- 1Department of Entomology, University of Kentucky, Lexington, KY, United States
- 2School of Economics and Management, China University of Petroleum – Beijing, Beijing, China
- 3Department of Entomology, Washington State University, Pullman, WA, United States
- 4Charles H. Dyson School of Applied Economics and Management, Cornell University, Ithaca, NY, United States
- 5Department of Horticulture, University of Kentucky, Lexington, KY, United States
Organic cucurbit growers face many challenges to production including insect pests, insect-vectored diseases, and non-vectored diseases. While Organic Material Review Institute (OMRI) -listed pesticides underperform at suppressing pests and diseases, some alternative pest management strategies hold promise, but little research exists on their cost-effectiveness. We compared the efficacy of mesotunnel systems (nylon-mesh netting row covers deployed over bent metal hoops) and OMRI-listed pesticides (fungicides and insecticides) on acorn squash across 2 years. During the early part of the season, before row covers were removed for pollination, we found 1.7 (2020) and 0.3 (2021) times more cucumber beetles in the uncovered plots compared to the mesotunnels. Powdery mildew incidence was 36 and 25% lower under the mesotunnels compared to the uncovered plots in 2020 and 2021, respectively. Marketable yield of acorn squash was 46–54% higher in the mesotunnels compared to the uncovered treatments. OMRI-listed pesticide treatments reduced powdery mildew incidence compared to untreated control treatments. However, for pest presence, OMRI-listed pesticide treatments did not differ from untreated control treatments in either year, nor did it differ in marketable yield in 2020. Finally, the mesotunnel-only system was 47% more profitable compared to the OMRI-listed pesticide treatment and 47% more profitable compared to the uncovered plots. These results highlight mesotunnels as an economically viable pest management strategy for organic cucurbit growers in the U.S.
1 Introduction
U.S. retail sales of organic foods rose more than five-fold between 2000 and 2020, reaching nearly $56 billion in annual sales (Carlson et al., 2023). Fruits and vegetables lead the organic market with nearly 40% of all sales (Carlson et al., 2023), making them central to the success of the organic market. However, production of some organic fruits and vegetables is severely challenged by pests and diseases because of a limited arsenal of OMRI (Organic Materials Review Institute)-listed insecticides and fungicides that often under-perform and are expensive to growers (Brockman et al., 2020; Dively et al., 2020). In fact, in the Southern U.S., organic growers rank controlling insect pests and diseases among the most important challenges facing organic production (Snyder et al., 2022).
For organic cucurbits, such as melons, cucumbers and winter squash, pests and diseases are particularly limiting. Striped and spotted cucumber beetles [Acalymma vittatum (F.) and Diabrotica undecimpunctata howardi Barber (Coleoptera: Chrysomelidae)], squash bugs [Anasa tristis De Geer (Hemiptera: Coreidae)], and the squash vine borer [Melittia cucurbitae Harris (Lepidoptera: Sesiidae)] pose the greatest insect threats to cucurbit production (Hoffmann et al., 2000; Brzozowski et al., 2016; Doughty et al., 2016; Middleton, 2018). While these pests cause extensive fruit and plant damage, they are also important vectors for pathogens including Erwinia tracheiphila Smith (bacterial wilt) and Serratia marcescens Bizio (cucurbit yellow vine disease, CYVD) (Rojas et al., 2015; Doughty et al., 2016). The bacterial wilt and the CYVD pathogens overwinter in the gut of cucumber beetles and squash bugs, respectively. These pathogens can be transmitted from vector insect to uninfected plants when saliva and feces enter areas of feeding damage. Further, uninfected insect vectors pick up E. tracheiphila and S. marcescens by feeding on infected cucurbits which allows for further disease spread. Once a plant is infected, the bacteria multiply and clog the vascular system in the stem. This causes severe wilting and ultimately leads to crop loss (Pair et al., 2004; Mueller et al., 2006; Seebold and Bessin, 2011; Doughty et al., 2016; Schuh and Grabowski, 2022).
Additionally, a major non-vectored disease, cucurbit powdery mildew, caused by either Podosphaera fusca (Fries) Braun & Shishkov or Erysiphe cichoracearum (de Candolle) Heluta, can greatly impact crop yield by reducing photosynthetic leaf surface area and by requiring separate management tactics from the insect-vectored diseases (Sharma et al., 2016). Symptoms of cucurbit powdery mildew are a white powdery growth that develops on both the top and undersides of leaf surfaces as well as petioles and stems. Older plants and plant parts are generally affected first. While fruit infection is unlikely, yields can be reduced when high colonization in the leaf surfaces reduces photosynthesis and limits nutrient availability for fruit growth (Sharma et al., 2016). Powdery mildew disease development is most severe when plant growth leads to low light penetration in the canopy and high relative humidity within the stand (Sharma et al., 2016). While dryness can be favorable for the spread of the disease, the presence of moisture or dew does not prevent the disease from developing (Schnathorst, 1965). It is estimated that U.S. cucurbit farmers lose nearly $100 million USD annually from these pest-disease complexes combined (Schroder et al., 2001).
OMRI-listed pesticide options are often ineffective at controlling both cucurbit and other insect pests in the field. While laboratory studies find that organic insecticides can effectively induce mortality in insect pests, control in the field is often lacking (Morehead and Kuhar, 2017; Dively et al., 2020). Lilley and Sanchez (2016) found that even with weekly insecticide applications, cucumber beetle populations remained above economic thresholds. Dively et al. (2020) cite many reasons for a lack of field control: differences in insect population density, number of applications, and residue coverage influenced by spray volume and canopy structure. Further, OMRI-listed pesticides can have non-target effects that harm pollinators and natural enemies (Morandin et al., 2005; Desneux et al., 2007; Bernardes et al., 2018).
Powdery mildew is most often managed by planting resistant varieties and by applying fungicides (McGrath, 2015). However, most fungicides are used preventatively as they must be applied before the disease develops. While some organic fungicide options, such as copper, can be very effective at controlling powdery mildew, there is concern that overuse of fungicides can lead to resistance development and detrimental effects on soil microbial communities, as well as potential phytotoxicity (Marine et al., 2016). Additionally, there is a lack of studies that compare the effectiveness of organic fungicides to untreated controls.
One promising alternative pest control strategy is the use of fabric or fine mesh row covers that exclude insect pests and insect vectored diseases. Low tunnels (45.72 cm tall) covered by a spunbond polypropylene fabric are traditionally used in season extension for protection against frost and cooler temperatures (Evans, 2016). Beyond season extension, low tunnels also increase growth, yield, water, and nitrogen use efficiency in addition to excluding pests (Acharya et al., 2019, 2020a,b). However, fabric low tunnels can trap heat and increase the temperature under the cover beyond the plant’s tolerance in warmer climates (Tillman et al., 2015; Skidmore et al., 2019). In contrast, mesotunnel systems are covered by nylon-mesh fabric that is durable, breathable and light-permeable, and is placed over the crop on 1.1 m tall bent conduit hoops. The use of mesotunnel systems has resulted in consistently large gains in marketable yield for organic muskmelon and acorn squash, as well as drastically reduced needs for insecticide and fungicide application (Hanna et al., 2016; Athey et al., 2021; Nelson et al., 2023). Skidmore et al. (2019) found in their cucurbit study that row cover plots contained about half the number of pests (striped and spotted cucumber beetles) as uncovered plots. Further, Nelson et al. (2023) found that they could reduce the need for insecticide sprays to zero with the use of a full-season mesotunnel stocked with commercial bumble bees for pollination.
Two major challenges of using fine mesh mesotunnel systems are the high upfront costs and increased labor required for their installation and maintenance. To date, few studies have compared the profitability of mesotunnels to OMRI-listed insecticides or low tunnels. In some experiments fine-mesh netting is less profitable than fabric row covers (Hanna et al., 2016) and in others, mesotunnels (fine-mesh netting) were marginally more profitable than low tunnels (fabric row covers) and uncovered systems with OMRI-listed insecticides (Nelson et al., 2023). Additionally, Rojas et al. (2011) found that in years where there was a high incidence of bacterial wilt, row covers that remained installed for long durations were more profitable than either row cover removal at anthesis or uncovered systems. However, in years where there was little to no bacterial wilt, all row covers and uncovered systems were equivalently profitable.
While row covers can reduce the impact of insect pests and vectored pathogens on different crops through exclusion, it is unknown if row covers interact with non-vectored diseases. Row covers alter air and soil temperatures and solar radiation (Lilley and Sanchez, 2016; Arancibia, 2018; Acharya et al., 2019, 2020a), which could influence non-vectored diseases such as powdery mildew. Row covers may influence the development of powdery mildew by modifying microclimatic conditions or acting as a partial barrier to the movement of spores, but whether row cover microclimate modifications enhance or reduce the growth and transmission of powdery mildew is unknown. In this study, we compare the impact of mesotunnels and OMRI-listed pesticides (fungicides and insecticides) on acorn squash: (1) insect pests and insect-vectored pathogen symptoms, (2) non-vectored powdery mildew symptoms, (3) abiotic microclimate conditions, and (4) the profitability of these management techniques. We hypothesized that the mesotunnels will reduce the number of insect pests and insect-vectored pathogen symptoms. We also hypothesized, that non-vectored powdery mildew symptoms will be greater under the mesotunnels, which will be accompanied by an increase in the relative humidity and change in the other abiotic microclimate conditions under the mesotunnels. Finally, we hypothesized that profitability will be greatest under the mesotunnel-only system.
2 Materials and methods
2.1 Site
We conducted field studies in the Organic Farming Unit of the University of Kentucky’s Horticulture Research Farm in Lexington, KY (37°58′25.92” N, 84°32′5.85” W) in 2020 and 2021. This 40.5 hectare farm is within USDA (United States Department of Agriculture) plant hardiness zone six. The farm is split into organic and conventional zones and includes a diverse array of specialty crops. In the certified organic section of the farm, in addition to the research plots, a Community Supported Agriculture program grows over 50 different types and varieties of vegetables, fruit and herbs and is holistically managed with crop rotation and cover crops.
2.2 Experimental design
The experimental design was a randomized block experiment. Within each block (N = 4), we randomized mesotunnel treatments (mesotunnel or uncovered) and OMRI-listed pesticide treatments (pesticides or untreated) in a two-by-two factorial design. The OMRI-listed pesticide treatments included insecticides that were applied following economic threshold guidelines for cucumber beetles and squash bugs (Brust et al., 1996; Burkness and Hutchison, 1998; Brust and Foster, 1999; Doughty et al., 2016), and at the first instance of squash vine borers. OMRI-listed fungicides were applied when the first evidence of powdery mildew was present. Each plot was approximately 6.4 m wide and 9.1 m long and included three plastic-mulch beds (0.9 m wide) spaced 0.9–1.2 m apart. Plots were separated from each other by approximately 2.1–2.4 m.
2.3 Field preparation
Fields were amended with organic compost (University of Kentucky Woodford County Farm) at a rate of approximately 4 tons per hectare and spaded in early spring (Table 1). After spading, fields were cultivated, and beds were formed with a Rain-Flo Plastic Layer (Rain-Flo Irrigation, East Earl, PA, United States) and the Kubota m9540 tractor (Kubota, Osaka, Japan). White plastic mulch (Berry Global, Evansville, IN, United States), and drip tape (20.32 cm aqua-traxx drip irrigation; The Toro Company, Bloomington, MN, United States) were used to form the beds. Simultaneous with plastic laying, NatureSafe 10-2-8. coarse fertilizer (Darling Ingredients, Irving, TX, United States) was applied at a rate of 16.3–18.1 kgs per hectare (Table 1).
