- 1School of Engineering and Built Environment, Griffith University, Brisbane, QLD, Australia
- 2Department of Fisheries and Marine Science, Noakhali Science and Technology University, Noakhali, Bangladesh
- 3Department of Applied Chemistry and Chemical Engineering, Noakhali Science and Technology University, Noakhali, Bangladesh
- 4Environmental Studies Department, University of California, Santa Cruz, Santa Cruz, CA, United States
- 5Department of Zoology, College of Science, King Saud University, Riyadh, Saudi Arabia
- 6Environmental and Life Sciences Programme, Faculty of Science, Universiti Brunei Darussalam, Gadong, Brunei Darussalam
The current knowledge regarding the presence of microplastics (MPs) in the digestive tracts of fish, particularly the non-edible parts, provides limited assistance in assessing the risk to human health and establishing guideline values for policy-making purposes. Therefore, we conducted the first investigation into MPs within the commercially significant catfish species S. silondia, form a major tropical estuary assessing their accumulation, characteristics, and associated risks in various tissues, particularly the consumable muscle. A sum of 132 MPs were identified, and the mean abundance of MPs were 7.40 ± 4.12 item/DT, 3.20 ± 1.69 item/gill and 2.60 ± 1.65 item/muscle found in digestive tract (DT), gill and muscle of the fish, respectively. The strong relationships of MPs among three different tissues (y = 0.6069x - 22.69, R2 = 0.7589) indicated the pathways and how MPs were influenced by the physiology of organisms. The prevalence of MPs in fish muscle demonstrated their trophic transmission to humans. Moreover, fishes with higher weight had higher MPs in their gill, and DT. The dominant type, color and size of MPs were fibrous (89.67%), violet (41%) and < 0.5 mm sized (94%), respectively. The high percentage of fibers suggests that they might have come from ropes, fishing nets or sewage. FTIR chemical composition analyses of microplastics (MPs) identified three primary polymer types: PET (44.5%), PE (33.3%), and PP (22.2%) in fish. These polymers are commonly associated with packaging materials, household wastes and plastic pipes. Contamination level assessment by CF (CF = 2.76) and PLI (PLI > 1) indicated moderately to highly contaminated fish with MPs ingestion. While the complete understanding of the direct negative effects of microplastics (MPs) on human health remains elusive, their presence in estuarine fish raises concerns for both human and ecosystem health. The findings will be useful for assessing the hazards to human health from exposure to MPs and for creating effective risk management plans to reduce those risks.
1 Introduction
Plastic pollution has emerged as a global environmental crisis, with its pervasive presence in oceans, rivers, and terrestrial ecosystems, posing a profound threat to biodiversity, ecosystems, and human health worldwide (Banik et al., 2022, 2024). Nowadays, human society cannot imagine a single day without using plastic-made products. For example, a typical person in Western Europe uses roughly 100 Kg of plastics annually, making it a significant production and commodity product. Since the 1980s, the demand for plastic materials have increased because of their lightweight, strong, durable, and reasonably priced (Li et al., 2016). Plastic waste production exceeded 284 million metric tons in 2020 alone (Harris et al., 2021), and projections suggest a staggering increase to 33 billion metric tons by the year 2050 (Crawford and Quinn, 2017). Unfortunately, inadequate handling and irresponsible disposal of plastic waste have made it ubiquitous, posing a significant hazard to both the environment and human health.
Microplastics (MPs) are tiny particles of plastic waste, typically measuring less than 5 mm in diameter (Nur et al., 2022). They exist in various forms such as microbeads, pellets, fibers, or fragments originating from our daily activities. Additionally, they result from the breakdown of primary plastic waste through processes like photooxidation and biodegradation (Cole et al., 2011; Diepens and Koelmans, 2018). MPs often exert significant negative effects on aquatic organisms, such as impaired reproduction and decreased nutritional uptake. These effects can persist due to the slow or limited biodegradability of MPs, which may endure in water for hundreds or even thousands of years. MPs from the environment accumulate in marine life, subsequently being ingested by larger fish, birds, or other predators. As these organisms are consumed by others further along the food chain, humans ultimately ingest the MPs passed from prey to predator. In addition, these MPs appear to be able to absorb extremely dangerous and common pollutants like PCBs (polychlorinated biphenyls) and pesticides like DDT and other harmful elements including polychlorinated biphenyls and phthalates, which have been linked to human cancer and a number of other major health issues. Therefore, while developing strategies to manage this kind of pollution, it is crucial to understand how plastic particles are accumulated in marine organisms and the ecological concerns that ensue.
Estuaries represent one of the most productive and ecologically dynamic ecosystems, providing a diverse range of products, services and deemed as significant nursery grounds for fish (Martinho et al., 2007; Hossain et al., 2023). The Meghna, the largest estuary in Bangladesh, is home to many a number of commercially important fish, including the national fish, Hilsa ilisha. However, agricultural waste, fecal waste, oil spills from passenger boats and fishing and numerous small industrial effluents that contain a significant number of synthetic wastes are the main sources of pollution entering estuarine systems (Rahman et al., 2019). As per the RPI index, the lower Meghna River Estuary has been categorized as a contaminated estuary, attributed to elevated concentrations of certain water parameters. This poses a significant threat to both fish populations and the local residents in coastal areas (Sharif et al., 2017). Recently, it has been determined that the Meghna river estuary has a significantly higher concentration of MPs discharging up to 3 billion pieces of MPs every day into the Bay of Bengal (Napper et al., 2021). Hence, they are anticipated to be gathered by the dwelling organisms from the estuary. The estuarine catfish, Silonia silondia, is a demersal fish species that is commercially significant and widely distributed in estuaries and rivers of Asian countries (IUCN Bangladesh, 2000; Hasan et al., 2002). It can provide us with useful information about the presence of MPs in the ecosystem. Therefore, research on fish consumption of MPs is essential to better understand the risk that it provides to human health and the estuarine ecosystem.
There have been numerous studies conducted worldwide on the assimilation of MPs by diverse aquatic species (Cheung et al., 2018; de Vries et al., 2020; Lin et al., 2020; Ghosh et al., 2021). There are few studies that have been focused on the accumulation of MPs in digestive tracts and gills of marine fishes in Bangladesh, such as Polynemus paradiseus, Carangoides chrysophrys, Setipinna phasa, Setipinna tenuifilis, Otolithoides pama and Priacanthus hamrur from the Bay of Bengal (Hossain et al., 2019, 2020; Ghosh et al., 2021; Siddique et al., 2022). However, these studies were mostly concentrated on MPs in digestive tracts (non-edible parts of fish) overlooking the consumable body parts (muscle) of fish. To assess the human health risk, it is crucial to know the accumulation rate of MPs in fish muscle. In addition, no research documenting the overall accumulation of MPs in different parts of fish including silond catfish (Silonia silondia) have been assessed in Bangladesh. Therefore, it is challenging to comprehend a complete pathway of how MPs influence the physiology of organisms and determine the widespread impact of MPs as an environmental pollutant without understanding the relationships between MPs abundance and biological parameters such as digestive tract (DT), gill and muscle weight, or length. Given the significance of estuarine habitat and the implications of MPs in ecosystems, this study attempted to document the presence and characterization of microplastics in the gastrointestinal tracts, gills, and muscles of cat fish, Silonia silondia collected from the lower Meghna river estuary. The specific objectives of this study were: (1) to get a preliminary account on abundance, type, shape, size, color and potential sources of microplastics (MPs) in DT, gill, and muscle of the fish, (2) to elucidate the relationship between microplastics abundance in DT, gill, as well as muscle and (3) to assess microplastics contamination levels in commercially important fish from Bangladesh in comparison with the results of previous studies. The result will help contribute a better understanding of the impacts of MPs on the estuarine ecology and potential human health hazards related due to their consumption.
