- 1Pilot Plant Development and Training Institute, King Mongkut’s University of Technology Thonburi, Bangkok, Thailand
- 2Department of Food Engineering, Faculty of Engineering, King Mongkut’s University of Technology Thonburi, Bangkok, Thailand
- 3Department of Microbiology, Faculty of Science, King Mongkut’s University of Technology Thonburi, Bangkok, Thailand
- 4School of Food Technology, Institute of Agricultural Technology, Suranaree University of Technology, Nakhon Ratchasima, Thailand
Wolffia is a protein-rich aquatic plant with the potential to help address food sustainability issues; however, a more efficient extraction process must be sought due to limited yield with conventional methods. This study aimed to investigate the effects of duckweed forms (fresh and dried), duckweed-to-aqueous solution ratio (DSR), as well as alkaline and ultrasonication extractions on yields, physicochemical and techno-functional properties of duckweed protein extracts (DPE). Three extraction methods were used: alkaline extraction (AE), ultrasound-assisted alkaline extraction (UAAE), and ultrasound-assisted water extraction (UAWE). The study showed fresh duckweed resulted in a 2.5-fold higher yield and protein recovery than oven-dried duckweed. UAE significantly enhanced extraction yield and protein recovery. The optimal extraction process was a DSR of 1:6 using UAAE at pH 8.5, resulting in a 16% yield and 34% protein recovery. Furthermore, the study found that UAE facilitated the extraction of non-polar/hydrophobic amino acids while AE proved to be efficient in extracting sulfur-containing amino acids. This study, for the first time, revealed the role of UAE and AE in promoting the extraction of different profiles of amino acids. The DPE produced using UAAE contained 65% protein, meeting the WHO/FAO/UNU recommended protein intake for preschool-aged children. Furthermore, the DPE exhibited excellent emulsifying properties and oil-holding capacity comparable to commercial soy protein isolates. Overall, UAAE was identified as a promising approach for producing techno-functional and nutritious protein ingredients from Wolffia. The resulting DPE proved to show great potential for functional plant-based food and feed applications.
1 Introduction
Currently, approximately one billion people worldwide lack access to nutritious diets, while the global demand for protein is expected to double by 2050 (Raja et al., 2022). The lack of diversity in dietary protein sources is one of the crucial factors that severely impacts food system sustainability (Colgrave et al., 2021; Raja et al., 2022). Therefore, any innovative approaches that enable the development of new alternative protein-rich ingredients to be applied in food, beverages, and feed products are urgently needed to bridge the protein diet gap. Apart from protein nutritional attributes, techno-functional property is a crucial factor in the applicability of alternative proteins. Recently, there has been a surge in research to develop novel, techno-functional, and nutritious protein ingredients from existing plants (Colgrave et al., 2021; Anoop et al., 2023).
Duckweed is a promising source of aquatic plant protein due to its high protein content and hypoallergenic properties (Turck et al., 2021). The FDA has filed it as GRAS or Generally Recognized as Safe (EFSA, 2021). Wolffia is the most prevalent genus of duckweed found in Asia, with W. arrhiza and W. globosa being the two most abundant species found in Thailand (Ruekaewma et al., 2015; Appenroth et al., 2018; Prosridee et al., 2023). The plant has a rapid growth rate, reproduces asexually, has a short life cycle, and requires only a small cultivation area, making it an emerging food protein source (Yang et al., 2020; Nieuwland et al., 2021; Anoop et al., 2023). Duckweed typically contains high-quality protein in terms of PDCAAS and amino acid content, making it a potential ingredient for protein manufacturers (Appenroth et al., 2017; Duangjarus et al., 2022; Pagliuso et al., 2022). However, the high water content of up to 97% in fresh duckweed (EFSA, 2021) presents a significant challenge for transportation and storage. Dried duckweed has emerged as a preferred alternative, but the drying process may affect the protein’s extractability and techno-functional properties, and there is no report to clarify this effect.
Plant protein is typically extracted through alkaline extraction and precipitation at the isoelectric point (pI). However, a more efficient extraction process is needed for Wolffia due to limited yield. Ultrasonication is an eco-friendly alternative, efficient, and applicable on a large scale for extracting bioactive compounds (Patist and Bates, 2008; Kingwascharapong et al., 2021; Sert et al., 2022). The mechanism behind UAE is that ultrasound waves generate cycles of compression and rarefaction, which create numerous vacuum bubbles within the liquid. When these bubbles implode, they generate high shear forces and temperatures, which aid in extracting components from the cells. The impacts of the combined use of AE and UAE extraction on the yield, physicochemical, and techno-functional properties of duckweed protein extract (DPE) have not yet been reported.
This study aimed to address the knowledge gaps by investigating the effects of duckweed form (fresh and dried), protein extraction methods, i.e., alkaline extraction (AE), ultrasound-assisted alkaline extraction (UAAE), and ultrasound-assisted water extraction (UAWE) on the production yield, protein recovery, physicochemical, and techno-functional properties, as well as amino acid profiles of the extracted Wolffia proteins. The findings of this study are of utmost importance as they provide crucial scientific data on the use of UAAE to produce techno-functional duckweed proteins for food and feed applications.
2 Materials and methods
2.1 Materials
In this study, fresh duckweeds (W. arrhiza) were purchased from Areeya Duckweed Farm (Kalasin Province, Thailand). The duckweeds were cultured in a closed hydroponic system. To ensure their purity, the samples were thoroughly cleaned with tap water to remove any foreign matter, then drained by using a cheesecloth, and packed in plastic bags. During transportation to King Mongkut’s University Laboratory, the duckweeds were kept at a temperature of 4 1°C. According to the proximate composition analysis, the duckweed contained 30.07 0.19% protein, 28.55 0.34% total dietary fiber, 18.78 0.02% ash, 16.28 0.58% carbohydrate, and 6.32 ± 0.03% lipid. For the study, three forms of duckweed were prepared: fresh granules, dried granules, and powder. The fresh duckweed samples were processed the day after arrival, while the dried duckweed was prepared on the same day of arrival. Drying was carried out using a hot air rotary oven (Obaking, OBX-G32, China) at a controlled temperature of 53 ± 1°C for 15 h to achieve a final moisture content of 5.5 ± 0.5% (wet basis, w.b.). The dried duckweed was ground using an ultra-centrifugal mill (Retsch, ZM200, Haan, Germany) with a ring sieve size of 250 μm to obtain duckweed powder. The powder was packed in an aluminum laminated pouch, and kept in a desiccator. Sample was used by 1 week.
2.2 Sample preparation
2.2.1 Cell disruption using ultrasonication
Duckweed powder (13 g, dry weight basis) was immersed in 430 g distilled water for 12 h to reach a moisture content of approximately 80 ± 1% (w.b.). This soaking condition provided a rehydrated duckweed suspension with a weight ratio of rehydrated duckweed powder to extract aqueous media of 1/6. For duckweed protein extract, or DPE, produced with ultrasound-assisted extraction (i.e., UAAE and UAWE), the rehydrated duckweed suspension was subjected to cell disruption using an ultrasonicator (Sonics & Materials, VCX-750 Vibra-cell, Oxon, United Kingdom). A 25-mm diameter titanium alloy tip was used for cell disruption. The sample was treated with ultrasonication at an operating condition of 20 kHz and a 70% amplitude, which provided a power of approximately 562.5 W for 18 min. This operating condition resulted in an energy intensity of 107 W/cm2. The ultrasonication was performed in cycles, with each duty cycle consisting of 30 s of ultrasonication followed by a 10-s pause. To prevent protein from thermal denaturation, the temperature of the duckweed suspension was controlled at approximately 10 ± 1°C by placing the glass beaker containing the sample suspension in an ice slurry bath. After the ultrasonication, the sample had a pH of 6.6 ± 0.1.
2.2.2 Preparation of duckweed protein extracts
In this study, duckweed protein extracts (DPEs) were produced using three different extraction techniques: alkaline extraction (AE), ultrasonication-assisted alkaline extraction (UAAE), and ultrasonication-assisted water extraction (UAWE). Table 1 shows DPEs preparation methods and abbreviation of the methods used in this study. For UAAE and UAWE samples, the rehydrated duckweed suspensions were subjected to ultrasonication to break the cells, followed by alkaline (for UAAE) or water (for UAWE) extraction, respectively. Protein extraction of samples AE and UAAE was performed using an alkali extraction method. The pH of the rehydrated duckweed suspension was adjusted to 8.5 with 1.0 and 0.5 M NaOH, and stirred continuously for 2 h at room temperature (25 1°C). The mixture was then centrifuged at 16,500 × g for 15 min at 4°C, and the supernatant was filtered through a two-layer cheesecloth. The pH of the supernatant was then lowered to 4.5 using 1 and/or 0.5 N HCl. The acidified sample was stirred for 30 min at room temperature before being centrifuged at 16,500 × g for 15 min to obtain a DPE pellet. For the UAWE sample, the same procedure was followed, except that the alkalization step was omitted. The resulting protein pellet was pre-frozen at 20°C and then freeze-dried at 35°C under a vacuum pressure of 20 Pa for 30 h (Christ, Alpha 1–4 LSCPlus, Germany) to obtain a freeze-dried sample with a final moisture content of 5.0 0.5% (w.b.).
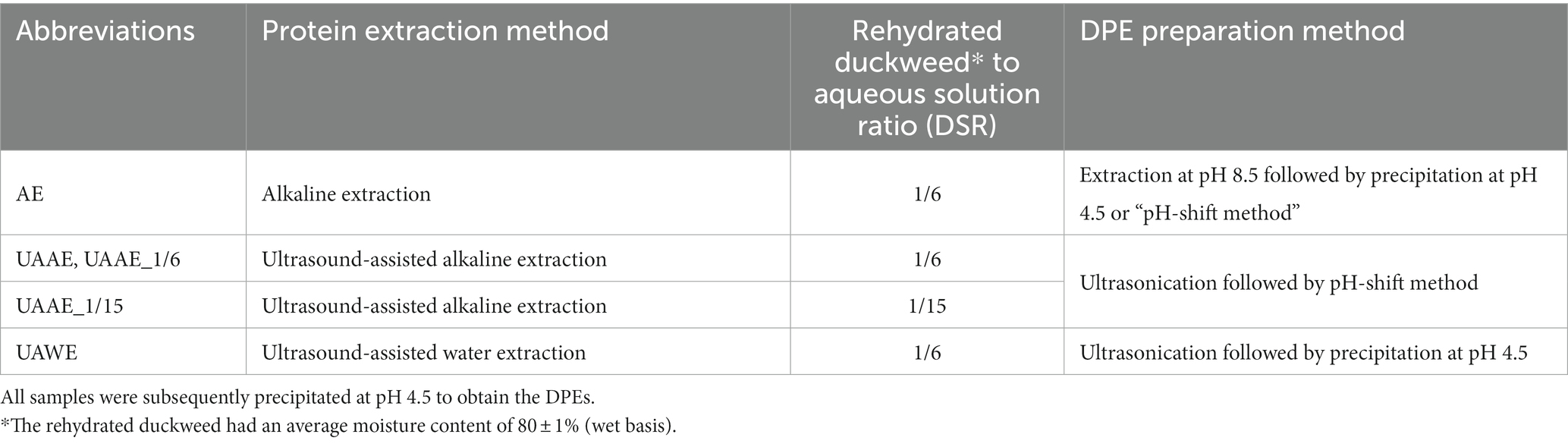
Table 1. Preparation methods of duckweed protein extracts (DPEs) and abbreviations of the methods used in the study.
