- 1School of Public Health, Jilin Medical University, Jilin, China
- 2Institute of Food Science and Nutrition, University of Sargodha, Sargodha, Pakistan
- 3Louisiana State University Health Sciences Center, New Orleans, LA, United States
This study aimed to characterize dietary fibers (DF) produced from papaya peel (PP) and seed (PS) using three different extraction methods (acidic: AC, enzymatic: EN and alkaline: AL). The scanning electron microscopy (SEM), Fourier transform infrared spectroscopy (FT-IR), thermal and rheological properties, X-ray diffraction (XRD), monosaccharide composition were adopted for characterizing DF samples. All the DF samples showed representative infrared spectral features and crystalline structure, whereas DF derived from PP and PS extracted by AC had looser and more complicated structures. DF derived from PP and PS extracted by EN displayed greater thermal stability among DFs. DF extracted by PP-AC and PS-EN showed a high degree of linearity in rheograms as compared to other DF samples. In addition, the extraction method also influenced the monosaccharide composition in PP and PS DFs samples. Furthermore, AC-extracted DFs, specifically PP-AC and PS-AC exhibited higher functional (water-holding capacity (WHC), oil-holding capacity (OHC), water swelling capacity (WSC), cholesterol-absorption capacity (CAC), bile acid absorption capacity (BAC), nitrite ion absorption capacity (NIAC), glucose absorption capacity (GAC)) and antioxidant properties (DPPH, ABTS and FRAP). Our results suggest that the PP and PS derived DF samples could potentially be used as functional food ingredient.
1 Introduction
Due to the nutritional advantages of tropical fruits consumption for overall human health, it has garnered increasing attention globally (Ameer et al., 2017). The papaya (Carica papaya L.), a tropical evergreen fruit tree that originated in Central America and Mexico, is well known. It is important to note that different fruit components such as pulp, seeds, and skin are used at various ripening stages. The preparation of papaya fruit produces large amounts of waste in the form of papaya peel (PP) and papaya seeds (PS). These enormous papaya waste streams, which typically harm ecosystems, could be used for product development and valorization (Feng et al., 2022; Jiang et al., 2022a). Recently, studies have shown that papaya fruit and its by-products (leaves, roots and stems) exhibit several health benefits. As a result they have the potential for various applications in formulations of diuretic medications, emmenagogue, anthelmintics, antidyseptic, stomachic, vermifuge, laxative, antirheumatic, antiasthmatic, tonic, rubefacient, poultice as well as anti-cancer herbal remedy and cure for inflated spleen, liver and freckles (Leitão et al., 2022). PS are frequently ground into powder, which is then used in a variety of food products, including sweet smoothies, desserts, juices, and tea infusions.
According to epidemiological research, studies on humans, animals, and other organisms have shown that dietary fiber (DF), the seventh essential nutrient, possess unique biological properties (Jiang et al., 2020, 2023). For example, soluble dietary fiber (SDF) plays a critical role in increasing viscosity, reducing blood sugar, and increasing plasma cholesterol, whereas insoluble dietary fiber (IDF) increases stool volume and promotes defecation (Jiang et al., 2021). However, there is no published report available on the composition and functional properties of DF isolated from PP and PS.
Currently, DF is extracted from a variety of sources using enzymatic, chemical, enzymatic-chemical, ultrasound-assisted, and microwave-assisted processes. The various processing conditions could alter the structural and functional characteristics of DF, which would then affect their physicochemical and functional activities (Jiang et al., 2021).
Different extraction procedures, enzymatic (EN) extraction, acidic (AC) extraction, and alkaline (AL) extraction methods were applied to extract DF from PP and PS. Furthermore, proximate composition, hydration, structural and functional characteristics, and antioxidant activities of PP and PS-derived DF by various extraction methods were compared. This study could provide insights to investigations on PP and PS derived DF and offer a theoretical basis for the deep processing of papaya fruit and the development of functional food additives.
2 Materials and methods
2.1 Materials and chemicals
Fresh papaya fruits were procured from a local supermarket located in Jilin, China. The supplied enzymes, such as protease and heat-stable α-amylase were purchased from Sigma Aldrich (St. Louis, United States). All chemicals and reagents used in this study were of analytical grade.
2.2 DF extraction from PP and PS
2.2.1 Preliminary treatment of papaya
The fresh papaya fruits were washed with cold water. The washed fruits were subjected to peeling followed by removal of peel and seed from papaya fruits. The isolated peel and seeds were then subjected to oven drying at 50°C. Next, PP and PS were milled using a lab-scale dry grinder (JYL-C020E, Joyoung Comp. Ltd., Jinan, China) for 1 min time interval. Then, both PP and PS powders were sifted by passing through 60-mesh sieve to obtain particles of uniform size of less than 500 μm. The finely milled powders of PP and PS were sealed in aluminum-laminated bags and kept at −20°C until further use.
2.2.2 Enzymatic (EN) extraction method
The EN extraction method for DF extraction from PP and PS was employed according to the reported methods of Kurek et al. (2018). Briefly, 10 grams of each PP and PS were gently mixed with 80 mL of phosphate buffer (20 mM, pH 6.8). Then, 1% of a-amylase (heat stable) was added to the reaction mixture, followed by stirring to create a suspension. Each PP and PS sample was incubated in shaking water bath at 75°C until a negative iodine test was achieved. The negative iodine test usually takes approximately 30 min. Then, cooling of the suspension was carried out at 60°C followed by pH adjustment up to 7.5 by addition of phosphate buffer (50 mL:20 mM). Protein digestion was then achieved by mixing protease (200 μL) with the suspension and the reaction mixture was allowed to stand for a period of 30 min in shaking water bath at 60°C. After performing the centrifugation at 5,000×g for 15 min, the residues were extracted followed by two rinses with distilled water and then oven-dried at 50°C to obtain the IDF extracted by PP-EN and PS-EN. Simultaneously, after collecting the supernatants, they were mixed with 95% ethanol (four-fold volumes and incubated for 2 h at ambient room temperature (25°C) for residues collection. Then, drying was performed in the fume hood for obtaining SDF from PP-EN and PS-EN. Prior to further analysis, IDF and SDF extracts were mixed together. The extraction yield of DF from PP-EN and PS-EN fractions were calculated based on the following formula given below in Eq. 1:
In aforementioned formula, the C denotes the TDF weight of PP-EN and PS-EN whereas, W denotes the weight of PP and PS powder.
2.2.3 Acid (AC) extraction method
Citric acid in specified amount (1% w/v) was employed for the extraction of acid-extracted fraction of DF from PS and PP according to the reported method of Yuliarti et al. (2015). For AC extraction, the PP and PS powders were mixed with citric acid in a ratio of 1:40 (w/v) and subjected to extraction of 2 h on a water bath at 40°C. Later, the PP-AC and PS-AC fractions were obtained as per the treatment described in Section 2.2.2. The extraction yield calculation from acidic fractions of PS and PP was carried out using the Eq. 1 given.
2.2.4 Alkali (AL) extraction method
The AL-extracted DF was extracted by employing 5% NaOH (w/v) solution as per the reported method of Wang et al. (2021) after certain modification. Briefly, NaOH solution in an amount of 400 mL was added to 10.0 g of PP and PS and the resultant solution mixture was subjected to 2 h extraction on water bath at 40°C followed by centrifugation at 5,000×g for 15 min. Then, the supernatants and residues were collected and the obtained DF was calculated according to the treatment described in Section 2.2.2. The extraction yield (%) was determined as per Eq. 1.
2.3 Proximate composition
The proximate parameters, such as protein, fat and ash content were calculated according to the AACC (2000) methods. In case of protein analysis, nitrogen content thus obtained was multiplied by a protein conversion factor of 6.25 in order to get crude protein content as given in Eq. 2.
2.4 Scanning electron microscopy (SEM)
Assessment of the effects on morphology and microstructure of DF extracted by different extraction methods was conducted by scanning electron microscope (SEM: SU8010, Hitachi, Tokyo, Japan). Specifically, the DFs from each extraction method were fixed on the specimen holder and plated on the sputter with the gold powder and SEM monitoring was carried out at operation voltage of 5 kV and image capturing was performed at 2000× magnifications.
