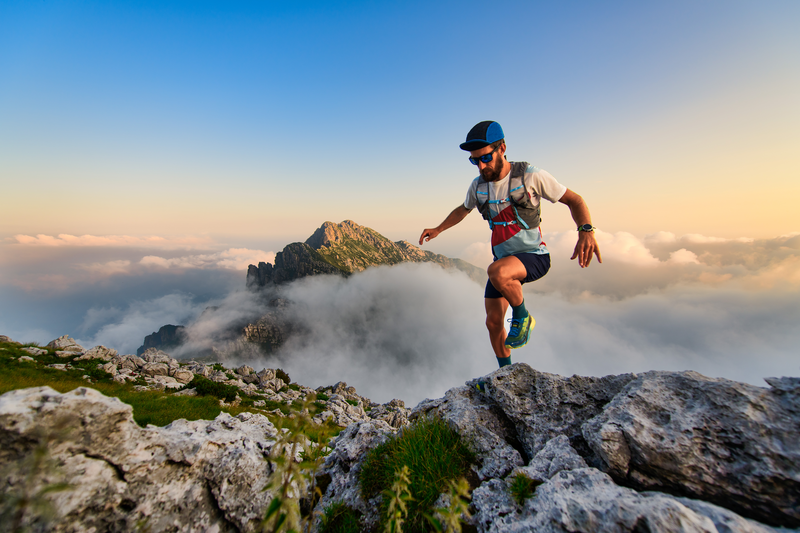
94% of researchers rate our articles as excellent or good
Learn more about the work of our research integrity team to safeguard the quality of each article we publish.
Find out more
ORIGINAL RESEARCH article
Front. Sustain. Food Syst. , 20 February 2024
Sec. Nutrition and Sustainable Diets
Volume 8 - 2024 | https://doi.org/10.3389/fsufs.2024.1321288
This article is part of the Research Topic Pushing The Frontiers Of Nutritional Life Cycle Assessment (nLCA) To Identify Globally Equitable And Sustainable Agri-Food Systems View all 15 articles
Introduction: Lamb production systems are under increasing pressure to reduce their environmental footprint, particularly emissions of greenhouse gases (GHGs) such as methane. However, the metrics used to express the carbon footprint of lamb seldom consider its nutritional density and contribution to balanced diets in humans. Lamb production systems vary considerably, from low-input pastoral systems to higher-input systems feeding concentrates for the latter ‘finishing’ period. To date, no studies have explored the effect of finishing diet on the carbon footprint of lamb meat on a nutritional basis.
Methods: Data from 444 carcasses were collected from four abattoirs across Wales, United Kingdom. Lambs were derived from 33 farms with one of four distinct finishing diets: forage crops (n = 5), grass (n = 11), concentrates (n = 7), and grass and concentrates (n = 15). Carcass data were analysed using mixed effects models. Significant differences were found in fatty acid composition of two large commercial cuts of meat from different finishing diets. To illustrate the effect of different measures of footprint, mass (kg dwt) and omega-3 polyunsaturated fatty acid content (g omega-3) were selected as functional units. GHG emission estimates were calculated using Agrecalc.
Results: The concentrates diet had the lowest average mass-based product emissions [25.0 kg CO2e/kg deadweight (dwt)] while the grass systems had the highest (28.1 kg CO2e/kg dwt; p < 0.001). The semimembranosus muscle cut from the forage crops diet had the lowest average nutrition-based product emissions (19.2 kg CO2e/g omega-3); whereas the same muscle cut from lambs finished on the grass and concentrates diet had the highest nutrition-based product emissions (29.4 kg CO2e/g omega-3; p < 0.001).
Discussion: While mass-based functional units can be useful for comparing efficiencies of different farming systems, they do not reflect how farming systems impact the nutritional differences of the final product. This study demonstrates the importance of considering nutrition when expressing and comparing the carbon footprints of nutrient-dense foods such as lamb. This approach could also help inform discussions around the optimal diets for lamb production systems from both a human nutrition and environmental sustainability perspective.
Lamb production systems are under increasing pressure to reduce their environmental footprint, particularly greenhouse gas (GHG) emissions such as methane (Garnett, 2011; Gerber et al., 2013; Jones et al., 2014a). In recent years, carbon footprinting of farms and the resultant produce (e.g., meat) has been increasingly used to estimate resultant environmental impacts (Edwards-Jones et al., 2009; de Vries and de Boer, 2010; Röös et al., 2013; Jones et al., 2014b). Calculating a farm’s carbon footprint offers the opportunity to identify sources of high emissions as well as compare emissions from different farming systems. However, such approaches rarely consider the carbon footprint of lamb relative to its nutritional density as a food product, as the standard functional unit for expressing lamb carbon footprint is per unit of product, e.g., kg CO2e/kg of liveweight (lwt) or kg CO2e/kg of deadweight (dwt; Edwards-Jones et al., 2009; Jones et al., 2014a,b; Ripoll-Bosch et al., 2013). While this mass-based functional unit is useful for comparing efficiencies of different farming systems (Hyland et al., 2016; McAuliffe et al., 2018a), it does not reflect the nutritional value of the product to humans. Several different approaches have been taken to address this, including using a nutritional functional unit to model carbon footprint while considering nutrient density (McLaren, 2021; McAuliffe et al., 2023a).
Ensuring an appropriate nutrient to use as a functional unit is paramount, as this can directly affect carbon footprint calculations. Previous research has used protein as a nutritional functional unit (e.g., Poore and Nemecek, 2018; Xu et al., 2018). Protein as a nutritional functional unit is useful due to simplicities in data processing; however, it can be considered a rudimentary approach as it does not reflect the impact of individual amino acids and intricacies associated with digestion and absorption (Sonesson et al., 2017; McLaren, 2021). Consequently, protein quality has been incorporated into nutritional functional units. For example, McAuliffe et al. (2023b) used an assessment called the Digestible Indispensable Amino Acid Score (DIAAS), which generates a protein quality “adjusted” functional unit. While this is a useful metric for studies comparing a single nutrient, a product’s complete nutritive value is not accurately reflected. Nutrition density scores (NDS) provide a single functional unit in which multiple nutrients can be assessed. The most cited approach for using NDS to express emissions is the Nutrient Rich Food (NRF9.3; Fulgoni et al., 2009) scoring system which accounts for nine nutrients including protein, selected minerals and vitamins, polyunsaturated fatty acids (PUFA) and three nutrients which are to be limited, namely, saturated fatty acids (SFA), sodium and added sugars. Given the complexities and importance of carbon footprinting for environmental targets, policy and consumers, the use of an appropriate functional unit is paramount for accurate determination of a product’s nutrient density and carbon footprint (Capper, 2021).
Research has identified that while protein and amino acid profiles of meat remain largely constant across the diets on which livestock are reared, fat content and lipid profiles are heavily influenced by animal nutrition (Scollan et al., 2006). Most notably, grass-based systems have been found to have higher levels of omega-3 PUFA than systems feeding concentrates (Fisher et al., 2000; Warren et al., 2008). Omega-3 PUFA is a functional unit of great importance due to its potential health benefits and nutraceutical properties in humans, e.g., reducing the risk of cardiovascular disease and other inflammatory diseases (Swanson et al., 2012). Consequently, omega-3 PUFA as a single nutrient functional unit has been explored to express emissions, particularly when comparing farming systems (McAuliffe et al., 2018b). Lamb production systems also vary across the world, from low-input pastoral systems to higher-input systems feeding concentrates for the latter ‘finishing’ period. In the United Kingdom, many farms are typically grass-based systems, but some will provide supplementary concentrates and/or forage crops [e.g., swede (Brassica napus) or stubble turnips (Brassica rapa)] during the autumn/winter finishing period as grass availability and quality reduces (Barry, 2013).
To date, no studies have explored the effect of finishing diets on the carbon footprint of lamb expressed on a nutritional basis. Using data gathered on farms adopting one of four distinct finishing diets and data from the produced meat, this study applies a dual approach to evaluate the impacts of diet on the carbon footprint of lamb expressed on both a mass and nutritional basis, using omega-3 PUFA in 1 kg of fresh muscle as a functional unit.