Production yield, protein yield, and protein recovery were calculated to discuss protein extractability. The production yield was the mass of the resulting DPE compared to the total mass of duckweed used for extraction. Protein yield was the protein mass in the resulting DPE compared to the total mass of duckweed used for extraction. Protein recovery was the protein mass in the resulting DPE compared to the total protein mass contained in duckweed used for extraction. All values were obtained using a dry weight basis and with triplicate measurements.
2.3 Analysis of physicochemical and techno-functional properties
2.3.1 Chemical composition
The protein content was evaluated based on the nitrogen combustion technique and the Dumas nitrogen analysis method of the sample was evaluated based on the studies of Grossmann et al. (2019) and Nieuwland et al. (2021). Calibration curves were prepared using EDTA with a known nitrogen content of 9.58%. Crude protein content was calculated using a nitrogen-to-protein conversion factor of 6.25 (Appenroth et al., 2017; EFSA, 2021; Duangjarus et al., 2022). Other compositions, including lipids, total dietary fibers, ash, and carbohydrates, were performed following the methods of Grossmann et al. (2019) and Nieuwland et al. (2021). The remaining percentage was indicated as the carbohydrate content.
2.3.2 Determination of amino acids
To determine the amino acid profile of duckweed protein extracts (DPE), a microwave extraction method was used with slight modifications to the procedure described by Moldoveanu (2005). The DPE was weighed for 19 ± 1 mg, dissolved in 10 mL of 6 N HCl containing 0.1% (v/v) phenol, and then flushed with nitrogen. The mixture was subjected to microwave digestion at 130°C for 30 min (MultiWave3000, Anton Paar GmbH, Graz, Austria) and then cooled down to ambient temperature. The digested sample was filtered through a 0.45-μm PTFE membrane. Finally, the amino acid in the DPE sample was analyzed via ion exchange chromatography using an amino acid analyzer (Biochrome 30 , Biochrom, Cambridge, United Kingdom).
2.3.3 Protein electrophoresis
Dried duckweed and duckweed protein extracts (DPEs) were dissolved in 5%SDS and heated at 95°C for 5 min. The protein solution was mixed with a sample buffer containing 0.125 M Tris–HCl (pH 6.8), 4% SDS, 20% glycerol, and 10% BME- at a ratio of 1:1. SDS-PAGE was performed according to the method of Tang and Yongsawatdigul (2020). Sample solutions (10 μg/μL) were loaded onto 10, 12.5, and 15% polyacrylamide gel and run at 60 V for 5 min, followed by 120 V for 90 min. The gel was then stained using 0.125% Coomassie Brilliant Blue R-250, and destained using a mixed solution of 25% methanol and 10% acetic acid. TriColor Broad Protein Ladder (3.5–245 kDa) was used to evaluate protein mass.
2.3.4 Solubility
The examination method was performed similar to the method of Cui et al. (2020) with a slight modification. Protein solubility of the samples in DI water having pH in the range of 3.0–9.0 was examined. The samples were then centrifuged at 16,500 g for 15 min, and the protein content in the supernatant was measured using the Carbon/Nitrogen Analyzer method (LECO, CN828, United States). The solubility profile of DPE was calculated as the percentage of protein in the supernatant compared to the total amount of initial protein.
2.3.5 Water holding capacity and oil holding capacity
WHC and OHC were determined using the method of Stone et al. (2015) with some modifications. Duckweed protein extract (0.1 g) was mixed with 10 mL of distilled water or soybean oil in a pre-weighed centrifuge tube and vortexed at high speed for 30 s. The sample was then incubated at room temperature for 30 min. Next, the hydrated sample was centrifuged at 15,000 g for 20 min. The supernatant was gently decanted, the precipitate was collected and reweighed. WHC and OHC were calculated using Eqs. (1, 2), respectively.
2.3.6 Emulsifying capacity and emulsifying stability
The emulsifying capacity and stability of the samples were determined according to the method described by Duangjarus et al. (2022) with a slight modification. DPE dissolved in distilled water at a concentration of 1% (w/v) was prepared and the pH was adjusted to 7.0 and 8.5. The fraction that was completely soluble in water was obtained by centrifugation, and the supernatant or DPE solution was collected for the test. Ten milliliters of the DPE solution were then homogenized with 10 mL of sunflower oil using a high-shear homogenizer (Ultra-Turrax IKA, T25, Staufen, Germany) for 1 min at room temperature. An additional 10 mL of sunflower oil was added, and the mixture was homogenized again at the same homogenization speed for 2 min. The homogenized sample was left to stand at ambient temperature for 24 h. The height of the emulsion layer (HEL) and the total height of the sample (HTS) were measured at t0 and t24. Emulsion capacity (EC) was calculated at t0 by dividing HEL0 by HTS0. Emulsion stability (ES) was calculated at t24 by determining EC24 and EC0, and then dividing EC24 by EC0. Both ES and EC were expressed as a percentage.
2.3.7 Statistical analysis
All analyses of the DPEs properties were conducted at least in duplicate. The experimental data is presented as To assess the differences among the data, an analysis of variance (ANOVA) was conducted, and Tukey’s range test was performed at a confidence level of 95% ( ) using the SPSS package software.
3 Results and discussion
3.1 Effects of raw material state on the extractability of DPEs.
As mentioned earlier, fresh duckweed has an extremely high-water content, which incurs additional costs and makes transportation logistics and industrial operations difficult (EFSA, 2021). To overcome this issue, it is necessary to dry the duckweed before protein production. However, drying can potentially affect the extractability and qualities of the duckweed protein. Therefore, the impact of hot-air drying on protein extractability and properties was investigated.
Figure 1 shows the production yield, protein yield, and protein recovery of the duckweed protein extract (DPE) produced from fresh and dried duckweed granules and ground dried duckweed, so-called duckweed powder. The results indicated that the extraction yield, protein yield, and protein recovery of DPE obtained from fresh granules were significantly higher than those obtained from dried granules by approximately 3.4, 3.3, and 2.9 folds, respectively However, the protein content of the two samples was not significantly different (p ≥ 0.05), with values of 67.1 and 67.3% for fresh and dried granules, respectively. The results could be explained through the change of microstructure undergoing hot-air drying.
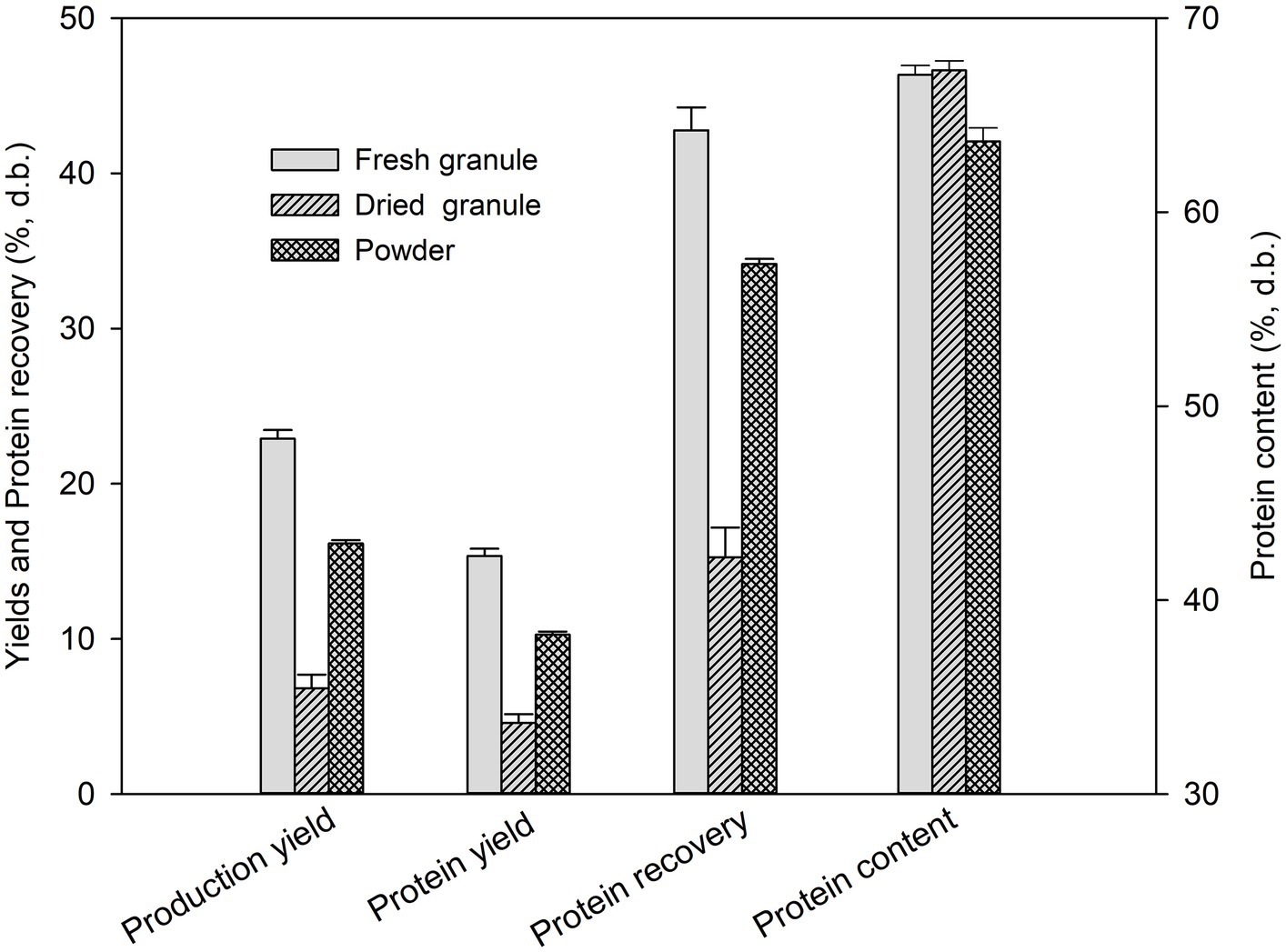
Figure 1. Yields, protein recovery, and protein contents of duckweed protein extracts prepared from fresh and dried duckweed granules and ground dried duckweed or duckweed powder.
