- 1Programa de Doctorado en Ciencias Agrarias, Facultad de Ingeniería Agronómica, Universidad del Tolima, Ibagué, Tolima, Colombia
- 2Programa de Ingeniería Agroecológica, Facultad de Ingeniería, Universidad de la Amazonia, Florencia, Caquetá, Colombia
- 3Centro de Investigaciones Amazónicas CIMAZ Macagual Cesar Augusto Estrada González, Grupo de Investigaciones Agroecosistemas y Conservación en Bosques Amazónicos-GAIA, Florencia, Colombia
- 4Centro Agronómico Tropical de Investigación y Enseñanza (CATIE), Turrialba, Costa Rica
Properly designed agroforestry systems (AFS) can generate optimal cocoa bean (BC) yields, produce co-products and provide ecosystem services. This study analyzes the interactions between climate, AFS structure and BC yield in six climatic zones across three natural regions of Colombia. A total of 305 plots of 1,000 m2 each were established in 132 farms where the cocoa-AFS structure, BC yield and climatic variables were determined. Five typologies of cocoa-AFS were obtained based on the characteristics of the shade canopy and the abundance of cocoa trees: “Highly diversified multistratum with high biomass” (HDMHB), “Diversified multistratum with high shade and abundance of Musaceae (DMHSM),” “Diversified multistratum with high abundance of cocoa trees (DMHDC),” “Diversified monostratum with low shade (DMLS)” and “monostratum with minimal shade (MMS).” In the departments of Huila and Caquetá, Andean and Amazonia regions, respectively, the HDMHB typology predominated, while in Meta, the Orinoquia region, it was MMS. In the temperate-humid zone, the DMHDC and DMHSM typologies were not found. A high floristic diversity of the shade canopy was found: 229 species; Caquetá registered the highest number (152). The most frequent canopy companion species were Musa paradisiaca, Cariniana pyriformis, Cedrela odorata, Psidium guajava, Musa sapientum, and Cordia alliodora. The highest abundance of cocoa trees occurs in areas with lower temperature and relative humidity and in AFS with lower abundance of fruit and timber trees. Zones with higher temperature and lower precipitation had higher abundance of timber species (r = 0.23). The BC yield is higher in areas with higher precipitation and is related to the lower abundance of individuals of timber and fruit species, and to the higher abundance of Fabaceae. The BC yield depends on the typology (p < 0.0001) of the cacao systems and was higher in DMHDC (1,148 kg ha−1 yr.−1). These results are key for the design of cocoa-AFS farms that maximize the integral production of BC, co-products and ecosystem services, approaching sustainable cocoa farming.
1 Introduction
Agroforestry systems (AFS) are considered as a solution to climate and food security challenges (Reppin et al., 2019; Ballesteros et al., 2022; Koutouleas et al., 2022). The inclusion of several tree species increases biodiversity, productivity and the provision of ecosystem services (Vaast and Somarriba, 2014; Wartenberg et al., 2017; Notaro et al., 2021; Numbisi et al., 2021), improving the well-being of farmers (Vaast and Somarriba, 2014; Hernández-Núñez et al., 2021b; Scudder et al., 2022) by obtaining benefits that are not achieved with monoculture production systems (Maney et al., 2022). The AFS are the association of trees as a shade canopy and a crop adapted to grow under it (Notaro et al., 2021). Their design depends on ecological, productive and local knowledge factors, such as the predominant vegetation, land tenure and decision making (Numbisi et al., 2021). This is the case of cocoa (Theobroma cacao L.), which is planted under different levels of shade across approximately 70% of plantations worldwide (Vaast and Somarriba, 2014), where shade is generated by one or more companion species (Deheuvels et al., 2012; Hosseini et al., 2017; Jagoret et al., 2018; Notaro et al., 2021; Schmidt et al., 2022).
The cocoa tree, native to the Amazon region (Motamayor et al., 2002), is one of the most important agricultural crops in tropical regions (Hosseini et al., 2017; Gonas et al., 2022). This production system is the livelihood of approximately five million rural households (Scudder et al., 2022), 80% of which present vulnerable conditions (Vaast and Somarriba, 2014). Cocoa planted under AFS allows multiple benefits, such as: (a) conservation of biodiversity and generation of ecosystem services (Deheuvels et al., 2014; Vaast and Somarriba, 2014; Asigbaase et al., 2019; Maney et al., 2022), (b) adaptive capacity to climate variability and change (Andrade et al., 2013; Salvador et al., 2019; Notaro et al., 2021; Zequeira-Larios et al., 2021; Hernández-Núñez et al., 2021a), (c) contribution to self-consumption, food security and food and nutritional sovereignty (Vaast and Somarriba, 2014; Hosseini et al., 2017; Saj et al., 2017; Asigbaase et al., 2019; Notaro et al., 2021; Gonas et al., 2022), (d) decrease in the economic vulnerability of households (Cerda et al., 2014; Vaast and Somarriba, 2014; Hosseini et al., 2017; Hernández-Nuñez et al., 2020; Notaro et al., 2021; Somarriba et al., 2021; Zequeira-Larios et al., 2021), and (e) synergy that makes the production system sustainable (Vaast and Somarriba, 2014; Hosseini et al., 2017; Wartenberg et al., 2017; Asigbaase et al., 2019; Notaro et al., 2021; Zequeira-Larios et al., 2021; Maney et al., 2022).
Despite the importance of cocoa AFS, their complexity can present difficulties at the production (such as increased incidence of pests and diseases) and technological level (such as pruning and fertilization management) (Correa et al., 2014; Vaast and Somarriba, 2014; Espinosa, 2016), which generate negative impacts on production (Correa et al., 2014; Sterling et al., 2015). These conditions threaten the sustainability of the crop, which has a direct impact on the well-being of rural households (Scudder et al., 2022). Additionally, the improvement of cocoa AFS, setting bean yield as the only criterion, invisibilize the multiple objectives that can be achieved (Vaast and Somarriba, 2014; Hernández-Nuñez et al., 2020), as the other products are as important as cocoa in contributing to household livelihoods (Vaast and Somarriba, 2014; Hosseini et al., 2017).
The design of the AFS must contemplate the physiological needs of the crop (Fedecacao, 2015; Hosseini et al., 2017; Suárez et al., 2018), the challenges and objectives of the rural household (Numbisi et al., 2021; Zequeira-Larios et al., 2021; Rodríguez et al., 2022) and the local biophysical and socioeconomic conditions (Reppin et al., 2019). The interactions of the above factors determine whether there are synergistic or competitive effects in the production system (Notaro et al., 2021). An adequate AFS design can achieve optimal cocoa bean yields, generate co-products and enhance the provision of ecosystem services, aspects that improve household well-being (Hosseini et al., 2017; Asigbaase et al., 2019; Hernández-Nuñez et al., 2020; Numbisi et al., 2021; Zequeira-Larios et al., 2021). Moreover, AFS are not static but change over farm age and production cycles (Numbisi et al., 2021).
This study analyzes the interactions between climatic conditions, shade canopy structure, floristic composition, pest and disease incidence and yield in cocoa production systems in Colombia. The guiding research questions were: How are plant species compositions in cocoa AFS in Colombia? What is the relationship between climatic conditions and shade canopy typologies in cocoa AFS? and How do AFS typologies affect pest and disease incidence and cocoa bean yield? The answers to the questions posed will provide academic inputs for the integrated management of cocoa production systems. Our results aim to find cocoa AFS based on the interaction and balance between a high production of dry cocoa beans and a high generation of companion species that contribute to security, diversify diets and have a greater capacity to adapt to climate change.
2 Materials and methods
2.1 Study area and selected population
The study was conducted in Colombia, in the departments of Huila, Meta and Caquetá, which are in the Andean, Orinoco and Amazon regions, respectively. These departments were taken as representative of the natural regions of greatest importance for cocoa cultivation. Sixteen municipalities in the three departments were selected based on their agro-climatic conditions and the participation of cocoa cultivation in the productive dynamics of the region, considering: (a) area planted, (b) bean yield, (c) marketing or processing, and (d) presence of cocoa organizations.
A total of 305 sample plots were selected in 132 cocoa producing farms (22, 51 and 59 in Meta, Huila and Caquetá, respectively) registered in the Cooperativa Agroindustrial de Cacaoteros del Meta -CACAOMET-, the Red de Asociaciones de Productores de Cacao del Huila -APROCAHUILA- and the Asociación Departamental de Cultivadores de Cacao y Especies Maderables del Caquetá -ACAMAFRUT-. The producers were randomly selected, proportionally to the total number in each department. Visits were made to the producers’ farms to identify cocoa plots under AFS, in which an area 1,000 m2 (50 × 20 m) was established in each plot, corresponding to 83, 100, and 122 in Meta, Huila and Caquetá, respectively (Jagoret et al., 2017; Suárez et al., 2018; Hernández-Nuñez et al., 2020). According to the Caldas Lang climate zone classification, 157 of these plots were located in the Humid Warm zone, 22 in Semi-Arid Warm, 31 in Semi-Humid Warm zone, 11 in Humid Temperate zone, 51 in Semi-Arid Temperate and 33 in Semi-Humid Temperate zone (supplementary) (Ideam, 2017).
2.2 Tree structure and floristic composition of cocoa production systems
In each plot, the position of each individual was projected on a Cartesian plane with respect to the south-west corner of the plot (Suárez et al., 2018; Hernández-Nuñez et al., 2020) and different dasometric measurements were taken: (a) trunk diameter at breast height (dbh); (b) mean crown diameter and; (c) height – total and at the base of the crown (Arango-Ulloa et al., 2009; Ngo Bieng et al., 2013). Only companion trees with dbh ≥ 2.5 cm were measured. In the case of cocoa and coffee trees, trunk diameter was measured at 50 and 15 cm height, respectively (Hernández-Núñez et al., 2021a). Botanical samples of the companion trees were taken for identification to species level at the Laboratorio de Malherbología y Agrobiodiversidad de la Universidad de la Amazonia. The companion species were classified according to their use as food, palms, legumes, timber, Musaceae and others. From this information, 74 variables were estimated, grouped into seven components: (1) shade; (2) height; and (3) the selection of variables was based on work by Ngo Bieng et al. (2013), Jagoret et al. (2017), Suárez et al. (2018), and Hernández-Nuñez et al. (2020).