This paper is based on data from a larger 5-year study that included four balanced design trials. The Welsh Lamb Meat Quality Project conducted research trials across the United Kingdom, exploring on-farm and processing factors that may influence meat eating and nutritional quality. The on-farm factors were investigated across four trials, and included treatments of breed type, lamb gender, muscle cut, lamb finishing diet, daily liveweight gain, seasonality, lamb sire, and processing factors including length of meat ageing period, carcass hanging and packaging (Hybu Cig Cymru – Meat Promotion Wales, 2023). Lamb numbers per treatment were balanced within each trial; however, numbers differed across trials due to lamb availability. Trials were conducted with four Protected Geographical Indication (PGI) approved Welsh abattoirs (DEFRA, 2021) that had previous experience of participating in large trials.
The abattoirs identified lamb producers that could supply lambs for the project (based on the specific trials treatments that were required, e.g., supply lambs of a certain sex, finished on specific diets). A minimum of 24 lambs per farm were needed to reach a target slaughter date. The overall study aim was to research Welsh lamb eating quality across the range of systems that reflect production across the year. As such, the diet of the lambs was representative of those at different seasons / time of year. For example, forage-based crops can only be sown and used for finishing lambs at certain times (Hybu Cig Cymru – Meat Promotion Wales, 2018).
Farm data were collected from 33 farms feeding one of four distinct finishing diets: forage crops (n = 5), grass (n = 7), concentrates (n = 6), and grass and concentrates (n = 15). The forage crop diet consisted of brassicas, fodder beet and forage rape. In the concentrates finishing system, lambs were all fed indoors on a diet of concentrates, barley, crimped barley or coarse mix, whereas the grass and the grass and concentrates diets were all fed outdoors and exclusively on grass and grass and concentrates, respectively. Farm data were self-reported by participating farmers using digital farm information surveys. All farms produced lambs to PGI Welsh lamb standards (DEFRA, 2021). In total, there were 60 lambs fed the forage crop diet, 90 lambs fed the grass diet, 66 lambs fed the concentrates diet, and 228 lambs fed the grass and concentrates diet (Table 1). Lambs were born between January 2020 and April 2022 and their age was recorded as the number of days between the average lambing date and the date of slaughter. Lambs consisted of several breeds: terminal sire (n = 382), hill (n = 38) and cross-breeds (n = 24). Previous studies have found breed could potentially affect meat-eating quality (Fisher et al., 2000; Arsenos et al., 2002), therefore breed was controlled for in the statistical design of the study. Terminal sire breeds included Aberfield, Abermax, Charollais, Lleyn, Primera, Suffolk and Texel. The hill breed type included Beulah Speckled Face, Welsh Mountain and Torddu. Lambs were a mixture of male (entire n = 288; castrated n = 72) and females (n = 84; Table 1). Individual lamb weights were recorded on a fortnightly basis over the 6-week finishing period to calculate their liveweight gain for that period. In cases where specific data were difficult to obtain or where any data were missing, recently published UK data or standardised estimates were used. This was sourced predominantly from SRUC’s Farm Management Handbook (Beattie, 2022) and Feedipedia (Heuzé et al., 2015). For example, data were collected for diet type; however, actual feed consumption was not included. Therefore, assumptions were made on forage and concentrate intake based on example finishing systems and values from SRUC’s Farm Management Handbook (Beattie, 2022).
Table 1. Summary of the mean key performance indicators (± standard error) over the 6-week finishing period and number of farms carbon footprinted for each finishing diet.
Lambs were selected at the target carcass weight of 16–22 kg and conformation grade of E, U, R and fat class 2, 3 L, 3H (Hybu Cig Cymru – Meat Promotion Wales, 2012). From the farms selected that provided whole farm data, 444 carcasses were available for analysis. Carcasses were weighed directly after slaughter to calculate the killing out percentage (KO%). Three of the largest lamb muscles used in other lamb sensory scientific studies (Bonny et al., 2018; Pannier et al., 2018; MSA, 2019; Pannier et al., 2019) were selected using the Meat Standards Australia cooking protocol, being the longissimus dorsi (Loin; n = 444), semimembranosus (Topside; n = 203) and gluteus medius (Chump cut; n = 96). The longissimus dorsi was analysed for all lambs (number of lambs from each diet, breed type and gender can be found in Section 2.1). The semimembranosus analysed included 36 lambs fed the forage crop diet, 36 lambs fed the grass diet, 18 lambs fed the concentrates diet, and 113 lambs fed the grass and concentrates diet. Lambs from the semimembranosus analysed also consisted of several breeds: terminal sire (n = 173), hill (n = 8) and cross-breeds (n = 22). All semimembranosus samples analysed came from ram lambs (n = 203). Eight days post slaughter, the muscle pH was recorded for each cut. Muscles were stored at −20°C until nutritional analysis.
Fatty acid composition was determined by the method of O’Fallon et al. (2007). Lean lamb muscle was hydrolysed with potassium hydroxide in methanol. The potassium hydroxide was neutralised, and the free fatty acids methylated by acid catalysis using sulphuric acid. Fatty acid methyl esters were extracted into hexane and analysed by GC-FID using a CP-SIL 88 column (100 m × 250 μm × 0.2 μm). Intramuscular fat was determined by the method of Folch et al. (1956) with the percentage of extracted fat calculated gravimetrically.
For total amino acid analysis, 100 g of fresh muscle was hydrolysed in constant boiling hydrochloric acid. Samples were then dried down, diluted and analysed on a Waters 2,695 pump/injector system. The individual amino acids were separated by ion exchange chromatography on a strong cation exchange resin using sodium citrate buffer gradients of increasing pH. The ninhydrin reagent was pumped using a Waters 1,515 isocratic pump. The ninhydrin reaction occurs in a heated reaction coil at 125°C, and the derivatized amino acids are detected using a Waters 2,487 variable wavelength UV/VIS detector.
Mineral analysis was carried out using a two-stage microwave digestion followed by Inductively Coupled Plasma Optical Emission Spectroscopy using wavelengths 238.2 and 213.9 nm for iron and zinc, respectively. ERM-BB184 Bovine Muscle from the Joint Research Centre of the European Commission was used as a quality control material. ISO 17034 certified reference standards for zinc and iron were purchased from ROMIL Ltd., Cambridge, United Kingdom.
The full nutritional analysis methods are available in the Supplementary material.
Baseline carbon footprints were calculated using Agrecalc (Agricultural Resource Efficiency Calculator).1 Agrecalc was developed by Scotland’s Rural College and has been found to be among the best-performing carbon accounting tools in terms of transparency, methodology and allocation for use on UK farms (Sykes et al., 2017). The system boundary for Agrecalc is “cradle-to-grave,” i.e., all emissions from agricultural production from the birth of the animal to the farm gate. The tool uses methods from the latest 2019 refinements to the 2006 IPCC Guidelines for National Greenhouse Gas Inventories and is certified to PAS2050 standards (2011). Agrecalc follows IPCC (2019) Tier 2 country-specific guidelines for all livestock and manure management CH4 and N2O emissions. Direct N2O emissions from soil following fertiliser and manure application also used IPCC (2019) Tier 2 calculations. IPCC (2019) Tier 1 methodology was used to calculate N2O emissions from crop residues and indirect N2O emissions. DEFRA, (2021) EFs were employed for calculating emissions relating to energy usage. Emissions for imported feed and embedded fertiliser were based on values from the Dutch Feedprint database (Vellinga et al., 2013) and Kool et al. (2012), respectively. Data required to calculate sequestration estimates were not provided, therefore, carbon sequestration was not considered in this study.
For conversion of non-CO2 gases, Agrecalc uses the global warming potential over a 100-year period (GWP100) published in the fourth assessment report (AR4) which are consistent with National Inventory reporting. Methane has a GWP100 of 25 and the value for N2O is 298 (IPCC, 2007). It is important to note that these values are different from those in the most recent assessment report (AR6; IPCC, 2023).