As shown by the microimages in Figure 2, the cell structure of duckweed undergoing hot air drying was shrinking and collapsing compared to fresh duckweed. As a result, the dried duckweed had a lower protein extractability due to its reduced ability to absorb water and swell. The microstructure changes of the dried duckweed cell matrix could adversely affect molecular diffusivity through the cells during protein extraction, thereby minimizing extraction efficiency. A similar result was obtained in the study of Nguyen et al. (2018), which observed that shrinkage of cell walls and porosity reduction in bulk tissue of fruits and vegetables undergoing hot-air drying significantly occurred, leading to a severe reduction in the rehydration properties of the dried products. These findings suggest that while hot air drying is necessary for commercial-dried duckweed preparation, it adversely affects production yield and protein recovery. Enhancing the surface area of dried duckweed cells exposed to extraction solution could help maximize the protein extractability.
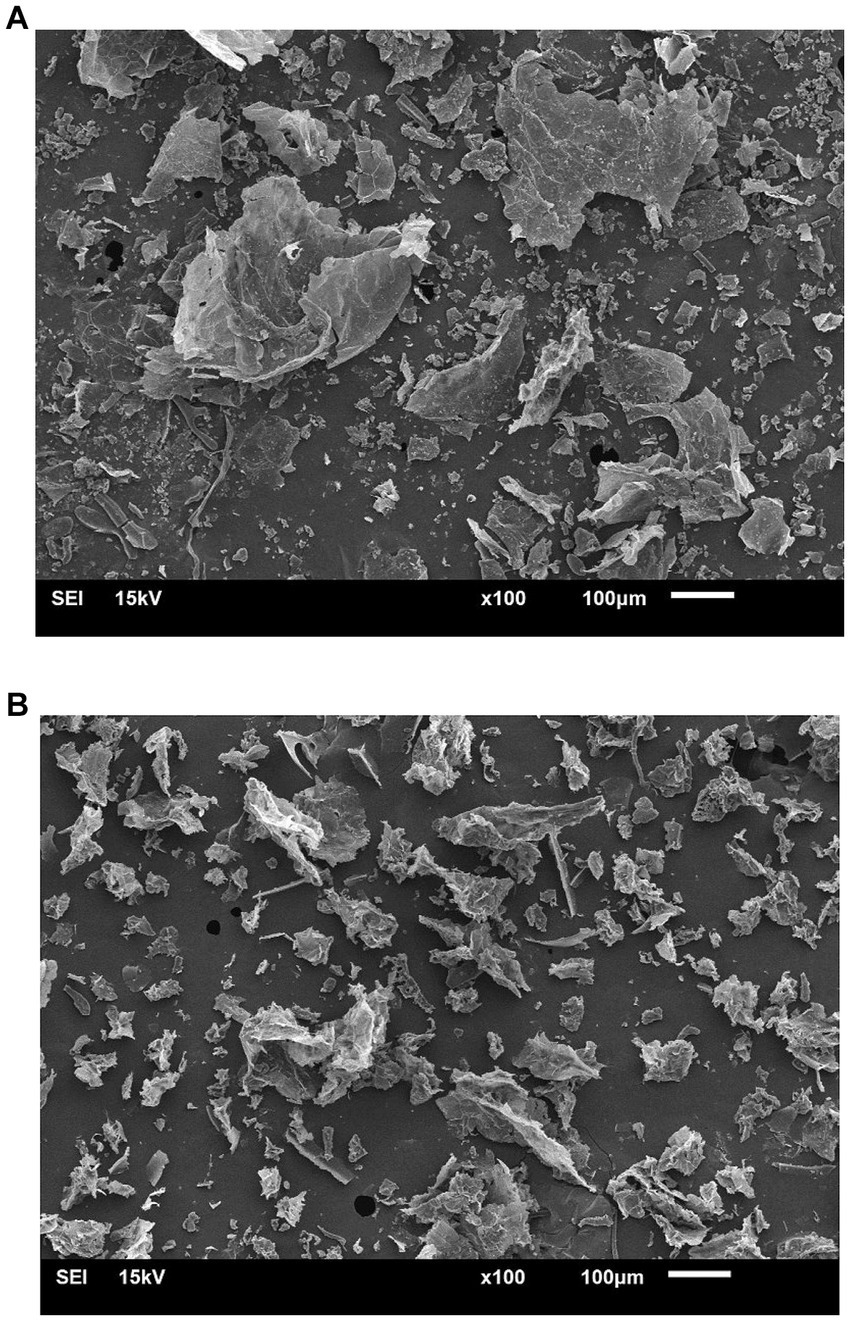
Figure 2. Scanning electron microimages of (A) fresh, and (B) dried duckweed particles undergoing ultrasonication in water using the duckweed-to-aqueous solution ratio of 1/6. The microimages were taken at a magnification of 100 . The scale bars are 100 μm.
To enhance the production yield and protein recovery of dried duckweed granules, their size was reduced to 250 μm using an ultra-centrifugal mill. As shown in Figure 1, the results indicate that using a powdery form resulted in a significant improvement in the production yield and protein recovery of DPE . The improvement was approximately 2.3 and 2.4 times, respectively, compared to using a granular form. However, protein content decreased from 67.3 to 63.7% ( ). This might be explained by the fact that other components in duckweed cells, such as lipids and polysaccharides, were co-extracted with the proteins, leading to a reduction in the protein content ratio of DPE. Many studies have strongly evidenced that ultrasonication effectively facilitates lipid extraction from various bio-feedstocks, such as plants, microalgae, and fungi, attributed to the great rupturing of the cells by the high-intensity ultrasonication waves (Yao et al., 2018; Stevanato and da Silva, 2019; Deng et al., 2022).
Moreover, the study showed that the yield of the yield and protein recovery of DPE produced from duckweed powder were only 1.3 and 1.2-fold lower than those obtained from fresh duckweed granules, respectively. This finding implies that using duckweed in powdery form could be a feasible and effective alternative to fresh duckweed in producing DPE, yielding almost comparable results. This study proposes a promising solution to the problem of using fresh duckweed as feedstock for commercial production of duckweed proteins, as mentioned previously.
3.2 Effects of duckweed-aqueous solution ratio on extractability, chemical composition, and techno-functional properties of DPEs
3.2.1 Extractability
The extraction of duckweed protein was studied using different duckweed-to-aqueous solution ratios (DSR) in the range of 1/6 to 1/25, as reported by Nieuwland et al. (2021), Duangjarus et al. (2022), and Inguanez et al. (2023). However, comparing the results of these studies can be challenging due to variations in the types of duckweed and moisture content used.
In our study, duckweed powder with an 80 ± 1% moisture content after 12-h rehydration was used as a starting material. Therefore, the DSR used in the studies of Nieuwland et al. (2021) and Duangjarus et al. (2022) were estimated at 80% moisture content and were found to be equivalent to 1/5.5 and 1/20, respectively. A DSR range of 1/6 to 1/20 was thus set to determine its effect on duckweed protein extractability.
As shown in Figure 3, the protein content, protein yield, and protein recovery of DPEs increased with an increase in the aqueous solution ratio up to 15. However, a further increase did not significantly improve extractability. The production yield of the DPEs was not considerably affected by the DSR within the studied range (p ≥ 0.05). The studies of Potin et al. (2019), Bello et al. (2023), and Shen et al. (2023) reported similar results, showing that extraction efficiency and yield increased as the solid-to-liquid ratio used in the UAE process increased. This could be explained because the higher solvent ratio helps dissolve the extracted compound, making the mixture less dense and promoting the cavitation effect, which improves the extraction efficiency.
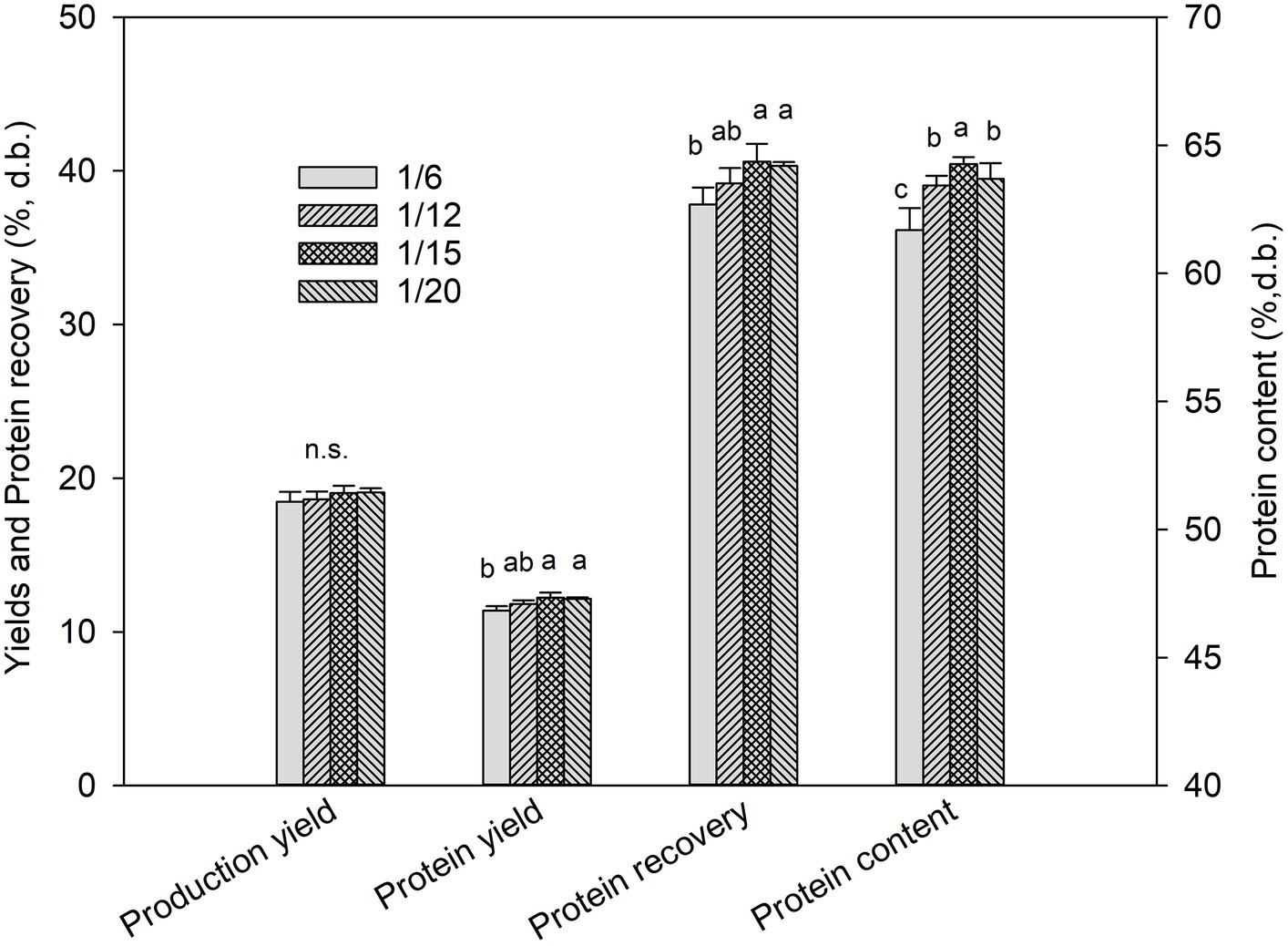
Figure 3. Yields, protein recovery, and protein contents of duckweed protein extracts prepared from duckweed powder using ultrasound-assisted alkaline extraction (UAAE) at a duckweed-to-solvent ratio (DSR) of 1/6, 1/12, 1/15, and 1/20. N.S. means not significantly different (p ≥ 0.05).
