- 1Centro Agronómico Tropical de Investigación y Enseñanza (CATIE), Turrialba, Costa Rica
- 2Ecole Nationale Supérieure Agronomique de Toulouse, Auzeville-Tolosane, France
- 3CIRAD, UMR SENS, Univ Montpellier, Montpellier, France
- 4CIRAD, UMR PHIM, Montpellier, France
- 5PHIM, Univ Montpellier, CIRAD, INRAE, Institut Agro, IRD, Montpellier, France
- 6CIRAD, AGAP Institut, Montpellier, France
- 7AGAP Institut, Univ Montpellier, CIRAD, INRAE, Institut Agro, Montpellier, France
Introduction: Insect pests cause important crop production losses worldwide. Their distribution and movement are affected by climate and land use change and agriculture intensification. Site colonization by insect pests is dependent on pest dispersal capability, the availability of resources, the presence of competitors or predators, the weather conditions and the characteristics of the surrounding landscape. Movement of pests between the plots might be considered in pest management strategies to counterbalance the traditional plot oriented strategies. In this study, our objective was to provide evidence of the movement of the coffee berry borer (CBB), the most important pest in coffee cultivation, from neighboring coffee plantations to adjacent land uses at different time periods of the coffee production cycle.
Methods: For 10 months we captured the CBB with funnel traps in 13 coffee plots that had an interface with forests, pastures, and abandoned coffee plantations in Costa Rica. At each interface, we established three transects with a minimum distance of 50 m between them, in the direction of the wind. Within each transect, we placed four traps 20 m apart. We fitted generalized linear mixed models to evaluate the relationship between CBB captures and the type of interface, the position of the trap, wind velocity, rainfall, temperature and relative humidity, and their interactions.
Results: Our findings suggest that CBB moves into adjacent land uses when the coffee resource in the plot is limited. This effect varies according to the interface and the position of the trap. We also found an interaction between the interface and the position of the trap with the wind and relative humidity.
Discussion: Our findings suggest that movement of the CBB partly depends upon the adjacent land uses. The forest creates a barrier to CBB movement and may prevent the transport of the CBB considering the action of the wind speed. The pasture may facilitate movement of the CBB through the action of the wind speed and infest coffee plantations beyond its dispersal capacity. Our results support the importance of considering the landscape context when developing CBB management strategies.
1 Introduction
The dispersion of insect pests results from an interaction among intrinsic biological characteristics of the species, biotic (availability of resources), and abiotic conditions of their environment. Successful colonization of sites by agricultural insect pests depends on the dispersal distance capacity of the individuals, the surrounding landscape characteristics (hostility of the matrix and energy wear), the availability of resources, the presence of competitors, predators, and the climatic conditions (Tischendorf and Fahrig, 2000; Laska et al., 2022). Most insect pests locate their hosts through visual and olfactory cues. A disruption of these signals can therefore reduce the incidence of attack on adjacent crops (O’Rourke and Petersen, 2017). Understanding insect pest dispersal strategies and the role of the landscape environment may shed light on control intervention (Hernández-Andrade et al., 2019).
The coffee berry borer (Hypothenemus hampei) is the most destructive pest in coffee cultivation, causing significant damage to the fruit and resulting in economic losses for farmers (Castaño et al., 2005). CBB is present in all coffee-producing regions (Baker et al., 1992; Damon, 2000; Dickson et al., 2019) across the world likely due to passive transport by wind, workers, vehicles, and commerce or by harvest-time workers moving from plantation to plantation (Damon, 2000). Once established in the plantation, the CBB female is the only one that can disperse from the infested berries by flying or walking to colonize new fruits (Damon, 2000; Benavides et al., 2012). The main peaks of the CBB dispersion by flight usually occur during the dry season, after rainfall and before the harvest period, induced by increased humidity and high temperatures (Baker et al., 1992; Mathieu et al., 1997b) and by olfactory stimuli mainly volatiles like alcohols, emitted by fruits during the ripening process (Mathieu et al., 2001). Approximately 10% of the fruits remain on the plant and the ground after fruit harvest (Chamorro Trejos et al., 1995). The CBB individuals can survive within these residual fruits, waiting for optimal conditions to emerge. They will partly constitute the new population that will colonize the new fruits of the following season of production.
This pest is known to have a limited flying capacity, but only a few studies have set up specific experiments to evaluate it. Studies assessing CBB movement within coffee plantations report up to 348 m under controlled conditions (Leefmans, 1923) and up to 30 m after coffee pruning in field conditions (Castaño et al., 2005). Using molecular markers, Gil et al. (2015) report that adults managed to fly up to 65 m. However, in the literature, there is little information about the movement of CBB in land uses adjacent to coffee plantations and how these land uses can facilitate or prevent the movement of the pest. On this matter, Johnson and Manoukis (2020) report a greater flight activity of the CBB in poorly managed coffee plantations than in abandoned coffee plantations and forests, while Olivas et al. (2011) demonstrate that CBB can move up to 140 m into adjacent land used from coffee plantations, being forests the land use showing the greatest friction to CBB movement. Moreover, other studies focus on the incidence of bored fruits rather than CBB movement, assessing the effect of landscape composition and configuration on the level of pest damage, and hypothesizing a barrier effect or the action of natural enemies in adjacent land uses (Avelino et al., 2012; Aristizábal and Metzger, 2019; Mosomtai et al., 2021; Vilchez-Mendoza et al., 2022).
In this study, we aimed to provide evidence on the movement of the CBB from coffee plantations to adjacent land uses and vice versa, throughout a coffee production cycle (from harvest to the appearance of the new fruits suitable for colonization). We hypothesize a spillover effect when coffee plantations do not have berries available (“hypothesis of absence of resources”) due to harvest and during the flowering period. In this case, the CBB tends to disperse into adjacent land uses to search for new resources outside the coffee plantation which would result in an increase in captures in traps far from the edge of the plantation. The dispersion can be prevented or facilitated by different land uses surrounding the coffee plot. Specifically, (1) the forest adjacent to coffee plots may act as a barrier for the dispersion of the CBB and may increase CBB predation, given its vegetation complexity and the presence of the CBB natural predators; (2) the pasture adjacent to coffee plots may facilitate the dispersion of the CBB, with few vegetative barriers and higher winds assisting in flight of the CBB; and (3) the abandoned coffee plots adjacent to coffee plots may contribute to an increase in CBB population, given that no management interventions are conducted in these plots.
