- 1Division of Environment Science, ICAR-Indian Agricultural Research Institute, New Delhi, India
- 2Division of Agronomy, ICAR-Indian Agricultural Research Institute, New Delhi, India
- 3Division of Plant Physiology, ICAR-Indian Agricultural Research Institute, New Delhi, India
- 4Division of Water Science and Technology, ICAR-Indian Agricultural Research Institute, New Delhi, India
- 5ICAR-National Bureau Agriculturally Important Microorganisms, Mau, Uttar Pradesh, India
- 6Krishi Vigyan Kendra, Sardar Vallabhbhai Patel University of Agriculture and Technology, Meerut, Uttar Pradesh, India
Natural Farming represents an agro-ecological methodology for farming that emphasizes regenerative practices with an aim to promote holistic ecological balance and reduce the dependence on external inputs as well as financial resources. Substantial concern has recently arisen over the need to promote agroecosystems that are more sustainable in order to improve the deteriorating soil health as well as reversing the yield plateau of crop. So, the current on farm field experiment was executed comprising of 8 treatments with different combination of natural farming inputs (Ghanjeevamrit, Jeevamrit, Beejamrit), organic fertilizer (such as FYM), integrated nutrient management (NPK, FYM, Azotobacter and Azolla) and in-organic(NPK) to examine and compare the consequence of natural farming inputs, organic fertilizer and in-organic dosage of fertilizer on soil nitrogen uptake, soil physicochemical properties, soil biological properties, soil microbial population and crop yields in a rice-wheat cropping system over two crop seasons 2021–23 [rice (Pusa-1509) and wheat (HD-3086)]. The study results demonstrated that there was significant (p < 0.05) increase in the soil’s nitrogen availability and nitrogen uptake with the use of natural farming inputs as compared to control treatment, whereas, natural farming treatments (TNF1, TNF2, TNF3, TMNF) were inferior than integrated nutrient management (TINM) and recommended doses of fertilizer (TRDF) treatment in case of nitrogen uptake by both rice and wheat crop. The soil enzymatic activity (Dehydrogenase, β-glucosidase, and urease), soil microbial biomass carbon and nitrogen, and soil microbial population (Bacteria, fungi, and actinomycetes) were significantly (p < 0.05) higher in treatment receiving natural farming inputs compare to in-organic fertilizer and organic fertilizer. A positive and significant correlation was observed between potential mineralization nitrogen and soil enzymatic activity (Dehydrogenase, β-glucosidase, and urease), soil microbial biomass carbon and nitrogen and soil microbial population (Bacteria, fungi, and actinomycetes). The crop yield at the end of experiment recorded to be highest in treatment TINM (75% RDF (In-organic) + 25% RDF (FYM) + BGA) i.e., (Rice- 4.76 t/ha and Wheat- 5.82 t/ha) compared to TRDF and TNF. A crop yield reduction of 14.2% was observed in treatment receiving natural farming inputs compare to TINM. A significant increase in crop yield was observed in TMNF (Jeevamrit (25%) + Ghanjeevamrit (25%) + 50% RDF through FYM + Beejamrit) compare to Tc (Control) and TFYM (Farmyard manure). Therefore, our study suggests that adoption of natural farming inputs over time can facilitate the enhancement of soil biological health of Inceptisol of Trans Gangetic Plain of India.
1 Introduction
Green revolution refers to a period of significant agricultural advancement in India that took place from mid-20th century onwards. As green revolution can be seen as subset of conventional farming practices, it was promoted and implemented on a large scale with the objective to increase food production and to address food shortage. It introduces modern farming techniques and technologies which include use of synthetic fertilizers, pesticides, herbicides and farm mechanization (John and Babu, 2021). Even though green revolution has effectively accomplished its core objective of augmenting agricultural productivity and ensuring food self-sufficiency in the country. But the exclusive dependence on inorganic agrochemical has engendered significant adverse repercussions on the environment. For instance, the widespread usage has resulted in soil degradation, depletion of soil microbiota and environmental pollution (Pingali, 2012). Livestock husbandry and the application of nitrogen-based fertilizers stand as the primary factors responsible for agriculture’s direct contribution to 20% of the country’s greenhouse gas emissions (Vetter et al., 2017; Anas et al., 2020). According to Craswell (2021), these fertilizers serve as the principal catalyst for nitrate contamination in surface water ecosystems. Land degradation is also underway in more than 30% of the nation’s entire geographic area (Kabiraj et al., 2022). The strain from the environment has a damaging impact not only on agriculture output and natural resources but also on human health as well. A significant variation in yield of agricultural crops is observed despite inculcating the best practices and intensive use of farm inputs. Additionally, the quality of agricultural land is declining, which increases the threat to the agro-ecology and soil resources. These perils encompass species extinction, desertification, climate change, as well as soil, air, water, and food supply contamination (Gupta, 2019). Synthetic agro-chemical impact human wellness, because residues from in-organic fertilizer used in crop and soil gets biomagnified and ultimately enter the digestive tracts of individuals who consume these dietary items. As a result, the human body is showing adverse effects on health, including interference of the immune, neurological, and hormonal systems (Nicolopoulou-Stamati et al., 2016). Every nation grappling with issues of poverty, starvation, and undernourishment will be compelled to accelerate agricultural expansion as a means to attain sustainable development goals, particularly as they endeavor to achieve the eradication of poverty, the elimination of hunger, and the establishment of a salubrious environment for all (Paroda, 2018). There is growing fear that any further efforts to maintain this chemical agriculture model will be futile in the long run and irreparably harm soil health. Agriculture’s sustainability is one of the world’s top issues right now. Returning to non-chemical agriculture has become crucial to achieving production sustainability. Organic farming is a methodology rooted in the principles and dynamics of living organisms, wherein all constituents, including soil, plants, farm animals, microorganisms, insects, farmers, and others, exhibit interdependence. According to Nagavani and Subbian (2015), using organic manures in conjunction with inorganic fertilizers is frequently seen as the answer to maintaining high levels of productivity while maintaining environmental safety. In the hunt for more farmer-friendly as well as eco-friendly alternative systems of farming. Several environmentalists, among them Subhash Palekar from India, Chao from Korea, and Masanobu Fukuoka from Japan, introduced the concept of natural farming, commonly referred to as zero budget natural farming (ZBNF) in India. Natural farming is an agro-ecological approach to agriculture that promotes the cultivation of plants in symbiosis with their surrounding ecosystem. The two main axes of natural farming are structural and agronomic. It entails the enrichment of soil fertility through the application of diverse agro-ecological principles, including strategies like crop diversification, mulching with crop residues, nutrient recycling, fostering beneficial biotic interactions. Simultaneously, it imposes limitations on the utilization of synthetic fertilizers and external inputs (Palekar, 2006). Contrarily, NF focuses on removing farmers’ dependence on outside inputs and financial markets in order to provide them autonomy by forbidding them to make any purchases from outside parties, particularly businesses (Rosset and Martínez-Torres, 2012). Natural farming inputs have a high microbial population, which when used enhances the soil flora and mineralizes the macro- and micronutrients in the soil so that plants can use them and provides a sustainable crop yield. Natural farming experienced a huge surge across the country with numerous states like Andhra Pradesh, Odisha, Himachal Pradesh, Chhattisgarh, Madhya Pradesh etc., adopting natural farming as state policy or a grassroots movement, Khadse et al. (2018). It requires scientific confirmation in terms of its impacts on production in various agro-climatic situations, cropping systems, and soil types in order for multiple state governments to accept natural farming as one of their state policies. It may be best to employ natural farming methods in conjunction with crop diversification along with intercropping to boost crop yield stability, preserve soil health as well as fertility and lower greenhouse gas emissions. With this perspective in consideration, the present investigation was conducted to examine the impact of natural farming on soil quality, nutrient absorption, and crop yield. The primary objective of the study was to.
Evaluate the impact of organic and natural farming inputs (Ghanjeevamrit, Jeevamrit, and Beejamrit) application in comparison with conventional management, on soil health, nitrogen availability and crop yield.
2 Material methods
2.1 Experimental site
The experiment was undertaken at a farm owned by the farmer (coordinates 28.53° N, 77.64° E) as illustrated in Figure 1, spanning the three-year period from 2021 to 2023. This farm is affiliated with the Indian Council of Agricultural Research (ICAR)-Krishi Vigyan Kendra (KVK), located in Gautambudh Nagar, Uttar Pradesh. The farm is situated at an approximate elevation of 200 m above sea level and experiences an average annual precipitation of 700 mm. The climate in this geographical area falls within the category of sub-tropical and semi-arid, characterized by an annual average temperature of 26 °C. The soil exhibited a sandy clay loam texture, with the following major properties at a depth of 0–20 cm: 46% sand, 33% silt, 20% clay, a pH of 8.3, a bulk density of 1.39 g/cm^3, and an organic carbon content of 0.47%. The initial soil nutrient levels, determined prior to seed sowing, were as follows: low available nitrogen (238 ± 0.5 kg/ha), medium levels of available phosphorus (53 ± 0.2 kg/ha), and available potassium (120 ± 0.8 kg/ha). The experiment involved a rice-wheat (Oryza sativa-Triticum aestivum) cropping system, utilizing Pusa 1,509 rice and HD-3086 wheat varieties. The experimental trial started with Rice (Kharif season-2021-22) till Wheat (Rabi season-2022-23). Before the initiation of the experimental trial, the site had been under continuous organic cultivation of cereals (rice and wheat) and legumes (mungbean) in rotation for more than 6 years. During the years 2021–22 and 2022–23, the total recorded rainfall was 16.3 mm and 18.7 mm, respectively. The average maximum temperatures during the growing seasons of 2021–22 and 2022–23 were 41.1°C and 42.2°C, while the average minimum temperatures were 10.2°C and 9.8°C, as depicted in Figure 2.
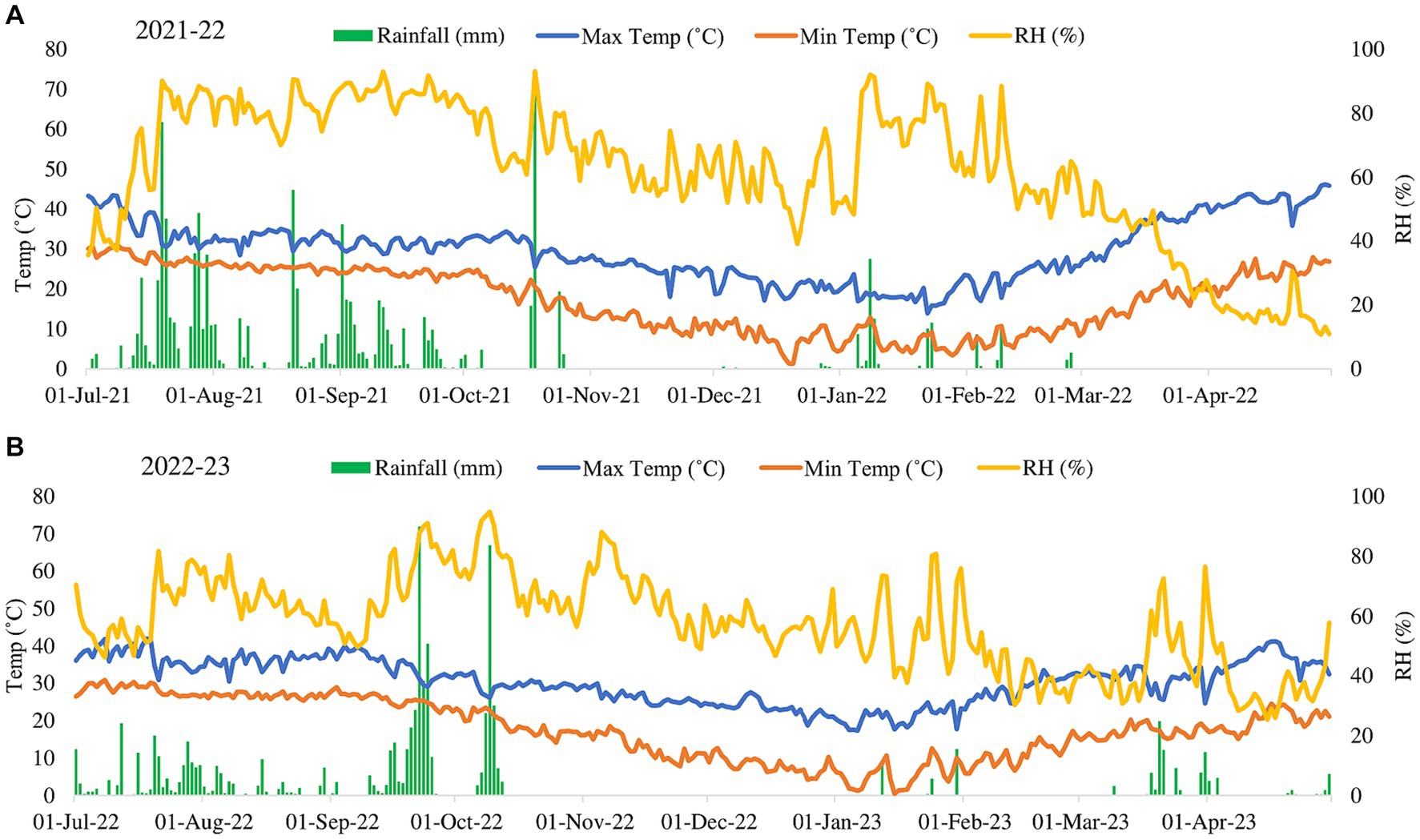
Figure 2. A and B explain as weather condition of the site of experiment during year 2021-22 and 2022-23 respectively.
2.2 Experimental design and treatments
The experimental field trial was executed following a randomized block design (RBD) with three replications, encompassing eight distinct treatments, namely Tc (No fertilizer), TRDF (NPK @ 150:60:40 kg/ha), TINM [75% Recommended dose of fertilizer (in-organic) + 25% Recommended dose of fertilizer (FYM) + BGA (Azotobacter for wheat) & (Azolla for rice)], TFYM (Recommended dose of fertilizer through FYM), TNF1 (Jeevamrit @ 1,000 L ha−1 in 3 split + Mulching), TNF2 (Ghanjeevamrit @ 1,000 kg ha−1 in 3 split + mulching), TNF3 (Jeevamrit (50%) + Ghanjeevamrit (50%) in 3 split + Mulching), TMNF (Jeevamrit (25%) + Ghanjeevamrit (25%) + 50% RDN through FYM in 3 split + Beejamrit + mulching). Conventional tillage has been applied from Tc to TFYM and minimum tillage in TNF1 to TMNF. The experimental field, situated within the affiliation of KVK-Gautambudhnagar, Uttar Pradesh. The Control treatment group has deliberately not received any organic treatments, facilitating the establishment of a rigorous and credible basis for comparison with the above designated treatment groups across various parameters. The detailed treatment information has been given in (Table 1). The nitrogen fertilizer, in the form of urea containing 46% nitrogen (N), was applied in three stages: 50% at sowing, 30% during tillering, and 20% at flowering. Phosphorus fertilizer, specifically Di-ammonium phosphate with 46% P2O5, was exclusively applied during the seedling stage. Potassium fertilizer, using potassium chloride with 50% K2O, was evenly distributed between the seedling and earring stages. The nutrient concentration of these input applications for different treatments is detailed in Table 2. Each experimental plot covered an area of 20 m2 (5 × 4).
2.3 Method of soil sample collection and analysis
Fresh soil samples were collected from the 0–15 cm soil layer at three distinct locations within each treatment, employing an 8 cm tube auger. This sampling procedure was conducted during multiple growth stages of the crop, including tillering, flowering, grain filling, and physiological maturity. In total, 24 fresh soil samples were gathered, air-dried for a duration of 7 days, passed through a 2 mm sieve, thoroughly mixed, and stored in plastic bags for subsequent analysis. For soil enzymatic activities, microbial biomass carbon, nitrogen and microbial population analysis fresh soil sample from anthesis stage was collected. The analysis of various soil properties was conducted in accordance with established standard procedures. The available nitrogen content was determined using the Kjeldahl digestion method (Kjeldahl, 1883). Available soil phosphorus and potassium were assessed using Olsen’s method and the ammonium acetate method, respectively (Hanway and Heidel, 1952; Olsen, 1954). Soil organic carbon content was determined through the Walkley and Black Method (Walkley and Black, 1934). The initial soil properties of the experimental field are presented in (Table 3).