As above reported, when a DSR ratio was used after 1/15, the resulting extraction yields were ineffective. This observation is consistent with the findings of Sun et al. (2018) and Liao et al. (2022), who also noted that using too much solvent in the UAE process can compromise extraction yields beyond a certain limit. This might be due to the excessive solvent volume that compromised the acoustic energy density, leading to a reduction in the cavitation effect and extraction efficiency. Additionally, using higher solvent ratios in an industrial-scale operation is impractical due to lower production capacity, higher effluents, and increased operating costs. The extraction ratio has been shown to affect the chemical composition of the extracted proteins, which can in turn affect the techno-function of the DPE. Based on these findings, DSRs of 1/6 and 1/15 were selected to prepare DPE for further analysis.
3.2.2 Chemical composition
As presented in Table 2, there was no significant difference in the protein and lipid contents of the DPE extracted at DSR of 1/6 and 1/15. However, increasing the aqueous solution ratio from 6 to 15 resulted in a considerable reduction in total dietary fiber from 8.93 0.16 to 1.39 0.01%. It was noted that hemicellulose and some dietary fibers were dissociated in an alkali solution (Maphosa and Jideani, 2016). Therefore, increasing the alkali solution ratio reduced the dietary fiber content of the DPEs. Additionally, the ash content of the DPE produced at the DSR of 1/15 was doubled compared to the DPE produced at the DSR of 1/6, which could be attributed to the higher amount of NaOH used for extraction. The results demonstrated the impact of the DSR on regulating the chemical composition and playing a role in the particle size distribution of the protein extracts.
3.2.3 Technofunctional properties
Table 3 shows techno-functional properties of DPEs produced at two different DSRs (1/6 and 1/15). The properties were evaluated in terms of water holding capacity (WHC), oil holding capacity (OHC), and emulsifying properties (emulsifying capacity or EC and emulsifying stability or ES). The results indicate that the DSR values in the range of 1/6 to 1/15 did not affect DPE’s functional properties. As a result, the DSR of 1/6 was selected for DPE preparation in subsequent studies.
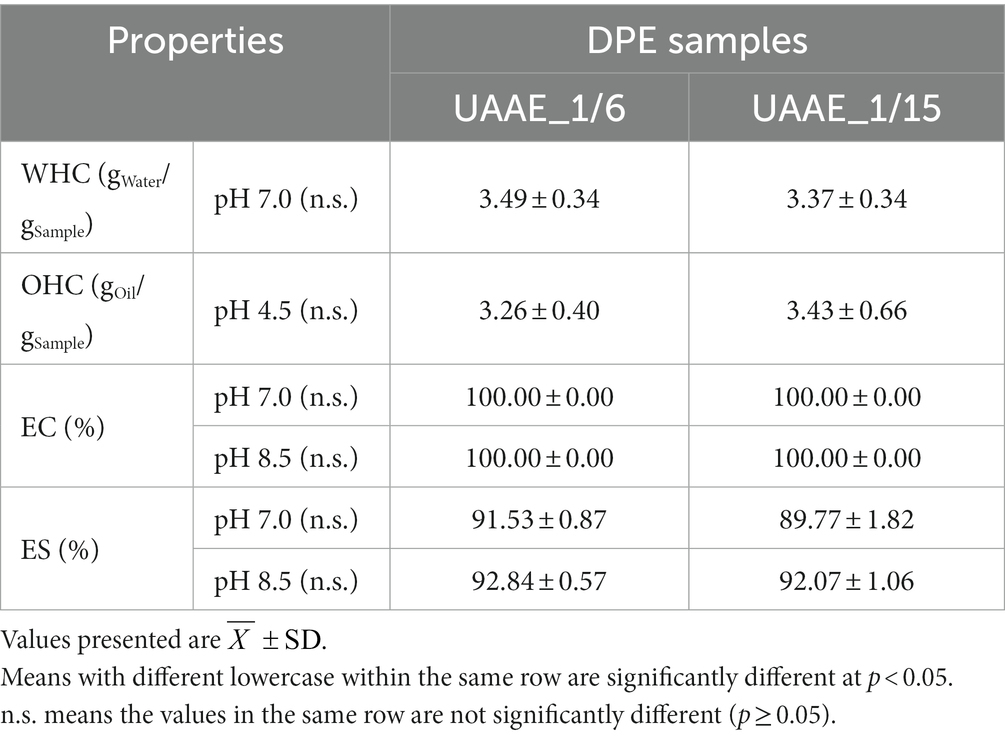
Table 3. Water holding capacity (WHC), oil holding capacity (OHC), and emulsifying properties, including emulsifying capacity (EC), and emulsifying stability (ES) of UAAE_1/6 and UAAE_1/15.
3.3 Effects of extraction methods on extractability, physicochemical, and techno-functional properties of DPEs
3.3.1 Extractability
The current study employed a combining method of alkaline extraction (AE) and UAE, so-called UAAE, to produce the duckweed protein extract (DPE) and compared it with AE and UAWE. Table 4 shows the production yield, protein yields, and protein recovery of DPE produced using AE, UAAE, and UAWE methods. The result shows that UAAE provided the highest production yield of 16.2%, followed by UAWE (11.9%) and AE (7.6%). Similarly, the protein yield and protein recovery were ranked from the highest to the lowest as UAAE, UAWE, and AE. For protein recovery, it indicates the efficiency of the processing to extract protein from raw material and recover it into the protein extracts. Based on the results, UAAE had the highest protein recovery of 34.2%, followed by UAWE with 24.6%, and AE with 17.1%. Comparing AE to UAAE extraction, both production yield and protein recovery of the DPE were improved by 2.1 times.
Besides, to understand how US and AE affect the extractability of duckweed proteins, we compared the protein yield obtained from each extraction method with its production yield. The extraction method exhibited a protein yield-to-production yield ratio close to 1.0, indicating that the technique provided DPE with high protein purity. It was found that the ratio number ranked from highest to lowest corresponded to AE (0.73) to UAAE (0.64) and UAWE (0.62) for the protein yield-to-production-yield ratio. The finding indicated that ultrasonication was crucial in promoting both protein and non-protein extraction, while alkaline treatment was necessary in facilitating protein extraction. Increasing the pH of the suspension undergoing ultrasonication extraction to an alkaline pH might provide a positive synergistic effect on protein extraction. The protein recovery and production yield of Wolffia protein achieved in this study were generally higher than in previous studies (Kingwascharapong et al., 2021; Duangjarus et al., 2022).
3.3.2 Chemical composition and amino acid composition
Chemical composition of DPEs obtained through different extractions, namely AE, UAAE, and UAWE, was investigated. The results presented in Table 5 showed that UAAE and UAWE exhibited lipid content approximately 2.3-fold higher than that obtained from AE. Despite having a lower protein content than DPE obtained via AE, the DPE produced using UAAE and UAWE exhibited significantly higher protein recovery due to the high protein extractability promoted by ultrasonication. These results suggest that ultrasonication was important in releasing proteins and other co-extracted components from the duckweed cells into the extraction aqueous media. This phenomenon was attributed to the high energy intensity of ultrasound waves that generate cavitation force, causing the breaking of cells and releasing various components from the duckweed cells. The high energy and shear force also facilitated the rupture or dissociation of long-chain molecules to shorter-chain molecules, which could subsequently form a complex with protein and/or co-extracted molecules (Kingwascharapong et al., 2021; Sert et al., 2022).
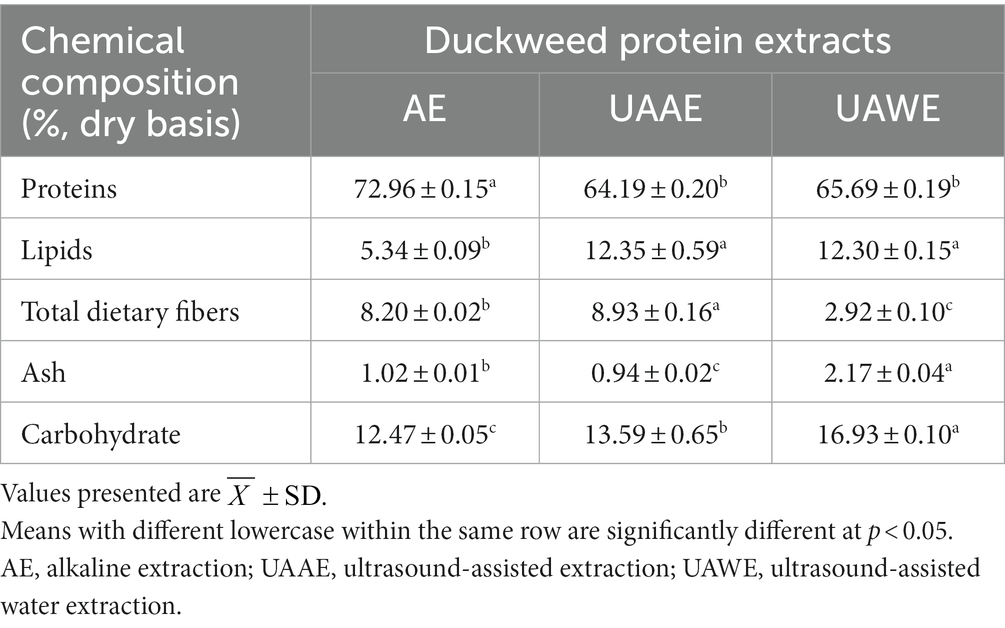
Table 5. Chemical composition of DPE produced using different extraction methods: AE, UAAE, and UAWE.
Furthermore, the results revealed that AE and UAAE contained higher amounts of dietary fiber than UAWE. This was because alkaline could extract dietary fibers from plant cells within a temperature range of 4–85°C (Devi et al., 2023; Liu et al., 2023). This mechanism involves the dissociation of hydrogen and covalent bonds, as well as the ester linkage of cellulose and hemicellulose components by the hydroxyl ion (Maphosa and Jideani, 2016; Tejada-Ortigoza et al., 2016). Therefore, AE and UAAE, which were extracted in an alkali solution, contained higher amounts of dietary fibers than UAWE.