‘Table Ace’ acorn squash (Cucurbita pepo) (Seedway, LLC. Hall, NY, United States) seeds were sown into 10, 72-cell trays (3.8 cm x 3.8 cm x 5.7 cm) using Vermont Compost (Vermont Compost Company, Montpelier, VT, United States; Table 1) and grown inside a greenhouse. Four-week-old acorn squash seedlings were transplanted in single rows with 61 cm in-row spacing and transplant holes were backfilled with woodchips (2020) and organic Promix (Premier Tech Horticulture, Delson, Quebec, Canada) (2021) to prevent weed emergence.
On the day of transplanting, a living mulch of teff (Eragrostis tef; Corvallis-nitro coat; Welter Seed, Onslow, IA, United States) was sown for weed control at a rate of 4.4 kg per hectare in 2020. In 2021, a rate of 6.5 kg per hectare was used to improve weed control. Additionally, in 2021 the teff was mowed to a height of 5.1–7.6 cm with a BCS flail mower (BCS America, Oregon City, OR, United States) 33 days after establishment to limit teff from shading the acorn squash later in the season.
After transplanting, we immediately installed mesotunnels over the respective treatments. Hoops were installed in each plot with 1.1 m tall bent, electrical conduit pipe hoops (Johnny’s Selected Seeds, Winslow, ME, United States) by evenly spacing five hoops on each of the two outside beds every 1.8 m and three hoops in the center bed every 3 m. Fine-mesh netting (60-g netting; Tek-Knit Industries Montreal, Quebec, Canada), cut to the appropriate dimensions, was installed above the hoops, and weighed down at the perimeter by rock bags.
To allow for insect-mediated pollination of acorn squash, we opened mesotunnels during flowering to allow wild pollinators to access squash flowers. In 2020, we completely removed the fine mesh net at flowering for 16 days before reestablishing the netting (on–off–on strategy). In 2021, we modified this approach by only opening the two ends of the tunnel by clipping the ends of the netting to the supporting hoops (open ends strategy). A previous experiment in 2020 found just opening the ends of the mesotunnel increased cucurbit yield compared to the entire removal of nets (Unpublished data). Mesotunnel ends were open for 21 days.
2.4 Arthropod and disease measurements
To determine the effect of pest management treatments on pest abundance, we deployed two pest abundance measurements. One week after transplanting, one sticky card, (6.35 cm x 8.89 cm) (Arbico Organics, Oro Valley, AZ, United States) was placed approximately 1.5 m inwards from each end of the center bed in each plot. The sticky cards were removed after 7 days. After the pollination was complete and the mesotunnel nets were reestablished, a second set of sticky cards were placed in each plot for 7 days. All insect pests of cucurbits present on sticky cards were quantified. Visual observations (scouting) of insect pests were conducted weekly on three flagged plants (at 3, 4.6, and 6.1 m) in the center bed of each plot. For 60 s, a trained observer counted the number of striped and spotted cucumber beetles, squash bug adults, nymphs, and eggs. Two squash vine borer pheromone traps (Great Lakes IPM, Vestaburg, MI, United States) were placed at the north and south ends of our experimental fields and monitored weekly for squash vine borer activity.
Weekly visual observations also guided the application of OMRI-listed insecticides and fungicides. For striped cucumber beetle, we used an economic threshold of one beetle per plant to decide on the application of insecticides (Brust et al., 1996; Burkness and Hutchison, 1998; Brust and Foster, 1999). There is no economic threshold published for the spotted cucumber beetle, so we used the same recommendations for striped cucumber beetle. We followed the published economic threshold of one adult squash bug per plant or one egg mass per plant to manage for squash bugs (Doughty et al., 2016). If the thresholds were met for either of the pests (cucumber beetles or squash bugs), we applied a mixture of kaolin clay (Surround WP, Tessenderlo Kerley, Inc., Phoenix, AZ, United States; 11.34 kg/0.4 hectares), pyrethrins and azadirachtin (Azera, MGK Company, Minneapolis, MN, United States; 1.42 L/0.4 hectares), and an adjuvant (Nu-Film P, Miller, Hanover, PA, United States; 118.29 mL/0.4 hectares) following recommended rates on a weekly basis. After the first observation of squash vine borers, we also included Bacillus thuringiensis (Javelin WG, Certis Biologicals, Columbia, MD, United States; 0.61 kg/0.4 hectares) within the insecticide mixture. At the first observation of powdery mildew, we also applied cuprous oxide (Nordox 75 WG, Brandt, Springfield, IL, United States; 0.57 kg/0.4 hectares) to treated plots. Weekly applications continued until 2 weeks before harvest in 2020 due to rain events and until the week of harvest in 2021.
At the end of the growing season, immediately before harvest, we evaluated wilt symptoms associated with Bacterial wilt and CYVD in each plot. We counted the number of wilted plants, including dead plants, in each of the beds across each treatment. We observed no wilt symptoms in the field in 2020, therefore we did not present the data.
During harvest in 2020 and 2021, we determined the severity of powdery mildew in each plot. We established a visual scale for assessing powdery mildew damage, with ratings based on the percentage of coverage on both the upper and under sides of leaves. The scale ranges from 0–20%, 20–40%, 40–60%, 60–80%, to 80–100%. One leaf from the approximate top or center of the plant was sampled from 10 acorn squash plants every 0.9 m in the central bed of each plot. The severity of powdery mildew was then estimated by averaging the percent coverage of the upper- and under-side of each leaf.
2.5 Yield measurements
Acorn squash was harvested once fruit were mature with a dark green color and an orange spot on the bottom. The number of fruits and total weight of fruits were recorded from 10 plants in the center bed of each plot. The fruits were graded according to USDA standards and divided into marketable yield (USDA number 1 and USDA number 2) and unmarketable yield (USDA Agricultural Marketing Service, 1983).
2.6 Temperature, humidity, and dew point measurements
Air temperature, humidity, and dew point were measured on even hours in all treatments from transplanting until harvest using temperature and relative humidity sensors (WatchDog B series; Spectrum Technologies, Inc., Aurora, IL, United States). One sensor was placed approximately 30.5 cm above the raised plastic bed in the central bed.
2.7 Statistical analysis
The visual observation data of pest insects and climate conditions were organized to capture changes resulting from mesotunnel management of pollinators given that nets were opened or removed during flowering. We aggregated measurements into three net-stages by averaging data collected during each stage. The “pre-flowering” net-stage ranged from transplant until the netting was removed for pollination. The “flowering” net-stage ranged from netting removal until the netting replacement at the conclusion of flowering. The “post-flowering” net-stage ranged from netting replacement until harvest.
To determine the impact of the mesotunnel and pesticide treatments, we analyzed data from 2020 and 2021 separately due to the use of the different mesotunnel pollination strategies within each year (on–off–on strategy in 2020 and open-ends strategy in 2021). For all analyses within each year, we applied Linear Mixed Models (LMM) with mesotunnel treatments, pesticide treatments, and their interaction as fixed effects and block as a random effect using R, version 4.0.3 (R Core Team 2020, R Foundation for Statistical Computing, Vienna, Austria) and the function ‘lmer’ [R-package ‘lme4’ (Bates et al., 2015)]. For visual observations of cucumber beetles and squash bugs, we also included the factor net-stage and all two- and three-way interactions as fixed effects to understand the pest abundance dynamics during periods when mesotunnels were closed (pre-flowering), opened (flowering), and re-closed (post-flowering). We tested each model for the assumptions of normality using the Shapiro-Wilks test on model residuals. For all data, we averaged subsamples within each experimental plot to help meet the assumptions of a normal distribution. A square-root transformation was applied to meet the assumptions of normality for some dependent variables. Post hoc pairwise contrasts were performed using the function ‘emmeans’ with a Tukey adjustment [R-package ‘emmeans’ (Lenth et al., 2019)]. However, for squash bug abundance the non-parametric Kruskal–Wallis test was used to assess the effects of mesotunnel treatments and pesticide treatments for each net-stage. For unmarketable yield, we used a Kruskal–Wallis test to assess the effects of mesotunnel and pesticide treatments for 2020, due to the lack of normality of the data.
Similar to the pest, disease, and yield data analysis, all climate data was analyzed separately by year. We tested each model for the assumptions of normality using the Shapiro–Wilks test on model residuals. All climate variables were highly non-normal, therefore we used a Kruskal–Wallis test to assess the effects of the mesotunnel treatments on temperature, dew point, and relative humidity. For air temperature, relative humidity, and dew point, the minimum, maximum, and mean values were averaged across all time points for each treatment during each net-stage.
2.8 Economic analysis
A partial budget analysis was conducted to compare the cost and economic efficiency of all treatments (Calkins and DiPietre, 1983). We collected mesotunnel-related cost data, including hoops, netting, rock bags, spraying pesticides, and weeding. Labor costs included mesotunnel preparation and setup, net cutting, rock bag filling, pollination-related labor, field clean-up after harvest, pesticide spraying, and weeding labor. Other costs including field preparation, seeds, irrigation materials, white plastic mulch, etc., were treated as common cost items.
We used an annual equivalent cost approach to convert the cost of the netting to the annual cost of using it over its assumed life of 5 years (Cui et al., 2022). Equation 1: Formula to calculate annual equivalent cost is shown below (where “r” is the interest rate and “t” is the assumed life of the asset):
Equation 1. Formula to calculate annual equivalent cost.
Similarly, the electrical conduit pipe and the conduit bender (a tool to bend the conduit into the hoop shape) were assumed to last for 10 years. The conduit bender was assumed to be used for all the subplots in the experiments, therefore, we divided the cost by the number of subplots in the experiment. Labor was classified as unskilled for all tasks except the spraying of OMRI-listed pesticides. We assumed an hourly wage rate of unskilled labor was $18 and skilled labor was $20. The sprayer cost was calculated by the annual equivalent cost equation, prorating the costs among treatments based on the number of pesticide-spray trips. For example, the annual equivalent cost for a 300-gallon-capacity boom sprayer with drawbar pull is around $489 based on a 10-year estimated lifetime. During 2021 experiments, 7-day sprays were applied in the OMRI-listed pesticide treatment. Dividing 489 by 365 days per year and multiplying by 7 results in an estimated sprayer cost of $9.38, which encompasses only the machine cost, but not the labor and pesticide costs.
Based on the collected information, we estimated total cost, marketable yield, revenue, profitability (revenue – total costs), and Net Present Value. Net Present Value was calculated by assuming a discount rate of 7% based on the estimate from Federal Energy Management Program and using the Microsoft Excel formula NPV (discount rate, cash flow) (Advisers, 2017; Cui et al., 2022). A robustness check using a 3% discount rate was conducted and does not change the main conclusions. To estimate revenue, we assumed retail prices for acorn squash were $4.40 per kg and wholesale prices were $2.65 per kg. We used the results from the retail price to report the revenue, net profit, and NPV.
3 Results
3.1 Cucumber beetles
For cucumber beetles sampled using sticky cards, we found uncovered treatments had nearly seven times more cucumber beetles than did the mesotunnel treatments in 2020 (Figure 1A, F1,12 = 54.9, p < 0.001). In 2021, uncovered treatments had almost three times more cucumber beetles on sticky cards than did the mesotunnel treatments (Figure 1B, F1,12 = 12.8, p = 0.004). There was no effect of the OMRI-pesticide treatments on cucumber beetles found on sticky cards (2020 F1,12 = 0.1, p = 0.766; 2021 F1,12 = 0.0, p = 0.965) nor was there an interaction between the mesotunnel and OMRI-pesticide treatments (2020 F1,12 = 2.1, p = 0.172; 2021 F1,12 = 0.3, p = 0.576).