2.4 Analysis of plant samples
The nitrogen content in both the grain and straw components was assessed through the Kjeldahl digestion method (Jackson et al., 1973). To estimate nitrogen uptake, the nutrient concentrations, expressed as percentages, were multiplied by the corresponding yields of grain and straw. The total nutrient quantity extracted by the crop was determined by summing the nutrient uptake from both grain and straw, as illustrated in Equation (1).
2.5 Potential nitrogen mineralization
Potential nitrogen mineralization of soil was analyzed by using anaerobic incubation method (Keeney, 1982). Collect the representative soil sample ensuring sufficient volume for analysis. Air dry the sample and pass it through 2 mm sieve the place 100 g of sample into glass jar and tightly close the lid to create anaerobic condition. The soil samples the incubated at 35°C for 1 week. Collect the soil sample from jar and use Kjeldahl method to measure the mineralized nitrogen in the soil sample.
2.6 Soil microbial properties
2.6.1 Soil biomass carbon
Soil microbial biomass carbon (MBC) was determined in accordance with the protocol delineated by Vance et al. (1987). A soil sample weighing 17.5 grams was collected from each treatment and placed in a hermetically sealed container. One milli liter of chloroform was introduced to the sample, which was subsequently fumigated. Additionally, a non-fumigated set was prepared in a 250 mL flask. These samples were kept in the dark for 24 h. Following incubation, chloroform was evaporated at 50°C within a Biological Oxygen Demand (BOD) chamber, and the caps were left open for the ensuing 20–24 h. Subsequently, 70 mL of a 0.5 M K2SO4 solution was added to these samples, and they were subjected to agitation for 30 min. The supernatant was isolated by filtering the samples through Whatman No. 42 filter paper. The absorbance of the supernatant was promptly assessed at 280 nm for both fumigated and non-fumigated samples. The MBC of the soil samples was then computed using the formula provided below. Equation (2) was employed for calculating the carbon content of the microbial biomass.
2.6.2 Soil biomass nitrogen
The method proposed by Vance et al. (1987) is widely acknowledged and frequently employed for the expeditious retrieval and measurement of microbial biomass nitrogen in soil. In the experimental procedure, representative soil samples are obtained from each treatment plot. Approximately 10 g of soil is weighed into a fumigation chamber, followed by the addition of 30 mL of chloroform. The chamber is sealed, and thorough shaking ensures even distribution of chloroform. Subsequently, the soil undergoes chloroform fumigation for 24–48 h, leading to the effective termination of microbial biomass. A distinct portion of the soil sample is preserved without fumigation, serving as a non-fumigated control. Following fumigation, nitrogen extraction from both fumigated and non-fumigated samples is carried out using a suitable extractant solution, such as 0.5 M K2SO4. Vigorous shaking is employed to facilitate the extraction of nitrogen. The nitrogen concentration in the extracts is then determined using the Kjeldahl digestion method. The calculation of Microbial Biomass Nitrogen (MBN) for the soil samples is executed using a provided formula, with Equation (3) specifically utilized for computing the nitrogen content of the microbial biomass. This systematic approach ensures a comprehensive evaluation of microbial biomass nitrogen in the soil.
2.6.3 Soil enzymatic activity
Soil samples for enzymatic analysis were collected at the anthesis stage of both the rice and wheat crops. The freshly obtained soil samples were promptly sifted through a 2 mm sieve. Subsamples were extracted for moisture content evaluation, which was determined by measuring the loss in weight after subjecting the subsample of soil to drying at 105°C for a period of 48 h. The assessment of enzymatic activity was performed on all treatment samples in triplicate, with an additional sample serving as the control. The enzymes under investigation included dehydrogenase, β-glucosidase, and urease, and their quantification was carried out through colorimetric analysis of reaction products resulting from the incubation of samples with the appropriate substrate under standardized conditions. The dehydrogenase activity was assessed following the procedure proposed by Casida et al. (1964). This enzymatic activity was determined by measuring the production of triphenyl formazan from triphenyl tetrazolium chloride, which served as a hydrogen acceptor. In this method, 1 gram of air-dried soil was carefully weighed and placed in a 15 mL screw cap tube. Three replicates were maintained for each experimental treatment. To these tubes, 0.2 mL of a 3% w/v solution of 2, 3, 5 triphenyl tetrazolium chloride was added. To ensure a thin layer of water on the soil surface, 0.5 mL of a 1% glucose solution was included. The tubes were then incubated at a controlled temperature of 30 ± 1°C. After 24 h of incubation, 10 mL of methanol was introduced, and the tubes were manually shaken for 1 min. Subsequently, these tubes were left in the dark for 6 h. The developed color intensity was quantified using a spectrophotometer set at 485 nm. Dehydrogenase activity was expressed as milligrams of Triphenyl formazan (TPF) produced per hour per gram of air-dry soil. A standard curve was constructed within a range of 0.005 mg TPF to 0.4 mg per 10 mL of methanol.
β-Glucosidase activity was assessed following the protocol outlined by Eivazi and Tabatabai (1988). In this procedure, Modified Universal Buffer (MUB), toluene, and p-nitrophenyl β-D-glucoside solution each of 4 mL, 0.2 mL and 1 mL were incubated, respectively, with 1 gram of soil at 37°C for 1 h. Subsequently, 0.5 M CaCl2 and 0.1 M THAM (Tris hydroxy methyl amino methane) each of 1 mL and 4 mL, respectively, were added, and the resulting soil solution was filtered using Whatman No. 1 filter paper. The intensity of yellow color solutions was assessed using a spectrophotometer at a wavelength of 490 nm. These measurements were then compared to a calibration curve established from standards that contained varying quantities of p-nitrophenol, specifically 0, 10, 20, 30, 40, and 50 mg per gram of soil, as measured at 490 nm. The comparison was made with respect to the reference readings from the established standards.
The quantification of urease activity in soil entails the assessment of urea hydrolysis by examining the remaining urea content subsequent to the soil’s incubation with a urea solution at a temperature of 37°C, following the method introduced by Bremner and Douglas (1971). The amount of urea that remains after a specific incubation period is used as the basis for estimating urease activity. Urea content is determined through a calorimetric method, which involves the chemical reaction of urea with diacetyl monoxime (DAM) in the presence of Thio-Semi carbazide (TSC), H3PO4, and H2SO4. Subsequently, the intensity of the resulting red coloration was quantified using a spectrophotometer at a wavelength of 527 nm. The calculation of soil urease activity was carried out according to Equation (4) below, where “B” represents the quantity (mg) of urea initially added, “A” signifies the amount (mg) of urea remaining after the time “t,” and “x” corresponds to the oven-dried quantity (in grams) of soil used during incubation. When time is measured in hours, urease activity can be expressed as milligrams of urea per gram of soil (mg urea g−1 soil).
The Geometric Mean (GMenz) of enzyme activity amalgamates data from all enzymes, computed for each treatment, employing the subsequent Equation (5), as previously described by Hinojosa et al. (2004).
2.6.4 Culturable soil microbial population
The quantification of viable microorganisms in rhizospheric soils was performed out using the serial dilution method, and the values were expressed as colony forming units (CFUs) per gram of soil, as described by Wollum et al., (1994). Rhizospheric soil samples were systematically diluted by mixing 10 g of soil with 90 mL of sterilized distilled water, with subsequent dilutions up to 10−8. For the quantification of bacteria, fungi, and actinobacteria, nutrient agar (comprising Peptone, Yeast Extract, Sodium Chloride, Agar) each added 5 g, 2 g, 5 g 15 g, respectively, and add sufficient distilled water to make up the volume by 1,000 mL, Potato Dextrose Agar (containing Potatoes, Dextrose, Agar) added 200 g, 20 g and 15 g respectively, and Actinomycetes isolation agar (with Sodium caseinate 2.0, L-Asparagine 0.10, Sodium propionate 4.0, Dipotassium phosphate 0.5, Magnesium sulfate 0.1, Ferrous sulfate 0.001, Agar 15.0) media from Himedia® were employed, respectively. Subsequently, the soil suspensions from the 10−5, 10−4, and 10−3 dilutions were spread onto petri plates in triplicates containing the respective media for bacterial, fungal, and actinobacterial counts. Bacterial, fungal, and actinomycetes colonies were enumerated after 1, 3–4, and 6–7 days of incubation at 28 ± 2°C, respectively.
2.7 Crop yield
The rice and wheat crops were harvested in October and April, respectively, and subsequently threshed to quantify their grain and straw yields per hectare, with moisture content adjusted to 12%.
2.8 Statistical analysis
The measurements acquired from the experimental study underwent analysis using OPSTAT Software, as described by Sheoran et al. (1998), to compute ANOVA. Subsequently, means were differentiated through Duncan’s multiple range test (DMRT) at a significance level of p = 0.05. Each variable’s data underwent assessment using a variance protocol analysis within a randomized block design, subject to verification for statistical significance via the “F” test, following the approach outlined by Gomez and Gomez (1984). The evaluation of the impact of different treatments on crop yield and soil microbial population was carried out using R software, version 4.3.1, as specified by R Development Core Team (2012). The standard error of means (SEm) and the least significant difference (LSD) were computed at a significance level of 5%. The correlation panel matrix was constructed using the “ggplot2” and “corrplot” packages within the R software, version 4.3.1.
3 Results
3.1 Soil chemical properties (pH, electrical conductivity, and soil organic carbon)
The results of the analysis of selected soil chemical properties are presented in (Table 4). Following the harvest of rice and wheat, soil pH and electrical conductivity (EC) exhibited no significant variations when different natural farming inputs were applied. However, a slight decrease in pH was observed in the TRDF treatment. Conversely, there was a marginal increase in these values across all other treatments over the years from 2021 to 2023. Notably, the application of various natural farming inputs significantly influenced the organic carbon content of the soil after the crop harvest. Specifically, the TNF3 treatment exhibited a significantly higher soil organic carbon content, measuring 0.51 and 0.58% for the years 2021–22 and 2022–23, respectively. This was on par with the TMNF treatment, followed by TNF1 and TNF2, which recorded values of 0.55 and 0.56%, respectively, at the end of the experiment. These values were statistically similar. In contrast, the control treatment displayed the lowest organic carbon content, with measurements of 0.40 and 0.42% for the years 2021–22 and 2022–23, respectively.
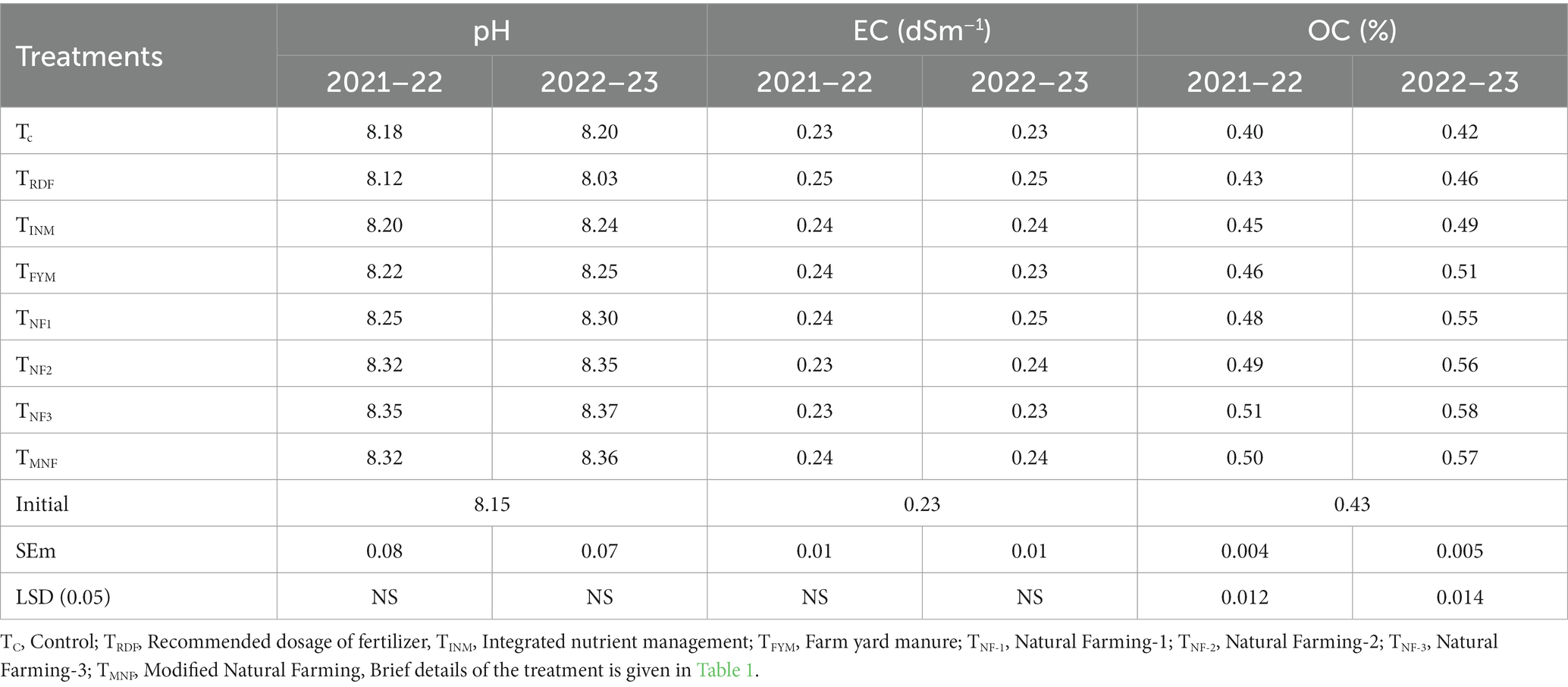
Table 4. Effect of different natural farming inputs (Ghanjeevamrit, Jeevamrit, and Beejamrit), organic (FYM) and in-organic fertilizer on soil chemical properties at harvest of wheat crop of 2021–22 and 2022–23.
3.2 Soil available nitrogen, phosphorus, and potassium
During both the agricultural seasons (2021–22 and 2022–23) of the rice-wheat cropping system, it was observed a noteworthy alteration in the levels of available nitrogen subsequent to the harvest of both rice and wheat crops. The treatment designated as “TINM” consistently exhibited the highest recorded levels of available nitrogen, registering values of 234.9 and 241.3 kg per ha−1 after the wheat harvest for the respective years 2021–22 and 2022–23. In contrast, the treatments denoted as TNF1, TNF2, and TNF3 consistently displayed significantly lower concentrations of available nitrogen following the wheat harvest, in comparison to the TINM treatment. Among the various natural farming inputs employed, the “TMNF” treatment consistently demonstrated the highest levels of available nitrogen for both years, with values of 229.3 and 237.8 kg ha−1 after the wheat harvest for the years 2021–22 and 2022–23, respectively. Specifically, the TINM treatment exhibited a percentage increase in soil-available nitrogen of 2.42 and 1.47% compared to TMNF after the wheat harvest for the years 2021–22 and 2022–23, respectively. It is noteworthy that the “TRDF” treatment consistently exhibited soil-available nitrogen values statistically comparable to those of the TINM treatment following both seasons of the rice-wheat cropping system. Conversely, the control treatment (Tc) consistently displayed the significantly lowest levels of available nitrogen. In comparison to TINM, TINM exhibited a percentage increase of 61.6 and 72.2% in soil-available nitrogen following the wheat harvest for the years 2021–22 and 2022–23, respectively (Figure 3).