Figure 4A shows the amino acid composition of three different DPEs: AE, UAAE, and UAWE. The five main amino acids (AAs) found in all three DPEs were Glu, Asp., Leu, Arg, and Ala. The amino acid content of the DPEs produced with UAE applied (i.e., UAAE and UAWE) was considerably increased. However, it was observed that sulfur amino acids (SAA) were not detected in UAWE, which was produced without alkaline extraction. In contrast, SAAs were detected in both DPEs produced using AE and UAAE, with a slightly higher amount compared to the raw material. These findings suggest a significant role of alkaline treatment in SAA recovery.
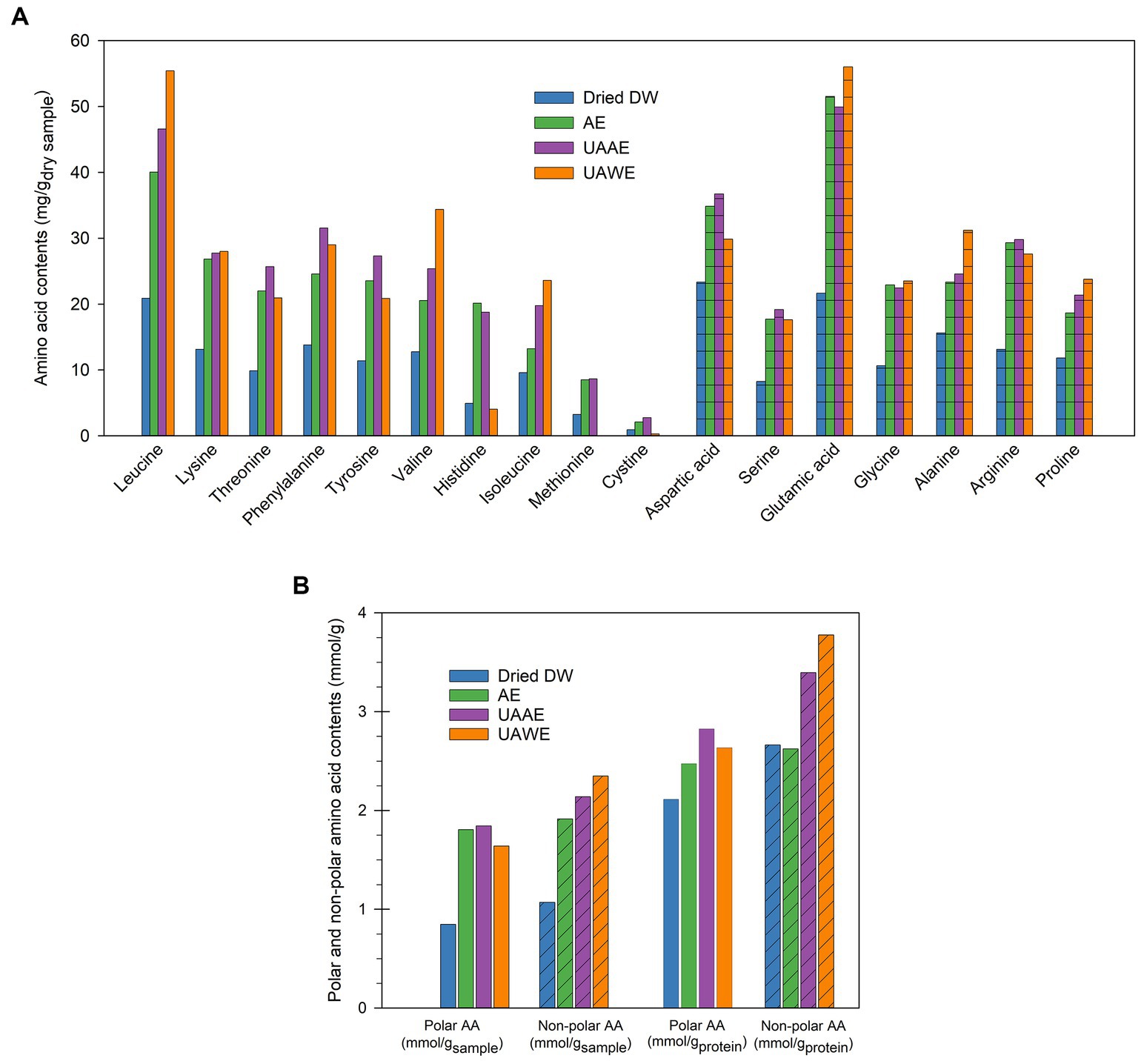
Figure 4. (A) Amino acid composition, and (B) Amount of polar and non-polar amino acids of duckweed protein extracts (DPEs) produced using alkaline extraction (AE), ultrasound-assisted alkaline extraction (UAAE), and ultrasound-assisted water extraction (UAWE) at a duckweed-to-aqueous solution ratio (DSR) of 1/6.
As recommended by FAO (2013), the values of EAAs recommended intake for preschool-aged children for His, Ile, Leu, Lys, Thr, Trp, Val, SAAs, and AAA (aromatic amino acids) are 15, 30, 59, 45, 23, 6, 39, 22, and 38 mg/gprotein, respectively. Notably, the application of UAAE led to an overall enhanced EAA value that meets the requirement. All EAAs of the DPE were even higher than the values recommended for adults.
Furthermore, ultrasonication increased the moles of polar and nonpolar AA by approximately 2.0–2.2 folds, as shown in Figure 4B. AE also enhanced the moles of polar and non-polar amino acids by about 1.8 and 2.2 folds, respectively. The extent and balance between polar and non-polar amino acids played a crucial role in determining the protein solubility, water-, and oil-holding capacity, and emulsifying properties of the protein extracts. The impact was investigated and reported in 3.3.6–3.3.7. This study reported for the first time the role of UAE and AE in promoting the extraction of different profiles of amino acids from duckweed. The new findings provide valuable data for future studies seeking to optimize the extraction of protein from food sources and develop protein extracts with improved nutritional value.
3.3.3 SDS-page
The protein profiles of duckweed powder (raw material) and DPEs produced using AE, UAAE, and UAWE analyzed by SDS-PAGE are shown in Figure 5. The results showed that duckweed powder exhibited more prominent bands at 25, 43, and 50 kDa, while UAWE showed more significant bands at 10, 25, and 50 kDa. On the other hand, UAAE exhibited stronger bands at 25, 50, and 240 kDa with a weaker band at 60 kDa. DPE obtained via AE showed stronger bands at 10, 50, and 240 kDa and a weaker band at 60 kDa. Notably, the band at 25 kDa was not detected in the AE sample.
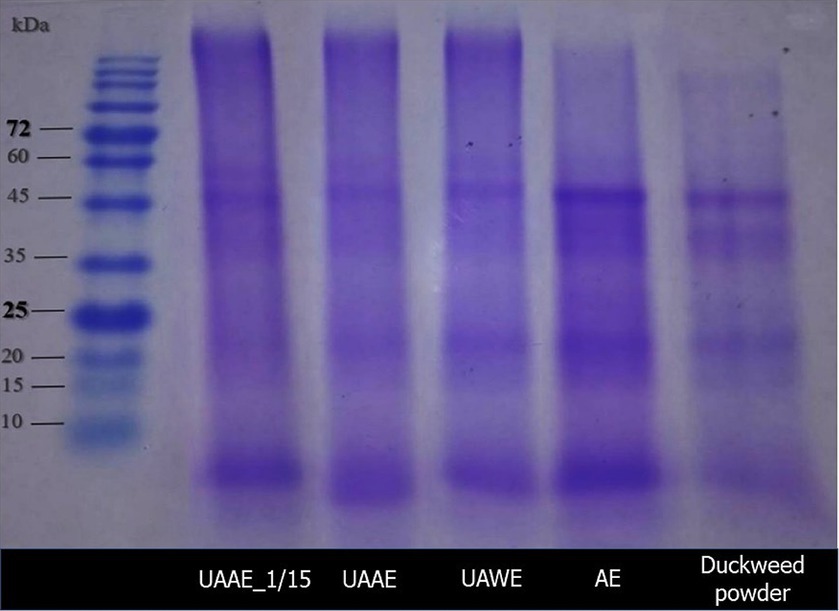
Figure 5. Sodium dodecyl-sulfate-polyacrylamide gel electrophoresis (SDS-PAGE) profile of duckweed powder (raw material) and duckweed protein extracts (DPEs) under reducing conditions.
Compared to UAAE and AE, UAWE exhibited a much more intense band at 50 kDa. However, proteins with Mw of 60 kDa and higher than 240 kDa were not detected. This observation suggested that the alkaline extraction induced changes in protein structure and composition, leading to isomerization and/or cross-linking of the proteins/peptide chains in the subsequent step of acidic precipitation. This was supported by several larger molecules found on the top of the gel in AE and UAAE.
Moreover, it was noticed that the band intensity of small protein molecules (10 kDa) was weaker in the UAAE sample as compared to the AE. This could be attributed to protein degradation caused by the high-intensity ultrasound. The 25-kDa band was detected in all samples produced with UAE applied, indicating that UAE induced protein–protein molecular interaction. These findings are consistent with previous studies (Kingwascharapong et al., 2021; Sert et al., 2022), which reported that ultrasonication induced molecular interactions of proteins-proteins and proteins-other components.
3.3.4 Particle size distribution and zeta potential
The functional properties of proteins in food are significantly influenced by their particle size. The size of protein particles in the bulk phase varies depending on the pH of the solution. Table 6 presents the volume median, Dv(50), or average particle size, of DPEs produced using AE, UAAE, and UAWE. The results showed that the Dv(50) of all three DPEs significantly increased when the pH decreased from 8.5 to 7.0 and 4.0 (p < 0.05). Additionally, it was observed that the use of UAE to produce DPE resulted in a decrease in the DPE’s particle size, regardless of whether alkaline extraction was used or not. The smaller particle size was attributed to the high shear force and cavitation generated by ultrasonication, which broke down protein molecules and other components. These findings were consistent with previous studies on the use of UAE to produce other plant proteins, as reported by Kingwascharapong et al. (2021) and Sengar et al. (2022). The study suggests that using UAE can effectively produce DPE with smaller particle sizes, which may in turn improve functional properties for various food applications. However, further study is needed to determine the optimal UAE conditions and particle size effects on DPE’s functional properties.