2 Methods
2.1 Study area
The study was conducted from August 2020 to June 2021, considering the periods of greatest coffee berry borer dispersion (April–June) when the rainy season begins and the first cohorts of fruits begin to be suitable for colonization and after the harvest period (December–January). The study was set up in the Central Biological Volcanic Corridor of Talamanca (CBVCT), situated in southeastern Costa Rica on the Caribbean slopes of Central Cordillera (Figure 1). This area offers climatic conditions that favor the CBB development, with an annual rainfall of 2,700 mm and 22°C of temperature on average. Wind direction is oriented east/northeast all year with an annual average speed of 2.77 m/s (National Meteorology Institute, SA).
2.2 Site selection
In total, 13 coffee plots characterized by an interface with either secondary degraded forest (n = 6), pasture (n = 5), or abandoned coffee (n = 3) were selected. These land uses correspond to the dominant land uses in our study landscape. These plots were selected using an updated land use map for the study region (Amante, 2020), through verification on the field and based on allowance of farmers to work in their lots. The selected abandoned coffee plantations have been abandoned for at least 2 years. Information about the structure and composition of the vegetation in these adjacent land uses was not available.
At each interface, three transects were located with a minimum distance of 50 m between each other (the largest was 60 m). Four coffee berry borer traps (Brocap® + commercial attractants which is a mixture of methanol–ethanol 3:1 volume) separated by 20 m between each other were placed along each transect to avoid interference between the traps. The traps were located at a height of 1.20 meters from the ground. The first trap was located within the coffee plot, the second one at the border between the coffee plot and the adjacent land use, and the following two traps were located within the adjacent land use at 40 and 60 m from the first trap (considered as the source of CBB) (Figure 2). Transects were arranged in the direction of the prevailing wind (north-northeast or south, south-west). We also made sure that transects were not located in areas with slopes higher than 30 degrees.
2.3 Data collection and processing
Traps were checked every 12 to 15 days and brought back to the laboratory for processing. As counting CBB is a tedious and time-consuming task, we considered the use of artificial intelligence through signal detection algorithms to identify and count coffee berry borer specimens collected in traps. To do so, we trained Yolov5 (Jocher et al., 2021) core based on the Corigan pipeline, an image analysis pipeline developed for small object detection using high-resolution images (Tresson et al., 2019). For training and validation, we used high-resolution photographs of CBB, taken under laboratory conditions. The pipeline was trained using photographs only containing CBB specimens, photographs in which CBB was combined with other species of Scolytinae, and photographs also including litter. This allowed us to replicate the conditions in which CBB samples are usually collected in the field. Photographs were taken using a Panasonic DMC-G2 camera with a light aperture of 3.5, exposure time of 1/125 s, an ISO of 100, and a focal length of 14 mm and at a resolution of 4,000 × 2,672 pixels of 180 dpi and 24bit depth. We used 318 photographs (with 4,818 CBB) for training and 30 photographs (with 1,430 CBB) to validate.1 Photographs were split into small segments to facilitate the training of the pipeline (Figure 3).
We took three photographs of each sample collected in the field. When the amount of CBB in a sample was substantial, the sample was divided into three parts to photograph each of them and then add up the number of individuals detected. Throughout the study, this procedure was carried out only for two samples with a high number of collected individuals. For the analysis, we decided to work with the maximum number of CBB detected by the pipeline in any of the three photographs. A total of 9,404 photographs were processed with the pipeline.
2.4 Climate variables
Climatic variables were obtained from the databases The Prediction of Worldwide Energy Resources (POWER).2 The selected variables for this study were wind [speed (m/s) and direction (degrees)], relative humidity (%), daily precipitation (mm), and temperature (°C). As the traps were checked in a time interval between 12 and 15 days, we estimated the means of the temperature, the relative humidity, and the wind (speed and direction) and the accumulated precipitation for each time interval.
2.5 Data analysis
To reduce the effect of transects within each site, we estimated the average CBB capture by site, trap position (coffee, edge, 20 m and 40 m), and date of capture (10 months of samples). All our analyses were performed with this reduced data set.
Subsequently, to test differences between the type of adjacent land use (forest, pasture, or abandoned coffee), trap position, and its interaction on the CBB captures, we fitted a generalized linear mixed model (glmm) using library lme4 (Bates et al., 2015) in R (R Core Team, 2023). We used a negative binomial distribution (function glmer.nb), and as a random effect, we included the site (farm) and position of the trap as the traps were operated for 10 months (approximately 20 evaluations). Following a significant interaction between land use and trap position, we used Tukey’s multiple comparison tests, using the emmeans (Lenth, 2023) and multcomp (Hothorn et al., 2008) libraries in R (R Core Team, 2023).
To add the effect of CBB capture time and its interaction with adjacent land use and trap position, we fitted a generalized additive mixed model (gamm) with negative binomial distribution. This model is similar to the generalized linear model with the difference that the evaluation time consists of a cubic smoothing base function, and the random effects are the site (farm) and the position of the trap to consider variation between the samples. In addition, we added to the model an autoregressive order-1 (AR1) on the residuals to consider repeated measures across time. This model was built using the mgcv library (Wood, 2004).
Finally, to test the relationship between climatic variables and CBB catches, we fitted a generalized linear mixed model separately for each climatic variable. The structure of the model looks like the previously mentioned model, including the climatic variable as a fixed effect, and the possible interaction with the adjacent land use and the position of the trap are added as a random effect. The fit of the models was evaluated by examining diagnostic graphs of the residuals and predict. For the glmm models, we utilized the DHARMa library (Hartig, 2022), while for the gamm model, we employed the gam.check function from the mgcv library (Wood, 2004).