2.5 Fourier transform infrared (FTIR) spectroscopy
FTIR spectrophotometry was employed for analyzing the organic functional groups of PP and PS DFs extracted by different extraction methods. Using an FTIR spectrophotometer (Tensor 27, Bruker Daltonics Inc., Bremen, Germany), the scanning was performed at spectrum wavelength ranging from 400 to 4,000 cm−1 at scan speed of <10 s for 4 scans at resolution of 4 cm−1. The obtainment of spectra was carried out in Attenuated Total Reflection (ATR) mode.
2.6 X-ray diffraction (XRD) analysis
The XRD patterns were measured by means of a diffractometer (7000S, Shimadzu, Kyoto, Japan) with copper Kα radiation at 40 kV (50 mA and 0.154 nm) as well as diffraction angle (2θ) ranged 4–40°C.
2.7 Weight loss measurement
Thermal properties were measured in terms of weight loss, which was calculated by means of a thermogravimetric analyzer (TGA, TG/DTA 8122, Rigaku, Tokyo, Japan), following the method reported by Du et al. (2019) for evaluating the DF samples. The measurement of weight loss was carried out over an operational temperature range of 30–600°C at a heating rate of 20°C/min under nitrogen atmosphere.
2.8 Rheological measurements
DFs solutions obtained from each extraction method were subjected to viscosity measurement using a rheometer (Discovery HR-1, TA, New Castle, DE, United States). Analysis was performed by means of steel cone geometry (gap = 56 μm: diameter = 60 mm). All measurements were recorded thrice at an operational temperature of 25°C (Jiang et al., 2022b).
2.9 HPLC determination of monosaccharides
Monosaccharides determination through HPLC was carried out through modified method of Jiang et al. (2022b). Each extracted sample was taken in an amount of 2 mg and dissolved in 1 mL of 2 M trifluoroacetic acid (TFA) and under sealed condition for a period of 2 h at 120°C in hydrothermal reactor. Prior to HPLC analysis, a standard filter with size of 0.45 μm was employed for filtration of aqueous layer. HPLC system of Dionex Thermo Ultimate 3,000 (Dionex Co., Sunnyvale, CA, United States) equipped with diode array detector (DAD) supplied by Thermo Fisher Sci. (Waltham, MA, United States) was employed for detection of monosaccharides. “The mobile phase was prepared by mixing acetonitrile (A) and phosphate buffer solution (0.1 mol/mL; PBS, pH 6.7) in a ratio of 82:18 (v/v). The analysis was performed using a Supersil ODS2 column (5 μm, 4.6 × 250 mm2) with an injection volume of 20 μL and a flow rate of 0.8 mL/min. Detection was carried out at a wavelength of 245 nm against reference standards.
2.10 Water holding capacity (WHC) and oil holding capacity (OHC)
WHC and OHC were determined as per the methods described by Raza et al. (2021). Briefly, 0.5 g of each extracted sample was taken in the centrifuge tube followed by addition of 5 mL of distilled water with samples and then was subjected to incubation at 37°C for period of 1 h. After the filtration of water, the WHC of samples were measured according to following Eq. 3.
Whereby, the W1 depicts total sample weight on dry basis and W2 represents total sample weight after the water was filtered out.
OHC of all extracted samples were determined by preparing mixture of each sample in specified amount of 0.5 g with soybean oil in centrifuge tube followed by incubation at 37°C for 1 h interval. Samples were centrifuged at 5,000×g for 10 min interval and OHC was measured using the following Eq. 4
Whereby, the W1 depicts total sample weight on dry basis and W2 represents total sample weight after removal of excessive soybean oil.
2.11 Water swelling capacity (WSC)
WSC was calculated for each DF sample according to the modified method of He et al. (2020). In brief, DF sample in a specified amount of accurately weighed 0.5 g was transferred to 26 mL of measuring cylinder and then was mixed with distilled water of 20 mL. The volume was calculated prior and after the completion of standing time of 2 h and WSC was measured in terms of volumetric change in DF dry sample after water swelling as per the Eq. 5 given:
Whereby, m0 represents DF sample dry weight, V1 represents initial volume and V2 indicates final volume after completion of standing time interval.
2.12 Cholesterol-adsorption capacity (CAC)
CAC values were measured as per the reported method of Jiang et al. (2022b). Each extracted sample (1 g) was mixed with 30 mL of diluted yolk solution. After the adjustment of pH to 2.0 or 7.0, samples were subjected to incubation at 37°C for 180 min. Absorbance of the supernatant was recorded spectrophotometrically at detection wavelength of 550 nm and calculated CAC values were represented in terms of mg/g as given in Eq. 6.
Where m is denoted as the mass of the constant sample weight (g); n1 is the cholesterol concentration of before adsorption (mg/mL), while n2 is the cholesterol concentration in the supernatant after centrifugation (mg/mL); V is volume (mL).
2.13 Bile acid-adsorption capacity (BAC)
BAC measurement was carried out as per the method reported by Jiang et al. (2022b). Each sample in specified amount of 1 g was mixed with 30 μL of sodium cholate (pH 7.0: 1–3 mg/mL) followed by incubation at 37°C for 2 h interval. Supernatant was analyzed for absorbance through spectrophotometer (UV-1800, Shimadzu Instruments Mfg. Co., Ltd., Kyoto, Japan) at detection wavelength of 620 nm.
2.14 Nitrite-ion adsorption capacity (NIAC)
NIAC was assessed as per the reported method of Jiang et al. (2021) with certain modifications. Each extracted sample (1 g) was mixed with 25 mL of sodium nitrite solution (100 μmol/L) and pH adjustment was carried out to 2.0 and 7.0 in terms of simulation of the pH conditions similar to human stomach and small intestine. Then, the samples were incubated for 120 min at 37°C. N-(1- naphthyl) ethylenediamine dihydrochloride/sulfanilic acid reagent was employed for determination of nitrite ion content spectrophotometrically at detection wavelength of 538 nm.
2.15 Glucose adsorption capacity (GAC)
GAC values were measured as per the method of Wang et al. (2021). Each sample (0.5 g) was mixed with 50 mL of glucose solution (conc. 50 mmol/L). Samples were continuously stirred for 2 h interval at 37°C followed by centrifugation of samples at 2,862×g for 5 min time interval. Determination of the final glucose concentration of collected supernatant was performed by a glucose assay kit (oxidaseperoxidase kit, Rongsheng Biotech Co., Ltd., Shanghai, China).
2.16 Preparation of DF samples extract
The DF samples were extracted according to the method described by Jiang et al. (2018). Homogenization and sonication treatments were performed sequentially (first the homogenization was performed followed by sonication). The obtained extract was employed for determination of antioxidant activities in later experiments.
2.17 Determination of antioxidant activities
Antioxidant activities, such as DPPH, ABTS and FRAP were assessed accorng to the methods described by Thaipong et al. (2006). A spectrophotometer was employed for measurement of the absorbance values of DPPH, ABTS and FRAP values at detections wavelengths of 515, 734 and 593 nm, respectively. The results of antioxidant activities were represented in terms of μmol trolox equivalent (TE)/g and standard curve linearity was ranged between 25 and 800 mM TE/g.
2.18 Statistical analysis
All the statistical analyses were performed by means of SPSS 20 0.0 software (SPSS Inc., IL, United States). All experiments were carried out in triplicate manner and results were represented in terms of mean ± standard deviation (SD) whereas analysis of variance (ANOVA) in conjunction with Duncan’s test were employed for assessment of the differences between the means at a significance level of p < 0.05.
3 Results and discussion
3.1 DF extraction yield and proximate composition
The results of DF yield and proximate composition of DF fraction from PP and PS obtained by various extraction methods are provided in Table 1. In the case of PP, DF extracted by AL exhibited the highest DF yield of 84.23%. Similarly, DF yield of PS was also analyzed and it was evident from results that PS-AL fraction exhibited the highest DF yield (81.13%). The probable reason for the highest DF recovery by alkaline extraction can be ascribed to the thorough destruction of cell wall and the disruption of compact molecules within the cell wall structure when exposed to an alkaline solution. This process results in the partial dissolution of hemicellulose in IDF, consequently leading to a higher extraction yield of DF (Sun et al., 2018). The protein content of DF extracted by PP-AC and PS-AC was the highest. Ash and fat contents in DF extracted by PP-AC and PS-AC was found to be much higher. Similar results have been reported by Wang et al. (2021) for the extraction yield and proximate compositions of IDF and SDF extracted from kiwi fruit using various extraction methods.
