- 1Department of Marine Biotechnology, Norwegian Institute of Food, Fisheries and Aquaculture Research (Nofima), Tromsø, Norway
- 2Department of Processing Technology, Norwegian Institute of Food, Fisheries and Aquaculture Research (Nofima), Stavanger, Norway
Farmed lumpfish (Cyclopterus lumpus) are used as cleaner fish in Norwegian aquaculture. However, once the fish reaches sexual maturity, it becomes less effective in combating lice and is often wasted or turned into silage. This raises ethical, economic, and sustainability concerns regarding whether the use is sustainable without increasing its standard of living and further use of the fish to higher value products. To improve the utilization of lumpfish, a study was conducted to investigate the differences in extraction efficiency by comparing product yield, protein content, and peptide size distribution after enzymatic hydrolysis of lumpfish using several commercial proteases (Corolase 8,000, Corolase 7,089, Endocut 01 L, Flavourzyme, and Food Pro PNL), and different pretreatment methods (pulsed electric field and high-pressure processing). The choice of enzyme did not affect the amino acid contents of the different hydrolysates. Furthermore, varying enzyme concentrations had a minor impact on the final product’s protein content. As anticipated, increased enzyme concentrations decreased the hydrolysates’ average molecular weight. The study found that biomass treated either chemically (NaOH and butanol) or mechanically with a pulsed electric field before enzymatic hydrolysis resulted in higher protein content and relatively higher amounts of collagen peptides. Initial bioactivity testing indicated that the hydrolysates had no toxic effects on hepatocellular carcinoma and non-malignant lung fibroblast cells. Previous studies have shown that farmed lumpfish contains all the essential amino acids and has high levels of EPA, DHA, B12, and D3 vitamins. The contents of environmental pollutants and heavy metals were also below the EU maximum levels. This study’s knowledge and results open for the potential use of lumpfish peptides and gelatin for, e.g., dietary supplements, feed, and biodegradable packaging.
1 Introduction
Lumpfish (Cyclopterus lumpus) is a marine finned fish found in the North Atlantic and adjacent oceans (Jansson et al., 2023). In the wild, the fish are mainly harvested for their roe, which is used as lumpfish caviar (Powell et al., 2018). In Norway, farmed lumpfish are used as a treatment for sea lice (Brooker et al., 2018) [Lepeophtheirus salmonis (Krøyer, 1837)] infestations on Atlantic salmon in the aquaculture industry (Imsland et al., 2014, 2018). Sea lice are marine parasites that attach to the fish’s body, feeding off their skin, mucus, and blood, making them more susceptible to other infections (e.g., bacteria, viruses, and fungi).1 Juvenile lumpfish is transferred to the salmon net cage at approximately six months old (initial weight ~ 25 g) (Ageeva et al., 2021). When they increase in size and reach sexual maturity, there is a decrease in efficacy as lice eaters. This makes the reuse of lumpfish as cleaner fish not feasible (Brooker et al., 2018). In 2022, approximately 17.6 M lumpfish were distributed for use as salmon lice control.2 After slaughtering the salmon stock in the net cage, the lumpfish is removed and destroyed (waste) or used for silage. The silage suitable for animal feed production can be sold for around 0.2 € per kilo. However, some salmon producers must pay to dispose of the lumpfish (Nøstvold et al., 2016).
The use of cleaner fish has raised ethical, economic, and sustainability concerns (Garcia de Leaniz et al., 2022) regarding whether the use of lumpfish is sustainable without increasing its standard of living and increasing the utilization of biomass to produce higher-value products. Usually, all applicable resources should follow the “food first”-principle and subsequently be used for the highest possible value (Vang et al., 2021). Recently, there has been a focus on new utilization areas for farmed cleaner fish. Zhuang et al. (2018) and Thong et al. (2023) tested the viability of lumpfish as food by exploring possible products that could be made in Vietnamese cuisine and understanding the stakeholders’ perception and acceptance of the fish. Studies have shown (Ageeva et al., 2021) that farmed lumpfish contains all the essential amino acids and has high levels of EPA, DHA, B12, and D3 vitamins. Contents of environmental pollutants and heavy metals were also below the EU maximum levels, indicating that the lumpfish can be further exploited for human consumption. Some work has been published on collagen extraction from lumpfish (Zhuang et al., 2018; Vate et al., 2023), but there are few (if any) publications regarding protein hydrolysis of whole lumpfish.
Many studies have been conducted on producing and using collagen, gelatin, and enzymatically produced hydrolysates from other aquatic side streams. These proteins have various applications in biomedical engineering (Milan et al., 2021), food supplements (Lin et al., 2020), cosmetics (Amnuaikit et al., 2022), and food packaging materials.3 Previous studies have shown that the skin and head of lumpfish can account for up to 54% of the biomass (Ageeva et al., 2021), and these parts are affluent in connective tissues, including collagen (Dave et al., 2019), possibly making it attractive for a protein source for further use in applications mentioned above. Leftover marine biomass has previously proven to be a potential source for generating natural bioactive peptides and proteins, focusing on bioactivities such as antimicrobial (Naghdi et al., 2023), anti-inflammatory (Giannetto et al., 2020), and ACE inhibition activities (Abbas et al., 2022).
This study aimed to explore differences in product yield, protein content, and peptide size distribution resulting from various commercial proteases and pretreatment techniques and find an efficient extraction method. The study is divided into three experimental setups: (1) extraction of lumpfish skin gelatin (LSG) and whole lumpfish gelatin (WLG), (2) testing commercial enzymes and concentrations for protein hydrolysis, and (3) testing chemical and mechanical pretreatment techniques in combination with enzymatic hydrolysis. Pretreatment of raw materials before enzymatic hydrolysis can significantly influence a hydrolysis process by providing more accessible sites for enzymes by increasing the surface area and by partially or fully unfolding the proteins (Asaithambi et al., 2022). The different pretreatments can also affect textural and sensory properties (Asaithambi et al., 2022). The hydrolysate’s proximate composition and peptide size distribution were analyzed to find potential differences. Initial bioactivity assays were performed to assess any potential biological activities of the hydrolysates.
2 Materials and methods
2.1 Materials and reagents
The lumpfish used in this study were sampled from salmon net cages in March 2020 from Karanes (Karlsøy, Troms, and Finnmark county, Norway) as described in Ageeva et al. (2021). Whole lumpfish and lumpfish skin was cut into 2 × 2 cm pieces, packed in vacuum bags (Scancell, Kuppenheim, Baden-Wurttemberg, Germany), and frozen at −40°C until further processing.
The proteases used for enzymatic hydrolysis were Corolase 8,000 (batch number R205464ST, 100000 BPU/g, AB Enzymes, Darmstadt, Germany), Corolase 7,089 (batch number F181360ST, 840 Uhb/g, AB Enzymes, Darmstadt, Germany), Food Pro PNL (batch number 4863924615, 1,600 U/g, DANISCO, Copenhagen, Denmark), Flavourzyme (batch number HPN00549, 1000 LAPU/g, Novozymes, Bagsværd, Denmark) and Endocut 01 L (batch number 1043, 180 NU/g, Tailorzyme, Herlev, Denmark). All the enzymes used comply with the recommended purity specifications for food-grade enzymes issued by the joint FAO/WHO Expert Committee on Food Additives and the Food Chemicals Codex.
Peptide standards for molecular weight (MW) distribution were purchased from Sigma-Aldrich: Carbonic Anhydrase from bovine erythrocytes (C7025, 29 kDa), Lysozyme from chicken egg white (L7651, 14.3 kDa), Cytochrome c from the bovine heart (C2037, 12.3 kDa), Aprotinin from bovine lung (A1153, 6.51 kDa), Insulin Chain B oxidized from bovine pancreas (I6383, 3.5 kDa), Renin Substrate Tetradecapeptide porcine (R8129, 1.76 kDa), Angiotensin II human (A9525, 1.05 kDa), Bradykinin Fragment 1–7 (B1651, 0.757 kDa), [D-Ala2]-Leucine enkephalin (E5008, 0.569 kDa), Val-Tyr-Val (V8376, 0.379 kDa), and L-Tryptophane (T0254, 0.204 kDa). All cell lines and microbes used for bioactivity screening were purchased at LGC standards: HepG2 (ATCC HB-8065™), MRC5 (ATCC CCL-171™), Enterococcus faecalis (ATCC 29212), Escherichia coli (ATCC 25922), Pseudomonas aeruginosa (ATCC 27853), Staphylococcus aureus (ATCC 25923), Streptococcus agalactiae (ATCC 12386), Candida albicans (ATCC 90028) and Staphylococcus epidermidis (ATCC 35984).
All chemicals used in this study have been purchased from Sigma Aldrich and VWR and solutions for electrophoresis through Thermo Scientific.