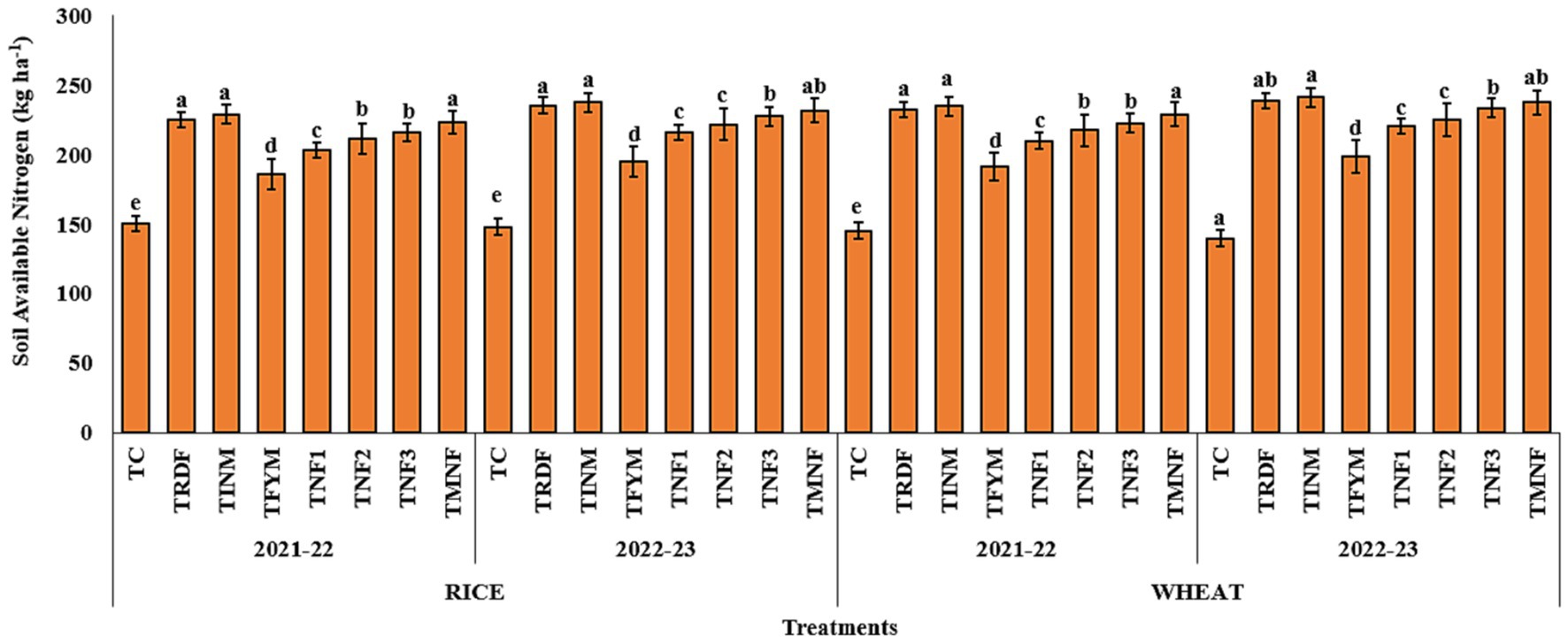
Figure 3. Effect of different nutrient management practices on soil available nitrogen. [TC: Control, TRDF: Recommended dosage of fertilizer, TINM, Integrated nutrient management; TFYM, Farm yard manure; TNF-1, Natural Farming-1; TNF-2, Natural Farming-2; TNF-3, Natural Farming-3; TMNF, Modified Natural Farming, Brief details of the treatment is given in Table 1]. Data refer to mean of three replicate and error bars depicts standard deviation. Bars with the same alphabet are not significantly different at p ≤ 0.05. Different alphabet indicates statistically significant differences within each treatment at p < 0.05 (Duncan test).
The available phosphorus content in the soil exhibited notable enhancements in treatments incorporating natural farming inputs, i.e., TNF1, TNF2, TNF3, and TMNF. In particular, the application of TMNF resulted in significantly elevated levels of available phosphorus after 2 years, amounting to 64.6 and 68.3 kg ha−1 after the wheat harvest for the years 2021–22 and 2022–23, respectively. These values were statistically akin to those observed in the TRDF and TINM treatments. Conversely, the TFYM treatment displayed lower levels of available soil phosphorus for both years, post-wheat harvest, measuring 54.8 and 58.5 kg ha−1 in comparison to the other treatment regimens. During the years 2021–22 and 2022–23, TNF1 and TNF2 exhibited no statistically significant differences in soil phosphorus content after 2 years of crop harvest. The control treatment consistently manifested substantially reduced levels of available phosphorus, with values of 47.2 and 46.3 kg ha−1 after the wheat harvest, representing a reduction of 36.8 and 47.5% compared to TMNF for the respective years (Figure 4).
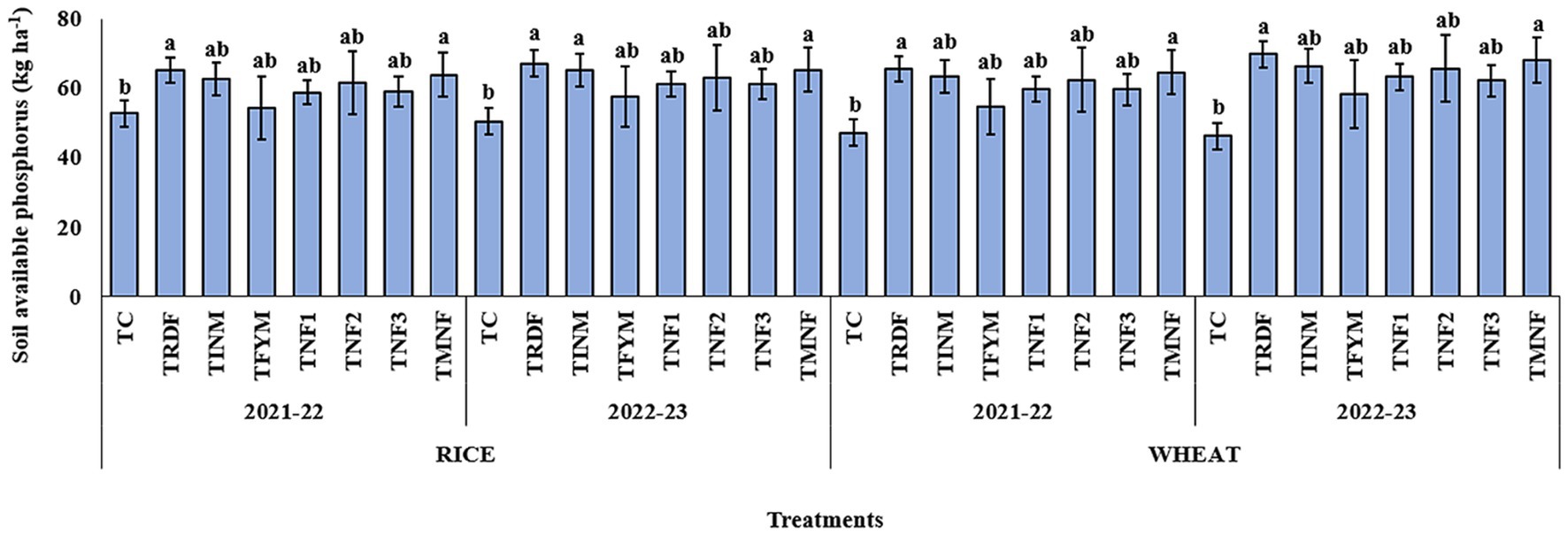
Figure 4. Effect of different nutrient management practices on soil available phosphorus. [TC, Control; TRDF, Recommended dosage of fertilizer; TINM, Integrated nutrient management; TFYM, Farm yard manure; TNF-1, Natural Farming-1; TNF-2, Natural Farming-2; TNF-3, Natural Farming-3; TMNF, Modified Natural Farming, Brief details of the treatment is given in Table 1]. Data refer to mean of three replicate and error bars depicts standard deviation. Bars with the same alphabet are not significantly different at p ≤ 0.05. Different alphabet indicates statistically significant differences within each treatment at p < 0.05 (Duncan test).
Diverse treatment approaches exerted significant influence on the soil’s available potassium content, as indicated in Figure 5. In the years 2021–22 and 2022–23, notably elevated levels of available potassium following the rice harvest were documented in the TINM treatment, registering at 135.6 and 139 kg ha−1 after the wheat harvest. These values demonstrated statistical similarity to those obtained in the TRDF treatment, followed closely by TMNF. TNF3 displayed levels statistically akin to TNF1 and TNF2 for both years, with available potassium quantities of 126.4 and 123.2 kg ha−1 following the wheat harvest for the years 2021–22 and 2022–23, respectively. In contrast, the control treatments consistently exhibited the most markedly reduced levels of available potassium, measuring 119.2 kg ha−1 after the wheat harvest in the second year of the crop.
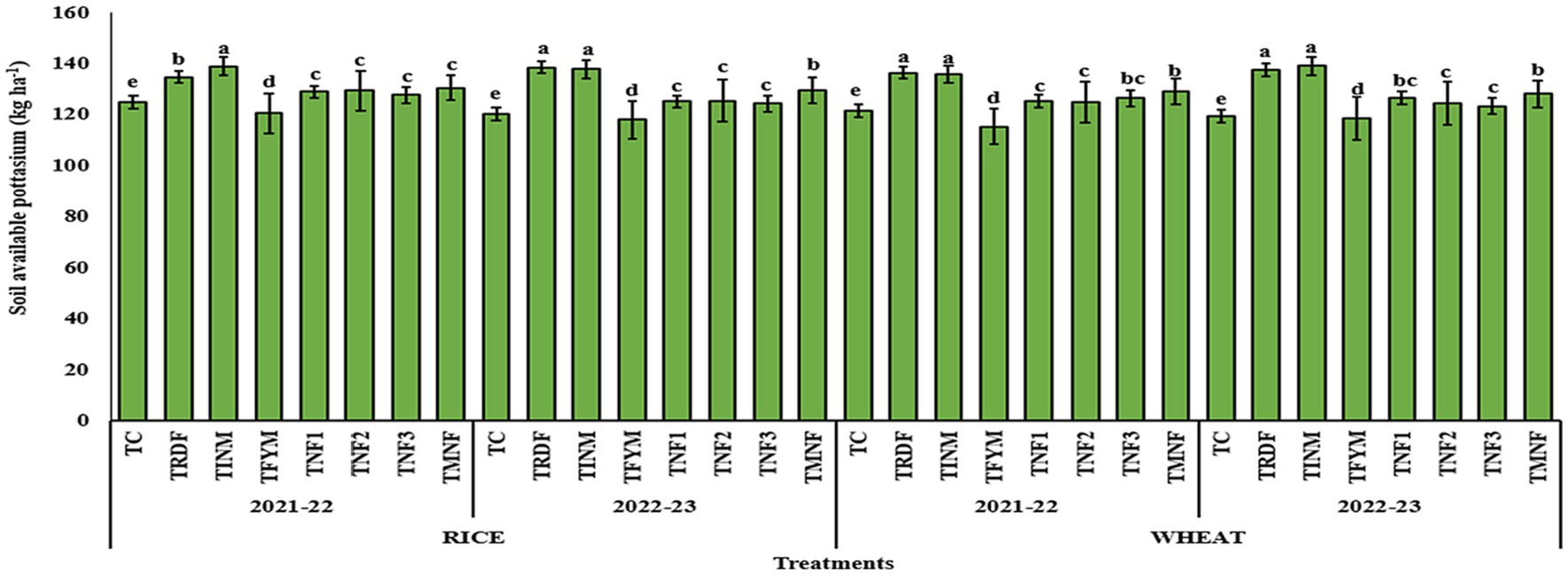
Figure 5. Effect of different nutrient management practices on soil available potassium. [TC, Control; TRDF, Recommended dosage of fertilizer; TINM, Integrated nutrient management; TFYM, Farm yard manure; TNF-1, Natural Farming-1; TNF-2, Natural Farming-2; TNF-3, Natural Farming-3; TMNF, Modified Natural Farming, Brief details of the treatment is given in Table 1]. Data refer to mean of three replicate and error bars depicts standard deviation. Bars with the same alphabet are not significantly different at p ≤ 0.05. Different alphabet indicates statistically significant differences within each treatment at p < 0.05 (Duncan test).
3.3 Potential nitrogen mineralization
The field management practices, including fertilization and crop rotation, had a significant impact on soil mineralized nitrogen, as depicted in Figure 6. The potential nitrogen mineralization exhibited extensive variation across different treatment groups and throughout the duration of the experiment. Relative to the control group (Tc), all other treatment groups demonstrated a noteworthy increase in potential nitrogen mineralization. The highest potential nitrogen mineralization, recorded after the 2022–23 wheat harvest, was observed in the TMNF treatment, with a value of 12.20 mg/kg. This represented a substantial 60.5% increase compared to the TRDF treatment, which had a potential nitrogen mineralization of 7.60 mg/kg. The TINM treatment exhibited a nitrogen mineralization value of 9.10 mg/kg. Among the treatments that received natural farming inputs (TNF1, TNF2, and TNF3), all showed statistically equivalent values in terms of potential nitrogen mineralization. In contrast, the lowest nitrogen mineralization was recorded in the control group, Tc, with a value of 4.5 mg/kg. This value was two-fold lower when compared to the TMNF treatment.
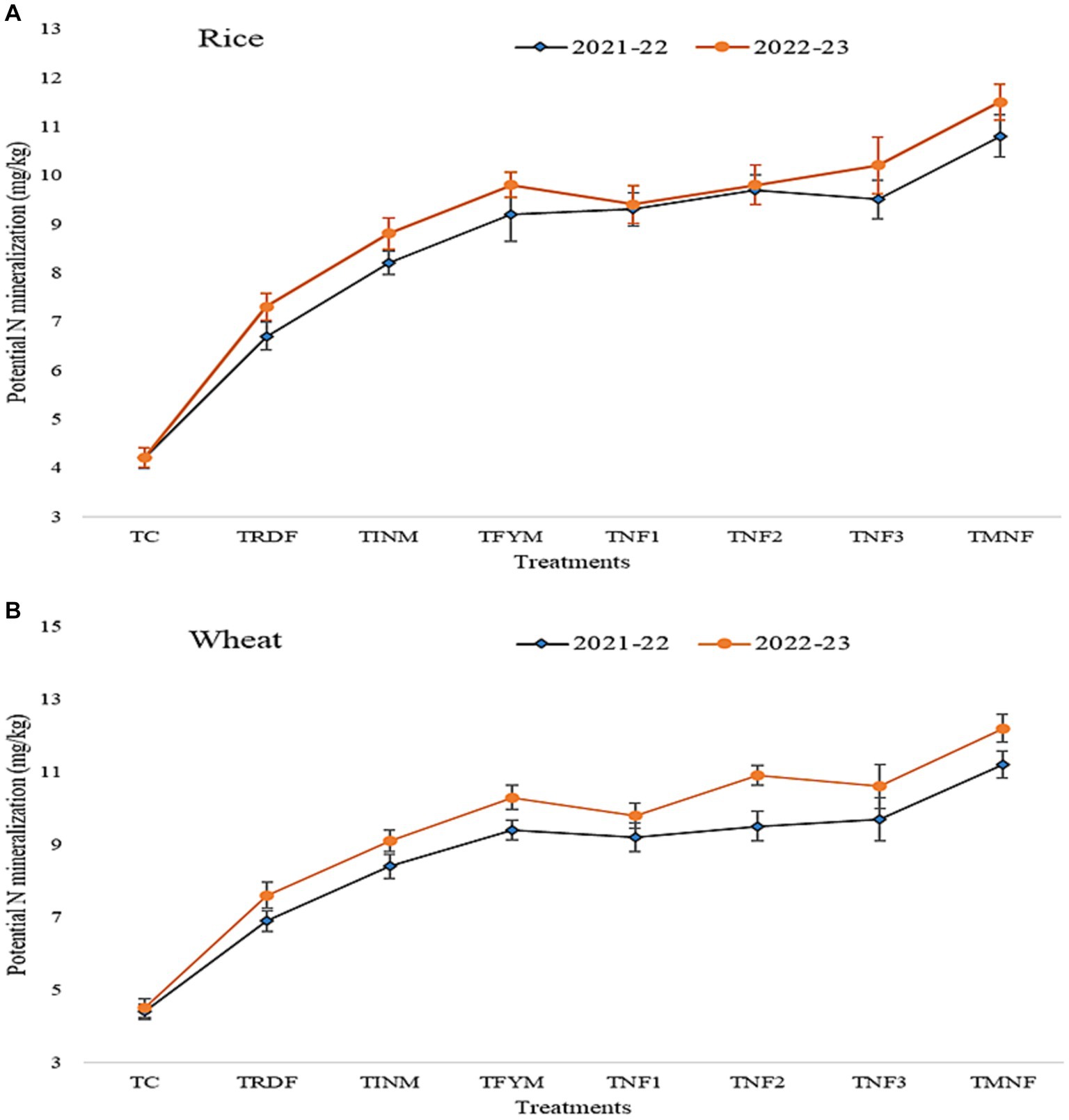
Figure 6. (A) and (B) explain as the effect different nutrient management practices on nitrogen mineralization for crop rice and wheat respectively. A is for Rice crop of 2021-22 and 2022-23 and B is for Wheat crop of 2021-22 and 2022-23.