2.3 Climatic conditions
The geographic position of the center of each sample plot was determined using a Global Positioning System with an accuracy of 3 m. For each plot, annual climatic information was obtained for a 20-year period (2000–2020) for the following variables: (a) mean temperature (°C), (b) relative humidity (%), and (c) precipitation (mm). This information was downloaded from “The POWER Project,” which provides solar and meteorological data sets from the National Aeronautics and Space Administration-NASA research (Sparks, 2018). The download was performed using the “nasapower” library in the R Core Team statistical software (R Core Team, 2021; Sparks, 2021).
2.4 Incidence and severity of pests and diseases in cocoa production systems
In each plot, the infestation and severity of damage caused by the insect Monalonium dissimulatum and the fungi Moniliophthora roreri and Phytophthora spp. were measured. The degree of external severity of M. roreri damage was estimated on 50 pods per plot, using the scale used by Sterling et al. (2015): 0, healthy pod; 1, oily spots; 2, swelling and/or premature ripening; 3, spot (necrosis); 4, mycelium up to 25% of the necrotic spot; and 5, mycelium covering more than 25% of the necrotic spot. A longitudinal cut was made on 50 pods to determine internal severity. The percentage of internal necrosis caused by M. roreri was measured and ranked from 0 to 5: 0, no necrosis; 1, 1–20%; 2, 21–40%; 3, 41–60%; 4, 61–80%; and 5, more than 80% of with necrosis (Sterling et al., 2015). The incidence of Phytophthora spp. and M. dissimulatum was determined by counting all the pods of the trees in the plot and identifying those affected (Vargas et al., 2005; Ramírez, 2016). The percentage of incidence was estimated as the ratio between number of affected pobs and numbers of evaluated cobs ×100.
The severity of Phytophthora spp. damage was estimated on 50 pods. This was categorized on a grade from 1 to 5: 1, symptom-free; 2, less than 2 mm affected; 3, affected between 2 mm and 2 cm; 4, affected up to 25%; and 5, spots on more than 25% of the pod (Ramírez, 2016). The severity of M. dissimulatum was estimated by counting the number of bites on all pods of all cocoa trees per plot. The severity of this affectation was ranked from 0 to 4, as follows: (0) zero stings; (1) 1–10 stings; (2) 11–25 stings; (3) 26–50 stings; (4) more than 50 stings (Vargas et al., 2005).
2.5 Cocoa bean yields
In each plot, 50 mature cobs of the main genotype of the lot were collected and the fresh kernels of each were weighed. Subsequently, grain yield during the peak production period of each department was estimated using the following formula proposed by Jagoret et al. (2017):
donde;
R: yield (kg ha−1 year−1).
NbPods: average number of pods/cacao tree.
Wbeans: average weight of fresh beans (kg pod−1).
TC: fresh grain to dry grain conversion ratio.
KkoDens: abundance of cocoa trees (individuals ha−1).
2.6 Data analysis
2.6.1 Typification of tree structure and floristic composition
The types of cocoa agroforestry systems were identified using the variables of the seven components of tree structure and floristic composition and the abundance of cocoa trees. The variables that make up each of the seven components of the cocoa-AFS were transformed to a scale between 0 and 1; based on this, seven indices were generated because of the sum of these transformed variables per component (Supplementary Table S1). With the seven indices and the variable “abundance of cocoa trees,” a classification of the plots was generated using a hierarchical cluster analysis, with Ward’s method and Euclidean distance (Balzarini et al., 2008).
The influence of the 74 variables of the seven components and the abundance of cocoa trees on the separation of the groups (Cocoa types-AFS) was estimated by an analysis of variance. The continuous variables were analyzed using linear mixed models (MLM), with the typologies as a fixed effect, based on cluster analysis, and the natural regions as a random effect. The model assumptions were evaluated by graphical inspection of the residuals. When heterogeneous variances between typologies were detected, the variance–covariance matrix was modeled (Di Rienzo et al., 2011). Discrete variables were analyzed using generalized linear mixed models (GLMM) with Poisson distribution (Di Rienzo et al., 2017). Fisher’s LSD test (p < 0.05) was used for mean comparisons. The association of clusters with departments and with climatic zones was performed by contingency table analysis. Relationships between cocoa tree and companion species variables were estimated with Spearman correlation analysis. Multiple relationships between the dasometric measures of companion species and cocoa trees were performed through a Principal Component Analysis (PCA) (Balzarini et al., 2008). The analyses were performed using the statistical software InfoStat (Di Rienzo et al., 2019) and R Core Team (R Core Team, 2021).
2.6.2 Climatic conditions, pests and diseases and dry cocoa bean production in cocoa agroforestry system types
Spearman correlation analysis was performed between climatic variables and component variables describing tree structure and floristic composition (supplementary). A tri-plot using Partial Least Squares Regression (PLS) was performed to order the plots according to the climatic variables (predictors) and the abundance of cocoa trees and accompanying individuals (dependent), identifying the types of cocoa agroforestry systems (Balzarini et al., 2008).
Additionally, an analysis of variance was performed to determine the influence of cocoa AFS types on pest and disease incidence and severity status (using MLGM) and cocoa bean yield (using MLM) with fixed effect of typology and random effect of department (Di Rienzo et al., 2011, 2017). Finally, a linear regression analysis was performed to determine the relationship between cocoa bean yield (response variable) and tree structure, shade and climate variables (independent or predictor variables) (Balzarini et al., 2008).
3 Results
3.1 Types of cocoa production systems in Colombia
The 305 plots were grouped into five types of cocoa agroforestry systems with significant difference (p < 0.0001) among them (Supplementary Table S1): Highly diversified multistratum with high biomass (HDMHB), Diversified multistratum with high shade and abundance of Musaceae (DMHSM), Diversified multistratum with high abundance of cocoa trees (DMHDC), Diversified monostratum with low shade (DMLS) and monostratum with minimum shade (MMS).
Highly diversified multistratum with high biomass (HDMHB) (31.2%, n = 95). This typology presented a high diversity of companion species (richness = 6.52 species, Shannon Weaver index = 1.43) and the highest diversity in potential uses (richness of 6.45 uses, Shannon Weaver index = 1.6) (Supplementary Table S1). This typology presented on average 788 individuals ha−1 of cocoa trees and 211 individuals ha−1 of companion species, which are distributed in the low, medium and high strata (64, 34 and 2%, respectively). The 32 and 29% of the companion species correspond to timber species and Musaceae, with 68 and 61 individuals ha−1, respectively. The accompanying individuals presented the largest basal area and dbh in the three strata and in all use categories with a dbh of 36.2 cm. This typology presented a high shade, generated by individuals of companion species (30.5%) (Supplementary Table S1).
Diversified multistratum with high shade and abundant Musaceae (DMHSM) (10.8%, n = 33). This typology presented high values of companion species diversity (richness = 6.73 species, Shannon Weaver index = 1.14) and diversity in potential uses (richness = 6 uses, Shannon Weaver index = 1.48 uses). This cacao AFS typology was characterized by having the lowest abundance of cocoa trees (574 individuals ha−1) and the highest abundance of companion species (481 individuals ha−1), most of them in the middle and low strata. These cocoa plantations had a high abundance of timber and moss species (169 individuals ha−1 and 140 individuals ha−1, respectively). Despite the high abundance of companion species, the individuals had small diameters (dbh = 12.3 cm). The shade accompanying cocoa in the plots of this typology was 36.8% (Supplementary Table S1).
Diversified multistratum with high abundance of cocoa trees (DMHDC) (15.7%, n = 48). This typology presented average values of companion species diversity (richness = 5 species, Shannon Weaver index = 1.2) and a high diversity of potential uses (richness = 6.4 uses, Shannon Weaver index = 1.6). This typology reached the highest abundance of cocoa trees (1,259 individuals ha−1) and 196 individuals ha−1 of companion trees. Of the companion trees, 68 and 32% are of low and medium stratum, respectively. A total of 86% of the companion individuals correspond to: Musaceae (42%) with 82 individuals ha−1, timber (25%) with 50 individuals ha−1 and food species (19%) with 36 individuals ha−1. It had the largest basal area (13.83 m2 ha−1). The accompanying shade to cocoa in the plots of this typology was 25%.
Diversified monostratum with Low shade (DMLS) (23.3%, n = 71). This typology was characterized by an average abundance of 726 individuals ha−1 of cocoa trees, and a low abundance of companion individuals (143 individuals ha−1), with no trees in the upper stratum. The highest percentage of accompanying individuals corresponded to Musaceae (34%), with 48 individuals ha−1. This typology presented a low percentage of shade from companion species (16.9%) (Supplementary Table S1).
Monostratum with minimum shade (MMS) (19.0%, n = 58). This typology presented the lowest diversity of companion species (richness = 2.3 species, Shannon Weaver index = 0.57), and potential uses (richness of 4.7 uses, Shannon Weaver index = 1.23 uses). It had an average abundance of 871 individuals ha−1 of cocoa trees and was characterized by having the lowest abundance of companion species (90 individuals ha−1) and no individuals in the upper stratum. It also had the lowest shade canopy (5.5%) (Supplementary Table S1).
Using the tree structure and floristic composition indices, the principal component analysis (PCA) explained 63% of the variance of the data with the first two axes. The first component allows sorting the PCAs in order of complexity, with HDMHB being the most complex, followed by DMHSM and DMHDC, then DMLS and finally the simplest, MMS. The abundance of cocoa trees only allows separating DMHSM from the rest. The variables with the strongest contribution to the separation in CP1 are the shade index and the height index. In the case of the indices that have more strength in CP2, the variables with the greatest contribution were the abundance of companion species (associated with DMHSM) and abundance of cocoa, associated with DMLS, DMHDC, HDMHB and MMS (Figure 1A). Significant negative correlations (p < 0.05) were found for the abundance, basal area and shade of cocoa trees with the abundance, basal area and height of companion individuals (Figure 1B).