3.2 SEM
In the current study, SEM was employed to elucidate the microstructures of DF fractions derived from all extraction methods. As shown in Figure 1, DF extracted by PP-AL and PS-AL exhibited more complicated structures with lesser degree of pore loosening as compared to other samples. In PP-AC and PS-AC extracted DFs, the surface irregularity, pore loosing, disintegration of cell walls and microstructure impairment were more prominent as compared to DF extracted from PP and PS by EN and AL. Severe structural impairment of DF extracted by PP-AC and PS-AC might be ascribed to the disintegration of sheet-like structure in DF of PP and PS. The SEM images indicate that AC condition exhibited significant effect on DF microstructure. Moreover, DFs with less compactness and looser spatial structures exhibit increased surface area, which may significantly affect the adsorption capacities of bile acid, water, oil, glucose and nitrite ion. Conclusively, it may be implied that the type of extraction method may lead to alteration in the DFs properties extracted from PS and PP. These results are in agreement with the findings of Wang et al. (2021), who reported significant effects of extraction methods on the morphology and microstructural properties of DF extracted from kiwi fruit.
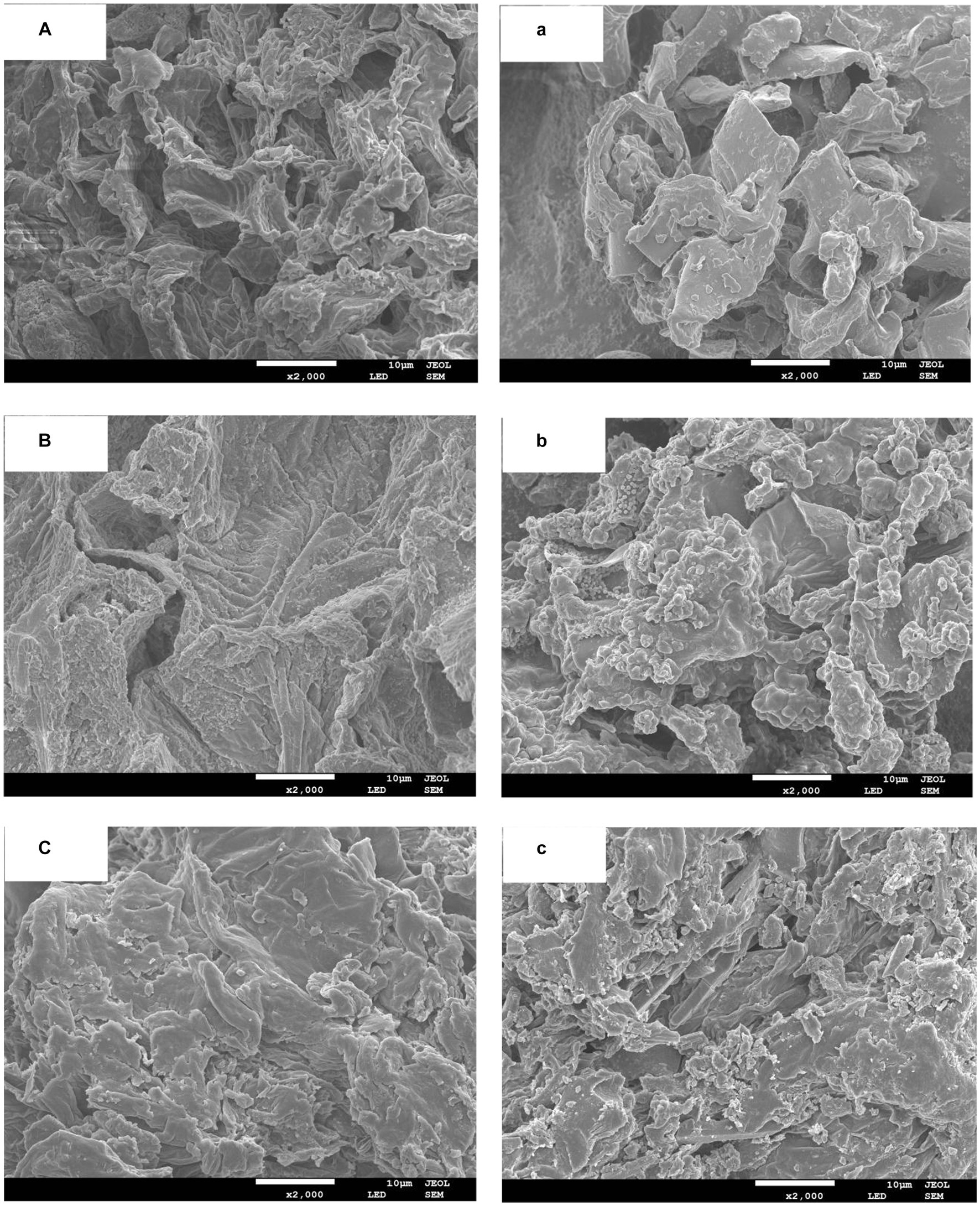
Figure 1. SEM images for DF extracted by PP-AC (A), PP-EN (B, b), PP-AL (C), PS-AC (a), PS-EN (b) and PS-AL (c).
3.3 FT-IR spectroscopy
Figure 2A shows the transmittance percentage for all DF fractions of PP and PS obtained by various extraction methods. The stretching vibrations of two broad peaks at IR regions of 3,427 cm−1 and 2,927 cm−1 were visible in all DF’s samples and were indicative of the stretching vibrations of hydroxyl (-OH) group and –CH of sugar methyl as well as methylene groups, respectively. The absorption peaks existing at IR regions of 1,200–1,400 cm−1 might be ascribed to the variable angle CH vibration. Furthermore, absorption peaks at these IR regions are reported in published literature as characteristic absorption peaks corresponding to saccharides in DF. Similarly, irrespective of the type of extraction method used, all DF fractions of PP and PS exhibited absorption peaks at IR regions ranged 1,000–1,300 cm−1 which might be attributable to the contraction IR vibration corresponding to ester C–O, while peaks presence in IR spectral range of 700–1,000 cm−1 may be ascribed to the characteristic absorption peaks of α- and β-pyran monosaccharides. The absorption peaks with weak intensities at IR spectral ranges of 3,414–3,480 cm−1 (vibrational stretching of –OH groups) and 2,856–2,923 cm−1 (vibrational stretching of C–H groups in case of polysaccharides polymers) in DF fractions of PS and PP might be attributed to the possible breakage of intermolecular bonds and disintegration of molecular structures after exposure to AC, EN and AL treatments. It was confirmed by the FTIR results that EN extraction caused less peak broadening in IR peaks as compared to the PP-, PS-AC, and PP-, PS-AL implying that EN extraction led to increased degree of hydrolysis followed by AL treatment in hemicellulose and pectin of PS and PP-derived DF’s samples. In all DF samples, the IR absorption peak at the region of 1,000 cm−1 was indicative of the C–O stretching vibration in C–O–C linkages (typical IR peak of xylan) which implied that DF comprised of xylan hemicellulose in its structural configuration. IR peaks existence at IR spectral region of 1,660 cm−1 in DF extracted by PS-AC and PP-AC corresponded to the bending or stretching vibration of aromatic lignin hydrocarbon whereas, this characteristic peak was not found in the case of DFs of PP and PS extracted by EN and AL methods, implying that EN and AL treatments led to increased degree of disintegration in lignin molecules. These results are in line with the previous studies reported by Wang et al. (2021) for DF extracted from kiwi fruit.