2.2 Methods
2.2.1 Gelatin extraction and verification
Gelatin extraction was performed as described by Arnesen and Gildberg (2007), with slight modifications. The extraction was performed once for lumpfish skin (LS) and whole lumpfish (WL). LS and WL were processed as described in section 2.1 and performed in a closed 1 Liter high viscosity glass reactor (IKA, Staufen, Germany) with impeller and temperature control. The raw material to solvent ratio was 1:3.3 with the various solutions. The raw material was incubated twice in 0.05 M NaOH solution for 30 min before being drained in a sieve. After washing with cold water to remove excess NaOH, the biomass was neutralized with 0.067 M sulfuric acid before treatment with 5 mM citric acid. Each acid step was performed for 30 min. The biomass was further washed with cold water before gelatin was extracted with water for 2 h at 55°C. Before lyophilization, the LSG and WLG extract were filtrated with grade 4 filter paper (Whatman, Cytiva, Medemblik, Holland). The following formula calculated the mass yield for gelatin extraction:
Protein recovery was measured by determining the amino acid content in the biomass and the dried WLG. Recovery is presented as percent hydroxyproline (Hyp) extracted compared to the Hyp content in the original biomass using the following formula:
To validate if gelatin had been extracted, SDS-PAGE was performed using Xcell SureLock Mini-Cell (Thermo Fisher Scientific, Waltham, United States) on NuPage 4–12% BisTris, 1.0 mm, mini protein gel in NuPage MOPS SDS running buffer. LSG and WLG were dissolved in water, and NuPage LDS Sample Buffer (4X) containing 5% 2-mercaptoethanol was boiled for 5 min. Samples (20 μg/well) were applied to sample wells and electrophoresed for 50 min at 200 V and 150 mA using SeeBlue Plus 2 pre-stained as a marker. The separated proteins were stained using NuPage Simply Blue Safe stain.
2.2.2 Enzymatic hydrolysis
Previous unpublished studies showed that the protein content (measured by the Kjeldahl method) and total amino acid content (Supplementary Tables S1, S2) after enzymatic hydrolysis on whole lumpfish vs. lumpfish skin was similar (ranging between 74 and 77%). Since filleting lumpfish is a time-consuming manual process (Figure 1) and therefore expensive, it was decided that the experimental work would be performed on whole lumpfish gutted with head. Firstly, the lumpfish was hydrolyzed using a selection of commercial proteases at different concentrations (Figure 2). Secondly, enzymatic hydrolysis was combined with mechanical and chemical pretreatment of the biomass (Figure 3), as described in section 2.2.3.
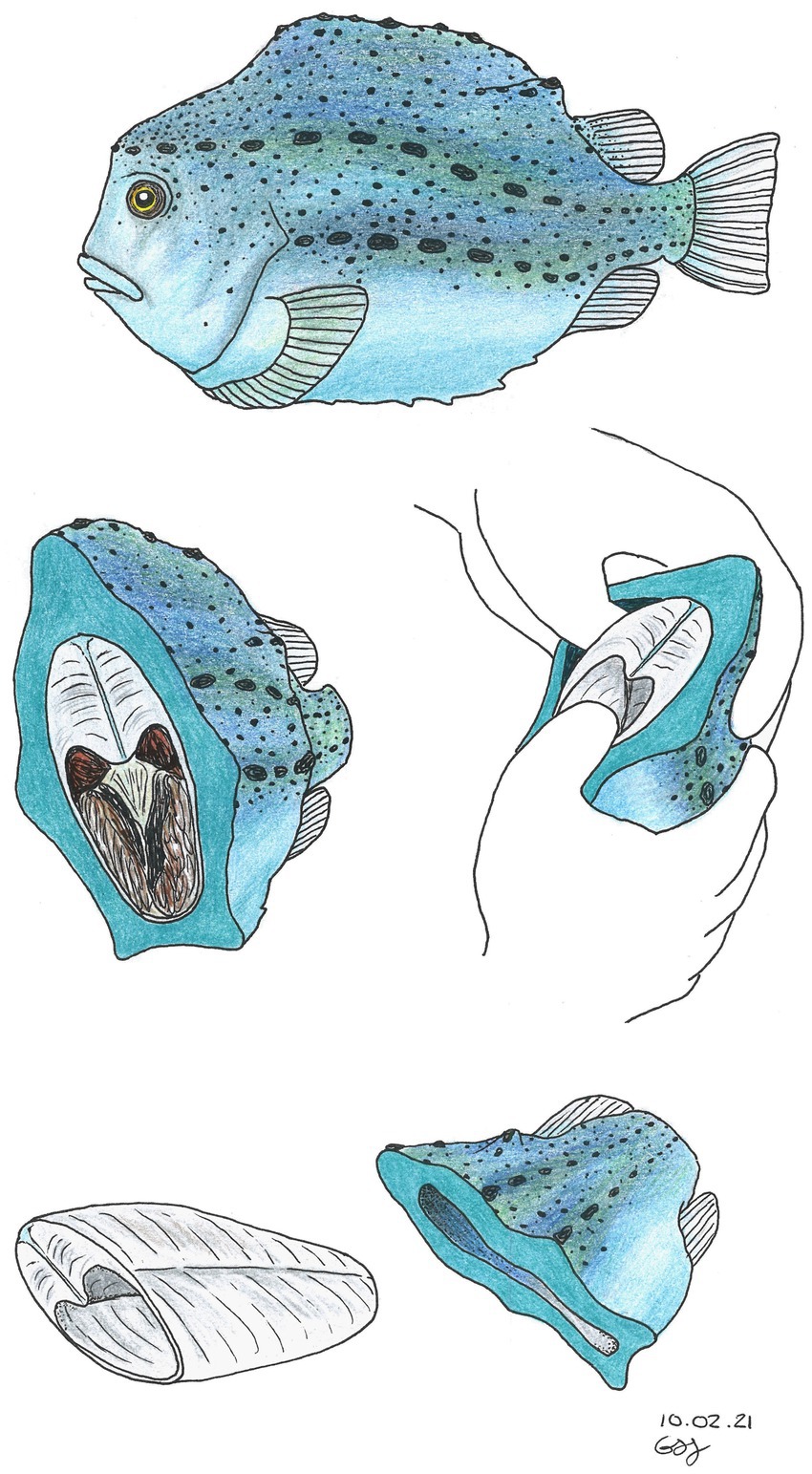
Figure 1. Illustration of manual lumpfish filleting. Illustration by Gunhild Seljehaug Johanson, Nofima, Tromsø, Norway.
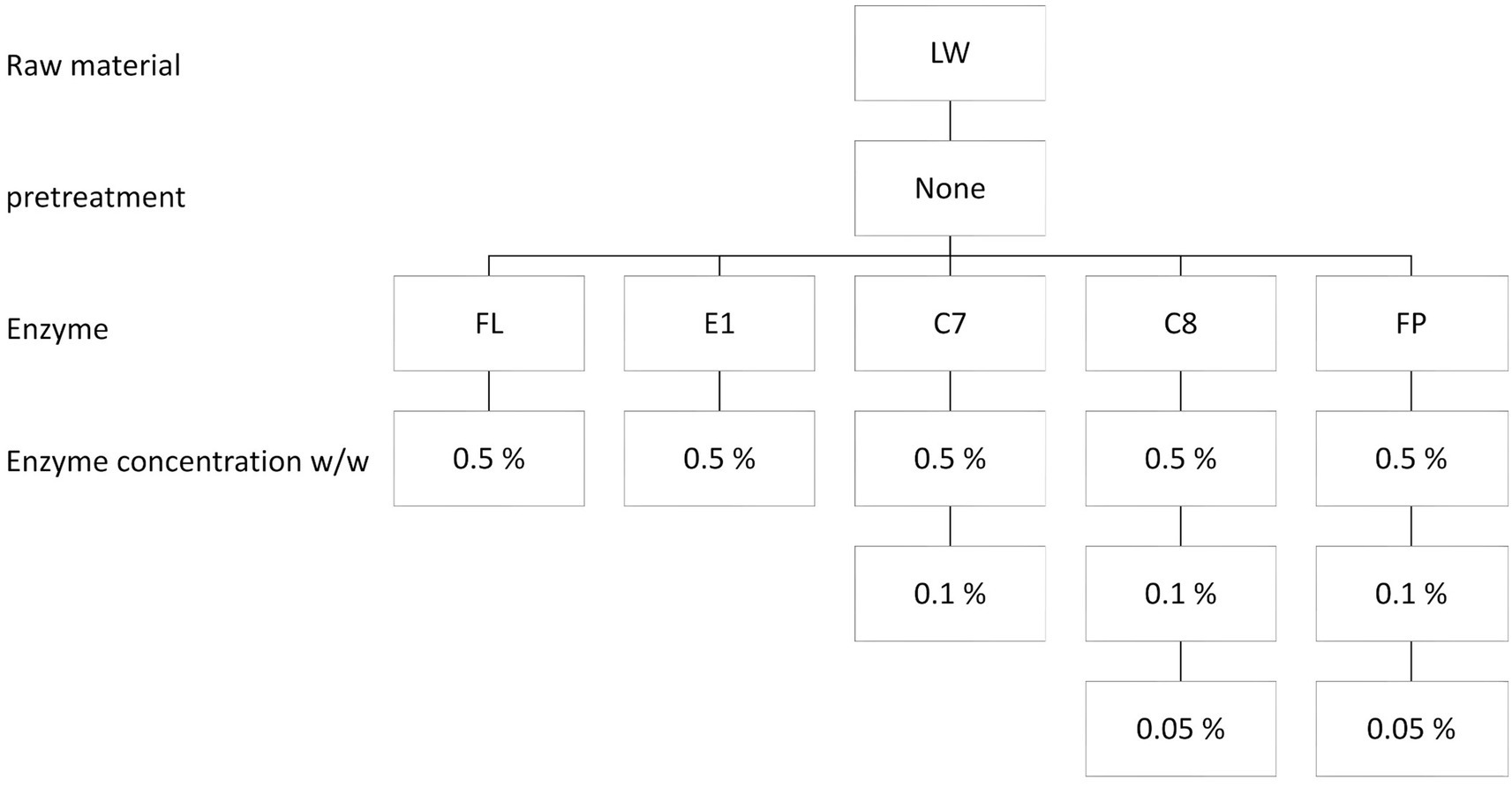
Figure 2. Experimental setup: Enzymatic hydrolysis using different enzymes and concentrations. Enzymatic hydrolysis of whole lumpfish (LW) using 5 proteases (Flavourzyme (FL), Endocut 01 L (E1), Corolase 7,089 (C7), Corolase 8000 (C8), and FoodProPNL (FP)) with enzyme concentration at 0.5% (w/w) was performed and evaluated. Subsequently, three enzymes were chosen based on protein yield from the first round (C7, C8, and FP), decreasing the enzyme concentration to 0.1% (w/w). Finally, two proteases were tested with an enzyme concentration of 0.05% (w/w) (C8 and FP).