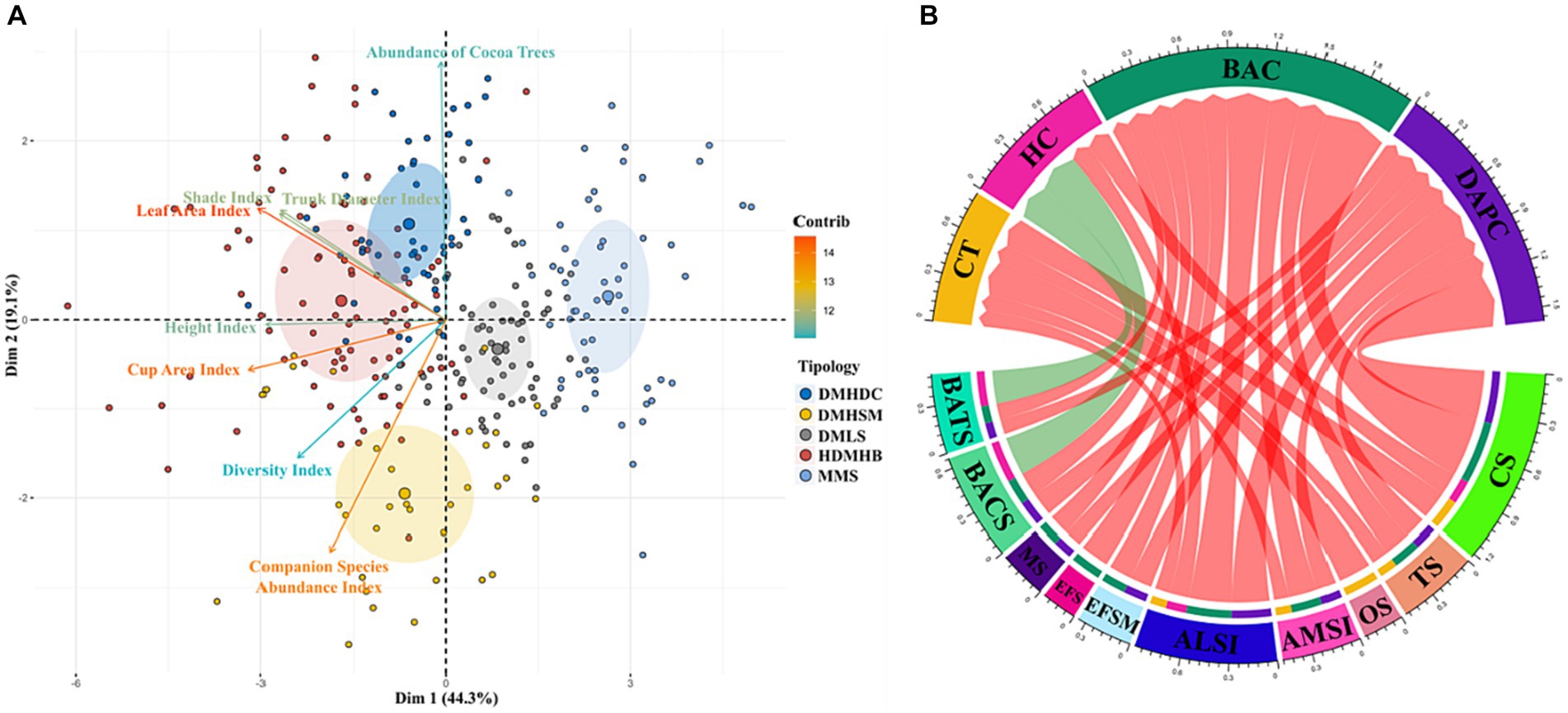
Figure 1. Variables of tree structure and floristic composition of cocoa production systems in the departments of Caquetá, Huila and Meta, Colombia. (A) Bi-plot constructed by principal component analysis using the component indices of tree structure and floristic composition of cocoa AFS and identifying the AFS types. (B) Significant Spearman correlations (p < 0.05) between variables of tree structure and floristic composition of cocoa companion species and cocoa trees. Green color: positive correlations; red color: negative correlations; width: strength of correlation. CS, Companion Species; TS, Timber Species; OS, Other Companion Species; AMSI, High stratum individuals; ALSI, Low stratum individuals; BALSS, Basal area of low stratum individuals; EFS, food species; MS, musaceous species; BACS, basal area of companion species; BATS, basal area timber individuals; BAC, basal area cocoa trees; HC, height cocoa trees; Cos, percentage shade cocoa trees; CT, number of cocoa trees; AS, palms; FSE, leguminous plants.
3.2 Spatial distribution of cocoa production system typologies
The distribution of cocoa production system typologies was heterogeneous among natural regions and climatic zones. In Huila, 86% of the systems corresponded to the typologies: HDMHB (42%), DMHDC (31%), DMLS (13%); while MMS and DMHSM only represented 9 and 5% of the total plots, respectively. In Meta, 75% of the plots corresponded to: MMS (42%) and DMLS (34%), while no HDMHB typology were found (Figure 2). In Caquetá, 68% of the plots were of the HDMHB (43%) and DMLS (25%) typologies; only 3% of the plots corresponded to DMHDC (Figure 2).
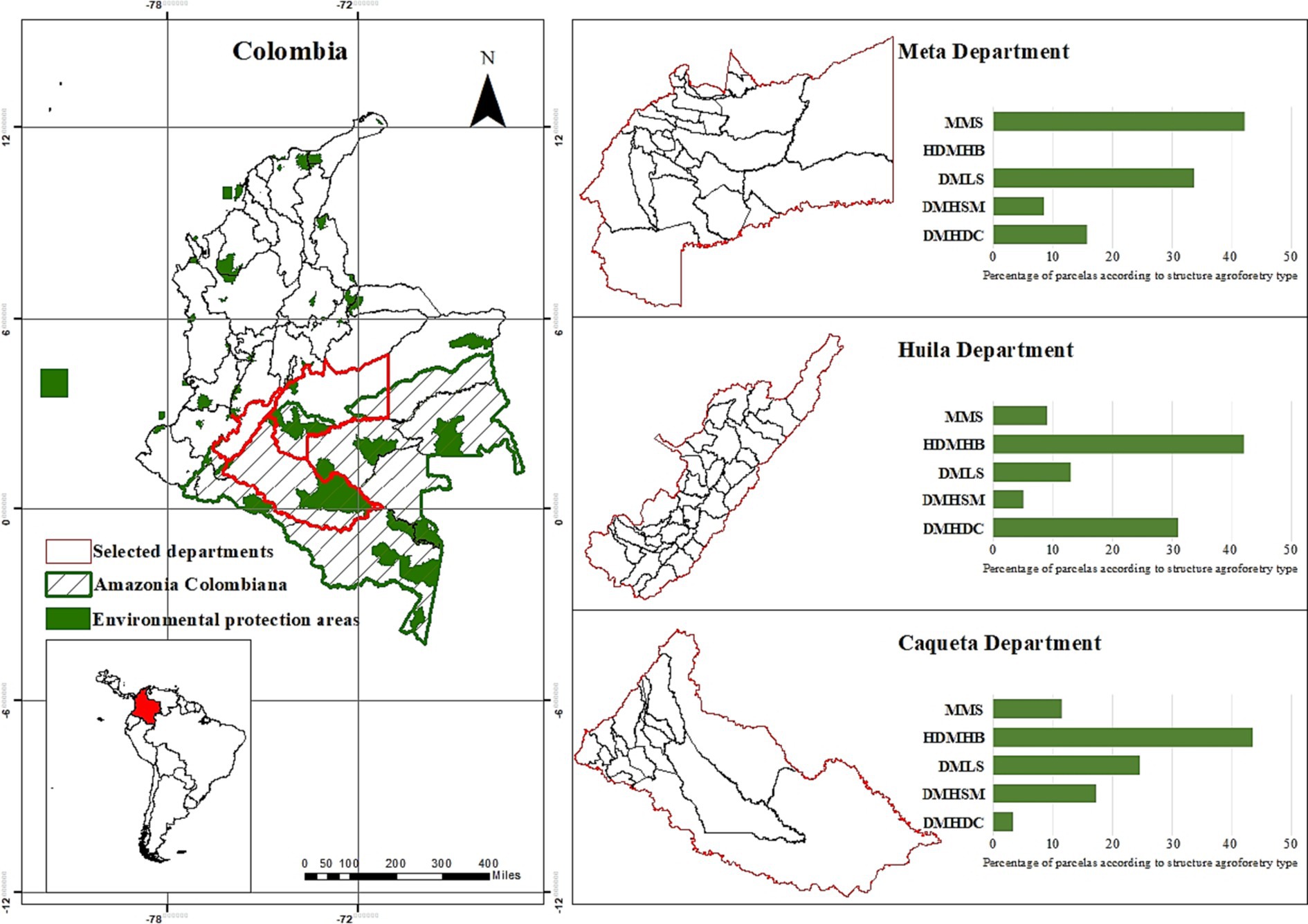
Figure 2. Spatial distribution of cocoa crop plots by typology in the departments of Caquetá, Huila and Meta, Colombia. Highly diversified multistratum with high biomass (HDMHB), Diversified multistratum with high shade and abundance of musaceae (DMHSM), Diversified multistratum with high abundance of cocoa trees (DMHDC), Diversified monostratum with low shade (DMLS), and Monostratum with minimum shade (MMS).
In the Humid Temperate zone, no plots of the DMHDC and DMHSM typologies were found; in this same zone, the largest number of plots corresponded to DMLS (45%) and MMS (36%). The HDMHB typology was uniformly presented in the different climatic zones. In the Humid Warm zone, the DMLS typology predominated, followed by HDMHB and MMS with 29, 23 and 22% of plots, respectively. In the Warm Semiarid and Warm Semi-humid zones, most of the plots were of the HDMHB typology with 41 and 55%, respectively. In the Temperate Semiarid zone, the DMHDC and HDMHB typologies were the most abundant (35% each); while the DMHSM and MMS typologies were the least present (4 and 6%, respectively). Finally, in the Semi-humid Temperate zone, the largest number of plots corresponded to HDMHB and MMS with 39 and 30%; while the least common were DMHSM and DMHDC with 3% of plots in each case.
3.3 Floristic diversity of cocoa production systems
A total of 229 species, corresponding to 54 taxonomic families (Figure 3A), were found in 30.5 ha of cocoa plantations in the three departments. Caquetá recorded the highest number of species (152, 66.3% of the total of the study) grouped in 112 genera and 49 families, with dominance of the species Musa paradisiaca L., Cariniana pyriformis Miers., Cedrela odorata L., Psidium guajava L., Musa sapientum L., and Cordia alliodora (Ruíz & Pav.) Oken. In Huila and Meta, a similar number of species (64 and 67), genera (57–52) and taxonomic families (28–25) were found, respectively; with the highest frequency of Musa paradisiaca L., Persea americana Mill. and Gliricidia sepium (Jacq.) Kunth ex Walp. found, while Musa sapientum L. was found only in Huila (Figures 3A,B).