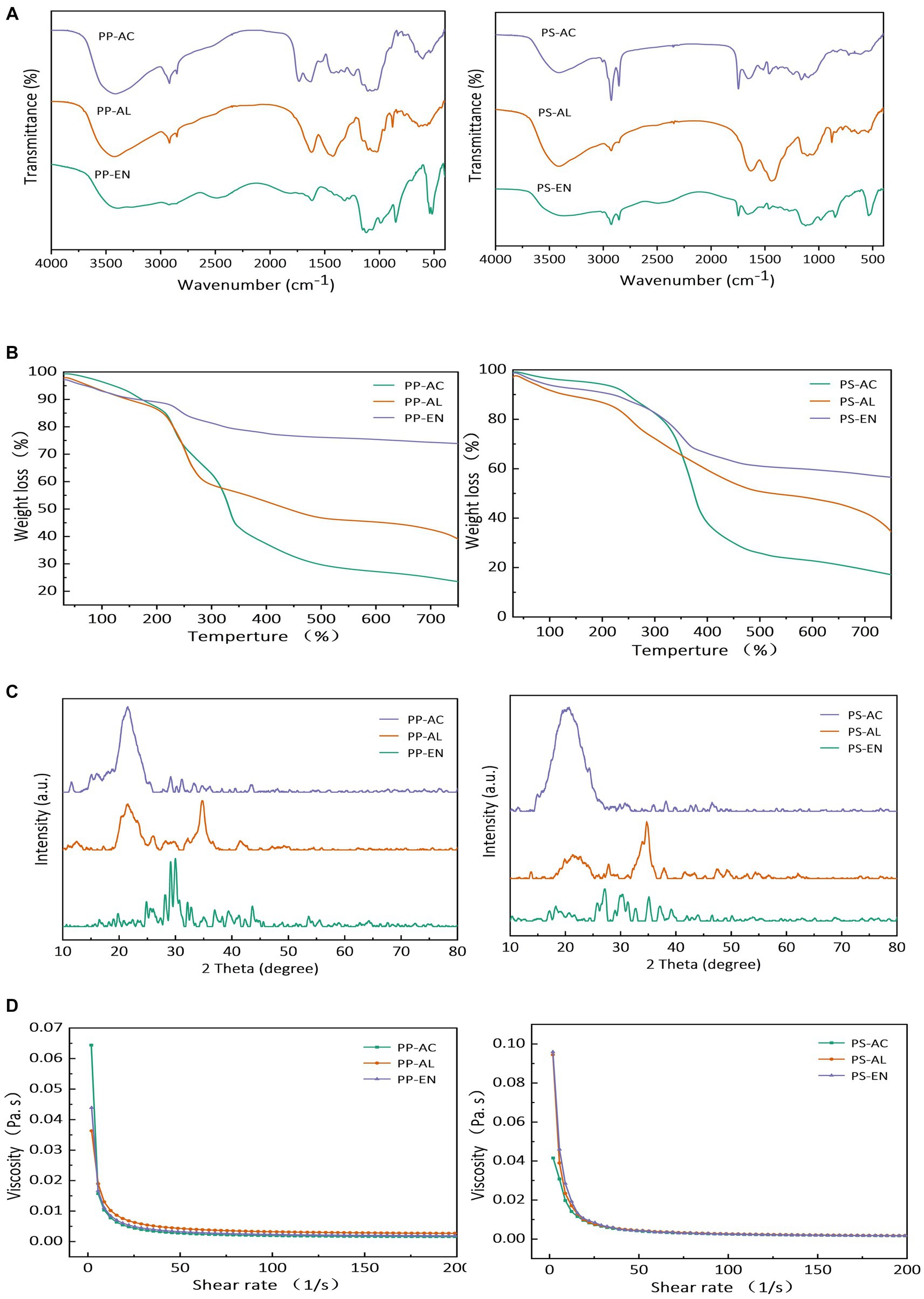
Figure 2. FT-IR spectra (A), thermal properties (B), X-ray diffraction (C) and rheogram plot (D) for DF extracted by PP-AC, PP-EN, PP-AL, PS-AC, PS-EN and PS-AL.
3.4 Thermal properties
TGA curves for all DF samples are depicted in Figure 2B. TGA curve is usually described in terms of three stages. During the initial stage at temperature range of 30–210°C, all DF samples irrespective of extraction method showed decreasing trend with respect to weight loss in conjunction with devolatilization occurrence at 120°C. In the case of both PP and PS, the highest weight loss was observed in case of DF samples by AC method followed by AL and EN methods with corresponding increase in the temperature. During initial stage of TGA analysis, the loss percentage in weight might be ascribed to the possibility of absorbed water evaporation from the DF samples extracted by various methods. It was also implied from the TGA curves that 210–400°C temperature range was for the second stage of TGA analysis and maximum degree of weight loss was occurred during this stage. This enhanced degree of weight loss during second stage might be attributable to the possible elevated degradation of pyrolytic polysaccharides mainly comprising of pectic polysaccharides and hemicelluloses. The weight loss observed in DFs extracted by PS-EN and PP-EN was relatively slower and proceeded in gradual manner, whereas weight loss was higher in the case of AL and AC methods during third stage for both PS and PP, which implied that DF’s from PS-EN and PP-EN exhibited relatively more thermal stability in comparison with those of other samples. The lower thermal weight loss observed for enzymatic extracted DF as EN method caused less polymer chain cleavage when compared to other methods of extraction. This may probably the main reason for higher thermal stability of enzymatic extracted fibre in such a way that enzymes had not degraded the main polysaccharides of extracts compared to acidic and/or alkaline treatments. The lower thermal weight loss observed for enzymatically extracted dietary fiber compared to acidic and alkaline extracted dietary fiber can be attributed to several factors related to the extraction methods and the nature of the fibers obtained. Here are some probable reasons, such as (1) enzymatic extraction often involves milder conditions compared to acidic or alkaline extraction. The use of enzymes is more specific and selective, allowing for the targeted breakdown of cell wall components without causing extensive degradation or modification of the fibers; (2) enzymatic extraction is less likely to disrupt the structural integrity of dietary fibers. Acidic and alkaline conditions can lead to chemical modifications and structural breakdown of fibers, making them more susceptible to thermal degradation; (3) acidic and alkaline extractions may leave residues of the chemicals used in the process, which can contribute to thermal weight loss during subsequent analysis. Enzymatic extraction typically involves fewer chemicals and, therefore, results in lower chemical residues that might contribute to thermal degradation (Huang et al., 2018; Ma et al., 2022; Jiang et al., 2023). These results were in agreement with the previously reported findings of Wang et al. (2021) for DF extracted from kiwi fruit.
3.5 XRD
The results of diffractograms from DF extracted by each method are demonstrated in Figure 2C. Generally, all the DF samples of PP extracted by various methods exhibited characteristics main signal peaks at 2θ angles of 14–15°, 21.5°, 22.32°, 23.5–24.5° and 30–31°. It has been reported in the published literature that signal peaks around 14–15° and 21–23.5°might be due to the presence of cellulose crystals. Cellulose exhibits crystalline configuration owing to Van der Waals forces and hydrogen bonding linkages in adjacent molecules. Moreover, the amorphousness in DFs exists because of non-crystalline cellulose, hemicellulose and lignin (Kulasinski et al., 2014). The peak existing at 2θ angle range of 21.2–23.5° was quite broader in DF extracted by PP-AC as compared to sharper peak in the case of PP-AL. PP-AL DFs showed existence of another broader peak at 34.23–37.5°whereas this peak intensity was much lower in case of PP-AC. PP-EN DFs showed two split peaks emergence at 38.23–32.51°. Regarding PS samples, DF extracted by AC (PS-AC) exhibited large peak with broad shape at 14.12–27.5° as compared to PS-AL, which showed very low signal peak intensity at same 2θ angle. PS-AL DFs exhibited sharp peak with medium intensity at 34.19–37.25°. For PS-EN DFs, small peaks emergence was noted at range of 25.28–39.53°. As far as the extractions methods are concerned, the peak positions and peak width were affected by AL and EN extraction methods, which implied that both AL and EN extraction methods resulted in significant disruption in the crystalline structures of PP- and PS-DF. Moreover, both AL and EN methods led to emergence of new signals peaks and consequently new crystalline formations in structural configurations of DF from EN and AL methods as compared to that was obtained by AC extraction method. The irregularity in peaks of DF extracted by PS-EN, PP-EN, PS-AL and PP-AL might be because of denaturation effects of enzymatic hydrolysis and NaOH solution. Similar results have been reported by Ma and Mu (2016) whereby authors have carried out the research regarding DF extraction from deoiled cumin and have reported the denaturation effects caused by enzymatic hydrolysis.