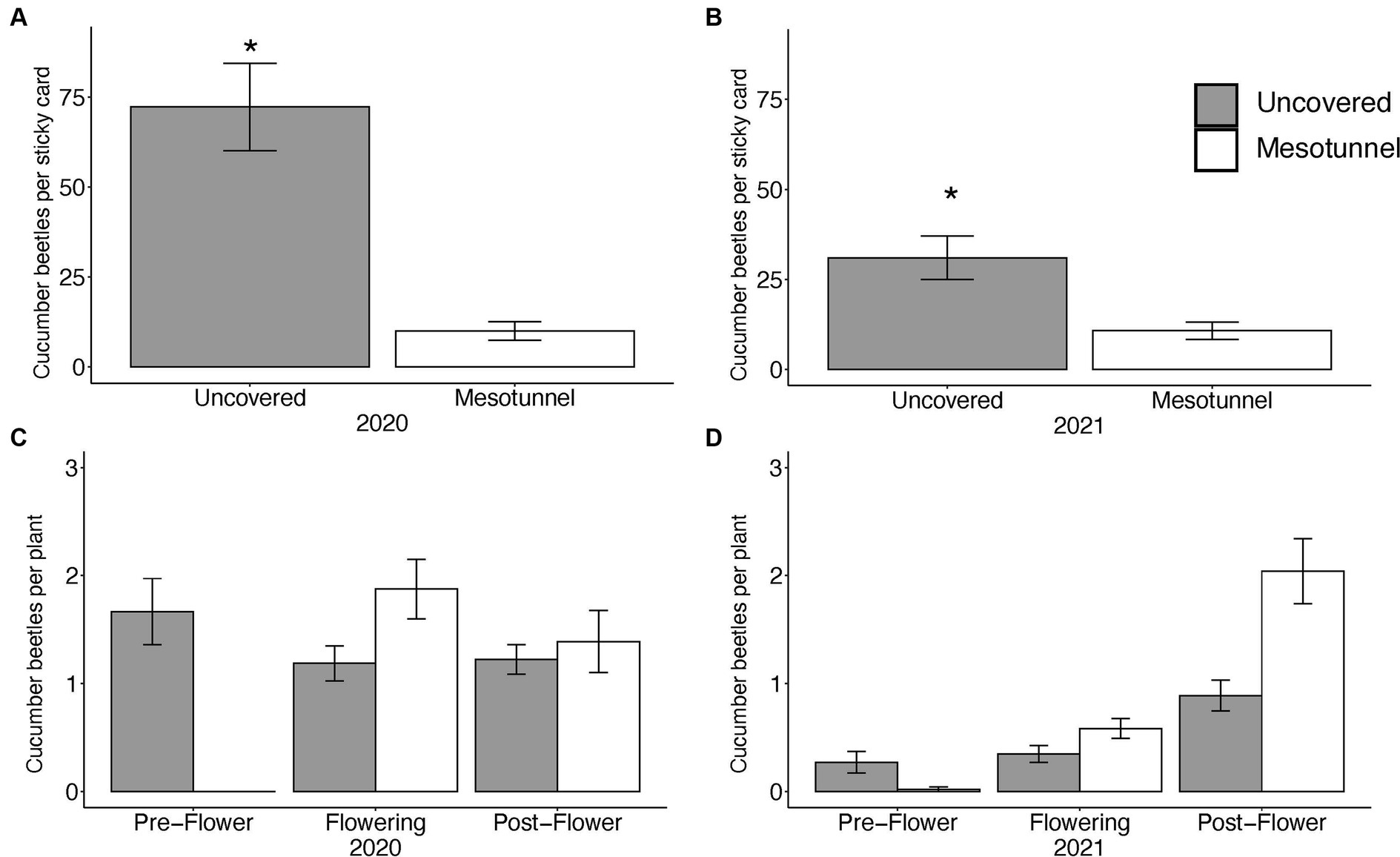
Figure 1. (A) Mean number of cucumber beetles on sticky traps in 2020. Uncovered treatments had nearly seven times more cucumber beetles than mesotunnel treatments. (B) Mean number of cucumber beetles on sticky traps in 2021. Uncovered treatments had nearly three times more cucumber beetles than mesotunnel treatments. Asterisks indicate significant effects at alpha = 0.05. (C) Number of cucumber beetles per visual survey (mean across all weeks) of acorn squash plants at each of the net-stages for 2020. There is an interaction between squash flower phenology and mesotunnel treatment. Pre-flower mesotunnel is signficantly different than any other net-stages and mesotunnel interactions in 2020. (D) Number of cucumber beetles per visual survey (mean across all weeks) of acorn squash plants at each of the net-stages for 2021. There is an interaction between squash flower phenology and mesotunnel treatment and the pre-flower mesotunnel interaction is different than any other net-stage and mesotunnel interactions.
Weekly visual surveys of cucumber beetles (per squash plant) revealed that the effect of the mesotunnel treatments varied across the pre-flower, flowering, and post-flower growth stages (net-stage factor) in both 2020 and 2021 (Figures 1C,D and Table 2). In the pre-flower stage, uncovered treatments had 1.7 and 0.3 times more cucumber beetles than did mesotunnel treatments in 2020 and 2021, respectively. However, in the flowering and post-flowering stages after mesotunnels had been opened (for pollination), the mesotunnel treatment tended to have more cucumber beetles than the uncovered treatments. In the flowering stage, the mesotunnel treatments had 1.6 and 2 times more cucumber beetles than did the uncovered treatments in 2020 and 2021, respectively. During the post-flowering stage in 2020 and 2021, the mesotunnel treatments had 1.2 and 2.2 times more cucumber beetles than did the uncovered treatments, respectively. There was no effect of the OMRI-pesticide treatments and no other significant interactions (Table 2).
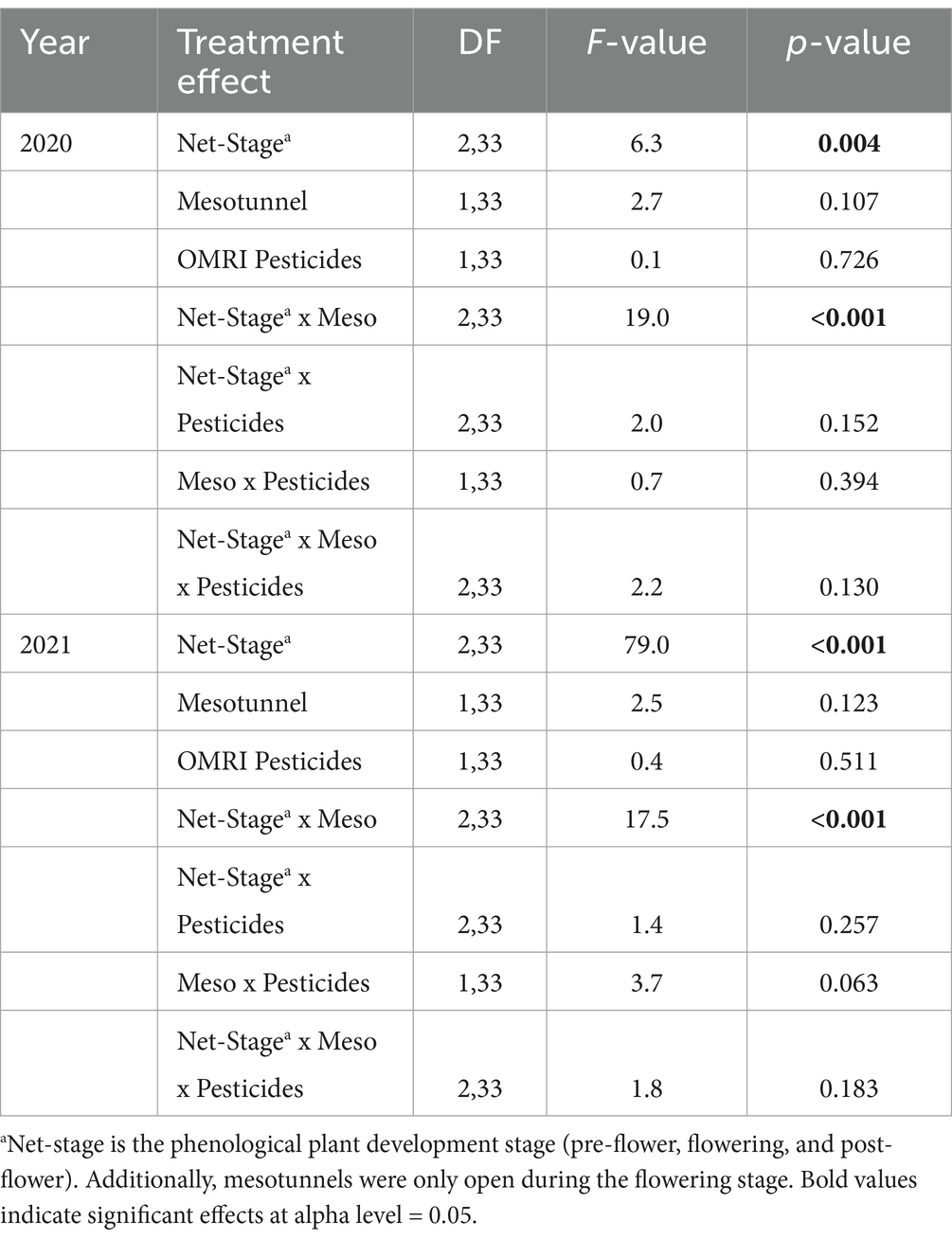
Table 2. Statistical comparisons of net-stage, mesotunnels, and OMRI pesticide treatment effects on the mean numbers of cucumber beetles observed in surveys from each year of the experiment.
3.2 Squash bugs
In 2020, squash bug adults were first observed within the second week of the experiment for the uncovered-untreated treatments, the first week for the uncovered, pesticide-only treatment, never in the mesotunnel-only treatment, and in the sixth week for the mesotunnel-pesticide treatment. In 2021, the first observations of squash bug adults were in the third or fourth week of the experiment for all treatments. The number of adult and nymphal squash bugs did not statistically differ between treatments across any net-stage in 2020 or 2021 (Tables 3, 4). Similarly, the number of squash bug eggs did not differ between treatments across any net-stage in 2020. However, the number of squash bug eggs was 6.5 times and 2.6 times greater in uncovered treatments compared to mesotunnel treatments in the flowering and post-flowering net-stages in 2021, respectively (Tables 3, 4).