3.4 Nitrogen uptake
The nitrogen uptake by both the rice and wheat crops exhibited considerable variation among the treatment groups across multiple years, as summarized in Figure 7. Nitrogen uptake from the soil reached its maximum value in the TINM treatment for both cropping seasons, regardless of the crop type. In the 2021–22 and 2022–23 seasons, TINM demonstrated the highest nitrogen uptake for both rice and wheat, with values of 124.09 and 127.57 kg ha−1 for rice and 160.19 and 170.98 kg ha−1 for wheat, respectively. Following TINM, TRDF recorded the second-highest nitrogen uptake, with values of 108.19 and 114.42 kg ha−1 for rice and 150.78 and 158.18 kg ha−1 for wheat in the same respective seasons. Notably, there were no statistically significant differences in nitrogen uptake between treatments TNF1 and TNF3, as well as between TINM and TNF2. The control treatments consistently exhibited the lowest nitrogen uptake during both years.
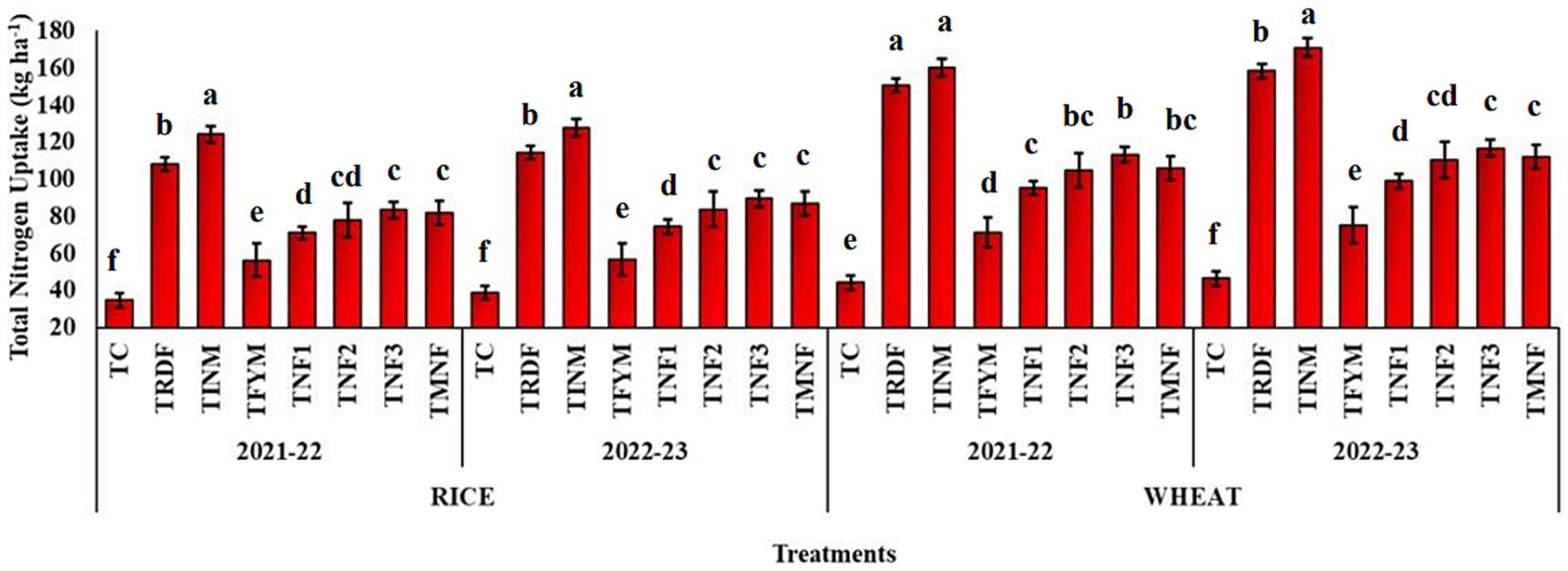
Figure 7. Effect of different nutrient management practices on total nitrogen uptake by rice and wheat during cropping season 2021–22 and 2022–23. [TC, Control; TRDF, Recommended dosage of fertilizer; TINM, Integrated nutrient management; TFYM, Farm yard manure; TNF-1, Natural Farming-1; TNF-2, Natural Farming-2; TNF-3, Natural Farming-3; TMNF, Modified Natural Farming, Brief details of the treatment is given in Table 1]. Data refer to mean of three replicate and error bars depicts standard deviation.
3.5 Soil biological properties
3.5.1 Soil microbial biomass carbon and nitrogen
Our experimental result revealed that treatment receiving natural farming inputs exert a substantial influence on the Microbial biomass carbon (MBC) and Microbial biomass nitrogen (MBN) content in soil. In the present investigation, specifically, the treatment denoted as TNF1, TNF2, TNF3, and TMNF exhibited a notably pronounced impact on the soil microbial biomass, as indicated in (Table 5). In both years under study, 2021–22 and 2022–23, the research trial yielded highest MBC values in the TMNF treatment for both rice (190.3 mg kg−1) and wheat (204.5 mg kg−1) during the last year of experiment. TNF3 also exhibited a relatively higher MBC content, which was statistically equivalent to that of TMNF. In the context of rice cultivation, TMNF demonstrated a substantial 44.9% increase in MBC when compared to the TRDF treatment. Similarly, during wheat cultivation, TMNF exhibited a substantial 53.5% increase in MBC relative to the TRDF treatment. Notably, analogous trends were observed for MBN content in the soil. The highest and lowest values for MBN were consistently recorded in the TMNF and TC treatments, respectively. During rice cultivation, TMNF displayed an MBN content of 88.9 mg kg-1, while TC had a content of 20.4 mg kg−1. During wheat cultivation, TMNF exhibited an MBN content of 95.4 mg kg−1, whereas TC displayed a content of 21.8 mg kg−1. This implies a remarkable percentage increase of 40.6% in MBN content during rice cultivation and an even more substantial 102.1% increase during wheat cultivation in the TMNF treatment compared to the TRDF treatment. Conversely, the lowest MBC levels were observed in the TC treatment, registering at 79.4 mg kg−1 and 78 mg kg−1 following the harvest of two consecutive rice and wheat seasons, respectively.
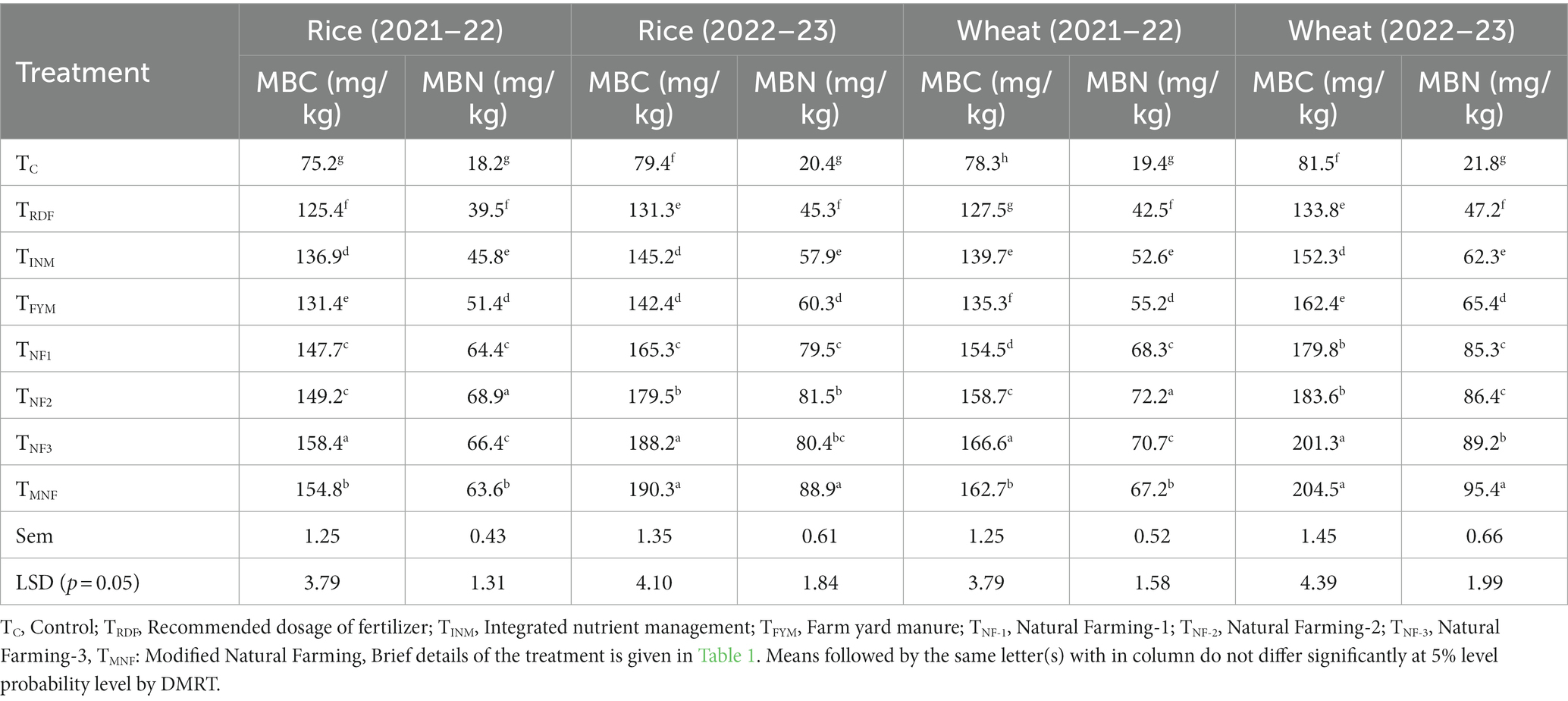
Table 5. Effect of different natural farming inputs (Ghanjeevamrit, Jeevamrit, and Beejamrit), organic (FYM) and in-organic fertilizer on soil MBC and MBN.
3.5.2 Soil enzymatic activity index
An important innovation in this study was the introduction of the integrated index GMenz, which serves as a dimensionless parameter facilitating the comparison of combined enzyme activity (dehydrogenase, β-glucosidase and urease) and soil sample quality. The GMenz values ranged from 7.85 to 32.83 across all treatments for both rice and wheat during a two-year experiment as shown in Figure 8. Over the course of this research, TMNF consistently exhibited the highest GMenz value, regardless of the crop type and year. At the end of the 2022–23 wheat crop season, TMNF displayed a remarkable 77.03% increase in GMenz value compared to TRDF, and a substantial 48.5% increase compared to TINM. In contrast, TC registered the lowest GMenz value, which was 7.99, making it four times lower than TMNF.
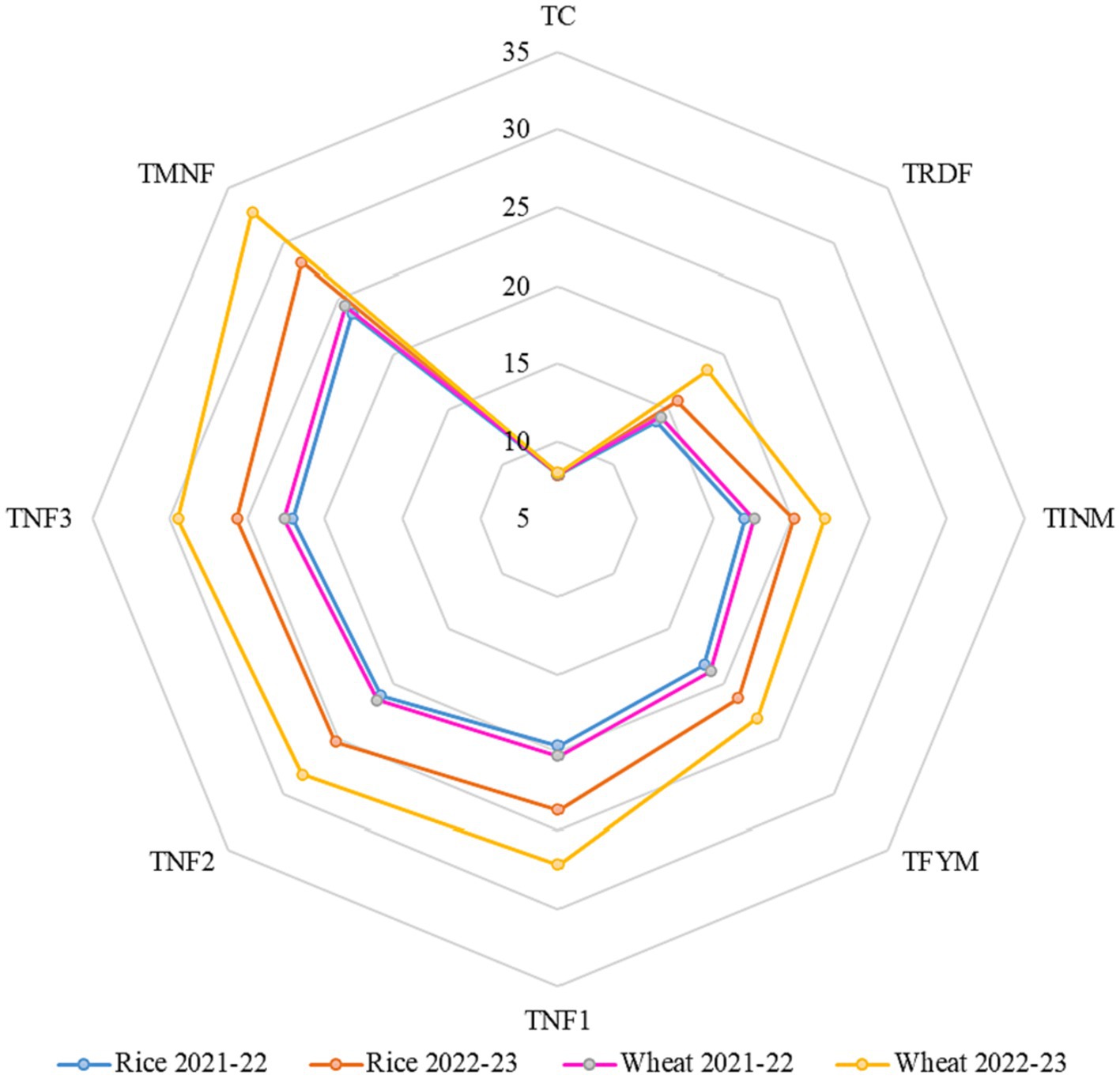
Figure 8. Effect of different nutrient management practices on the geometric mean of assayed enzyme activities (relative value). [TC, Control; TRDF, Recommended dosage of fertilizer, TINM, Integrated nutrient management; TFYM, Farm yard manure; TNF-1, Natural Farming-1; TNF-2, Natural Farming-2; TNF-3, Natural Farming-3; TMNF, Modified Natural Farming, Brief details of the treatment is given in Table 1].