Emissions from Agrecalc were expressed as both GHG emissions per unit of product (i.e., kg CO2e/kg of deadweight (dwt)) and GHG emissions per unit of nutrition. To calculate the latter, the value of mg omega-3 measured in 100 g of fresh muscle, determined as described in Section 2.2, was converted to the equivalent in g omega-3 in 1 kg of fresh muscle. The calculated GHG emissions per kg dwt were then divided by this to give kg CO2e/g omega-3, giving the GHG emissions per unit of nutrition.
For individual variables, models were fitted using mixed effects models in R (R Core Team, 2022). Models were fitted using the lme4 package (Bates et al., 2015) and value of ps were calculated using Satterthwaite’s method from the lmerTest package (Kuznetsova et al., 2017). In all models, Farm was included as a random effect and models included diet, breed type and gender as factors. This approach allowed the analysis of the data that was unbalanced in breed and gender while controlling for any differences in these factors not of direct interest. Gender was not included for the semimembranosus models as all the lambs in this group were male. Pairwise differences were calculated using the emmeans package (Lenth, 2023) using a Tukey correction for multiple comparisons. After fitting diagnostic plots for all models were checked for any evidence of heterogeneity of variance or non-normality of errors. For a few variables a log (or log+1) transformation was applied to correct for heterogeneity of variance. Data were plotted using the ggstats package (Larmarange, 2023).
To assess the effect of diet on finishing system performance, a one-way ANOVA and Tukey pairwise-comparison were performed on individual key performance indicators (KPIs). A one-way ANOVA was conducted to assess the effect of diet on mass-based product emissions and a two-way ANOVA was used to test for an association between diet and muscle cut on nutrition-based product emissions. Multiple pairwise-comparison between the means of groups were then performed using Tukey multiple pairwise-comparisons. The level of statistical significance was set at 5% for all tests in this study.
Lamb growth and weights varied between finishing diets. Lambs from the forage crops and concentrates diet had significantly higher liveweights at the start of the finishing period compared to lambs on grass and grass and concentrates diet (Table 1). Lamb age varied at the start of the finishing period to reflect the inherent differences in the production and seasonality of the different finishing systems according to industry practice. Lambs from the grass and concentrates diet had significantly higher liveweight gain and total weight gain over the finishing period than lambs from all other diets. Lambs on the forage crops diet had the highest liveweight at slaughter whereas the grass diet had the lowest liveweight at slaughter (Table 1). Killing out percentages did not vary significantly between diets. Lambs from the grass only diet had significantly lower carcass weights compared to lambs on all other diets (Table 1).
Although not directly related to the finishing diet and likely influenced by how lambs were selected, time on farm varied between lambs from different finishing systems. Lambs from the concentrates diet were on farm for the longest time (mean 9.2 ± 0.17 months) compared to lambs from: the forage crops diet which were on farm for 8.5 ± 0.19 month (p > 0.05), grass diet which were kept for 6.0 ± 0.22 months (p < 0.001) and grass and concentrates diet which were on farm for the least time at 5.2 ± 0.11 months (p < 0.001).
There was no significant difference between the amino acid content of gluteus medius across the four diets (Supplementary Table 1; p > 0.05). As expected, there were also no significant differences found in the iron content of both the longissimus dorsi and semimembranosus across all four diets (p > 0.05), the iron content of muscle is more associated with age than diet (Pannier et al., 2014). Additionally, there was no significant differences in the zinc content in the semimembranosus across all diets, however, diet did have an effect on the zinc content of the longissimus dorsi (p < 0.001).
Fat percentage varied significantly between finishing diets in both the longissimus dorsi and semimembranosus (p < 0.05). Differences were noted in the total fatty acid composition and saturated fatty acid in the longissimus dorsi across the four diets (p < 0.05); however, there were no differences found in total fatty acid content of the semimembranosus across diets (Table 2; discussed in Section 4.1). There were significant differences in the total omega-3 PUFA content in the longissimus dorsi across the four finishing diets (p < 0.001), with the highest and lowest being reported in muscle from the grass and concentrate diets, respectively. The analysis controlled for the differences in breed type (longissimus dorsi and semimembranosus) and gender (longissimus dorsi only). There was not a consistent pattern among fatty acids, with breed type and gender being significantly different in some but not all of the variables (full results can be found in Supplementary Table 2). For the variable of interest (omega-3 PUFA), breed type had a significant effect in the semimembranosus (p < 0.05) but not in the longissimus dorsi (p > 0.05). There was also a significant difference in omega-3 PUFA between genders in the longissimus dorsi (p < 0.05).
Table 2. Estimated marginal mean (± standard error) fatty acid composition of lamb meat from four finishing diets averaged over breed type and gender for longissimus dorsi and breed type for semimembranosus.
There were significant differences in levels of palmitic acid (C16:0) and stearic acid (C18:0) across the four diets in the longissimus dorsi muscle (p < 0.05), with no differences detected in the semimembranosus (Table 2). Linoleic acid (C18:2 n-6) was significantly greater in the concentrate diet and lowest in the grass diet in the longissimus dorsi muscle. There was no difference between C18:2 n-6 levels from lamb finished on the forage crops and grass and concentrate diet.
Lamb from the forage crops diet and grass diet had significantly higher alpha-linolenic acid (C18:3 n-3) in both the longissimus dorsi and semimembranosus with levels being reported as 62 and 61 mg/100 g and 71 and 73 mg/100 g, respectively, compared to the concentrate diet where 42 mg/100 g was reported for both muscles. There were differences in levels of eicosapentaenoic acid (C20:5 n-3; p < 0.001), docosapentaenoic acid (C22:5 n-3; p < 0.05) and docosahexaenoic acid (C22:6 n-3; p < 0.001) across diets in the longissimus dorsi muscle, however, no differences in any long chain omega-3 PUFA was noted in the semimembranosus.
Omega-3 PUFA is known to have a variety of health benefits such as reduced risk of cardiovascular disease and other inflammatory diseases (Swanson et al., 2012). Omega-3 PUFA composition of lamb is also known to vary significantly between animal diets, particularly between grass and concentrate feeding (Fisher et al., 2000; Warren et al., 2008). Our finding are in line with other previous studies. Grams of omega-3 in 1 kg of fresh muscle (kg CO2e/g-omega-3) was selected as a functional unit to express emissions on a nutritional basis.
Mass-based product emissions varied significantly from 21.8–36.4 kg CO2e/kg dwt across finishing diets (p < 0.001). There were significant differences in mass-based product emissions between all diets (p < 0.05) apart from the forage crops and grass and concentrates diets (p > 0.05). Lambs from the concentrates diet had the lowest average mass-based product emissions (25.0 kg CO2e/kg dwt) while those from the grass systems had the highest (28.1 kg CO2e/kg dwt; Figure 1; p < 0.001). Variation in mass-based product emissions was also seen within the same diets, for example, grass and concentrates diet, highest mass-based product emissions (36.4 kg CO2e/kg dwt) were more than 1.6 times higher than the lowest (22.2 kg CO2e/kg dwt).
Figure 1. Mean emissions estimates (± standard error) for longissimus dorsi and semimembranosus muscle cuts for each finishing diet expressed as mass-based product emissions and nutrition-based product emissions. Different lower-case letters indicate statistically significant differences in diet within each individual emissions measure at the 5% level.
Further variation was seen when accounting for omega-3 content, with nutrition-based emissions ranging from 12.1–73.8 kg CO2e/g omega-3. Nutrition-based emissions were greater for longissimus dorsi than for semimembranosus across all diets other than for grass and concentrates, although this difference was not statistically significant (p > 0.05). Significant differences in nutrition-based product emissions between the two muscle cuts were only found in the forage crops diet (p < 0.01; data not shown). The semimembranosus cut of lambs from the forage crops diet had the lowest average nutrition-based product emissions (19.2 kg CO2e/g omega-3; Figure 1), whereas the semimembranosus cut of lambs from the grass and concentrates diet had the highest nutrition-based product emissions (29.4 kg CO2e/g omega-3; p < 0.001).
