.201200117
Ferraz, P. F. P., Ferraz, G. A. E. S., Leso, L., Klopčič, M., Barbari, M., and Rossi, G. (2020). Properties of conventional and alternative bedding materials for dairy cattle. J. Dairy Sci. 103, 8661–8674. doi: 10.3168/jds.2020-18318
Frondelius, L., Lindeberg, H., and Pastel, M. (2020). Recycled manure solids as a bedding material: udder health, cleanliness and integument alterations of dairy cows in mattress stalls. Agricult. Food Sci. 29, 420–431. doi: 10.23986/afsci.95603
Hansen, M. N., Henriksen, K., and Sommer, S. G. (2006). Observations of production and emission of greenhouse gases and ammonia during storage of solids separated from pig slurry: effects of covering. Atmos. Environ. 40, 4172–4181. doi: 10.1016/j.atmosenv.2006.02.013
Hjorth, M., Christensen, K. V., Christensen, M. L., and Sommer, S. G. (2010). Solid-liquid separation of animal slurry in theory and practice. A review. Agron. Sustain. Dev. 30, 153–180. doi: 10.1051/agro/2009010
Holly, M. A., Larson, R. A., Powell, J. M., Ruark, M. D., and Aguirre-Villegas, H. (2017). Greenhouse gas and ammonia emissions from digested and separated dairy manure during storage and after land application. Agricult. Ecosyst. Environ. 239, 410–419. doi: 10.1016/j.agee.2017.02.007
Husfeldt, A. W., Endres, M. I., Salfer, J. A., and Janni, K. A. (2012). Management and characteristics of recycled manure solids used for bedding in Midwest freestall dairy herds. J. Dairy Sci. 95, 2195–2203. doi: 10.3168/jds.2011-5105
Jansson, S. L. (1958). Tracer studies on nitrogen transformations in soil with special attention to mineralization-immobilization relationships. Kungliga Lantbrukshogskolans Annaler 24, 101–361.
Jensen, E. S. (1994). Mineralization-immobilization of nitrogen in soil amended with low C:N ratio plant residues with different particle sizes. Soil Biol. Biochem. 26, 519–521. doi: 10.1016/0038-0717(94)90185-6
Jensen, L. S., Salo, T., Palmason, F., Breland, T. A., Henriksen, T. M., Stenberg, B., et al. (2005). Influence of biochemical quality on C and N mineralisation from a broad variety of plant materials in soil. Plant Soil 273, 307–326. doi: 10.1007/s11104-004-8128-y
Kirchmann, H., and Lundvall, A. (1993). Relationship between N immobilization and volatile fatty acids in soil after application of pig and cattle slurry. Biol. Fertil. Soils 15, 161–164. doi: 10.1007/BF00361605
Leach, K. A., Charlton, G. L., Green, M. J., Lima, E., Gibbons, J., Black, D., et al. (2022). Bedding system influences lying behaviour in dairy cows. Vet. Rec. 190:e1066. doi: 10.1002/vetr.1066
Lukehurst, C. T., Frost, P., and Al Seadi, T. (2010). Utilisation of Digestate From Biogas Plants as Biofertilizer. IEA Bioenergy. Available online at: https://www.ieabioenergy.com/blog/publications/utilisation-of-digestate-from-biogas-plants-as-biofertiliser/ (accessed June 17, 2024).
