- 1College of Agriculture, Yangtze University, Jingzhou, Hubei, China
- 2Rice Research Institute, Anhui Academy of Agricultural Sciences, Hefei, Anhui, China
Reducing greenhouse gas (GHG) emissions and quantifying the carbon footprint (CF) of rice-cropping systems in the context of food security is an important step toward the sustainability of rice production. Exploring the key factors affecting emission reduction in rice production is important to properly evaluate the impact of China’s rice-cropping systems on global climate change. This review provides an overview of the direct and indirect CF in rice-cropping systems; analyzes the influencing factors in terms of rice-based cropping systems, varieties and agronomic practices; and proposes mitigation strategies. Different studies have shown that direct and indirect GHG emissions in rice-based cropping systems accounted for 38.3 to 95.5% and 4.5 to 61.7% of total emissions, respectively. And the CFs of ratoon rice, rice–wheat, rice–maize, rice–rapeseed, and rice–fish systems ranged from 316,9 kg CO2-eq kg−1 to 258,47 kg CO2-eq kg−1, which are lower than that in a double-rice planting system. High-yielding rice, drought-resistant rice, and other hybrids can mitigate GHG emissions from paddy fields by 3.7 ~ 21.5%. Furthermore, organic matter, water, tillage, straw incorporation, conservation tillage, reduced nitrogen fertilizer use, and added biochar and methane inhibitors could reduce emissions. Therefore, through reasonable agronomic measures, variety selection and optimal layout of rice-based rotation systems, the carbon neutral rate of rice production can be improved to help the national carbon sequestration and emission reduction target.
1 Introduction
A series of ecological and environmental problems caused by global warming have become a major challenge that humans must confront (Fu et al., 2015). Global warming is caused by the increased concentration of greenhouse gases (GHGs) in the atmosphere and leads to a phenomenon widely known as the “greenhouse effect,” which directly leads to extreme weather such as droughts, floods, typhoons, and catastrophic precipitation (Hussain et al., 2015). The average surface temperatures will rise by 2.2°C to 3.5°C by the middle of the century if the rate of global warming is not controlled [IPCC (Intergovernmental Panel on Climate Change), 2023]. Climate change is seriously affecting agricultural production. The significant increase in temperatures has seriously contributed to the increased frequency of crop disasters and reduced yields. The three major GHGs that contribute to global climate change are carbon dioxide (CO2), methane (CH4) and nitrous oxide (N2O). World food security and even human survival will face serious challenges if effective measures are not taken to achieve net zero GHG emissions [IPCC (Intergovernmental Panel on Climate Change), 2021]. Therefore, carbon sequestration and emission reduction are widely focused on to mitigate current climate change. According to the IPCC thematic report, the world needs to achieve CO2 emissions neutrality by 2050 and with net zero emissions of all GHGs thereafter. The common plan for world development is to achieve carbon peak and neutrality by 2050. China aims to achieve peak carbon emissions by 2030 and carbon neutrality by 2060 with stronger policies and measures (UNGA-75th 2020).
The carbon footprint (CF) is an indicator used to account for carbon emissions based on the ecological footprint, primarily measuring the degree of pressure on natural resources from human activities over the entire life cycle (Wackernagel and Rees, 1998). Agricultural production is one of the major contributors to carbon emissions, 12% of total anthropogenic emissions (Walling and Vaneeckhaute, 2020). Agricultural CF refers to calculating the sum of GHG emissions and consumption “from cradle to grave” in the agricultural production system based on the life-cycle assessment (LCA) method and evaluation of the impact on climate change in the form of CO2-eq (Wiedmann and Minx, 2008; Yan et al., 2015; Xu et al., 2020). Rice-cropping systems are a vital part of agricultural systems. In the past two decades, total rice production has increased from 160,65 to 208,49 million tons, and the total rice sowing area has increased from 265,07 to 294,50 thousand hectares in China (NBSC 2023), which is largely attributed to the rapid increase in agronomic inputs. Therefore, exploring the CF of rice-cropping systems is essential to mitigate global warming in China. Paddy fields are important sources of agricultural GHG emissions, especially CH4 and N2O emissions, which account for 12 ~ 26% and 7 ~ 11% of the total emissions from global agricultural fields, respectively (IPCC, 2014). Therefore, accounting for the CF of the agricultural production process is essential in China to reduce carbon emissions caused by agricultural activities. This study describes the CF and emission reduction measures of rice-cropping systems in China, with the aim of providing solutions and support for saving energy and reducing emission in rice production.
2 Carbon footprint of Paddy fields
In rice-cropping systems, CF includes indirect emissions from the production, storage, and transportation of various agricultural inputs and direct emissions from paddy fields (Zhou et al., 2023). Specifically, in rice-cropping systems, the sum of CH4 emissions from paddy fields, N2O emissions from nitrogen (N) application, and CO2 emissions from respiration are called direct emissions. Moreover, rice-cropping system indirect emissions refer to GHG emissions resulting from rice production, storage, consumption, waste chains and transportation of agricultural input production, such as human inputs, fertilizers, fuel consumption, and pest and weed control.
2.1 Direct emission of CF of paddy fields
Direct GHG emissions under conventional farming account for 75.7% of total emissions, but those from organic farming account for 90.3% (Arunrat et al., 2021). Of all gases that contribute to the greenhouse effect, N2O is the most destructive to the ozone layer, being 298 times more destructive than CO2 by mass over a 100-year 43 time span (Tian et al., 2020). As of 2016, anthropogenic sources contributed, on average, 43% to the total N2O emission, of which emissions from nitrogen additions in agriculture and other sectors contributed around 70% (Lal et al., 2020). The largest emissions under organic and conventional rice farming are CH4, followed by N2O, accounting for 45 and 10% of the overall GHG emissions of CF, respectively (Arunrat et al., 2021).
Paddy fields are considered an important source of atmospheric CH4. CH4 production by methanogenic bacteria is one of the end products of organic matter mineralization under anaerobic conditions (Qian et al., 2023). Extreme reduction conditions lead to the conversion of organic carbon to CH4 through methanogenesis (Inubushi et al., 2001). The CH4 generated in the soil undergoes dissolved diffusion through the water–air and soil–water interfaces, is lost by the ebullient, transported to the roots by diffusion, converted to gaseous CH4 in the aerenchyma and cortex, and subsequently released to the atmosphere through plant micropores (Hussain et al., 2015; Figure 1). CH4 is the main sources of direct emissions, accounting for 59.7 ~ 85.7% of direct emissions (Qian et al., 2023; Zhen et al., 2023). CH4 contributed more than 60% of the total GHG emissions of the organic, rice–fish coculture, and conventional rice farming systems (Zhen et al., 2023).
N2O production mainly result from microbial nitrogen transformations, that is, mediated by the processes of soil nitrification, denitrification, and heterotrophic reduction of nitrate-nitrogen to ammonium (Kuypers et al., 2018). Long-term flooding of paddy fields results in a unique soil profile that leads to the development of oxidizing and reducing layers within the cultivated layer (Xing et al., 2009). N2O diffuses into the atmosphere mainly from water, plants, and the concentration gradient between soil and water (Figure 2). N2O is released mainly through the soil surface in the absence of floodwater (Yan et al., 2000).
CO2 emissions from paddy fields mainly come from biotic and abiotic processes, and are less than those of CH4 and N2O (Figure 3). Biological processes include the activity of plants and microorganisms in the soil, and abiotic processes are mainly the oxidation of carbon-containing materials in the soil. But in paddy field studies, CO2 emission or C sequestration from soil were not considered because soil organic matter is typically maintained or increased in intensive and irrigated rice production system (Cassman et al., 1995; Bronson et al., 1997). Rice is a C3 plant with low efficiency of CO2 assimilation, especially photorespiration. Soil CO2 emissions are mainly derived from soil respiration. Carbon accumulation in flooded soil results in lower emissions mainly due to poor carbon oxidation (anaerobic) conditions.
2.2 Indirect emission of CF of paddy fields
In recent years, increasing attention has been given to indirect carbon emissions from rice production. Indirect carbon emissions in rice-cropping systems emanate from carbon emitted during the use of fertilizer, electricity and diesel oil, fuel combustion, machinery inputs, labor, irrigation, herbicides, pesticides, seeds, trays, and other material inputs (Adviento-Borbe et al., 2007; Fuentes-Ponce et al., 2022).