Significant differences were found in the total fatty acid composition and saturated fatty acids in the longissimus dorsi, but not in the semimembranosus across the four finishing diets. This it is likely due to the longissimus dorsi having a higher total fat content than the semimembranosus (Supplementary Table 1). Differences were found in the fatty acid composition of the semimembranosus across finishing diets, however, these differences were not significant. This may be due to the lower number of semimembranosus samples analysed (n = 203) compared to the longissimus dorsi (n = 444), due to this study being part of a larger research trial looking at multiple variables, one being muscle/cut. Nonetheless, significant differences were found in C18:3 n-3 and the n-6/n-3 ratio in the semimembranosus of lambs across finishing diets, which was ultimately a key focus of the study.
The total fat content for lamb meat was highest in the longissimus dorsi from the forage crops diet and lowest in semimembranosus from the grass and concentrates diet. Pasture feeding is often associated with lower meat fat content as found by Fisher et al. (2000) and Nuernberg et al. (2008), who reported 1963 vs. 1853 mg/100 g and 2,100 vs. 1800 mg/100 g muscle in concentrate- and grass-fed lamb, respectively. Conversely, Demirel et al. (2006) reported lambs finished on grass hay had higher total fatty acid, compared to concentrate feeding. This is similar to the saturated fatty acid composition in the longissimus dorsi in the current study, where again the grass and concentrates diet was lowest. However, the saturated fatty acid composition did not differ significantly between the diets in the semimembranosus.
Levels of C18:2 n-6 were higher in lambs that had been fed concentrates as part of or as a sole dietary component. This is unsurprising as concentrates are rich in linoleic acid, whereas grass and forage crops would have relatively low levels. Lambs from the grass and concentrates diet had significantly less C18:2 n-6 compared to the concentrates diet. The mixture of grass and concentrates at dietary components will dilute the amount of C18:2 n-6 being deposited into muscle (Scollan et al., 2017). This dominant C18:2 n-6 influence is also reflected in the n-6/n-3 ratio, which is highest for the concentrate diet and lowest for the grass diet.
The total omega-3 PUFA composition varied across the four diets, with the forage crops and grass diet having the highest amount and the lowest being reported in the concentrates diet for both muscle cuts. Studies in lamb have reported total omega-3 PUFA as 102 and 44 mg/100 g of meat (Fisher et al., 2000), and 78 and 67 mg/100 g of meat (Kitessa et al., 2010) in animals fed on grass and concentrate diets, respectively. This was supported by a study concluding that lambs reared on grass had significantly higher total omega-3 PUFA levels compared to lambs reared on a grass and concentrate and concentrate and hay diet (Boughalmi and Araba, 2016).
Lamb from the forage crops diet and grass diet had significantly higher C18:3 n-3 in the longissimus dorsi compared to the concentrate diet. It is well acknowledged that grass is rich in C18:3 n-3. This is because plant chloroplasts can uniquely synthesise (de novo) long chain fatty acids (>18 carbons; Harwood, 1999). Levels of C18:3 n-3 in grass and other plants are influenced by season, species, location and environment (e.g., temperature and light exposure; Elgersma et al., 2003; Mir et al., 2006; Tsvetkova and Angelow, 2010; Yalcin et al., 2011; De Brito et al., 2017). This also explains why forage crops and other plant-based materials have high levels of C18:3 n-3. The ‘grass effect’ is reflected in the data presented, particularly by the titration effect seen between the grass, grass and concentrates and concentrate diets, where any impact is diluted. There were some significant differences reported for the long chain omega-3 PUFAs (C20:5, C22:5 and C22:6 n-3) across the four finishing diets which is contrary to the findings of others (Fisher et al., 2000; Demirel et al., 2006). Higher levels of long chain omega-3 PUFAs including C20:5 n-3 and C22:6 n-3 were found in the grass and forage crops in the longissimus dorsi. Although lamb diets consisting solely of grass have very little amounts of long chain omega-3 PUFAs (as pasture species are primarily dominant in C18:3 n-3), small increases are not surprising as conversion of C18:3 n-3 to longer chain omega-3 via elongation and desaturation processes can occur in the lamb (Bessa et al., 2015). Nutrition and genetics are the two most influencing factors affecting fatty acid composition in muscle (Scollan et al., 2014; Dervishi et al., 2019), meaning any variation seen is likely due to lambs being on a grass-based diet more so than the actual species composition in the grazed pastures (Dierking et al., 2010; Scollan et al., 2017).
Due to the differences in omega-3 PUFA composition between the four diets, grams of omega-3 was selected as a functional unit to express emissions on a nutritional basis. While the n-6/n-3 ratio was also considered for use as a nutritional functional unit, we focus on omega-3 PUFA because it accounts for absolute amounts, rather proportions of fatty acids present (EFSA Panel on Dietetic Products, Nutrition, and Allergies (NDA), 2010). Omega-3 PUFA is known to vary between grass and concentrate based diets (Fisher et al., 2000; Warren et al., 2008), and has been previously used as a functional unit to express emissions while comparing farming systems (McAuliffe et al., 2018b). Additionally, omega-3 PUFA is important in human nutrition with documented health benefits such as reducing the risk of cardiovascular disease and other inflammatory diseases (EFSA Panel on Dietetic Products, Nutrition, and Allergies (NDA), 2010; Swanson et al., 2012).
Mass-based product emissions varied significantly across finishing systems, which largely reflects the variation in efficiencies between the different diets. The concentrates diet had the lowest average mass-based product emissions while the grass systems had the highest. Although lambs from the concentrates diet were on farm for longer and the bought-in feed would lead to greater embedded GHG emissions, concentrates have a lower fibre content which can result in lower CH4 production (Yan et al., 2010; van Wyngaard et al., 2018). Lambs on the concentrates diet also had higher carcass weights and KO% compared to the lambs form the grass diet, resulting in lower emissions per kg of product. Considerable variation was also seen in mass-based product emissions of finishing systems within the same diets. This highlights the difference in efficiencies of finishing systems within the same diet. This could be explained by animal health issues (e.g., lameness or gastrointestinal worm challenge), the quality of the diet offered, and genetic variation.
The significantly higher omega-3 PUFA content of the forage crops diet resulted in the semimembranosus cuts from this diet having the lowest nutrition-based product emissions. Similarly, grass systems had the lowest nutrition-based product emissions for the longissimus dorsi due to lambs from the grass diets having the highest omega-3 PUFA content of the longissimus dorsi. The grass and concentrates diet had the highest nutrition-based product emissions for both the longissimus dorsi and semimembranosus. This is likely a result of their initially higher mass-based product emissions and relatively lower omega-3 PUFA content compared to that of the forage crops and grass diets. The concentrates diet had lowest omega-3 PUFA content for both the longissimus dorsi and semimembranosus resulting in higher nutrition-based product emissions. However, as the concentrates diet had the lowest mass-based emissions to begin with, this effect is somewhat masked.
Across all diets except the forage crops diet, there was no significant difference in nutrition-based product emissions between the longissimus dorsi and semimembranosus. This is due to the similar average omega-3 PUFA content between longissimus dorsi and semimembranosus. For all systems, except for the grass and concentrate diet, nutrition-based product emissions were higher in the longissimus dorsi than in the semimembranosus. This could be explained by the forage crops and grass diets having higher omega-3 PUFA contents in their semimembranosus cuts than in their longissimus dorsi. This is likely due to longissimus dorsi having a higher SFA and lower PUFA content than the semimembranosus. Fowler et al. (2019) also found the longissimus dorsi of lambs in extensive systems had lower omega-3 PUFA content than the semimembranosus. However, the forage crops diet showed significant differences in nutrition-based product emissions between longissimus dorsi and semimembranosus. This result should be treated with caution due to the small number of farms in this study on the forage crops diet as well as the variation of feeds and therefore fatty acid composition of lambs within the forage crops diet. For example, the forage crops diet consisted of finishing systems feeding brassica, fodder beet and forage rape, which may all affect the nutritional composition of lambs differently. Even within diets that were finished on solely grass, grass quality will vary between farms and therefore this will likely impact the nutritional composition of lambs, particularly omega-3 PUFA content (Howes et al., 2015).