2 Materials and methods
2.1 Study area
The lower Meghna River estuary, spanning 160 kilometers, stretches from the southern region of Chandpur to Tetulia. It is characterized by the Tetulia and Shahbazpur rivers, with a combined width of approximately 40 km at the coastline. The basin area of this estuary measures 1,574,223.9 km2. Known for its diverse and dynamic physiography, the Meghna River estuary is marked by intricate geomorphological features (Hossain et al., 2023). As one of the largest river estuaries in the world, it encompasses a vast deltaic region formed by the confluence of the Meghna, Padma, and Brahmaputra rivers. The physiography is shaped by a network of distributaries, tidal channels, and extensive mudflats. This estuary is categorized into three zones based on tidal range: micro-tidal (0-2 m) extending from Tetulia river through to Chandpur, meso-tidal (2–4 m) covering the west coast of Bangladesh to north Hatiya, south Bhola, and the south and west coast of Hatiya, and macro-tidal (4 ~ 6 m) encompassing the east coast of Hatiya to the south. Tidal influences play a significant role, creating tidal creeks and mudbanks that contribute to the ever-changing landscape. Mangrove forests, including the Sundarbans, fringe the estuary, providing crucial ecological habitats. The interaction of freshwater discharge from upstream rivers and saline tidal waters gives rise to a complex estuarine ecosystem, influencing sedimentation patterns and fostering biodiversity. The Estuary was selected for study on MPs for several reasons. Firstly, it is a transboundary river of Bangladesh, India and Nepal and a vital habitat for various key stone fish species (e.g., Lates calcarifer, Mughil cephalus, Hilsha illisha etc.), offering insight into impacts of MPs on aquatic ecosystems. Secondly, the estuary faces substantial anthropogenic pressures, such as industrial and agricultural runoff, which contribute to microplastic pollution (Hossain et al., 2023). Thirdly, the dynamic characteristics, influenced by tidal currents and sediment movement, could influence microplastic distribution, necessitating investigation. In addition, previous studies have identified the Meghna estuary as a microplastic contamination hotspot, emphasizing its importance for further research (Hossain et al., 2023).
2.2 Fish sample collection and preparation
Shillong catfish (Silonia silondia) samples were collected from the lower Meghna River estuary near central coast of Bangladesh. Selection of the species, S. silondia fish from the Meghna estuary of Bangladesh was driven by various factors. Firstly, the commercial importance of S. silondia raised concerns about food safety and fisheries management amidst microplastic contamination. Moreover, it is abundant in coastal waters of Bangladesh made it a practical and representative species for such research. Additionally, S. silondia has a potential role as a keystone species or ecosystem health indicator in Bangladesh prompted interest in its MP contamination. The habitat preferences, potentially in environments prone to plastic pollution such as rivers or estuaries, provided additional rationale for the study. For this study, fresh fish specimens were collected from March 2022 to April 2022 form the study area using the estuarine set bag net (ESBN or Behundi jal). Fish samples were collected from the estuary in March and April for some specific reasons. Firstly, these months typically offer stable environmental conditions, providing a representative snapshot of MP contamination levels in fish. Additionally, March and April may coincide with spawning or migration periods for some fish species, potentially affecting their exposure to and ingestion of microplastics. To catch fish, the net was anchored was set at 2–3 m shallow coastal waters of at sampling sites within the estuary, strategically positioned to intercept fish as they move with the current. As the net was designed with a bag-shaped enclosure that allows fish to enter but makes it difficult for them to escape. Once set, the net was left in place for 3–4 h, depending on environmental conditions. Then the net was carefully retrieved, and any captured fish were collected from the bag-shaped enclosure. Once collected, the fishes were transferred to containers filled with water from the estuary and handled with care to minimize stress. Morphometric data, including species identification and size measurements, were recorded for each specimen. In this study, a total of 10 fish specimens were utilized, with each specimen consisting of 10 replicates of gills, gastrointestinal tract, and muscle tissue. Consequently, a total of 50 samples were examined across all specimens. The average individual weight of the fish was 80.69 ± 9.70 g. The samples were then transferred to the Laboratory for Ecology, Environment and Biodiversity (LEEB) in Noakhali, and kept in a − 20°C refrigerator for further MPs analysis. The fish samples were then defrosted at room temperature in an iron tray, and the blood, debris, and sediment from the fish body were washed away with distilled water. Using an electronic weighing balance (BSA224S, Sartorius, China), the morphometric features of each specimen, including standard length (SL), total length (TL), fork length (FL), and body weight, were recorded following Ory et al. (2018). Each specimen was dissected individually using scissors, a scalpel, and forceps in an iron tray to assess the frequency of MPs ingestion in fish. Gills, 5 g of fish muscle tissue, and the gastrointestinal tract or Digestive tract (GIT/DT) were all removed, weighed, and placed in a petri dish (Karami et al., 2017; Hossain et al., 2019). To minimize the risk of contamination during acidic digestion, the fish tissues were transferred to a 1 L glass beaker and securely covered with aluminum foil (Ghosh et al., 2021).
2.3 Digestion of fish sample and extraction of MPs
H2O2 (Scharlab, Spain) digestion techniques were employed for the digestion of fish tissue, with a few minor adjustments (i.e., density separation by NaCl after digestion was skipped because there wasn’t much organic matter left after digestion). Then, fish tissue (GIT, gills, and muscle) was placed separately to a 1 L beaker containing 30% hydrogen peroxide (H2O2) at a ratio of 1:20 (w/v), which was thought to be more efficient at breaking down biogenic material than sodium hydroxide (NaOH) and hydrochloric acid (HCl) (Mathalon and Hill, 2014; Avio et al., 2015). Prior to proceeding to the subsequent phase, the acid-tissue combinations were assembled on a laboratory bench and allowed to sit at room temperature for 5 min. Once H2O2 had evaporated, the beaker was heated on a magnetic hotplate stirrer at a temperature of 55 to 65°C with 75 rpm (Avio et al., 2015). Nevertheless, if the organic matter persisted when the H2O2 had nearly evaporated to 1 milliliter, an additional 1–2 milliliters of 30% H2O2 were injected until the organic matter was nearly completely digested (Masura et al., 2015). Following digestion to remove all tissue, samples were placed in a density separator for 24 h (Coppock et al., 2017). A 47 mm diameter 5.0 μm Cellulose Nitrate filter paper from Minipore i (India) was used to filter the separated solution.
2.4 Microscopic analysis and polymer identification of MPs
The identification and quantification of MPs particles from the filter were carried out using a light stereomicroscope (Leica EZ4E, Germany) with 8× to 35× magnification (Hidalgo-Ruz et al., 2012; Cheung et al., 2016). To count the MPs of the filter paper, each quadrate of filter paper was checked carefully (Lots et al., 2017). The MPs count in each sample was manually assessed under microscopy through visual inspection and tallying. Additionally, in some cases, photos of each quadrat were taken and counted using software Size measurements were made using the images, which were taken using a high-resolution camera mounted to the microscope. ImageJ software (version 2.0.0) was used to determine the size of MPs (Laglbauer et al., 2014). Using a hot needle test, the non-plastic compounds were removed (De Witte et al., 2014). The type, color, and sizes of MPs were determined (Hossain et al., 2021). Relatively larger particles from filter papers were chosen to distinguish the different polymers.
To identify the polymer type, a potassium bromide (KBr) pellet technique and FTIR analysis using the 8400S model by Shimadzu Corporation, Japan (wavenumber range: 4,000–400 cm-1), were employed. This involved combining 200 mg of KBr powder with 1–3 mg of finely crushed microplastic (MPs) samples, compressing the mixture for 1 min under a regular pressure of 10 tons using a Shimadzu (IR Prestige-21) hydraulic press to create a transparent pellet. The entire system was kept under evacuation during pellet production. The resulting pellet was analyzed using an FTIR spectrometer with a resolution of 2 cm-1 over 30 scans. To enhance the identification process, automated contrast with a comprehensive spectrum library was utilized. The FTIR spectra were compared to those in previously published studies (Noda et al., 2007; Jung et al., 2018) to ensure accurate identification, avoiding reliance solely on automated libraries.
2.5 Contamination and health hazard assessment of MPs in fish
To evaluate ecological risk in both terrestrial and aquatic ecosystems, the contamination factor (CF) and pollutant load index (PLI) are frequently used (Tomlinson et al., 1980). This study utilized the count of MPs in samples fish from the Meghna River estuary to assess human and environmental risk. The equations provided in Supplementary Table S1 were utilized to compute the Pollution Load Index (PLI), with categorization based on the values recommended by Tomlinson et al. (1980). Remarkably, there was a lack of prior scientific inquiry into microplastic contamination in the lower Meghna River estuary for the establishment of baseline levels in fish. Thus, the minimal concentration of MPs in the digestive tract (DT), gill, and muscle was identified as the background value for various fish species.
2.6 Control of contamination
Prior to use, all liquids (distilled water and hydrogen peroxide) underwent filtration using Cellulose Nitrite Filter paper, featuring a 47 mm diameter and a 5 μm pore size. Instruments used in the study were cleaned and rinsed with purified distilled water. Rigorous protocols were followed throughout the process of fish sample collection, transportation, and preservation, as well as during the dissection of fish tissues (gill, gastrointestinal tract, and muscle), aimed at preventing contamination. Dissected tissues were placed in petri dishes and covered with aluminum foil paper to mitigate the risk of airborne fiber contamination (De Witte et al., 2014; Devriese et al., 2015). To establish a baseline for comparison, a completely blank sample without fish tissue underwent the same processing methodology (Li et al., 2015), and these blank samples showed no presence of MPs.