3.5.3 Soil microbial population
Throughout the research conducted in both the years 2021–22 and 2022–23, the investigation revealed that the highest bacterial population was consistently observed in the TMNF treatment, with subsequent populations in TNF3, indicating (33.8 and 34.4 × 106 cfu g−1 soil) during rice-wheat crop in the respective years. Conversely, the TRDF treatment exhibited significantly lower bacterial populations, registering only (19.4 and 20.2 × 106 cfu g−1 soil) compared to all other treatments utilizing natural farming inputs. This data underscores a substantial 70.2% increase in bacterial population in TMNF as compared to the TRDF treatment during the final crop. It further elucidates the findings related to the fungal and actinomycetes populations in the soil for the years 2021–22 and 2022–23. Remarkably, the TMNF treatment consistently displayed the highest populations, recording 10.3 and 11.5 × 103 cfu g−1 soil for fungi and 23.5 and 24.8 × 105 cfu g−1soil for actinomycetes in the respective years. Following this, TNF3 exhibited the second-highest fungal population with 9.8 and 10.4 × 103 cfu g−1 soil, whereas TNF2 recorded the second-highest actinomycetes population with 23.6 and 24.8 × 105 cfu g−1 soil. Conversely, the control treatment consistently demonstrated the lowest fungal and actinomycetes populations, measuring only (4.1 and 4.3 × 103 cfu g−1 of soil for fungi and 11.4 and 11.7 × 105 cfu g−1 of soil) for actinomycetes during the respective years. These observations indicate a consistent increase in microbial counts over time. Importantly, when the organic inputs, including Farm Yard Manure (FYM), Ghanjeevamrit, Jeevamrit, and mulching, were applied collectively, the microbial population in the soil exhibited a much higher count than when any of these inputs were applied individually (Figures 9, 10).
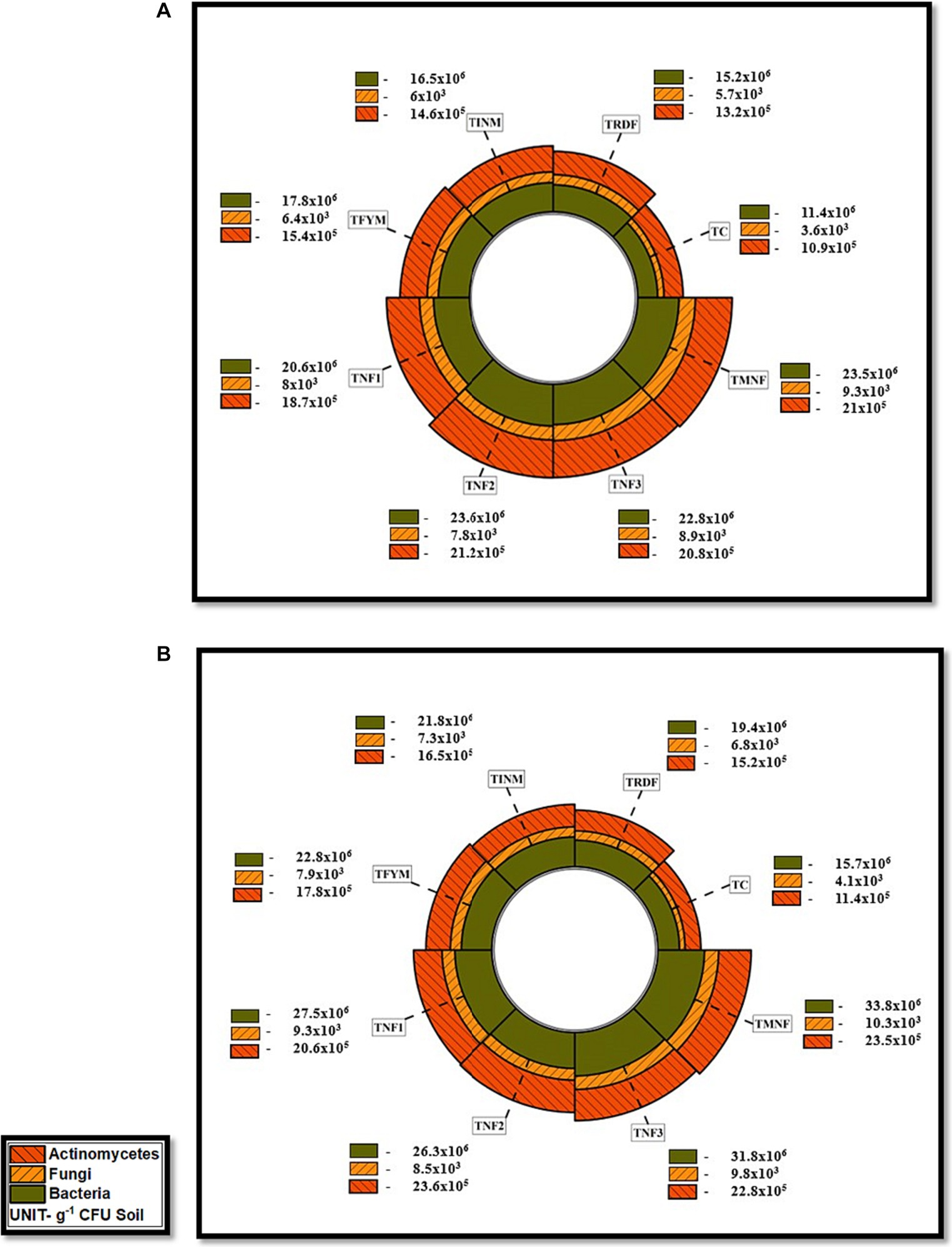
Figure 9. Effect of different natural farming inputs (Ghanjeevamrit, Jeevamrit, and Beejamrit), organic (FYM) and in-organic fertilizer on soil microbial population (A) rice crop (2021–22) and (B) rice crop (2022–23). LSD value (p > 0.05): for Bacteria 0.70 ×106and 0.67 ×106, Fungi 0.17 × 103 and 0.22 × 103 and Actinomycetes 0.43 × 105 and 0.50 × 105 in 2021–22 and 2022–23, respectively. [TC, Control; TRDF, Recommended dosage of fertilizer; TINM, Integrated nutrient management; TFYM, Farm yard manure; TNF-1, Natural Farming-1; TNF-2, Natural Farming-2; TNF-3, Natural Farming-3; TMNF, Modified Natural Farming, Brief details of the treatment is given in Table 1]. Data refer to mean of three replicates as shown in radial bar on a polar coordinate system.
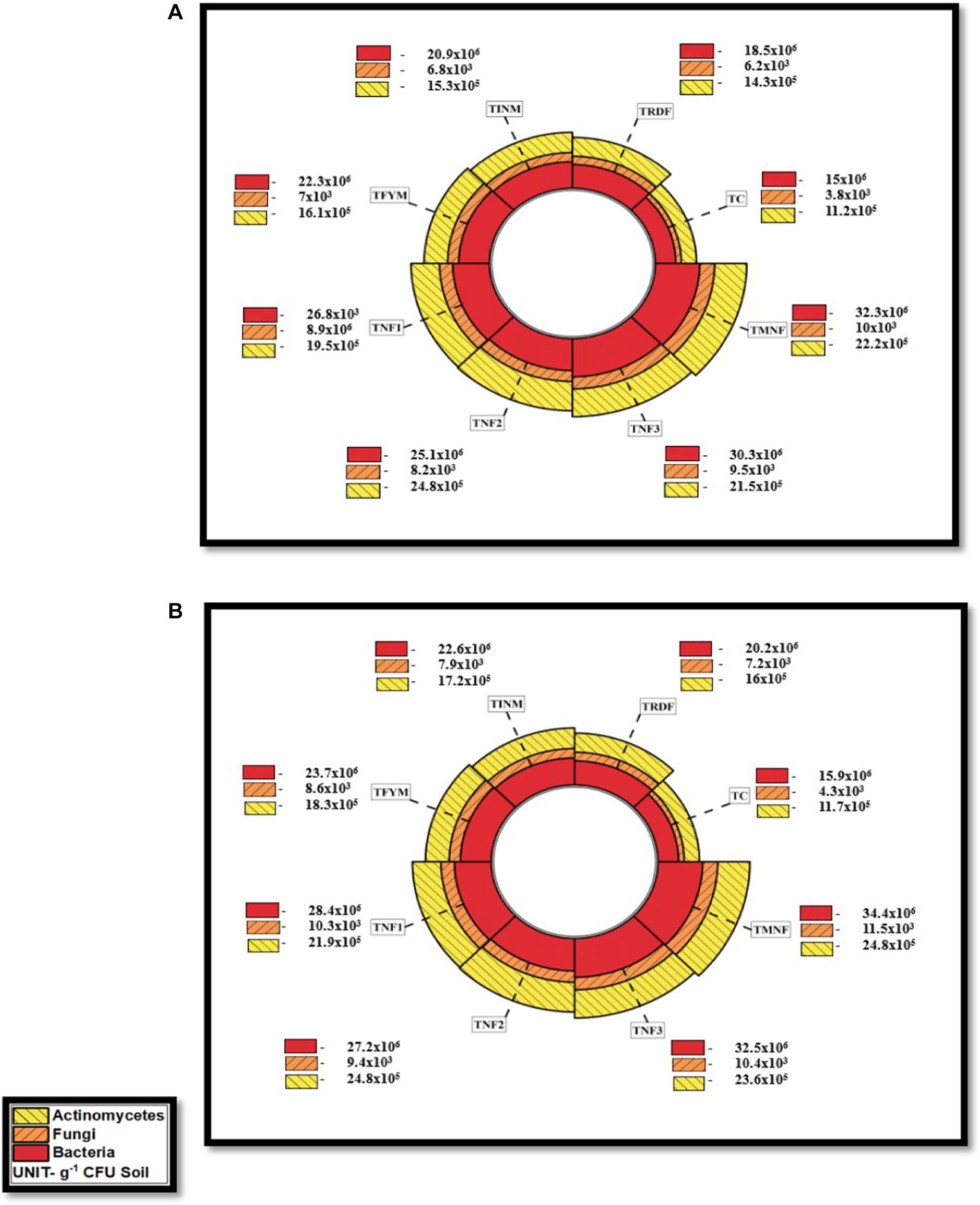
Figure 10. Effect of different natural farming inputs (Ghanjeevamrit, Jeevamrit, and Beejamrit), organic (FYM) and in-organic fertilizer on soil microbial population during (A) wheat crop (2021–22) and (B) wheat crop (2022–23). LSD value (p > 0.05): for Bacteria 0.74 × 106 and 0.69 × 106, Fungi 0.23 × 103, and 0.24 × 103 and Actinomycetes 0.52 × 105 and 0.53 × 105 in 2021–22 and 2022–23, respectively. [TC, Control; TRDF, Recommended dosage of fertilizer; TINM, Integrated nutrient management; TFYM, Farm yard manure; TNF-1, Natural Farming-1; TNF-2, Natural Farming-2; TNF-3, Natural Farming-3; TMNF, Modified Natural Farming, Brief details of the treatment is given in Table 1]. Data refer to mean of three replicates as shown in a radial bar chart on a polar coordinate system.
3.6 Crop yield
The outcome of a crop, which is often contingent upon the development of yield attributes, is primarily determined by the grain yield. The utilization of an integrated nutrient source substantially enhances the grain yield of rice and wheat. Particularly, during the end of the Kharif season in 2022, the highest grain yield, amounting to 4.76 t ha−1, was observed in the treatment denoted as TINM when compared to the TRDF treatment and other treatments employing natural farming inputs such as TNF1, TNF2, TNF3, and TMNF. Notably, TINM demonstrated a remarkable 9.3% increase in rice grain yield in comparison to TRDF, which yielded 4.31 t ha−1. Among the various natural farming inputs, TMNF yielded the highest grain yield at 4.08 t ha−1, although this result was not statistically distinguishable from the yield of TNF2, which amounted to 3.81 t ha−1. Over the course of the two-year experimental trial, spanning from 2021–22 to 2022–23, it was evident that significant differences in wheat yield existed among the various treatments. The highest wheat yield recorded at the end of the experimental trial was in the TRDF treatment, yielding 5.82 t ha−1, which was statistically equivalent to the yield of 5.70 t ha−1 observed in the TINM treatment. There was a notable decrease in yield observed in the treatments employing natural farming inputs. Among the natural farming inputs, TNF2 yielded the highest wheat yield at 4.19 t ha−1, which was not statistically distinct from the yield of 4.14 t ha−1 observed in the TMNF treatment (Figure 11).
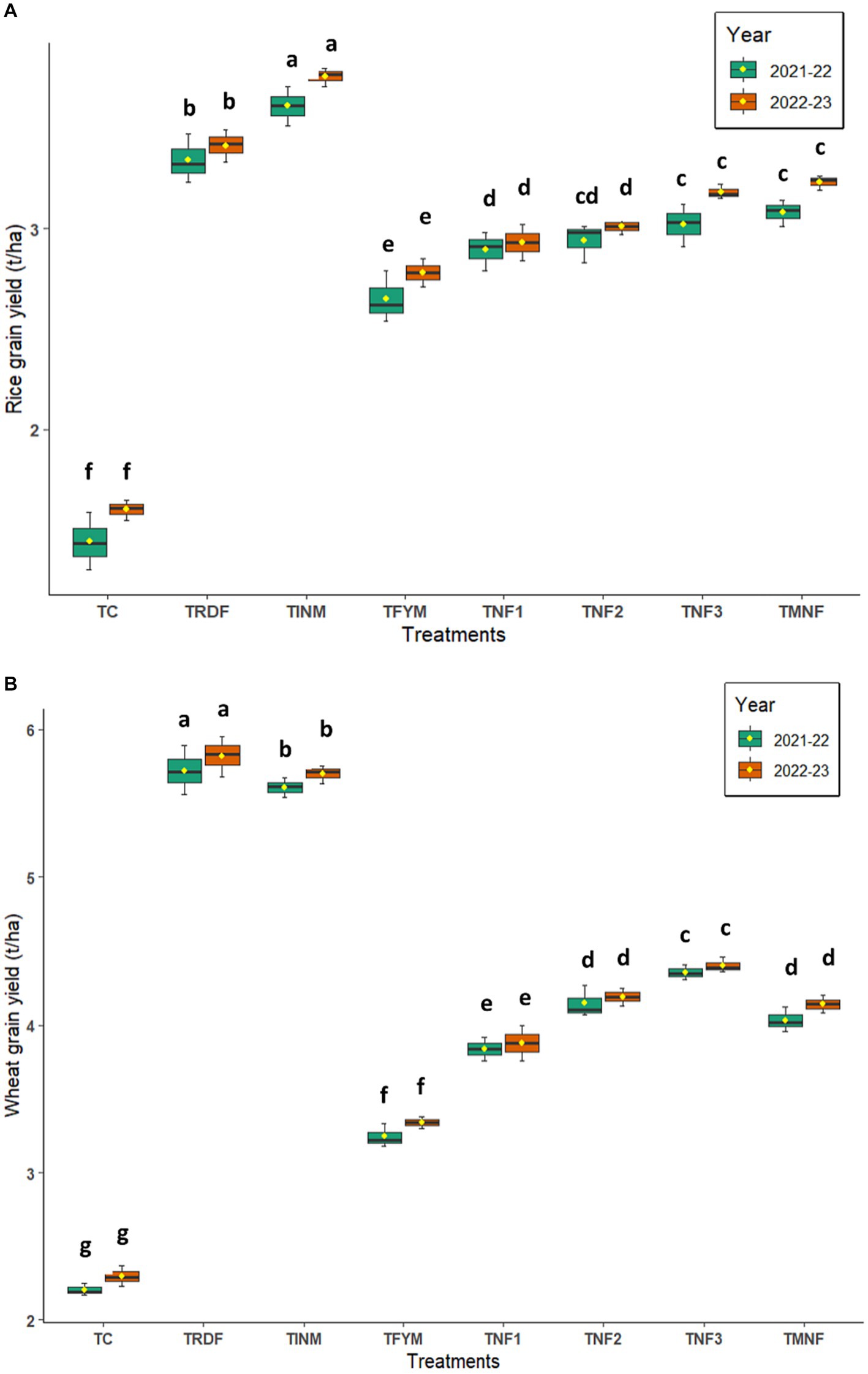
Figure 11. Box plot of different natural farming inputs (Ghanjeevamrit, Jeevamrit, and Beejamrit), organic (FYM) and in-organic fertilizers on (A) rice grain yield and (B) wheat grain yield. [TC, Control; TRDF, Recommended dosage of fertilizer; TINM, Integrated nutrient management; TFYM, Farm yard manure; TNF-1, Natural Farming-1; TNF-2, Natural Farming-2; TNF-3, Natural Farming-3; TMNF, Modified Natural Farming, Brief details of the treatment is given in Table 1]. Box plots of chemicals analyzed in the present study (logarithmic scale).