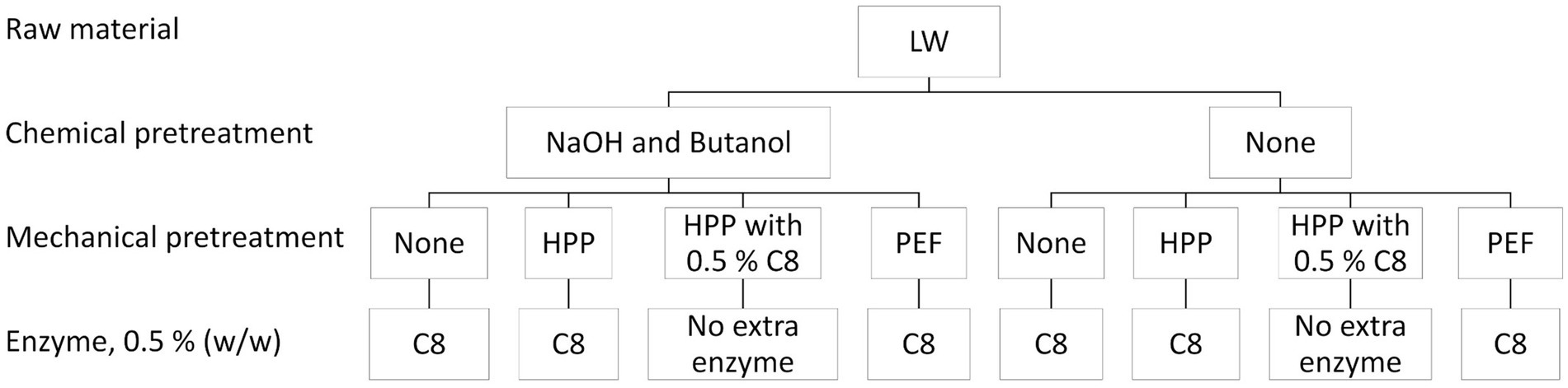
Figure 3. Experimental setup: Chemical and mechanical pretreatments and combinations of pretreatments, followed by enzymatic hydrolysis. Enzymatic hydrolysis of whole lumpfish (LW) chemical and mechanical pretreated using Corolase 8000 (C8) in all trials. Mechanical pretreatments are high-pressure processing (HPP) and pulsed electric field (PEF), and chemical pretreatments include lye (NaOH) and butanol steps.
For enzymatic hydrolysis, the biomass was thawed at 4°C for 24 h before being mixed with tap water at a 1:1 ratio (w/v). All hydrolyses were carried out once for each enzyme concentration in closed 1 Liter high viscosity glass reactors (IKA, Staufen, Germany) with impeller and temperature control. The mixture was heated to the optimum temperature for each protease used: Flavourzyme (FL): 50°C, Endocut 01 L (E1): 50°C, Corolase 7,089 (C7): 55°C, Corolase 8,000 (C8): 65°C and FoodProPNL (FP): 55°C. The reaction was initiated by adding the enzyme of choice to the desired concentration (Figures 2, 3). The hydrolyses were conducted for 60 min at 40 rpm before inactivation at 90°C for 15 min. The samples were coarsely filtered through a sieve before centrifugation at 8,000 rpm for 20 min at 18°C in an Avanti JXN-26 B38623 high-speed centrifuge (Beckman, Indianapolis, United States). The supernatant containing water-soluble peptides was further filtered through depth filter sheets (Seitz T2600, PALL) to remove excess fat and any remaining particles. The solution was frozen at −80°C before lyophilization in a FreeZone Plus 12 Liter Cascade Console Freeze Dry System (LABCONCO, Kansas City, United States). The following formula calculated the mass yield for each hydrolysis:
2.2.3 Pretreatment procedures
The biomass of lumpfish was subjected to various mechanical and chemical pretreatments and combinations of these treatments before enzymatic hydrolysis (as illustrated in Figure 3). These pretreatments were conducted to investigate if they could affect the product yield, protein content, and peptide size distribution following enzymatic hydrolysis of the biomass. The following enzymatic hydrolysis was performed as described in section 2.2.2. One batch of lumpfish (4 kg) underwent a chemical pretreatment using 0.1 M NaOH in 1:10-ratio (w/v) for three days to remove non-collagenous proteins to investigate if it was possible to obtain a product with higher collagen peptide content. The lumpfish was further washed with water until a neutral pH was achieved. The fish was mixed with 10% butyl alcohol in a ratio of 1:10 (w/v) for 24 h to remove lipids and thoroughly washed with water to remove excess butanol. The biomass was packed and stored at −20°C until further processing.
The pilot dual equipment (Elea Technology GmbH, Quakenbrück, Germany) was used for the lumpfish’s mechanical pretreatment pulsed electric field (PEF). The treatment was done in a 10 L batch chamber with an electrode distance of 24 cm. Tap water (20°C) was used in the process. The fish: water ratio was 1:3 (approximately 500 g fish to 1,500 g water). The water was changed between each run. The following conditions were applied: electrode voltage 24 kV; frequency 30 Hz; pulse count 800; pulse width 6 μs. The measured energy supplied to lumpfish and water was 4.5 ± 0.1 KJ/kg. After the treatment, the batch was placed in a sieve and dripped for 2 min. Then, it was placed in a clean, food-grade plastic bag, vacuum packed, and frozen at 18°C until further handling. A sample of the drip water was collected and lyophilized before the protein content was analyzed using the Kjeldahl method.
High-pressure processing (HPP) was used as a second mechanical pretreatment of the lumpfish. The fish was vacuum-packed (95% vacuum) in food-grade plastic bags. The HPP was performed at an ambient temperature of 200 MPa for 15 min in a high hydrostatic pressure machine QFP 2 L-700 (Avure Technologies Inc., Columbus, OH, United States). The come-up time was approximately 55 s, whereas the pressure release was immediate. The duration of treatment did not include the come-up time. The samples were frozen and stored at −18°C until further processing.
2.2.4 Chemical characterization
Moisture and ash contents were determined following the standard methods of the Nordic-Baltic Committee on Food Analysis (NMKL) 23 (NMKL, 2022) and 173 (NMKL, 2005), respectively. The Kjeldahl method (NMKL 6) (NMKL, 2003) was applied for protein content analysis, and crude protein was estimated based on N × 6.25. Total amino acid content was determined as described by Szkudzińska et al. (2017), with minor modifications. The run time was set to 32 min with a flow of 0.4 mL/min, giving 18 amino acids (including cysteine and taurine).
The peptide size distribution was determined by size exclusion chromatography (SEC), as previously described (Wubshet et al., 2017) with minor differences: The stationary phase was a BioSep-SEC-s2000 column (300 × 7.8 mm) (Phenomenex, Værløse, Denmark) on a Shimadzu HPLC system (Shimadzu, Nishinokyo Kuwabara-cho, Nakagyo-ku, Japan). The injection volume was 10 μL. Each injection was performed in two parallels and separated at 30°C. The mobile phase consisted of 30:70:0.05 acetonitrile: water: trifluoracetic acid. Isocratic elution was carried out at a 0.90 mL/min flow rate. After 17 min, the mobile phase was changed to 0.10 M NaH2PO4 and maintained for 3 min for column washing. Elution conditions were restored at 20 min, and the column was re-equilibrated for an additional 25 min. The column was calibrated using standards of known MW ranging from 0.2 to 29 kDa (details in 2.1 Materials and reagents). The parallels were compared in the interval 5–17 min (the window of compound elution according to standards) by calculating Pearson correlation based on retention time and intensity as measured by the PDA at 214 nm with 640 ms intervals.
2.2.5 Bioactivity studies
The five fish protein hydrolysates produced with 0.5% (w/w) enzyme (FP, C7, C8, E1, and FL) were evaluated for bioactivity in various assays. All bioactivity testing was performed by the analytical platform Marbio (UiT – the Arctic University of Norway, Tromsø, Norway). The hydrolysates were dissolved in ddH2O to 10 mg/mL and screened for bioactivities at 100, 50, and 25 μg/mL. Potential growth-promoting or toxic/antiproliferative activities of the hydrolysates toward human cells were assayed using two cell lines, the hepatocellular carcinoma cell line HepG2 and the non-malignant lung fibroblast cell line MRC5, in an MTS in vitro cell proliferation assay as described previously (Hansen et al., 2019).
The hydrolysates were assayed for antimicrobial activities against five bacterial isolates and one fungal strain (E. faecalis, E. coli, P. aeruginosa, S. aureus, S. agalactiae, and C. albicans) in a minimal inhibitory concentration assay, as previously described (Jenssen et al., 2021). In addition, inhibition of biofilm formation against a biofilm-forming S. epidermidis isolate was assayed, as previously described (Jenssen et al., 2021). To evaluate the potential antioxidative activities of the hydrolysates, a ferric-reducing ability of plasma (FRAP) assay was performed according to the method of Benzie and Strain using Trolox as a reference Benzie and Strain (1996).