This study found marginally lower nutrition-based emissions for lamb production systems than previous studies. McAuliffe et al. (2018a) noted lambs on upland and lowland systems had nutrition-based emissions of 30.0 kg CO2e/g omega-3 and 28.7 kg CO2e/g omega-3, respectively. These values are higher than both cuts from the forage crops, grass and concentrates diets found in the present study. However, these differences must be interpreted with caution as different carbon footprinting tools have been used to calculate emissions estimates in this study. Additionally, our study found higher omega-3 PUFA content in lambs across some diets, e.g., 146 mg/100 g from the semimembranosus from the grass diet compared to published values, which reported levels of 103 mg/100 g of meat (Whittington et al., 2006).
The present study highlights the importance of nutritional functional unit when considering health and wellbeing implications of products, especially given the diversity in nutritional fatty acid composition in ruminant products. Using omega-3 PUFA as a nutritional functional unit demonstrated its value and warrants further consideration given the numerous reported benefits optimal consumption has on human health and well-being (Jacobson et al., 2012; Givens, 2015; Singh et al., 2016). Although the lamb in this study will unlikely have a nutraceutical effect at a normal portion size, the aim of this study was to explore the effect of finishing diet on the carbon footprint of lamb expressed on a nutritional basis rather than making recommendations on lamb portion sizes.
This study has uniquely used real farm data to highlight the importance of shifting from mass-based functional units to nutrient-based functional units. While mass-based functional units such as per kg dwt still have a valuable place in comparing production efficiencies of farms, they do not reflect the degree of nutrition provided by consumption of the meat produced from each system.
Some appropriate assumptions had to be made to calculate carbon footprints for each finishing system where some farm data were unavailable. For example, although data were collected for diet type, actual feed consumption was not recorded. Although such assumptions and default values regularly have to be applied in farm carbon footprint studies (Edwards-Jones et al., 2009; Ripoll-Bosch et al., 2013; McAuliffe et al., 2018a), there may be an over- and/or under-estimations of emission estimates as a result. Ensuring a larger sample size with an equal number of finishing systems from each diet would reduce unequal variances between diets and improve the statistical power of results. Nonetheless, although breed type and gender were unbalanced between treatments, farms were selected for this study to represent a cross-section of lamb finishing systems, and therefore these differences in production and seasonality are reflected in the results. For example, hill breeds will more likely be associated with grass-based finishing systems as opposed to concentrates. However, for the variables such as breed type (e.g., hill and cross- breeds) which have lower numbers in each group, there will inevitably be a greater level of uncertainty in the results.
Using a single nutrient functional unit does not reflect the products’ complete nutritive value. Focusing on a single nutrient functional unit could lead to an under or over-supply of other key nutrients. In this study, we have focused purely on omega-3 PUFA, however, there would likely be variation in a number of other fatty acids between finishing diets, for example, conjugated linoleic acid (CLA) which have a high nutraceutical value. Future studies should therefore consider CLA and indeed the full fatty acid profile. Moreover, lamb can provide a considerable range of nutritional benefits that were not considered in this study. Although many parameters (52 fatty acid parameters, 19 amino acid parameters, and two mineral parameters) were collected for this study, measurement of other key nutrients (e.g., vitamins and certain minerals) would generate a fuller nutrient density score (Fulgoni et al., 2009). Moreover, nutrient density scores often consider the daily recommended intake of each nutrient. Nutrients collected in this study were from 100 g of fresh muscle, so future work would need to consider cooking losses of meat if a nutrient density score was to be created. However, nutrient density scores are not without their limitations. The outcomes of nLCAs which employ a nutrient density score are highly dependent on the nutrients which are included in the metric. This means some metrics are more suitable for some foods than others, and other important aspects of nutrition (such as the bioavailability of nutrients and interaction between nutrients) are not captured (Bianchi et al., 2020). Moreover, foods are rarely consumed in isolation and therefore future nLCA studies should consider nutrition at a diet-level (McAuliffe et al., 2018b). Recently, some studies have taken a novel approach which involves a diet-level assessment that accounts for the foods’ effect on human health. For example, Stylianou et al. (2016) developed the Combined Nutritional and Environmental Life Cycle Assessment (CONE-LCA). The CONE-LCA uses a traditional LCA approach and predicts health outcomes following changes in diet, using epidemiological data based on the nutritional quality of food. However, these outcomes will obviously depend on the initial diet and its nutritional status of the individuals making the dietary change.
As with all LCA studies, the results of nLCA depend upon the type of LCA (attributional vs. consequential), where system boundaries are drawn, and the allocation method they employ (Silva, 2021). Clearly, nLCAs also require an extra layer of data relating to the nutritional value of food, introducing additional sources of variation. Studies often rely on a range of external databases for this nutritional information. Although not an issue in this study, data availability and quality are major limitations of nLCA. This includes both primary data from agricultural production and secondary data from agricultural databases. When utilising primary data, there can be concerns of the representativeness of data, particularly if data comes from a single, specific year (Notarnicola et al., 2017). With secondary data, databases exhibit significant variability in terms of detail and completeness and are often biassed towards conventional production in high-income countries (Teixeira, 2015; Carvalho et al., 2023). Moreover, some nLCA studies may require additional information such as nutritional intake recommendation, interactions with other foods, and food processing and preparation (McLaren, 2021). Again, while this was not a limitation in the current study, the lack of available high-quality data will likely limit the wider use and application of nLCA.
Despite the assumptions and limitations of this study, a novel functional unit has been successfully used to compare four finishing diets of lambs and has highlighted the importance of considering nutrition when expressing GHG emissions.
This preliminary assessment is the first of its kind to use real farm and carcass data to assess the effect of finishing diet on lamb carbon footprints expressed on a nutritional basis. Despite recognised limitations, this study has demonstrated the need to consider nutrition when expressing carbon footprints. When a mass-based functional unit was employed, grass diets had on average the highest carbon footprint, however, when omega-3 PUFA content was accounted for, the grass diet had the lowest carbon footprint for the longissimus dorsi. While mass-based functional units can be useful for comparing efficiencies of different farming systems, they do not reflect the function of the final product, human nutrition. Therefore, future work should consider both mass-based and nutrition-based functional units when comparing different farming systems. Future studies should also collect a comprehensive set of carcass and nutritional parameters for emissions to be expressed through a full nutrient density score. This would allow us to accurately determine the role nutrient density of a product plays in environmental sustainability of livestock farming.
The datasets presented in this article are not readily available because the data analysed in this study was obtained from Hybu Cig Cymru – Meat Promotion Wales. Requests to access these datasets should be directed to ET, ZXRob21hc0BoeWJ1Y2lnLmN5bXJ1.
The animal studies were approved by Hybu Cig Cymru – Meat Promotion Wales, Ty Rheidol, Parc Merlin, Glanyrafon Industrial Estate, Aberystwyth SY23 3FF. The studies were conducted in accordance with the local legislation and institutional requirements. Written informed consent was obtained from the owners for the participation of their animals in this study.
LM: Data curation, Formal analysis, Visualization, Writing – original draft. LP: Data curation, Project administration, Writing – original draft. JG: Formal analysis, Writing – review & editing. NS: Funding acquisition, Supervision, Writing – review & editing. AN: Writing – review & editing. ET: Conceptualization, Investigation, Supervision, Writing – review & editing. ES: Data curation, Investigation, Writing – review & editing. CM: Investigation, Resources, Writing – review & editing. AW: Investigation, Resources, Writing – review & editing. SC: Investigation, Resources, Writing – review & editing. LF: Conceptualization, Writing – review & editing. APW: Supervision, Writing – review & editing.