3 Results
3.1 Capture
In the 10 months that the traps operated at the different sites, we captured 148,913 individual coffee berry borers: 39% of the captures occurred in the traps that are within the coffee plantation at 20 m of the edge, 26% in the traps located at the edge, 18 and 17% in the trap located within the adjacent land uses at 20 m and 40 m of the edge, respectively; 36% of the CBB was captured in the traps located on the transects at the interface between coffee and forest, 29% in the traps located at the interface between coffee and abandoned coffee, and 35% was captured in the traps located at the interface between coffee and pasture. On average, in the coffee–forest transect 36.77 CBB were captured per trap per sampling session (approximately 12 days), in the coffee–abandoned coffee transect 59.51 CBB were captured per trap, and in the coffee–pasture transect 71.58 CBB were captured per trap per sampling session.
The maximum abundance of CBB captured per land use was recorded at the end of the dry season and after the first rains (Figures 4A) where there is an increase in relative humidity (Figures 4A) and temperature (Figure 4C) and reduce wind speed (Figure 4B). During the peak in CBB capture, pasture recorded the highest abundance of CBB in the traps (Figure 4D). The period where the lowest number of CBB was obtained was at the end of the harvest.
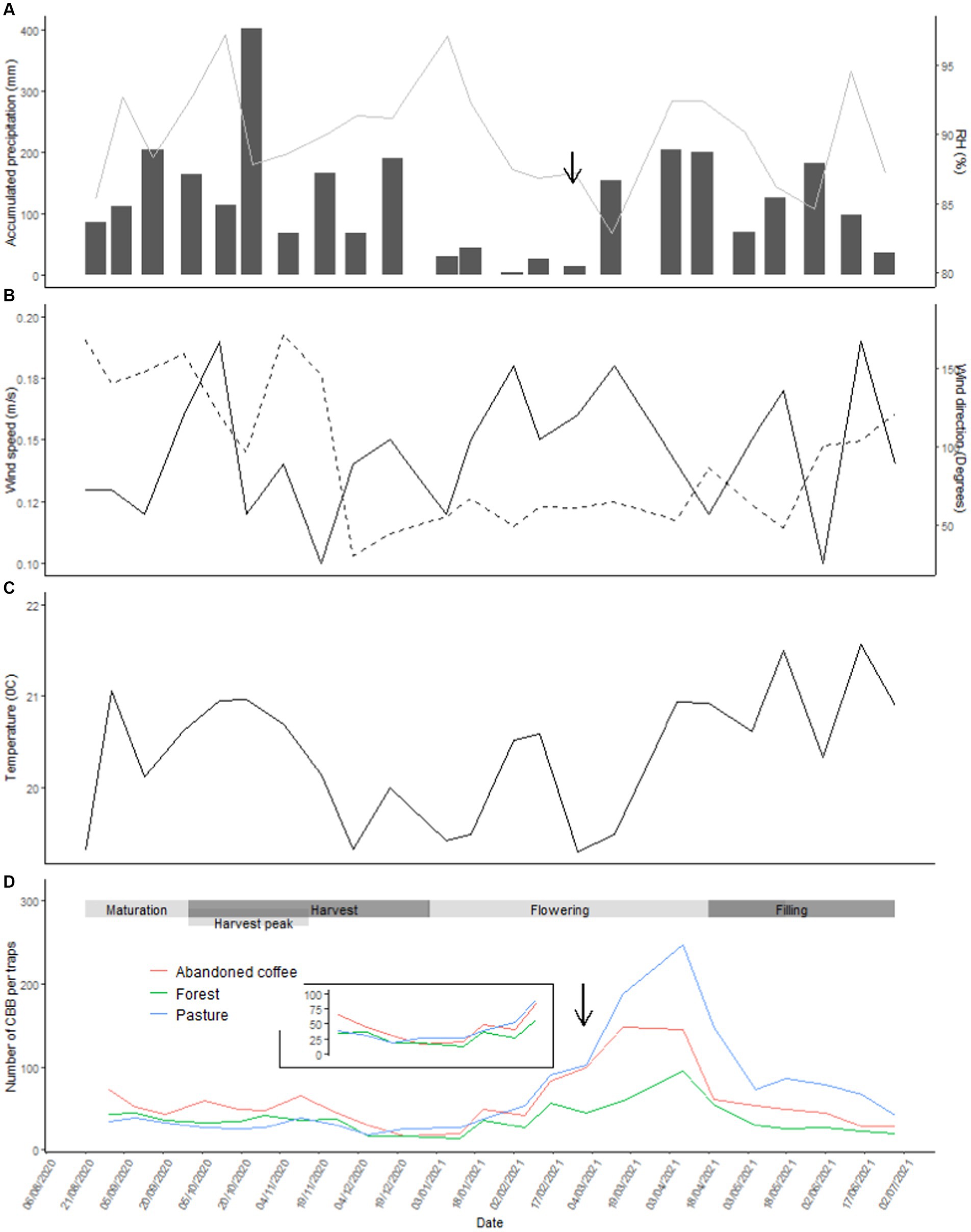
Figure 4. Capture of CBB throughout the sampling period and climatic variables: (A) accumulated precipitation (bars) and relative humidity (line), (B) wind speed and direction (dotted line), (C) temperature, and (D) average abundance of CBBs per land uses. The arrow indicates the time of year when there is a rapid increase in CBB catches.
3.2 Effect of land use and trap position
We found a significant interaction between adjacent land use and trap position (χ26 = 53.11, p < 0.0001; Supplementary material 1) on the number of captured CBB. The highest abundance of CBB was captured in the traps located inside the coffee plantation on the transect between coffee and pasture. The traps located on the edges did not show a different abundance of CBB regardless of the adjacent land uses (Table 1). The traps located at 20 and 40 m within the abandoned coffee plantations showed a higher abundance of CBB in comparison with those located in other land use at 20 m (Table 1).