3 Results and discussion
3.1 Proximate composition of raw material
The proximate composition of the lumpfish used in this study has been published previously (Ageeva et al., 2021). The results showed that the lumpfish had high moisture levels (91.5% w/w) and low levels of protein (5.7%), fat (1.3%), and ash (1.6%). For comparison, a previous study by Dave et al. (2019) and (Lin et al. (2020) observed an almost identical proximate composition with 91.08% moisture, 5.02% protein, 1.59% fat, and 1.72% ash. The total amino acid content of whole lumpfish used in this study was 46.67 g/100 g (analyzed on dry weight), and it contained all essential amino acids (Supplementary Table 3).
3.2 Verification, yield, and amino acid composition of gelatin
Lumpfish skin and whole lumpfish were used as the starting point for gelatin extraction. The gelatin samples contained significant components in the form of α-chains and β-chains, as shown in Figure 4. The molecular weights of the α1- and α2-chains were larger than 100 kDa. The β-chain had a molecular weight > 200 kDa. This result suggests that the extraction conditions did not cause any degradation to the α-chains and β-chains. The mass yield of LSG and WLG were 1.61 and 0.63%, respectively. Vate et al. (2023) have previously shown a mass yield of 1.24% of native collagen isolated from lumpfish (gutted with head). The total amino acid content of LSG and WLG indicated higher protein content in the LSG than in the WLG sample (Table 1). LSG also has a higher amount of glycine (Gly), Hyp and proline (Pro) compared to WLG, which is expected since connective tissue (like skin) contains a more significant amount of collagen compared to filets (Liaset and Espe, 2008). Based on the amino acid composition of lumpfish biomass, the recovery of Hyp was 0.17 and 1.71% in extracted WLG and LSG, respectively. Based on the low mass yield and low recovery, it is believed that the process used for gelatin extraction is not satisfactory from an economic point of view. The continued work focused on complete protein extraction from whole lumpfish, using different pretreatments (both chemical and mechanical) and enzymatic hydrolysis.
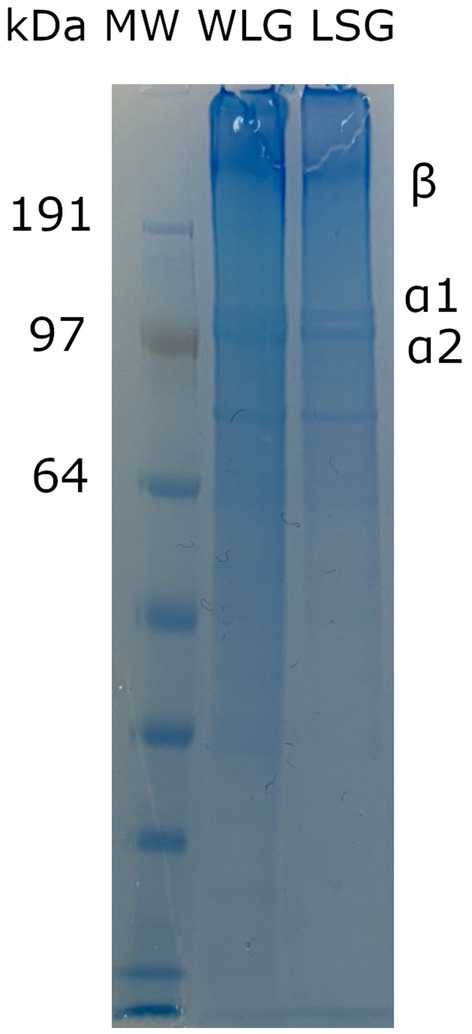
Figure 4. SDS-polyacrylamide gel electrophoresis (SDS-PAGE) pattern of gelatin from whole lumpfish (WLG) and lumpfish skin (LSG). MW: molecular weight marker. Numbers represent the molecular weight of different protein bands when run in a MOPS buffer.
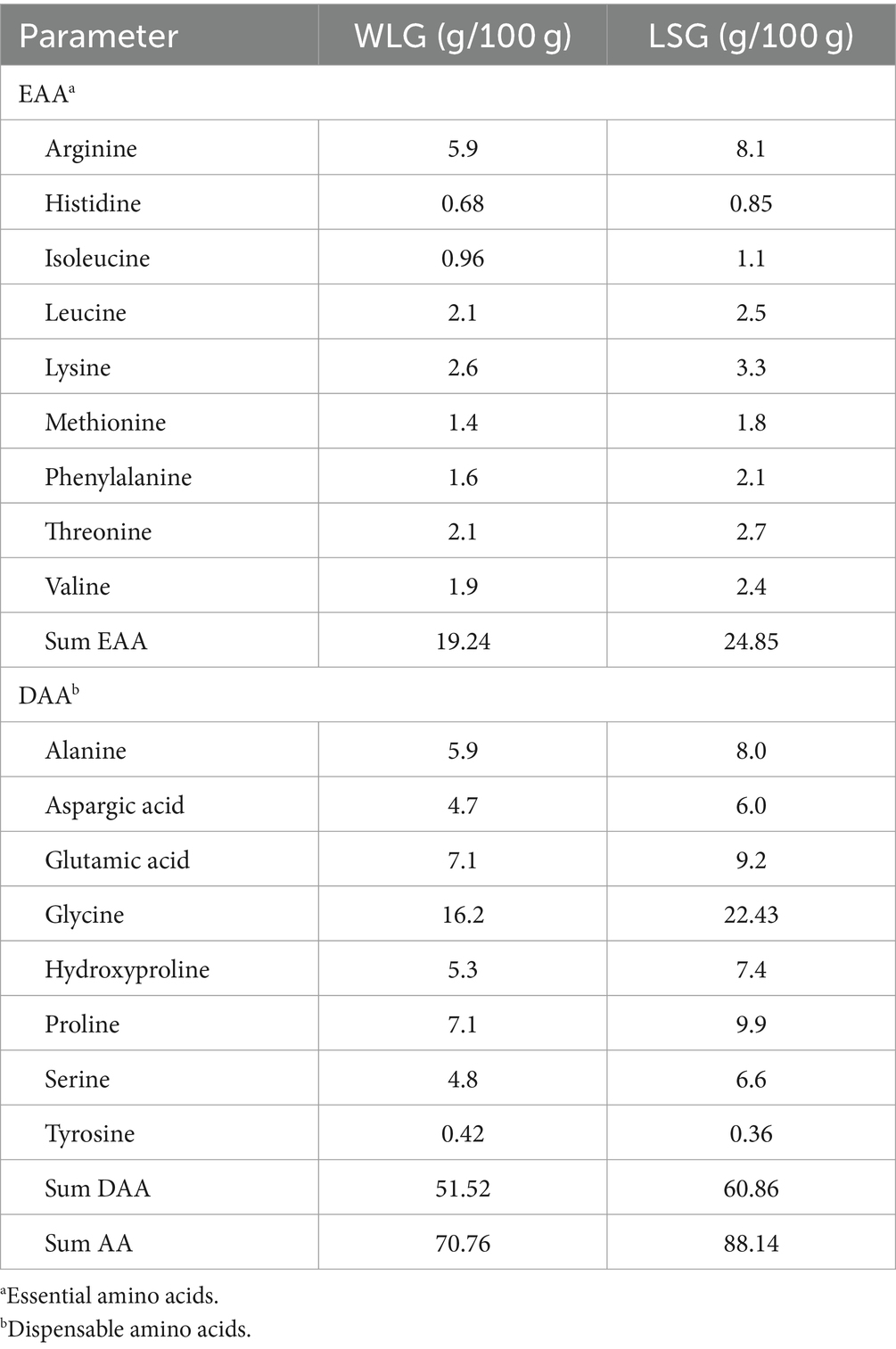
Table 1. TOTAL amino acid composition (g/100 g) of gelatin extracted from whole lumpfish, not gutted (WLG), and lumpfish skin (LSG).
3.3 Chemical characterization of hydrolysates produced without pretreatment
The hydrolysis trials produced two fractions: fish protein hydrolysate and sediment. There were no visible lipid layers or emulsions in the hydrolysates, indicating that the amount of lipids not removed during the filtration is incorporated either in the fish protein hydrolysates or in the sediment (not analyzed).
Using the different commercial enzymes at a concentration of 0.5%, the mass yield ranged between 5.35 and 6.73% (Table 2). Reducing enzyme concentration led to a correlating decrease in mass yield. In a commercial setting, a reduction in yield and product loss must be evaluated against the costs saved on the reduced use of enzymes.