2.7 Statistical analysis
Before statistical analysis, normality distribution of data and homogeneity of variance were tested. Initially, descriptive statistics encompassed calculating metrics such as the mean, median, standard deviation, and range for variables such as microplastic abundance and fish body attributes (e.g., weight, gut weight etc.). Parametric statistical analyses such as One-way Analysis of Variance (ANOVA), was applied to compare the abundance of MPs among the specimens and the relationship between fish traits (i.e., body weight, gill weight, DT weight, MPs/g gill and MPs/g DT). Post-hoc tests, like Tukey’s HSD (Honestly Significant Difference) test, were subsequently employed to identify specific group differences if ANOVA results were deemed significant. In order to evaluate the relationships between fish biological traits, such as body size and weight, and microplastic abundance Pearson’s test was used (Peters and Bratton, 2016). Subsequently, regression analysis is utilized to formally model the relationship between microplastic contamination and fish body characteristics. The threshold for significance was either <0.05 or < 0.01. The computer packages, PAST (PAleontological Statistics; Version 4.03), Origin Pro 2022 (9.9), IBM SPSS statistics (Version 25), R Studio (with R version 3.5.1),1 ggplot2 (version 3.1.0) (Ghosh et al., 2021), were used for the statistical analyses.
3 Results and discussion
3.1 Abundance of MPs in fish of the Meghna River estuary
All the thirty samples from ten specimens of S. silondia contained MPs with an average number of 13.20 ± 6.55 MPs/individual (Figure 1; Table 1). MPs were present in samples of the digestive tract, gill and muscle as 5.5 ± 1.25 MPs/g, 3.10 ± 1.50 MPs/ g, and 0.52 ± 0.33 MPs/g, respectively. One-way ANOVA showed that the occurrence of MPs highly significantly differed (F = 26.24, p < 0.01) among the DT, gill and muscle of investigated species. This indicates that the distribution of MPs in different organs of S. silondia is not uniform and that the digestive tract and gills are more likely to contain higher concentrations of MPs than the muscle. The presence of MPs in fish muscle was surprising. The MPs accumulated in the flesh may originated from the digestive system, as indicated by their abundance in the digestive tract. The transport of very small-sized MPs through the digestive system, facilitated by digestive processes and the circulation of fluids within the fish, including blood, potentially enables their passage into other tissues such as the muscle tissue. Karami et al. (2017), suggested that direct pathways for MPs to reach fish muscles are skin, gills and gastrointestinal. The minimum size of plastic that can enter a fish’s vein or blood vessel depends on various factors, including the species of fish, the size of the plastic particle, and the conditions under which the fish ingests the plastic (Akhbarizadeh et al., 2018). Generally, smaller microplastic particles have a higher likelihood of entering a fish’s bloodstream. Plastic particles smaller than the diameter of a human hair—less than 20 micrometers can enter blood stream.
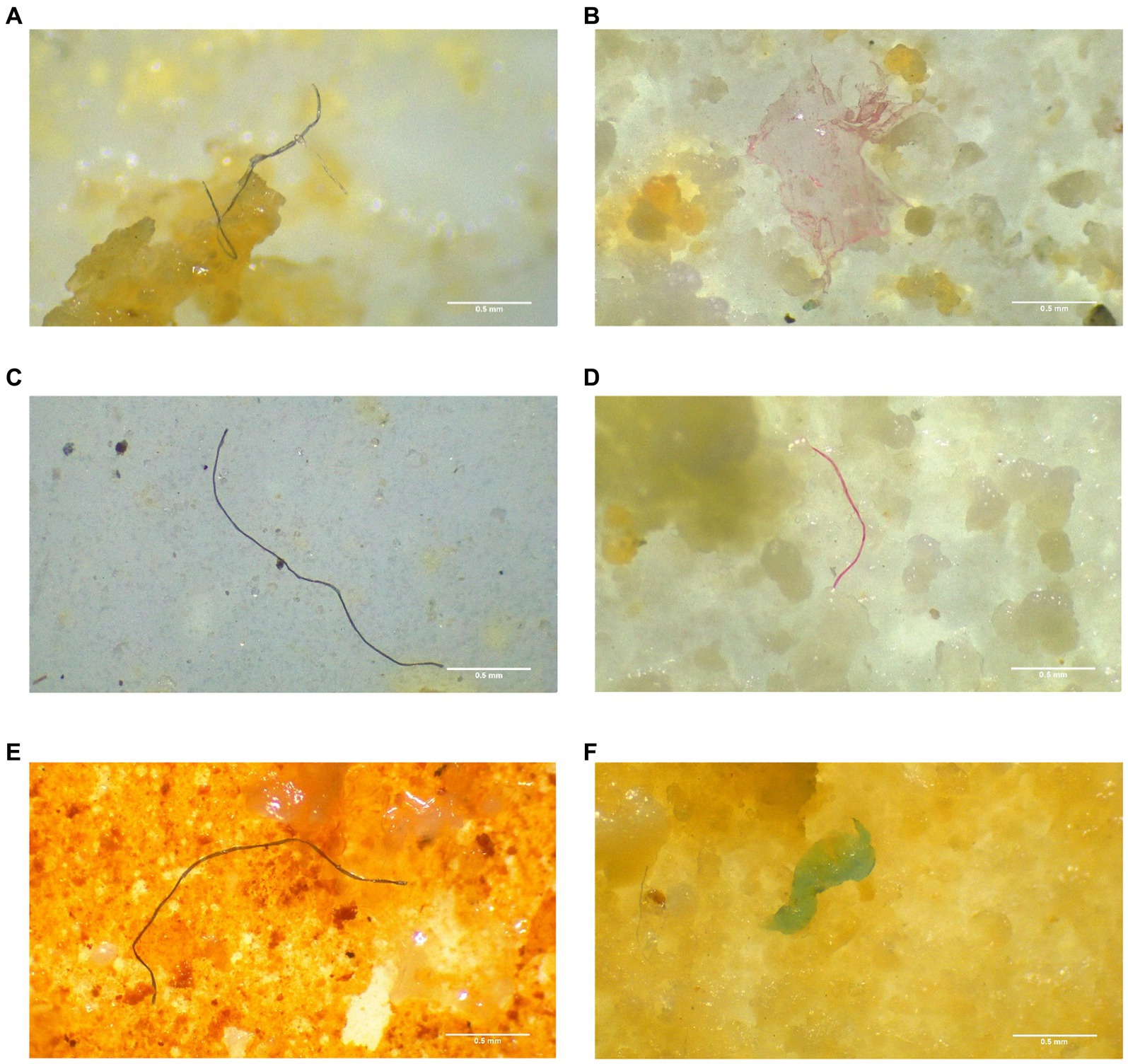
Figure 1. Different types of microplastics in the studied fish; (A) violet fiber, (B) pink film, (C) blue fiber, (D) red fiber, (E) black fiber, (F) green film.
According to the results of Tukey’s pairwise comparisons, MPs/g gill showed highly significant difference (p < 0.01) from MPs/ g DT, MPs/ g muscle, and MPs/g BW whereas MPs/ g muscle did not differ significantly from MPs/ g DT (p < 0.05), and MPs/g BW (p < 0.05). Moreover, there was a positive correlation between the abundance of MP in different body parts and the body weight (r = 0.87116, p < 0.01), gill weight (r = 0.66248, p < 0.05), and DT weight (r = 0.90117, p < 0.01). This suggests that fish with greater weight tend to exhibit higher concentrations of MPs in their gills, digestive tract, and muscle. The regression analysis revealed strong relationships among MPs across three distinct tissues (y = 0.6069x − 22.69, R2 = 0.7589), suggests that microplastics might be influencing the health or growth of fish by organismal physiology (Figure 2). The findings helps in identifying patterns or trends that might not be apparent from simple correlation. These findings align with previous studies conducted by Hossain et al. (2019) and Yagi et al. (2022).