Indirect GHG emissions as a percentage of total emissions under rice-based cropping systems range from 4.5 to 61.7% (Tables 1, 2). Indirect emissions under rice-cropping systems in the middle and lower reaches range from 1.86 Mt. CO2-eq to 8.09 Mt. CO2-eq, accounting for 23.8 ~ 34.2% of total emissions (Qin, 2011; Cheng, 2015; Xia, 2019). In the double-season rice and ratoon rice systems, indirect GHGs in the first season accounted for 11.4 ~ 17.3%, and those in the second season accounted for 5.2 ~ 6.8% of the annual total indirect GHGs (Xu et al., 2022). Urea and phosphate fertilizer (to a lesser extent) were the most responsible for increasing indirect emissions (Fuentes-Ponce et al., 2022; Table 3). The indirect emissions of CO2 from agricultural inputs were obviously greater than those from farm operations in each cropping system. Indirect GHG emissions from agriculture inputs under fertilizer, electricity and diesel were higher than other farm inputs (Xue et al., 2016; Jiang et al., 2020; Li et al., 2020; Ling et al., 2021; Huang et al., 2022; Xu et al., 2022). Indirect emissions of GHGs arising from the production of agricultural inputs, fuel combustion, and use of machinery may contribute as much as half of the total GHG emissions (Mosier et al., 2005; Adviento-Borbe et al., 2007). In addition to fertilizers, the share of indirect GHG emissions from herbicides, labor and irrigation in addition to fertilizers was still high in multiple rice-cropping systems (Chen et al., 2020; Ghosh et al., 2022). In addition, under organic and conventional rice farming treatments, conventional cultivation had the highest indirect GHG emissions of herbicides, insecticides and transportation (Arunrat et al., 2021).
3 Influencing factors of the CF of paddy fields
Variations in cropping system, cultivar., tillage type, fertilizer, irrigation, and additive substance among different cropping systems impact soil properties, microbial abundance and activity, and crop growth, leading to differences in carbon emissions (Tables 3, 4).
3.1 Cropping systems
The higher resource use efficiency and lower carbon emission in the optimized rice rotation systems can improve soil aeration and promote microorganism activity, microbial cycling, and retention of carbon and N. Under different rotation systems, Rice–maize or rice–wheat are highly effective strategies for reducing CF and enhancing the net C sink as well as maintaining high grain yield (Janz et al., 2019; Jiang et al., 2020; Huang et al., 2022). The introduction of a rice–maize system into a double-season rice system provides a feasible system to significantly reduce the CF by 35.0 ~ 41.7% (Jiang et al., 2020). One of the reasons for this is that maize, as a C4 crop, is more yielding than C3 crops such as rice and wheat (Huang et al., 2022). On the other hand, aerobic conditions during the growing season of maize and wheat can improve the nutrient availability of the following rice by accelerating soil organic matter mineralization (Jiang et al., 2020; Huang et al., 2022). The rice–wheat rotation showed the highest total CH4 emissions in the rice season, but total N2O emissions were lowest compared to rice–green manure rotation and rice–fallow rotation (Hu et al., 2016; Xu, 2017). The annual CF of rice–wheat rotation is the lowest, followed by rice–shrimp cropping, and the highest is in cold-soaked rice fields (“medium rice-winter soak”) (Xu, 2020). The carbon emissions per unit area and CF per unit yield of the rice season in the spring maize-late rice system were reduced by 496 kg CO2-eq·ha−1 and 0.24 kg CO2-eq·kg−1 compared to the double season rice, respectively (Jiang et al., 2019a,b). Higher agricultural inputs and GHG emissions resulted in a higher global warming potential (GWP) in double-season rice than in ratoon rice. Zhou et al. (2023) compared the three rice systems, and the average annual GWP of double-season rice was 152,66 kg CO2-eq ha−1, which was 104.9 and 70.2% higher than those of middle-season rice and ratoon rice systems, respectively. Similar results were also reported for ratoon rice, which had a lower CF than double-season rice (Qiao, 2019; Sun et al., 2019; Xu et al., 2022). The two experiments demonstrated that ratoon rice had a 27.4 ~ 40.7% lower annual CF than double-season rice (Huang et al., 2022; Xu et al., 2022).
Furthermore, integrated rice and aquatic animal production systems are rapidly and increasingly being developed. Choosing rice–fish, rice–duck and rice–crayfish systems can reduce GHG emissions through biological intercropping with rice (Table 5, 6; Xu, 2017; Ling et al., 2021; Jiang et al., 2022). In different integrated rice-cropping systems, the rice–duck systems increased N2O emissions by 4.2 ~ 5.2% while reducing total CH4 emissions from rice fields by 8.80 ~ 16.68% compared with the conventional cultivation mode (Xu et al., 2017). Given that CH4 emission contributed to 85.83 ~ 96.22% of GWP, the great reduction in CH4 emission led to a significantly lower GWP of the rice–duck systems. Across the two cropping systems, the CF of the rice–fish system was 0.8 times lower than that of the organic rice system (Zhen et al., 2023). In the straw return treatments of the integrated rice–crayfish system, GHG emissions were approximately 7.5% lower than those of the fallow rice system (Ling et al., 2021). The CFs were 141,26 kg CO2-eq·ha−1 and 131,40 kg CO2-eq·ha−1 for single rice and rice–crayfish systems, respectively, and the CF per unit production value and CF per unit nutrient density of the rice–shrimp systems were 81.4 and 49.3% lower than those of single rice, respectively (Jiang et al., 2022). The above phenomenon may be attributed that crayfish or shrimp hiding dig burrows in field, which increases the redox potential of soil and thus decreasing CH4 emission (Ling et al., 2021; Jiang et al., 2022).
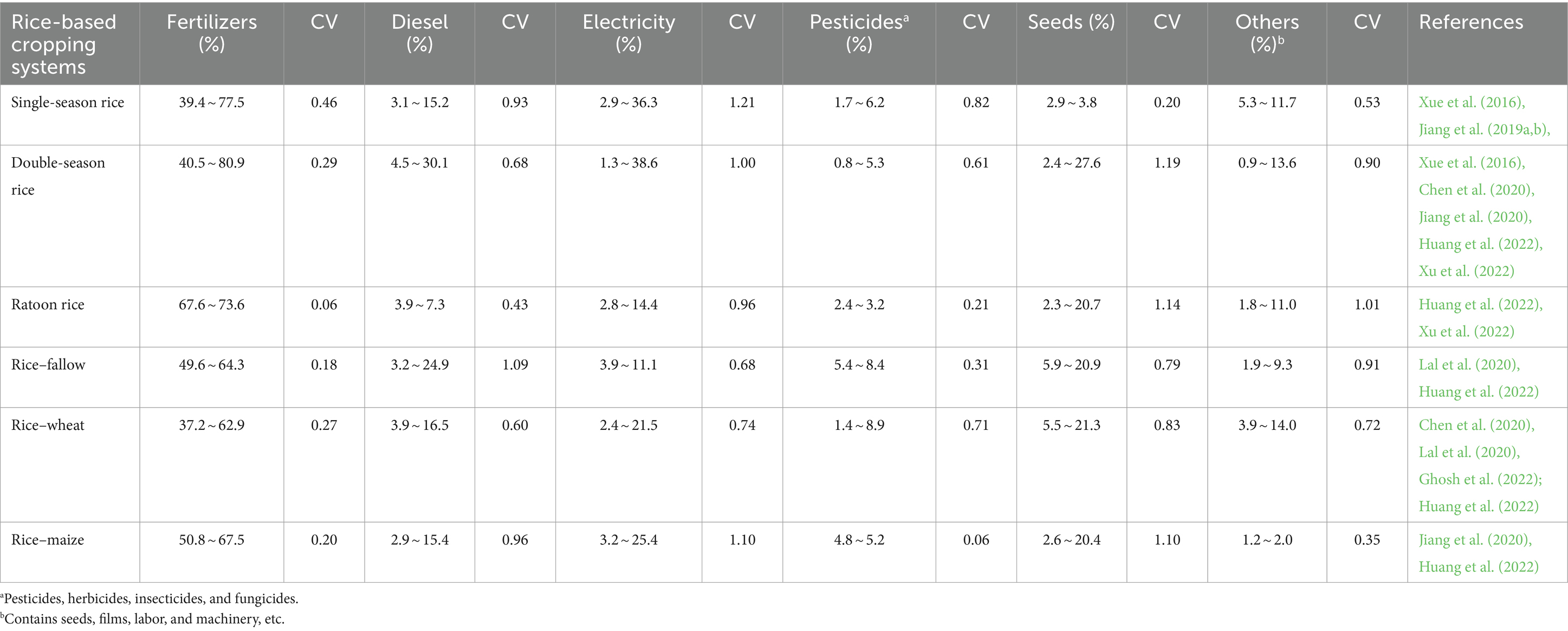
Table 5. Share of source-wise greenhouse gas (GHG) indirect emissions (%) in different rice-based cropping systems.