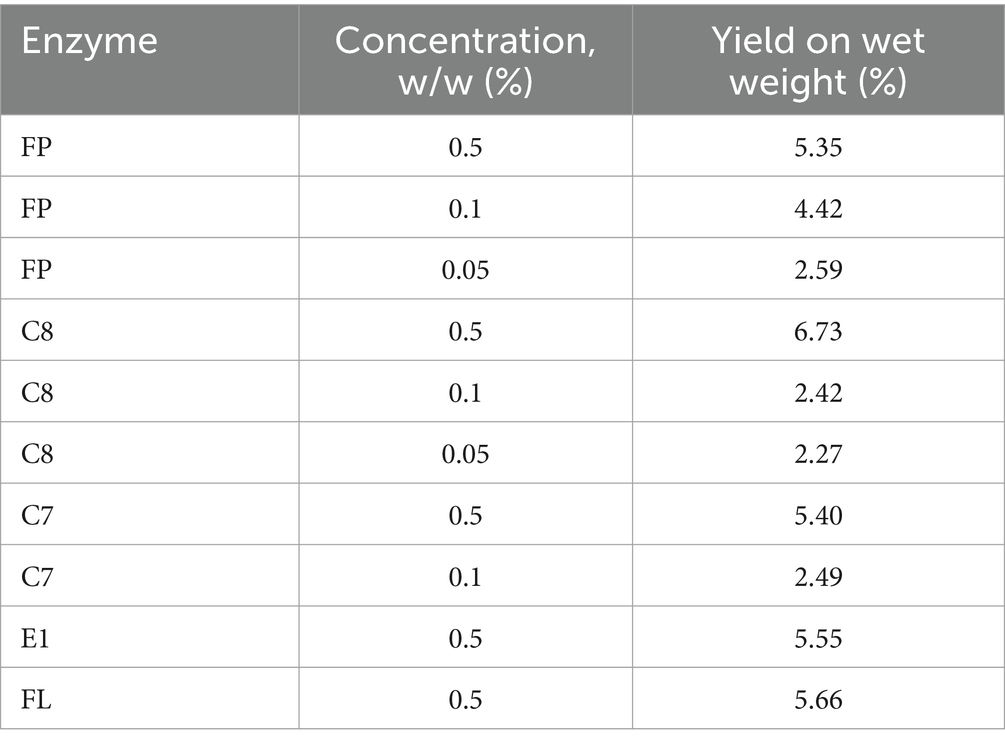
Table 2. Overview of hydrolysate yield influenced by protease and protease concentration using FoodProPNL (FP), Corolase 8,000 (C8), Corolase 7,089 (C7), Endocut 01 L (E1), and Flavourzyme (FL), n = 1.
The lyophilized fish protein hydrolysates had between 4.9–7.4% moisture and 72–81% Kjeldahl protein (Table 3). There were no significant differences in Kjeldahl protein content when comparing the proteases used at 0.5% (w/w). Surprisingly, a reduction in enzyme concentration from 0.5 to 0.1% gave higher Kjeldahl protein for all enzymes tested (FP, C8, and C7). Decreasing the enzyme concentration to 0.05% showed a reduction in Kjeldahl protein content compared to 0.1% enzyme. However, the 10-fold decrease in the enzyme concentration did not significantly impact the Kjeldahl protein measurements. The sum of total amino acids is the most accurate estimate for protein content in a product (Mæhre et al., 2018), and the levels of total amino acids (Table 3) were lower than Kjeldahl protein in the samples. This is expected when using the protein factor 6.25 in Kjeldahl analysis, which was previously shown to be inaccurate for fish fractions. It has been found that using a protein factor of 5.6 is more accurate (Aspevik et al., 2021). When factor 5.6 is applied to the current results (data not shown), the levels are more similar than the sum of total amino acids.
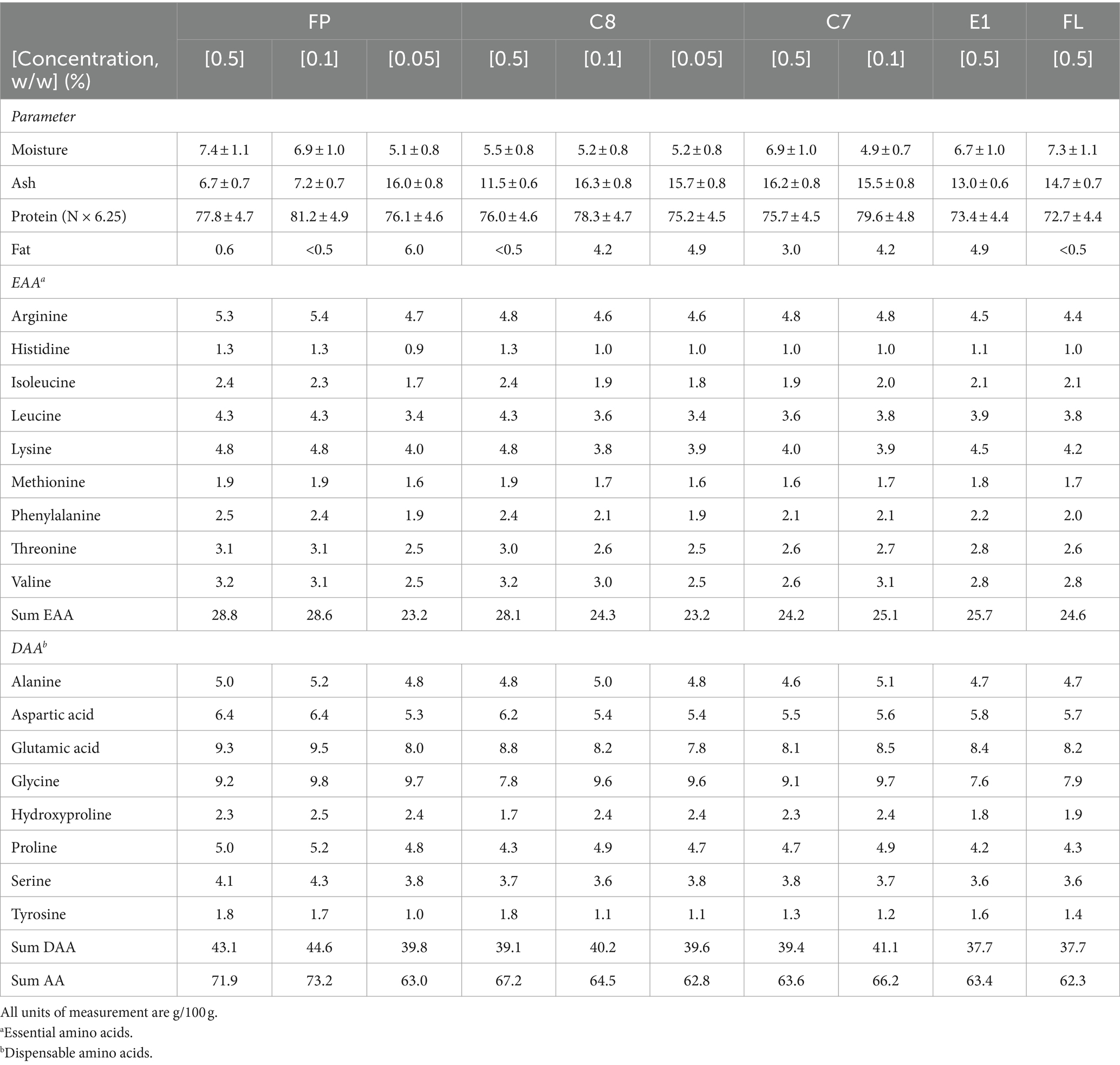
Table 3. Proximate and total amino acid composition of lumpfish hydrolysates using Food Pro PNL (FP), Corolase 8,000 (C8), Corolase 7,089 (C7), Endocut 01 L (E1), and Flavourzyme (FL).
However, the actual total amino acid content is expected to be higher than displayed in Table 3 since some amino acids, such as asparagine and glutamine, are hydrolyzed to their aspartic and glutamic acid forms, respectively. In addition, other amino acids cannot be determined 100% correctly using this method. For example, tryptophan is completely degraded during the reaction, while sulfur-containing amino acids (e.g., cysteine, methionine) cannot be determined with certainty due to partial degradation of the amino acids. Furthermore, amino acids such as tyrosine, serine, and threonine may have lower recovery due to the nature of acid hydrolysis. The hydrolysates produced in this study contained all the essential amino acids. The choice of enzyme did not notably influence the amino acid contents in the different hydrolysates.
SEC is a method to elucidate the relative size of compounds in a complex sample, e.g., a proteinaceous sample after hydrolysis. The analysis can be used to find the hydrolysis process with the most desired peptide size distribution. Most bioactive peptides have molecular mass in the range of 400–2,000 Da (Zaky et al., 2022), and intestinal digestibility is affected by the size of the peptides (Korhonen and Pihlanto, 2006). In this study, SEC was used to evaluate the effect of enzyme choice and concentration on the peptide size distribution of the respective hydrolysates. All hydrolysates produced at different enzyme concentrations (without pretreatment) were analyzed, and the total average MW was calculated (Table 4). At 0.5% enzyme concentration, the average MW of the peptides in the hydrolysates ranged from 961 Da (FP) to 2,763 Da (FL). At 0.1% enzyme concentration, the same trend can be seen. However, at 0.05% enzyme concentration, C8 seems more efficient in peptide degradation than FP, with a total average MW of 2,318 and 2,670, respectively. The effect of enzyme concentration can be measured as the change in total average MW for peptides produced by the same enzyme. A reduction in total average MW can be observed for all tested enzymes with increased enzyme concentration. This can be expected as higher concentrations of enzymes typically increase protein hydrolysis. The same can be observed in the relative size distribution of peptides in each hydrolysate, shown in Table 4, where the peptides have been divided into categories based on size. Enzyme kinetics can be affected by concentrations (Juárez-Enríquez et al., 2022), possibly explaining this phenomenon.