Table 1. Marginal means and confidence intervals (95%) of the number of CBBs captured in each adjacent land use and trap position.
3.3 Effect of land use, trap position, and time
We also find a significant interaction between adjacent land use, trap position, and time on the number of captured CBBs. Effects of land use and trap position were more evident at certain times of the year (Supplementary material 2) mainly at the end of the dry season and after the first rain from April to June (in red) and at the end of the harvest (in gray) (Figure 5). Traps placed within the active coffee plantations and near the pastures captured a greater number of CBB compared to those placed in coffee plantations bordering forests or abandoned coffee fields. In contrast, in the traps located on the edges, there were no differences between the uses of land adjacent to the coffee plantation. On the other hand, in the traps located within the abandoned coffee plantations at 20 and 40 meters from the edge of the active coffee, a greater abundance of CBB was found than in the traps in the other land uses in the same positions. An even higher abundance of CBB was found in these traps than in the adjacent active coffee plantations. In addition, during the harvest period, the traps inside the forest (20 and 40 m) had a higher number of CBBs than the traps located in pastures (gray region).
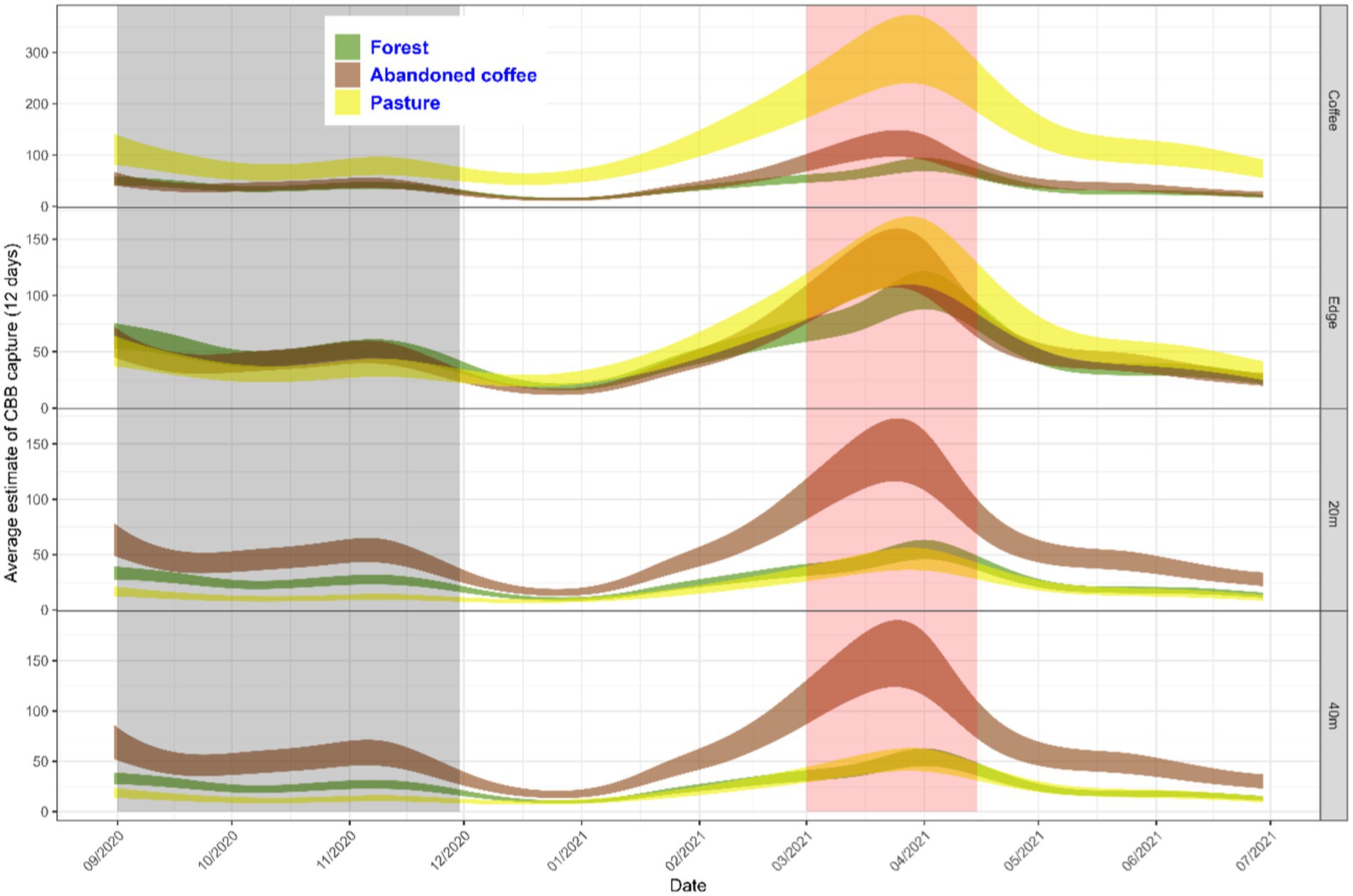
Figure 5. Estimated number of CBBs over time based on adjacent land use and trap position. The orange-colored area corresponds to the period of berry development and the peak of greatest CBB emergence from the residual berries of the previous harvest.
3.4 Relationship between meteorological conditions and abundance of CBB captures
We found an interaction (χ211 = 24.53, p = 0.0046; Supplementary material 1) between the adjacent land uses, the position of the trap, and the wind speed (Figure 6A). Specifically in the traps located inside the coffee plantation at the interface with pasture, the abundance of CBB was positively affected by wind speed. On the other hand, in the trap located 40 m from the edge within the pasture, the effect between the abundance of CBB and wind speed was negative (Figure 6A). For all the other position of the traps and adjacent land use (forest and abandoned coffee), there was no effect between the number of CBB collected and the wind speed.