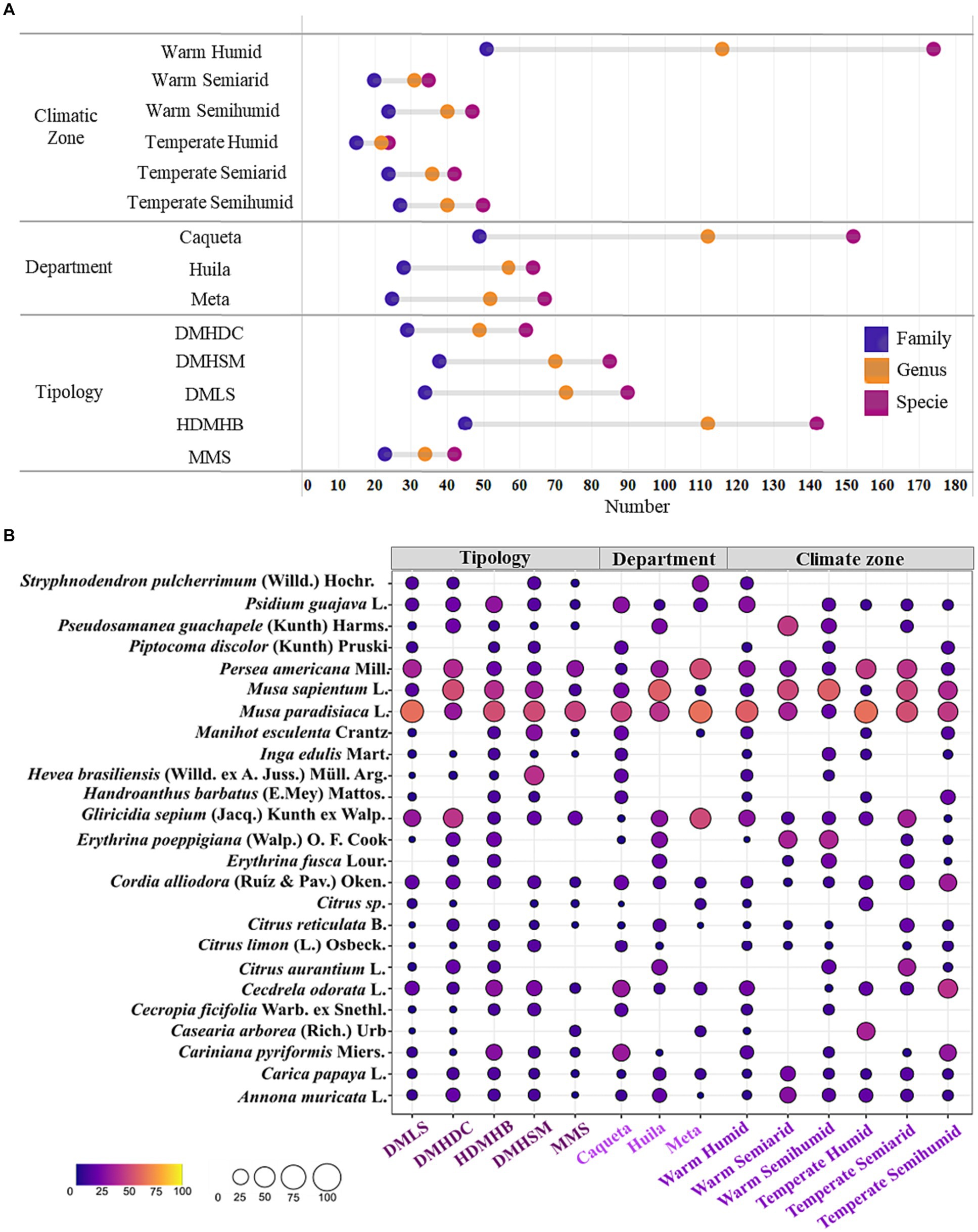
Figure 3. Floristic diversity of cocoa AFS in the typologies, departments and climatic zones in the departments of Caquetá, Huila and Meta, Colombia. (A) Taxonomic richness at family, genus and species level; purple, orange and lilac dots denote the number of families, genera and species, respectively. (B) Frequency expressed as percentage of species presence. The colors and sizes of the circles refer to the frequency (0–100%).
Musa paradisiaca L. was the most frequent species in all typologies (48–63% of the plots) (Figure 3B). However, the abundance of Musaceae presented significant differences between typologies (p < 0.0001) (Supplementary Table S1), with the highest abundance of Musa paradisiaca L. and Musa sapientum L. (174 and 142 individuals ha−1, respectively) in DMHSM; in addition, this typology showed a high abundance of Hevea brasiliensis (Willd. ex A. Juss.) Müll. Arg. and Manihot esculenta Crantz, which are present in 42 and 27% of the plots, respectively (Figures 3A,B). The HDMHB cocoa plantations had the highest number of species (142), with high frequency of Psidium guajava L., Cedrela odorata L., Cariniana pyriformis Miers, Erythrina poeppigiana (Walp.) O. F. Cook and Persea americana Mill (Figures 3A,B). The MMS typology presented the lowest richness: 23 families, 34 genera and 42 species, where Musa paradisiaca L. and Persea americana Mill. were in 48 and 29% of the plots with an abundance of 49 and 68 individuals ha−1, respectively. The highest species richness was reported in humid warm climate zones, with Musa paradisiaca L., Persea americana Mill., Gliricidia sepium (Jacq.) Kunth ex Walp. and Psidium guajava L. and Cedrela odorata L. being the most frequent (Figures 3A,B). In general, the cocoa plantations hosted a high diversity of companion species, with a maximum of 20 species per plot, with an average of five species.
3.4 Relationships between climatic conditions, tree structure and floristic composition
Significant correlations (p < 0.05) were found between the variables describing tree structure and climatic conditions (Figure 4B). The highest abundance of cocoa trees occurred in areas where temperature and relative humidity were lower (r = −0.34 and − 0.18, respectively) (Figure 4B). The correlations between abundance and climatic conditions were different according to the categories of uses of the shade canopy species. For example, in areas of high temperature and low precipitation, a higher abundance of timber species was found (r = 0.23) (Figures 4A,B). The abundance of Musaceae was higher in areas with lower relative humidity (Figures 4A,B).
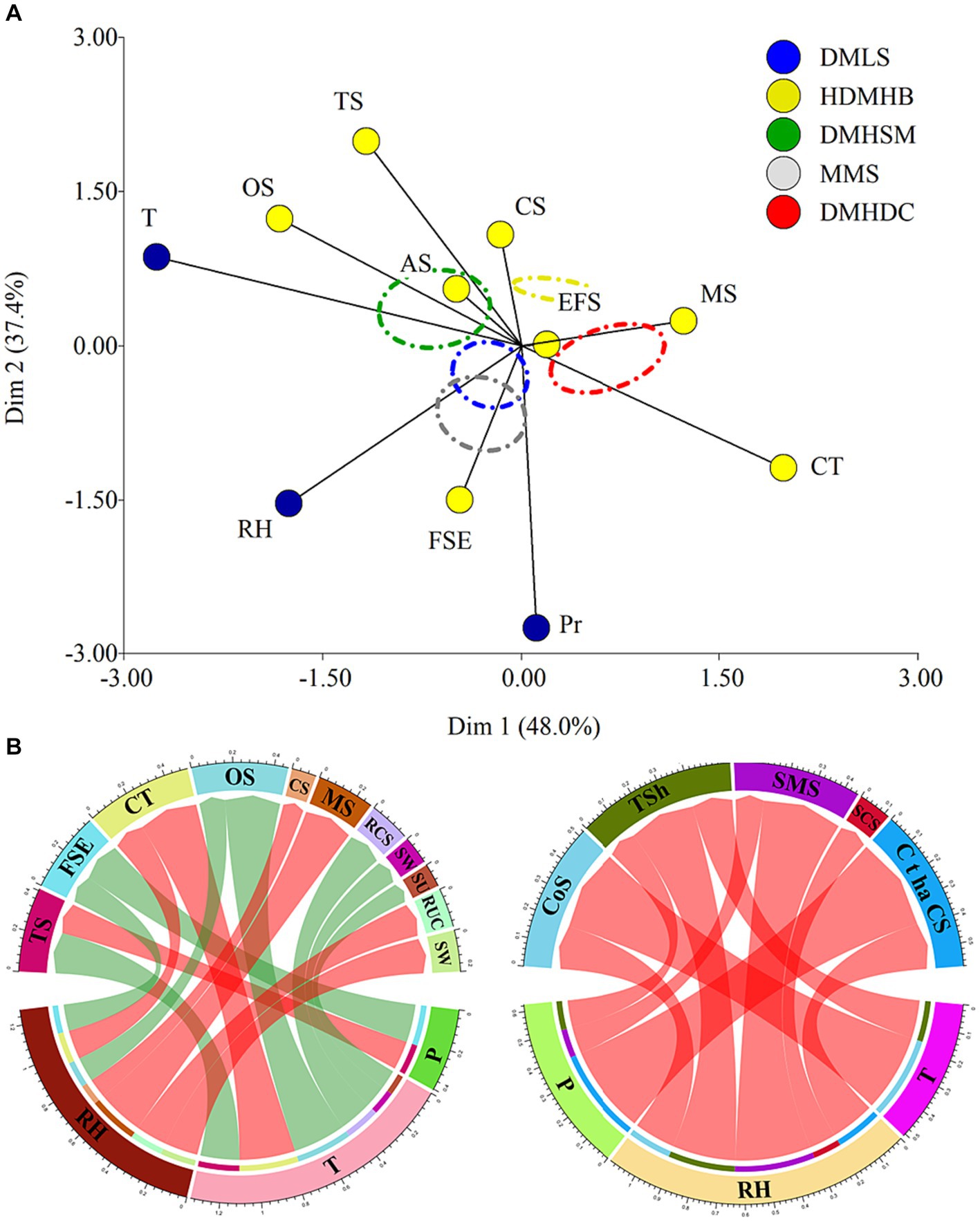
Figure 4. Relationships between climatic conditions and tree structure and floristic composition variables in cocoa crops in the departments of Caquetá, Huila and Meta, Colombia. (A) Tri-plot constructed by PLS using climatic variables as predictors (blue dots), abundance of cocoa trees and accompanying individuals as dependent (yellow dots) and identifying cocoa production system typologies. (B) Significant Pearson correlations (p < 0.05) between tree structure variables and floristic composition of cocoa production systems and climatic variables; green color represents positive correlations; red color represents negative correlations; width represents the strength of the correlation. CS, companion species; TS, timber species; OS, other companion species; EFS, food species; MS, Musaceae species; CT, number of cocoa trees; AS, palms; FSE, leguminous plants; T, mean annual temperature; RH, annual relative humidity; P, annual precipitation.