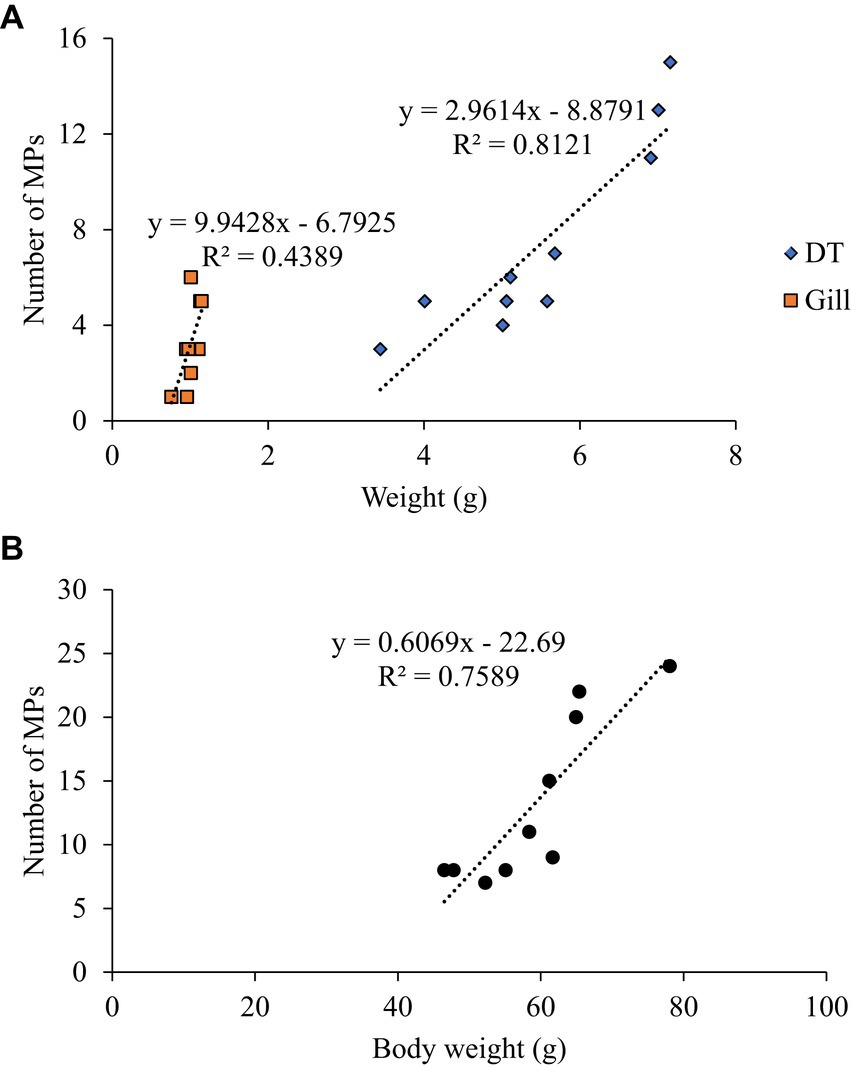
Figure 2. The relationship between MPs abundance and (A) digestive tract (DT) weight and gill weight and (B) body weight.
The results of this study were contrasted with those of other studies carried out globally (Table 2). The number of MPs in muscle ranging from 1.78 ± 0.26 to 6.03 ± 0.47 item/g of fish tissue in the Tunisian coast was higher than that of the present investigation (Zitouni et al., 2020). In addition, the number of MPs in DT/g of S. silondia was found to be lower than Polynemus paradiseus, Carangoides chrysophrys, Setipinna phasa, Setipinna tenuifilis, Otolithoides pama and Priacanthus hamrur fishes from Bay of Bengal (Ghosh et al., 2021; Hossain et al., 2022) and Scomber japonicus, and Trichiurus japonicus fish from west coast of Kyushu, Japan (Yagi et al., 2022). In contrast, Otolithoides pama, Harpadon nehereus, Anodontostoma chacunda, Coilia neglecta, Harpadon translucens and Megalaspis cordyla from Bay of Bengal had lower MPs levels in DT compared to this study (Ghosh et al., 2021; Hossain et al., 2022).
While the widespread presence of microplastics in fish and shellfish has been extensively documented worldwide, including in Bangladesh, there has been limited investigation into the presence of MPs in other protein sources such as beef and chicken. In Bangladesh, studies on microplastic contamination from terrestrial meats or alternative protein sources are scarce, making it challenging to compare results. However, research on this emerging topic is gaining attraction due to its presence in various food items, including fish, drinking water, salt, sugar, and ecosystems like land, rivers and oceans, raising concerns about animal health and food safety. However, studies from other regions have revealed that a significant portion of proteins, including pork, beef, chicken, tofu, and various plant-based alternatives, contain MPs at comparable levels to fish. Highly processed foods like fish sticks, chicken nuggets, tofu, and plant-based burgers were found to have slightly higher MP content per gram compared to minimally processed proteins like chicken breast, although the difference was statistically insignificant. According to Baechler et al. (2024), the mean number of microplastics consumed in a single serving for seafood, terrestrial meat, and plant-based protein was 120 ± 320, 32 ± 61, and 40 ± 69 MPS, respectively. This ubiquity of MP across protein types suggests that the source of proteins, whether from the ocean, land, or animal production facilities, does not distinctly influence overall MP contamination levels.
The occurrence of varied MPs in different species may depend on the feeding habits and the surrounding environments (Romeo et al., 2015; Jabeen et al., 2017; Ory et al., 2017). While consuming food from the water column or sifting through contaminated debris, fish might intentionally or unintentionally absorb MPs (Cannon et al., 2016). In a recent study, 500–20,000 MPs/km2 were recorded from the surface waters of Bay of Bengal (Eriksen et al., 2018) which might affects the transfer of MPs in fish. Hence, further investigation is recommended to monitor and understand the contamination level of MPs in marine ecosystems, and the risk of exposure to organisms therein.
3.2 Morphometric characteristics of MPs in different tissues of fish
Nearly all identified MPs in S. silondia were fiber of diversified color and size (Figure 3). In DT, the types of MPs followed the decreasing order of fiber (78.4%) > films (16.2%) > fragment (5.4%) whereas fiber (90.6%) and films (9.4%) were abundant in fish gill (Figure 3A). Further, only fiber was found in the muscle of experimented fish. Further, colored MPs (88% of total recorded MPs) were predominant in the experimented fish and the rest were (12%) transparent. The MP color followed the decreasing order of violet (41%) > pink (17%) > blue (13%) > red (13%) > green (4%). Transparent, pink, violet, blue, and red colored MPs were common in DT, gill and muscle (Figure 3B). Besides, most of MPs (94% of total recorded MPs) recorded in this study were < 0.5 mm size range, followed by 0.5 − 1 mm (5%), and 1 − 5 mm (1%). Maximum number of 0.5 −1 mm (6.8%) and 1 −5 mm (1.4%) size range MPs were predominant in the DT (Figure 3C). In contrast, all the MPs (100%) in the muscle were < 0.5 mm size range.
Studies from various countries have also documented a variety of MPs in freshwater and marine organisms (Li et al., 2015, 2016; Kolandhasamy et al., 2018; Frias and Nash, 2019; Teng et al., 2019). Their findings revealed that fiber was the predominant type of MPs in fishes (Desforges et al., 2015; Steer et al., 2017; Hossain et al., 2019) which supported our present findings. In this study, it was discovered that fibers comprised 86% of all microplastics (MPs), consistent with previous findings indicating that fish from the Spanish coast contained 83% fibers (Compa et al., 2018), 70% fibers in fish from the Mediterranean Sea (Güven et al., 2017), and 71% fibers in fish from the coast of Spain (Bellas et al., 2016). Fibers in coastal waters can originate from a range of sources, such as fishing nets and ropes, municipal waste, household and laundry effluents, etc., (Browne et al., 2011; Hossain et al., 2021; Banik et al., 2022).
Six various colors of MPs were noticed, including transparent, violet, pink, red, blue, and green, which was in line with earlier findings (Bellas et al., 2016; Ory et al., 2017, 2018; Hossain et al., 2019). Fish of the clade Clupeiformes, such as sardines, have been found to inadvertently consume MPs that mimic their native diet when they transition from filter feeders to carnivores (Collard et al., 2017). However, colored MPs change to transparent MPs throughout the process of transporting them to aquatic ecosystems (Kalogerakis et al., 2017; Wu et al., 2018). The prevalence of colored MPs suggests that they may have synthetic and organic sources, which need for in-depth and long-term research.