Lybæk, R., and Kjær, T. (2019). Pre-assessment of the circular economic benefits and challenges of biogas production in Denmark when utilizing sand bedding in dairy cow stables. J. Clean. Product. 219, 268–277. doi: 10.1016/j.jclepro.2019.01.241
Meng, X., Ma, C., and Petersen, S. O. (2022). Sensitive control of N2O emissions and microbial community dynamics by organic fertilizer and soil interactions. Biol. Fertil. Soils 58, 771–788. doi: 10.1007/s00374-022-01662-9
Möller, K., and Müller, T. (2012). Effects of anaerobic digestion on digestate nutrient availability and crop growth: a review. Eng. Life Sci. 12, 242–257. doi: 10.1002/elsc.201100085
Norris, M., Johnstone, P. R., Dexter, M. M., Selbie, D. R., Houlbrooke, D. J., Sharp, J. M., et al. (2019). Predicting nitrogen supply from dairy effluent applied to contrasting soil types. N. Zeal. J. Agricult. Res. 62, 438–456. doi: 10.1080/00288233.2018.1508478
Pedersen, J., Hafner, S. D., and Adamsen, A. P. S. (2022). Effectiveness of mechanical separation for reducing ammonia loss from field-applied slurry: assessment through literature review and model calculations. J. Environ. Manag. 323:116196. doi: 10.1016/j.jenvman.2022.116196
Powell, J. M., Wattiaux, M. A., Broderick, G. A., Moreira, V. R., and Casler, M. D. (2006). Dairy diet impacts on fecal chemical properties and nitrogen cycling in soils. Soil Sci. Soc. Am. J. 70, 786–794. doi: 10.2136/sssaj2005.0286
Senbayram, M., Chen, R., Budai, A., Bakken, L., and Dittert, K. (2012). N2O emission and the N2O/(N2O+N2) product ratio of denitrification as controlled by available carbon substrates and nitrate concentrations. Agricult. Ecosyst. Environ. 147, 4–12. doi: 10.1016/j.agee.2011.06.022
Smirnova, N., Demyan, M. S., Rasche, F., Cadisch, G., and Muller, T. (2014). Calibration of CO2 trapping in alkaline solutions during soil incubation at varying temperatures using a respicond VI. Open J. Soil Sci. 4:7. doi: 10.4236/ojss.2014.45019
Sørensen, P. (1998). Effects of storage time and straw content of cattle slurry on the mineralization of nitrogen and carbon in soil. Biol. Fertil. Soils 27, 85–91. doi: 10.1007/s003740050404
Sørensen, P., Ladd, J. N., and Amato, M. (1996). Microbial assimilation of C-14 of ground and unground plant materials decomposing in a loamy sand and a clay soil. Soil Biol. Biochem. 28, 1425–1434. doi: 10.1016/S0038-0717(96)00150-2
Sørensen, P., Pedersen, B. N., Thomsen, I. K., Eriksen, J., and Christensen, B. T. (2023). Plant availability and leaching of 15N-labelled mineral fertilizer residues retained in agricultural soil for 25 years: a lysimeter study. J. Plant Nutr. Soil Sci. 186, 441–450. doi: 10.1002/jpln.202200288
Sørensen, P., Weisbjerg, M. R., and Lund, P. (2003). Dietary effects on the composition and plant utilization of nitrogen in dairy cattle manure. J. Agricult. Sci. 141, 79–91. doi: 10.1017/S0021859603003368
Swedish Board of Agriculture (2021). Rules and General Guidance (SJVFS 2004:62) on Environmental Concern in Agriculture as Regards Plant Nutrients. Jönköping: Swedish Board of Agriculture.
Van Soest, P. J., Robertson, J. B., and Lewis, B. A. (1991). Methods for dietary fiber, neutral detergent fiber, and nonstarch polysaccharides in relation to animal nutrition. J. Dairy Sci. 74, 3583–3597. doi: 10.3168/jds.S0022-0302(91)78551-2
Växa Sverige (2024). Cattle Statistics 2024. Jönköping. Available online at: www.vxa.se/statistik (accessed June 17, 2024).
Webb, J., Sørensen, P., Velthof, G., Amon, B., Pinto, M., Rodhe, L., et al. (2013). “Chapter seven—an assessment of the variation of manure nitrogen efficiency throughout Europe and an appraisal of means to increase manure-N efficiency,” in Advances in Agronomy, ed. D. L. Sparks (Cambridge, MA: Academic Press), 7.
Keywords: bedding material, solid fraction, straw, sawdust, slurry separation, soil incubation experiment
Citation: Andersson K, Dahlin AS, Sørensen P and Delin S (2024) Bedding material properties and slurry C/N ratio affect the availability of nitrogen in cattle slurry applied to soil. Front. Sustain. Food Syst. 8:1393674. doi: 10.3389/fsufs.2024.1393674
Received: 29 February 2024; Accepted: 02 July 2024;
Published: 26 July 2024.
Edited by:
Harald Menzi, Independent researcher, Bern, SwitzerlandReviewed by:
Elio Dinuccio, University of Turin, ItalyJoachim Deru, Louis Bolk Instituut, Netherlands
Copyright © 2024 Andersson, Dahlin, Sørensen and Delin. This is an open-access article distributed under the terms of the Creative Commons Attribution License (CC BY). The use, distribution or reproduction in other forums is permitted, provided the original author(s) and the copyright owner(s) are credited and that the original publication in this journal is cited, in accordance with accepted academic practice. No use, distribution or reproduction is permitted which does not comply with these terms.
*Correspondence: Karin Andersson, karin.i.andersson@slu.se