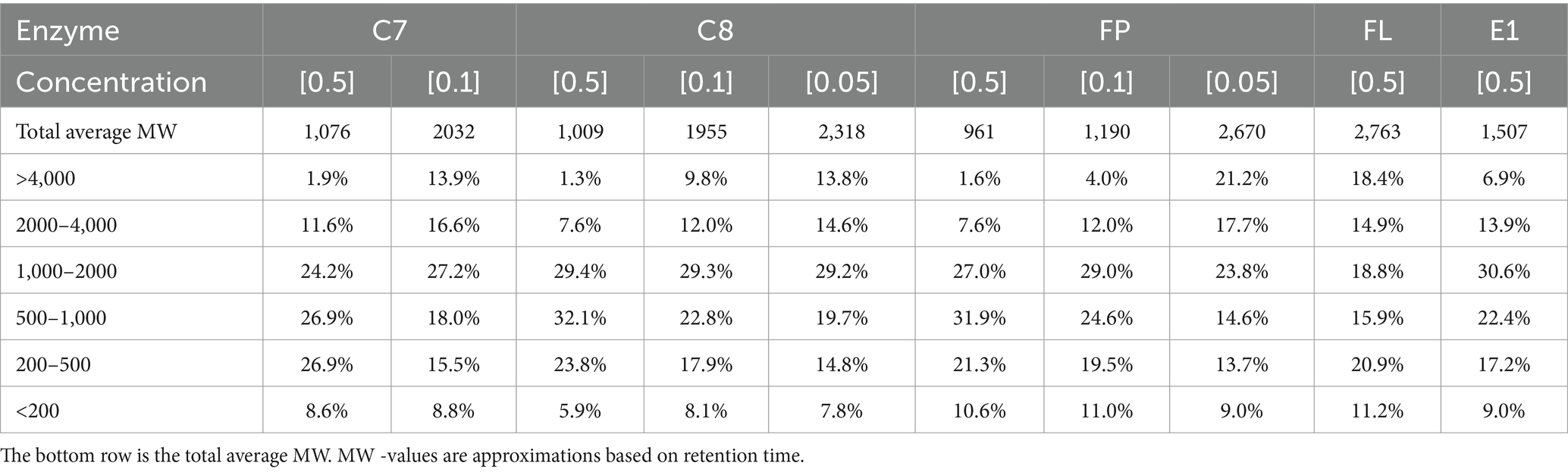
Table 4. Molecular weight (MW) distribution is categorized into six categories for all hydrolysates and concentrations.
3.4 Chemical characterization of hydrolysates produced using pretreatment and combinations of pretreatments
Samples treated chemically and using PEF pretreatment on non-chemically pretreated samples (Tables 5, 6) resulted in a higher overall protein content with relatively higher amounts of the amino acids Gly, Pro, and Hyp, which are characteristic of collagen (Vate et al., 2023). This indicates that these hydrolysates contain higher amounts of collagen peptides. The mass yield, however, is lower in these samples compared to non-chemically pretreated samples, which is expected as the chemicals used to remove compounds such as non-collagen proteins and fat. During PEF pretreatment, the water changes continuously, and the mass yield results indicate that some compounds are removed during this treatment. A test sample from the PEF-processing drip water showed high amounts of protein on a dry weight basis. Drip loss during preprocessing steps can give lower yields but might result in a product with higher collagen purity.
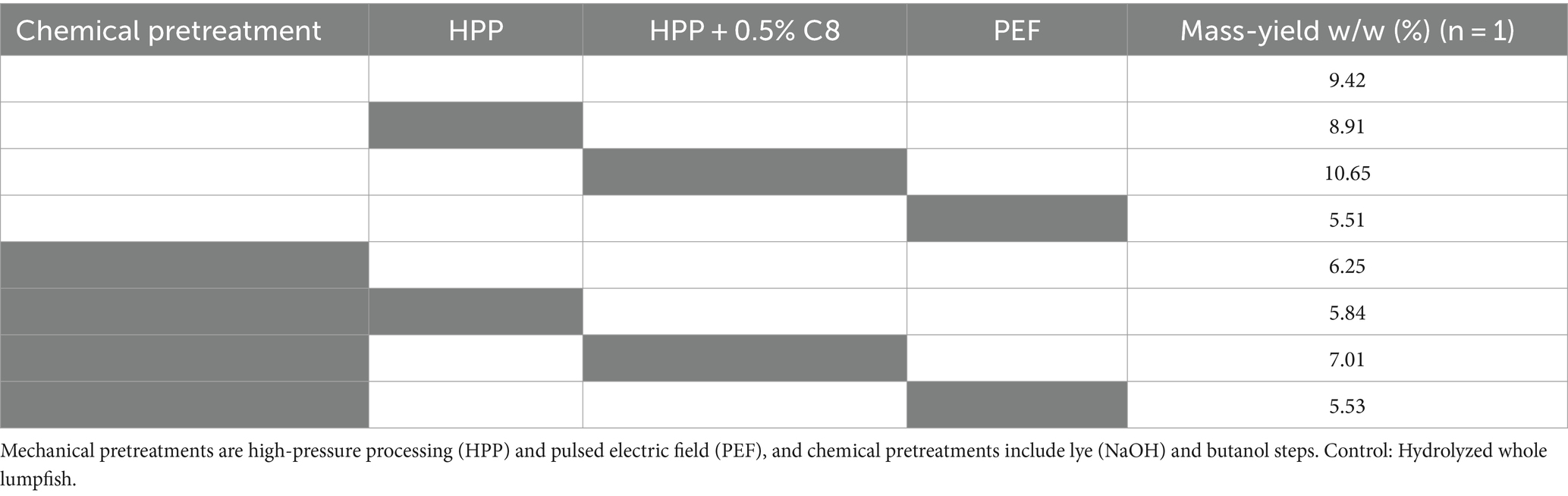
Table 5. Overview of hydrolysate yield influenced by mechanical and chemical pretreatment using Corolase 8,000 (C8).
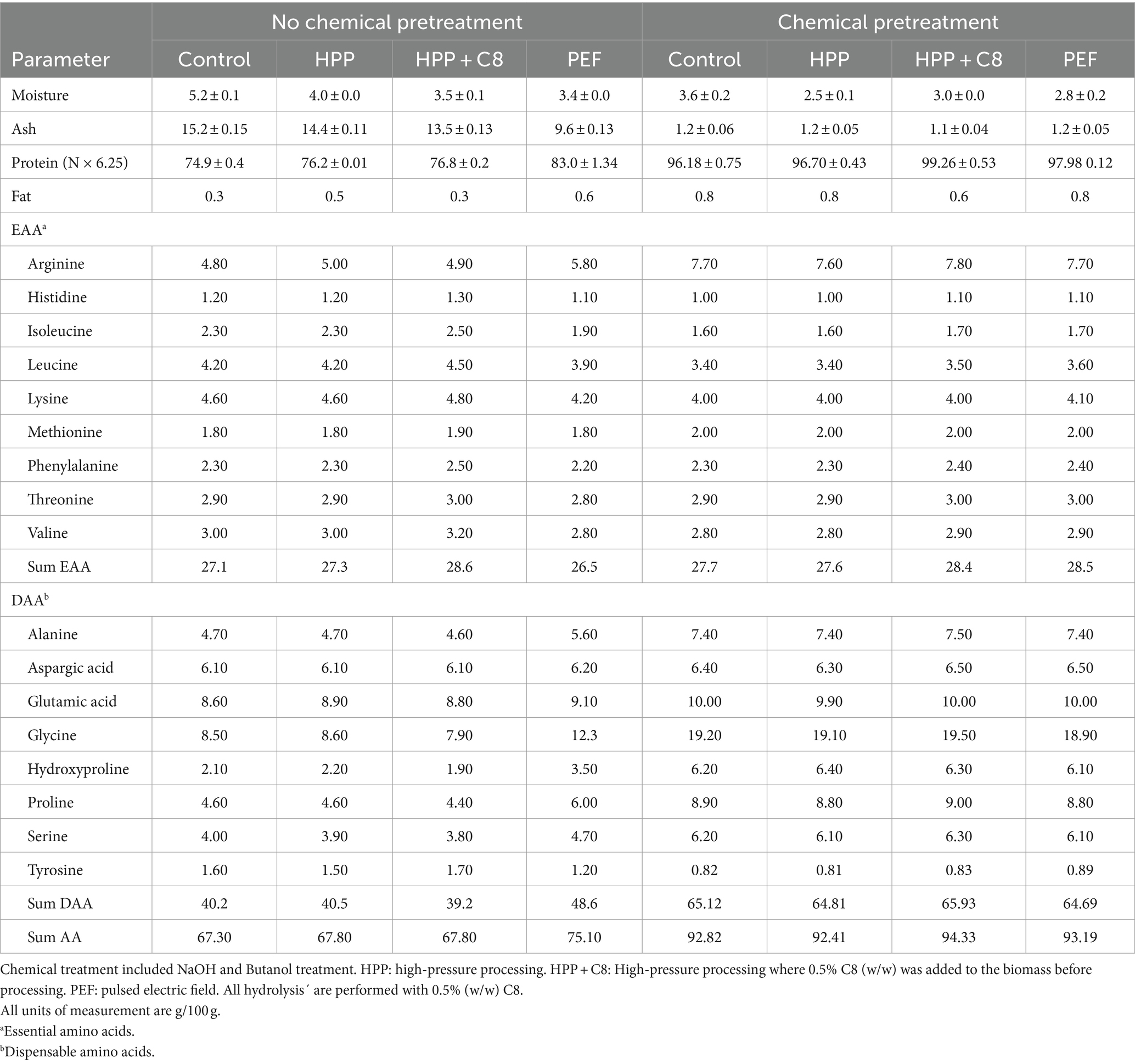
Table 6. Proximate analysis and total amino acid composition of hydrolysates where lumpfish was pretreated before hydrolysis.