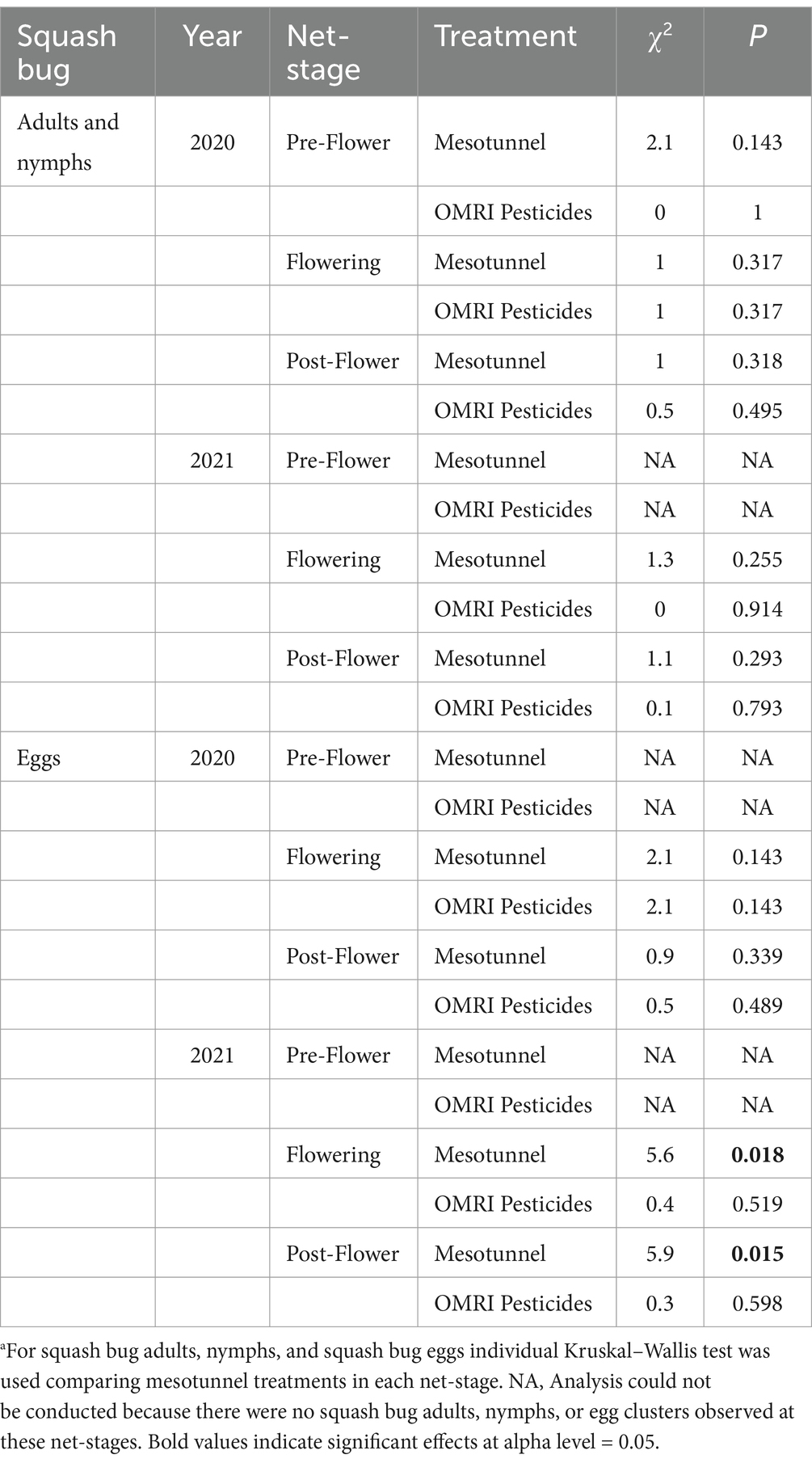
Table 3. Statistical comparisonsa of the net-stage, mesotunnel, and OMRI pesticide treatments on the combined relative abundance of squash bug adults, nymphs and eggs across 2020 and 2021.
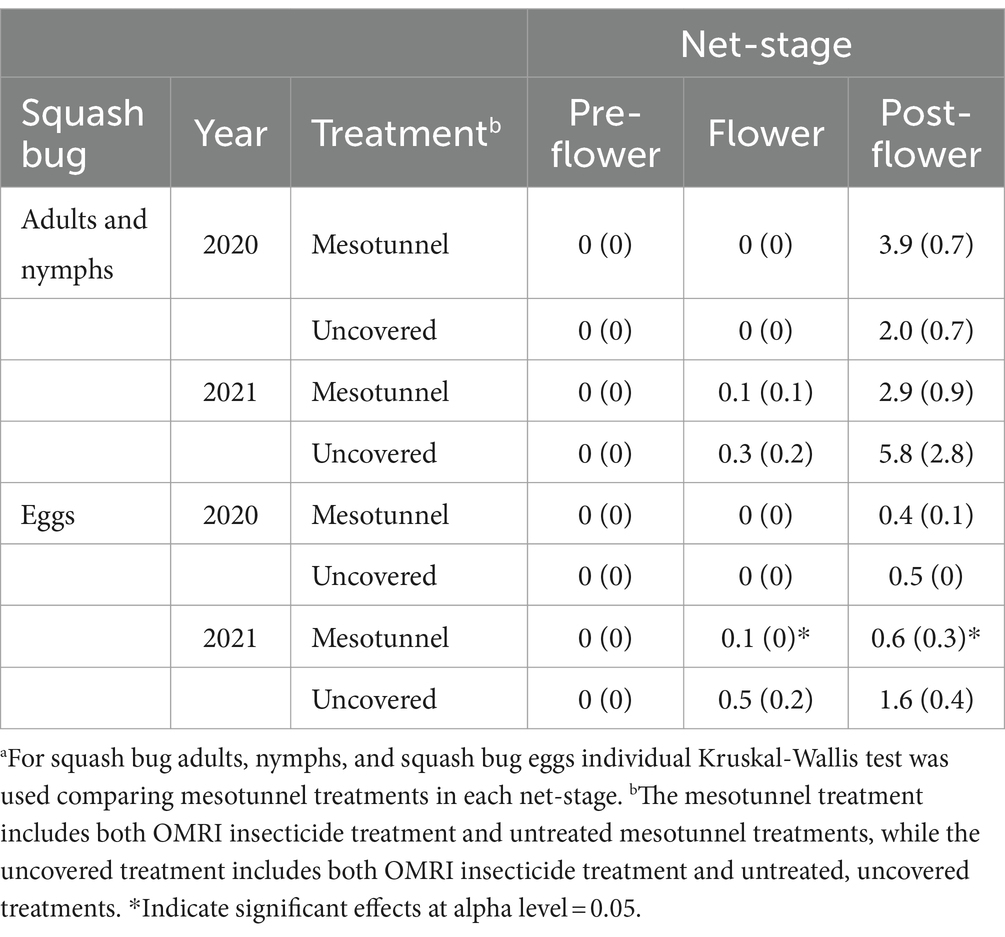
Table 4. Mean ± SE of the relative abundance of squash bug adults and nymphs and eggs at each net-stage in 2020 and 2021 for mesotunnel and uncovered treatmentsa.
3.3 Insect-vectored and non-vectored diseases
In 2020, no wilting symptoms indictive of bacterial wilt or CYVD were observed. In 2021, there was no difference in the occurrence of wilting symptoms across mesotunnel treatments (F1,12 = 0.1, p = 0.7232), OMRI-pesticide treatments (F1,12 = 0.2, p = 0.6712), or the interaction between treatments (F1,12 = 0.1, p = 0.7767).
In 2020, the uncovered treatments had 1.4 times more powdery mildew incidence compared to the mesotunnel treatments (Figure 2A). There was 1.9 times more powdery mildew incidence in untreated treatments relative to the OMRI-pesticide treatments (Figure 2B and Table 5), however there was no interaction between treatments. In 2021, there was 1.3 times more powdery mildew incidence in the uncovered treatments compared to the mesotunnel treatments (Figure 2C and Table 5). However, there was no effect of the OMRI-pesticide treatments nor an interaction between mesotunnel and OMRI-pesticide treatments in 2021 (Figure 2D and Table 5).
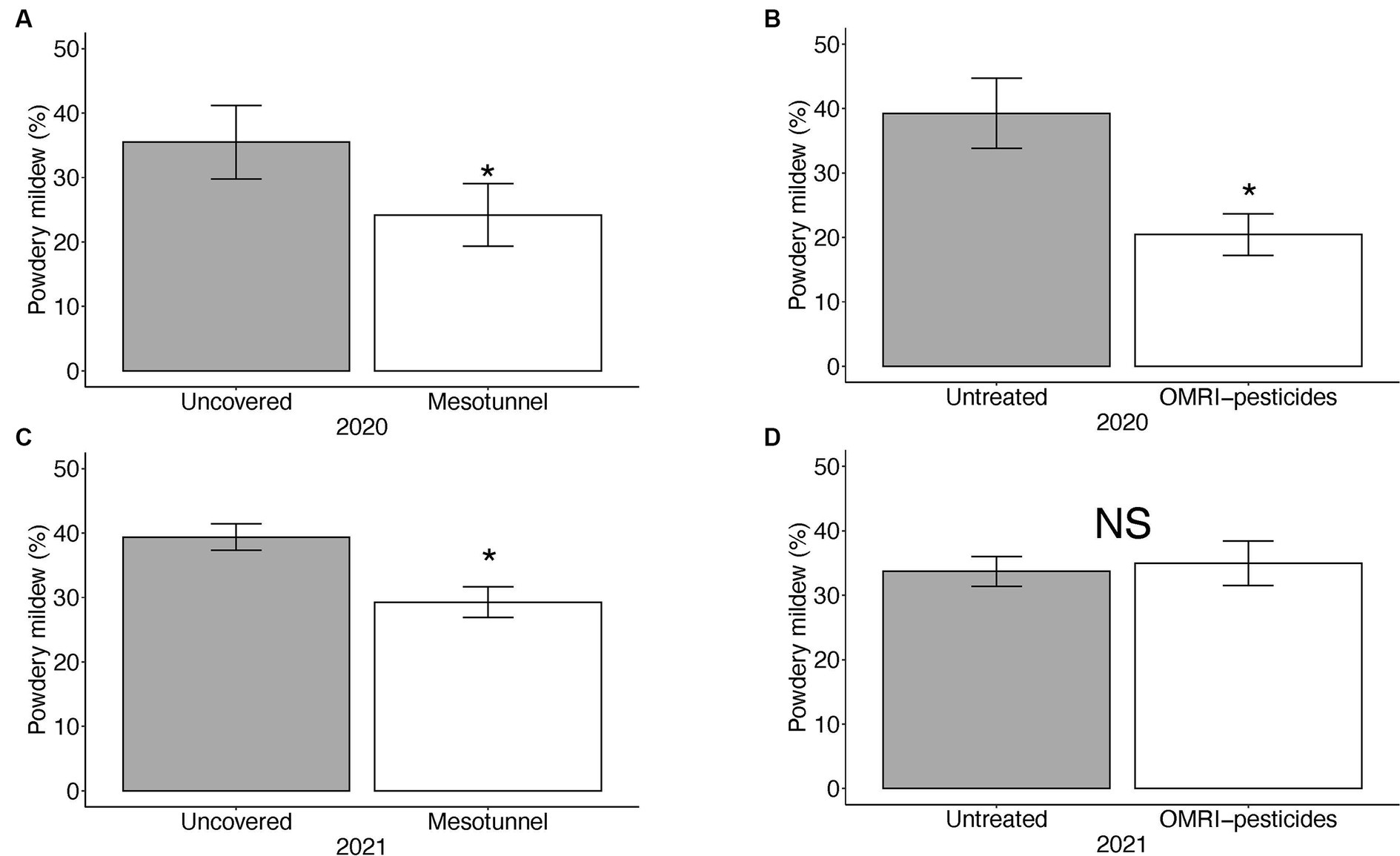
Figure 2. Mean percent of powdery mildew incidence per 10 acorn squash leaf samples per treatment. (A) There was an effect of mesotunnel and (B) OMRI pesticides on the powdery mildew incidence in 2020. (C) There was an effect of mesotunnels only on powdery mildew in 2021. (D) There was no effect of OMRI pesticides on powdery mildew incidence in 2021. Asterisks indicate significant effects at alpha level = 0.05. NS = not significant.