3.6 Viscosity
The viscosity of all DF samples was measured as a function of shear rate and the results are demonstrated in Figure 2D. In the case of PP-DF samples, all the extractions methods showed similar rheograms, and DF extracted by PP-AL showed slightly decreasing trend in viscosity. However, PP-AL exhibited slightly lower viscosities as compared to those of PP-AC and PP-EN. Similarly, for PS samples, the rheograms of PS-AC and PS-AL exhibited less degree of curvature in comparison with that of PS-EN. Moreover, it is worthwhile to note that charge-transfer complexes (CTC) and hydrogen bonding linkages present in the DF’s polymer chains may affect the viscosity parameter (Jiang et al., 2023). DF extracted by PP-AC and PS-EN showed a high degree of linearity in rheograms as compared to other DF samples and differences in the viscosities of PS and PP samples extracted by AL and EN methods. This may be ascribed to the structural modifications, greater interactions of particles, alterations in cluster structures of DF, and breakage of partial branching, thereby increasing the apparent viscosity (Jiang et al., 2022b). Moreover, EN hydrolysis and NaOH solution caused destruction of the molecular structures owing to breakage of partial branching patterns and reduction of internal molecular linkages and tightness (Bi et al., 2020). These results were in line with the previously reported study of Jiang et al. (2022b) for DF extracted from Sanchi flower.
3.7 Monosaccharide composition
The results of DF samples monosaccharide composition by determined by HPLC are shown in Table 2 and Figure 3, exhibiting the molar ratio of monosaccharides in DF derived from PP and PS by various extraction methods. It was found that all DF samples consisted of mannose, rhamnose, glucuronic acid, galacturonic acid, glucose, galactose, xylose and arabinose. The dominant monosaccharides of DF derived from PP and PS samples were found to be glucose, galacturonic acid and galactose. Previous studies have reported that the main monosaccharides of DF are glucose, galactose and arabinose from DF of calamondin pomace and sweet potato (Huang and Ma, 2019; Neela and Fanta, 2019). Glucose is mainly derived from starch and cellulose, and the content of DF extracted by PP-AC and PS-AC glucose is relatively high, which may be because the AC environment promotes the hydrolysis of cellulose in the cell wall. In both DFs from PS and PP, the higher molar ratio of galactose might be ascribed to the enhanced hydrolysis of cell wall cellulose after exposure to AC and AL extraction methods. Moreover, the increased galactose in DF extracted by PP-AC and PS-AC could be the main contribution to the increased SDF content, thereby affecting the water holding capacity of PP and PS DFs. The DF derived PP samples had higher galacturonic acid than those of DF derived PS samples, indicating that PP contains more pectin, which had good physical and functional properties. Galacturonic acid is the main component of pectin. However, higher xylose observed in DF derived from PS samples, indicating a higher presence of xylan hemicellulose. The relatively high content of rhamnose in DF extracted by PP (AC and AL) and PS (AC and AL) indicates that SDF contains more pectin. Pectin always contains varying amounts of neutral sugars, such as D-galactose, L-rhamnose, L-arabinose, and D-xylose. Some of these neutral sugars are constituents of side chains to the galacturonan backbone (Flutto, 2003). The amounts of mannose, galacturonic acid and glucuronic acid in PP- and PS-AC decreased compared to the PP- and PP-EN amount, while the glucose amount increased, which was possibly due to the breakage of glycosidic bond by AC extraction, as well as the formation of glucose (Wang et al., 2021). Based on our results, the different extraction methods had impacts on PP- and PS-derived DFs.
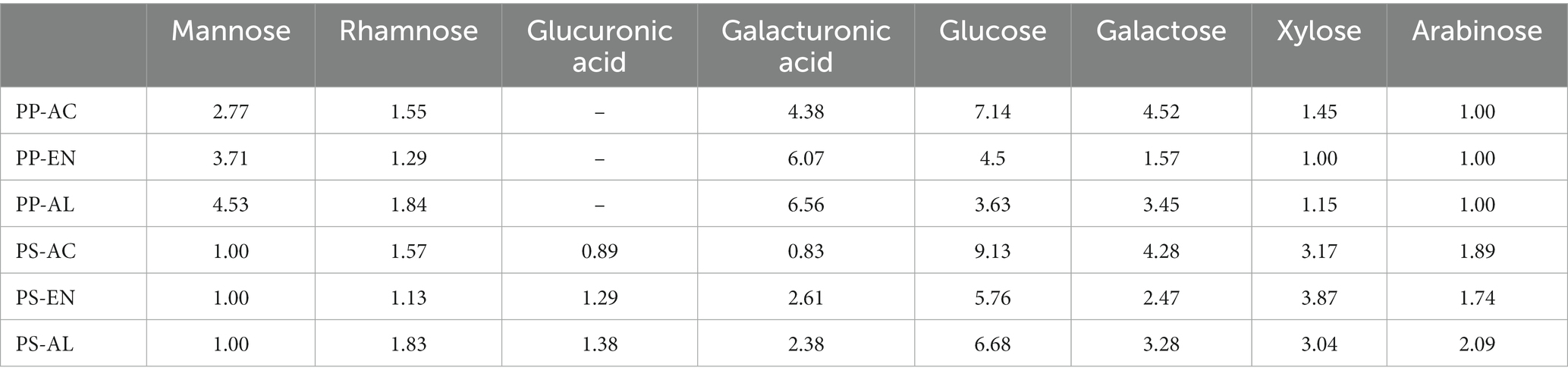
Table 2. Molar ratio of monosaccharide components of DF extracted by PP-AC, PP-EN, PP-AL, PS-AC, PS-EN and PS-AL.
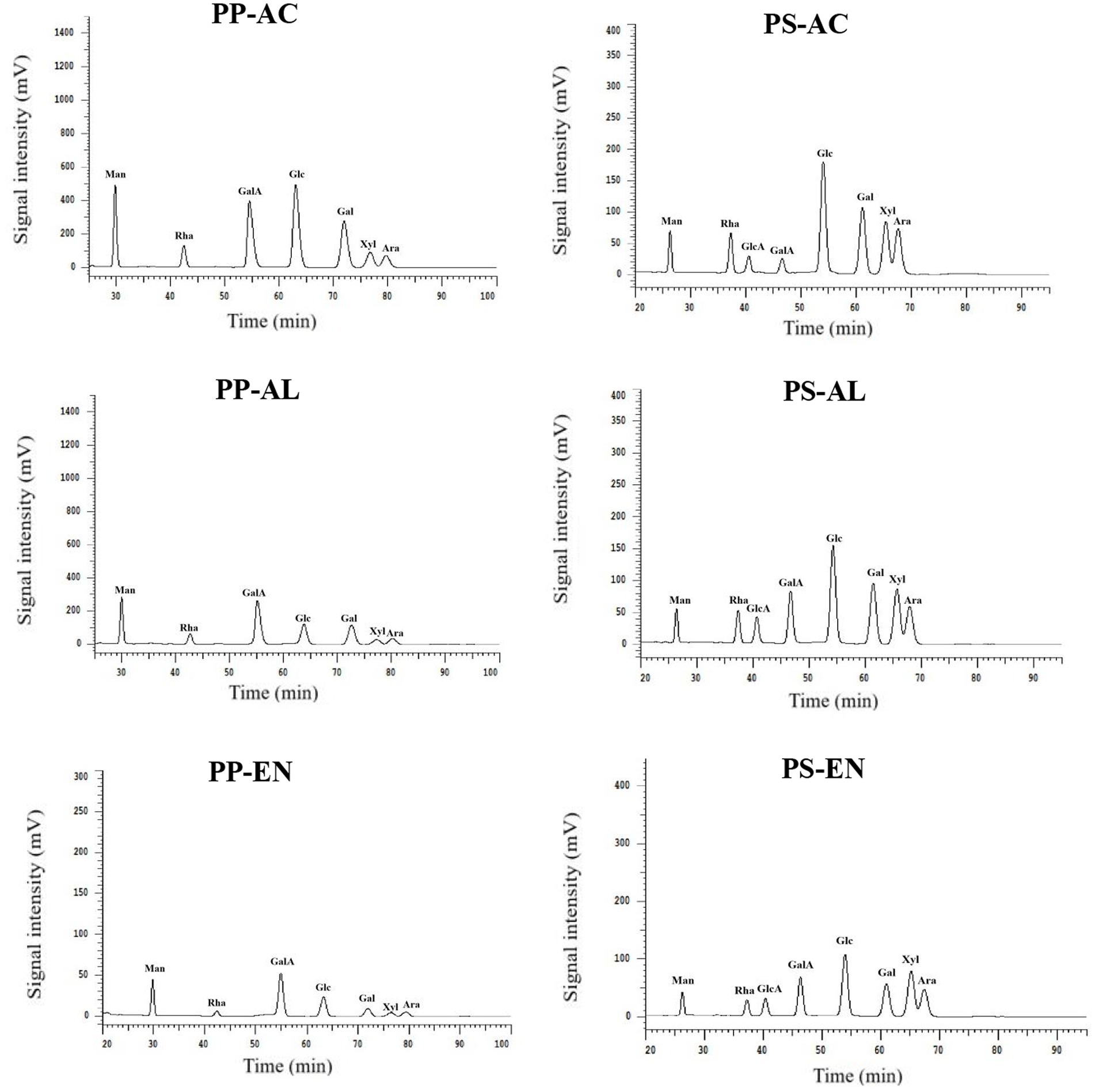
Figure 3. HPLC grams of monosaccharide standards and monosaccharide compositions for DF extracted by PP-AC, PP-EN, PP-AL, PS-AC, PS-EN and PS-AL.