The shade accompanying the cocoa tree presented negative correlations (p < 0.05) with precipitation and relative humidity variables (r = −0.12 and −0.18, respectively). Richness and Shannon Weaver index presented positive correlations with temperature (r = 0.20 and 0.14, respectively). On the contrary, Shannon Weaver index and diversity of potential uses were significantly (p < 0.05) and negatively correlated with relative humidity (r = −0.20 and −0.19, respectively) (Figure 4B).
3.5 Relationship between pest and disease attack, bean production, cocoa farm type and climatic conditions
Cocoa bean yield showed statistical differences (p < 0.0001) between typologies, where DMHDC had the highest value (1,148 kg ha−1 yr.−1). DMHSM had the lowest yield (655 kg ha−1 yr.−1), 42.9% lower than DMHDC. The MMS, HDMHB and DMLS typologies reached intermediate yields (855, 806 and 767 kg ha−1 yr.−1, respectively) (supplementary). Grain index, pod index, wet to dry grain transformation coefficient and grain weight did not show significant differences (p > 0.05) between typologies.
Cocoa bean yield was affected by different conditions of shade canopy, climate and phytosanitary status. In areas with higher rainfall, cocoa bean yield was higher; the opposite behavior occurred in areas with high incidence of Monilia, and high values of timber trees and Musaceae (p < 0.05) (Table 1). Bean yield was negatively affected (p < 0.05) by the incidence of Monalonion and Phytophthora (r = −0.23 and r = −0.18, respectively). Similarly, internal and external severity of Monilia reduced yield (r = −0.19 and r = −0.16, respectively). The abundance of timber, fruit, Musaceae and other species was negatively related (p < 0.05) with cocoa yield, while the abundance of Fabaceae was positively correlated (p < 0.05) with this variable.
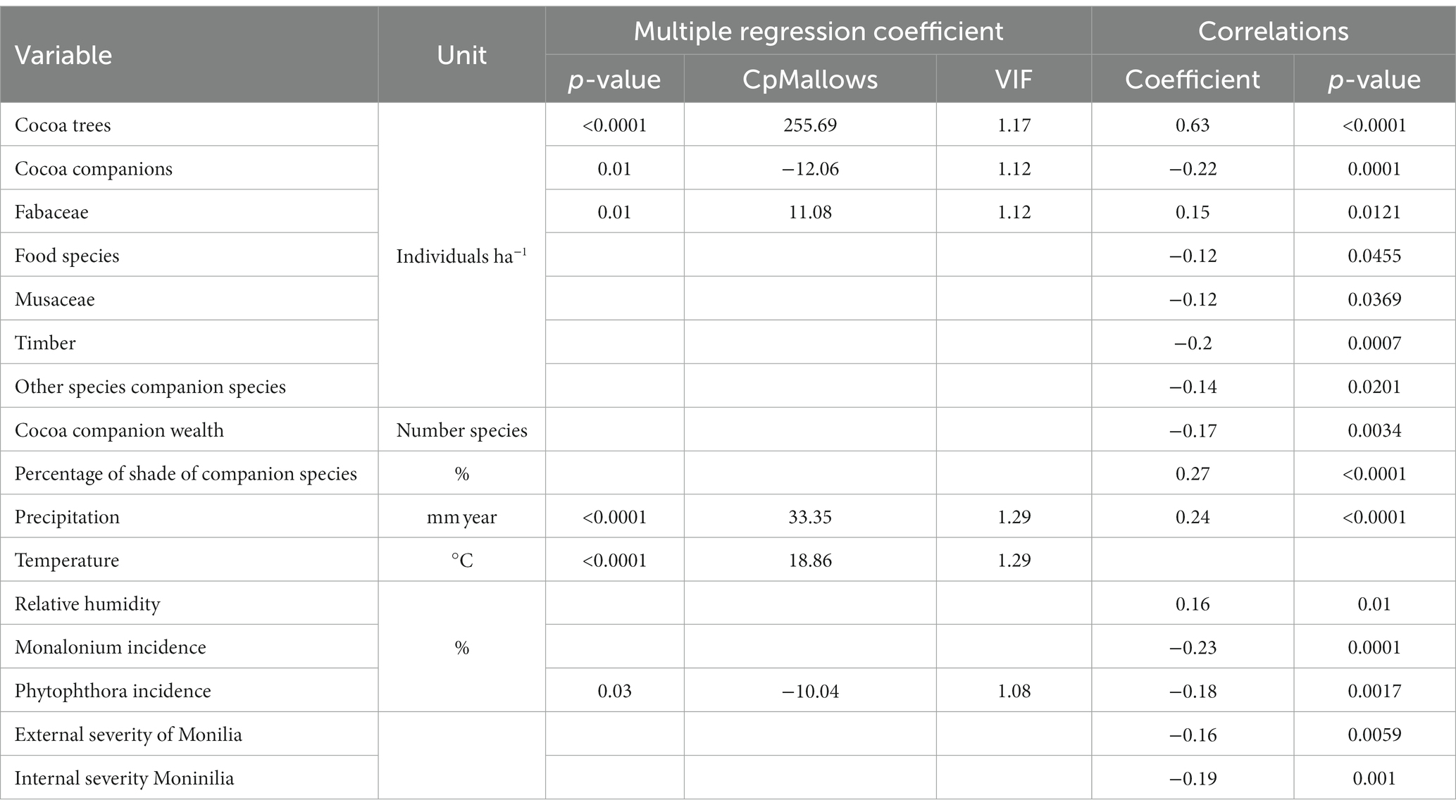
Table 1. Multiple regression coefficient and univariate correlations between the different variables evaluated and the response variable (dry cocoa bean yield per hectare) in cocoa crops in the departments of Caquetá, Huila and Meta, Colombia.
4 Discussion
Cocoa production in Colombia is carried out under different AFS, without an exclusive design by zones. In this regard, Ndo et al. (2023) indicate that the specific diversity leads to a diversity of spatial structures that can be adopted by communities, as a number of species vary greatly from one farm to another. This defines the different production and sustainability approaches that cocoa crops can have, which although, in a general way can be defined as cocoa planted in monoculture or in AFS (Jaimes et al., 2022), the latter has different variations in its design (Suárez et al., 2018; Notaro et al., 2020); where, the number and type of plants are important factors in defining the spatial structure adopted by farmers (Ndo et al., 2023).
In the three areas studied, five types of cocoa plantations were found with differences in their structure and floristic composition (i.e., number and type of species, percentage of shade): Highly diversified multistratum with high biomass (HDMHB), diversified multistratum with high shade and abundance of Musaceae (DMHSM), diversified multistratum with high abundance of cocoa trees (DMHDC), diversified monostratum with low shade (DMLS) and monostratum with minimum shade (MMS). This has a contradiction with different technical approaches and research, where it is indicated that an adequate plantation should have a certain level of shade and number of species (Fedecacao, 2015). For example, the DMHDC and DMHSM typologies presented, respectively, a higher and lower abundance of cocoa trees than recommended by the technical guidelines of Fedecacao (2015): 600–700 trees ha−1. The first of these typologies was above the average reported in Central America and the second is similar to the average of plantations in Africa (Cerda et al., 2014). Ballesteros et al. (2022) considered the low density of trees (400 individuals ha−1) as one of the disadvantages of the competitiveness of cocoa farms. The abundance of companion species was not uniform between zones either, as high abundance was found in the DMHSM typology with 481 individuals ha−1 and low abundance in MMS with 90 individuals ha−1. Research has reported in Ivory Coast, Indonesia, Cameroon, Central American countries and the Colombian Amazon approximately 237, 205, 196, 200, and 127 accompanying individuals ha−1, respectively (Cerda et al., 2014; Suárez et al., 2018). In Africa, they reported 30 accompanying individuals ha−1, corresponding 45 different families, 129 genera and 213 species (Sanial et al., 2023). This is consistent with other research, which has found that the spatial configuration of cocoa trees often deviates from agronomic recommendations (Numbisi et al., 2021).
The dichotomy between the designs of cocoa-based AFS found and the technical recommendations may occur because, as indicated by Lavoie et al. (2023), the design of the AFS does not obey a single factor but is given by the convergence of local conditions (social and environmental), external conditions (projects) and the farmer’s own capabilities and projections. For example, farmers are more aware of the multiple uses of cocoa companion species (Zequeira-Larios et al., 2021), which can lead to a higher abundance of these species, as occurs in the DMHSM typology. This reaffirms the potential of cocoa-AFS for multiple production objectives (Vaast and Somarriba, 2014). This behavior goes against the institutional trend of increasing the abundance of cocoa planting, but is in line with the vision of traditional farmers because the low density of cocoa trees allows them to plant diverse crops for their livelihood (Ballesteros et al., 2022). In addition, with appropriate management practices, a balance between bean yield and co-product generation can be achieved (Hernández-Nuñez et al., 2020). For example, the HDMHB typology presented a cocoa bean yield of 855 kg ha−1 year−1, which is higher than that reported by different studies (Escobar Ramírez et al., 2021; Gama-Rodrigues et al., 2021; Asitoakor et al., 2022b) and presented an average abundance of 211 accompanying individuals ha−1, mainly of Musaceae, timber species and food generators, which increase the provision of ecosystem services. This is related to what has been suggested by different authors who indicate that the cocoa-AFS can be a socioeconomically and ecologically viable system (Notaro et al., 2021; Gonas et al., 2022), which emphasizes the need to eliminate the bias of studying AFS-cocoa focused on cocoa bean yields without evaluating the complementary economic benefits (Ballesteros et al., 2022).
One factor that agreed with the ranges reported by different authors and technical guides was the percentage of shade, since no typology exceeded 36%. This is in agreement with different authors, who indicate that this should be less than 50% (Zequeira-Larios et al., 2021), where the most recommended is 30%, since a higher shade causes limitations in cocoa bean yield (Ballesteros et al., 2022). However, in departments such as Caquetá, which have fewer daylight hours (3.8 daylight hours day−1), a lower percentage of shade is necessary as reported by Suárez et al. (2018). In this department, there was a higher frequency of the HDMHB typology, which has a high percentage of shade. This can cause a higher incidence of pests and diseases and limit cocoa productivity (Jaimes et al., 2011; Vaast and Somarriba, 2014; Ortíz et al., 2015; Sterling et al., 2015), which was consistent with the present results, where the higher the percentage of shade, the greater the severity of Monilia.