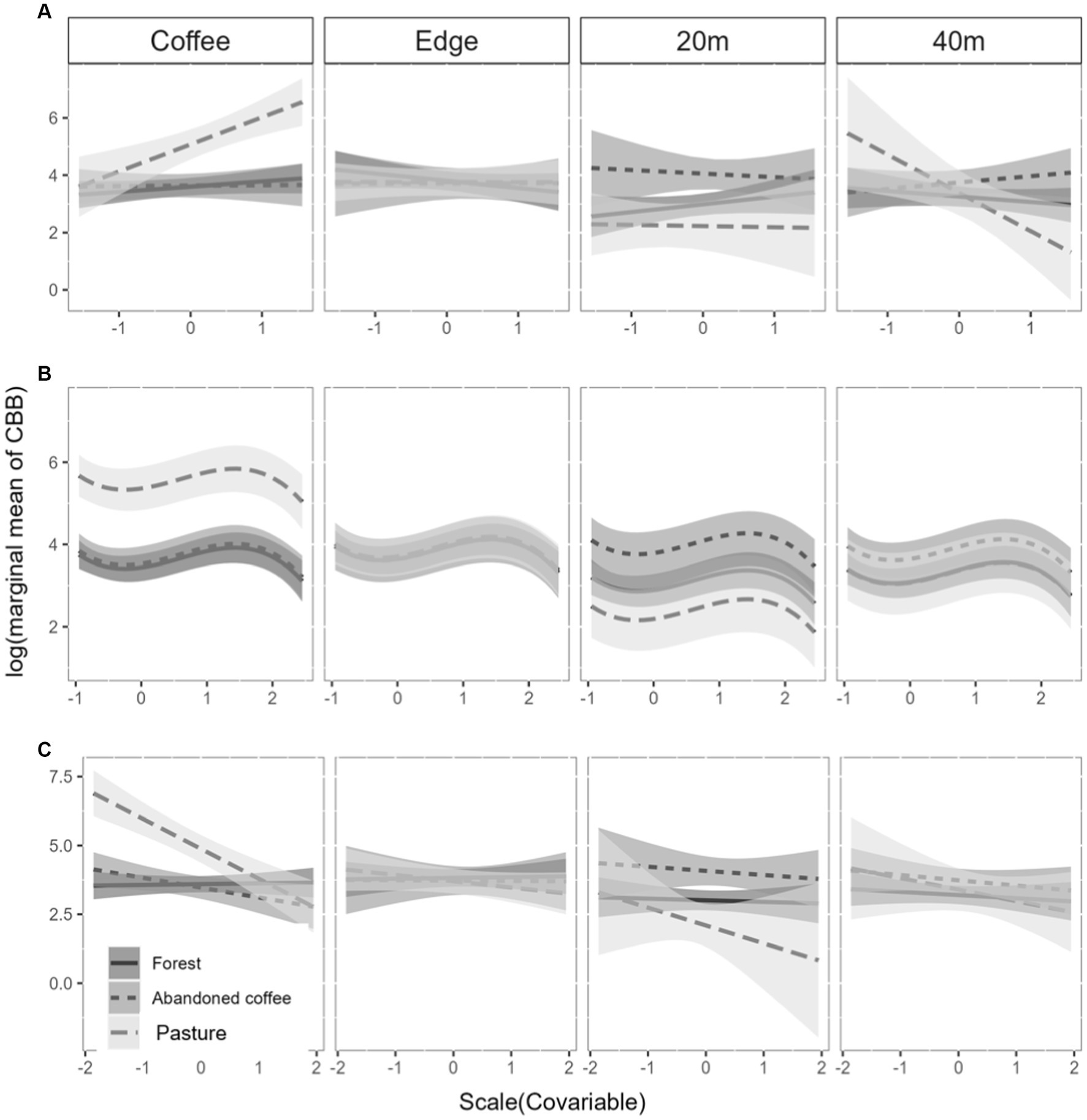
Figure 6. Relationship of the number of CBB, adjacent land use, the spatial arrangement of the traps, and scaled agroclimatic variables: (A) wind speed, (B) rainfall, and (C) relative humidity. The y-axis is the logarithm of scale.
The precipitation showed a non-linear effect (χ21 = 12.84, p = 0.0013; Supplementary material 1) with the abundance of CBB, but the effect was the same in all land uses whatever the traps position (Figure 6B). There was an interaction between relative humidity and adjacent land use and trap position (χ211 = 29.99, p = 0.0015; Supplementary material 1). Relative humidity had a negative effect on the abundance of CBBs captured in traps located within the coffee plantation at the interface with pasture and at 40 m within this land use (Figure 6C). The temperature did not show any effect with the captures of CBB (χ21 = 0.84, p = 0.35; Supplementary material 1).
4 Discussion
Our study demonstrated that female CBBs can fly out of coffee patches and move to adjacent land uses in search of resources. We found that the CBB population outside coffee plantations accounts for approximately 26% of the total population, in contrast to the 4% reported by Olivas et al. (2011) in the same landscape. The differences in the number of CBB outside coffee between these two studies may be due to differences in the study design and considered land uses as Olivas et al. (2011) set 100-m transects from forest to sugar cane and pastures only.
We captured CBB throughout the sampling period as reported by Aristizábal et al. (2017) in a study carried out on coffee farms in Hawaii. We did not find evidence to support our hypothesis (due to lack of resources) in periods of resource shortages. However, our experimental design provides some information about spillover effects at the edge and between the different land uses. We expected higher catches in the traps that are farthest from the edges of the coffee plantations during the period of scarcity of suitable resources (at the end of the harvest and during the flowering season), which is more favorable for CBB movement. Nevertheless, in the forest and pasture, the tendency was to decrease as the traps were farther from the edge and regardless of the time of year. This pattern supports the low capacity of movement of CBB (Gil et al., 2015). In the case of abandoned coffee, the abundance of trapped CBB was similar regardless of the position of the traps, reinforcing the hypothesis of a local population of CBB.