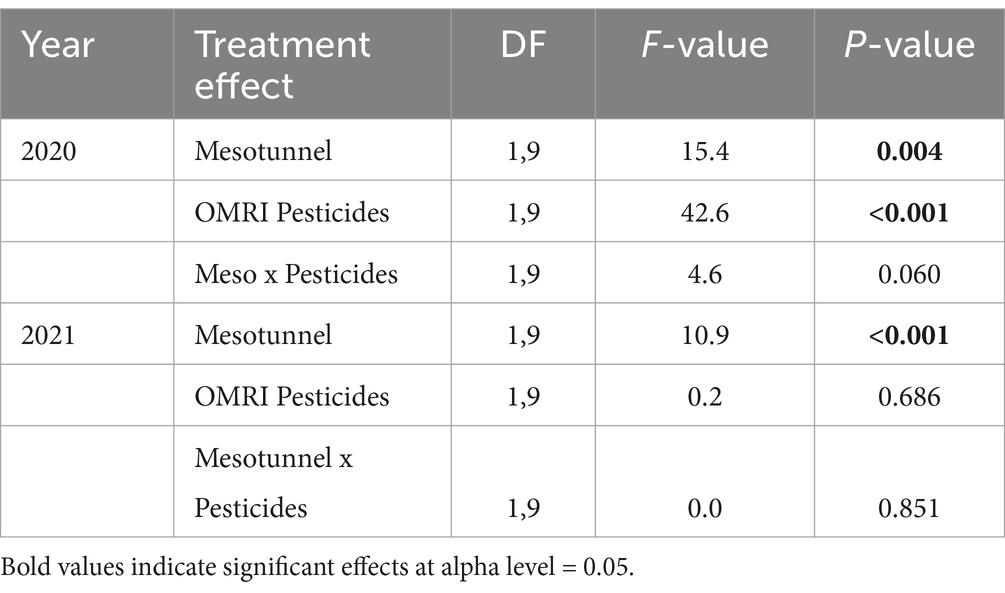
Table 5. Statistical comparisons of mesotunnel, OMRI Pesticides, and the interaction of mesotunnel and OMRI pesticides on powdery mildew incidence across 2020 and 2021.
3.4 Squash yield
The mesotunnel treatments had 54 and 46% higher marketable yield compared to the uncovered treatments in 2020 and 2021, respectively (Figures 3A,C and Table 6). The OMRI-pesticide treatments had no effect on marketable yield in 2020 (Figure 3B) and there was no interaction between treatments. In 2021, marketable yield in the untreated treatments tended to be lower than the OMRI-pesticide treatments (Figure 3D and Table 6). We found no difference in unmarketable yield between treatments for either 2020 (Supplementary Table S1) or 2021 (Supplementary Tables S2, S3).
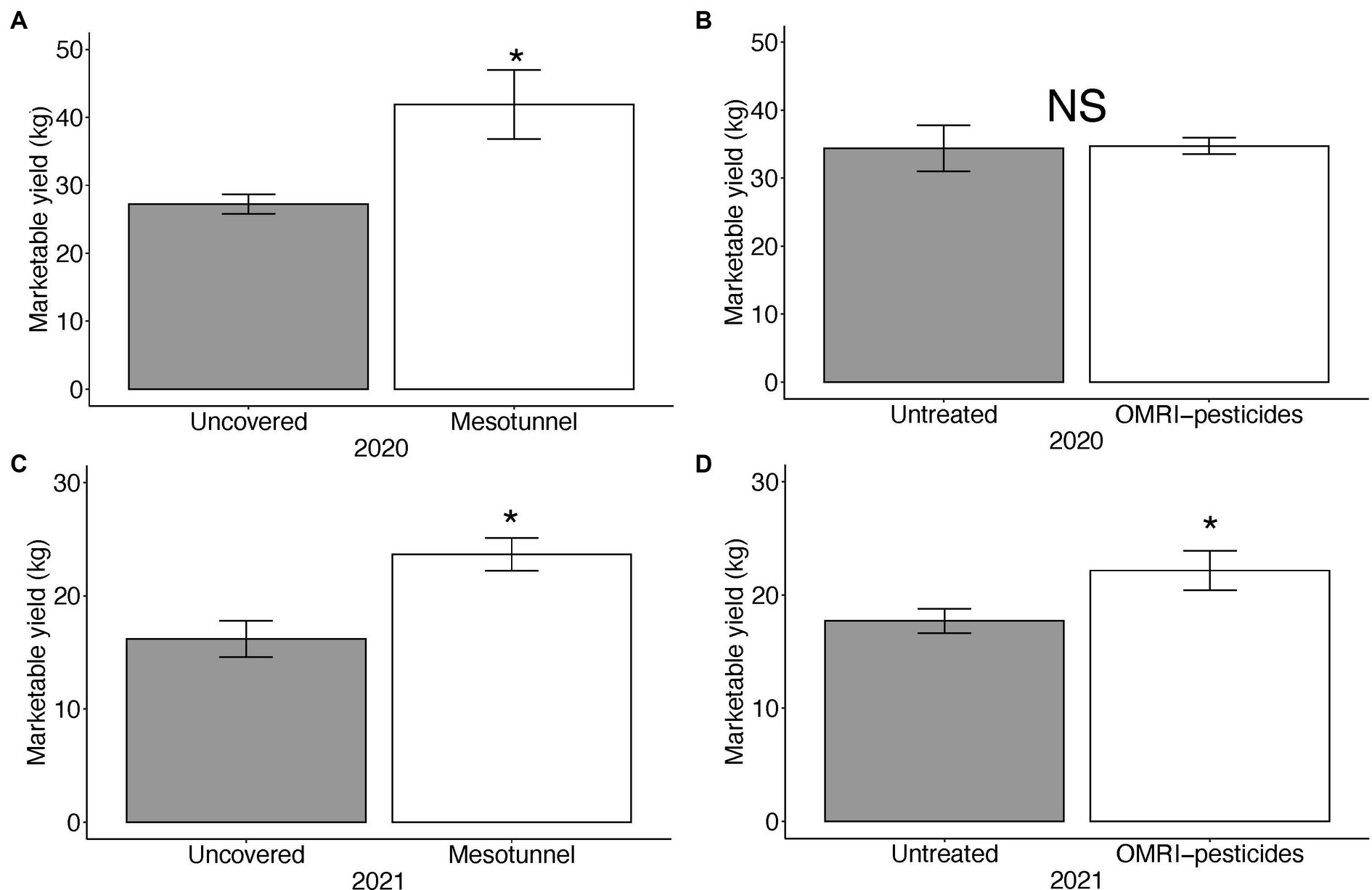
Figure 3. Mean marketable weight (kg.) of acorn squash yield across 2020 and 2021. (A) There was an effect of mesotunnels on marketable yield in 2020, (B) but not of OMRI pesticides. There was an effect of mesotunnels (C) and a slight effect of OMRI pesticides (D) on yield in 2021. Asterisks indicate significant effects at alpha level = 0.05. NS = not significant.
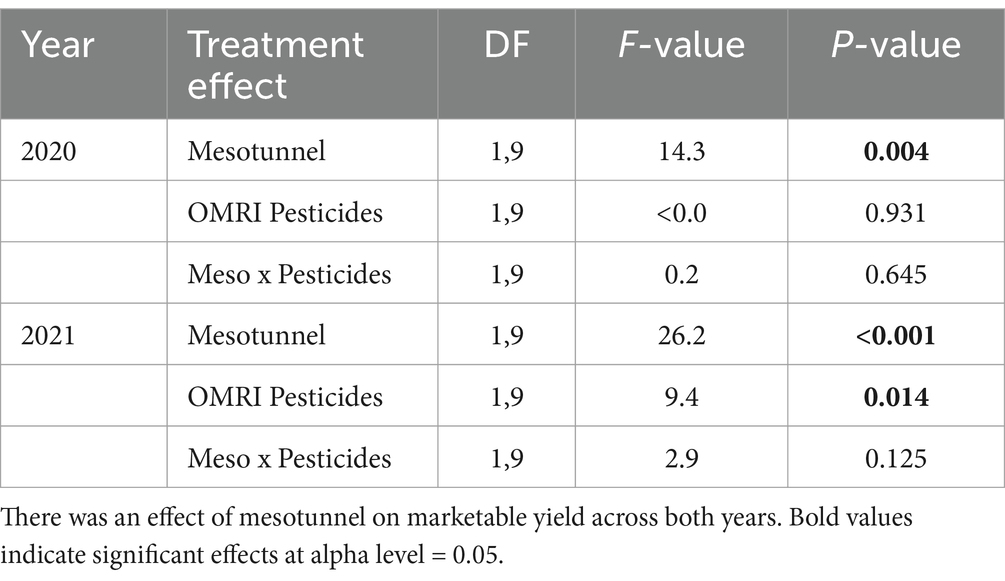
Table 6. Statistical comparisons of mesotunnel, OMRI pesticides, and the interaction of mesotunnel and OMRI pesticides on the marketable weight (kg) of acorn squash.
3.5 Microclimate
We found small differences in the minimum, mean, and maximum temperature, humidity, and dewpoint between the mesotunnel and uncovered treatments in some net-stages and years of the study (Supplementary Tables S4–S6 and Supplementary Figures S1–S3). However, with both years of data combined, the mesotunnel treatments did not alter temperature, humidity, or dewpoint compared to the uncovered treatments (Table 7 and Supplementary Figure S4).
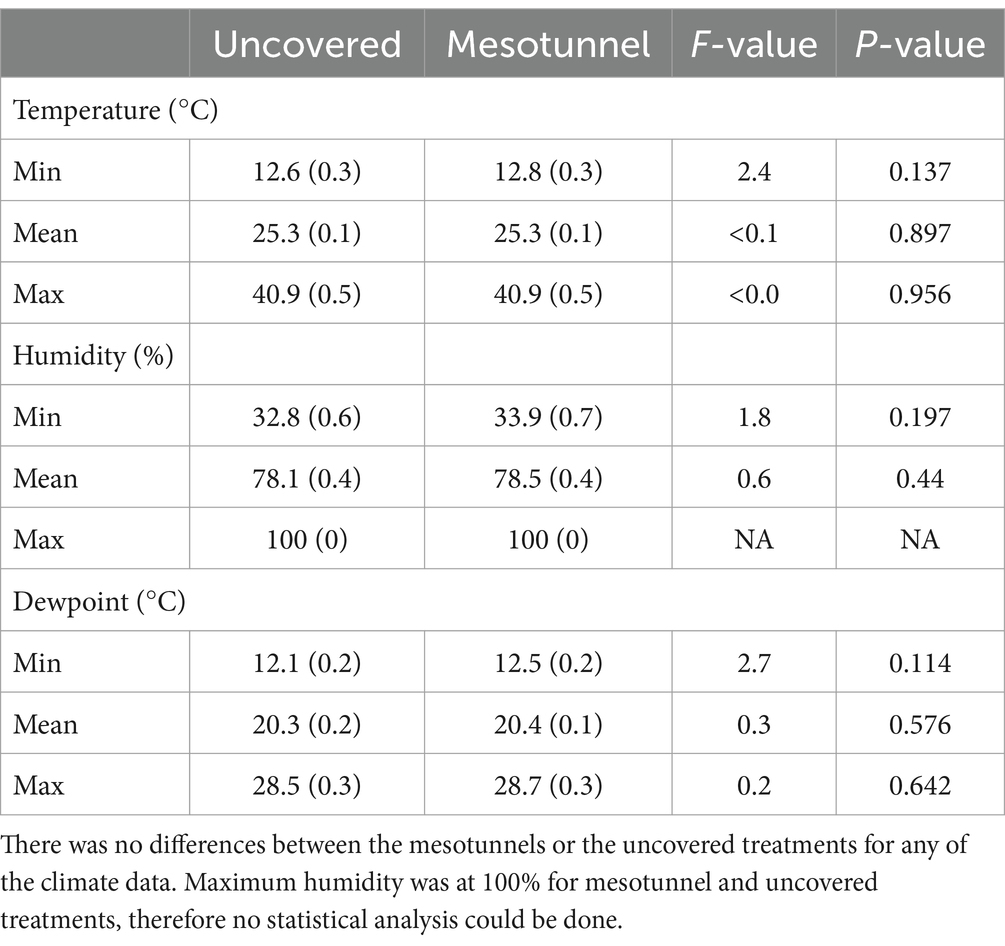
Table 7. Statistical comparisons and the means ± standard errors across both years of the climate data for mesotunnel treatments and uncovered treatments.