Research has found different reasons that define the number of companion species to cocoa and the type of species (Salazar-Díaz and Tixier, 2017; Guelly et al., 2021) and is that the diversity of crop species generates a diversity of phenological, morphological and physiological characteristics, whose combination influences the agroecological functioning of FFS through facilitation or competition effects (Notaro et al., 2022). One factor of importance when establishing companion species is to regulate shade for cocoa, as it directly influences the agronomic yield of cocoa beans (Ngo Bieng et al., 2013; Jagoret et al., 2017; Wartenberg et al., 2017; Asare et al., 2018; Suárez et al., 2018). In some cases, farmers have found it necessary to remove forest tree species to achieve high grain yields (Anglaaere et al., 2011). This is due to a trade-off between yields and biodiversity within cropping systems (Notaro et al., 2022). However, authors such as (Trebissou et al., 2021), indicate that competition between trees occurs from the early years in cocoa plantations. Also, Hernández-Nuñez et al. (2020) concluded that proper management of companion species can generate high cocoa bean yields and different environmental and economic services.
Defining the percentage of shade for cocoa is accompanied by different reasons that the producer may have to determine the type of species and amount to plant. Among them, the various environmental, economic and food security services they can generate (Vaast and Somarriba, 2014). Some of these are the improvement of soil conditions (Anglaaere et al., 2011), biodiversity conservation and generation of ecosystem services (Deheuvels et al., 2014; Vaast and Somarriba, 2014; Asigbaase et al., 2019; Maney et al., 2022), adaptive capacity to climate variability and change (Andrade et al., 2013; Salvador et al., 2019; Notaro et al., 2021; Zequeira-Larios et al., 2021; Hernández-Núñez et al., 2021a), food production that diversify the diet and economic income of farmers (Vaast and Somarriba, 2014). Although environmental services are of global interest, Sanial et al. (2023) indicate that there are socioeconomic variables that are more determinant than environmental variables in making decisions on the association of trees to cocoa.
In our study, the typologies in Caquetá recorded a high number of companion species with potential timber and other uses. In some of these cases, this occurs because cocoa planting projects incorporate timber species as companion species (Rodríguez et al., 2022). In other cases, it may be due to the fact that the planting was generated in areas of natural regeneration, where cocoa replaces one of the strata and the upper strata are kept as shade (Jaimes et al., 2022). This is an important practice, as it reduces establishment and maintenance costs (Rodríguez et al., 2022). Furthermore, retained trees provide shade for cocoa and leaf mulch from shade trees and nutrients stored in the forest ground ensure productivity (Hosseini et al., 2017; Asigbaase et al., 2019; Côte et al., 2022). Additionally, these trees enable biodiversity conservation (Vaast and Somarriba, 2014), carbon sequestration (Hernández-Núñez et al., 2021a) and enhance better connectivity, which allows the movement of forest species between remnant patches of primary forest in a wider matrix than open land cocoa-AFS (Maney et al., 2022). These aspects are of high importance in departments such as Caquetá, which belong to the Amazon, but have high deforestation rates (Capdevilla et al., 2023).
Timber trees also diversify household income. In studies by Notaro et al. (2021) and Gonas et al. (2022) the species with high abundance were Cordia alliodora (Ruíz & Pav.) Oken, a species that also appears with high frequency in the plots of our study along with Cedrella odorata L. Authors such as Reppin et al. (2019), report the importance of other forest species for household provisioning services, such as construction and firewood. In the present investigation, this type of species has high frequency, mainly in the DMHSM typology. Hernández-Núñez et al. (2021a) report that these species also represent an important accumulation of carbon, which contributes to climate change mitigation.
The DMHDC and DMLS cocoa AFS present a high number of mosaic and fruit species, with high abundance and frequency of Musaceae and Persea americana Mill species, which are in agreement with global trends reported by different authors (Cerda et al., 2014; Deheuvels et al., 2014; Gonas et al., 2022; Asitoakor et al., 2022a; Ndo et al., 2023). In studies in the Peruvian Amazon, the species with the highest abundance was Musaceae (316 individuals in total) (Gonas et al., 2022); in cocoa crops in Ghana, Persea americana was the most common shade tree species (Asitoakor et al., 2022a). In research in Cameroon, fruit trees belonging to different families and species are one of the key components of cocoa-AFS in humid forest areas, accounting for up to 80% of the agrobiodiversity (Ndo et al., 2023). These types of companion species have a high potential for commercialization (Notaro et al., 2021), which diversifies household income sources (Asitoakor et al., 2022a; Jaimes et al., 2022). In addition, they provide food for household consumption, resulting in savings in household expenses (Hosseini et al., 2017). Also, they are shade generators for cocoa (Gonas et al., 2022), which also increases soil fertility by providing organic matter (Notaro et al., 2021).
This trend towards the predominance of fruit and timber trees may indicate that it would be advantageous to plant more citrus or other fruit trees and decrease cocoa trees (Notaro et al., 2021). This may be an indication of the deliberate transformation of the landscape by farmers from natural pioneer species traditionally grown with cocoa to species that provide food and medicinal benefits (Zequeira-Larios et al., 2021; Gonas et al., 2022). Concordant with these statements, the results of this research indicate that there is a negative correlation between cocoa tree abundance and companion species, mainly fruit and timber species. Zequeira-Larios et al. (2021) found in two communities in Mexico differences between the densities of cocoa trees and companion species, indicating that in one community (Tabasco) farmers are more focused on selling cocoa; in this way they keep cocoa trees in constant renewal and maintain shade trees and in (Chiapas), farmers in Chiapas obtain income from cocoa and fruit trees.
Under this scenario and despite the benefits that different authors have raised about companion trees (Andrade et al., 2013; Cerda et al., 2014; Deheuvels et al., 2014; Vaast and Somarriba, 2014; Hosseini et al., 2017; Saj et al., 2017; Wartenberg et al., 2017; Salvador et al., 2019; Hernández-Nuñez et al., 2020; Somarriba et al., 2021; Hernández-Núñez et al., 2021a; Maney et al., 2022), there is a need for proper practices on these trees in the design of cocoa-AFS (Gonas et al., 2022). This is due to factors such as the difficulty and time needed to harvest these trees, the higher space requirements of these species compared to cocoa trees, the sale of some fruit crops is sometimes not possible due to the lack of commercial contacts, which reduces the economic value of these associated species (Notaro et al., 2021), nutrient competition or negative allelopathic effects for cocoa (Jaimes et al., 2022). Finally, this trend of simplification within cocoa agroforests leads to the creation of agrochemical-dependent cocoa systems, called conventional cocoa systems, which smallholder farmers cannot manage due to high input costs (Asigbaase et al., 2019). This has reached extreme consequences, such as the removal of shade trees on their farms due to perceived competition for light, water and nutrients (Asitoakor et al., 2022b), demonstrating that there is a lack of coordinated definition and implementation of cocoa-based AFS, causing sustainable harvesting to be missed (Esche et al., 2022).
5 Conclusion
In Colombia there are five types of agroforestry designs based on cocoa according to the components of the tree structure, floristic composition and the abundance of cocoa trees. We found AFS Highly diversified multistratum with high biomass, diversified multistratum with high shade and abundance of Musaceae, diversified multistratum with high abundance of cocoa trees, diversified monostratum with low shade and monostratum with minimal shade. The most frequent typology was Highly diversified multistratum with high biomass, with an average of 788 individuals ha−1 of cocoa trees and 211 individuals ha−1 of companion species.
A total of 229 plant species were found within the cocoa-AFS in the area studied. The department of Caquetá, located in the Colombian Amazon, registered the highest number of companion species (66.3% of the total of the study) with a dominance of Musa paradisiaca L., Cariniana pyriformis Miers., Cedrela odorata L., Psidium guajava L., Musa sapientum L. and Cordia alliodora (Ruíz & Pav.) Oken. In Huila and Meta, a similar number of species was found (64 and 67 species); with the highest frequency of Musa paradisiaca L., Persea americana Mill. and Gliricidia sepium (Jacq.) Kunth ex Walp; Musa sapientum L. was found only in Huila. In all typologies Musa paradisiaca L. was the most frequent species (48 to 63% of the plots); however, its abundance differed among typologies.
Relationships were found between the components of tree structure, floristic composition and abundance of cocoa trees and climatic conditions. The highest abundance of cocoa trees was found in areas where temperature and relative humidity were lower. In areas of high temperature and low precipitation, a greater abundance of timber species was found, and the abundance of Musaceae was higher in areas with lower relative humidity. The Shannon Weaver index and the diversity of potential uses were negatively correlated with relative humidity.
Cocoa bean yields differed between typologies, with DMHDC and DMHSM having the highest (1,148 kg ha−1 yr.−1) and lowest (655 kg ha−1 yr.−1) values. The results allow us to conclude that cocoa bean yield was affected by different conditions of shade canopy, climate and phytosanitary status. In areas with higher rainfall, cocoa bean yield was higher; the opposite behavior occurred in areas with high incidence of Monilia, and high values of timber trees and Musaceae. It was found that the abundance of timber trees, fruit trees, Musaceae and other species was negatively related to cocoa yield, while the abundance of Fabaceae was positively correlated with this variable. The HDMHB typology, which presented a cocoa bean yield of 855 kg ha-1 year-1, higher than reported by different studies, an average abundance of companion species ha-1, mainly Musaceae, timber species and food generators, is a typology that allows to have significant income from cocoa beans and also allows a good provision of ecosystem services, contributions to food security and diversification of economic income, which can partially conclude that it is a typology with high potential to promote in future cocoa farms. However, it is important to solve future research questions that will help to make decisions based on data that integrate more components, such as what is the income derived from the companion species, what is the contribution of companion species to food and nutritional security of households, and what is the contribution of companion species to the food and nutritional security of households?
Finally, we conclude that these typologies have differentiated conditions of cocoa bean production and co-product generation. We found typologies in which there is a high diversity of companion species that generate different ecosystem services and have a high cocoa bean yield. These typologies are important because they become efficient production systems, contributing significantly to the well-being of the rural household by promoting food security, conservation of diversity, generation of extra income and adaptation and mitigation of climate change; aspects that are considered as challenges within the dynamics of the new rurality in Colombia. On the contrary, we found typologies that, although they have a high abundance of companion species, these are generally of natural regeneration processes and do not represent a current or potential use for households and, in addition, have low cocoa yields. These types of production systems can have a demotivating effect on rural producers. However, the design of the AFS is not the only factor that affects bean yield and the generation of co-products, therefore, new research questions arise, such as: What is the relationship between the social conditions of rural households and the agronomic conditions of the crop?
Data availability statement
The raw data supporting the conclusions of this article will be made available by the authors, without undue reservation.