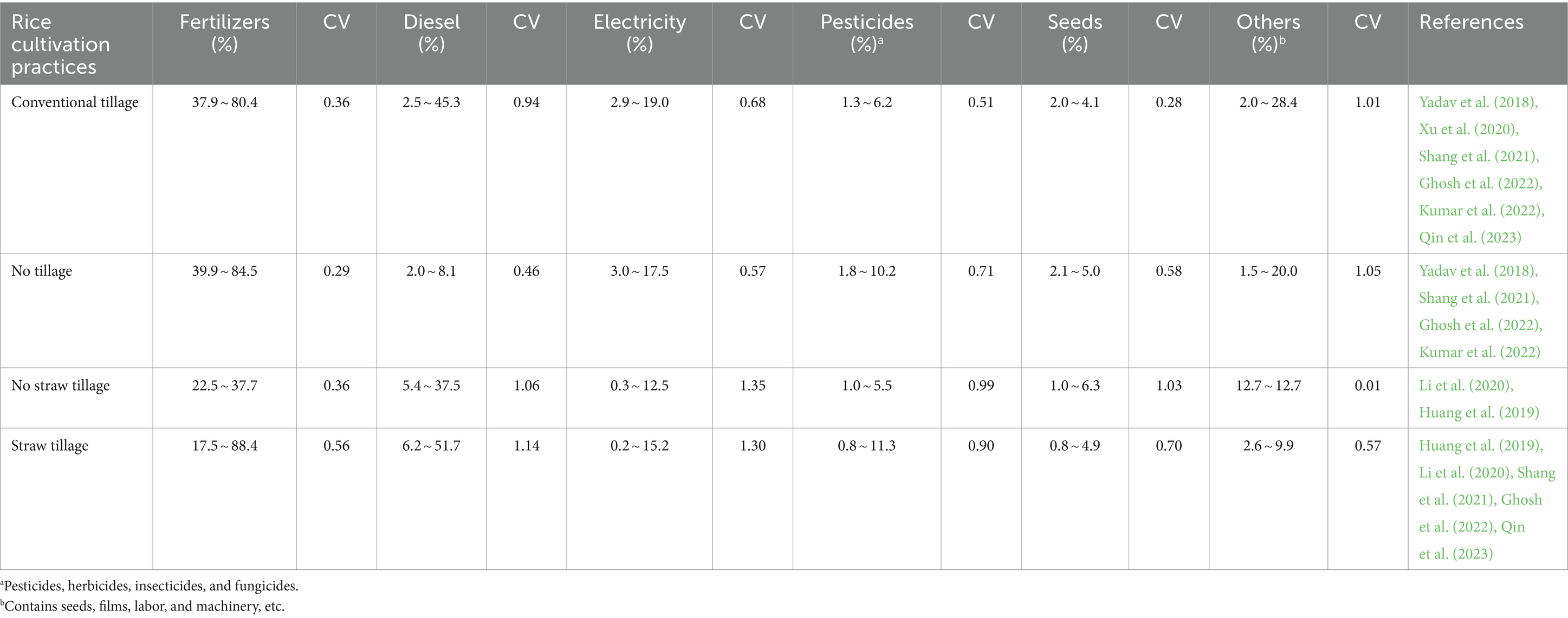
Table 6. Share of source-wise greenhouse gas (GHG) indirect emissions (%) in different rice cultivation practices.
3.2 Rice variety
Rice variety is an important factor affecting GHG emissions, with differences in CH4 and N2O emissions between different rice varieties reaching 6 and 14 times, respectively (Riya et al., 2012). Drought-resistant rice varieties may benefit climate change mitigation and adaptation efforts, because it can reduce GHG emissions by significantly reducing irrigation water use (Luo, 2010; Serraj et al., 2011; Xu et al., 2015). The aerobic rice system saved 14.6 and 19.3% of the CF of rice production over shallow lowland rice and rice intensification systems, respectively (Dash et al., 2022). The drought resistant rice reduced CH4, N2O and CO2 emissions by 21.5, 3.7 and 9.8% compared with the typical variety planted in flooded and wet intermittent irrigation, respectively (Xu et al., 2015). This phenomenon might be partly due to significant differences in the morphological characteristics, amounts of root exudates, microbial communities and plant litter decomposition among different rice varieties (Aulakh et al., 2001; Liechty et al., 2020).
Developing large panicles benefits rice production by increasing yield and produces low CH4 emissions because rice varieties with large panicles may reduce CH4 emissions mainly by controlling CH4 production (Das and Baruah, 2010; Jiang et al., 2016). The more rice tiller number, stems and leaves the plant has the greater the rate of rice plant-mediated transport, thus promoting CH4 emissions. Research has shown that CH4 emissions are positively correlated with the rice plant height, and the CH4 emissions of taller varieties with a plant height of 120 cm are 2.9 times higher than those of shorter varieties with a plant height of 90 cm (Ding et al., 1999). This phenomenon occurs because the oxidizing effect on CH4 is greater than the production and transport effects in high rice plants. Therefore, short-stalked rice varieties are more advantageous than tall-stalked rice varieties for regulating carbon emissions.
Furthermore, high-yielding varieties are in fact also emission-reducing varieties, mainly by changing photosynthetic product allocation to improve the harvest index and reduce the carbon source required by methanogenic bacteria (Chen, 2017). A strongly CO2-responsive cultivar (hybrid rice) was observed to significantly reduce GHG emissions (Yu et al., 2021; Qiu et al., 2023). Higher content of dissolved organic matter, dissolved sugars, NH4-N, and NO3-N in Yongyou 1,540 varieties reduced microbial abundance and CF (Ding et al., 2022). In addition, strongly CO2-responsive rice can distribute photosynthetic products more fully to the roots to increase the C/N, promote the conversion of N fertilizer to microbial N and inhibit N2O from nitrification and denitrification. In terms of response to x [CO2], the strongly CO2-responsive rice cultivar could reduce N2O emissions by 38.3 ~ 41.9% relative to the weakly CO2-responsive cultivar (Yu et al., 2021).
3.3 Agronomic practices
3.3.1 Tillage
In addition to the influence of rice-cropping systems and variety, agronomic practices of soil conditions are more influential. Different tillage practices significantly affect soil respiration and the surface and subsurface microenvironments, leading to changes in soil organic carbon fixation and carbon emissions. Conservative tillage reduces the inefficient evaporation of soil water, thus reducing the irrigation of crop water requirements improving water use efficiency and ultimately reducing carbon emissions (Follett, 2001; Shang et al., 2021; Ghosh et al., 2022). Conservative tillage, which aims at minimize carbon costs and resource use efficiency, has led to significant reduction in total estimated GHG emissions and improved carbon efficiency (Das et al., 2020; Gangopadhyay et al., 2022; Ghosh et al., 2022). The no-till approach resulted in a higher content of macroaggregates, which favored the production of more N2O for denitrification to proceed, and straw incorporation provided more reaction substrate for denitrification, but residual nutrients were released into the atmosphere by burning to CO2 (Freibauer et al., 2004; López-Fando and Pardo, 2011). Dry direct-seeding and transplanting showed significant differences in CH4 emissions and GWP but not N2O emissions compared with wet direct-seeding (Hang, 2015). Tillage destroys the original structure of the soil and accelerates soil disturbance and soil organic matter decomposition, causing changes in soil redox potential and soil moisture, promoting soil carbon emissions, and reducing the oxidation of methane by the soil (Meng et al., 2006).
3.3.2 Fertilizer
Soil CF is greatly influenced by the type and structure of fertilizer application, the amount of fertilizer applied and the mix of different fertilizers. The CF of rice production were positively correlated with N fertilizer rates. Fertilizer is the main sources of indirect emissions, accounting for 41.1 ~ 75.9% of indirect emissions. GHG emissions from fertilizers account for 17.54 ~ 88.39% of indirect emissions in different rice-based cropping systems (Tables 3, 4; Supplementary Tables S1, S2). Fertilizer application indirectly affects gas emissions by influencing soil pH, Eh, temperature and bacterial concentration. Urea application reduces plant residues and soil organic matter and promotes the decomposition of organic matter, while urea itself is gradually hydrolyzed in the soil and inhibits oxidation, increasing emissions (Cai, 2009).