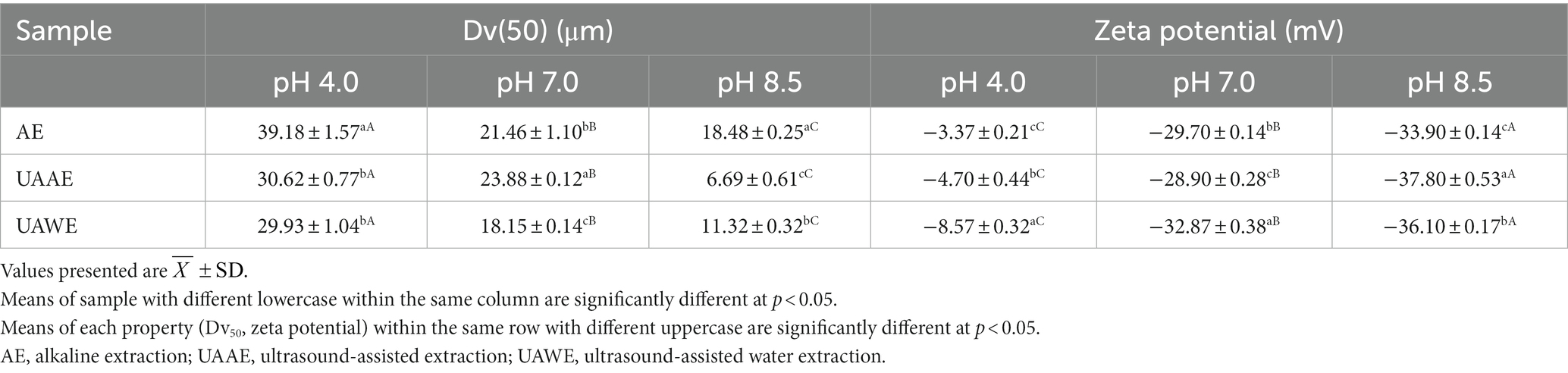
Table 6. Median volume or volume average particle size, Dv(50), at pH 4.0, 7.0 and 8.5 of the differently prepared DPEs: AE, UAAE, and UAWE.
Surface net charge plays a crucial role in determining protein functional properties. The zeta potential (ζ) values of all DPEs were measured at pH 8.5, 7.0, and 4.0 and are presented in Table 6 The zeta potential of three different DPEs in aqueous solutions at three different pH levels (8.5, 7.0, and 4.0) were all negative and significantly different from each other (p < 0.05). As the pH decreased from 8.5 to 4.0, the negative values of the DPEs decreased This could be attributed to the protonation of the carboxyl and amino groups of the protein, caused by shifting pH from alkaline to acidic values (Ladjal-Ettoumi et al., 2015).
The DPE solution with higher absolute zeta potential values suggested the greater electrostatic repulsion of the molecules, which tended to have less aggregation, thereby exhibiting smaller particles as previously reported. The higher absolute zeta potential values observed in the DPE solution suggested greater electrostatic repulsion among the molecules. This, in turn, led to reduced aggregation and resulted in smaller particles, as previously reported. The result was consistent with many studies that applied ultrasonication to other types of protein extraction, which found that UAE played an important role in particle size reduction and surface charge property enhancement of the protein extracts (Li et al., 2020; Sengar et al., 2022; Liu et al., 2023; Rao et al., 2023).
3.3.5 Protein solubility
Figure 6 shows the solubility of DPEs produced using AE, UAAE, and UAWE. The solubility of proteins was observed to be at its minimum when the pH was around 4, and it increased as the pH either decreased or increased. The pH is known as the isoelectric point (pI), where a protein’s net charge becomes zero.
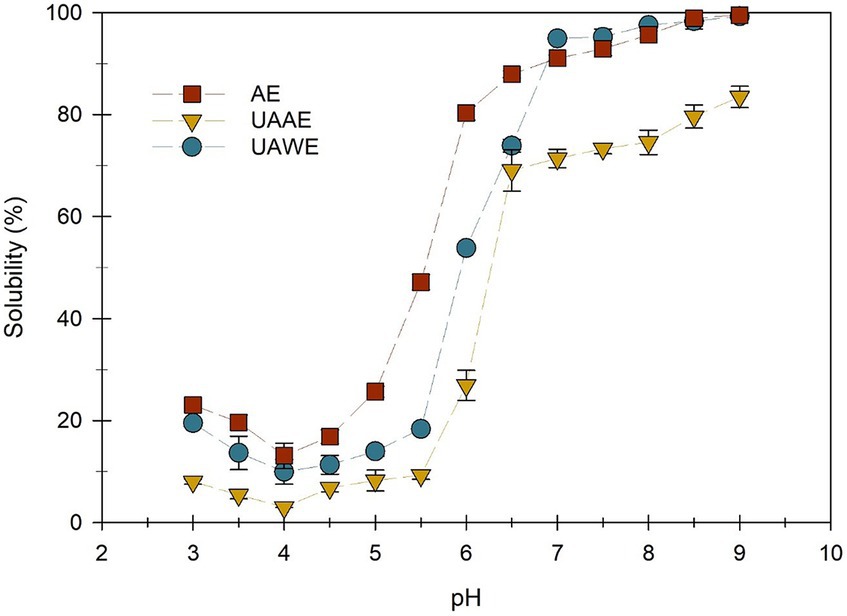
Figure 6. Solubility of duckweed protein extracts (DPEs) measured as a function of pH. The DPEs were produced using alkaline extraction (AE, −■-), ultrasound-assisted alkaline extraction (UAAE, −▼-), and ultrasound-assisted water extraction (UAWE, -●-) at a duckweed-to-solvent ratio of 1/6.
The result of this study was consistent with the existing report on the relationship between pH and protein solubility. According to Anoop et al. (2023), leaf proteins typically have a pI between 4.0 and 6.0. The pI of all DPEs examined in this study was found to be approximately 4.0. When the pH deviated from the pI value, the solubility of these proteins significantly increased. This can be explained by the increase in the net charge on the protein surface, which results in stronger repulsive electrostatic forces between protein molecules and their interaction with water.
The solubility of UAAE was significantly lower than AE. This could be because proteins were aggregated and/or denatured during the high-intensity ultrasonication. Additionally, the solubility of DPE produced via UAAE was lower than that prepared by UAWE in both acidic and alkaline conditions. Furthermore, it was noteworthy that the poorer solubility could be attributed to the high amount of dietary fibers co-extracted with the proteins during the alkaline extraction, as indicated by the higher dietary fiber content in UAAE sample (Table 5). According to Turck et al. (2021), more than 90% of dietary fibers found in duckweed are water-insoluble, which could account for the lower solubility of the DPE produced via the UAAE approach. The solubility of protein is a critical factor in maintaining the stability of emulsions, as higher solubility allows proteins to effectively dissociate and migrate to the water/oil interface and provide stabilization for oil droplets (McClements et al., 2022). However, extracts with a high proportion of dietary fibers can help stabilize the emulsion system by increasing aqueous phase viscosity. A similar finding was observed in the study of He et al. (2020).
3.3.6 Emulsifying properties
The emulsifying capacity and stability of protein indicate the protein’s ability to form and stabilize emulsion, respectively. The former capacity involves the ability of the protein molecules to rapidly adsorb on the oil droplet surfaces upon homogenization. At the same time, the latter property is associated with the protein’s ability to prevent or retard the oil droplet aggregation after forming the emulsion (McClements et al., 2022). Emulsion-based foods need both properties but with different balances between the two properties depending on the type of food. The effects of the ultrasound and alkaline treatments on emulsifying properties were investigated. The emulsifying properties, including emulsifying capacity (EC) and emulsifying stability (ES) of the resulting DPEs, are presented in Table 7. Additionally, the appearance of all emulsion samples after storing for 1 and 24 h is presented in Supplementary Figure S1.
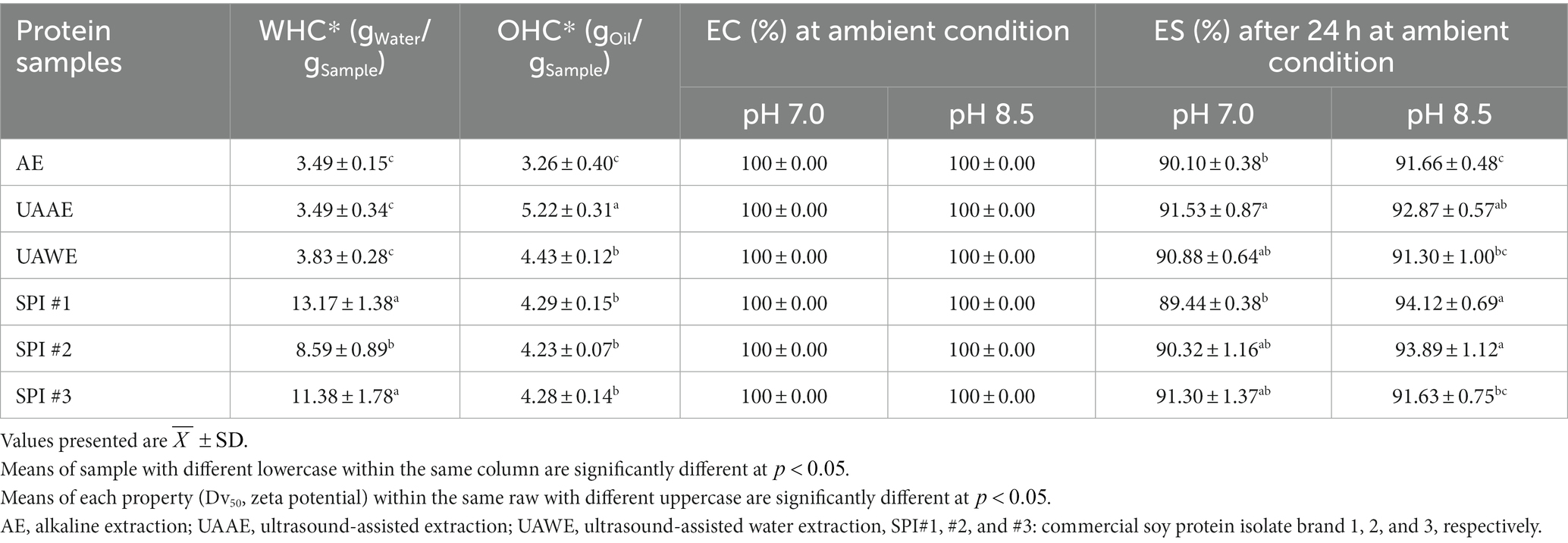
Table 7. Water holding capacity (WHC), oil holding capacity (OHC), emulsifying capacity (EC), and emulsifying stability (ES) of differently prepared DPEs: AE, UAAE, and UAWE, and 2 commercial soy protein isolates (SPI).
All three DPEs demonstrated 100% emulsifying capacity (EC) at pH 7.0 and 8.5, with emulsion stability (ES) of 90–92% after 24 h. While the protein content of UAAE and UAWE was lower than AE, their emulsifying properties were not significantly different ( ). Moreover, the protein solubility of UAAE was lower than the other two DPEs. Despite these differences, the emulsifying properties of all three DPEs showed no significant difference ( ). According to the study of McClements et al. (2022), smaller protein particles tend to migrate faster towards the oil–water interface, which promotes them to effectively stabilize the oil droplets. This is likely the reason for the comparatively high EC of the UAAE, as the protein particles in the sample were smaller, and therefore more effective at stabilizing the emulsion though the UAAE sample had lower protein content than the AE sample.