Author contributions
HH-N: Conceptualization, Data curation, Formal analysis, Investigation, Methodology, Project administration, Writing – original draft, Writing – review & editing. JS: Conceptualization, Data curation, Formal analysis, Methodology, Writing – original draft, Writing – review & editing. HA: Conceptualization, Data curation, Investigation, Methodology, Writing – original draft, Writing – review & editing. JA: Conceptualization, Data curation, Methodology, Writing – original draft. RN: Conceptualization, Data curation, Methodology, Writing – original draft. DG: Conceptualization, Data curation, Investigation, Methodology, Writing – original draft. GG: Conceptualization, Data curation, Investigation, Methodology, Writing – original draft. IG-M: Conceptualization, Formal analysis, Methodology, Writing – review & editing. FC: Conceptualization, Data curation, Formal analysis, Methodology, Supervision, Validation, Writing – original draft, Writing – review & editing.
Funding
The author(s) declare financial support was received for the research, authorship, and/or publication of this article. This publication was funded by the Universidad de la Amazonia and Universidad del Tolima, Colombia.
Acknowledgments
The authors wish to thank the following collaborators: Ministry of National Education (MEN), Cooperativa Agroindustrial de Cacaoteros del Meta (CACAOMET), the Red de Asociaciones de Productores de Cacao del Huila (APROCAHUILA), the Asociación Departamental de Cultivadores de Cacao y Especies Maderables del Caquetá (ACAMAFRUT), the Unviersidad de la Amazonia and the Unviersidad del Tolima.
Conflict of interest
The authors declare that the research was conducted in the absence of any commercial or financial relationships that could be construed as a potential conflict of interest.
Publisher’s note
All claims expressed in this article are solely those of the authors and do not necessarily represent those of their affiliated organizations, or those of the publisher, the editors and the reviewers. Any product that may be evaluated in this article, or claim that may be made by its manufacturer, is not guaranteed or endorsed by the publisher.
Supplementary material
The Supplementary material for this article can be found online at: https://www.frontiersin.org/articles/10.3389/fsufs.2024.1295992/full#supplementary-material
References
Andrade, H., Figueroa, J., and Silva, D. (2013). Almacenamiento de carbono en cacaotales (Theobroma cacao) en armero-guayabal (Tolima, Colombia). Sci. Agroalimentaria 1, 6–10.
Anglaaere, L. C. N., Cobbina, J., Sinclair, F. L., and Mcdonald, M. A. (2011). The effect of land use systems on tree diversity: farmer preference and species composition of cocoa-based agroecosystems in Ghana. Agrofor. Syst. 81, 249–265. doi: 10.1007/s10457-010-9366-z
Arango-Ulloa, J., Bohorquez, A., Duque, M. C., and Maass, B. L. (2009). Diversity of the calabash tree (Crescentia cujete L.) in Colombia. Agrofor. Syst. 76, 543–553. doi: 10.1007/s10457-009-9207-0
Asare, R., Markussen, B., Asare, R. A., Anim-Kwapong, G., and Ræbild, A. (2018). On-farm cocoa yields increase with canopy cover of shade trees in two agro-ecological zones in Ghana. Clim. Dev. 11, 435–445. doi: 10.1080/17565529.2018.1442805
Asigbaase, M., Sjogersten, S., Lomax, B. H., and Dawoe, E. (2019). Tree diversity and its ecological importance value in organic and conventional cocoa agroforests in Ghana. PLoS One 14:e0210557. doi: 10.1371/journal.pone.0210557
Asitoakor, B. K., Asare, R., Ræbild, A., Ravn, H. P., Eziah, V. Y., Owusu, K., et al. (2022a). Influences of climate variability on cocoa health and productivity in agroforestry systems in Ghana. Agric. For. Meteorol. 327:109199. doi: 10.1016/j.agrformet.2022.109199
Asitoakor, B. K., Vaast, P., Ræbild, A., Ravn, H. P., Eziah, V. Y., Owusu, K., et al. (2022b). Selected shade tree species improved cocoa yields in low-input agroforestry systems in Ghana. Agric. Syst. 202:103476. doi: 10.1016/j.agsy.2022.103476
Ballesteros, W., Valencia, J. C., and Navia-Estrada, J. F. (2022). Assessment of a cocoa-based agroforestry system in the southwest of Colombia. Sustain. For. 14, 1–17. doi: 10.3390/su14159447
Balzarini, M. G., Gonzalez, L., Tablada, M., Casanoves, F., Di Rienzo, J. A., and Robledo, C. W. (2008). InfoStat. Córdoba, Argentina: Manual del Usuario.
Capdevilla, D. A. G., Bermúdez, O. B., and Aguirre, M. A. (2023). Alternativas Comunitarias a los Procesos de Deforestación en la Amazonía Colombiana. Caso el Caraño, Caquetá. Historia Ambiental Latinoamericana Caribeña Revista Solcha 13, 19–52. doi: 10.32991/2237-2717.2023v13i1.p19-52
Cerda, R., Deheuvels, O., Calvache, D., Niehaus, L., Saenz, Y., Kent, J., et al. (2014). Contribution of cocoa agroforestry systems to family income and domestic consumption: looking toward intensification. Agrofor. Syst. 88, 957–981. doi: 10.1007/s10457-014-9691-8
Correa, J., Castro, S., and Coy, J. (2014). Estado de la Moniliasis del cacao causada por Moniliophthora roreri en Colombia. Acta Agronóm. 63, 388–399. doi: 10.15446/acag.v63n4.42747
Côte, F. X., Rapidel, B., Sourisseau, J. M., Affholder, F., Andrieu, N., Bessou, C., et al. (2022). Levers for the agroecological transition of tropical agriculture. Agron. Sustain. Dev. 42, 1–11. doi: 10.1007/s13593-022-00799-z
Deheuvels, O., Avelino, J., Somarriba, E., and Malezieux, E. (2012). Vegetation structure and productivity in cocoa-based agroforestry systems in Talamanca, Costa Rica. Agric. Ecosyst. Environ. 149, 181–188. doi: 10.1016/j.agee.2011.03.003
Deheuvels, O., Rousseau, G. X., Soto Quiroga, G., Decker Franco, M., Cerda, R., Vílchez Mendoza, S. J., et al. (2014). Biodiversity is affected by changes in management intensity of cocoa-based agroforests. Agrofor. Syst. 88, 1081–1099. doi: 10.1007/s10457-014-9710-9
Di Rienzo, J., Casanoves, F., Balzarini, M., Gonzalez, L., Tablada, M., and Robledo, C. (2019). Infostat versión 2019. Córdoba, Argentina: Universidad Nacional de Córdoba
Di Rienzo, J., Macchiavelli, R., and Casanoves, F. (2011). Modelos lineales mixtos: aplicaciones en InfoStat. 1st Córdoba: Grupo InfoStat, 193
Di Rienzo, J.A., Macchiavelli, R.E., and Casanoves, F. (2017). Modelos lineales generalizados mixtos: aplicaciones en InfoStat.
Esche, L., Schneider, M., Milz, J., and Armengot, L. (2022). The role of shade tree pruning in cocoa agroforestry systems: agronomic and economic benefits. Agrofor. Syst. 97, 175–185. doi: 10.1007/s10457-022-00796-x
Escobar Ramírez, C. H., Córdoba-Gaona, Ó. D. J., Correa Londoño, G. A., and Martínez Bustamante, E. G. (2021). Yield of cocoa under different agroforestry systems in a dry tropical forest in western Colombia. Bioagro 34, 39–50. doi: 10.51372/bioagro341.4
Espinosa, J. A. (2016). Características estructurales y funcionales de un faro agroecológico a partir de las experiencias de productores cacaoteros de las regiones de los departamentos de Nariño, Meta, Caquetá y Tolima Doctorado en Agroecología Tesis de Doctorado, Universidad de Antioquia.
Fedecacao (2015). Guía técnica para el cultivo del Cacao. Bogotá: Federación Nacional de Cacaoteros.
Gama-Rodrigues, A. C., Müller, M. W., Gama-Rodrigues, E. F., and Mendes, F. A. T. (2021). Cacao-based agroforestry systems in the Atlantic Forest and Amazon biomes: an ecoregional analysis of land use. Agric. Syst. 194:103270. doi: 10.1016/j.agsy.2021.103270
Gonas, M., Rubio, K. B., Rojas Briceno, N. B., Pariente-Mondragon, E., and Oliva-Cruz, M. (2022). Tree diversity in agroforestry systems of native fine-aroma cacao, Amazonas, Peru. PLoS One 17:e0275994. doi: 10.1371/journal.pone.0275994
Guelly, K. A., Pereki, H., and Djiwa, O. (2021). Perceptions ethnoculturelles des services écosystémiques rendus par les agroforêts à base de cacaoyer au Togo. Base. 25, 208–222. doi: 10.25518/1780-4507.19153
Hernández-Núñez, H. E., Andrade Castañeda, H. J., Suárez, J. C., Gutiérrez, D., Gutiérrez García, G. A., Trujillo, E., et al. (2021a). Almacenamiento de carbono en sistemas agroforestales en los Llanos Orientales de Colombia. Biol. Trop. 69, 352–368. doi: 10.15517/rbt.v69i1.42959
Hernández-Núñez, H. E., Gutiérrez-Montes, I., Bernal-Núñez, A. P., Gutiérrez-García, G. A., Suárez, J. C., Casanoves, F., et al. (2021b). Cacao cultivation as a livelihood strategy: contributions to the well-being of Colombian rural households. Agric. Hum. Values 39, 201–216. doi: 10.1007/s10460-021-10240-y
Hernández-Nuñez, H. E., Gutiérrez-Montes, I., Gutiérrez, G. A., Sánchez, J., Suárez, J. C., and Casanoves, F. (2020). Agronomic state of cacao cultivation: its relationship with the capitals endowment of the Colombian rural households. Agrofor. Syst. 94, 2367–2380. doi: 10.1007/s10457-020-00556-9
Hosseini, S., Trueman, S. J., Nevenimo, T., Hannet, G., Bapiwai, P., Poienou, M., et al. (2017). Effects of shade-tree species and spacing on soil and leaf nutrient concentrations in cocoa plantations at 8 years after establishment. Agric. Ecosyst. Environ. 246, 134–143. doi: 10.1016/j.agee.2017.06.003
Ideam (2017). Atlas climatológico de Colombia. Bogotá: Instituto de Hidrología, Meteorología y Estudios Ambientales - IDEAM.