The mitigation of soil CH4 and N2O emissions under urea deep placement decreased the total GHG emissions by 34.0% and the CF by 46.0% (Liou et al., 2003). The application of inorganic N fertilizer significantly promoted rice field emissions, mainly because increasing the soil N content provided a substrate for the nitrification denitrification process and influenced the nitrification denitrification reaction process (Gregorich et al., 2005; Xiang et al., 2007; Ma et al., 2010). Nitrate N had some inhibitory effect on CH4 oxidation capacity, but compared to nitrate N fertilizers, long-term application of ammonium N can reduce CH4 oxidation capacity by tens of times (Liou et al., 2003; Yang et al., 2010). Foliar N fertilization not only reduces fertilizer losses but also reduces CH4 and N2O emissions (Das and Adhya, 2014). The method of fertilizer application and the amount of fertilizer applied also affect the carbon footprint of rice fields. Chemical fertilizer application reduced CH4 emissions when N phosphorus (P), and potassium (K) levels were essentially constant, while additional organic fertilizer application promoted emissions (Nan et al., 2020). The CF of the rice-cropping system varied significantly among fertilizer combinations, and N and K application and N, P, and K application were 2.9 and 38.2% lower than no fertilizer application, respectively (Qin et al., 2023). However, the positive effect of fermented digestate (organic fertilizer) on CH4 emissions from paddy fields was also much lower than that of “fresh” organic fertilizer. Soil CO2 emissions showed a decreasing trend with increasing N application levels in the range of 0 ~ 270 kg N ha−1 (Wilson and Al-Kaisi, 2008). Thus, the timing of fertilizer, N deep placement, balanced inorganic fertilization, foliar N fertilization, nitrate N, and ammonium N can increase grain yield, reduce CF, and enhance the net ecosystem economic benefit from rice fields (Yang et al., 2010; Das and Adhya, 2014; Liu et al., 2020).
3.3.3 Irrigation
Irrigation, as the second largest source of carbon inputs, was approximately 22% of total carbon inputs in crop production in China over 1993–2007 (Cheng et al., 2011). Gas emissions from electricity used for irrigation account for 1.27 ~ 38.64% of total indirect emissions (Tables 3, 4). Continuous irrigation creates an anaerobic environment in paddy soils to promote GHG emissions. The combined greenhouse effects of irrigation practices that conserve water, such as intermittent irrigation and moist irrigation profiles, were only 10% of those under continuous flooding (Jiang et al., 2023; Maris et al., 2015). Nitrification and denitrification processes are directly influenced by soil moisture, and proper water content can promote both nitrification and denitrification. Intermittent irrigation has been shown to be an effective measure to reduce emissions by alternating anaerobic and aerobic environments in paddy fields changing the redox potential of the soil to reduce GHG emissions (Xu et al., 2015). The most striking difference is that alternate wetting and drying irrigation reduced the CF, while continuous flooding irrigation CF showed an increase of 1.86 times (Du et al., 2022). Compared to conventional flooding paddies, maintaining a saturated soil water content and maintaining 80% of the field capacity significantly reduced the CF by 30.7 and 34.7%, respectively (Xu et al., 2020).
3.3.4 Additive substances
There are also many proven farming management practices that can sequester carbon and reduce emissions in rice ecosystems. For example, biochar addition during the rice growing season reduces GHG emissions (Woolf et al., 2010; Xie et al., 2013; Qi et al., 2020). Research findings suggest that the application of biochar in the rice–wheat system significantly decreases CH4 and N2O emissions by 11.2 ~ 17.5% and 19.5 ~ 26.3%, respectively (Wu et al., 2019). The application of biochar to farmland can reduce GHG emissions while cultivating soil carbon pools, thereby improving crop quality and achieving high ecological and environmental benefits (Ge et al., 2020; Qi et al., 2020). In addition, one of the most effective ways to reduce GHG emissions from rice paddies is by using methane inhibitors (essentially humic acid), which accelerate the conversion of soil organic matter to humus, thereby significantly reducing the substrate suppression emissions required for methane formation. The annual accumulation of CH4 in green manure–rice significantly reduced the CF, mainly because green manure reduced the abundance of methanogens by reducing the soil C/N ratio (Zhong et al., 2021). Furthermore, plastic film mulching could also significantly reduce GHG emissions (Berger et al., 2013; Gao et al., 2014).
4 Emission mitigation options in rice-cropping systems
Agricultural carbon sequestration and emission reduction has received a great deal of attention at home and abroad as an important way to effectively mitigate the greenhouse effect. As an important source of GHG emissions, paddy fields pose a serious threat to global warming. Therefore, for the sustainable development of mankind, control of GHG emissions from paddy field rice-cropping systems is urgently needed. Carbon sequestration and reduction could be considered from soil, energy consumption and plants in rice-cropping systems (Figure 4). Evidently, reasonable mitigation measures for direct and indirect emission impact factors are essential.
In the past few decades, China has made fruitful achievements in cropping systems, rotation systems, and integrated cropping systems, which are prerequisites for climate change mitigation in agriculture. To reduce farmland emissions by adjusting the cropping structure, reducing the proportion of winter fallow fields and increasing the proportion of straw returned to the field are practical and feasible technical ways to further improve the carbon sequestration potential of rice-cropping systems. The ratoon rice exhibited the lowest GHG intensity among the single-season and double-season rice, which showed that ratoon rice is a cropping system with relatively high yield and low GHG emissions (Zhou et al., 2023). Considering the reduction in inputs and GHG emissions but the high economic efficiency of ratoon rice systems, ratoon rice is recommended to grow in the region where thermal energy is more than that required for planting single-season rice but not enough for planting double-season rice (Qiao, 2019; Xu et al., 2022; Zhou et al., 2023). In addition, the replacement of rice–follow with rice–maize, rice–wheat, rice–fish, rice–duck or rice–crayfish are highly effective strategies for reducing CF as well as maintaining high grain yield (Janz et al., 2019; Jiang et al., 2020; Huang et al., 2022).
Innovative varieties for rice have been developed to conserve nutrients, energy, and water to achieve sustainable yields and mitigate GHG emissions. Noteworthy, for the short-term reduction of GHG emissions, aerobic rice, large panicle size rice and drought resistant rice varieties are good options (Luo, 2010; Serraj et al., 2011; Xu et al., 2015; Yun et al., 2019; Dash et al., 2022). In addition, hybrid rice is great option, for instance strongly CO2-responsive cultivars and high-yielding varieties (Qiu et al., 2023). Therefore, mastering the characteristics and development trend of variety renewal and technology improvement can provide a scientific basis for high-yielding low-carbon rice crop technology innovation.
Low-carbon rice management techniques such as no-till, conservation tillage rice, deep application of N fertilizer, residue retention and intermittent water-saving irrigation can enhance the soil agglomeration structure to reduce CF. Determining the fertilizer application ratio according to the growth needs of rice combined and delaying the application period of N fertilizer can also reduce N2O emissions, while the reduction of GHG emissions from rice fields can also be achieved by applying controlled release fertilizers and additives. In addition, nitrate N, urea N and slow-release fertilizers with high N utilization and emission reduction should be selected, and techniques such as off-root fertilization should be used to reduce GHG emissions due to soil microbial activity (Freibauer et al., 2004; López-Fando and Pardo, 2011; Hang, 2015). In terms of irrigation, the use of intermittent irrigation and moist irrigation profiles, can also reduce the CF (Jiang et al., 2023; Maris et al., 2015). In addition, biochar, methane inhibitors and mulching plastic film can also alleviate GHG emissions (Woolf et al., 2010; Xie et al., 2013; Qi et al., 2020; Zhong et al., 2021).
In addition to reducing emissions in terms of cropping systems, variety, and agronomic practices, emission reduction measures can also be considered in terms of indirect emissions. Indirect emissions from electricity for irrigation and labor inputs are just behind fertilizer inputs (29.2 ~ 41.1%), accounting for 16.3 ~ 33.9% and 21.0 ~ 29.3% of indirect emissions, respectively (Chen et al., 2020). Therefore, decreased GHG emissions from the rice-cropping system can be selecting varieties, reducing the use of fertilizers, especially nitrogen fertilizer, irrigation water and tillage.
5 Conclusion and prospects
The CF is influenced by the rice-based cropping systems, varieties, tillage methods, fertilizer types, irrigation conditions, and added emission reduction materials of the rice crop systems. Emissions can be reduced in rice-cropping systems by implementing the following six strategies: (1) choose ratoon rice, rice–maize, rice–wheat, rice–fish, rice–duck, and rice–crayfish cropping systems; (2) choose aerobic rice, large panicle size rice, drought-resistant rice, and the strongly CO2-responsive rice cultivar; (3) choose deep N placement, balanced inorganic fertilization, nitrate N, ammonium N, and slow-release fertilizers; (4) choose no-till, wetting and drying irrigation, and intermittent irrigation methods; (5) add methane inhibitors and mulching plastic film; and (6) reduce farm machinery fuel consumption, electrical energy, labor inputs and disease control inputs. However, farmers are currently focusing on economic efficiency, so future research will focus on how to reduce GHG emissions while ensuring profitability for different rice-cropping systems. Additionally, understanding the social drivers of GHG emissions in rice-cropping systems is one of the future research directions.