3.7 Correlation analysis
Correlation analysis of (BP: bacterial population; FP: fungal population; AP: actinomycetes population; MBC: microbial biomass carbon; MBN: microbial biomass nitrogen; DHA: dehydrogenase activity; UrA: urease activity; BGA: beta-glucosidase activity; PMN: potential mineralizable nitrogen) for rice and wheat crop during 2021–22 and 2022–23 has been shown in Figures 12, 13. The PMN during flowering stage was significantly (p = 0.001) positive correlated with BP (R2 = 0.818 and 0.901), FP (R2 = 0.929 and 0.942), AP (R2 = 0.890 and 0.906), MBC (R2 = 0.918 and 0.942), MBN (R2 = 0.926 and 0.940), DHA (R2 = 0.834 and 0.801), UrA (R2 = 0.748 and 0.742) and BGA (R2 = 0.972 and 0.973) in rice and wheat, respectively.
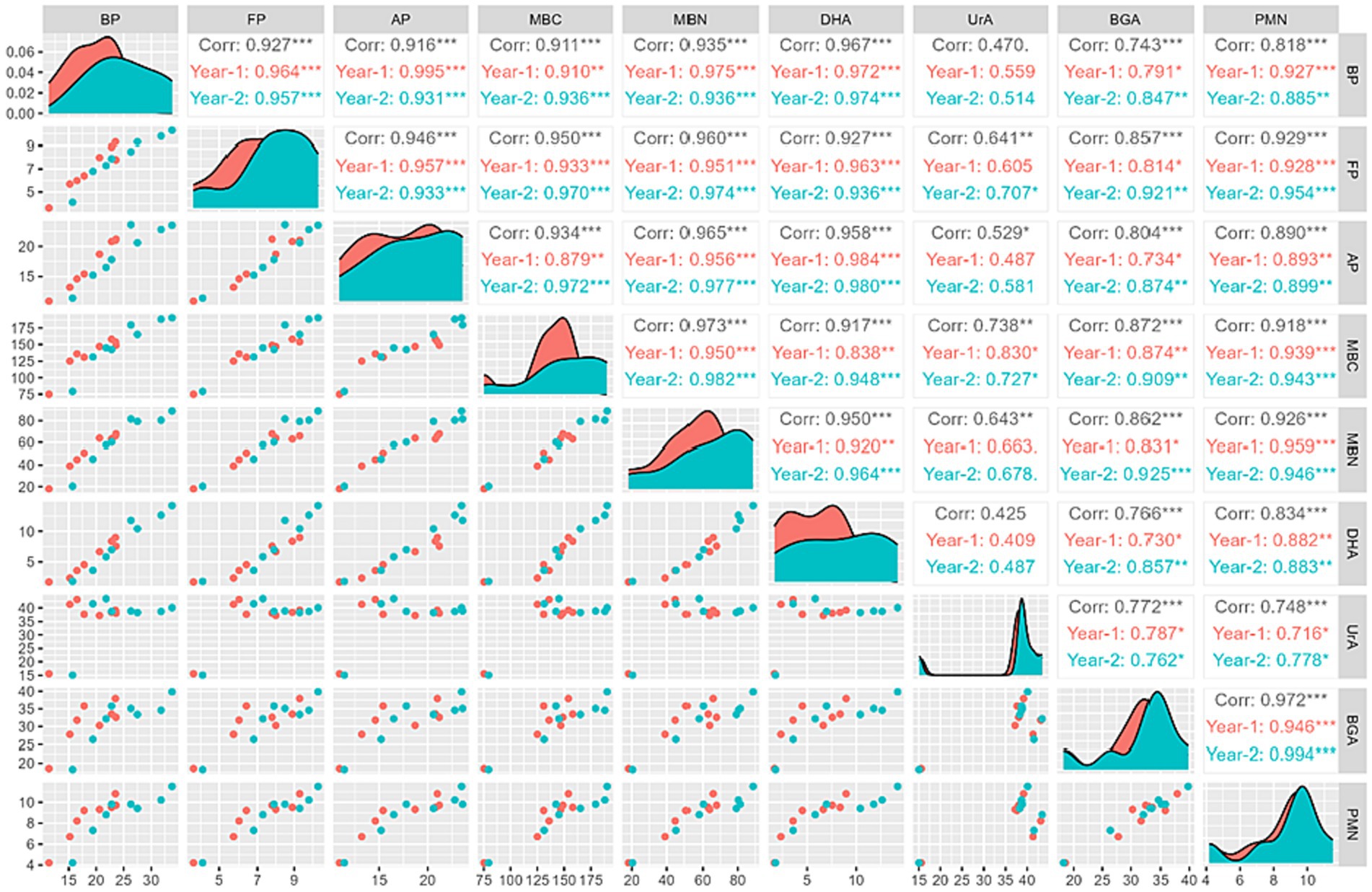
Figure 12. Correlation panel graph in rice (BP, bacterial population; FP, fungal population; AP, actinomycetes population; MBC, microbial biomass carbon; MBN, microbial biomass nitrogen; DHA, dehydrogenase activity; UrA, urease activity; BGA, beta-glucosidase activity; PMN, potential mineralizable nitrogen).
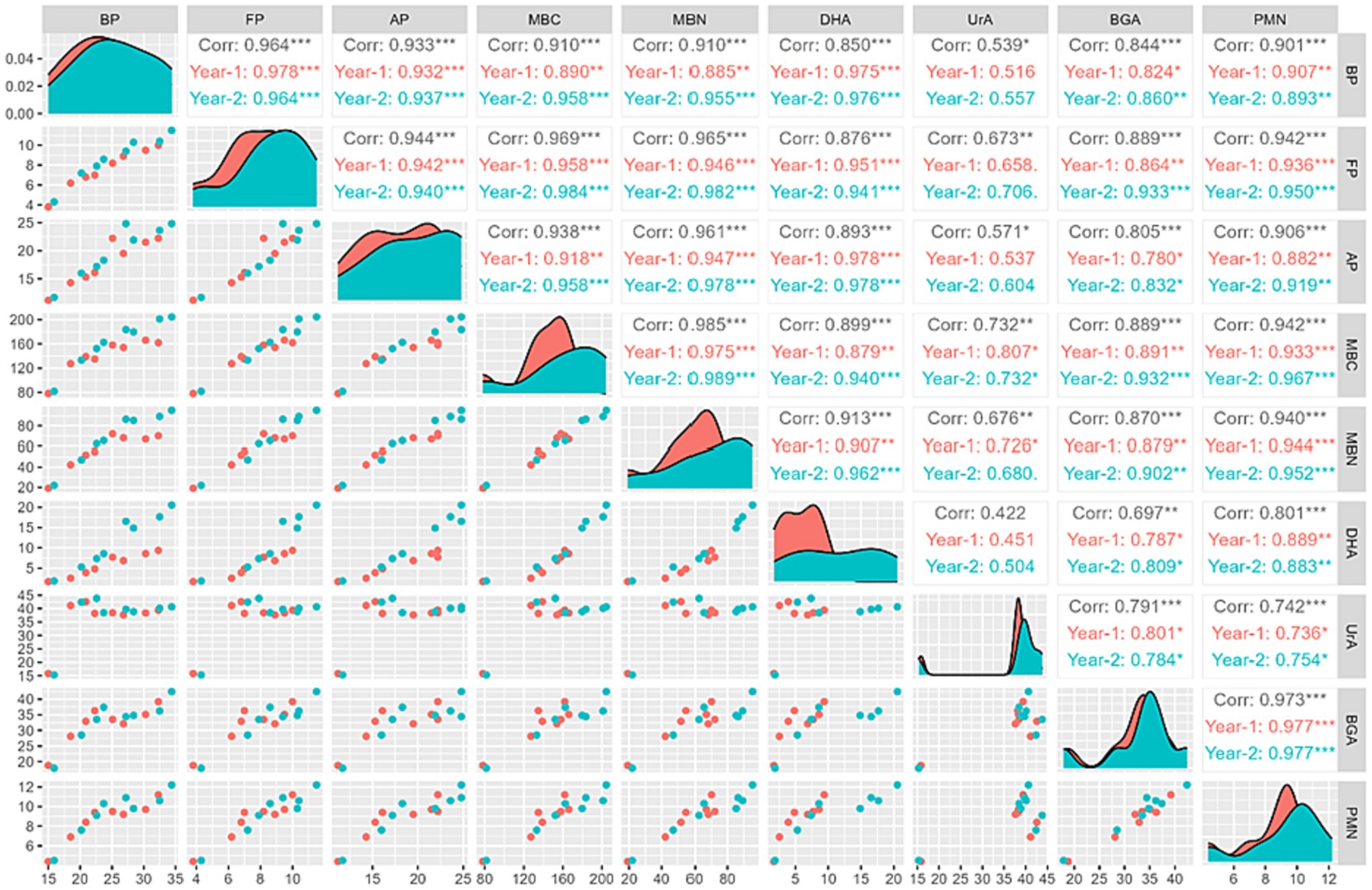
Figure 13. Correlation panel graph in wheat (BP, bacterial population; FP, fungal population; AP, actinomycetes population; MBC, microbial biomass carbon; MBN, microbial biomass nitrogen; DHA, dehydrogenase activity; UrA, urease activity; BGA, beta-glucosidase activity; PMN, potential mineralizable nitrogen).
4 Discussion
The study was conducted to evaluate the effect of different natural farming and organic inputs on nitrogen uptake, soil biological health and crop yield in rice-wheat cropping system. After the rice and wheat harvests spanning from 2021 to 2023, it was observed that the soil’s pH and electrical conductivity (EC) remained relatively stable, despite the application of diverse natural farming inputs. Notably, a minor reduction in pH was observed in the TRDF, while all other treatment groups showed slight increases in pH and EC values over time (Yadav et al., 2022). Soil organic matter by interacting with both biotic and abiotic soil component plays a significant role in improving soil health and restoring soil fertility (Gurmu, 2019). Numerous studies have investigated the comparative impacts of synthetic fertilizers and organic amendments on soil properties and crop production. It was found that the enhanced organic carbon content in the soil, resulting from the application of cow-based solid and liquid manure, could be attributed to the direct incorporation of organic materials and improved root growth. Subsequent decomposition of these organic materials appeared to contribute to the increased organic carbon content in the soil (Choudhary et al., 2022; Rautela et al., 2022). In our own investigation, the higher organic carbon content in all soil samples treated with natural farming inputs and organic amendments was primarily associated with the introduction of organic materials in comparison to mineral fertilizer and untreated control groups (Hu et al., 2023). Soil nutrient availability is a dynamic process that is highly responsive to intensive agricultural practices, environmental changes, and is typically constrained by both the quantity and quality of nutrients supplied through various physiochemical mechanisms (Rana et al., 2021). Previous research has consistently demonstrated a positive correlation between the use of various organic amendments and increased nutrient availability in soil (Darjee et al., 2023; Ramalingappa et al., 2023). In our current investigation, the incorporation of Ghanjeevamrit, Jeevamrit, Beejamrit, and Farm Yard Manure (FYM) resulted in a notable enhancement of the pool of available major nutrients in the soil. This phenomenon could be attributed to the accelerated mineralization of nitrogen, facilitated by heightened microbial activity within these treatments, in conjunction with the application of natural farming inputs (Darjee et al., 2022). Aulakh et al. (2018) also reported similar findings while studying the impact of farmyard manure and Jeevamrit in a maize-wheat organic production system in Punjab. However, some studies have indicated an increase in available nitrogen in the soil when optimizing Jeevamrit doses and application timing to boost wheat productivity under natural farming systems (Kaur and Saini, 2021). During our study, variations in the available phosphorus content were observed among the different treatment groups. This divergence may be due to the increased release of organic acids during mineralization in the case of treatments combining Ghanjeevamrit and Jeevamrit, which enhanced soil phosphorus levels by rendering native phosphates more soluble (Naresh et al., 2018). Interestingly, similar observations of increased soil available phosphorus content were made in studies focused on the application of Jeevamrit to enhance soil properties in zero-budget natural farming fields (Saharan et al., 2023). Our findings align with those of Sharma and Chadak (2022), who investigated residual soil fertility, nutrient uptake, and okra yield influenced by bioorganic nutrient sources. Notably, treatments with organic amendments exhibited significantly lower values of soil available potassium, possibly due to the absence of external potassium inputs and the depletion of the local potassium reservoir by plants. This depletion, however, was mitigated by the accumulation of microflora and fauna resulting from the addition of Jeevamrit and Ghanjeevamrit (Al-Jabori et al., 2011). Over the course of our research trial, an increase in potassium content was observed, which may be attributed to the ability of various natural farming amendments to increase soil potassium availability, promote potassium release, and reduce potassium fixation within the soil (Bader et al., 2021). This aligns with the findings of Wafaa and Mona (2015), who noted that the decomposition of organic fertilizers facilitates the dissolution of potassium-containing minerals in response to naturally occurring acids, such as fulvic and humic acids. This process releases potassium ions, subsequently elevating their concentration in the soil solution. The pattern of nitrogen uptake exhibited an elevated trend in both conventional fertilization methods and integrated nutrient management practices. Specifically, the microbial inoculum, or Jeevamrit, applied at various intervals to the soil, played a pivotal role in augmenting nutrient availability and subsequently enhancing enzymatic activity. As noted by Choudhary et al. (2022), Jeevamrit as well as Ghanjeevamrit significantly amplifies microbial activity in the soil, thereby enhancing the accessibility of nutrients to crops. Nitrogen availability frequently presents limitations within agro-ecosystems. Soil organic matter stands as the primary source of nitrogen for both crops and microorganisms, achieved through the process of mineralization. Superior-quality organic matter is characterized by a low carbon-to-nitrogen (C: N) ratio and an ample nitrogen content, sufficient to support the growth of microorganisms and crops. In most scenarios, soil suffers from nitrogen deficiency, primarily due to its meager or substandard organic matter.
The introduction of natural farming inputs, which tend to be nitrogen-rich, to the soil typically enhances the quality of soil organic matter. Nitrogen mineralization, on the other hand, exhibits variances among the various natural farming and organic inputs, with the most pronounced nitrogen mineralization observed in the treatment receiving an integrated blend of Farm Yard Manure (FYM), Ghanjeevamrit, and Jeevamrit (citations). The composting process serves to stabilize organic compounds, diminishing the proportion of soluble carbon and nitrogen forms (Masunga et al., 2016). In another study, it was revealed that only a minor fraction of organic nitrogen in composts underwent mineralization over time (Zhao et al., 2013). Consequently, even when manure is abundant in nitrogen, the release of nitrogen into the soil proceeds at a slow pace. Tits et al. (2014) noted restricted nitrogen release in composts derived from vegetable, fruit, and garden waste, despite their elevated nitrogen content (1.5% of fresh weight). Lazicki et al. (2020) also observed that nitrogen mineralization was more substantial in animal manure, such as cow dung, compared to composts (7 and 1% of applied organic nitrogen, respectively). This was the case despite their similar C:N ratios and nitrogen contents (10.1 and 9.3, respectively, and 1.2 and 2.2%, respectively).