The samples that underwent chemical pretreatment have lower ash values compared to non-treated samples (Table 6). This implies that the chemical pretreatment removes some non-protein compounds, such as mineral residues. The biomass that was not chemically pretreated was not washed with fresh water before processing. Therefore, the remaining minerals from seawater could also be a factor in increasing the ash values.
3.5 Initial bioactivity assessment of hydrolysates
The five fish protein hydrolysates produced with 0.5% (w/w) enzyme (FP, C7, C8, E1, and FL) were evaluated for bioactivity in a selection of assays (Figure 5). The two human cell lines, HepG2 and MRC-5, were screened mainly to exclude any potential toxic activities of the hydrolysates and to investigate any potential growth-promoting effects. Compared to the HepG2 cells, an increase in cell survival (up to 134%) was observed, indicating growth-promoting effects on the cells. The assays were performed with one biological replicate and three technical replicates, so further studies must be conducted for confirmational data. The same was observed in the non-malignant cell line MRC-5 (up to 171% cell survival). The results strongly indicate that the hydrolysates do not exert any toxic effects on the cells. On the contrary, they are boosting cell growth and survival. Further studies investigating potential growth-promoting effects should be considered for an extended panel of relevant cell lines, including primary muscle cells. An increase in cell proliferation has been observed after treatment with protein hydrolysates in several previous studies against a variety of cell lines (Yang et al., 2019; Chotphruethipong et al., 2021; Jung et al., 2022). The hydrolysates were assayed for antioxidative activities in a FRAP assay, and no activity was observed (Supplementary Table 4).
The hydrolysates produced with 0.5% (w/w) enzyme (FP, C7, C8, E1, and FL) were screened against five bacterial strains, three Gram-positive and two Gram-negative, at 100, 50, and 25 μg/mL in a minimal inhibitory concentration assay. None of the hydrolysates gave any considerable growth inhibition against any tested bacteria (Supplementary Table 5). No growth inhibition was observed against Candida albicans at any assayed concentrations (Supplementary Table 5). In the assay for biofilm inhibition, some effects were observed for the hydrolysates produced using the Corolase enzymes (C7 and C8) (Figure 6). At the highest concentration (100 μg/mL), the C7 hydrolysate resulted in a biofilm formation of 43%. No growth reduction was observed for the bacterium, indicating that the activity was specific for biofilm formation, not targeting bacterial growth.
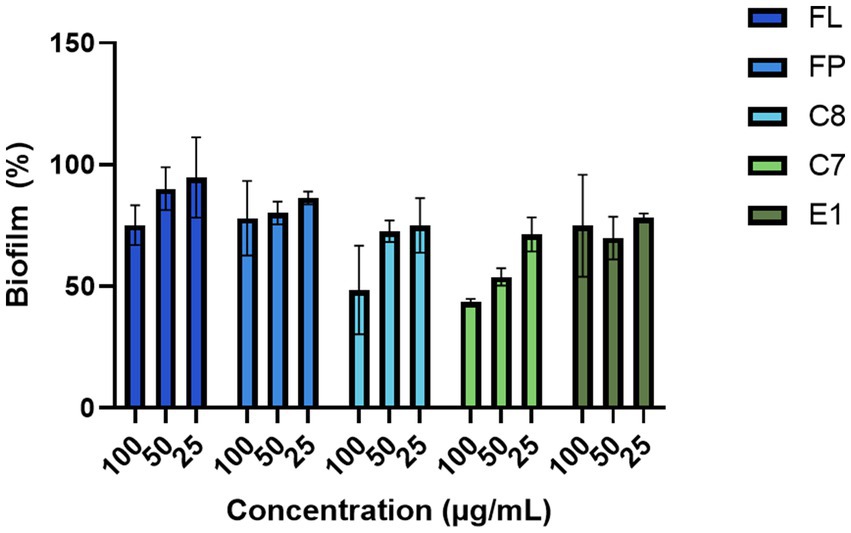
Figure 6. Inhibition of bacterial biofilm formation by the protein hydrolysates against the biofilm-producing S. epidermidis (n = 3).
4 Conclusion
Farmed lumpfish is only partially utilized after being used in sea lice treatment in aquaculture, and it is essential to find a better sustainable alternative for this biomass. One way to achieve this is to use the leftover biomass as a source for proteins, oils, and other molecules that can be used in various products such as food, feed, cosmetics, and packaging.
However, the low yield and recovery from the gelatin extraction process of lumpfish raw material makes the current method economically unsound. LSG is, however, high in collagen-associated amino acids, and further studies on gel strength and biological and physical properties need to be analyzed to evaluate its potential in different products. The complete protein extraction from whole lumpfish has both benefits and disadvantages. Chemical pretreatment increases the protein content by higher collagen peptide content but reduces the mass yield. Different pretreatments and combinations will suit different product categories and should be adapted to the products being developed. Yield is vital in bulk productions, e.g., feed, while collagen peptides are essential for tissue engineering and wound healing. The processing price will also be affected and should be considered when establishing a production pipeline.
Initial bioactivity testing showed no toxic effects of the hydrolysates on hepatocellular carcinoma and non-malignant lung fibroblast cells. Additionally, the hydrolysates seemed to boost cell growth and survival. The hydrolysates should be assayed at higher concentrations and with more replicates to obtain more information about this activity.
Using cleaner fish has raised ethical, economic, and sustainability concerns, and this practice may disappear over the years. However, it is crucial to ensure that if lumpfish is used as cleaner fish, it will be utilized in the best possible way. Reutilizing leftover biomass from the marine environment as a source of proteins, oils, and other molecules with nutritional or technical properties can minimize waste and benefit various industries and society.
Data availability statement
The original contributions presented in the study are included in the article/Supplementary material, further inquiries can be directed to the corresponding author.
Ethics statement
Ethical approval was not required for the studies on animals in accordance with the local legislation and institutional requirements because only commercially available established cell lines were used.
Author contributions
KL: Conceptualization, Data curation, Formal analysis, Investigation, Methodology, Project administration, Resources, Supervision, Validation, Visualization, Writing – original draft, Writing – review & editing. IM: Methodology, Resources, Writing – review & editing. TR: Methodology, Resources, Validation, Writing – original draft, Writing – review & editing. MJ: Formal analysis, Resources, Writing – original draft, Writing – review & editing. BV: Methodology, Writing – review & editing. RS: Conceptualization, Formal analysis, Funding acquisition, Methodology, Project administration, Validation, Visualization, Writing – original draft, Writing – review & editing.
Funding
The author(s) declare financial support was received for the research, authorship, and/or publication of this article. This project has received funding from the European Union’s Horizon 2020 research and innovation program under grant agreement 817992 and by the Norwegian Research Council as a part of the research project «From waste to food – sustainable exploitation of farmed lumpfish» with project number 301494.
Acknowledgments
The authors thank Gunhild Seljehaug Johanson (Nofima) for the illustration in Figure 1.
Conflict of interest
The authors declare that the research was conducted without any commercial or financial relationship that could be constructed as a potential conflict of interest.
Publisher’s note
All claims expressed in this article are solely those of the authors and do not necessarily represent those of their affiliated organizations, or those of the publisher, the editors and the reviewers. Any product that may be evaluated in this article, or claim that may be made by its manufacturer, is not guaranteed or endorsed by the publisher.
Supplementary material
The Supplementary material for this article can be found online at: https://www.frontiersin.org/articles/10.3389/fsufs.2024.1346548/full#supplementary-material
Footnotes
1. ^ https://www.hi.no/en/hi/temasider/species/sea-lice
2. ^https://www.fiskeridir.no/Akvakultur/Tall-og-analyse/Akvakulturstatistikk-tidsserier/Rensefisk.
3. ^ Implementation of gelatin for environmentally friendly food packaging - Nofima
References
Abbas, A. A., Shakir, K. A., and Walsh, M. K. (2022). Functional properties of collagen extracted from catfish (Silurus triostegus) waste. Food Secur. 11:633. doi: 10.3390/foods11050633
Ageeva, T. N., Lorentzen, G., Nilsen, H. A., and Lian, K. (2021). Lumpfish (Cyclopterus lumpus) used as cleaner fish: characterization and suitability for human consumption. Appl. Food Res. 1:100008. doi: 10.1016/j.afres.2021.100008
Amnuaikit, T., Shankar, R., and Benjakul, S. (2022). Hydrolyzed fish collagen serum from by-product of food industry: cosmetic product formulation and facial skin evaluation. Sustain. For. 14:16553. doi: 10.3390/su142416553
Arnesen, J. A., and Gildberg, A. (2007). Extraction and characterization of gelatine from Atlantic salmon (Salmo salar) skin. Bioresour. Technol. 98, 53–57. doi: 10.1016/j.biortech.2005.11.021
Asaithambi, N., Singha, P., and Singh, S. K. (2022). “Recent application of protein hydrolysates in food texture modification” in Critical reviews in food science and nutrition.