Data availability statement
All data analyses were performed with licensed software, and generated or analyzed during this study are included in this published article.
Author contributions
YJ: Conceptualization, Data curation, Formal analysis, Investigation, Methodology, Software, Visualization, Writing – original draft, Writing – review & editing. YZ: Conceptualization, Funding acquisition, Supervision, Writing – review & editing. ZL: Conceptualization, Supervision, Writing – review & editing. KF: Supervision, Writing – review & editing. XS: Supervision, Writing – review & editing. YX: Funding acquisition, Supervision, Writing – review & editing. WW: Data curation, Funding acquisition, Methodology, Project administration, Supervision, Visualization, Writing – review & editing. HZ: Data curation, Methodology, Project administration, Supervision, Visualization, Writing – review & editing.
Funding
The author(s) declare that financial support was received for the research, authorship, and/or publication of this article. This work was supported by the National Key Research and Development Program of China (2022YFD2301402), the Young Talents Program of Anhui Academy of Agricultural Sciences (QNYC-201904), and the Discipline Leading Talents Program of Anhui Academy of Agricultural Sciences (LJRC-202102).
Acknowledgments
The authors wish to thank the reviewers, whose contributions significantly improved the quality of the paper, and Min Xi and Yan Zhou for their technological support.
Conflict of interest
The authors declare that the research was conducted in the absence of any commercial or financial relationships that could be construed as a potential conflict of interest.
Publisher’s note
All claims expressed in this article are solely those of the authors and do not necessarily represent those of their affiliated organizations, or those of the publisher, the editors and the reviewers. Any product that may be evaluated in this article, or claim that may be made by its manufacturer, is not guaranteed or endorsed by the publisher.
Supplementary material
The Supplementary material for this article can be found online at: https://www.frontiersin.org/articles/10.3389/fsufs.2024.1375092/full#supplementary-material
References
Adviento-Borbe, M., Haddix, M., Binder, D., Walters, D., and Dobermann, A. (2007). A soil greenhouse gas fluxes and global warming potential in four high-yielding maize systems. Glob. Chang. Biol. 13, 1972–1988. doi: 10.1111/j.1365-2486.2007.01421.x
Alam, M. A., Huang, J., Khan, M. N., Daba, N. A., Zhang, L., Shen, Z., et al. (2023). Effects of long-term organic and inorganic fertilization on greenhouse gas emissions and soil nutrient stoichiometry in a rice-rice-fallow cropping system. Agric. Ecosyst. Environ. 357:108695. doi: 10.1016/j.agee.2023.108695
Arunrat, N., Sereenonchai, S., and Wang, C. (2021). Carbon footprint and predicting the impact of climate change on carbon sequestration ecosystem services of organic rice farming and conventional rice farming: a case study in Phichit province Thailand. J. Environ. Manag. 289:112458. doi: 10.1016/j.jenvman.2021.112458
Aulakh, M. S., Wassmann, R., Bueno, C., Kreuzwieser, J., and Rennenberg, H. (2001). Characterization of root exudates at different growth stages of ten rice (Oryza sativa L.) cultivars. Plant Biol. 3, 139–148. doi: 10.1055/s-2001-12905
Bakhshandeh, E., Jamali, M., Emadi, M., and Francaviglia, R. (2022). Greenhouse gas emissions and financial analysis of rice paddy production scenarios in northern Iran. Agric. Water Manag. 272:107863. doi: 10.1016/j.agwat.2022.107863
Berger, S., Kim, Y., Kettering, J., and Gebauer, G. (2013). Plastic mulching in agriculture-friend or foe of N2O emissions? Agric. Ecosyst. Environ. 167, 43–51. doi: 10.1016/j.agee.2013.01.010
Bronson, K. F., Cassman, K. G., Wassmann, R., Olk, D. C., van Noordwijk, M., and Garrity, D. P. (1997). “Soil carbon dynamics in different cropping systems in principal ecoregions of Asia” in Management of Carbon Sequestration in soil. eds. R. Lal, J. M. Kimble, R. F. Follett, and B. A. Stewart (Boca Raton: CRC Press).
Cai, Z. C. (2009). Methane and nitrous oxide emissions from rice-based ecosystems. first Edn. China (in Chinese with English abstract): University of Science and Technology of China Press.
Cassman, K. G., De Datta, S. K., Olk, D. C., Alcantara, J. M., Samson, M. I., Descalsota, J. P., et al. (1995). “Yield decline and the nitrogen economy of long-term experiments on continuous, irrigated rice systems in the tropics” in Soil management: Experimental basis for sustainability and environmental quality. eds. R. Lal and B. A. Stewart (Boca Raton: Lewis/CRC Publishers).
Chen, Z.D. (2017) Farming practices on carbon sequestration and emission mitigation in double rice field and adoption behavior of low carbon technology by rice farmers. Thesis, Beijing, China, College of agriculture and biotechnology China agricultural university
Chen, Z. D., Xu, C. C., Ji, L., Feng, J. F., Li, F. B., Zhou, X. Y., et al. (2020). Effects of multi-cropping system on temporal and spatial distribution of carbon and nitrogen footprint of major crops in China. Glob. Ecol. Conserv. 22:e00895. doi: 10.1016/j.gecco.2019.e00895
Cheng, C. (2015). Effects of water-saving irrigation and conservation tillage on cropping growth and greenhouse gases emissions in double rice-cropping systems. Jiang Xi, China: Thesis. Jiang Xi agricultural university.
Cheng, K., Pan, G. X., Smith, P., Luo, T., Li, L. Q., Zheng, J. W., et al. (2011). Carbon footprint of China’s crop production—an estimation using agro-statistics data over 1993-2007. Agric. Ecosyst. Environ. 142, 231–237. doi: 10.1016/j.agee.2011.05.012
Das, S., and Adhya, T. K. (2014). Effect of combine application of organic manure and inorganic fertilizer on methane and nitrous oxide emissions from a tropical flooded soil planted to rice. Geoderma 213, 185–192. doi: 10.1016/j.geoderma.2013.08.011
Das, K., and Baruah, K. K. (2010). Methane emission associated with anatomical and morphophysiological characteristics of rice (Oryza sativa) plant. Physiol. Plant. 134, 303–312. doi: 10.1111/j.1399-3054.2008.01137
Das, T. K., Nath, C. P., Das, S., Biswas, S., Bhattacharyya, R., Sudhishri, S., et al. (2020). Conservation agriculture in rice-mustard cropping system for five years: impacts on crop productivity, profitability, water-use efficiency, and soil properties. Field Crop Res. 250:107781. doi: 10.1016/j.fcr.2020.107781
Dash, P. K., Bhattacharyya, P., Padhy, S. R., Nauak, A. K., Poonam, A., and Mohanty, S. (2022). Life cycle greenhouse gases emission from five contrasting rice production systems in the tropics. Pedosphere 33, 960–971. doi: 10.1016/j.pedsph.2022.11.001
Ding, H., Hu, Q. Y., Cai, M. L., Cao, C. G., and Jiang, Y. (2022). Effect of dissolved organic matter (DOM) on greenhouse gas emissions in rice varieties. Agric. Ecosyst. Environ. 330:107870. doi: 10.1016/j.agee.2022.107870
Ding, A., Willis, C. R., Sass, R. L., and Fisher, F. M. (1999). Methane emissions from rice fields: effect of plant height among several rice cultivars. Glob. Biogeochem. Cycles 13, 1045–1052. doi: 10.1029/1999GB900084
Du, X. Z., Hao, M., Guo, L. J., Li, S. H., Hu, W. L., Sheng, F., et al. (2022). Integrated assessment of carbon footprint and economic profit from paddy fields under microbial decaying agents with diverse water regimes in Central China. Agric. Water Manag. 262:107403. doi: 10.1016/j.agwat.2021.107403
Follett, R. F. (2001). Soil management concepts and carbon sequestration in cropland soils. Soil Tillage Res. 61, 77–92. doi: 10.1016/S0167-1987(01)00180-5
Freibauer, A., Rounsevell, M. D. A., Smith, P., and Verhagen, J. (2004). Carbon sequestration in the agricultural soils of Europe. Geoderma 122, 1–23. doi: 10.1016/j.geoderma.2004.01.021
Fu, C. H., Large, S., Knight, B., Richardson, A. J., Bundy, A., Reygondeau, G., et al. (2015). Relationships among fisheries exploitation, environmental conditions, and ecological indicators across a series of marine ecosystems. J. Mar. Syst. 148, 101–111. doi: 10.1016/j.jmarsys.2015.01.004
Fuentes-Ponce, M. H., Gutierrez-Díaz, J., Flores-Macías, A., Gonzalez-Ortega, E., Mendoza, A. P., Sanchez, L. M. R., et al. (2022). Direct and indirect greenhouse gas emissions under conventional, organic, and conservation agriculture. Agric. Ecosyst. Environ. 340:108148. doi: 10.1016/j.agee.2022.108148
Gangopadhyay, S., Banerjee, R., Batabyal, S., Das, N., Mondal, A., Chandra, P. S., et al. (2022). Carbon sequestration and greenhouse gas emissions for different rice cultivation practices. Sustain. Product. Consump. 34, 90–104. doi: 10.1016/j.spc.2022.09.001
Gao, B., Ju, X. T., Su, F., Meng, O., Oenema, Q. F., Christie, P., et al. (2014). Nitrous oxide and methane emissions from optimized and alternative cereal cropping systems on the North China plain: a two-year field study. Sci. Total Environ. 472, 112–124. doi: 10.1016/j.scitotenv.2013.11.003
Ge, X. G., Cao, Y. H., Zhou, B. Z., Xiao, W. F., Tian, X. K., and Li, M. H. (2020). Combined application of biochar and N increased temperature sensitivity of soil respiration but still decreased the soil CO2 emissions in moso bamboo plantations. Sci. Total Environ. 730:139003. doi: 10.1016/j.scitotenv.2020.139003
Ghosh, S., Das, T. K., Rana, K. S., Biswas, D. R., Das, D. K., Singh, G., et al. (2022). Energy budgeting and carbon footprint of contrasting tillage and residue management scenarios in rice-wheat cropping system. Soil Tillage Res. 223:105445. doi: 10.1016/j.still.2022.105445
Gregorich, E. G., Rochette, P., VandenBygaart, A. J., and Angers, D. A. (2005). Greenhouse gas contributions of agricultural soils and potential mitigation practices in eastern Canada. Soil Tillage Res. 83, 53–72. doi: 10.1016/j.still.2005.02.009
Hang, X. N. (2015). Impacts of rice planting pattern and straw recycling on crop yield and greenhouse gas emission under rice-barley cropping system. Nanjing: Nanjing Agricultural University, Nanjing, China (in Chinese with English abstract).