3.6 Economic
Total labor use (hours) was lowest in the uncovered-untreated treatment, followed by the pesticide-only, then the mesotunnel-only, and finally the mesotunnel-pesticide treatment (Supplementary Table S7). The mesotunnel-only treatment required only 2.7% more labor hours compared with the pesticide-only treatment.
Similarly, the uncovered-untreated treatment had the lowest total costs associated with production, followed by the mesotunnel-only, then the pesticide-only, and finally the mesotunnel -pesticide treatment (Table 8). In 2020 and 2021, total costs for mesotunnel-only treatment were 8 and 1% lower than the pesticide-only treatment, respectively. Total costs for mesotunnel-only, pesticide-only, and the mesotunnel-pesticide treatments were double that of the uncovered- untreated treatment in both 2020 and 2021.
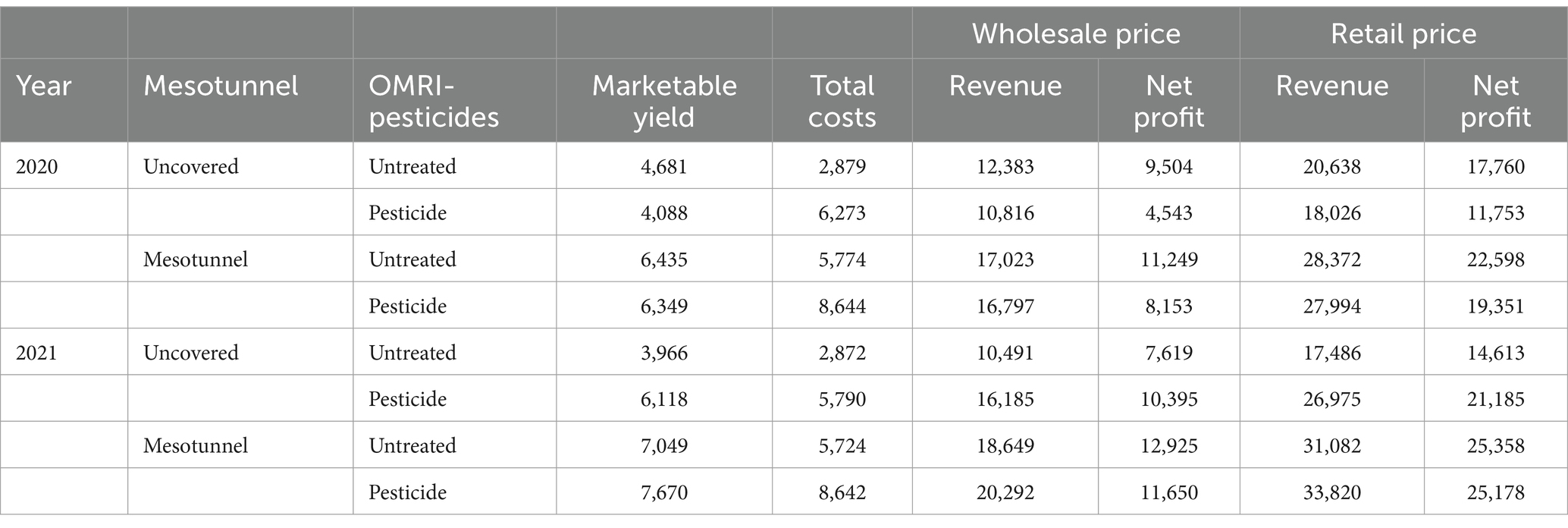
Table 8. Marketable yield (kg), revenue and profit ($) of acorn squash from 2020 and 2021 for 0.4 hectares (1 acre) plot for each treatment.
Retail prices ($4.40/kg) greatly increased the revenue generated per 0.4 hectares relative to wholesale prices ($2.65/kg) (Table 8). Below for simplicity, the revenue generated across treatments are summarized for retail prices, however patterns across treatments were consistent among retail and wholesale prices. In 2020 and 2021, the mesotunnel-only treatment had 57 and 15% greater retail revenue than the pesticide-only treatment. Compared to the uncovered-untreated treatment, the mesotunnel-only treatment had 37 and 78% greater retail revenue in 2020 and 2021. The mesotunnel-pesticide treatment had 55 and 25% greater retail revenue than the pesticide-only treatment in 2020 and 2021, respectively. Compared to the uncovered-untreated treatment, the mesotunnel-pesticide treatment had 36 and 93% greater retail revenue in 2020 and 2021.
The retail net profit was highest in the mesotunnel-only treatment in both 2020 and 2021, while the mesotunnel-pesticide treatment followed closely behind. In 2020 and 2021, the mesotunnel-only treatment had 92 and 20% higher retail net profit than the pesticide-only treatment, respectively. Compared to the uncovered-untreated treatment, the mesotunnel-only treatment had 27 and 74% higher retail net profit in 2020 and 2021. The mesotunnel-pesticide treatment had 65 and 19% higher retail net profit than the pesticide-only treatment in 2020 and 2021, respectively. Compared to the uncovered-untreated treatment, the mesotunnel-pesticide treatment had 9 and 72% higher retail net profit in 2020 and 2021.
The mesotunnel-only treatment had the highest Net Present Value across 2020 and 2021 (Table 9). The mesotunnel-only treatment increased the Net Present value by 47% increase in net profit, followed by the mesotunnel-pesticide treatment (36% or $10,588 per 0.4 hectares) compared to the pesticide-only treatment. Compared to the uncovered-untreated control, the mesotunnel-only treatment also has a 47% ($13,907 per 0.4 hectares) increase in net profit, followed by the mesotunnel-pesticide treatment (36% or $10,714 per 0.4 hectares). We also conducted a robustness check applying the 3% discount rate to generate NPV (Table 9), which shows similar results and confirms the stability of our analysis.
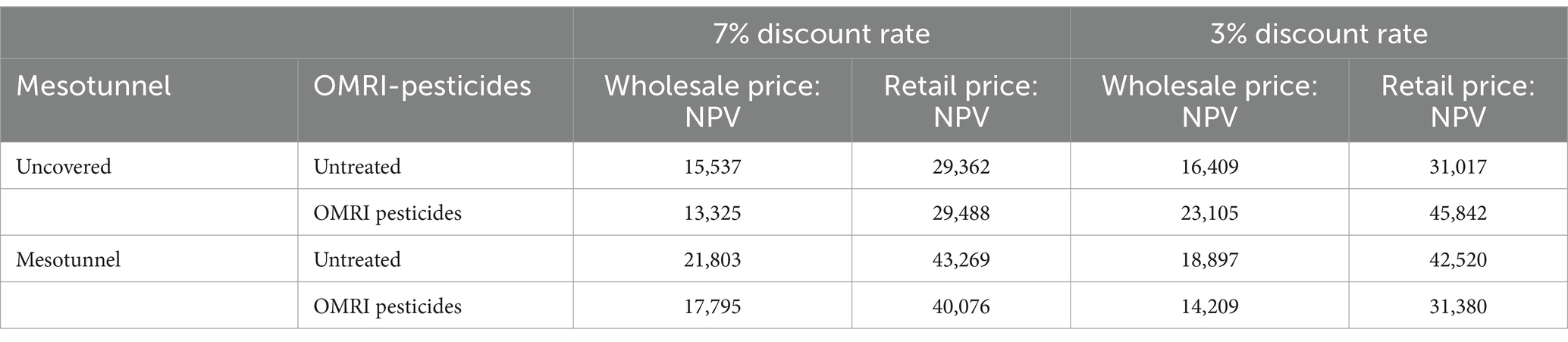
Table 9. Net present values ($ per 0.4 hectares) across 2020 and 2021 net profits of acorn squash for retail and wholesale prices using a 7% discount rate and a 3% discount rate for a robustness check.
4 Discussion
This study highlights three important findings regarding the mesotunnel system: (1) Mesotunnels reduced insect pests and increased marketable yield, but their effects on pests depended on the stages of net management for pollination. (2) Mesotunnels reduced the incidence of powdery mildew. (3) Mesotunnels increased profitability relative to uncovered controls and OMRI-listed pesticide treatments. Together these results offer support that mesotunnels are a highly efficacious pest management strategy that reduce the reliance on organic insecticide use and are cost-effective for organic producers.
4.1 Row covers reduce pests and increase yield
Our findings are consistent with prior evidence that row covers reduce insect damage and pest abundance and increase marketable yield in a variety of crops (Moreno et al., 2002; Rojas et al., 2011; Gogo et al., 2014; Kuesel et al., 2019; Nair et al., 2019; Skidmore et al., 2019; Brockman et al., 2020; Acharya et al., 2020b; Athey et al., 2021; Nelson et al., 2023). We found that mesotunnels reduce cucumber beetles significantly during the early developmental stages of cucurbit growth when nets were closed (before flowering). However, we did find more cucumber beetles under mesotunnels compared to uncovered treatments during the flowering and post-flowering net-stages. Given that acorn squash, like many cucurbits, is highly dependent on bee pollination for fruit set, mesotunnels are often partially opened or removed entirely during flowering. Once the mesotunnels have been opened for pollination, the acorn squash plants can be colonized by cucumber beetles. Given that row covers increase the growth and size of cucurbit plants (Acharya et al., 2019, 2020a) it is likely that cucumber beetles were more attracted to mesotunnel plants during the flowering stage resulting in greater abundance per plant. We also observed larger and longer flowering blooms under mesotunnels (Unpublished data) which could have been more attractive to cucumber beetles; larger flowers have been found to be more attractive to cucumber beetles (Theis et al., 2014). After the mesotunnels were closed after flowering, it is possible that insect pests were trapped within the tunnels resulting in a higher density per plant through the post-flowering net-stage. Striped cucumber beetles can complete one to three generations per year with egg incubation taking 5-9 days and larval development taking 2–4 weeks (Legault, 2007). Therefore, it is possible the flowering and post-flowering net-stages (~35 days) is sufficient for the beetles to complete one life cycle and increase in abundance by the end of the post-flowering net-stage.
Despite observing higher cucumber beetle abundance in mesotunnels after flowering, we still found that marketable yields were higher in the mesotunnel treatments compared to the uncovered treatments. Prior evidence suggests that early feeding damage on cotyledon and the first-leaf stage can cause yield reductions due to a loss of foliar surface area and a reduction in plant vigor (Brewer et al., 1987). Larger, mature plants may be more resistant to cucumber beetle damage because they have a greater leaf surface area per beetle and they have larger root systems that are better able to regenerate damaged plant tissue (Brewer et al., 1987). Furthermore, greenhouse studies have found that only 10% of squash plants that have reached the first and third true leaf stages show symptoms of CYVD compared to 60% of squash plants that have reached the un-expanded cotyledon stage (Bruton et al., 2003). Our results provide evidence that protecting cucurbits from insect pests early in development is more important for cucurbit yield than protecting cucurbits at later developmental stages (past flowering).
While we found fewer squash bug eggs under mesotunnels in the flowering and post-flowering net-stages (in 2021), we found no effect of mesotunnels on adults and nymphs in either year. The effect of mesotunnels on squash bugs may have deviated from the observed effect on cucumber beetles because squash bug abundance increased later in the season during the flowering and post-flowering stages. Cucumber beetles were present in sufficient densities to cause damage at the time of planting, while squash bugs lagged behind one to 6 weeks after planting.