The author(s) declare financial support was received for the research, authorship, and/or publication of this article. This work was funded by the Red Meat Development Programme (RMDP) delivered by Hybu Cig Cymru – Meat Promotion Wales (HCC), which was supported by the Welsh Government Rural Communities – Rural Development Programme 2014–2020, funded by the European Agricultural Fund for Rural Development and the Welsh Government. The research was also part of the Knowledge Economy Skills Scholarships (KESS 2) funded by the Welsh Government’s European Social Fund, part-funded by Hybu Cig Cymru – Meat Promotion Wales (BUK2E030).
The authors would like to thank the farmers and abattoirs that participated in this study.
The authors declare that the research was conducted in the absence of any commercial or financial relationships that could be construed as a potential conflict of interest.
All claims expressed in this article are solely those of the authors and do not necessarily represent those of their affiliated organizations, or those of the publisher, the editors and the reviewers. Any product that may be evaluated in this article, or claim that may be made by its manufacturer, is not guaranteed or endorsed by the publisher.
The Supplementary material for this article can be found online at: https://www.frontiersin.org/articles/10.3389/fsufs.2024.1321288/full#supplementary-material
Arsenos, G., Banos, G., Fortomaris, P., Katsaounis, N., Stamataris, C., Tsaras, L., et al. (2002). Eating quality of lamb meat: effects of breed, sex, degree of maturity and nutritional management. Meat Sci. 60, 379–387. doi: 10.1016/S0309-1740(01)00147-4
Barry, T. N. (2013). The feeding value of forage brassica plants for grazing ruminant livestock. Anim. Feed Sci. Technol. 181, 15–25. doi: 10.1016/j.anifeedsci.2013.01.012
Bates, D., Maechler, M., Bolker, B., and Walker, S. (2015). Fitting linear mixed-effects models. Using lme4. J. Stat. Softw. 67, 1–48. doi: 10.18637/jss.v067.i01
Beattie, A. , (2022). Farm Management Handbook 2022/23. SAC Consulting, Farm Advisory Service (FAS), United Kingdom.
Bessa, R. J. B., Alves, S. P., and Santos-Silva, J. (2015). Constraints and potentials for the nutritional modulation of the fatty acid composition of ruminant meat. Eur. J. Lipid Sci. Technol. 117, 1325–1344. doi: 10.1002/ejlt.201400468
Bianchi, M., Strid, A., Winkvist, A., Lindroos, A.-K., Sonesson, U., and Hallström, E. (2020). Systematic evaluation of nutrition indicators for use within food LCA studies. Sustain. For. 12:8992. doi: 10.3390/su12218992
Bonny, S. P. F., O’Reilly, R. A., Pethick, D. W., Gardner, G. E., Hocquette, J.-F., and Pannier, L. (2018). Update of meat standards Australia and the cuts based grading scheme for beef and sheepmeat. J. Integr. Agric. 17, 1641–1654. doi: 10.1016/S2095-3119(18)61924-0
Boughalmi, A., and Araba, A., (2016). Effect of feeding management from grass to concentrate feed on growth, carcass characteristics, meat quality and fatty acid profile of Timahdite lamb breed. Small Ruminant Res. 144, 158–163. doi: 10.1016/j.smallrumres.2016.09.013
Capper, J. L. (2021). Current issues and controversies in assessing the environmental impacts of livestock production. CABI Rev. 2021. doi: 10.1079/PAVSNNR202116044
Carvalho, C., Correia, D., Costa, S. A., Lopes, C., and Torres, D. (2023). Assessing the environmental impact of diet – influence of using different databases of foods’ environmental footprints. J. Clean. Prod. 416:137973. doi: 10.1016/j.jclepro.2023.137973
De Brito, G. F., Ponnampalam, E. N., and Hopkins, D. L. (2017). The effect of extensive feeding systems on growth rate, carcass traits, and meat quality of finishing lambs. Compr. Rev. Food Sci. Food Saf. 16, 23–38. doi: 10.1111/1541-4337.12230
de Vries, M., and de Boer, I. J. M. (2010). Comparing environmental impacts for livestock products: a review of life cycle assessments. Livest. Sci. 128, 1–11. doi: 10.1016/j.livsci.2009.11.007
DEFRA . Product Specification: Welsh Lamb (PGI). (2021). Available at: https://assets.publishing.service.gov.uk/government/uploads/system/uploads/attachment_data/file/786674/pfn-welsh-lamb-spec.pdf
Dervishi, E., González-Calvo, L., Blanco, M., Joy, M., Sarto, P., Martin-Hernandez, R., et al. (2019). Gene Expression and Fatty Acid Profiling in Longissimus thoracis Muscle, Subcutaneous Fat, and Liver of Light Lambs in Response to Concentrate or Alfalfa Grazing. Front. Genet. 10. doi: 10.3389/fgene.2019.01070
Demirel, G., Ozpinar, H., Nazli, B., and Keser, Ö. (2006). Fatty acids of lamb meat from two breeds fed different forage: concentrate ratio. Meat Sci. 72, 229–235. doi: 10.1016/j.meatsci.2005.07.006
Dierking, R. M., Kallenbach, R. L., and Roberts, C. A. (2010). Fatty acid profiles of Orchardgrass, tall fescue, perennial ryegrass, and alfalfa. Crop Sci. 50, 391–402. doi: 10.2135/cropsci2008.12.0741
Edwards-Jones, G., Plassmann, K., and Harris, I. M. (2009). Carbon footprinting of lamb and beef production systems: insights from an empirical analysis of farms in Wales, UK. J. Agric. Sci. 147, 707–719. doi: 10.1017/S0021859609990165
EFSA Panel on Dietetic Products, Nutrition, and Allergies (NDA) (2010). Scientific opinion on dietary reference values for fats, including saturated fatty acids, polyunsaturated fatty acids, monounsaturated fatty acids, trans fatty acids, and cholesterol. EFSA J. 8:1461. doi: 10.2903/j.efsa.2010.1461
Elgersma, A., Ellen, G., Van Der Horst, H., Muuse, B. G., Boer, H., and Tamminga, S. (2003). Influence of cultivar and cutting date on the fatty acid composition of perennial ryegrass (Lolium perenne L.). Grass Forage Sci. 58, 323–331. doi: 10.1046/j.1365-2494.2003.00384.x
Fisher, A. V., Enser, M., Richardson, R. I., Wood, J. D., Nute, G. R., Kurt, E., et al. (2000). Fatty acid composition and eating quality of lamb types derived from four diverse breed× production systems. Meat Sci. 55, 141–147. doi: 10.1016/S0309-1740(99)00136-9
Folch, J., Lees, M., and Sloane-Stanely, G. H. (1956). A simple method for isolation and purification of total lipids from animal tissue. J. Biol. Chem. 226, 497–509. doi: 10.1016/S0021-9258(18)64849-5
Fowler, S. M., Morris, S., and Hopkins, D. L. (2019). Nutritional composition of lamb retail cuts from the carcases of extensively finished lambs. Meat Sci. 154, 126–132. doi: 10.1016/j.meatsci.2019.04.016
Fulgoni, V. L. III, Keast, D. R., and Drewnowski, A. (2009). Development and validation of the nutrient-rich foods index: a tool to measure nutritional quality of foods. J. Nutr. 139, 1549–1554. doi: 10.3945/jn.108.101360
Garnett, T. (2011). Where are the best opportunities for reducing greenhouse gas emissions in the food system (including the food chain)? The challenge of global food sustainability. Food Policy 36, S23–S32. doi: 10.1016/j.foodpol.2010.10.010
Gerber, P. J., Hristov, A. N., Henderson, B., Makkar, H., Oh, J., Lee, C., et al. (2013). Technical options for the mitigation of direct methane and nitrous oxide emissions from livestock: a review. Animal 7, 220–234. doi: 10.1017/S1751731113000876
Givens, D. I. (2015). Manipulation of lipids in animal-derived foods: can it contribute to public health nutrition? Eur. J. Lipid Sci. Technol. 117, 1306–1316. doi: 10.1002/ejlt.201400427
Harwood, J.L. (1999). Plant Fatty Acid Synthesis. Available at: https://lipidlibrary.aocs.org/chemistry/physics/plant-lipid/plant-fatty-acid-synthesis. Accessed 10th September 2023.