Hu, A. Y., Sun, X., and Liu, Q. (2016). Effects of different rotation systems on greenhouse gas (CH4 and N2O) emissions in the Taihu Lake region, China. Chin. J. Appl. Ecol. 27, 99–106. doi: 10.13287/j.1001-9332.201601.031
Huang, J. X., Pan, J., Liu, W. R., Yang, G. L., Xiao, X. P., Zheng, H. B., et al. (2019). Carbon footprint of different agricultural systems in China estimated by different evaluation metrics. J. Clean. Prod. 225, 939–948. doi: 10.1016/j.jclepro.2019.04.044
Huang, J. D., Yu, X., Zhang, Z. L., Peng, S. B., Liu, B., Tao, X., et al. (2022). Exploration of feasible rice-based crop rotation systems to coordinate productivity, resource use efficiency and carbon footprint in Central China. Eur. J. Agron. 141:126633. doi: 10.1016/j.eja.2022.126633
Hung, N. V., Sande, B. O., Quilty, J., Balingbing, C., Castalone, A. G., Romasanta, R., et al. (2019). An assessment of irrigated rice production energy efficiency and environmental footprint with in-field and off-field rice straw management practices. Nature 9:16887. doi: 10.1038/s41598-019-53072-x
Hussain, S., Peng, S. B., Fahad, S., Khaliq, A., Huang, J. L., Cui, K. H., et al. (2015). Rice management interventions to mitigate greenhouse gas emissions: a review. Environ. Sci. Pollut. Res. 22, 3342–3360. doi: 10.1007/s11356-014-3760-4
Inubushi, K., Sugii, H., Nishino, S., and Nishino, E. (2001). Effects of aquatic weeds on methane emission from submerged paddy soils. Am. J. Bot. 88, 975–979. doi: 10.2307/2657078
IPCC (Intergovernmental Panel on Climate Change) (2014). Climate change 2014: Synthesis report. Contribution of working groups I, II and III to the fifth assessment report of the intergovernmental panel on climate change (Cambridge: Cambridge University Press).
IPCC (Intergovernmental Panel on Climate Change) (2021). “Climate change 2021: the physical science basis” in Contribution of working group I to the sixth assessment report of the intergovernmental panel on climate change (Cambridge: Cambridge University Press).
IPCC (Intergovernmental Panel on Climate Change) (2023). Climate change 2023: Synthesis report. Global warming of 1.5°C, climate change and land, the ocean and cryosphere in a changing climate. Cambridge: Cambridge University Press.
Janz, B., Weller, S., Kraus, D., Racela, H., Kiese, R., Wassmann, R., et al. (2019). Greenhouse gas footprint of diversifying rice-cropping systems: impacts of water regime and organic amendments. Agric. Ecosyst. Environ. 270-271, 41–54. doi: 10.1016/j.agee.2018.10.011
Jiang, J. Y., Huang, Y., and Zong, L. G. (2023). Influence of water controlling and straw application on CH4 and N2O emmissions from rice filed. China Environmental Science, 105–109. (in Chinese with English abstract). Available at: https://kns.cnki.net/kcms2/article/abstract?v=3uoqIhG8C44YLTlOAiTRKgchrJ08w1e7ZCYsl4RS_3iXSorujdrhY0wsXB-_3ahdNOYTQ_z1iXlPjy-LYLcS_St_c4DsmbDr&uniplatform=NZKPT&src=copy.
Jiang, Z. H., Lin, J. D., Liu, Y. Z., Mo, C. Y., and Yang, J. P. (2020). Double paddy rice conversion to maize-paddy rice reduces carbon footprint and enhances net carbon sink. J. Clean. Prod. 258:120643. doi: 10.1016/j.jclepro.2020.120643
Jiang, Y., Tian, Y. T., Sun, Y. N., Zhang, Y., Hang, X. N., Deng, A. X., et al. (2016). Effect of rice panicle size on paddy field CH4 emissions. Biol. Fertil. Soils 52, 389–399. doi: 10.1007/s00374-015-1084-2
Jiang, R., Xu, Q., Li, J. Y., Dai, L. X., Ao, D. C., Dou, Z., et al. (2022). Sensitivity and uncertainty analysis of carbon footprint evaluation: a case study of rice-crayfish coculture in China. Chin. J. Eco-Agric. 30, 1577–1587. doi: 10.12357/cjea.20220188
Jiang, Z. H., Yang, X., Liu, Y. Z., Lin, J. D., Wu, Y. X., and Yang, J. P. (2019a). Comparison of carbon footprint of spring maize-late rice and early rice-late rice-cropping patterns. J. Ecol. 39, 8091–8099,
Jiang, Z. H., Zhong, Y. M., Yang, J. P., Wu, Y. X. Y., Li, H., and Zheng, L. (2019b). Effect of nitrogen fertilizer rates on carbon footprint and ecosystem service of carbon sequestration in rice production. Sci. Total Environ. 670, 210–217. doi: 10.1016/j.scitotenv.2019.03.188
Kumar, R., Mishra, J. S., Mali, S. S., Mondal, R. S., Meena, R., Lal, B. K., et al. (2022). Comprehensive environmental impact assessment for designing carbon-cum-energy efficient, cleaner and eco-friendly production system for rice-fallow agro-ecosystems of South Asia. J. Clean. Prod. 331:129973. doi: 10.1016/j.jclepro.2021.129973
Kuypers, M., Marchant, H. K., and Kartal, B. (2018). The microbial nitrogen-cycling network. Nat. Rev. Microbiol. 16, 263–276. doi: 10.1038/nrmicro.2018.9
Lal, B., Gautama, P., Panda, B. B., Tripathi, R., Shahid, M., Bihari, P., et al. (2020). Identification of energy and carbon efficient cropping system for ecological sustainability of rice fallow. Ecol. Indic. 115:106431. doi: 10.1016/j.ecolind.2020.106431
Leon, A., and Izumi, T. (2022). Impacts of alternate wetting and drying on rice farmers’ profits and life cycle greenhouse gas emissions in a Giang Province in Vietnam. J. Clean. Prod. 354:131621. doi: 10.1016/j.jclepro.2022.131621
Leon, A., Minamikawa, K., Izumi, T., and Chiem, N. H. (2021). Estimating impacts of alternate wetting and drying on greenhouse gas emissions from early wet rice production in a full-dike system in a Giang Province, Vietnam, through life cycle assessment. J. Clean. Prod. 285:125309. doi: 10.1016/j.jclepro.2020.125309
Li, S. H., Guo, L. J., Cao, C. G., and Li, C. F. (2020). Effects of straw returning levels on carbon footprint and net ecosystem economic benefits from rice-wheat rotation in Central China. Environ. Sci. Pollut. Res. 28, 5742–5754. doi: 10.1007/s11356-020-10914-w
Liechty, Z., Santos-Medellín, C., Edwards, J., Nguyen, B., Mikhail, D., Eason, S., et al. (2020). Comparative analysis of root microbiomes of rice cultivars with high and low methane emissions reveals differences in abundance of methanogenic archaea and putative upstream fermenters. m Systems 5, e00897–e00819. doi: 10.1128/mSystems.00897-19
Ling, L., Shuai, Y. J., Xu, Y., Zhang, Z. S., Wang, B., You, L. Z., et al. (2021). Comparing rice production systems in China: economic output and carbon footprint. Sci. Total Environ. 791:147890. doi: 10.1016/j.scitotenv.2021.147890