Our study did not observe high incidence of bacterial wilt or CYVD. In fields where there has been a history of bacterial wilt or CYVD, alternative mesotunnel strategies may be deployed. We deployed part-season pollination strategies (on–off–on in 2020, opened ends in 2021), which allow wild pollinators to visit squash flowers for pollination, but also allow pests to breach the mesotunnel. A full season mesotunnel strategy that maintains nets on during the entire season can be achieved by stocking tunnels with commercial bumble bee colonies. When high disease pressure from bacterial wilt or CYVD is present, a full season mesotunnel increased marketable yield four times higher than uncovered control (Nelson et al., 2023).
4.2 Effect of mesotunnels on powdery mildew
Contrary to our hypothesis, we found 36% (2020) and 25% (2021) lower incidence of powdery mildew in mesotunnel treatments compared to the uncovered treatments. Few studies have compared the effect of row covers on the incidence of powdery mildew. Minter and Bessin (2014) noted that full season row covers appeared to have less incidence of powdery mildew than part-season row covers. To our knowledge, no other row cover studies have specifically looked at powdery mildew incidence.
Mesotunnels may reduce powdery mildew by altering the microclimate or by acting as a physical barrier. Some research suggests that powdery mildew develops best at 35°C and 70% relative humidity (Sharma et al., 2016), and under low light conditions (Cheah et al., 1996). However, temperature and relative humidity did not differ significantly across mesotunnel and uncovered treatments. Further, mesotunnels reduce solar radiance by approximately 8% (Dubois Agrinovation, 2022) which should increase powdery mildew incidence. Yet in our study we found lower incidence under mesotunnels. Mesotunnels may also act as partial physical barriers to powdery mildew spores. While this has never been studied in mesotunnel systems, polyculture studies that manipulate host and non-host densities show that non-host plants can act as physical barriers to pathogen spore movement. For example Luo et al. (2021) and Villegas-Fernández et al. (2021) found that different plant canopy structures and different non-host crops reduced powdery mildew on wheat and pea. Ratnadass et al. (2012) also found that air-borne and splash-borne pathogens can be affected by plant physical barriers. Thus, it is plausible that row covers also act as a partial physical barrier to pathogen spores. Future studies should investigate the use of spore traps inside and outside of row covers to determine whether the mesotunnel can act as a physical barrier.
4.3 Mesotunnels increase profitability
Lastly, we found support for our hypothesis that the mesotunnel-only treatment is the most profitable. Perhaps surprisingly, the material and labor costs of implementing mesotunnels alone were lower or roughly equivalent to the pesticide-only treatment (Table 8). OMRI-listed pesticides are expensive and the six to seven applications per year do require significant labor hours making mesotunnels competitive with organic pesticide management strategies. However, the revenue generated by the mesotunnel-only treatment was roughly equivalent to the mesotunnel-pesticide and was far greater than the uncovered-untreated and the pesticide-only treatments. Similar to our study, Nelson et al. (2023) found that mesotunnel systems had greater total costs compared to uncovered, untreated treatments. However, the mesotunnel system had significantly greater cost efficiencies due to the higher marketable yield beneath the mesotunnels in organic muskmelon (Nelson et al., 2023). Rojas et al. (2011) also found that mesotunnel costs were much higher than the uncovered, untreated control. However, in some years with high bacterial wilt disease, the mesotunnels had a higher net return than the uncovered, untreated treatments (Rojas et al., 2011). These studies provide strong evidence that mesotunnels may be an economically efficient strategy for organic pest management of cucurbit pests and the associated diseases. In conditions with higher wilt and CYVD, mesotunnels may be even more cost effective than uncovered or insecticide treatments.
At the same time, 46% of organic farmers perceive accessing labor as a non-production challenge (Snyder et al., 2022). The increased labor requirements of mesotunnels at large scales may act as a barrier to adoption of this practice. This study did not attempt to compare the number of workers needed to manage mesotunnels relative to other treatments at this scale. However, growers have reported that 3–8 workers are needed to manage mesotunnels at approximately 0.4 hectares (Personal communications). This is likely a greater labor requirement than that needed to manage the same area with insecticide use but is likely not greater than the labor required to manage a vegetable operation that would implement mesotunnels at a 0.4 hectare scale. For example, for a 4–5 hectare (10–12 acres) diversified vegetable operation (University of Kentucky Community Supported Agriculture) typically employs five full time workers and 6–10 workers during peak summer harvest (Durbin et al., 2022). To help guide growers seeking to adopt mesotunnels, future research should identify the appropriate scales of mesotunnel use for different sized operations and the corresponding number of workers needed to do so.
It should also be noted that the pesticide-only treatment was relatively ineffective at suppressing pests, was costly, and did not increase yield making it roughly as profitable as the uncovered-untreated treatment. However, in 2020, the pesticide-only treatment did reduce the incidence of powdery mildew relative to the uncovered-untreated treatment but was only marginally different from the mesotunnel treatments. Furthermore, the pesticide-only treatment was not consistent every year, as it did not reduce powdery mildew relative to the mesotunnel-only treatments or the uncovered-untreated treatment in 2021. The total costs for the pesticide-only treatment were greater than the uncovered-untreated control and the mesotunnel-only treatment. One of the insecticides used, Azera, can cost up to $415 for one gallon. Due to this underperformance and low revenue generation, it is not surprising that the high costs of this management technique limit their cost-effectiveness.
5 Conclusion
We find support that row covers limit insect pests at vital stages of plant growth, limit non-vectored diseases, such as powdery mildew and increase marketable yield. We did not find that the microclimate underneath the mesotunnel was significantly different than the uncovered treatments. While the costs of the mesotunnel system are roughly the same as the OMRI-listed insecticides, they increase yield, making mesotunnels more cost effective than OMRI-listed insecticides. This study provides strong evidence that mesotunnels may be an ecologically and economically efficient strategy for pest management of cucurbit pests and their associated diseases.
Data availability statement
The raw data supporting the conclusions of this article will be made available by the authors, without undue reservation.
Author contributions
KF: Conceptualization, Data curation, Formal analysis, Methodology, Project administration, Writing – original draft, Writing – review & editing. NC: Formal analysis, Writing – review & editing. RK: Formal analysis, Writing – review & editing. WZ: Conceptualization, Writing – review & editing, Formal analysis, Methodology. RB: Conceptualization, Supervision, Writing – review & editing. MW: Conceptualization, Supervision, Writing – review & editing. DG: Conceptualization, Formal analysis, Funding acquisition, Supervision, Writing – review & editing.
Funding
The author(s) declare that financial support was received for the research, authorship, and/or publication of this article. This experiment was supported by OREI ‘Resilient Systems for Sustainable Management of Cucurbit Crops’ Grant # 2019–51300-30248 and USDA Hatch grant (KY008079).
Acknowledgments
We would like to thank Chelsea Avery, Briana Bazile, Robert Brockman, Isaac D’Souza, Karina Garcia, Alexis Gauger, Colby Grant, Nate Haan, Viktor Halmos, Sarah Jones, Holly Meyers, Emily Pfeufer, Delia Scott, and Kantima Thongjued for their help in reviewing, scouting, sampling and/or harvest. We would also like to thank Steve Diver, Aaron German, Jay Tucker, and Neil Wilson at the University of Kentucky Horticulture Research Farm for their assistance and guidance in field set up and irrigation maintenance.
Conflict of interest
The authors declare that the research was conducted in the absence of any commercial or financial relationships that could be construed as a potential conflict of interest.
Publisher’s note
All claims expressed in this article are solely those of the authors and do not necessarily represent those of their affiliated organizations, or those of the publisher, the editors and the reviewers. Any product that may be evaluated in this article, or claim that may be made by its manufacturer, is not guaranteed or endorsed by the publisher.
Supplementary material
The Supplementary material for this article can be found online at: https://www.frontiersin.org/articles/10.3389/fsufs.2024.1347924/full#supplementary-material
References
Acharya, T. P., Reiter, M. S., Welbaum, G., and Arancibia, R. A. (2020a). Nitrogen uptake and use efficiency in sweet basil production under low tunnels. HortScience 55, 429–435. doi: 10.21273/hortsci14515-19
Acharya, T. P., Welbaum, G. E., and Arancibia, R. A. (2019). Low tunnels reduce irrigation water needs and increase growth, yield, and water-use efficiency in Brussels sprouts production. HortScience 54, 470–475. doi: 10.21273/hortsci13568-18
Acharya, T. P., Welbaum, G. E., and Arancibia, R. A. (2020b). Low tunnels reduce insect populations, insecticide application, and chewing insect damage on Brussels sprouts. J. Econ. Entomol. 113, 2553–2557. doi: 10.1093/jee/toaa154
Advisers, C. o. E. (2017). Discounting for public policy: Theory and recent evidence on the merits of updating the discount rate Washington, D.C: Council of Economic Advisers Issue Brief. Blacksburg, VA: Virginia Cooperative Extension.
Arancibia, R. (2018). Low tunnels in vegetable crops: beyond season extension. Blacksburg, VA: Virginia Cooperative Extension.
Athey, K. J., Peterson, J. A., Dreyer, J., Harwood, J. D., and Williams, M. A. (2021). Effect of breathable row covers and ground cover on Pest insect levels and cucurbit yield. J. Econ. Entomol. 115, 193–200. doi: 10.1093/jee/toab212
Bates, D., Mächler, M., Bolker, B., and Walker, S. (2015). Fitting linear mixed-effects models using lme4. J. Stat. Softw. 67, 1–48. doi: 10.18637/jss.v067.i01
Bernardes, R. C., Barbosa, W. F., Martins, G. F., and Lima, M. A. P. (2018). The reduced-risk insecticide azadirachtin poses a toxicological hazard to stingless bee Partamona helleri (Friese, 1900) queens. Chemosphere 201, 550–556. doi: 10.1016/j.chemosphere.2018.03.030
Brewer, M. J., Story, R. N., and Wright, V. L. (1987). Development of summer squash seedlings damaged by striped and spotted cucumber beetles (Coleoptera: Chrysomelidae). J. Econ. Entomol. 80, 1004–1009. doi: 10.1093/jee/80.5.1004
Brockman, R., Kuesel, R., Archer, K., O'Hearn, K., Wilson, N., Scott, D., et al. (2020). The impact of plant essential oils and fine mesh row covers on flea beetle (Chrysomelidae) Management in Brassicaceous Greens Production. Insects 11:714. doi: 10.3390/insects11100714
Brust, G. E., and Foster, R. E. (1999). New economic threshold for striped cucumber beetle (Coleoptera: Chrysomelidae) in cantaloupe in the Midwest. J. Econ. Entomol. 92, 936–940. doi: 10.1093/jee/92.4.936
Brust, G. E., Foster, R. E., and Buhler, W. G. (1996). Comparison of insecticide use programs for managing the striped cucumber beetle (Coleoptera: Chrysomelidae) in muskmelon. J. Econ. Entomol. 89, 981–986. doi: 10.1093/jee/89.4.981
Bruton, B., Mitchell, F., Fletcher, J., Pair, S., Wayadande, A., Melcher, U., et al. (2003). Serratia marcescens, a phloem-colonizing, squash bug-transmitted bacterium: causal agent of cucurbit yellow vine disease. Plant Dis. 87, 937–944. doi: 10.1094/PDIS.2003.87.8.937
Brzozowski, L., Leckie, B. M., Gardner, J., Hoffmann, M. P., and Mazourek, M. (2016). Cucurbita pepo subspecies delineates striped cucumber beetle (Acalymma vittatum) preference. Hortic. Res. 3:16028. doi: 10.1038/hortres.2016.28
Burkness, E. C., and Hutchison, W. D. (1998). Action thresholds for striped cucumber beetle (Coleoptera: Chrysomelidae) on ‘Carolina’ cucumber. Crop Prot. 17, 331–336. doi: 10.1016/S0261-2194(98)00021-0
Calkins, P. H., and DiPietre, D. D. (1983). Farm business management: Successful decisions in a changing environment New York: Macmillan Co.