Higher populations of CBB females were captured at the end of the dry season and the beginning of the rainy season (April–May) due to the appearance of the first suitable fruits. In fact, during this period, the main cohort of berries is older than 120 days. At this stage, coffee berries start to be suitable for CBB female attacks (Montoya and Cardenas-Murillo, 1994; Benavides et al., 2012) due to the maximum moisture content and the average dry weight approximately 0.14 g (Salazar et al., 1994). This phenological stage might stimulate the emergence of flying females from residual berries in the soil or on plants from the previous harvest (Dufour et al., 1997; Pereira et al., 2012). This reminds us of the importance of the sanitary harvest to control the CBB populations to prevent the colonization of the new generations of berries (Mathieu et al., 1997a,b; Benavides et al., 2012; Pereira et al., 2012; Johnson and Manoukis, 2020; Vilchez-Mendoza et al., 2022).
After this peak of captures, there are more berries suitable and less CBB captured in the traps, maybe due to the competition between the volatiles emitted by the berries and the attractants of the traps. During this period, the CBBs are still captured outside the adjacent land uses. The largest captures are recorded in abandoned coffee plantations. We assumed that in this land use, there are still some coffee plants that produce some fruits that could be infested by CBB. The population collected may be an on-site population that is not related to the movements of individuals from nearby active coffee plantations. Gil et al. (2015) show that less than 10% of the dispersing population of CBB are able to disperse at distance > to 65 m in coffee plantations.
During the harvest period, two small peaks of CBB captures are observed. We consider that it is an effect not only of the removal of berries by harvest action that favors the emergence of females but also an effect of environmental conditions. The peak of rain and daylight that occurred during this period might stimulate the emergence of CBB females (Mathieu et al., 1997a).
The correlation of the accumulated rainfall of each sampling session with the CBB capture indicates that the increase in rainfall increases the CBB captured up to a point where the rain might become detrimental and reduce the number of CBB captured (Figure 6B). The coffee plantations adjacent to pastures registered a greater abundance of captured CBB females than the coffee plantations adjacent to the forest and the abandoned coffee. We believe that the adjacency to the forest provides natural enemies which may remove CBBs that are flying close to the edges. There is evidence of the effect of proximity to the forest on the reduction of bored berries (Avelino et al., 2012; Karp et al., 2013; Aristizábal and Metzger, 2019; Vilchez-Mendoza et al., 2022).
Finally, in the present study, our data suggest that the movement of CBB might be governed by wind action. The interaction between the speed of the wind and the positions of the traps in the coffee plantations adjacent to pastures corroborates the action of the wind in facilitating the movement of the CBB (Baker, 1984). In the trap located inside the coffee plantation, there is greater capture when the wind speed increases, possibly because of the CBB movement inside the coffee plantation. In the trap located at 40 m from the edge of the pasture, there is a negative relationship between the wind and the amount of CBB captured. One hypothesis could be that CBB that enters the pasture is transported by the wind beyond the location of the traps (more than 40 m). According to Alonzo (1984), the flight of coffee berry borer adult females is reduced to a few meters, unless they take advantage of air currents. A dispersion at large distances might depend on speed conditions and wind direction (Benavides, 2010). In contrast, the non-interaction of the wind with the position of the traps in coffee plantations adjacent to forests and abandoned coffee areas may be due to the resistance generated by these land uses to the wind. Johnson and Manoukis (2020) also suggest that vegetation surrounding coffee farms may act as a physical barrier and recommend the use of physical barriers or border crops densely planted to inhibit the migration of CBB. Future studies should consider variables related to vegetation structure to better understand potential mechanisms related to the resistance offered by adjacent land uses.
5 Conclusion
Our study highlights the significance of adjacent land uses in the dispersal of CBB. Specifically, our results suggest that open agricultural systems such as pastures can facilitate the movement of CBB by wind, possibly beyond CBB dispersal ability. Other dominant open systems in Ventral American landscapes include sugarcane and diverse annual crops that could have a similar effect on CBB populations as pastures in our study.
Abandoned coffee areas in our landscape are a source of CBB for adjacent coffee plantations, hosting CBB populations even up to 2 years after abandonment. In our study area, the gradual abandonment of coffee cultivation due to low productivity and changes in market prices makes the management of these abandoned areas a priority.
Our results suggest that forests can be considered as natural barriers to the movement of CBB, which is probably related to their greater vegetation complexity that modifies the direction and speed of wind and prevents CBB transport.
Considering the importance of landscape configuration and composition on CBB incidence in coffee plantations, we suggest that the management that abandoned coffee areas should be considered in which remnant coffee plants are removed or shade management is done to promote the growth of secondary vegetation. In addition, the use of vegetation barriers and the consideration of buffer areas between coffee cultivation and adjacent land uses should be considered.
Finally, the information generated in our study on CBB incidence in coffee plantations and adjacent land uses can be used to assign permeability values in studies of CBB movement using tools such as graph or circuit theory, an alternative to more expensive dispersion studies in the field.
Data availability statement
The original contributions presented in the study are included in the article/Supplementary material, further inquiries can be directed to the corresponding author.
Author contributions
SV-M: Conceptualization, Formal analysis, Investigation, Methodology, Writing – original draft. AR: Methodology, Writing – review & editing. PB: Conceptualization, Supervision, Writing – review & editing. CC: Supervision, Writing – review & editing. LB: Conceptualization, Methodology, Resources, Supervision, Writing – review & editing.
Funding
The author(s) declare financial support was received for the research, authorship, and/or publication of this article. The author(s) declare the work was funded by the Agroforesta platform and CATIE.
Acknowledgments
We would like to acknowledge the farmers who allowed us to work on their farms, Amilkar Moncada and Kennet Barbosa for their support in the data collection, and the Agroforesta platform for its logistical and financial support.
Conflict of interest
The authors declare that the research was conducted in the absence of any commercial or financial relationships that could be construed as a potential conflict of interest.
Publisher’s note
All claims expressed in this article are solely those of the authors and do not necessarily represent those of their affiliated organizations, or those of the publisher, the editors and the reviewers. Any product that may be evaluated in this article, or claim that may be made by its manufacturer, is not guaranteed or endorsed by the publisher.