Liou, R. M., Huang, S. N., and Lin, C. W. (2003). Methane emission from fields with differences in nitrogen fertilizers and rice varieties in Taiwan paddy soils. Chemosphere 50, 237–246. doi: 10.1016/S0045-6535(02)00158-3
Liu, T. Q., Li, S. H., Guo, L. G., Cao, C. G., Li, C. F., Zhai, Z. B., et al. (2020). Advantages of nitrogen fertilizer deep placement in greenhouse gas emissions and net ecosystem economic benefits from no-tillage paddy fields. J. Clean. Prod. 263:121322. doi: 10.1016/j.jclepro.2020.121322
López-Fando, C., and Pardo, M. T. (2011). Soil carbon storage and stratification under different tillage systems in a semi-arid region. Soil Tillage Res. 111, 224–230. doi: 10.1016/j.still.2010.10.011
Luo, L. J. (2010). Breeding for water-saving and drought-resistance rice (WDR) in China. J. Exp. Bot. 61, 3509–3517. doi: 10.1093/jxb/erq185
Ma, E. D., Ma, J., Xu, H., Cai, Z. C., and Ba, M. Y. H. (2010). Effects of rice straw returning methods in whmeat-growing season on CH4 emissions from following rice-g growing season. Ecol. Environ. Sci. 19, 729–732. doi: 10.16258/j.cnki.1674-5906.2010.03.031
Mandal, U. K., Bhardwaj, A. K., Lama, T. D., Nayak, D. B., Samui, D., Burman, K. K., et al. (2021). Net ecosystem exchange of carbon, greenhouse gases, and energy budget in coastal lowland double cropped rice ecology. Soil Tillage Res. 212:105076. doi: 10.1016/j.still.2021.105076
Maris, S. C., Teira-Esmatges, M. R., Arbones, A., and Rufat, J. (2015). Effect of irrigation, nitrogen application, and a nitrification inhibitor on nitrous oxide, carbon dioxide and methane emissions from an olive (Olea Europaea L.) orchard. Sci. Total Environ. 538, 966–978. doi: 10.1016/j.scitotenv.2015.08.040
Meng, F. J., Guan, G. H., Zhang, Q. Z., Juan, Y. J., Qu, B., and Kuang, X. (2006). Seasonal variation in soil respiration under different long-term cultivation practices on high yield farmland in the North China plain. Journal of environmental. Science 26, 992–999. doi: 10.13671/j.hjkxxb.2006.06.020
Mosier, A., Halvorson, A., Peterson, G., Robertson, G., and Sherrod, L. (2005). Measurement of net global warming potential in three agroecosystems. Nutr. Cycl. Agroecosyst. 72, 67–76. doi: 10.1007/s10705-004-7356-0
Nan, W. G., Li, S. Q., Dong, Z. B., and Yao, P. W. (2020). CH4 ffuxes and diffusion within soil proffles subjected to different fertilizer regimes on China’s loess plateau. Agric. Ecosyst. Environ. 287:106679. doi: 10.1016/j.agee.2019.106679
Qi, L., Pokharel, P., Ni, C. S., Gong, X. Q., Zhou, P., Niu, H. D., et al. (2020). Biochar changes thermal activation of greenhouse gas emissions in a rice-lettuce rotation microcosm experiment. J. Clean. Prod. 247:119148. doi: 10.1016/j.jclepro.2019.119148
Qian, H., Zhu, X., Huang, S., Linquist, B., Kuzyakov, Y., Wassmann, R., et al. (2023). Greenhouse gas emissions and mitigation in rice agriculture. Nat. Rev. Earth Environ. 4, 716–732. doi: 10.1038/s43017-023-00482-1
Qiao, J. D. (2019). Effects of cultivation modes on soil fertility, greenhouse gases emission and yield in ratoon Rice fields. Thesis. Wuhan, China: Huazhong agricultural university.
Qin, X. B. (2011). Mitigation of greenhouse gas intensity from typical double rice field of Central China. Beijing, China: Chinese Academy of Agricultural Sciences.
Qin, X. B., Lu, Y. H., Wan, Y. F., Wang, B., Nie, J., Li, Y., et al. (2023). Rice straw application improves yield marginally and increases carbon footprint of double cropping paddy rice (Oryza sativa L.). Field Crop Res. 291:108796. doi: 10.1016/j.fcr.2022.108796
Qiu, Z. J., He, X. H., Yu, H. Y., Zhu, C. W., and Shen, W. S. (2023). Differential responses of soil bacterial communities to elevated CO2 between strongly CO2-responsive and weakly CO2-responsive rice cultivars. Sci. Total Environ. 869:161843. doi: 10.1016/j.scitotenv.2023.161843
Riya, S., Zhou, S., Watanabe, Y., Sagehashi, M., Terada, A., and Hosomi, M. (2012). CH4 and N2O emissions from different varieties of forage rice (Oryza sativa L.) treating liquid cattle waste. Sci. Total Environ. 419, 178–186. doi: 10.1016/j.scitotenv.2012.01.014
Serraj, R., McNally, K. L., Slamet-Loedin, I., Kohli, A., Haefele, S. M., Atlin, G., et al. (2011). Drought resistance improvement in rice: an integrated genetic and resource management strategy. Plant Product. Sci. 14, 1–14. doi: 10.1626/pps.14.1
Shang, Q. Y., Cheng, C., Wang, J. J., Luo, K., Zeng, Y. J., and Yang, X. X. (2021). Net global warming potential, greenhouse gas intensity and carbon footprint as affected by different tillage systems from Chinese double-cropping paddy fields. Soil Tillage Res. 209:104947. doi: 10.1016/j.still.2021.104947
Sun, H., Feng, Y., Ji, Y., Shi, W., Yang, L., and Xing, B. (2018). N2O and CH4 emissions from N fertilized rice paddy soil can be mitigated by wood vinegar application at an appropriate rate. Atmos. Environ. 185, 153–158. doi: 10.1016/j.atmosenv.2018.05.015
Sun, M., Zhan, M., Zhao, M., Tang, L. L., Qin, M. G., Cao, C. G., et al. (2019). Maize and rice double cropping benefits carbon footprint and soil carbon budget in paddy field. Field Crop Res. 243:107620. doi: 10.1016/j.fcr.2019.107620
Tian, H., Xu, C. R., Thompson, R., Winiwarter, W., Suntharalingam, P., Davidson, E., et al. (2020). A comprehensive quantification of global nitrous oxide sources and sinks. Nature 586, 248–256. doi: 10.1038/s41586-020-2780-0
Tseng, M. C., Roel, A., Deambrosi, E., Zorrilla, G., Riccetto, S., and Pittelkow, C. M. (2020). Towards actionable research frameworks for sustainable intensification in high-yielding rice systems. Nature 10:9975. doi: 10.1038/s41598-020-63251-w
Wackernagel, M., and Rees, W. (1998). Our ecological footprint: reducing human impact on the earth. United Kingdom: New society publishers.
Walling, E., and Vaneeckhaute, C. (2020). Greenhouse gas emissions from inorganic and organic fertilizer production and use: a review of emission factors and their variability. J. Environ. Manag. 276:111211. doi: 10.1016/j.jenvman.2020.111211
Wiedmann, T., and Minx, J. (2008). “A definition of carbon footprint” in Ecological economics research trends. ed. C. C. Pertsova (Hauppauge, NY, USA: Nova Science Publishers).