3.8 Functional properties
3.8.1 WHC, OHC and WSC
The results regarding effects of different extraction methods on hydration properties of PP and PS-derived DF are graphically represented in Figure 4. WHC property is indicative of water retention capacity of foodstuffs when food materials are processed by applying various processing techniques, such as centrifugation and compression. Among all samples, DF extracted by PP-AC exhibited the highest WHC, whereas lowest WHC was observed with DF extracted by PP-AL. WHC comprises linked water, physically trapped water and hydrodynamic water. WHC is an inherent property of foods, which is linked with hydrophilic site, diverse DF surface area, structures and densities. Generally, DF samples exhibiting high WHC may cause prevention of foods shrinkage and viscosity alteration. Similar results have been reported regarding WHC of DF extracted from kiwifruit (Wang et al., 2021) and grapefruit peel (Gan et al., 2020).
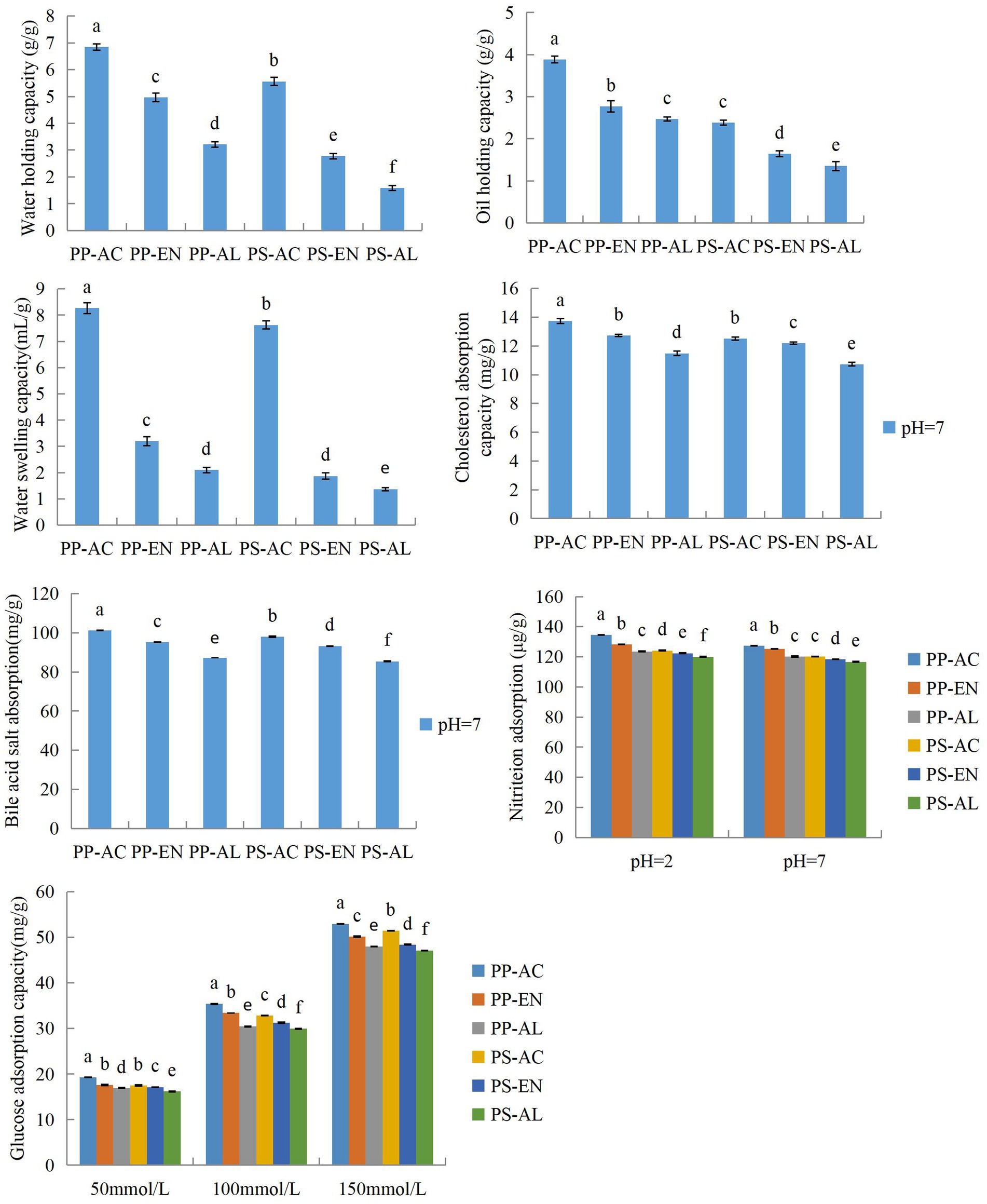
Figure 4. Hydration and functional properties for DF extracted by PP-AC, PP-EN, PP-AL, PS-AC, PS-EN and PS-AL. Values followed by different letters (a–f) in the same bar represent statistically significant differences at p < 0.05.
Conversely, the OHC is defined as the natural ability of DF to maintain the oil droplets after subjecting DF to shear mixing with oil followed by centrifugation. OHC of DF exhibits high significance in food applications, such as fat loss prevention during cooking. Among all samples, DF extracted by PP-AC exhibited the highest OHC, but DF extracted by PS-AL had lowest OHC. The high OHC in PP DFs might be attributable to the structure loosening after exposure to AC conditions. Typically, foods fortified with DFs from PP and PS, may serve as good source for related food products. Furthermore, they can help prevent water synersis during foods formulation and act as emulsifier for high-fat foods owing to their high OHC and WHC (Lv et al., 2017; Jia et al., 2019).
WSC is described in terms of ratio between volume occupied by DF subjected to immersion in excessive water after reaching equilibrium and actual weight of DF. There are two reported mechanisms for DF interaction with water: (i) capillary structures hold water as a consequence of surface tension strength, (ii) water retention because of dipole forms and hydrogen bonds. Free polar groups and particle sizes have significant contributory roles to enhance WSC of DFs. Besides, among all PP samples for WSC, DF extracted by PP-AC exhibited the highest WSC value of 8.27 mL/g. As far as PS-derived DFs samples concerned, PS-AC exhibited the highest WSC value of 7.63 mL/g. WSC particularly has positive correlation with that of pectin content and results implied that AC extraction by citric acid caused less degradation of pectin whereas, NaOH extraction led to the highest probable degradation of pectin in cell wall, Consequently, decreasing tendency in WSC was observed both in DFs samples from PS and PP. These results were in line with the previous findings reported by Ma and Mu (2016) regarding effects of different extraction methods on WSC of DF extracted from deoiled cumin.
3.8.2 CAC
The CAC values for all DF samples extracted by various extraction methods are depicted in Figure 4. CAC involves the estimation of total cholesterol mass absorbed by DF. In case of physical adsorption, important roles are played by temperature, surface area, porosity and particle size. Regarding chemical adsorption, hydrophobicity and electrostatic charges play a pivotal role. DF cholesterol absorption may cause a decrease in serum cholesterol levels. In the case of PP-derived DF samples at pH 7.0, the highest CAC value was exhibited by DF extracted by PP-AC followed by PP-EN and PP-AL. Moreover, CAC value of DF from PP was higher than those of DF from PS derived from various extraction methods. The possible reason for the enhanced CAC in the case of the PP-AC and PS-AC DFs might be ascribed to the loosening of the IDF’s structural framework due to AC hydrolysis. The results for acid treated DF suggested that it has more hydrophilic groups interacting with water molecules (resulting in higher WHC and WSC). The main probable reason for increased WHC and CAC of acid extracted samples may be higher porosity of fiber structure. Furthermore, the higher CAC observed for DF extracted by PP-AC might be attributed to the truth that the surface of PP-AC had more small particles, forming a honeycomb structure to bind more cholesterol.