Heuzé, V., Tran, G., Delagarde, R., Bastianelli, D., and Lebas, F., (2015). Feedipedia-animal feed resources information system. Rome Italy: FAO.
Howes, N. L., Bekhit, A. E.-D. A., Burritt, D. J., and Campbell, A. W. (2015). Opportunities and implications of pasture-based lamb fattening to enhance the long-chain fatty acid composition in meat. Compr. Rev. Food Sci. Food Saf. 14, 22–36. doi: 10.1111/1541-4337.12118
Hybu Cig Cymru – Meat Promotion Wales , (2012). Know what you’re looking at A guide to stock judging. Available at: https://meatpromotion.wales/images/resources/HCC_Llyfryn_YFC_cropped.pdf
Hybu Cig Cymru – Meat Promotion Wales . (2018). Lamb finishing systems. Available at: https://meatpromotion.wales/images/resources/LAMB_FINSIHING_ENGLISH_VERSION.pdf
Hybu Cig Cymru – Meat Promotion Wales . (2023). Welsh Lamb Meat Quality Project – Final Project Report. Available at: https://meatpromotion.wales/en/industry-projects/red-meat-development-programme/cynllun-safon-bwyta-cig-oen/results
Hyland, J. J., Styles, D., Jones, D. L., and Williams, A. P. (2016). Improving livestock production efficiencies presents a major opportunity to reduce sectoral greenhouse gas emissions. Agric. Syst. 147, 123–131. doi: 10.1016/j.agsy.2016.06.006
IPCC (2007) in Climate change 2007: synthesis report. contribution of working groups I, II and III to the fourth assessment report of the intergovernmental panel on climate change. eds. R. K. Pachauri and A. Reisinger (Geneva, Switzerland: IPCC), 104.
IPCC (2019) in Refinement to the 2006 IPCC guidelines for National Greenhouse gas Inventories. eds. E. Calvo Buendia, K. Tanabe, A. Kranjc, J. Baasansuren, M. Fukuda, and S. Ngarize, et al. (Switzerland: IPCC)
IPCC (2023) in Summary for policymakers. In: climate change 2023: synthesis report. contribution of working groups I, II and III to the sixth assessment report of the intergovernmental panel on climate change. eds. Core Writing Team , H. Lee and J. Romero (Geneva, Switzerland: IPCC), 1–34.
Jacobson, T. A., Glickstein, S. B., Rowe, J. D., and Soni, P. N. (2012). Effects of eicosapentaenoic acid and docosahexaenoic acid on low-density lipoprotein cholesterol and other lipids: a review. J. Clin. Lipidol. 6, 5–18. doi: 10.1016/j.jacl.2011.10.018
Jones, A. K., Jones, D. L., and Cross, P. (2014a). The carbon footprint of lamb: sources of variation and opportunities for mitigation. Agric. Syst. 123, 97–107. doi: 10.1016/j.agsy.2013.09.006
Jones, A. K., Jones, D. L., and Cross, P. (2014b). The carbon footprint of UK sheep production: current knowledge and opportunities for reduction in temperate zones. J. Agric. Sci. 152, 288–308. doi: 10.1017/S0021859613000245
Kitessa, S., Liu, S., Briegel, J., Pethick, D., Gardner, G., Ferguson, M., et al. (2010). Effects of intensive or pasture finishing in spring and linseed supplementation in autumn on the omega-3 content of lamb meat and its carcass distribution. Anim. Prod. Sci. 50, 130–137. doi: 10.1071/AN09095
Kool, A., Marinussen, M., Blonk, H., and Consultants, B. (2012). LCI data for the calculation tool Feedprint for greenhouse gas emissions of feed production and utilization. GHG Emissions of N, P and K fertiliser production :20.
Kuznetsova, A., Brockhoff, P. B., and Christensen, R. H. B. (2017). lmerTest package: tests in linear mixed effects models. J. Stat. Softw. 82, 1–26. doi: 10.18637/jss.v082.i13
Larmarange, J , (2023). Ggstats: extension to 'ggplot2' for plotting stats. R package version 0.3.0. Available at: https://CRAN.R-project.org/package=ggstats.
Lenth, R , (2023). Emmeans: estimated marginal means, aka least-squares means. R package version 1.8.5. Available at: https://CRAN.R-project.org/package=emmeans.
McAuliffe, G. A., Takahashi, T., Beal, T., Huppertz, T., Leroy, F., Buttriss, J., et al. (2023a). Protein quality as a complementary functional unit in life cycle assessment (LCA). Int. J. Life Cycle Assess. 28, 146–155. doi: 10.1007/s11367-022-02123-z
McAuliffe, G. A., Takahashi, T., and Lee, M. R. F. (2018b). Framework for life cycle assessment of livestock production systems to account for the nutritional quality of final products. Food and Energy Security 7:e00143. doi: 10.1002/fes3.143
McAuliffe, G. A., Takahashi, T., Lee, M. R. F., Jebari, A., Cardenas, L., Kumar, A., et al. (2023b). A commentary on key methodological developments related to nutritional life cycle assessment (nLCA) generated throughout a 6-year strategic scientific programme. Food Energy Security. 12:e480. doi: 10.1002/fes3.480
McAuliffe, G. A., Takahashi, T., Orr, R. J., Harris, P., and Lee, M. R. F. (2018a). Distributions of emissions intensity for individual beef cattle reared on pasture-based production systems. J. Clean. Prod. 171, 1672–1680. doi: 10.1016/j.jclepro.2017.10.113
McLaren, S. , (2021). Integration of environment and nutrition in life cycle assessment of food items: opportunities and challenges. FAO, Rome, Italy.
Mir, P., Bittman, S., Hunt, D., Entz, T., and Yip, B. (2006). Lipid content and fatty acid composition of grasses sampled on different dates through the early part of the growing season. Can. J. Anim. Sci. 86, 279–290. doi: 10.4141/A05-050
MSA , (2019). Meat standards Australia sheepmeat information kit. Meat and Livestock Australia. Available at: https://www.mla.com.au/globalassets/mla-corporate/marketing-beef-and-lamb/documents/meat-standards-australia/msa-sheep-tt-july-2019-lr.pdf
Notarnicola, B., Sala, S., Anton, A., McLaren, S. J., Saouter, E., and Sonesson, U. (2017). The role of life cycle assessment in supporting sustainable Agri-food systems: a review of the challenges. J. Clean. Prod. 140, 399–409. doi: 10.1016/j.jclepro.2016.06.071
Nuernberg, K., Fischer, A., Nuernberg, G., Ender, K., and Dannenberger, D. (2008). Meat quality and fatty acid composition of lipids in muscle and fatty tissue of Skudde lambs fed grass versus concentrate. Small Rumin. Res. 74, 279–283. doi: 10.1016/j.smallrumres.2007.07.009
O’Fallon, J. V., Busboom, J. R., Nelson, M. L., and Gaskins, C. T. (2007). A direct method for fatty acid methyl ester synthesis: application to wet meat tissues, oils and feedstuffs. J. Anim. Sci. 85, 1511–1521. doi: 10.2527/jas.2006-491
Pannier, L., Corlett, M., Payne, C., and Pethick, D., (2019). Meat and Livestock Australia limited - cuts based MSA lamb development – lamb turn off and packaging effects. Meat and Livestock Australia Limited. Available at: https://www.mla.com.au/contentassets/3d4209c303ae44558f170a92e248f1d2/l.eqt.1810_final_report_.pdf
Pannier, L., Gardner, G. E., O’Reilly, R. A., and Pethick, D. W. (2018). Factors affecting lamb eating quality and the potential for their integration into an MSA sheepmeat grading model. meat science, quality and integrity for global consumers. The 64th international congress of meat science and technology. Melbourne, Australia 144, 43–52. doi: 10.1016/j.meatsci.2018.06.035
Pannier, L., Pethick, D. W., Boyce, M. D., Ball, A. J., Jacob, R. H., and Gardner, G. E. (2014). Associations of genetic and non-genetic factors with concentrations of iron and zinc in the longissimus muscle of lamb. Meat Science, Australian Sheep CRC Meat: Meat Sci. Special Issue 96, 1111–1119. doi: 10.1016/j.meatsci.2013.08.013
Poore, J., and Nemecek, T. (2018). Reducing food’s environmental impacts through producers and consumers. Science 360, 987–992. doi: 10.1126/science.aaq0216
R Core Team , (2022). R: A language and environment for statistical computing. R Foundation for Statistical Computing, Vienna, Austria.