Wilson, H. M., and Al-Kaisi, M. M. (2008). Crop rotation and nitrogen fertilization effect on soil CO2 emissions in central lower. Appl. Soil Ecol. 39, 264–270. doi: 10.1016/j.apsoil.2007.12.013
Woolf, D., Amonette, J. E., Perrott, A. S., Lehmann, J., and Joseph, S. (2010). Sustainable biochar to mitigate global climate change. Nat. Commun. 1:56. doi: 10.1038/ncomms1053
Wu, Z., Zhang, X., Dong, Y. B., Li, B., and Xiong, Z. Q. (2019). Biochar amendment reduced greenhouse gas intensities in the rice-wheat rotation system: six-year field observation and meta-analysis. Agric. For. Meteorol. 278:107625. doi: 10.1016/j.agrformet.2019.107625
Xia, F. (2019). Study on yield, resource utilization efficiency and environmrntal cost of different planting patterns in the middle reaches of Yangtze river. Wuhan, China: Huazhong Agricultural University.
Xiang, H. Y., Zhu, B., Wang, Y. Y., Li, K., and Duan, W. X. (2007). Effects of nitrogen fertilizer for maize on de nitrification loss and N2O emission in purple soil. J. Zhejiang Univ. 33, 574–583. doi: 10.3321/j.issn:1008-9209.2007.05.018
Xie, Z. B., Xu, Y. P., Liu, G., Liu, Q., Zhu, J. G., et al. (2013). Impact of biochar application on nitrogen nutrition of rice, greenhouse-gas emissions and soil organic carbon dynamics in two paddy soils of China. Plant Soil 370, 527–540. doi: 10.1007/s11104-013-1636-x
Xing, G. X., Zhao, X., Xiong, Z. Q., Yan, X., Hua, X., Xie, Y., et al. (2009). Nitrous oxide emission from paddy fields in China. Acta Ecol. Since 29, 45–50. doi: 10.1016/j.chnaes.2009.04.006
Xu, S. (2017). Investigation on soil carbon sequestration and mitigation of greenhouse gas emission by the integrated rice-azolla cropping system in double rice cropped region. Beijing, China: China Agricultural University.
Xu, X. Y. (2020). Studies on greenhouse gas emissions and influencing factors of three middle-season paddy fields. Wuhan, China: Huazhong Agricultural University.
Xu, Y., Ge, J. Z., Tian, S. Y., Li, S. Y., Nguy-Robertson, A. L., Zhan, M., et al. (2015). Effects of water-saving irrigation practices and drought resistant rice variety on greenhouse gas emissions from a no-till paddy in the central lowlands of China. Sci. Total Environ. 505, 1043–1052. doi: 10.1016/j.scitotenv.2014.10.073
Xu, Q., Hu, K. L., Yao, Z. S., and Zuo, Q. (2020). Evaluation of carbon, nitrogen footprint and primary energy demand under different rice production systems. Ecol. Indic. 117:106634. doi: 10.1016/j.ecolind.2020.106634
Xu, Y., Liang, L. Q., Wang, B., Xiang, J. B., Gao, M. T., Fu, Z. Q., et al. (2022). Conversion from double-season rice to ratoon rice paddy fields reduces carbon footprint and enhances net ecosystem economic benefit. Sci. Total Environ. 813:152550. doi: 10.1016/j.scitotenv.2021.152550
Xu, G. H., Liu, X., Wang, Q. S., Yu, X. C., and Hang, Y. H. (2017). Integrated rice-duck farming mitigates the global warming potential in rice season. Sci. Tot. Environ. 575, 58–66. doi: 10.1016/j.scitotenv.2016.09.233
Xue, J. F., Pu, C., Liu, S. L., Zhao, X., Zhang, R., Chen, F., et al. (2016). Carbon and nitrogen footprint of double rice production in southern China. Ecol. Indic. 64, 249–257. doi: 10.1016/j.ecolind.2016.01.001
Yadav, G. S., Babu, S., Das, A., Mohapatra, K. P., Singh, R., Avasthe, R. K., et al. (2020). No-till and mulching enhance energy use efficiency and reduce carbon footprint of a direct-seeded upland rice production system. J. Clean. Prod. 271:122700. doi: 10.1016/j.jclepro.2020.122700
Yadav, G. S., Das, A., Lal, R., Babu, S., Meena, R. S., Saha, P., et al. (2018). Energy budget and carbon footprint in a no-till and mulch based rice-mustard cropping system. J. Clean. Prod. 191, 144–157. doi: 10.1016/j.jclepro.2018.04.173
Yan, M., Cheng, K., Luo, T., Yan, Y., Pan, G. X., and Rees, R. M. (2015). Carbon footprint of grain crop production in China e based on farm survey data. J. Clean. Prod. 104, 130–138. doi: 10.1016/j.jclepro.2015.05.058
Yan, X., Shi, S., Du, L., and Xing, G. (2000). Pathways of N2O emission from rice paddy soil. Soil Biol. Biochem. 32, 437–440. doi: 10.1016/S0038-0717(99)00175-3
Yang, X., Shang, Q., Wu, P., Liu, J., Shen, Q., Guo, S., et al. (2010). Methane emissions from double rice agriculture under long-term fertilizing systems in Hunan, China. Agric. Ecosyst. Environ. 137, 308–316. doi: 10.1016/j.agee.2010.03.001
Yu, H. Y., Huang, Q., Wang, T. Y., Zhang, G. F., Ma, J., Zhu, C. W., et al. (2021). Effect of elevated CO2 on N2O emissions from different rice cultivars in rice fields. Environmental. Science 42, 3924–3930. doi: 10.13227/j.hjkx.202012211
Yun, C., Li, S. Y., Zhang, Y. J., Chen, Y., Li, S. Y., Zhang, Y. J., et al. (2019). Rice root morphological and physiological traits interaction with rhizosphere soil and its effect on methane emissions in paddy fields. Soil Biol. Biochem. 129, 191–200. doi: 10.1016/j.soilbio.2018.11.015
Zhang, G. B., Song, K. F., Huang, Q., Zhu, X. L., Gong, H., Ma, J., et al. (2022). Heavy metal pollution and net greenhouse gas emissions in a rice-wheat rotation system as influenced by partial organic substitution. J. Environ. Manag. 307:114599. doi: 10.1016/j.jenvman.2022.114599
Zhen, H. Y., Feng, X., and Muhammad, A. W. (2023). Solutions to neutralize greenhouse gas emissions of the rice value chain-a case study in China. Sustain. Product. Consump. 35, 444–452. doi: 10.1016/j.spc.2022.11.023
Zhong, C., Liu, Y., Xu, X. T., Yang, B. J., Aamer, M., Zhang, P., et al. (2021). Paddy-upland rotation with Chinese milk vetch incorporation reduced the global warming potential and greenhouse gas emissions intensity of double rice-cropping system. Environ. Pollut. 276:116696. doi: 10.1016/j.envpol.2021.116696
Zhou, Y. J., Ji, Y. L., Zhang, M., Xu, Y. Z., Li, Z., Tu, D. B., et al. (2023). Exploring a sustainable rice-cropping system to balance grain yield, environmental footprint and economic benefits in the middle and lower reaches of the Yangtze River in China. J. Clean. Prod. 404:136988. doi: 10.1016/j.jclepro.2023.136988
Keywords: rice-cropping system, carbon footprint, greenhouse gas emissions, emission reduction, China
Citation: Ji Y, Zhou Y, Li Z, Feng K, Sun X, Xu Y, Wu W and Zou H (2024) Carbon footprint research and mitigation strategies for rice-cropping systems in China: a review. Front. Sustain. Food Syst. 8:1375092. doi: 10.3389/fsufs.2024.1375092
Edited by:
Roberto Valdivia, Oregon State University, United StatesReviewed by:
Juan Fernando Hirzel, Agricultural Research Institute (Chile), ChileApurbo Chaki, Bangladesh Agricultural Research Institute, Bangladesh
Copyright © 2024 Ji, Zhou, Li, Feng, Sun, Xu, Wu and Zou. This is an open-access article distributed under the terms of the Creative Commons Attribution License (CC BY). The use, distribution or reproduction in other forums is permitted, provided the original author(s) and the copyright owner(s) are credited and that the original publication in this journal is cited, in accordance with accepted academic practice. No use, distribution or reproduction is permitted which does not comply with these terms.
*Correspondence: Wenge Wu, d3V3ZW5nZUB2aXAuc2luYS5jb20=; Huawen Zou, em91aHVhd2VuQHlhbmd0emV1LmVkdS5jbg==