Carlson, A., Greene, C., Raszap Skorbiansky, S., Hitaj, C., Ha, K., Cavigelli, M., et al. (2023). US organic production, markets, consumers, and policy, 2000–2021. ERR-315, U.S. Department of Agriculture, Economic Research Service.
Cheah, L., Page, B., and Cox, J. (1996). “Epidemiology of powdery mildew (Sphaerotheca fuliginea) of squash”, in: Proceedings of the New Zealand Plant Protection Conference), 147–151.
Cui, X., Li, Y., Wang, X., and Wang, Z. (2022). Analysis of the net present value and equivalent annual cost in optimal machine life, in: 2022 7th International Conference on Financial Innovation and Economic Development (ICFIED 2022): Atlantis Press), 2929–2933.
Desneux, N., Decourtye, A., and Delpuech, J.-M. (2007). The sublethal effects of pesticides on beneficial arthropods. Annu. Rev. Entomol. 52, 81–106. doi: 10.1146/annurev.ento.52.110405.091440
Dively, G. P., Patton, T., Barranco, L., and Kulhanek, K. (2020). Comparative efficacy of common active ingredients in organic insecticides against difficult to control insect pests. Insects 11:614. doi: 10.3390/insects11090614
Doughty, H. B., Wilson, J. M., Schultz, P. B., and Kuhar, T. P. (2016). Squash bug (Hemiptera: Coreidae): biology and Management in Cucurbitaceous Crops. J. Integr. Pest Manag. 7:8. doi: 10.1093/jipm/pmv024
Dubois Agrinovation (2022). “ProtekNet brochure 2022”, Available at: https://duboisag.com/us_en/exclusion-insect-netting-knitted-60g-proteknet.html. (ed.) D. Agrinovation.
Durbin, K., Williams, M., Jacobsen, K., and Rudolph, R. (2022). Community supported agriculture (CSA) production manual of the organic farming unit at the University of Kentucky. Kentucky: Masters of Science.
Evans, W. B. (2016). “Season extension in organic systems” in Organic farming for sustainable agriculture. ed. D. Nandwani (Cham: Springer International Publishing Ag), 293–314.
Gogo, E. O., Saidi, M., Itulya, F. M., Martin, T., and Ngouajio, M. (2014). Eco-friendly nets and floating row covers reduce pest infestation and improve tomato (Solanum lycopersicum L.) yields for smallholder farmers in Kenya. Agronomy 4, 1–12. doi: 10.3390/agronomy4010001
Hanna, H. M., Polk, D. N., Rosentrater, K. A., and Steward, B. L. (2016). “Economic analysis of row cover insect exclusion for cucurbit crops” in 2016 ASABE annual international meeting (St. Joseph, Michigan: American Society of Agricultural and Biological Engineers), 1.
Hoffmann, M. P., Ayyappath, R., and Kirkwyland, J. J. (2000). Yield response of pumpkin and winter squash to simulated cucumber beetle (Coleoptera: Chrysomelidae) feeding injury. J. Econ. Entomol. 93, 136–140. doi: 10.1603/0022-0493-93.1.136
Kuesel, R., Scott Hicks, D., Archer, K., Sciligo, A., Bessin, R., and Gonthier, D. (2019). Effects of fine-mesh exclusion netting on pests of blackberry. Insects 10:249. doi: 10.3390/insects10080249
Legault, G. (2007). Effect of kaolin on the striped cucumber beetle (Acalymma vittatum) and cucumber growth and development. Montreal Quebec: McGill University.
Lenth, R., Singmann, H., Love, J., Buerkner, P., and Herve, M. (2019). Emmeans - Estimated Marginal Means, aka Least-Squares Means R package version 1.8/4–1/).
Lilley, J. M., and Sanchez, E. S. (2016). The potential of strip tillage and rowcovers for organic cucurbit production. HortTechnology 26, 628–636. doi: 10.21273/horttech03405-16
Luo, C., Ma, L., Zhu, J., Guo, Z., Dong, K., and Dong, Y. (2021). Effects of nitrogen and intercropping on the occurrence of wheat powdery mildew and stripe rust and the relationship with crop yield. Front. Plant Sci. 12:637393. doi: 10.3389/fpls.2021.637393
Marine, S. C., Newark, M. J., Korir, R. C., and Everts, K. L. (2016). Evaluation of rotational biopesticide programs for disease management in organic cucurbit production. Plant Dis. 100, 2226–2233. doi: 10.1094/PDIS-02-16-0252-RE
McGrath, M. T. (2015). “Cucurbit powdery mildew in the USA” in Fungicide resistance in plant pathogens (Tokyo: Springer), 401–417.
Middleton, E. (2018). Biology and management of squash vine borer (Lepidoptera: Sesiidae). J. Integ. Pest Manag. 9:8. doi: 10.1093/jipm/pmy012
Minter, L. M., and Bessin, R. T. (2014). Evaluation of native bees as pollinators of cucurbit crops under floating row covers. Environ. Entomol. 43, 1354–1363. doi: 10.1603/en13076
Morandin, L. A., Winston, M. L., Franklin, M. T., and Abbott, V. A. (2005). Lethal and sub-lethal effects of spinosad on bumble bees (Bombus impatiens Cresson). Pest Manag. Sci. 61, 619–626. doi: 10.1002/ps.1058
Morehead, J. A., and Kuhar, T. P. (2017). Efficacy of organically approved insecticides against brown marmorated stink bug, Halyomorpha halys and other stink bugs. J. Pest. Sci. 90, 1277–1285. doi: 10.1007/s10340-017-0879-3
Moreno, D. A., Víllora, G., Hernández, J., Castilla, N., and Monreal, L. R. (2002). Yield and chemical composition of Chinese cabbage in relation to thermal regime as influenced by row covers. J. Am. Soc. Hortic. Sci. 127, 343–348. doi: 10.21273/JASHS.127.3.343
Mueller, D. S., Gleason, M. L., Sisson, A. J., and Massman, J. M. (2006). Effect of row covers on suppression of bacterial wilt of muskmelon in Iowa. Plant Health Prog. 7:6. doi: 10.1094/PHP-2006-1020-02-RS
Nair, A., Lang, K., and Bilenky, M. (2019). Effect of row cover materials and cultivars in broccoli production systems. Iowa State University Research and Demonstration Farms Progress Reports 2018.
Nelson, H. M., González-Acuña, J. F., Nair, A., Cheng, N., Mphande, K., Badilla-Arias, S., et al. (2023). Comparison of row cover Systems for Pest Management in organic muskmelon in Iowa. HortTechnology 33, 103–110. doi: 10.21273/HORTTECH05096-22
Pair, S. D., Bruton, B. D., Mitchell, F., Fletcher, J., Wayadande, A., and Melcher, U. (2004). Overwintering squash bugs harbor and transmit the causal agent of cucurbit yellow vine disease. J. Econ. Entomol. 97, 74–78. doi: 10.1093/jee/97.1.74
Ratnadass, A., Fernandes, P., Avelino, J., and Habib, R. (2012). Plant species diversity for sustainable management of crop pests and diseases in agroecosystems: a review. Agron. Sustain. Dev. 32, 273–303. doi: 10.1007/s13593-011-0022-4
Rojas, E. S., Batzer, J. C., Beattie, G. A., Fleischer, S. J., Shapiro, L. R., Williams, M. A., et al. (2015). Bacterial wilt of cucurbits: resurrecting a classic pathosystem. Plant Dis. 99, 564–574. doi: 10.1094/PDIS-10-14-1068-FE
Rojas, E. S., Gleason, M., Batzer, J., and Duffy, M. (2011). Feasibility of delaying removal of row covers to suppress bacterial wilt of muskmelon (Cucumis melo). Plant Dis. 95, 729–734. doi: 10.1094/PDIS-11-10-0788
Schnathorst, W. (1965). Environmental relationships in the powdery mildews. Annu. Rev. Phytopathol. 3, 343–366. doi: 10.1146/annurev.py.03.090165.002015
Schroder, R. F., Martin, P. A., and Athanas, M. M. (2001). Effect of a phloxine B-cucurbitacin bait on diabroticite beetles (Coleoptera: Chrysomelidae). J. Econ. Entomol. 94, 892–897. doi: 10.1603/0022-0493-94.4.892
Schuh, M., and Grabowski, M. (2022). Bacterial wilt of cucurbits. Minnesota: University of Minnesota Extension.
Seebold, K. W., and Bessin, R. T. (2011). Yellow vine decline of cucurbits. Cooperative extension service. College of Agriculture, University of Kentucky. Plant Pathology Fact Sheet: PPFS-VG-12. Available at: http://plantpathology.ca.uky.edu/files/ppfs-vg-12.pdf (accessed March 14, 2023).
Sharma, A., Katoch, V., and Rana, C. (2016). “18 important diseases of cucurbitaceous crops and their management” in Handbook of cucurbits growth, cultural practices and physiology. 1st ed, Ed. Mohammad Pessarakli, CRC Press. 301–320.
Skidmore, A., Wilson, N., Williams, M., and Bessin, R. (2019). Integrating rowcovers and strip tillage for pest management in summer squash and muskmelon production. HortTechnology 29, 923–932. doi: 10.21273/horttech04221-18
Snyder, L., Schonbeck, M., and Vélez, T. (2022). 2022 National Organic Research Agenda: Outcomes and recommendations from the 2020 National Organic and transitioning farmer surveys and focus groups. Santa Cruz, CA: Organic Farming Research Foundation.
Theis, N., Barber, N. A., Gillespie, S. D., Hazzard, R. V., and Adler, L. S. (2014). Attracting mutualists and antagonists: plant trait variation explains the distribution of specialist floral herbivores and pollinators on crops and wild gourds. American Journal of Botany, 101, 1314–1322.
Tillman, J., Nair, A., Gleason, M., and Batzer, J. (2015). Evaluating strip tillage and rowcover use in organic and conventional muskmelon production. HortTechnology 25, 487–495. doi: 10.21273/horttech.25.4.487
USDA Agricultural Marketing Service (1983). United States standards for grades of fall and winter type squash and pumpkin [online]. Washington D.C: United States Department of Agriculture.
Keywords: cucurbits, row cover, cucumber beetles, powdery mildew, organic, profitability, mesotunnel
Citation: Fiske K, Cheng N, Kuesel R, Zhang W, Bessin R, Williams MA and Gonthier D (2024) Row covers limit pests and disease and increase profit in organic acorn squash. Front. Sustain. Food Syst. 8:1347924. doi: 10.3389/fsufs.2024.1347924
Edited by:
Matteo Dainese, University of Verona, ItalyReviewed by:
Kacie Athey, University of Illinois at Urbana-Champaign, United StatesTej Acharya, University of Georgia, United States
Copyright © 2024 Fiske, Cheng, Kuesel, Zhang, Bessin, Williams and Gonthier. This is an open-access article distributed under the terms of the Creative Commons Attribution License (CC BY). The use, distribution or reproduction in other forums is permitted, provided the original author(s) and the copyright owner(s) are credited and that the original publication in this journal is cited, in accordance with accepted academic practice. No use, distribution or reproduction is permitted which does not comply with these terms.
*Correspondence: Kathleen Fiske, krfi223@uky.edu