The emulsifying capacity (EC) of all three DPEs was 100% at pH 7.0 and 8.5, with emulsion stability (ES) ranging between 90 and 92% after 24 h. Although the protein content of DPE produced by UAAE and UAWE was lower than that obtained via AE, their emulsifying properties were not significantly different ( ). Furthermore, the protein solubility of DPEs produced using UAAE was lower than the other two DPEs. Despite these variations, all three DPEs showed no significant difference in their emulsifying properties ( ). As previously mentioned, DPE produced via UAAE had smaller particles at pH 8.5. Smaller protein particles typically exhibit better emulsifying capacity and stability than larger particles, as they migrate quickly to the oil–water interface and effectively stabilize the oil droplet. This could contribute to the good emulsifying property of the DPE produced using UAAE.
The ES at pH 7.0 of DPE produced via UAAE and UAWE were 91.53 and 90.88%, respectively, whereas the ES at pH 8.5 of the UAAE and UAWE were 92.87 and 91.3%, respectively. The ES of three commercial SPIs at pH 7.0 was 90.32–91.30%, and at pH 8.5 was 91.63–94.12%. The ES of UAAE and UAWE were comparable to the commercial SPIs (p ≥ 0.05). However, DPE produced via AE exhibited a lower ES than the commercial SPI at pH 7.0 and 8.5 (p < 0.05). It is worth noting that despite having lower protein content than SPI, all DPEs produced via UAE had comparable emulsifying properties to SPI. This finding implied that the emulsifying properties of the DPEs were not attributed to only the protein content. However, other components like carbohydrates and dietary fibers in the DPEs also co-contributed to improve emulsifying properties. Typically, dietary fibers and carbohydrates added into the emulsion system have been noted to play a role in emulsion capacity and stability improvement. These are generated via Pickering emulsion formation and enhance the continuous phase’s viscosity to retard the oil droplet aggregation, respectively. The DPE produced using ultrasonication proved to have excellent potential for emulsion application and exhibited emulsifying properties comparable to commercial SPI.
3.3.7 Water holding capacity and oil holding capacity
WHC and OHC are terms used to describe the ability of solid or semi-solid materials to retain water and oil in the sample under the influence of external forces, pressure, or heat applied. WHC plays a crucial role in determining the juiciness of foods, and it also impacts weight loss and cooking yield in food processes. As shown in Table 7, the WHC of three DPEs (AE, UAAE, and UAWE) was similar, ranging from approximately 3.5–3.8 gWater/gSample. It is important to note that the testing method and conditions can significantly affect WHC values, so comparisons with other studies must be made with caution.
An article reviewed by Ma et al. (2022) presented experimental data on the WHC of various plant protein extracts. Their results were obtained through an experimental set comparable to ours. The report showed that depending on the type of protein extract, WHC ranged from 0.8–1.0, 1.3–4.9, and 1.1–7.9 gWater/gSample for protein flour, protein concentrates, and protein isolates, respectively. Protein contents ranged from 23–27%, 63–77%, and 81–98% dry mass. All DPEs had WHC values comparable to most plant protein concentrates and many protein isolates. The DPE produced through UAAE and UAWE had even higher WHC than other protein concentrates.
Regarding OHC, Table 7 shows that DPEs produced through UAAE exhibited slightly higher OHC than the other two DPEs and three commercial soy protein isolates (SPI#1, #2, and #3). The OHC values (gOil/gSample) of DPEs were found to be significantly different (p < 0.05) and ranked from highest to lowest as UAAE (5.2) > UAWE (4.4) > AE (3.3). The three commercial SPIs had OHC values of about 4.2–4.3 gOil/gSample. OHC of UAWE was not significantly different from that of three commercial SPI. Typically, proteins’ hydrophilic and polar amino acid residues enhance their water-holding ability. On the other hand, proteins with high levels of hydrophobic amino acid residues tend to have good OHC (Tang et al., 2021; Ma et al., 2022). As previously reported, the DPE produced through UAAE and UAWE contained high levels of non-polar amino acids. Moreover, dietary fibers are known to improve WHC and OHC (Mehta et al., 2013; Zhang et al., 2020). As previously mentioned, AE resulted in the DPE with high dietary fiber. An analysis of the correlation among the content of dietary fibers, the amount of polar and non-polar amino acids, and water- and oil-holding capacity was conducted and is shown in Supplementary Figure S2. The results strongly suggest that the good OHC of the DPE produced via UAAE in this work was not solely due to its protein content. The amounts of non-polar amino acids and dietary fiber contents also played important roles in promoting OHC.
This finding is consistent with the reports of Miravalles et al. (2019)and Ma et al. (2022), which stated that the WHC and OHC of protein extracts are not always determined by their protein content alone. They are also influenced by the proportion and extent of hydrophilic and hydrophobic amino acid residues on the protein surface and other co-extracted components. The DPE produced using UAAE had a high ratio of non-polar amino acids, total amino acids, and dietary fiber content. As a result, it exhibited the highest OHC compared to the other two DPEs.
The limited techno-functional properties of most plant proteins, such as poor solubility and emulsifying properties, have been reported as factors that can restrict their use in food products (Colgrave et al., 2021). However, this study demonstrated that the DPE produced via UAAE shows promise as a possible alternative for food product applications such as bakeries, emulsion-based foods, and beverages.
4 Conclusion
The study demonstrated the production of techno-functional DPE using a combined method of UAE and AE, known as UAAE. The study found that UAE was effective in extracting non-polar or hydrophobic amino acids, while AE was effective in extracting sulfur-containing amino acids. The resulting DPE had an excellent essential amino acid content, meeting WHO/FAO/UNU’s protein recommendations for preschool-aged children. Additionally, AE also enhanced dietary fiber extraction, which helped stabilize emulsion. The DPE produced using UAAE had good emulsifying properties and OHC, comparable to commercial SPIs. The findings also revealed that protein recovery and production yields for the DPE produced from dried duckweed in powdery form were almost the same as those obtained from fresh duckweed in granular form, providing significant data applicable to industrial production. The DPE produced using UAAE proved to have excellent potential for protein-rich food, beverage, and feed applications. The ongoing study is currently investigating the gastrointestinal digestibility of DPE and the potential bioactivities of the digested peptides. The results will be presented in the near future. Moreover, we are also focusing on improving the DPE’s greenish appearance and grassy flavor and developing zero-discharge production processes.
Data availability statement
The original contributions presented in the study are included in the article/Supplementary material, further inquiries can be directed to the corresponding author.
Author contributions
CN: Investigation, Writing – review & editing. SW: Conceptualization, Formal analysis, Project administration, Writing – original draft, Writing – review & editing, Validation. PV: Writing – review & editing. JY: Funding acquisition, Writing – review & editing.
Funding
The author(s) declare financial support was received for the research, authorship, and/or publication of this article. This research was fully funded by the National Research Council of Thailand (NRCT) under Project number N42A65050548. The article publishing charges (APCs) is co-funded by King Mongkut’s University of Technology Thonburi.
Acknowledgments
The authors extend their sincere gratitude to Miss Supanooch Poungchawanwong for her assistance with the preliminary trial on Wolffia protein preparation during the early stage of the project.
Conflict of interest
The authors declare that the research was conducted in the absence of any commercial or financial relationships that could be construed as a potential conflict of interest.
Publisher’s note
All claims expressed in this article are solely those of the authors and do not necessarily represent those of their affiliated organizations, or those of the publisher, the editors and the reviewers. Any product that may be evaluated in this article, or claim that may be made by its manufacturer, is not guaranteed or endorsed by the publisher.
Supplementary material
The Supplementary material for this article can be found online at: https://www.frontiersin.org/articles/10.3389/fsufs.2024.1343615/full#supplementary-material
References
Anoop, A. A., Pillai, P. K. S., Nickerson, M., and Ragavan, K. V. (2023). Plant leaf proteins for food applications: opportunities and challenges. Compr. Rev. Food Sci. Food Saf. 22, 473–501. doi: 10.1111/1541-4337.13079
Appenroth, K.-J., Sree, K. S., Bog, M., Ecker, J., Seeliger, C., Böhm, V., et al. (2018). Nutritional value of the duckweed species of the genus Wolffia (Lemnaceae) as human food. Front. Chem. 6, 1–13. doi: 10.3389/fchem.2018.00483
Appenroth, K.-J., Sree, K. S., Böhm, V., Hammann, S., Vetter, W., Leiterer, M., et al. (2017). Nutritional value of duckweeds (Lemnaceae) as human food. Food Chem. 217, 266–273. doi: 10.1016/j.foodchem.2016.08.116
Bello, I., Adeniyi, A., Mukaila, T., and Hammed, A. (2023). Optimization of soybean protein extraction with ammonium hydroxide (NH4OH) using response surface methodology. Foods 3, 1515. doi: 10.3390/foods12071515
Cui, L., Bandillo, N., Wang, Y., Ohm, J. B., Chen, B., and Rao, J. (2020). Functionality and structure of yellow pea protein isolate as affected by cultivars and extraction pH, Food Hydrocoll. 108, 106008. doi: 10.1016/j.foodhyd.2020.106008
Colgrave, M. L., Dominik, S., Tobin, A. B., Stockmann, R., Simon, C., Howitt, C. A., et al. (2021). Perspectives on future protein production. J. Agric. Food Chem. 69, 15076–15083. doi: 10.1021/acs.jafc.1c05989
Deng, Y., Wang, W., Zhao, S., Yang, X., Xu, W., Guo, M., et al. (2022). Ultrasound-assisted extraction of lipids as food components: mechanism, solvent, feedstock, quality evaluation and coupled technologies – a review. Trends Food Sci. Technol. 122, 83–96. doi: 10.1016/j.tifs.2022.01.034
Devi, S. R., Kumari, T., and Deka, S. C. (2023). Extraction of dietary fiber and phytochemicals from bottle gourd seeds (Lagenaria siceraria), its physicochemical properties and application in food model. Food Chem Adv. 2, 100252–100258. doi: 10.1016/j.focha.2023.100252
Duangjarus, N., Chaiworapuek, W., Rachtanapun, C., Ritthiruangdej, P., and Charoensiddhi, S. (2022). Antimicrobial and functional properties of duckweed (Wolffia globosa) protein and peptide extracts prepared by ultrasound-assisted extraction. Food Secur. 11:2348. doi: 10.3390/foods11152348
EFSA (2021). Technical report on the notification of fresh plants of Wolffia arrhiza and Wolffia globosa as a traditional food from a third country pursuant to article 14 of regulation (EU) 2015/2283. EFSA Support. Pub. 18:EN-6658. doi: 10.2903/sp.efsa.2021.EN-6658
FAO. (2013). Dietary Protein Quality Evaluation in Human Nutrition: Report of an FAO Expert Consultation. Rome, Italy: FAO Food and nutrition paper.