3.8.3 BAC
The BAC values for all DF samples extracted by various extraction methods are calculated at pH 7 and results are depicted in Figure 4. Bile acids biosynthesis occurs in liver, where cholesterol serves as precursor molecule. DFs exhibit bile acid binding ability, which promotes bile acid elimination, and increases conversion to cholesterol-bind acid, thereby lowering serum cholesterol levels as well as incidence of cardiovascular disease (Feng et al., 2017). Higher BAC value were found in case of DF extracted by PP-AC and PS-AC. Besides, DF extracted by PP-AC had slightly higher values than those of PS-AC. It was evident from the results that BAC values exhibited differences and were significantly affected by the type of sample (peel or seed of papaya) and type of extraction method used for extraction. It has also been suggested by previous studies that gel properties and anionic group content of DFs may exert significant influence on BAC of DFs (Niu et al., 2013; Feng et al., 2017). Furthermore, BAC linkage has also been reported with those of DF internal structure, particle sizes and surface properties (Jiang et al., 2022b).
3.8.4 NIAC
The NIAC values for all DF samples extracted by various extraction methods were measured at two different pH levels (2.0 and 7.0) and results are shown in Figure 4. At gastric acid conditions, the NO2− ions reaction may pose a risk of cancer in conjunction with fetal malformations (Gan et al., 2020). Among PP samples, DF extracted by PP-AC exhibited the highest NIAC values at both pH levels of 2 and 7. In the case of DFs samples from PS, the DFs extracted by AC exhibited the highest NIAC values. As compared to AC extraction, EN and AL extractions caused slightly decreasing tendency with NIAC values at both pH levels of 2 and 7, respectively. In this study, DF extracted by PP-AC had the highest NIAC at pH 2.0 (134 μg/g), but it was still lower than that of kiwifruit SDF (138 μg/g) (Wang et al., 2021) and grapefruit peel-derived SDF (219.43 μg/g) (Gan et al., 2020). Besides, the NIAC values depended on the DF extraction methods, suggesting that the PP and PS-derived DF samples potentially absorbed nitrite ions, which could be excreted with feces.
3.8.5 GAC
The GAC values for all DF samples extracted by various extraction methods were calculated at glucose concentration levels of 50–150 mmol/L and results depicted in Figure 4. Intestinal juice contains glucose and DF has the ability to bind glucose, which may lead to lowering of postprandial serum glucose. Therefore, GAC is regarded as an important DFs functional property. Moreover, it has also been suggested by previous studies that increased GAC values might be because of increased number of cavities and enhanced surface area. Among PP samples at glucose concentration of 50–150 mmol/L, DF extracted by PP-AC had the highest GAC value compared to other samples. In the case of PS-derived DF samples at 50–150 mmol/L, PS-AC DF samples also had the highest GAC value among all samples. In addition, the higher GAC value was observed for PP-DF samples when compared to PS-DF samples. The results indicate that extraction methods and DF from different parts (peel and seed) might affect GAC, and that PP and PS-derived DF samples exhibited in vitro hypoglycemic potential.
3.9 Antioxidant activities
All DF samples were analyzed for their DPPH, ABTS and FRAP activities and results are demonstrated in Table 3. Comparatively, among all extraction methods, the PP and PS DFs samples extracted by AC exhibited the highest DPPH, ABTS and FRAP values. Moreover, DF derived from PP had higher antioxidant activities than those of DF derived from PS samples. According to relevant literature, the DPPH free radical scavenging ability of DF is related to the content of phenolic substances and the structure of phenolic acids in raw materials (Esposito et al., 2005; Guo and Beta, 2013). In addition, some studies have found that the reducing power of the crushed fiber was improved, which may be due to the change of the fiber structure caused by the shear force during the crushing process, resulting in the release of some phenolic substances (Zhu et al., 2015). Here, the high reducing power of DF extracted by PP-AC and PS-AC may be due to the release or exposure of some phenolic substances in PP and PS caused by the treatment of AC during the extraction process.
4 Conclusion
In this study, the structural and functional characteristics, as well as antioxidant activities of DFs produced from PP and PS are characterized using different extraction methods. In the case of TFC yield, DF extracted by AL method from PP and PS exhibited the highest content of 84.23% and 81.13%, respectively. DF extracted by PP-, PS-AC had looser and more complicated structures. The extraction methods not only impacted the structural characteristics of DFs, but also affected their functional and antioxidant properties. Among the extraction methods, DFs extracted by PP-AC and PS-AC had the best functional and antioxidant properties. Additionally, PP DFs had higher functional and antioxidant activities than PS DFs among the different extraction methods. Therefore, PP DFs extracted by AC method display great potential for applications in functional food industry. Extracting DF is a form of deep processing of PP, which can increase the added value of papaya industry.
Data availability statement
The original contributions presented in the study are included in the article/supplementary material, further inquiries can be directed to the corresponding author.
Author contributions
XF: Conceptualization, Investigation, Methodology, Writing – original draft, Writing – review & editing. KA: Conceptualization, Methodology, Writing – original draft, Writing – review & editing. GJ: Conceptualization, Data curation, Formal analysis, Funding acquisition, Investigation, Methodology, Software, Validation, Writing – original draft, Writing – review & editing. KR: Investigation, Methodology, Writing – original draft, Writing – review & editing.
Funding
The author(s) declare financial support was received for the research, authorship, and/or publication of this article. This work was supported by the Foundation of the Education Department of Jilin Province (No. JJKH20230539KJ).
Conflict of interest
The authors declare that the research was conducted in the absence of any commercial or financial relationships that could be construed as a potential conflict of interest.
Publisher’s note
All claims expressed in this article are solely those of the authors and do not necessarily represent those of their affiliated organizations, or those of the publisher, the editors and the reviewers. Any product that may be evaluated in this article, or claim that may be made by its manufacturer, is not guaranteed or endorsed by the publisher.
References
AACC . (2000). International. Approved methods of the AACC. St. Paul, MN, USA: American Association of Cereal Chemists.