In this study, smaller sized MPs were predominant which were more bio-available. This might happen due to the smaller body weight (59.14 ± 9.4 g), and limited food selectivity (Cole et al., 2013). As opposed to this, the larger size MPs predominated in Bombay-ducks, which may be related to their higher body weight (129.64 ± 23.92 g) and carnivorous feeding habits of that fish (Hossain et al., 2019). In contrast to muscle, the gills and DT recorded comparatively bigger sized MPs. This could occur because bigger plastic particles can enter the DT readily (Abbasi et al., 2018). The presence of MPs in S. silondia provides the specific indication about the possible consequences of MPs in the marine and estuarine environment of Bangladesh, and necessitate future study across a larger variety of species and habitats.
3.3 Polymer characterization
Figure 4 depicts the FTIR spectra of polymers found in MPs in the studied fish. When MPs samples were extracted for FTIR analysis, three different forms of polymers were identified, with PET, PE, and PP appearing in decreasing order. The predominant polymer identified in the samples was PET, constituting 44.5% of the total samples. PE accounted for 33.3% of the MPs found in S. silondia fish, while PP was observed to be the least prevalent at 22.2%. Due to the decadence, deterioration, and aging of MPs over time, certain similar peaks of the detected polymers were missing from the spectrum (Sathish et al., 2020; Banik et al., 2022; Nur et al., 2022). However, the detected plastic polymers were widely distributed in the coastal habitats of Bangladesh (Banik et al., 2022; Nur et al., 2022). The most common polymer, PET, is utilized in household waste and plastic piping, both of which can potentially enter aquatic environments via surface runoff and river discharge. PE may have been utilized for food packaging as well as leveling purposes in daily-used shampoo, containers of soap, oil, and other cosmetic goods (Wu et al., 2021). Generally, PP finds widespread global use in plastic containers, food and beverage packaging, and wraps. However, plastic waste left by visitors and residents contributes to marine plastic pollution in this area. Further research is essential to identify specific sources of MPs, and implement measures to curb their pollution in aquatic ecosystems.
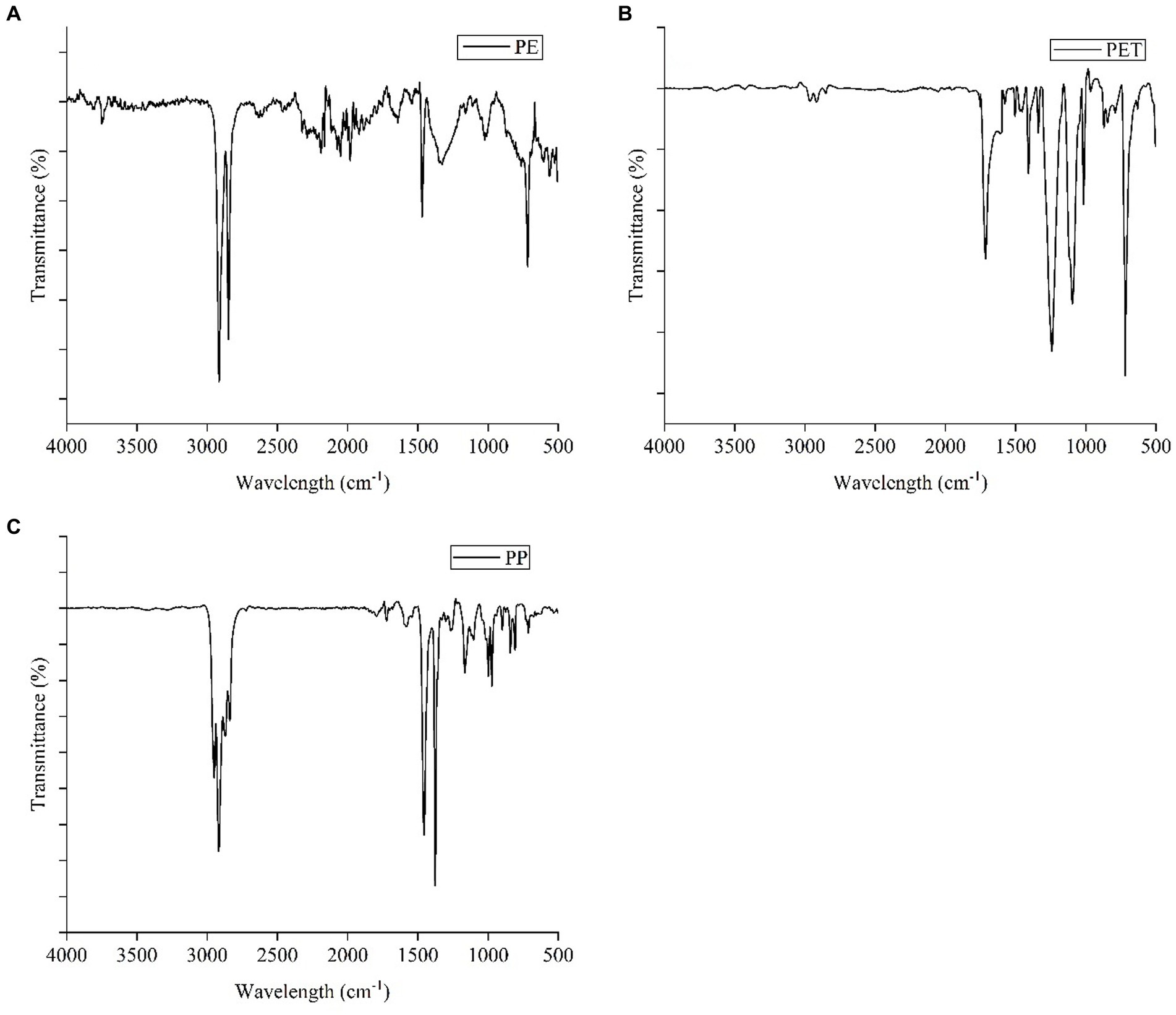
Figure 4. Polymeric characterization of MPs from different tissues of S. silondia: (A) PE, (B) PET and (C) PP.
3.4 Assessment of contamination level by CF and PLI values
The utilization of contamination factor (CF) is crucial in evaluating microplastic pollution as it provides a quantitative measure of the extent to which an organism or environment is affected. By considering factors such as concentration and distribution, contamination factors offer valuable insights for informed decision-making and effective mitigation strategies in addressing the growing concern of microplastic pollution. In this study, the CF values of digestive tract (2.47) and fish muscle (2.6) suggested that these fish organs were moderately contaminated level (1 < CF < 3) with MPs but gill (3.2) exhibited considerable contamination (3 < CF < 6).
The adoption of Pollution Load Index (PLI) is essential for assessing microplastic pollution as it integrates multiple parameters, providing a comprehensive overview of the pollution magnitude. This holistic approach aids in understanding the cumulative impact of various microplastic sources, facilitating targeted interventions and sustainable management practices for environmental conservation. PLI is used to quantify the extent of MP pollution (Pan et al., 2021; Ranjani et al., 2021). The PLI values of the fish organ samples were > 1, implying that the S. silondia fish were contaminated. PLI scores were assigned in the following descending order: gill (2.75) > muscle (2.22) > digestive tract (2.17). Based on the ratio between the occurrence of MPs and the background value, it appears that the polymer type of MPs has a minimal impact on the PLI (Nur et al., 2022). However, the presence of MPs in sea water is influenced by human activities such as industrialization, water transportation, fishing and so on (Jang et al., 2020; Pan et al., 2021). MPs abundance is also closely associated to socioeconomic aspects like as population density, industrialization level, economic development status, human lifestyle, and intensity of fishing (Lechner et al., 2014). A rise in the number of MPs was caused by the degree of economic development in some areas, such as those with a significant tourism industry (Xiong et al., 2018). Lower Meghna River estuary is a major fishing base in Bangladesh, and plastics-based aquaculture floating rafts are widely used within the bay. As a result, fishery farming becomes a substantial source of MPs in this area, influencing the value of PLI.
4 Conclusion
The present study revealed microplastics contamination from a commercially-important fish species from lower Meghna River estuary. The average MP abundance in S. silondia was 13.20 ± 6.55 items/individual, which was higher than several other species reported earlier. MPs were detected in the digestive tract (DT), gills, and muscle tissues, with higher abundance observed in the DT compared to the gills and muscles. Additionally, the presence of MPs showed a positive correlation with the weight of the digestive tract (DT), gills, and the overall body weight of the fish. This suggests that individuals with greater body weight tend to accumulate higher concentrations of MPs in their gills, digestive tract, and muscles. This implies that fish habitat and feeding behavior play crucial roles in determining the presence of MPs in their bodies. Additionally, the majority of the particles were fibrous, violet and pink in color, and smaller in size (<0.5 mm), characteristics typically associated with the synthetic origin, breakdown, and degradation of fishing equipment such as plastic lines, ropes, and nets. MPs predominance in DT suggests that fish may deliberately consume MPs because they resemble natural meals or may be mistaken for prey, or may be transferred trophically as microplastics. The ecological risk of S. silondia ingesting MPs was indicated by the PLI and CF value. These findings indicate that S. silondia has been subjected to microplastic (MP) contamination in its natural habitat, raising concerns about the potential health risks posed to humans who consume it, despite the current lack of direct evidence of harmful effects of MPs on human health. The study emphasizes the need for further research to better understand the mechanisms underlying in high prevalence of microplastic ingestion as well as to evaluate the risks associated with consuming estuarine fish that have ingested microplastics, and their potential negative effects on human health.