Ripoll-Bosch, R., De Boer, I. J. M., Bernués, A., and Vellinga, T. V. (2013). Accounting for multi-functionality of sheep farming in the carbon footprint of lamb: a comparison of three contrasting Mediterranean systems. Agric. Syst. 116, 60–68. doi: 10.1016/j.agsy.2012.11.002
Röös, E., Sundberg, C., Tidåker, P., Strid, I., and Hansson, P. A. (2013). Can carbon footprint serve as an indicator of the environmental impact of meat production? Ecol. Indic. 24, 573–581. doi: 10.1016/j.ecolind.2012.08.004
Scollan, N., Hocquette, J.-F., Nuernberg, K., Dannenberger, D., Richardson, I., and Moloney, A. (2006). Innovations in beef production systems that enhance the nutritional and health value of beef lipids and their relationship with meat quality. Meat Sci., 52nd international congress of meat science and technology (52nd ICoMST) 13-18 august 2006 Dublin. Theatr. Irel. 74, 17–33. doi: 10.1016/j.meatsci.2006.05.002
Scollan, N. D., Dannenberger, D., Nuernberg, K., Richardson, I., MacKintosh, S., Hocquette, J.-F., et al. (2014). Enhancing the nutritional and health value of beef lipids and their relationship with meat quality. Meat Sci. Advan. Beef Safety Throu. Res. Inno: Prosafebeef. 97, 384–394. doi: 10.1016/j.meatsci.2014.02.015
Scollan, N. D., Price, E. M., Morgan, S. A., Huws, S. A., and Shingfield, K. J. (2017). Can we improve the nutritional quality of meat? Proc. Nutr. Soc. 76, 603–618. doi: 10.1017/S0029665117001112
Silva, D.A.L. (2021). Life cycle assessment (LCA)—definition of goals and scope, in J.A. OliveiraDe, D.A. Lopes Silva, F.N. Puglieri, and Y.M.B. Saavedra (Eds.), Life cycle engineering and Management of Products: Theory and practice. Springer International Publishing, Cham, pp. 45–69.
Singh, S., Arora, R. R., Singh, M., and Khosla, S. (2016). Eicosapentaenoic acid versus docosahexaenoic acid as options for vascular risk prevention: a fish story. Am. J. Ther. 23, e905–e910. doi: 10.1097/MJT.0000000000000165
Sonesson, U., Davis, J., Flysjö, A., Gustavsson, J., and Witthöft, C. (2017). Protein quality as functional unit – a methodological framework for inclusion in life cycle assessment of food. J. Clean. Prod. 140, 470–478. doi: 10.1016/j.jclepro.2016.06.115
Stylianou, K. S., Heller, M. C., Fulgoni, V. L., Ernstoff, A. S., Keoleian, G. A., and Jolliet, O. (2016). A life cycle assessment framework combining nutritional and environmental health impacts of diet: a case study on milk. Int. J. Life Cycle Assess. 21, 734–746. doi: 10.1007/s11367-015-0961-0
Swanson, D., Block, R., and Mousa, S. A. (2012). Omega-3 fatty acids EPA and DHA: health benefits throughout life. Adv. Nutr. 3, 1–7. doi: 10.3945/an.111.000893
Sykes, A. J., Topp, C. F., Wilson, R. M., Reid, G., and Rees, R. M. (2017). A comparison of farm-level greenhouse gas calculators in their application on beef production systems. J. Clean. Prod. 164, 398–409. doi: 10.1016/j.jclepro.2017.06.197
Teixeira, R. F. M. (2015). Critical appraisal of life cycle impact assessment databases for Agri-food materials. J. Ind. Ecol. 19, 38–50. doi: 10.1111/jiec.12148
Tsvetkova, V., and Angelow, L. (2010). Influence of the season on the total lipids and fatty acid composition of grasses at the different altitudes in the region of the middle rhodopes. Bulgarian J. Agr. Sci. 16, 748–753.
Vellinga, T.V., Blonk, H., Marinussen, M., Zeist, W.J.van, and Starmans, D.J. (2013). Methodology used in FeedPrint: a tool quantifying greenhouse gas emissions of feed production and utilization (no. 674). Wageningen UR Livestock Res.
van Wyngaard, J. D. V., Meeske, R., and Erasmus, L. J., (2018). Effect of concentrate feeding level on methane emissions, production performance and rumen fermentation of Jersey cows grazing ryegrass pasture during spring. Animal Feed Sci. Techn. 241, 121–132. doi: 10.1016/j.anifeedsci.2018.04.025
Warren, H. E., Scollan, N. D., Nute, G. R., Hughes, S. I., Wood, J. D., and Richardson, R. I. (2008). Effects of breed and a concentrate or grass silage diet on beef quality in cattle of 3 ages. II: Meat stability and flavour. Meat Sci. 78, 270–278. doi: 10.1016/j.meatsci.2007.06.007
Whittington, F., Dunn, R., Nute, G., Richardson, R., and Wood, J. (2006). Effect of pasture type on lamb product quality. New Developments in Sheepmeat Quality 9th Annual Langford Food Industry Conference. 24–25, May 27–31.
Xu, Z., Xu, W., Peng, Z., Yang, Q., and Zhang, Z. (2018). Effects of different functional units on carbon footprint values of different carbohydrate-rich foods in China. J. Clean. Prod. 198, 907–916. doi: 10.1016/j.jclepro.2018.07.091
Yalcin, H., Öztürk, I., Tulukçu, E., and Sağdic, O. (2011). Influence of the harvesting year and fertilizer on the fatty acid composition and some physicochemical properties of linseed (Linum usitatissimum L.). J. Consum. Prot. Food Saf. 6, 197–202. doi: 10.1007/s00003-010-0631-x
Keywords: sheep systems, farm management, fatty acids, human nutrition, environmental impacts of meat, Omega-3, nutritional LCA, sustainable agriculture
Citation: McNicol LC, Perkins LS, Gibbons J, Scollan ND, Nugent AP, Thomas EM, Swancott EL, McRoberts C, White A, Chambers S, Farmer L and Williams AP (2024) The nutritional value of meat should be considered when comparing the carbon footprint of lambs produced on different finishing diets. Front. Sustain. Food Syst. 8:1321288. doi: 10.3389/fsufs.2024.1321288
Received: 13 October 2023; Accepted: 09 February 2024;
Published: 20 February 2024.
Edited by:
Graham A. McAuliffe, Rothamsted Research, United KingdomReviewed by:
Judith Capper, Harper Adams University, United KingdomCopyright © 2024 McNicol, Perkins, Gibbons, Scollan, Nugent, Thomas, Swancott, McRoberts, White, Chambers, Farmer and Williams. This is an open-access article distributed under the terms of the Creative Commons Attribution License (CC BY). The use, distribution or reproduction in other forums is permitted, provided the original author(s) and the copyright owner(s) are credited and that the original publication in this journal is cited, in accordance with accepted academic practice. No use, distribution or reproduction is permitted which does not comply with these terms.
*Correspondence: Louise C. McNicol, bHNtMjBmcWpAYmFuZ29yLmFjLnVr
†These authors have contributed equally to this work and share first authorship
Disclaimer: All claims expressed in this article are solely those of the authors and do not necessarily represent those of their affiliated organizations, or those of the publisher, the editors and the reviewers. Any product that may be evaluated in this article or claim that may be made by its manufacturer is not guaranteed or endorsed by the publisher.
Research integrity at Frontiers
Learn more about the work of our research integrity team to safeguard the quality of each article we publish.