Ameer, K., Shahbaz, H. M., and Kwon, J. H. (2017). Green extraction methods for polyphenols from plant matrices and their byproducts: a review. Compr. Rev. Food Sci. F. 16, 295–315. doi: 10.1111/1541-4337.12253
Bi, C. H., Yan, Z. M., Wang, P. L., Alkhatib, A., Zhu, J. Y., Zou, H. C., et al. (2020). Effect of high pressure homogenization treatment on the rheological properties of citrus peel fiber/corn oil emulsion. J. Sci. Food Agric. 100, 3658–3665. doi: 10.1002/jsfa.10398
Du, X., Bai, X., Gao, W., and Jiang, Z. (2019). Properties of soluble dietary fibre from defatted coconut flour obtained through subcritical water extraction. Int. J. Food Sci. Technol. 54, 1390–1404. doi: 10.1111/ijfs.14071
Esposito, F., Arlotti, G., Maria, B. A., Napolitano, A., Vitale, D., and Fogliano, V. (2005). Antioxidant activity and dietary fibre in durum wheat bran by-products. Food Res. Int. 38, 1167–1173. doi: 10.1016/j.foodres.2005.05.002
Feng, X., Ameer, K., Ramachandraiah, K., Wu, Z., Huo, N., Bai, X., et al. (2022). Effects of papaya (Carica papaya L.) seed supplementation on quality attributes, adsorption capacities, and in vitro starch digestibility of wheat bread. J. Food Meas. Charact. 16, 3226–3239. doi: 10.1007/s11694-022-01415-0
Feng, Z., Dou, W., Alaxi, S., Niu, Y., and Yu, L. (2017). Modified soluble dietary fiber from black bean coats with its rheological and bile acid binding properties. Food Hydrocoll. 62, 94–101. doi: 10.1016/j.foodhyd.2016.07.032
Flutto, L. (2003). Pectin | properties and determination. Encycl. Food Sci. Nutr. 2, 4440–4449. doi: 10.1016/B0-12-227055-X/00901-9
Gan, J., Huang, Z., Yu, Q., Peng, G., Chen, Y., Xie, J., et al. (2020). Microwave assisted extraction with three modifications on structural and functional properties of soluble dietary fibers from grapefruit peel. Food Hydrocoll. 101:105549. doi: 10.1016/j.foodhyd.2019.105549
Guo, W., and Beta, T. (2013). Phenolic acid composition and antioxidant potential of insoluble and soluble dietary fibre extracts derived from select whole-grain cereals. Food Res. Int. 51, 518–525. doi: 10.1016/j.foodres.2013.01.008
He, Y., Li, W., Zhang, X., Li, T., Ren, D., and Lu, J. (2020). Physicochemical, functional, and microstructural properties of modified insoluble dietary fiber extracted from rose pomace. J. Food Sci. Technol. 57, 1421–1429. doi: 10.1007/s13197-019-04177-8
Huang, Y. L., and Ma, Y. S. (2019). Optimization of the extrusion process for preparation of soluble dietary fiber-enriched calamondin pomace and its influence on the properties of bread. J. Food Sci. Technol. 56, 5444–5453. doi: 10.1007/s13197-019-04015-x
Huang, L., Zhang, X., Xu, M., An, S., Li, C., Huang, C., et al. (2018). Dietary fibres from cassava residue: physicochemical and enzymatic improvement, structure and physical properties. AIP Adv. 8:105035. doi: 10.1063/1.5054639
Jia, M., Chen, J., Liu, X., Xie, M., Nie, S., Chen, Y., et al. (2019). Structural characteristics and functional properties of soluble dietary fiber from defatted rice bran obtained through Trichoderma viride fermentation. Food Hydrocoll. 94, 468–474. doi: 10.1016/j.foodhyd.2019.03.047
Jiang, G., Feng, X., Wu, Z., Li, S., Bai, X., Zhao, C., et al. (2021). Development of wheat bread added with insoluble dietary fiber from ginseng residue and effects on physiochemical properties, in vitro adsorption capacities and starch digestibility. LWT 149:111855. doi: 10.1016/j.lwt.2021.111855
Jiang, G., Feng, X., Zhao, C., Ameer, K., and Wu, Z. (2022a). Development of biscuits supplemented with papaya seed and peel: effects on physicochemical properties, bioactive compounds, in vitro absorption capacities and starch digestibility. J. Food Sci. Technol. 59, 1341–1352. doi: 10.1007/s13197-021-05143-z
Jiang, G. H., Nam, S. H., and Eun, J. B. (2018). Effects of peeling, drying temperature, and sodium metabisulfite treatment on physicochemical characteristics and antioxidant activities of Asian pear powder. J. Food Process. Preserv. 42:e13526. doi: 10.1111/jfpp.13526
Jiang, G., Ramachandraiah, K., Tan, C., Cai, N., Ameer, K., and Feng, X. (2023). Modification of ginseng insoluble dietary fiber by enzymatic method: structural, rheological, thermal and functional properties. Foods 12:2809. doi: 10.3390/foods12142809
Jiang, G., Ramachandraiah, K., Wu, Z., and Ameer, K. (2022b). The influence of different extraction methods on the structure, rheological, thermal and functional properties of soluble dietary fiber from Sanchi (Panax notoginseng) flower. Foods 11:1995. doi: 10.3390/foods11141995
Jiang, G., Wu, Z., Ameer, K., Li, S., and Ramachandraiah, K. (2020). Particle size of ginseng (Panax ginseng Meyer) insoluble dietary fiber and its effect on physicochemical properties and antioxidant activities. App. Biol. Chem. 63, 1–10. doi: 10.1186/s13765-020-00558-2
Kulasinski, K., Keten, S., Churakov, S. V., Guyer, R., Carmeliet, J., and Derome, D. (2014). Molecular mechanism of moisture-induced transition in amorphous cellulose. ACS Macro Lett. 3, 1037–1040. doi: 10.1021/mz500528m
Kurek, M., Karp, S., Wyrwisz, J., and Niu, Y. (2018). Physicochemical properties of dietary fibers extracted from gluten-free sources: quinoa (Chenopodium quinoa), amaranth (Amaranthus caudatus) and millet (Panicum miliaceum). Food Hydrocoll. 85, 321–330. doi: 10.1016/j.foodhyd.2018.07.021
Leitão, M., Ribeiro, T., García, P. A., Barreiros, L., and Correia, P. (2022). Benefits of fermented papaya in human health. Foods 11:563. doi: 10.3390/foods11040563
Lv, J. S., Liu, X. Y., Zhang, X. P., and Wang, L. S. (2017). Chemical composition and functional characteristics of dietary fiber-rich powder obtained from core of maize straw. Food Chem. 227, 383–389. doi: 10.1016/j.foodchem.2017.01.078
Ma, Q., Ma, Z., Wang, W., Mu, J., Liu, Y., Wang, J., et al. (2022). The effects of enzymatic modification on the functional ingredient-dietary fiber extracted from potato residue. LWT 153:112511. doi: 10.1016/j.lwt.2021.112511
Ma, M. M., and Mu, T. H. (2016). Effects of extraction methods and particle size distribution on the structural, physicochemical, and functional properties of dietary fiber from deoiled cumin. Food Chem. 194, 237–246. doi: 10.1016/j.foodchem.2015.07.095
Neela, S., and Fanta, S. W. (2019). Review on nutritional composition of orange-fleshed sweet potato and its role in management of vitamin a deficiency. Food Sci. Nutr. 7, 1920–1945. doi: 10.1002/fsn3.1063
Niu, Y., Xie, Z., Zhang, H., Sheng, Y., and Yu, L. L. (2013). Effects of structural modifications on physicochemical and bile acid-binding properties of psyllium. J. Agric. Food Chem. 61, 596–601. doi: 10.1021/jf3043117
Raza, H., Ameer, K., Ma, H., Liang, Q., and Ren, X. (2021). Structural and physicochemical characterization of modified starch from arrowhead tuber (Sagittaria sagittifolia L.) using tri-frequency power ultrasound. Ultrason. Sonochem. 80:105826. doi: 10.1016/j.ultsonch.2021.105826
Sun, J., Zhang, Z., Xiao, F., Wei, Q., and Jing, Z. (2018). Ultrasound-assisted alkali extraction of insoluble dietary fiber from soybean residues. IOP Conf. Ser.: Mater. Sci. Eng. 392:052005. doi: 10.1088/1757-899X/392/5/052005
Thaipong, K., Boonprakoba, U., Crosby, K., Cisneros-Zevallosc, L., and Byrnec, D. H. (2006). Comparison of ABTS, DPPH, FRAP, and ORAC assays for estimating antioxidant activity from guava fruit extracts. J. Food Compos. Anal. 19, 669–675. doi: 10.1016/j.jfca.2006.01.003
Wang, K., Li, M., Wang, Y., Liu, Z., and Ni, Y. (2021). Effects of extraction methods on the structural characteristics and functional properties of dietary fiber extracted from kiwifruit (Actinidia deliciosa). Food Hydrocoll. 110:106162. doi: 10.1016/j.foodhyd.2020.106162
Yuliarti, O., Goh, K. K., Matia-Merino, L., Mawson, J., and Brennan, C. (2015). Extraction and characterisation of pomace pectin from gold kiwifruit (Actinidia chinensis). Food Chem. 187, 290–296. doi: 10.1016/j.foodchem.2015.03.148
Keywords: papaya, dietary fiber, microstructure, antioxidant properties, functional food
Citation: Feng X, Ameer K, Jiang G and Ramachandraiah K (2024) Effects of extraction methods on the structural characteristics and functional properties of dietary fiber extracted from papaya peel and seed. Front. Sustain. Food Syst. 8:1340961. doi: 10.3389/fsufs.2024.1340961
Edited by:
Zhen Chen, Xinyang Normal University, ChinaReviewed by:
Maria Simona Chiş, University of Agricultural Sciences and Veterinary Medicine of Cluj-Napoca, RomaniaAsad Mohammad Amini, University of Kurdistan, Iran
Copyright © 2024 Feng, Ameer, Jiang and Ramachandraiah. This is an open-access article distributed under the terms of the Creative Commons Attribution License (CC BY). The use, distribution or reproduction in other forums is permitted, provided the original author(s) and the copyright owner(s) are credited and that the original publication in this journal is cited, in accordance with accepted academic practice. No use, distribution or reproduction is permitted which does not comply with these terms.
*Correspondence: Guihun Jiang, jiangguihun@163.com; Karna Ramachandraiah, kramac@lsuhsc.edu
†These authors have contributed equally to this work