In the current investigation, it has been determined that soil microbial carbon and nitrogen are profoundly influenced by both organic and natural farming inputs, namely Ghanjeevamrit, Jeevamrit, and Beejamrit. This influence may be attributed to the substantial presence of organic matter in the soil, which provides a nutrient-rich substrate conducive to microbial proliferation (Hicks et al., 2021). The improved MBC and MBN compare in natural farming inputs plot might be due to residue retention (mulching), tillage reduction as well as addition of Farm Yard Manure (FYM), liquid manure (Jeevamrit), and solid manure (Ghanjeevamrit), are incorporated into the soil, they serve as a nutritional source for microorganisms as well as, supply of organic matter provided sufficient C resources for the growth and reproduction of soil microorganisms which in turn resulting in an accumulation of microbial biomass carbon and nitrogen, whereas absence of residue retention (mulching) and organic manure and presence of conventional tillage in control plot would have resulted in such low MBC and MBN content (Ramalingappa et al., 2023). Microbial Biomass Carbon (MBC) is notably more responsive to cultural practices than Soil Organic Carbon (SOC) and represents a substantial component of SOC. In soils treated with natural farming inputs, the highest levels of MBC and Microbial Biomass Nitrogen (MBN) were recorded (Yang et al., 2018). This observation suggests that natural farming inputs may have functioned as substrates and sources of soluble nutrients conducive to the proliferation of soil microorganisms. These findings align with previous research, indicating that the application of Jeevamrit substantially elevates soil MBC and MBN (Rathore et al., 2023). Soil enzymatic activity is frequently employed as a measure of soil quality, relates to soil nutrient transformation and also strongly correlated with the amount of organic matter in the soil (Merino et al., 2019). The enzymes selected in the current study, are dehydrogenase, β-glucosidase and urease, which are one of the most adequate, important and most sensitive bioindicators, relating to soil fertility (Wolińska and Stępniewska, 2012). Furthermore, according to Choudhary et al. (2022), there was a favourable correlation between soil enzymatic activity and microbial biomass. The utilization of organic sources in the soil may enhance dehydrogenase activity as well as β-glucosidase by increasing substrate availability, promoting biological activity, and stabilizing extracellular enzymes through humic substance complexation (Adak et al., 2014). Higher enzyme activity was seen in the organic amendment treatments, such as TINM, TFYM, TNF1, TNF2, TNF3, and TMNF. which is consistent with the results reported by Rao et al. (2019), Ghasal et al. (2023) and suggests that these treatments can enhance the cycling of soil nutrients (such as C, N, and P). Soil enzymatic activity is a commonly utilized metric for assessing soil quality, as it is intricately linked to soil nutrient transformations and exhibits a robust correlation with the quantity of organic matter within the soil (Merino et al., 2019). In the present study, the selected enzymes, specifically dehydrogenase, β-glucosidase, and urease, stand as highly suitable, crucial, and exceedingly sensitive bioindicators with strong ties to soil fertility (Wolińska and Stępniewska, 2012). Moreover, in accordance with Choudhary et al. (2022), a positive association was observed between soil enzymatic activity and microbial biomass. The incorporation of organic sources into the soil has the potential to elevate dehydrogenase and β-glucosidase activities by augmenting the availability of substrates, fostering biological activity, and stabilizing extracellular enzymes through complexation with humic substances (Adak et al., 2014). Notably, enhanced enzyme activity was evident in treatments involving organic amendments such as TINM, TFYM, TNF1, TNF2, TNF3, and TMNF. These findings align with results reported by Rao et al. (2019) and Ghasal et al. (2023), indicating that these treatments have the capacity to bolster the cycling of soil nutrients, including carbon (C), nitrogen (N), and phosphorus (P). Soil biodiversity is recognized as a fundamental component underpinning the integrity, functionality, and long-term sustainability of soil ecosystems (Zhao et al., 2014). In our investigation, it was observed that the microbial community, in terms of richness, exhibited relatively higher values in the treatment receiving Ghanjeevamrit, Jeevamrit, and Beejamrit, in comparison to other fertilizer regimens. However, it’s worth noting that while these responses were statistically significant when compared to the inorganic fertilizer treatment and the control group, they did not reach statistical significance when compared among the treatments utilizing various natural farming inputs. These findings align with the outcomes of Patro et al. (2009), which emphasizes that the metabolic activity of the microbial population in the soil is reflected in soil enzyme activity. The improved microbial population in the soil may be attributed to the combined impact of Ghanjeevamrit, Jeevamrit, and mulching (Saharan et al., 2023). Moreover, Ghanjeevamrit was found to have positive effects on soil properties, including the potential reduction of bulk density (Kumari et al., 2022), enhancement of soil aeration, and the provision of carbon as an energy source for the multiplication and survival of microbes present in Jeevamrit (Dhiman et al., 2023). According to Siddappa (2015), the application of Jeevamrit alone may lead to noticeably lower microbial activity because fungi, bacteria, and actinomycetes lack access to an organic carbon source necessary for their further proliferation. Conversely, the application of Ghanjeevamrit alone may result in a deficiency of microbial inoculum, which is typically present in Jeevamrit. Such a treatment approach might contribute to a more stable agroecosystem and promote sustainable crop production. In the present study, variations in crop yields over time were evident in response to the diverse fertilizer regimens applied. The majority of characteristics contributing to yield exhibited an increase, primarily attributed to improved growth characteristics resulting from heightened nitrogen absorption and the efficient translocation of assimilates from source to sink (Mangaraj et al., 2022). This upturn in grain yield can be linked to the appropriate allocation of resources from source to sink and the timely availability of nutrients (Laila et al., 2022). Aulakh et al. (2018), while conducting research on nutrient sources for organic rice (Oryza sativa)-wheat (Triticum aestivum) cropping systems in north-west India, reported similar outcomes. Darjee et al. (2022) and Iqbal et al. (2022) also documented enhanced growth attributes of rice and wheat yields in plots where Farm Yard Manure (FYM) was combined with chemical fertilizers. These results can likely be ascribed to the fulfillment of the crop’s nutritional requirements through improved nutrient availability throughout the crop’s life cycle, thereby enhancing growth and ultimately resulting in higher wheat seed yields. These findings closely align with those of Sutar et al. (2019), who observed similar outcomes with the application of Jeevamrit at a rate of 1,000 L/ha. Additionally, Gupta and Bhadauria (2022) reported an increase in wheat yields when investigating the impact of zero-budget natural farming on nutrient content and uptake in wheat (Triticum aestivum L.).
5 Conclusion
Our experimental trial concluded that application of natural farming inputs (Ghanjeevamrit, Jeevamrit, Beejamrit) in rice-wheat cropping system, had shown significant improvement in soil biological quality and soil microbial community as shown in (Figure 14). The data on the build-up of macro nutrient (N, P, K) also improved. Remarkably, a considerable boost in nitrogen uptake was observed in crops treated with natural farming inputs over control treatment. This phenomenon can be ascribed to the expedited mineralization of native and applied nutrients, facilitated by the accumulation of microflora. In contrast the treatment with exclusive use of in-organic fertilizer had an adverse impact on soil biological quality, reduced microbial richness and poor soil enzymatic activity. The natural farming inputs, employing Ghanjeevamrit, Jeevamrit, Beejamrit, and farmyard manure (FYM) in combination resulted in significant higher grain yield of rice and wheat than control and treatment having FYM only but lower yields than treatments supplied nutrients through integrated nutrient management and in-organic fertilizers. Overall, our research suggests that introducing natural farming inputs into intensive agricultural systems can mitigate the detrimental effects resulting from excessive use of synthetic fertilizers. However, to determine the optimal strategy, further investigations into the types, application rates, and frequency of these natural farming inputs are essential. These studies should also consider the interplay between various soil types and the specific cultivated crop species. Additionally, extensive research on the characterization of natural farming inputs are essential to substantiate our findings on a larger scale. Further investigations should focus on developing site-specific and comprehensive packages of practices to enhance adaptability within the farming community. Therefore, our study suggests that TINM & TRDF could be an option for the higher yield of rice and wheat however TMNF and TNF3 are best options for improving soil biological health in long term. Further a long-term study on Natural farming may be taken to confirm the effect on the production and profitability. Hence, advocating natural farming to various stakeholders is warranted, given its positive impact on soil health and cost reduction in regions favoring organic farming.
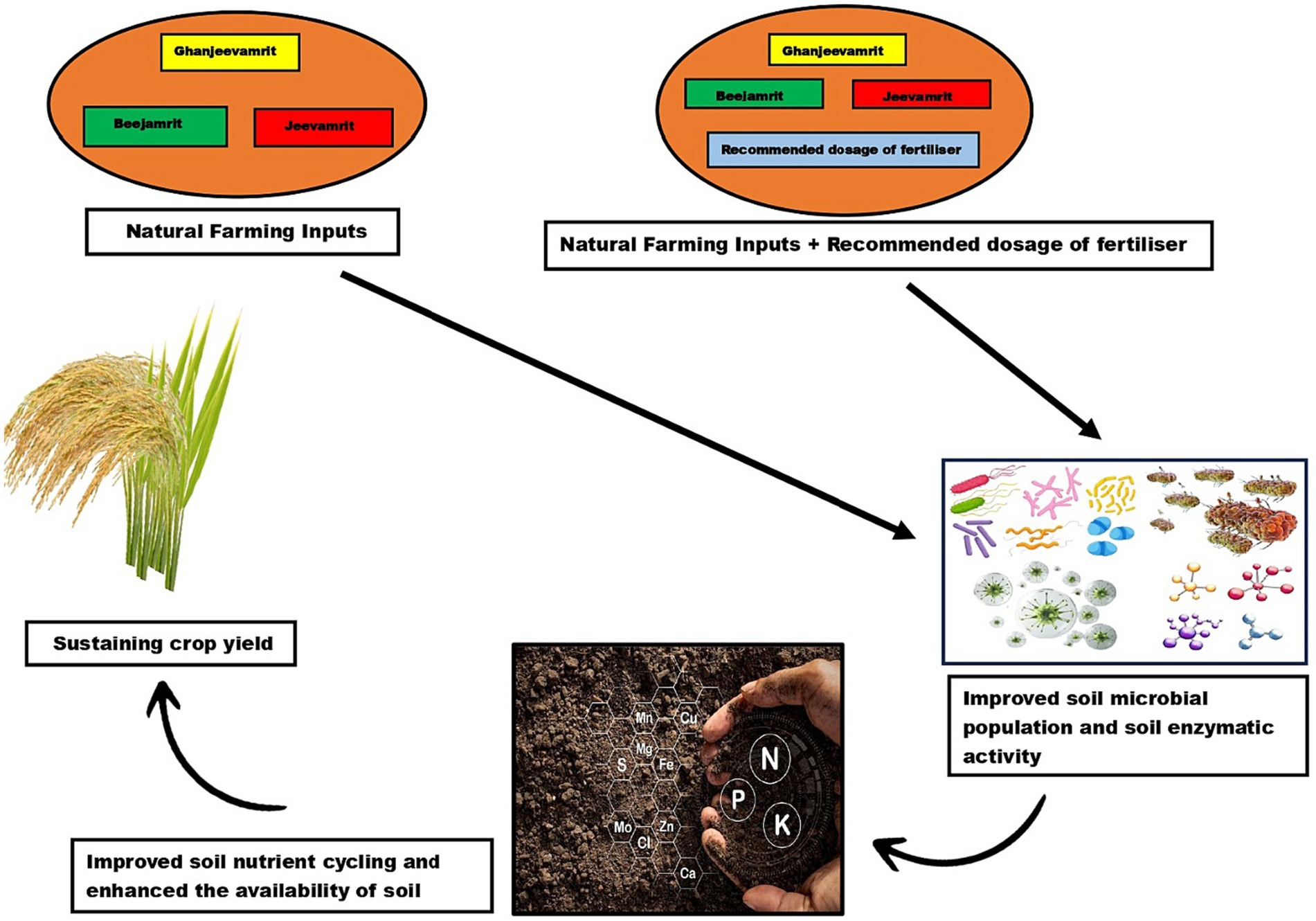
Figure 14. Pictorial representation of application of natural farming inputs (Ghanjeevamrit, Jeevamrit, Beejamrit) and natural farming inputs integrated with recommended dosage of fertilizers showing significant improvement in soil biological quality, soil microbial community, soil nutrient availability, and sustaining crop yield.
Data availability statement
The original contributions presented in the study are included in the article/supplementary material, further inquiries can be directed to the corresponding authors.
Author contributions
SD: Conceptualization, Investigation, Methodology, Resources, Validation, Writing – original draft, Writing – review & editing, Software. RS: Conceptualization, Investigation, Methodology, Resources, Validation, Writing – original draft, Writing – review & editing, Formal analysis, Supervision, Visualization. SD: Investigation, Writing – review & editing. RP: Investigation, Writing – review & editing. ND: Investigation, Writing – review & editing. PS: Investigation, Writing – review & editing. MR: Resources, Writing – review & editing. GA: Data curation, Writing – review & editing. SP: Data curation, Writing – review & editing. PR: Investigation, Writing – review & editing. MS: Investigation, Methodology, Resources, Visualization, Writing – review & editing.
Funding
The author(s) declare that no financial support was received for the research, authorship, and/or publication of this article.
Acknowledgments
The authors acknowledge the ICAR-Indian Agricultural Research Institute and University Grant Commission, New Delhi, India for providing necessary research facilities.
Conflict of interest
The authors declare that the research was conducted in the absence of any commercial or financial relationship that could be constructed as a potential conflict of interest.
Publisher’s note
All claims expressed in this article are solely those of the authors and do not necessarily represent those of their affiliated organizations, or those of the publisher, the editors and the reviewers. Any product that may be evaluated in this article, or claim that may be made by its manufacturer, is not guaranteed or endorsed by the publisher.
References
Adak, T., Singha, A., Kumar, K., Shukla, S. K., Singh, A., and Kumar Singh, V. (2014). Soil organic carbon, dehydrogenase activity, nutrient availability and leaf nutrient content as affected by organic and inorganic source of nutrient in mango orchard soil. J. Soc. Sci. Plant Nutr. 14, 394–406. doi: 10.4067/S0718-95162014005000031
Al-Jabori, J. S. J., Al-Obaed, B. S. O., and Al-Amiri, A. H. F. (2011). Effect of soil gypsum content and kind of organic matter on status and behavior of potassium. Tikrit J. Agric. Sci. 11, 299–310.
Anas, M., Liao, F., and Verma, K. K. (2020). Fate of nitrogen in agriculture and environment: agronomic, eco-physiological and molecular approaches to improve nitrogen use efficiency. Bio. Res. 53:47. doi: 10.1186/s40659-020-00312-4
Aulakh, C. S., Singh, H., Walia, S. S., Phutela, R. P., and Singh, G. (2018). Evaluation of nutrient sources for organic production of rice (Oryza sativa)-wheat (Triticum aestivum) cropping system in north-West India. Indian J. Agron. 63, 137–144.
Bader, B. R., Taban, S. K., Fahmi, A. H., Abood, M. A., and Hamdi, G. J. (2021). Potassium availability in soil amended with organic matter and phosphorous fertiliser under water stress during maize (Zea mays L.) growth. J. Saudi Soc. Agric. Sci. 20, 390–394. doi: 10.1016/j.jssas.2021.04.006
Bremner, J. M., and Douglas, L. A. (1971). Inhibition of urease activity in soils. Soil Biology and Biochemistry 3, 297–307.
Casida, L. E. Jr., Klein, D. A., and Santoro, T. (1964). Soil dehydrogenase activity. Soil Sci. 98, 371–376. doi: 10.1097/00010694-196412000-00004
Choudhary, R., Kumar, R., Sharma, G., and Sharma, P. (2022). Effect of natural farming on yield performances, soil health and nutrient uptake in wheat+ gram inter cropping system in sub-temperate regions of Himachal Pradesh. J. Crop Weed. 18, 1–8. doi: 10.22271/09746315.2022.v18.i2.1566
Craswell, E. (2021). Fertilizers and nitrate pollution of surface and ground water: an increasingly pervasive global problem. SN Appl. Sci. 3:518. doi: 10.1007/s42452-021-04521-8
Darjee, S., Pooja, L. R., Khandelwal, A., Dhar, S., Shrivastava, M., and Singh, R. (2022). Integrated application of inorganic and bio-fertilizers affects nitrogen losses and yield of wheat (Triticum aestivum L.). Ann. Agric. Res. 43, 127–133.