Data availability statement
The original contributions presented in the study are included in the article/Supplementary material, further inquiries can be directed to the corresponding authors.
Ethics statement
The animal study was approved by Research Cell, Noakhali Science and Technology University. The study was conducted in accordance with the local legislation and institutional requirements.
Author contributions
MH: Conceptualization, Supervision, Writing – original draft. FP: Formal analysis, Writing – original draft. MA: Data curation, Formal analysis, Investigation, Writing – original draft. A-AN: Investigation, Methodology, Writing – original draft. PB: Data curation, Investigation, Methodology, Writing – original draft. PS: Writing – review & editing. BP: Data curation, Funding acquisition, Resources, Software, Writing – review & editing. TA: Validation, Writing – review & editing. JY: Writing – review & editing.
Funding
The author(s) declare that financial support was received for the research, authorship, and/or publication of this article. This study was supported by Researchers Supporting Project Number (RSP2024R144), King Saud University, Riyadh, Saudi Arabia.
Acknowledgments
The authors would like to extend their sincere appreciation to volunteers who helped during sampling, and the Researchers Supporting Project Number (RSP2024R144), King Saud University, Riyadh, Saudi Arabia.
Conflict of interest
The authors declare that the research was conducted in the absence of any commercial or financial relationships that could be construed as a potential conflict of interest.
The author(s) declared that they were an editorial board member of Frontiers, at the time of submission. This had no impact on the peer review process and the final decision.
Publisher’s note
All claims expressed in this article are solely those of the authors and do not necessarily represent those of their affiliated organizations, or those of the publisher, the editors and the reviewers. Any product that may be evaluated in this article, or claim that may be made by its manufacturer, is not guaranteed or endorsed by the publisher.
Supplementary material
The Supplementary material for this article can be found online at: https://www.frontiersin.org/articles/10.3389/fsufs.2024.1372059/full#supplementary-material
Footnotes
References
Abbasi, S., Soltani, N., Keshavarzi, B., Moore, F., Turner, A., and Hassanaghaei, M. (2018). Microplastics in different tissues of fish and prawn from the Musa estuary, Persian gulf. Chemosphere 205, 80–87. doi: 10.1016/j.chemosphere.2018.04.076
Akhbarizadeh, R., Moore, F., and Keshavarzi, B. (2018). Investigating a probable relationship between microplastics and potentially toxic elements in fish muscles from northeast of Persian Gulf. Environmental Pollution, 232, 154–163.
Avio, C. G., Gorbi, S., and Regoli, F. (2015). Experimental development of a new protocol for extraction and characterization of microplastics in fish tissues: first observations in commercial species from Adriatic Sea. Mar. Environ. Res. 111, 18–26. doi: 10.1016/j.marenvres.2015.06.014
Baalkhuyur, F. M., Bin Dohaish, E. J. A., Elhalwagy, M. E. A., Alikunhi, N. M., AlSuwailem, A. M., Røstad, A., et al. (2018). Microplastic in the gastrointestinal tract of fishes along the Saudi Arabian Red Sea coast. Mar. Pollut. Bull. 131, 407–415. doi: 10.1016/j.marpolbul.2018.04.040
Banik, P., Anisuzzaman, M., Bhattacharjee, S., Marshall, D. J., Yu, J., Nur, A. A. U., et al. (2024). Quantification, characterization and risk assessment of microplastics from five major estuaries along the northern Bay of Bengal coast. Environmental Pollution, 342:123036.
Baechler, B. R., De Frond, H., Dropkin, L., Leonard, G. H., Proano, L., and Mallos, N. J. (2024). Public awareness and perceptions of ocean plastic pollution and support for solutions in the United States. Front. Mar. Sci. 10:1323477. doi: 10.3389/fmars.2023.1323477
Banik, P., Hossain, M. B., Nur, A. A. U., Choudhury, T. R., Liba, S. I., Yu, J., et al. (2022). Microplastics in sediment of Kuakata Beach, Bangladesh: occurrence, spatial distribution, and risk assessment. Front. Mar. Sci. 9:348. doi: 10.3389/fmars.2022.860989
Bellas, J., Martínez-Armental, J., Martínez-Cámara, A., Besada, V., and Martínez-Gómez, C. (2016). Ingestion of microplastics by demersal fish from the Spanish Atlantic and Mediterranean coasts. Mar. Pollut. Bull. 109, 55–60. doi: 10.1016/j.marpolbul.2016.06.026
Bessa, F., Barría, P., Neto, J. M., Frias, J. P. G. L., Otero, V., Sobral, P., et al. (2018). Occurrence of microplastics in commercial fish from a natural estuarine environment. Mar. Pollut. Bull. 128, 575–584. doi: 10.1016/j.marpolbul.2018.01.044
Browne, M. A., Crump, P., Niven, S. J., Teuten, E., Tonkin, A., Galloway, T., et al. (2011). Accumulation of microplastic on shorelines woldwide: sources and sinks. Environ. Sci. Technol. 45, 9175–9179. doi: 10.1021/es201811s
Cannon, S. M. E., Lavers, J. L., and Figueiredo, B. (2016). Plastic ingestion by fish in the southern hemisphere: a baseline study and review of methods. Mar. Pollut. Bull. 107, 286–291. doi: 10.1016/j.marpolbul.2016.03.057
Cheung, P. K., Cheung, L. T. O., and Fok, L. (2016). Seasonal variation in the abundance of marine plastic debris in the estuary of a subtropical macro-scale drainage basin in South China. Sci. Total Environ. 562, 658–665. doi: 10.1016/j.scitotenv.2016.04.048
Cheung, L. T. O., Lui, C. Y., and Fok, L. (2018). Microplastic contamination of wild and captive Flathead Grey mullet (Mugil cephalus). Int. J. Environ. Res. Public Health 15:597. doi: 10.3390/ijerph15040597
Cole, M., Lindeque, P., Fileman, E., Halsband, C., Goodhead, R., Moger, J., et al. (2013). Microplastic ingestion by zooplankton. Environ. Sci. Technol. 47, 6646–6655. doi: 10.1021/es400663f
Cole, M., Lindeque, P., Halsband, C., and Galloway, T. S. (2011). Microplastics as contaminants in the marine environment: a review. Mar. Pollut. Bull. 62, 2588–2597. doi: 10.1016/j.marpolbul.2011.09.025
Collard, F., Gilbert, B., Compère, P., Eppe, G., Das, K., Jauniaux, T., et al. (2017). Microplastics in livers of European anchovies (Engraulis encrasicolus, L.). Environ. Pollut. 229, 1000–1005. doi: 10.1016/j.envpol.2017.07.089
Compa, M., Ventero, A., Iglesias, M., and Deudero, S. (2018). Ingestion of microplastics and natural fibres in Sardina pilchardus (Walbaum, 1792) and Engraulis encrasicolus (Linnaeus, 1758) along the Spanish Mediterranean coast. Mar. Pollut. Bull. 128, 89–96. doi: 10.1016/j.marpolbul.2018.01.009
Coppock, R. L., Cole, M., Lindeque, P. K., Queirós, A. M., and Galloway, T. S. (2017). A small-scale, portable method for extracting microplastics from marine sediments. Environ. Pollut. 230, 829–837. doi: 10.1016/j.envpol.2017.07.017
Crawford, C. B., and Quinn, B. (2017). “Plastic production, waste and legislation” in Microplastic Pollutants. eds. C. B. Crawford and B. Quinn (Amsterdam: Elsevier Science), 39–56.