Supplementary material
The Supplementary material for this article can be found online at: https://www.frontiersin.org/articles/10.3389/fsufs.2024.1356650/full#supplementary-material
Footnotes
1. ^See the algorithm in https://github.com/SVMendoza/Detection-and-count-CBB.
References
Alonzo, F. R. (1984). Aspectos ecológicos de la broca Hypothenemus hampei (Coleoptera: Solytidae) In: IICA- PROMECAFE. El problema de la broca (Hypothenemus hampei, Ferr.) (Coleoptera: Scolytidae) y la caficultura: Aspectos relacionados con importancia, daño, identificación, Biolo’. San José, Costa Rica: IICA-PROMECAFE, 71–136.
Amante, B. L. (2020) ‘La conectividad funcional y los servicios ecosistémicos proporcionados por abejas en los agropaisajes del Corredor Biológico Volcánica Central Talamanca, Costa Rica’, Tesis. CR Turrialna: CENTRO AGRONÓMICO TROPICAL DE INVESTIGACIÓN Y ENSEÑANZA, DIVISIÓN DE EDUCACIÓN, pp. 1–125.
Aristizábal, L. F., Shriner, S., Hollingsworth, R., and Arthurs, S. (2017). ‘Flight Activity and Field Infestation Relationships for Coffee Berry Borer in Commercial Coffee Plantations in Kona and Kau Districts, Hawaii’, Journal of Economic Entomology, 110, pp. 2421–2427. doi: 10.1093/jee/tox215
Aristizábal, N., and Metzger, J. P. (2019). Landscape structure regulates pest control provided by ants in sun coffee farms. J. Appl. Ecol. 56, 21–30. doi: 10.1111/1365-2664.13283
Avelino, J., Romero-Gurdián, A., Cruz-Cuellar, H. F., and Declerck, F. A. J. (2012). Landscape context and scale differentially impact coffee leaf rust, coffee berry borer, and coffee root-knot nematodes. Ecol. Appl. 22, 584–596. doi: 10.1890/11-0869.1
Baker, P. S. (1984). ‘Some aspects of the behavior of the coffee berry borer in relation to its control in southern Mexico (Coleoptera, Scolytidae)’, Entomologica Mexicana, 61(January), pp. 9–24.
Baker, P. S., Ley, C., Balbuena, R., and Barrera, J. F. (1992). Factors affecting the emergence of Hypothenemus hampei (coleoptera: Scolytidae) from coffee berries. Bull. Entomol. Res. 82, 145–150. doi: 10.1017/S000748530005166X
Bates, D., Mächler, M., Bolker, B., and Walker, S. (2015). Fitting linear mixed-effects models using lme4. J. Stat. Softw. 67:1406. doi: 10.18637/jss.v067.i01
Benavides, P., Gongora, C., and Bustillo, A. (2012). “IPM program to control coffee berry borer Hypothenemus hampei, with emphasis on highly pathogenic mixed strains of Beauveria bassiana, to overcome insecticide resistance in Colombia’” in Insecticides - Advances in Integrated Pest Management. ed. F. K. Perveen
Benavides, P. M. (2010) Cómo se dispersa la broca a partir de cafetales zoqueados infestados. 38th. Colombia: Cenicafé.
Castaño, A., Benavides, P., and Baker, P. S. (2005). Dispersión de Hypothenemus hampei en cafetales zoqueados. Cenicafé 56, 142–150.
Chamorro Trejos, G., Cárdenas Murillo, R., and Herrera Herrera, A. (1995). Evaluación económica y de la calidad en taza del café proveniente de diferentes sistemas de recolección manual, utilizables como control en cafetales infestados de Hypothenemus hampei. Cenicafé (Colombia) 46, 164–175.
Damon, A. (2000). A review of the biology and control of the coffee berry borer, Hypothenemus hampei (coleoptera: Scolytidae). Bull. Entomol. Res. 90, 453–465. doi: 10.1017/s0007485300000584
Dickson, B. G., Albano, C. M., Anantharaman, R., Beier, P., Fargione, J., Graves, T. A., et al. (2019). Circuit-theory applications to connectivity science and conservation. Conserv. Biol. 33, 239–249. doi: 10.1111/cobi.13230
Dufour, B. (1997). ‘Lutte biologique contre le scolyte des baies du caféier en période de post récolte: Méthodes d’évaluation’, Plantations, Recherche, Developpement, 4, pp. 115–122.
Gil, Z. N., Benavides, P., Souza, O. D., Acevedo, F. E., and Lima, E. (2015). Molecular markers as a method to evaluate the movement of Hypothenemus hampei (Ferrari). J. Insect Sci. 15, 72–78. doi: 10.1093/jisesa/iev058
Hartig, F. (2022) ‘DHARMa: residual diagnostics for hierarchical (multi-level / mixed) regression models’. R package version 0.4.6. Available at: https://cran.r-project.org/package=DHARMa.
Hernández-Andrade, A., Parra-Gómez, L., Ferrer, M. M., Montañez-Escalante, P. I., and Jiménez-Osornio, J. (2019). Agrodiversity of hylocereus undatus in maya homegardens: management and genetic variability. Herpetologica 39, 530–548. doi: 10.2993/0278-0771-39.4.530
Hothorn, T., Bretz, F., and Westfall, P. (2008). Simultaneous inference in general parametric models. Biom. J. 50, 346–363. doi: 10.1002/bimj.200810425
Jocher, G., Stoken, A., Borovec, J., NanoCode,, Chaurasia, A., TaoXie,, et al. (2021). Ultralytics/yolov5: v5.0 - YOLOv5-P6 1280 models, AWS, Supervise.ly and YouTube integrations. Zenodo. doi: 10.5281/zenodo.4679653
Johnson, M. A., and Manoukis, N. C. (2020). Abundance of coffee berry borer in feral, abandoned and managed coffee on Hawaii Island. J. Appl. Entomol. 144, 920–928. doi: 10.1111/jen.12804
Karp, D. S., Mendenhall, C. D., Sandí, R. F., Chaumont, N., Ehrlich, P. R., Hadly, E. A., et al. (2013). Forest bolsters bird abundance, pest control and coffee yield. Ecol. Lett. 16, 1339–1347. doi: 10.1111/ele.12173
Laska, A., Przychodzka, A., Puchalska, E., Lewandowski, M., Karpicka-Ignatowska, K., and Skoracka, A. (2022). Mechanisms of dispersal and colonisation in a wind-borne cereal pest, the haplodiploid wheat curl mite. Sci. Rep. 12, 1–12. doi: 10.1038/s41598-021-04525-9
Leefmans, S. (1923). ‘De Koffiebessenboeboek (Stephanoderes hampei Ferrari = coffeae Hagedorn)’, Levenswijze en oecologie. Meded. Van het. Instituut voor Plantenz 57:94
Lenth, R. (2023) ‘Emmeans: Estimated marginal means, aka least-squares means’. R package version 1.8.5, Available at: https://cran.r-project.org/package=emmeans.