Darjee, S., Shrivastava, M., Langyan, S., Singh, G., Pandey, R., Sharma, A., et al. (2023). Integrated nutrient management reduced the nutrient losses and increased crop yield in irrigated wheat. Arch. Agron. Soil Sci. 69, 1298–1309. doi: 10.1080/03650340.2022.2084535
Dhiman, M., Rana, N., and Ghabru, A. (2023). Isolation and screening of agriculturally important Bacteria (PGPR) from organic sources of nutrient (Panchgavya, Jeevamrit and farm yard manure) for future use. Asian J. Adv. Agric. 22, 43–52. doi: 10.9734/ajaar/2023/v22i3443
Eivazi, F., and Tabatabai, M. A. (1988). Glucosidases and galactosidases in soils. Soil Biol 20, 601–606. doi: 10.1016/0038-0717(88)90141-1
Ghasal, P. C., Mishra, R. P., Choudhary, J., Dutta, D., Bhanu, C., Meena, A. L., et al. (2023). Evaluation of integrated crop management, organic management and natural farming in basmati rice-wheat system under upper indo-Gangetic Plains. J. Plant Nutr. 1-11, 1–11. doi: 10.1080/01904167.2023.2209117
Gomez, K. A., and Gomez, A. A. (1984). Statistical procedures for agricultural research in An International Rice Research Institute Book, 2nd edition (New York: A Wiley–Inter–science Publication John wiley & sons).
Gupta, G. S. (2019). Land degradation and challenges of food security. Rev. Eur. Stud. 11:63. doi: 10.5539/res.v11n1p63
Gupta, K., and Bhadauria, S. S. (2022). Effect of zero budget natural farming on nutrient content and uptake of wheat (Triticum aestivum L.). Pharma Innov. J. 11, 2305–2308.
Gurmu, G. (2019). Soil organic matter and its role in soil health and crop productivity improvement. For. Ecol. Manag. 7, 475–483. doi: 10.14662/ARJASR2019.147
Hanway, J. J., and Heidel, H. (1952). Soil analysis methods as used in Iowa state college soil testing laboratory, bulletin 57. Iowa, USA: Iowa State College of Agriculture 131.
Hicks, L. C., Lajtha, K., and Rousk, J. (2021). Nutrient limitation may induce microbial mining for resources from persistent soil organic matter. Ecology 102:e03328. doi: 10.1002/ecy.3328
Hinojosa, M. B., García-Ruíz, R., Viñegla, B., and Carreira, J. A. (2004). Microbiological rates and enzyme activities as indicators of functionality in soils affected by the Aznalcóllar toxic spill. Soil Biol. Biochem. 36, 1637–1644. doi: 10.1016/j.soilbio.2004.07.006
Hu, Q., Liu, T., Ding, H., Li, C., Tan, W., Yu, M., et al. (2023). Effects of nitrogen fertilizer on soil microbial residues and their contribution to soil organic carbon and total nitrogen in a rice-wheat system. Appl. Soil Ecol. 181:104648. doi: 10.1016/j.apsoil.2022.104648
Iqbal, A., Ali, I., Yuan, P., Khan, R., Liang, H., Wei, S., et al. (2022). Combined application of manure and chemical fertilizers alters soil environmental variables and improves soil fungal community composition and rice grain yield. Front. Microbiol. 13:856355. doi: 10.3389/fmicb.2022.856355
Jackson, W. A., Flesher, D., and Hageman, R. H. (1973). Nitrate uptake by dark-grown corn seedlings: some characteristics of apparent induction. Plant Physiol. 51, 120–127. doi: 10.1104/pp.51.1.120
John, D. A., and Babu, G. R. (2021). Lessons from the aftermaths of green revolution on food system and health. Front. Sustain. Food Syst. 5:644559. doi: 10.3389/fsufs.2021.644559
Kabiraj, S., Duraisekaran, E., and Ramaswamy, M. (2022). Combination of remote-sensing spectral indices to classify the areas of land degradation in west Burdwan district. India. Environ. Earth Sci. 81:218. doi: 10.1007/s12665-022-10388-4
Kaur, P., and Saini, J. P. (2021). Optimization of Jeevamrit doses and application time for enhancing productivity of wheat under natural farming system. J. Pharmacogn. Phytochem. 10, 405–408.
Khadse, A., Rosset, P. M., Morales, H., and Ferguson, B. G. (2018). Taking agroecology to scale: the zero-budget natural farming peasant movement in Karnataka. India. J. Peasant Stud. 45, 192–219. doi: 10.1080/03066150.2016.1276450
Keeney, D. R. (1982). Nitrogen—availability indices. Methods of soil analysis: Part 2 chemical and microbiological properties, 9:711–733.
Kjeldahl, C. (1883). A new method for the determination of nitrogen in organic matter. Z. Anal. Chem. 22, 366–382. doi: 10.1021/ac60085a028
Kumari, S., Meena, L., Raghavendra, K. J., and Karwal, M. (2022). Jeevamrit/Jeevamrutha: organic concoctions for natural farming. Agric. Food e-Newsl. 4, 40–42.
Laila, N., Paul, N. C., Imran, S., Sarkar, M. A. R., Sarkar, S. K., and Paul, S. K. (2022). Assessing the influence of integrated nutrient management on growth performance of aromatic fine rice. Arch. Agric. Environ. Sci. 7, 174–184. doi: 10.26832/24566632.2022.070205
Lazicki, P., Geisseler, D., and Lloyd, M. (2020). Nitrogen mineralization from organic amendments is variable but predictable. J. Environ. Qual. 49, 483–495. doi: 10.1002/jeq2.20030,
Mangaraj, S., Paikaray, R. K., Maitra, S., Pradhan, S. R., Garnayak, L. M., Satapathy, M., et al. (2022). Integrated nutrient management improves the growth and yield of rice and greengram in a rice—greengram cropping system under the coastal plain agro-climatic condition. Plan. Theory 11:142. doi: 10.3390/plants11010142
Masunga, R. H., Uzokwe, V. N., Mlay, P. D., Odeh, I., Singh, A., Buchan, D., et al. (2016). Nitrogen mineralization dynamics of different valuable organic amendments commonly used in agriculture. Appl. Soil Ecol. 101, 185–193. doi: 10.1016/j.apsoil.2016.01.006
Merino, A., Jiménez, E., Fernández, C., Fontúrbel, M. T., Campo, J., and Vega, J. A. (2019). Soil organic matter and phosphorus dynamics after low intensity prescribed burning in forests and shrubland. J. Environ. Manag. 234, 214–225. doi: 10.1016/j.jenvman.2018.12.055
Nagavani, A. V., and Subbian, P. (2015). Effect of organics and inorganics on soil microbial population and enzymatic activity in maize-maize cropping system. Progress. Agric. 15, 9–14.
Naresh, R. K., Kumar, M., Kumar, S., Chowdhary, U., Kumar, Y., Mahajan, N. C., et al. (2018). Zero budget natural farming viable for small farmers to empower food and nutritional security and improve soil health: a review. J. Pharmacogn. Phytochem. 7, 1104–1118.
Nicolopoulou-Stamati, P., Maipas, S., Kotampasi, C., and Stamatis, P. (2016). Chemical pesticides and human health: the urgent need for a new concept in agriculture. Front. Public Health 4:148. doi: 10.3389/fpubh.2016.00148
Olsen, S. R. (1954). Estimation of available phosphorus in soils by extraction with sodium bicarbonate (No. 939). Washington, DC: US Department of Agriculture.
Palekar, S. (2006). Natural Farming Research, development and extension movement, Zero budget natural farming: Five layers Palekar’s model (part I) Zero Budget Amravati, Maharashtra, India.
Paroda, R. S. (2018). “A strategy for doubling farmers' income” in Reorienting Indian agriculture: Challenges and opportunities ed. K. Tim (Wallingford UK: CAB International) 274–286.
Patro, H., Patro, L., Swain, S. C., and Tarai, R. K. (2009). Effect of organic source and nitrogen levels on soil microbial biomass nitrogen in Rice under Rice-wheat cropping system. Asian J. Exp. Sci. 23, 109–113.
Pingali, P. L. (2012). Green revolution: impacts, limits, and the path ahead. Proc. Natl. Acad. Sci. U. S. A. 109, 12302–12308. doi: 10.1073/pnas.0912953109
R Development Core Team . (2012). R: A language and environment for statistical computing. Vienna, Austria: R Foundation for Statistical ComputingISBN 3- 900051-07-0
Ramalingappa, P. L., Shrivastava, M., Dhar, S., Bandyopadhyay, K., Prasad, S., Langyan, S., et al. (2023). Reducing options of ammonia volatilization and improving nitrogen use efficiency via organic and inorganic amendments in wheat (Triticum aestivum L.). PeerJ 11:e14965. doi: 10.7717/peerj.14965
Rana, A., Sharma, G. D., Kumar, N., and Sankhyan, N. K. (2021). Performance of new genotype of Indian mustard (Brassica juncea (L.) Czernj. Cosson) as influenced by sowing time and nutrient management. Himachal J. Agric. Res. 47, 206–213.
Rao, D. L. N., Aparna, K., and Mohanty, S. R. (2019). Microbiology and biochemistry of soil organic matter, carbon sequestration and soil health. Indian J. Fert. 15, 124–138.
Rathore, G., Kaushal, R., Sharma, V., Sharma, G., Chaudhary, S., Dhaliwal, S. S., et al. (2023). Evaluation of the usefulness of fermented liquid organic formulations and manures for improving the soil fertility and productivity of Brinjal (Solanum melongena L.). Agriculture 13:417. doi: 10.3390/agriculture13020417
Rautela, S., Bains, G., Singh, D. K., Jahan, S., and Thi, T. (2022). Effect of organic, inorganic and integrated nutrient amendments on growth parameters of basmati rice (Oryza sativa L.). Pharma Innov. J. 11, 321–325.
Rosset, P. M., and Martínez-Torres, M. E. (2012). Rural social movements and agroecology: context, theory, and process. Ecol. Soc. 17:17. doi: 10.5751/ES-05000-170317
Saharan, B. S., Tyagi, S., Kumar, R., Vijay Om, H., Mandal, B. S., and Duhan, J. S. (2023). Application of Jeevamrit improves soil properties in zero budget natural farming fields. Agriculture 13:196. doi: 10.3390/agriculture13010196
Sharma, R., and Chadak, S. (2022). Residual soil fertility, nutrient uptake, and yield of okra as affected by bioorganic nutrient sources. Commun. Soil Sci. Plant Anal. 53, 2853–2866. doi: 10.1080/00103624.2022.2094397
Sheoran, O. P., Tonk, D. S., Kaushik, L. S., Hasija, R. C., and Pannu, R. S. (1998). Statistical software package for agricultural research workers. Recent advances in information theory, statistics & computer applications by DS Hooda & RC Hasija Department of Mathematics Statistics, Hisar: CCS HAU 8, 139–143.
Siddappa, (2015). Use of jeevamrutha and farm yard manure on growth and yield of field bean (Lablab purpureus var. lignosus). M.Sc. Thesis, Bengaluru, India: University of Agricultural Sciences.
Sutar, R., Sujith, G. M., and Devakumar, N. (2019). Growth and yield of cowpea (Vigna unguiculata (L.) Walp) as influenced by jeevamrutha and panchagavya application. Legum. Res. 42, 824–828. doi: 10.18805/LR-3932
Tits, M., Elsen, A., Bries, J., and Vandendriessche, H. (2014). Short-term and long-term effects of vegetable, fruit and garden waste compost applications in an arable crop rotation in Flanders. Plant Soil 376, 43–59. doi: 10.1007/s11104-012-1318-0
Vance, E. D., Brookes, P. C., and Jenkinson, D. S. (1987). An extraction method for measuring soil microbial biomass C. Soil Biol. Biochem. 19, 703–707. doi: 10.1016/0038-0717(87)90052-6
Vetter, S. H., Sapkota, T. B., Hillier, J., Stirling, C. M., Macdiarmid, J. I., Aleksandrowicz, L., et al. (2017). Greenhouse gas emissions from agricultural food production to supply Indian diets: implications for climate change mitigation. Agric. Ecosyst. Environ. 237, 234–241. doi: 10.1016/j.agee.2016.12.024
Wafaa, S. M., and Mona, A. O. (2015). Impact of feldspar acidulation on potassium dissolution and pea production. Int. J. ChemTech Res. 8, 01–10.
Walkley, A., and Black, I. A. (1934). An examination of the Degtjareff method for determining soil organic matter, and a proposed modification of the chromic acid titration method. Soil Sci. 37, 29–38. doi: 10.1097/00010694-193401000-00003
Wolińska, A., and Stępniewska, Z. (2012). Dehydrogenase activity in the soil environment. Dehydrogenases 10, 183–210. doi: 10.5772/48294
Wollum, A. G. (1994). “Soil sampling for microbiological analysis” in Methods of Soil Analysis: Part 2 Microbiological and Biochemical Properties. eds. P. J. Bottomley, J. S. Angle, and R. W. Weaver, vol. 5 (Hoboken: Wiley), 1–14.
Yadav, N., Thakur, K. S., and Mehta, D. K. (2022). A practice to enhance soil physico-chemical properties and viable microbial count as effected by organic nutrient sources in garden pea under mid hill zone of Himachal Pradesh. Indian J. Agric. Res. 56, 626–628. doi: 10.18805/IJARe.A-5979
Yang, Y., Yu, K., and Feng, H. (2018). Effects of straw mulching and plastic film mulching on improving soil organic carbon and nitrogen fractions, crop yield and water use efficiency in the loess plateau. China. Agric. Water Manag. 201, 133–143. doi: 10.1016/j.agwat.2018.01.021
Zhao, B., Chen, J., Zhang, J., Xin, X., and Hao, X. (2013). How different long-term fertilization strategies influence crop yield and soil properties in a maize field in the North China plain. J. Plant Nutr. Soil Sci. 176, 99–109. doi: 10.1002/jpln.201200076
Keywords: Ghanjeevamrit, Jeevamrit, Beejamrit, nitrogen uptake, soil microbial population
Citation: Darjee S, Singh R, Dhar S, Pandey R, Dwivedi N, Sahu PK, Rai MK, Alekhya G, Padhan SR, Ramalingappa PL and Shrivastava M (2024) Empirical observation of natural farming inputs on nitrogen uptake, soil health, and crop yield of rice-wheat cropping system in the organically managed Inceptisol of Trans Gangetic plain. Front. Sustain. Food Syst. 8:1324798. doi: 10.3389/fsufs.2024.1324798
Edited by:
G. K. Sujayanand, Indian Institute of Pulses Research, IndiaReviewed by:
Vesna Vasić, Educons University, SerbiaSushil K. Sharma, National Institute of Biotic Stress Management, India
Copyright © 2024 Darjee, Singh, Dhar, Pandey, Dwivedi, Sahu, Rai, Alekhya, Padhan, Ramalingappa and Shrivastava. This is an open-access article distributed under the terms of the Creative Commons Attribution License (CC BY). The use, distribution or reproduction in other forums is permitted, provided the original author(s) and the copyright owner(s) are credited and that the original publication in this journal is cited, in accordance with accepted academic practice. No use, distribution or reproduction is permitted which does not comply with these terms.
*Correspondence: Sibananda Darjee, darzee.sd.06@gmail.com; Renu Singh, renu_icar@yahoo.com
†ORCID: Sibananda Darjee https://orcid.org/0000-0002-6356-4570
Renu Singh https://orcid.org/0000-0001-8857-2027
Shiva Dhar https://orcid.org/0000-0001-9226-2742
Renu Pandey https://orcid.org/0000-0002-9244-8579
Pramod Kumar Sahu https://orcid.org/0000-0002-6712-5460
Gunturi Alekhya https://orcid.org/0009-0002-3018-8836
Smruti Ranjan Padhan https://orcid.org/0000-0001-8230-0637
Manoj Shrivastava https://orcid.org/0000-0003-1124-6770