de Vries, A. N., Govoni, D., Árnason, S. H., and Carlsson, P. (2020). Microplastic ingestion by fish: body size, condition factor and gut fullness are not related to the amount of plastics consumed. Mar. Pollut. Bull. 151:110827. doi: 10.1016/j.marpolbul.2019.110827
De Witte, B., Devriese, L., Bekaert, K., Hoffman, S., Vandermeersch, G., Cooreman, K., et al. (2014). Quality assessment of the blue mussel (Mytilus edulis): comparison between commercial and wild types. Mar. Pollut. Bull. 85, 146–155. doi: 10.1016/j.marpolbul.2014.06.006
Desforges, J. P. W., Galbraith, M., and Ross, P. S. (2015). Ingestion of microplastics by zooplankton in the Northeast Pacific Ocean. Arch. Environ. Contam. Toxicol 69, 320–330. doi: 10.1007/s00244-015-0172-5
Devriese, L. I., van der Meulen, M. D., Maes, T., Bekaert, K., Paul-Pont, I., Frère, L., et al. (2015). Microplastic contamination in brown shrimp (Crangon crangon, Linnaeus 1758) from coastal waters of the southern North Sea and channel area. Mar. Pollut. Bull. 98, 179–187. doi: 10.1016/j.marpolbul.2015.06.051
Diepens, N. J., and Koelmans, A. A. (2018). Accumulation of plastic debris and associated contaminants in aquatic food webs. Environ. Sci. Technol. 52, 8510–8520. doi: 10.1021/acs.est.8b02515
Eriksen, M., Liboiron, M., Kiessling, T., Charron, L., Alling, A., Lebreton, L., et al. (2018). Microplastic sampling with the AVANI trawl compared to two neuston trawls in the bay of Bengal and South Pacific. Environ. Pollut. 232, 430–439. doi: 10.1016/j.envpol.2017.09.058
Frias, J. P. G. L., and Nash, R. (2019). Microplastics: finding a consensus on the definition. Mar. Pollut. Bull. 138, 145–147. doi: 10.1016/j.marpolbul.2018.11.022
Ghosh, G. C., Akter, S. M., Islam, R. M., Habib, A., Chakraborty, T. K., Zaman, S., et al. (2021). Microplastics contamination in commercial marine fish from the bay of Bengal. Reg. Stud. Mar. Sci. 44:101728. doi: 10.1016/j.rsma.2021.101728
Güven, O., Gökdağ, K., Jovanović, B., and Kıdeyş, A. E. (2017). Microplastic litter composition of the Turkish territorial waters of the Mediterranean Sea, and its occurrence in the gastrointestinal tract of fish. Environ. Pollut. 223, 286–294. doi: 10.1016/j.envpol.2017.01.025
Harris, L. S., Gill, H., and Carrington, E. (2021). Microplastic changes the sinking and resuspension rates of marine mussel biodeposits. Marine Pollution Bulletin, 165:112165.
Hasan, M. F., Molla, A. H., Ahsan, M. S., and Alam, M. T. (2002). Physicochemical properties and fatty acid distribution pattern in lipids of Eutropiichthys vacha hamilton-buchanan. Fam. Schilbeidae 5, 696–698. doi: 10.3923/pjbs.2002.696.698
Hidalgo-Ruz, V., Gutow, L., Thompson, R. C., and Thiel, M. (2012). Microplastics in the marine environment: a review of the methods used for identification and quantification. Environ. Sci. Technol. 46, 3060–3075. doi: 10.1021/es2031505
Hossain, M. J., AftabUddin, S., Akhter, F., Nusrat, N., Rahaman, A., Sikder, M. N. A., et al. (2022). Surface water, sediment, and biota: the first multi-compartment analysis of microplastics in the Karnafully river, Bangladesh, Mar. Pollut. Bull 180, 113820. doi: 10.1016/j.marpolbul.2022.113820
Hossain, M. B., Banik, P., Nur, A. A. U., and Rahman, T. (2021). Abundance and characteristics of microplastics in sediments from the world’s longest natural beach, Cox’s bazar, Bangladesh. Mar. Pollut. Bull. 163:111956. doi: 10.1016/j.marpolbul.2020.111956
Hossain, M. S., Rahman, M. S., Uddin, M. N., Sharifuzzaman, S. M., Chowdhury, S. R., Sarker, S., et al. (2020). Microplastic contamination in Penaeid shrimp from the Northern Bay of Bengal. Chemosphere 238:124688. doi: 10.1016/j.chemosphere.2019.124688
Hossain, M. S., Sobhan, F., Uddin, M. N., Sharifuzzaman, S. M., Chowdhury, S. R., Sarker, S., et al. (2019). Microplastics in fishes from the Northern Bay of Bengal. Sci. Total Environ. 690, 821–830. doi: 10.1016/j.scitotenv.2019.07.065
Hossain, M. B., Sultana, J., Pingki, F. H., Nur, A. A. U., Mia, M. S., Bakar, M. A., et al. (2023). Accumulation and contamination assessment of heavy metals in sediments of commercial aquaculture farms from a coastal area along the northern bay of Bengal. Front. Environ. Sci. 11:1148360. doi: 10.3389/fenvs.2023.1148360
IUCN Bangladesh, (2000). Red Book of Threatened Fishes of Bangladesh. Bangladesh IUCN-the World Conservation Union. 116.
Jabeen, K., Su, L., Li, J., Yang, D., Tong, C., Mu, J., et al. (2017). Microplastics and mesoplastics in fish from coastal and fresh waters of China. Environ. Pollut. 221, 141–149. doi: 10.1016/j.envpol.2016.11.055
Jang, M., Shim, W. J., Cho, Y., Han, G. M., Song, Y. K., and Hong, S. H. (2020). A close relationship between microplastic contamination and coastal area use pattern. Water Res. 171:115400. doi: 10.1016/j.watres.2019.115400
Jung, M. R., Horgen, F. D., Orski, S. V., Rodriguez, C. V., Beers, K. L., Balazs, G. H., et al. (2018). Validation of ATR FT-IR to identify polymers of plastic marine debris, including those ingested by marine organisms. Mar. Pollut. Bull. 127, 704–716. doi: 10.1016/j.marpolbul.2017.12.061
Kalogerakis, N., Karkanorachaki, K., Kalogerakis, G. C., Triantafyllidi, E. I., Gotsis, A. D., Partsinevelos, P., et al. (2017). Microplastics generation: onset of fragmentation of polyethylene films in marine environment mesocosms. Front. Mar. Sci. 4:84. doi: 10.3389/fmars.2017.00084
Karami, A., Golieskardi, A., Choo, C. K., Romano, N., Ho, Y. B., and Salamatinia, B. (2017). A high-performance protocol for extraction of microplastics in fish. Science of the total environment, 578, 485–494. doi: 10.1016/j.scitotenv.2016.10.213
Kolandhasamy, P., Su, L., Li, J., Qu, X., Jabeen, K., and Shi, H. (2018). Adherence of microplastics to soft tissue of mussels: a novel way to uptake microplastics beyond ingestion. Sci. Total Environ. 610-611, 635–640. doi: 10.1016/j.scitotenv.2017.08.053
Laglbauer, B. J. L., Franco-Santos, R. M., Andreu-Cazenave, M., Brunelli, L., Papadatou, M., Palatinus, A., et al. (2014). Macrodebris and microplastics from beaches in Slovenia. Mar Pollut Bull 89, 356–366. doi: 10.1016/j.marpolbul.2014.09.036
Lechner, A., Keckeis, H., Lumesberger-Loisl, F., Zens, B., Krusch, R., Tritthart, M., et al. (2014). The Danube so colourful: a potpourri of plastic litter outnumbers fish larvae in Europe’s second largest river. Environ. Pollut. 188, 177–181. doi: 10.1016/j.envpol.2014.02.006
Li, J., Qu, X., Su, L., Zhang, W., Yang, D., Kolandhasamy, P., et al. (2016). Microplastics in mussels along the coastal waters of China. Environ. Pollut. 214, 177–184. doi: 10.1016/j.envpol.2016.04.012
Li, J., Yang, D., Li, L., Jabeen, K., and Shi, H. (2015). Microplastics in commercial bivalves from China. Environ. Pollut. 207, 190–195. doi: 10.1016/j.envpol.2015.09.018
Lin, L., Ma, L. S., Li, H. X., Pan, Y. F., Liu, S., Zhang, L., et al. (2020). Low level of microplastic contamination in wild fish from an urban estuary. Mar. Pollut. Bull. 160:111650. doi: 10.1016/j.marpolbul.2020.111650
Lots, F. A. E., Behrens, P., Vijver, M. G., Horton, A. A., and Bosker, T. (2017). A large-scale investigation of microplastic contamination: abundance and characteristics of microplastics in European beach sediment. Mar. Pollut. Bull. 123, 219–226. doi: 10.1016/j.marpolbul.2017.08.057
Martinho, F., Leitão, R., Viegas, I., Dolbeth, M., Neto, J. M., Cabral, H. N., et al. (2007). The influence of an extreme drought event in the fish community of a southern Europe temperate estuary. Estuar. Coast. Shelf Sci. 75, 537–546. doi: 10.1016/j.ecss.2007.05.040
Masura, J., Baker, J., Foster, G., and Arthur, C., (2015). Laboratory Methods for the Analysis of Microplastics in the Marine Environment: Recommendations for Quantifying Synthetic Particles in Waters and Sediments. United States: NOAA technical memorandum NOS-OR&R. Available at: https://repository.library.noaa.gov/view/noaa/10296
Mathalon, A., and Hill, P. (2014). Microplastic fibers in the intertidal ecosystem surrounding Halifax Harbor, Nova Scotia. Mar. Pollut. Bull. 81, 69–79. doi: 10.1016/j.marpolbul.2014.02.018
Napper, I. E., Baroth, A., Barrett, A. C., Bhola, S., Chowdhury, G. W., Davies, B. F. R., et al. (2021). The abundance and characteristics of microplastics in surface water in the transboundary Ganges River. Environ. Pollut. 274:116348. doi: 10.1016/j.envpol.2020.116348
Noda, I., Dowrey, A. E., Haynes, J. L., and Marcott, C. (2007). “Group frequency assignments for major infrared bands observed in common synthetic polymers” in Physical Properties of Polymers Handbook. ed. J. E. Mark (New York, NY: Springer), 395–406.