Mathieu, F., Brun, L. O., and Frérot, B. (1997a). Factors related to native host abandonment by the coffee berry borer Hypothenemus hampei (Ferr.) (Col., Scolytidae). J. Appl. Entomol. 121, 175–180. doi: 10.1111/j.1439-0418.1997.tb01389.x
Mathieu, F., Gaudichon, V., Brun, L. O., and Frérot, B. (2001). Effect of physiological status on olfactory and visual responses of female Hypothenemus hampei during host plant colonization. Physiol. Entomol. 26, 189–193. doi: 10.1046/j.1365-3032.2001.00232.x
Mathieu, F., Marchillaud, C., and Frcrot, B. (1997b). ‘Trapping of the coffee berry borer Hypothenemus hampei Ferr. (Col., Scolytidae) within a mesh-enclosed environment: interaction of olfactory and visual stimuli,’ Journal of Applied Entomology 121(1–5) 185–186. doi: 10.1111/j.1439-0418.1997.tb01390.x
Montoya, S., and Cardenas-Murillo, R. (1994). Biología de Hypothenemus hampei (Ferrari) en frutos de café de diferentes edades. Cenicafé 45, 5–13.
Mosomtai, G., Azrag, A. G. A., Babin, R., Abdel-Rahman, E. M., Odindi, J., Mutanga, O., et al. (2021). Functional land cover scale for three insect pests with contrasting dispersal strategies in a fragmented coffee-based landscape in Central Kenya. Agric. Ecosyst. Environ. 319, 107558–107510. doi: 10.1016/j.agee.2021.107558
O’Rourke, M. E., and Petersen, M. J. (2017). Extending the “resource concentration hypothesis” to the landscape-scale by considering dispersal mortality and fitness costs. Agric. Ecosyst. Environ. 249, 1–3. doi: 10.1016/j.agee.2017.07.022
Olivas, A., Rivera, C., Dufour, B., Hidalgo, E., DeClerck, F., and Avelino, J. (2011). “Micro-landscape context effects on the dispersal of coffee berry borer (Hypothenemus hampei)” in Costa Rica’, 23rd international conference on coffee science (ASIC) (Bali: Association Scientifique Internationale du Cafe´ (ASIC))
Pereira, A. E., Vilela, E. F., Tinoco, R. S., de Lima, J. O. G., Fantine, A. K., Morais, E. G. F., et al. (2012). Correlation between numbers captured and infestation levels of the coffee berry-borer, hypothenemus hampei: a preliminary basis for an action threshold using baited traps. Int. J. Pest Manag. 58, 183–190. doi: 10.1080/09670874.2012.676219
R Core Team (2023) R: A language and environment for statistical computing R Foundation for Statistical Computing, Vienna, Austria. Available at: https://www.r-project.org/.
Salazar, G., Chaves, B., Riaño, N. M., Arcila, J., and Jaramillo, A. (1994). Crecimiento del fruto de Coffea arabica var. Colombia. Cenicafé 45, 41–50.
Tischendorf, L., and Fahrig, L. (2000). On the usage and measurement of landscape connectivity. Oikos 90, 7–19. doi: 10.1034/j.1600-0706.2000.900102.x
Tresson, P., Tixier, P., Puech, W., Bagny Beilhe, L., Roudine, S., Pagès, C., et al. (2019). CORIGAN: assessing multiple species and interactions within images. Methods Ecol. Evol. 10, 1888–1893. doi: 10.1111/2041-210X.13281
Vilchez-Mendoza, S., Romero-Gurdián, A., Avelino, J., DeClerck, F., Bommel, P., Betbeder, J., et al. (2022). Assessing the joint effects of landscape, farm features and crop management practices on berry damage in coffee plantations. Agric. Ecosyst. Environ. 330:107903. doi: 10.1016/j.agee.2022.107903
Keywords: Hypothenemus hampei, pest management, weather, edge effects, Costa Rica
Citation: Vilchez-Mendoza SJ, Ronin A, Bommel P, Cilas C and Bagny Beilhe L (2024) Quantifying movement of the coffee berry borer at the interface between coffee plantations and adjacent land uses. Front. Sustain. Food Syst. 8:1356650. doi: 10.3389/fsufs.2024.1356650
Edited by:
Fábio Luiz Partelli, Federal University of Espirito Santo, BrazilReviewed by:
Melissa Johnson, Agricultural Research Service (USDA), United StatesJaved Sidiqi, McGill University, Canada
Copyright © 2024 Vilchez-Mendoza, Ronin, Bommel, Cilas and Bagny Beilhe. This is an open-access article distributed under the terms of the Creative Commons Attribution License (CC BY). The use, distribution or reproduction in other forums is permitted, provided the original author(s) and the copyright owner(s) are credited and that the original publication in this journal is cited, in accordance with accepted academic practice. No use, distribution or reproduction is permitted which does not comply with these terms.
*Correspondence: Sergio J. Vilchez-Mendoza, svilchez@catie.ac.cr