Grossmann, L., Ebert, S., Hinrichs, J., and Weiss, J. (2019). Formation and stability of emulsions prepared with a water-soluble extract from the microalga Chlorella protothecoides. J. Agric. Food Chem. 67, 6551–6558. doi: 10.1021/acs.jafc.8b05337
He, K., Zhang, X., Li, Y., Li, B., and Liu, S. (2020). Water-insoluble dietary-fibers from Flammulina velutiper used as edible stabilizers for oil-in-water Pickering emulsions. Food Hydrocoll. 101, 105519–105518. doi: 10.1016/j.foodhyd.2019.105519
Inguanez, L., Zhu, X., Mallia, J. O., Tiwari, B. K., and Valdramidis, V. P. (2023). Extractions of protein-rich Alaria esculenta and Lemna minor by the use of high-power (assisted) ultrasound. Sustain. For. 15:8024. doi: 10.3390/su15108024
Kingwascharapong, P., Chaijan, M., and Karnjanapratum, S. (2021). Ultrasound-assisted extraction of protein from Bombay locusts and its impact on functional and antioxidative properties. Sci. Rep. 11:17320. doi: 10.1038/s41598-021-96694-w
Ladjal-Ettoumi, Y., Boudries, H., Chibane, M., and Romero, A. (2015). Pea, chickpea and lentil protein isolates: physicochemical characterization and emulsifying properties. Food Biophys. 11, 43–51. doi: 10.1007/s11483-015-9411-6
Li, Y., Cheng, Y., Zhang, Z., Wang, Y., Mintah, B. K., Dabbour, M., et al. (2020). Modification of rapeseed protein by ultrasound-assisted pH shift treatment: ultrasonic mode and frequency screening, changes in protein solubility and structural characteristics. Ultrason. Sonochem. 69:105240. doi: 10.1016/j.ultsonch.2020.105240
Liao, J., Xue, H., and Li, J. (2022). Extraction of phenolics and anthocyanins from purple eggplant peels by multi-frequency ultrasound: effects of different extraction factors and optimization using uniform design. Ultrason. Sonochem. 90:106174. doi: 10.1016/j.ultsonch.2022.106174
Liu, S., Xie, Y., Li, B., Li, S., Yu, W., Ye, A., et al. (2023). Structural properties of quinoa protein isolate: impact of neutral to high alkaline extraction pH. Food Secur. 12:2589. doi: 10.3390/foods12132589
Ma, K. K., Grossmann, L., Nolden, A. A., McClements, D. J., and Kinchla, A. J. (2022). Functional and physical properties of commercial pulse proteins compared to soy derived protein. Future Foods 6:100155. doi: 10.1016/j.fufo.2022.100155
Maphosa, Y., and Jideani, V. A. (2016). Dietary fiber extraction for human nutrition-a review. Food Rev. Int. 32, 98–115. doi: 10.1080/87559129.2015.1057840
McClements, D. J., Lu, J., and Grossmann, L. (2022). Proposed methods for testing and comparing the emulsifying properties of proteins from animal, plant, and alternative sources. Coll. Interf. 6:20019. doi: 10.3390/colloids6020019
Mehta, N., Ahlawat, S. S., Sharma, D. P., and Dabur, R. S. (2013). Novel trends in development of dietary fiber rich meat products-a critical review. J Food Sci Technol. 52, 633–647. doi: 10.1007/s13197-013-1010-2
Miravalles, L. A., Jeske, S., Bez, J., Detzel, A., Busch, M., Krueger, M., et al. (2019). Membrane filtration and isoelectric precipitation technological approaches for the preparation of novel, functional and sustainable protein isolate from lentils. Eur. Food Res. Technol. 245, 1855–1869. doi: 10.1007/s00217-019-03296-y
Moldoveanu, S. C. (2005). Analysis of protein amino acids in tobacco using microwave digestion of plant material. Beitr. Tab. Int. Contrib. 21, 451–465. doi: 10.2478/cttr-2013-0813
Nguyen, T. K., Mondor, M., and Ratti, C. (2018). Shrinkage of cellular food during air drying. J. Food Eng. 230, 8–17. doi: 10.1016/j.jfoodeng.2018.02.017
Nieuwland, M., Geerdink, P., Engelen-Smit, N. P. E., Van der Meer, I. M., America, A. H. P., Mes, J. J., et al. (2021). Isolation and gelling properties of duckweed protein concentrate. ACS Food Sci. Technol. 1, 908–916. doi: 10.1021/acsfoodscitech.1c00009
Pagliuso, D., Grandis, A., Fortirer, J. S., Camargo, P., Floh, E. I. S., and Buckeridge, M. S. (2022). Duckweeds as promising food feedstocks globally. Agronomy 12:796. doi: 10.3390/agronomy12040796
Patist, A., and Bates, D. (2008). Ultrasonic innovations in the food industry: from the laboratory to commercial production. Innov. Food Sci. Emerg. Technol. 9, 147–154. doi: 10.1016/j.ifset.2007.07.004
Potin, F., Lubbers, S., Husson, F., and Saurel, R. (2019). Protein extraction conditions affect extraction yield and protein quality. J. Food Sci. 84, 3682–3690. doi: 10.1111/1750-3841.14850
Prosridee, K., Oonsivilai, R., Tira-aumphon, A., Singthong, J., Oonmetta-aree, J., and Oonsivilai, A. (2023). OPtimum aquaculture and drying conditions for Wolffia arrhiza (L.) Wimn. Heliyon. 9:e19730. doi: 10.1016/j.heliyon.2023.e19730
Raja, K., Kadirvel, V., and Subramaniyan, T. (2022). Seaweeds, an aquatic plant-based protein for sustainable nutrition-a review. Future Foods 5:100142. doi: 10.1016/j.fufo.2022.100142
Rao, M. V., Sunil, C. K., Rawson, A., Chidanand, D. V., and Venkatachlapathy, N. (2023). Modifying the plant proteins techno-functionalities by novel physical processing technologies: a review. Crit. Rev. Food Sci. Nutr. 63, 4070–4091. doi: 10.1080/10408398.2021.1997907
Ruekaewma, N., Piyatiratitivorakul, S., and Powtongsook, S. (2015). Culture system for Wolffia globosa L. (Lemnaceae) for hygiene human food. Songkhlanakharin J. Sci. Technol. 37, 575–580.
Sengar, A. S., Thirunavookarasu, N., Choudhary, P., Naik, M., Surekha, A., Sunil, C. K., et al. (2022). Application of power ultrasound for plant protein extraction modification and allergen reduction. A review. Appl. Food Res. 2, 100–219. doi: 10.1016/j.afres.2022.100219
Sert, D., Rohm, H., and Struck, S. (2022). Ultrasound-assisted extraction of protein from pumpkin seed press cake: impact on protein yield and techno-functionality. Food Secur. 11:4029. doi: 10.3390/foods11244029
Shen, L., Pang, S., Zhong, M., Sun, Y., Qayum, A., and Liu, Y. (2023). A comprehensive review of ultrasonic assisted extraction (UAE) for bioactive components: principles, advantages, equipment, and combined technologies. Ultrason. Sonochem. 101, 1–24. doi: 10.1016/j.ultsonch.2023.106646
Stevanato, N., and da Silva, C. (2019). Radish seed oil: ultrasound-assisted extraction using ethanol as solvent and assessment of its potential for ester production. Ind. Crop. Prod. 132, 283–291. doi: 10.1016/j.indcrop.2019.02.032
Stone, A. K., Karalash, A., Tyler, R. T., Warkentin, T., and Nickerson, M. T. (2015). Functional attributes of pea protein isolates prepared using different extraction methods and cultivars. Food Res. Int. 76, 31–38. doi: 10.1016/j.foodres.2014.11.017
Sun, J., Zhang, Z., Xiao, F., Wei, Q., and Jing, Z. (2018). Ultrasound-assisted alkali extraction of insoluble dietary fiber from soybean residues. IOP Conf. Ser. Mater. Sci. Eng. 392:52005. doi: 10.1088/1757-899X/392/5/052005
Tang, X., Shen, Y., Zhang, Y., Schilling, M. W., and Li, Y. (2021). Parallel comparison of functional and physicochemical properties of common pulse proteins. LWT 146:111594. doi: 10.1016/j.lwt.2021.111594
Tang, L., and Yongsawatdigul, J. (2020). Physicochemical properties of tilapia (Oreochromis niloticus) actomyosin subjected to high intensity ultrasound in low NaCl concentrations. Ultrason. Sonochem. 63:104922. doi: 10.1016/j.ultsonch.2019.104922
Tejada-Ortigoza, V., Garcia-Amezquita, L. E., Serna-Saldívar, S. O., and Welti-Chanes, J. (2016). Advances in the functional characterization and extraction processes of dietary fiber. Food Eng. Rev. 8, 251–271. doi: 10.1007/s12393-015-9134-y
Turck, D., Bohn, T., Castenmiller, J., De Henauw, S., Hirsch-Ernst, K. I., Maciuk, A., et al. (2021). Safety of the extension of use of 2′-fucosyllactose (2'-FL) as a novel food pursuant to regulation (EU) 2015/2283. EFSA J. 21, 1–13. doi: 10.2903/j.efsa.2023.8334
Yang, G. L., Feng, D., Liu, Y. T., Lv, S. M., Zheng, M. M., and Tan, A. J. (2020). Research progress of a potential bioreactor: duckweed. Biomol. Ther. 11, 1–14. doi: 10.3390/biom11010093
Yao, S., Mettu, S., Law, S. Q. K., Ashokkumar, M., and Martin, G. J. O. (2018). The effect of high-intensity ultrasound on cell disruption and lipid extraction from high solids viscous slurries of nannochloropsis sp. biomass. Algal Res. 35, 341–348. doi: 10.1016/j.algal.2018.09.004
Keywords: alternative proteins, duckweed, pH shift, protein extraction, ultrasound-assisted extraction
Citation: Nitiwuttithorn C, Wongsasulak S, Vongsawasdi P and Yongsawatdigul J (2024) Effects of alkaline and ultrasonication on duckweed (Wolffia arrhiza) protein extracts’ physicochemical and techno-functional properties. Front. Sustain. Food Syst. 8:1343615. doi: 10.3389/fsufs.2024.1343615
Edited by:
B. N. Dar, Islamic University of Science and Technology, IndiaReviewed by:
Md. Anisur Mazumder, Bangladesh Agricultural University, BangladeshSajad Ahmad Wani, Islamic University of Science and Technology, India
Copyright © 2024 Nitiwuttithorn, Wongsasulak, Vongsawasdi and Yongsawatdigul. This is an open-access article distributed under the terms of the Creative Commons Attribution License (CC BY). The use, distribution or reproduction in other forums is permitted, provided the original author(s) and the copyright owner(s) are credited and that the original publication in this journal is cited, in accordance with accepted academic practice. No use, distribution or reproduction is permitted which does not comply with these terms.
*Correspondence: Saowakon Wongsasulak, c2Fvd2Frb24ud29uQGttdXR0LmFjLnRo