Nur, A. A. U., Hossain, M. B., Banik, P., Choudhury, T. R., Liba, S. I., Umamaheswari, S., et al. (2022). Microplastic contamination in processed and unprocessed sea salts from a developing country and potential risk assessment. Chemosphere 308:136395. doi: 10.1016/j.chemosphere.2022.136395
Ory, N., Chagnon, C., Felix, F., Fernández, C., Ferreira, J. L., Gallardo, C., et al. (2017). Low prevalence of microplastic contamination in planktivorous fish species from the Southeast Pacific Ocean. Mar Pollut Bull 127, 211–216. doi: 10.1016/j.marpolbul.2017.12.016
Ory, N. C., Gallardo, C., Lenz, M., and Thiel, M. (2018). Capture, swallowing, and egestion of microplastics by a planktivorous juvenile fish. Environ. Pollut. 240, 566–573. doi: 10.1016/j.envpol.2018.04.093
Pan, Z., Zhang, C., Wang, S., Sun, D., Zhou, A., Xie, S., et al. (2021). Occurrence of microplastics in the gastrointestinal tract and gills of fish from Guangdong, South China. J. Mar. Sci. Eng. 9:981. doi: 10.3390/jmse9090981
Peters, C. A., and Bratton, S. P. (2016). Urbanization is a major influence on microplastic ingestion by sunfish in the Brazos River basin, Central Texas, USA. Environ. Pollut. 210, 380–387. doi: 10.1016/j.envpol.2016.01.018
Rahman, M. S., Hossain, M. S., Ahmed, M. K., Akther, S., Jolly, Y. N., Akhter, S., et al. (2019). Assessment of heavy metals contamination in selected tropical marine fish species in Bangladesh and their impact on human health. Environ. Nanotechnol. Monit. Manag. 11:100210. doi: 10.1016/j.enmm.2019.100210
Ranjani, M., Veerasingam, S., Venkatachalapathy, R., Mugilarasan, M., Bagaev, A., Mukhanov, V., et al. (2021). Assessment of potential ecological risk of microplastics in the coastal sediments of India: a meta-analysis. Marine Pollution Bulletin, 163:111969.
Romeo, T., Pietro, B., Pedà, C., Consoli, P., Andaloro, F., and Fossi, M. C. (2015). First evidence of presence of plastic debris in stomach of large pelagic fish in the Mediterranean Sea. Mar. Pollut. Bull. 95, 358–361. doi: 10.1016/j.marpolbul.2015.04.048
Sathish, M. N., Jeyasanta, I., and Patterson, J. (2020). Occurrence of microplastics in epipelagic and mesopelagic fishes from Tuticorin, Southeast coast of India. Sci. Total Environ 720:137614. doi: 10.1016/j.scitotenv.2020.137614
Sharif, A. S. M., Bakar, M. A., and Bhuyan, M. S. (2017). Assessment of water quality of the lower Meghna river estuary using multivariate analyses and RPI. Intern. J Chem. Pharm. Technol 2, 57–73.
Siddique, M. A. M., Uddin, A., Rahman, S. M. A., Rahman, M., Islam, M. S., and Kibria, G. (2022). Microplastics in an anadromous national fish, Hilsa shad Tenualosa ilisha from the bay of Bengal, Bangladesh. Mar. Pollut. Bull. 174:113236. doi: 10.1016/j.marpolbul.2021.113236
Steer, M., Cole, M., Thompson, R. C., and Lindeque, P. K. (2017). Microplastic ingestion in fish larvae in the western English Channel. Environ. Pollut. 226, 250–259. doi: 10.1016/j.envpol.2017.03.062
Teng, J., Wang, Q., Ran, W., Wu, D., Liu, Y., Sun, S., et al. (2019). Microplastic in cultured oysters from different coastal areas of China. Sci. Total Environ. 653, 1282–1292. doi: 10.1016/j.scitotenv.2018.11.057
Tomlinson, D. L., Wilson, J. G., Harris, C. R., and Jeffrey, D. W. (1980). 1980. Problems in the assessment of heavy-metal levels in estuaries and the formation of a pollution index. Helgoländer Meeresun. 33, 566–575. doi: 10.1007/BF02414780
Wu, F., Misra, M., and Mohanty, A. K. (2021). Challenges and new opportunities on barrier performance of biodegradable polymers for sustainable packaging. Prog. Polym. Sci 117:101395. doi: 10.1016/j.progpolymsci.2021.101395
Wu, C., Zhang, K., and Xiong, X. (2018). Microplastic pollution in inland waters focusing on Asia. Handb. Environ. Chem. 58, 85–99. doi: 10.1007/978-3-319-61615-5_5
Xiong, X., Zhang, K., Chen, X., Shi, H., Luo, Z., and Wu, C. (2018). Sources and distribution of microplastics in China’s largest inland lake – Qinghai Lake. Environ. Pollut. 235, 899–906. doi: 10.1016/j.envpol.2017.12.081
Yagi, M., Kobayashi, T., Maruyama, Y., Hoshina, S., Masumi, S., Aizawa, I., et al. (2022). Microplastic pollution of commercial fishes from coastal and offshore waters, Japan. Mar. Pollut. Bull. 174:113304. doi: 10.1016/j.marpolbul.2021.113304
Zitouni, N., Bousserrhine, N., Belbekhouche, S., Missawi, O., Alphonse, V., Boughatass, I., et al. (2020). First report on the presence of small microplastics (≤ 3 μm) in tissue of the commercial fish Serranus scriba (Linnaeus. 1758) from Tunisian coasts and associated cellular alterations. Environ. Pollut. 263:114576. doi: 10.1016/j.envpol.2020.114576
Keywords: microplastics, estuarine catfish, human health, risk assessment, Meghna estuary
Citation: Hossain MB, Pingki FH, Azad MAS, Nur A-AU, Banik P, Sarker PK, Paray BA, Arai T and Yu J (2024) Accumulation, tissue distribution, health hazard of microplastics in a commercially important cat fish, Silonia silondia from a tropical large-scale estuary. Front. Sustain. Food Syst. 8:1372059. doi: 10.3389/fsufs.2024.1372059
Edited by:
Matthew Chidozie Ogwu, Appalachian State University, United StatesReviewed by:
Mohammad Mahfujul Haque, Bangladesh Agricultural University, BangladeshMd. Kamal Hossain, Bangladesh Council of Scientific and Industrial Research (BCSIR), Bangladesh
Copyright © 2024 Hossain, Pingki, Azad, Nur, Banik, Sarker, Paray, Arai and Yu. This is an open-access article distributed under the terms of the Creative Commons Attribution License (CC BY). The use, distribution or reproduction in other forums is permitted, provided the original author(s) and the copyright owner(s) are credited and that the original publication in this journal is cited, in accordance with accepted academic practice. No use, distribution or reproduction is permitted which does not comply with these terms.
*Correspondence: M. Belal Hossain, YmVsYWwuaG9zc2FpbkBuc3R1LmVkdS5iZA==; Pallab Kumer Sarker, cHNhcmtlckB1Y3NjLmVkdQ==