Aspevik, T., Steinsholm, S., Vang, B., Carlehög, M., Arnesen, J. A., and Kousoulaki, K. (2021). Nutritional and sensory properties of protein hydrolysates based on Salmon (Salmo salar), mackerel (Scomber scombrus), and herring (Clupea harengus) heads and backbones. Front. Nutr. 8:8. doi: 10.3389/fnut.2021.695151
Benzie, I. F. F., and Strain, J. J. (1996). The ferric reducing ability of plasma (FRAP) as a measure of “antioxidant power”: the FRAP assay. Anal. Biochem. 239, 70–76. doi: 10.1006/abio.1996.0292
Brooker, A. J., Papadopoulou, A., Gutierrez, C., Rey, S., Davie, A., and Migaud, H. (2018). Sustainable production and use of cleaner fish for the biological control of sea lice: recent advances and current challenges. Vet. Rec. 183:383. doi: 10.1136/vr.104966
Chotphruethipong, L., Binlateh, T., Hutamekalin, P., Sukketsiri, W., Aluko, R. E., and Benjakul, S. (2021). In vitro antioxidant and wound-healing activities of hydrolyzed collagen from defatted Asian sea bass skin as influenced by different enzyme types and hydrolysis processes. RSC Adv. 11, 18144–18151. doi: 10.1039/D1RA03131G
Dave, D., Liu, Y., Clark, L., Dave, N., Trenholm, S., and Westcott, J. (2019). Availability of marine collagen from Newfoundland fisheries and aquaculture waste resources. Bioresour. Technol. Rep. 7:100271. doi: 10.1016/j.biteb.2019.100271
Garcia de Leaniz, C., Gutierrez Rabadan, C., Barrento, S. I., Stringwell, R., Howes, P. N., Whittaker, B. A., et al. (2022). Addressing the welfare needs of farmed lumpfish: knowledge gaps, challenges and solutions. Rev. Aquac. 14, 139–155. doi: 10.1111/raq.12589
Giannetto, A., Esposito, E., Lanza, M., Oliva, S., Riolo, K., di Pietro, S., et al. (2020). Protein hydrolysates from anchovy (Engraulis encrasicolus) waste: in vitro and in vivo biological activities. Mar. Drugs 18:86. doi: 10.3390/md18020086
Hansen, K. Ø., Andersen, J. H., Bayer, A., Pandey, S. K., Lorentzen, M., Jørgensen, K. B., et al. (2019). Kinase Chemodiversity from the Arctic: the Breitfussins. J. Med. Chem. 62, 10167–10181. doi: 10.1021/acs.jmedchem.9b01006
Imsland, A. K. D., Hanssen, A., Nytrø, A. V., Reynolds, P., Jonassen, T. M., Hangstad, T. A., et al. (2018). It works! Lumpfish can significantly lower sea lice infestation in large-scale salmon farming. Biol. Open 7:bio036301. doi: 10.1242/bio.036301
Imsland, A. K., Reynolds, P., Eliassen, G., Hangstad, T. A., Nytrø, A. V., Foss, A., et al. (2014). Assessment of growth and sea lice infection levels in Atlantic salmon stocked in small-scale cages with lumpfish. Aquaculture 433, 137–142. doi: 10.1016/j.aquaculture.2014.06.008
Jansson, E., Faust, E., Bekkevold, D., Quintela, M., Durif, C., Halvorsen, K. T., et al. (2023). Global, regional, and cryptic population structure in a high gene-flow transatlantic fish. PLoS One 18:e0283351. doi: 10.1371/journal.pone.0283351
Jenssen, M., Rainsford, P., Juskewitz, E., Andersen, J. H., Hansen, E. H., Isaksson, J., et al. (2021). Lulworthinone, a new dimeric Naphthopyrone from a marine fungus in the family Lulworthiaceae with antibacterial activity against clinical methicillin-resistant Staphylococcus aureus isolates. Front. Microbiol. 12:740. doi: 10.3389/fmicb.2021.730740
Juárez-Enríquez, E., Levario-Gómez, A., Ochoa-Reyes, E., Tirado-Gallegos, J. M., Baeza-Jiménez, R., and Buenrostro-Figueroa, J. (2022). “Chapter 34 - significance of enzyme kinetics in food processing and production” in Value-Addition in Food Products and Processing Through Enzyme Technology (Academic Press).
Jung, H., Jung, D., Lee, J., Ki, W., Lee, J. M., Kim, E. M., et al. (2022). Bioactive peptides in the pancreatin-hydrolysates of whey protein support cell proliferation and scavenge reactive oxygen species. Anim. Cells Syst. 26, 232–242. doi: 10.1080/19768354.2022.2130425
Korhonen, H., and Pihlanto, A. (2006). Bioactive peptides: production and functionality. Int. Dairy J. 16, 945–960. doi: 10.1016/j.idairyj.2005.10.012
Liaset, B., and Espe, M. (2008). Nutritional composition of soluble and insoluble fractions obtained by enzymatic hydrolysis of fish-raw materials. Process Biochem. 43, 42–48. doi: 10.1016/j.procbio.2007.10.007
Lin, Y., Cai, X., Wu, X., Lin, S., and Wang, S. (2020). Fabrication of snapper fish scales protein hydrolysate-calcium complex and the promotion in calcium cellular uptake. J. Funct. Foods 65:103717. doi: 10.1016/j.jff.2019.103717
Mæhre, H. K., Dalheim, L., Edvinsen, G., Elvevoll, E., and Jensen, I. J. (2018). Protein determination—method matters. Food Secur. 7:5. doi: 10.3390/foods7010005
Milan, E. P., Rodrigues, M. Á. V., Martins, V. C. A., Plepis, A. M. G., Fuhrmann-Lieker, T., and Horn, M. M. (2021). Mineralization of phosphorylated fish skin collagen/Mangosteen scaffolds as potential materials for bone tissue regeneration. Molecules 26:2899. doi: 10.3390/molecules26102899
Naghdi, S., Rezaei, M., Tabarsa, M., and Abdollahi, M. (2023). Parallel extraction of Sulfated polysaccharides and protein hydrolysate from skipjack tuna head and their bioactive and functional properties. Food Bioprocess Technol. 16, 1258–1279. doi: 10.1007/s11947-022-02988-w
NMKL Nitrogen determination in foods and feeds according to Kjeldahl (codex endorsed method), NMKL (2003).
NMKL Moisture and ash. Gravimetric determination in meat and meat products NMKL 23, 1991, 3. ed. REPEALED (2022).
Nøstvold, B.H., Kvalvik, I, Voldnes, G., and Jentoft, A.R. Etterbruk av rognkjeks - Fra lusespiser til middagsmat in Nofima’s reports 43/2016, Nofima, Editor. (2016).
Powell, A., Treasurer, J. W., Pooley, C. L., Keay, A. J., Lloyd, R., Imsland, A. K., et al. (2018). Use of lumpfish for sea-lice control in salmon farming: challenges and opportunities. Rev. Aquac. 10, 683–702. doi: 10.1111/raq.12194
Szkudzińska, K., Smutniak, I., Rubaj, J., Korol, W., and Bielecka, G. (2017). Method validation for determination of amino acids in feed by UPLC. Accred. Qual. Assur. 22, 247–252. doi: 10.1007/s00769-017-1281-9
Thong, N. T., Ngoc, Q.T.K., and Voldnes, G. (2023). Consumer’s perception and acceptance of lumpfish used in salmon cages. Aquacult Int doi: 10.1007/s10499-023-01273-7
Vang, B., Lian, K., Berntssen, M., Ørnsrud, R., Sele, V., Solstad, R. G., et al. (2021). Utfordringer som hindrer økt utnyttelse av marint restråstoff og marine arter in Nofima’s reports 29/2021. Editor: Nofima.
Vate, N. K., Strachowski, P., Undeland, I., and Abdollahi, M. (2023). Structural and functional properties of collagen isolated from lumpfish and starfish using isoelectric precipitation vs salting out. Food Chem. 18:100646,
Wubshet, S. G., Måge, I., Böcker, U., Lindberg, D., Knutsen, S. H., Rieder, A., et al. (2017). FTIR as a rapid tool for monitoring molecular weight distribution during enzymatic protein hydrolysis of food processing by-products. Anal. Methods 9, 4247–4254. doi: 10.1039/C7AY00865A
Yang, F., Jin, S., and Tang, Y. (2019). Marine collagen peptides promote cell proliferation of NIH-3T3 fibroblasts via NF-κB Signaling pathway. Molecules 24:4201. doi: 10.3390/molecules24224201
Zaky, A. A., Simal-Gandara, J., Eun, J. B., Shim, J. H., and Abd el-Aty, A. M. (2022). Bioactivities, applications, safety, and health benefits of bioactive peptides from food and by-products: a review. Front. Nutr. 8:8. doi: 10.3389/fnut.2021.815640
Keywords: lumpfish (Cyclopterus lumpus), protein hydrolysates, enzymatic hydrolysis, pretreatment, pulsed electric field, high-pressure processing
Citation: Lian K, Maribu I, Rode TM, Jenssen M, Vang B and Solstad RG (2024) More sustainable use of aquaculture cleaner fish: collagen-rich protein hydrolysates from lumpfish (Cyclopterus lumpus) – effects of biomass, pretreatment, and enzyme choice. Front. Sustain. Food Syst. 8:1346548. doi: 10.3389/fsufs.2024.1346548
Edited by:
Chunhong Yuan, Iwate University, JapanReviewed by:
Chanthima Phungamngoen, Khon Kaen University, ThailandBin Wang, Zhejiang Ocean University, China
Mehdi Nikoo, Urmia University, Iran
Copyright © 2024 Lian, Maribu, Rode, Jenssen, Vang and Solstad. This is an open-access article distributed under the terms of the Creative Commons Attribution License (CC BY). The use, distribution or reproduction in other forums is permitted, provided the original author(s) and the copyright owner(s) are credited and that the original publication in this journal is cited, in accordance with accepted academic practice. No use, distribution or reproduction is permitted which does not comply with these terms.
*Correspondence: Kjersti Lian, kjersti.lian@nofima.no