Jagoret, P., Michel, I., Ngnogué, H. T., Lachenaud, P., Snoeck, D., and Malézieux, E. (2017). Structural characteristics determine productivity in complex cocoa agroforestry systems. Agron. Sustain. Dev. 37, 1–12. doi: 10.1007/s13593-017-0468-0
Jagoret, P., Ngnogue, H. T., Malézieux, E., and Michel, I. (2018). Trajectories of cocoa agroforests and their drivers over time: lessons from the Cameroonian experience. Eur. J. Agron. 101, 183–192. doi: 10.1016/j.eja.2018.09.007
Jaimes, Y., Aránzazu, F., Rodríguez, E., and Martínez, N. (2011). Behavior of introduced regional clones of Theobroma cacao toward the infection Moniliophthora roreri in three different regions of Colombia. Agronomia Colombiana 2, 171–178.
Jaimes, Y. Y., Carvajal-Rivera, A. S., Galvis-Neira, D. A., Carvalho, F. E. L., and Rojas-Molina, J. (2022). Cacao agroforestry systems beyond the stigmas: biotic and abiotic stress incidence impact. Front. Plant Sci. 13:921469. doi: 10.3389/fpls.2022.921469
Koutouleas, A., Sarzynski, T., Bordeaux, M., Bosselmann, A. S., Campa, C., Etienne, H., et al. (2022). Shaded-coffee: a nature-based strategy for coffee production under climate change? A review. Front. Sustain. Food Syst. 6:877476. doi: 10.3389/fsufs.2022.877476
Lavoie, A., Thomas, E., and Olivier, A. (2023). Local working collections as the foundation for an integrated conservation of Theobroma cacao L. in Latin America. Front. Ecol. Evol. 10:10. doi: 10.3389/fevo.2022.1063266
Maney, C., Sassen, M., and Hill, S. L. L. (2022). Modelling biodiversity responses to land use in areas of cocoa cultivation. Agric. Ecosyst. Environ. 324:107712. doi: 10.1016/j.agee.2021.107712
Motamayor, J. C., Risterucci, A. M., Lopez, P. A., Ortiz, C. F., Moreno, A., and Lanaud, C. (2002). Cacao domestication I: the origin of the cacao cultivated by the Mayas. Heredity (Edinb) 89, 380–386. doi: 10.1038/sj.hdy.6800156
Ndo, E. G. D., Akoutou Mvondo, E., Bidzanga Nomo, L., Bella Manga, F., Ambang, Z., and Cilas, C. (2023). Farmer’s strategies in the choice of citrus spatial structures in cocoa-based agroforests in Cameroon. Agrofor. Syst. 97, 659–672. doi: 10.1007/s10457-023-00817-3
Ngo Bieng, M. A., Gidoin, C., Avelino, J., Cilas, C., Deheuvels, O., and Wery, J. (2013). Diversity and spatial clustering of shade trees affect cacao yield and pathogen pressure in Costa Rican agroforests. Basic Appl. Ecol. 14, 329–336. doi: 10.1016/j.baae.2013.03.003
Notaro, M., Collado, C., Depas, J. K., Dumovil, D., Denis, A. J., Deheuvels, O., et al. (2021). The spatial distribution and height of associated crops influence cocoa tree productivity in complex agroforestry systems. Agron. Sustain. Dev. 41, 1–14. doi: 10.1007/s13593-021-00716-w
Notaro, M., Deheuvels, O., and Gary, C. (2022). Participative design of the spatial and temporal development of improved cocoa agroforestry systems for yield and biodiversity. Eur. J. Agron. 132:126395. doi: 10.1016/j.eja.2021.126395
Notaro, M., Gary, C., and Deheuvels, O. (2020). Plant diversity and density in cocoa-based agroforestry systems: how farmers’ income is affected in the Dominican Republic. Agrofor. Syst. 94, 1071–1084. doi: 10.1007/s10457-019-00472-7
Numbisi, F. N., Alemagi, D., Degrande, A., and Van Coillie, F. (2021). Farm rejuvenation-induced changes in tree spatial pattern and live biomass species of cocoa agroforests in Central Cameroon: insights for tree conservation incentives in cocoa landscapes. Sustain. For. 13, 1–22. doi: 10.3390/su13158483
Ortíz, C., Torres, M., and Hernández, S. (2015). Comparación de dos sistemas de manejo del cultivo del cacao, en presencia de Moniliophthora roreri, en México. Rev. Fitotec. Mex. 38, 191–196. doi: 10.35196/rfm.2015.2.191
R Core Team (2021). R: A language and environment for statistical computing. Vienna, Austria. Available at: https://www.R-project.org/
Ramírez, J. (2016). Pérdidas económicas asociadas a la pudrición de la mazorca del cacao causada por Phytophthora spp.,y Moniliophthora roreri (Cif y Par) Evans et al., en la hacienda Theobroma, Colombia. Revist. Protec. Vegetal. 31, 42–49.
Reppin, S., Kuyah, S., De Neergaard, A., Oelofse, M., and Rosenstock, T. S. (2019). Contribution of agroforestry to climate change mitigation and livelihoods in Western Kenya. Agrofor. Syst. 94, 203–220. doi: 10.1007/s10457-019-00383-7
Rodríguez, T., Bonatti, M., Löhr, K., Lana, M., Del Río, M., and Sieber, S. (2022). Analyzing influencing factors to scale up agroforestry systems in Colombia: a comparative ex-ante assessment of cacao farming and cattle ranching in two regions. Agrofor. Syst. 96, 435–446. doi: 10.1007/s10457-022-00730-1
Saj, S., Durot, C., Mvondo Sakouma, K., Tayo Gamo, K., and Avana-Tientcheu, M. L. (2017). Contribution of associated trees to long-term species conservation, carbon storage and sustainability: a functional analysis of tree communities in cacao plantations of Central Cameroon. Int. J. Agric. Sustain. 15, 282–302. doi: 10.1080/14735903.2017.1311764
Salazar-Díaz, R., and Tixier, P. (2017). Effect of plant diversity on income generated by agroforestry systems in Talamanca, Costa Rica. Agrofor. Syst. 93, 571–580. doi: 10.1007/s10457-017-0151-0
Salvador, P., Cámara, L. D. C., Martínez, J. L., Sánchez, R., and Valdés, E. (2019). Diversidad, estructura y carbono de la vegetación arbórea en sistemas agroforestales de cacao. Madera Bosques 25, 1–14. doi: 10.21829/myb.2019.2511638
Sanial, E., Ruf, F., Louppe, D., Mietton, M., and Hérault, B. (2023). Local farmers shape ecosystem service provisioning in west African cocoa agroforests. Agrofor. Syst. 97, 401–414. doi: 10.1007/s10457-021-00723-6
Schmidt, J. E., Firl, A., Hamran, H., Imaniar, N. I., Crow, T. M., and Forbes, S. J. (2022). Impacts of shade trees on the adjacent cacao rhizosphere in a young diversified agroforestry system. Agronomy 12, 1–16. doi: 10.3390/agronomy12010195
Scudder, M., Wampe, N., Waviki, Z., Applegate, G., and Herbohn, J. (2022). Smallholder cocoa agroforestry systems; is increased yield worth the labour and capital inputs? Agric. Syst. 196:103350. doi: 10.1016/j.agsy.2021.103350
Somarriba, E., Peguero, F., Cerda, R., Orozco-Aguilar, L., López-Sampson, A., Leandro-Muñoz, M. E., et al. (2021). Rehabilitation and renovation of cocoa (Theobroma cacao L.) agroforestry systems. Agron. Sustain. Develop. 41, 1–19. doi: 10.1007/s13593-021-00717-9
Sparks, A. (2018). Nasapower: a NASA POWER global meteorology, surface solar energy and climatology data client for R. J. Open Source Soft. 3:1035. doi: 10.21105/joss.01035
Sparks, A. (2021). Nasapower: NASA-POWER data from R. R package version 4.0.0. Available at: https://CRAN.R-project.org/package=nasapower
Sterling, C. A., Hermida, M. A., Rodríguez, C. H., Salas, Y. M., Nieto, M. N., and Caicedo, D. F. (2015). Reacción a Moniliophthora roreri en Theobroma spp. en Caquetá, Colombia. Summa Phytopathol. 41, 183–190. doi: 10.1590/0100-5405/2026
Suárez, J. C., Ngo Bieng, M. A., Melgarejo, L. M., Di Rienzo, J. A., and Casanoves, F. (2018). First typology of cacao (Theobroma cacao L.) systems in Colombian Amazonia, based on tree species richness, canopy structure and light availability. PLoS One 13:e0191003. doi: 10.1371/journal.pone.0191003
Trebissou, C. I., Tahi, M. G., Munoz, F., Sanchez, L., N’Guetta, S. P. A., Cilas, C., et al. (2021). Cocoa breeding must take into account the competitive value of cocoa trees. Eur. J. Agron. 128:126288. doi: 10.1016/j.eja.2021.126288
Vaast, P., and Somarriba, E. (2014). Trade-offs between crop intensification and ecosystem services: the role of agroforestry in cocoa cultivation. Agrofor. Syst. 88, 947–956. doi: 10.1007/s10457-014-9762-x
Vargas, A., Somarriba, E., and Carballo, M. (2005). Dinámica poblacional del chinche (Monalonion dissimulatum Dist.) y daño de mazorcas en plantaciones orgánicas de cacao del Alto Beni, Bolivia. Agroforestería Am. 43, 72–76.
Wartenberg, A. C., Blaser, W. J., Gattinger, A., Roshetko, J. M., Van, M., and Six, J. (2017). Does shade tree diversity increase soil fertility in cocoa plantations? Agric. Ecosyst. Environ. 248, 190–199. doi: 10.1016/j.agee.2017.07.033
Keywords: sustainable agriculture, agroforestry systems, ecosystem services, self-consumption, floristic composition
Citation: Hernández-Nuñez HE, Suárez JC, Andrade HJ, Acosta JRS, Núñez RD, Gutiérrez DR, Gutiérrez GA, Gutiérrez-Montes I and Casanoves F (2024) Interactions between climate, shade canopy characteristics and cocoa production in Colombia. Front. Sustain. Food Syst. 8:1295992. doi: 10.3389/fsufs.2024.1295992
Edited by:
Roberto Valdivia, Oregon State University, United StatesReviewed by:
Philippe Vaast, DGDRS CIRAD, FranceAthina Koutouleas, The University of Copenhagen, Denmark
Copyright © 2024 Hernández-Nuñez, Suárez, Andrade, Acosta, Núñez, Gutiérrez, Gutiérrez-Montes and Casanoves. This is an open-access article distributed under the terms of the Creative Commons Attribution License (CC BY). The use, distribution or reproduction in other forums is permitted, provided the original author(s) and the copyright owner(s) are credited and that the original publication in this journal is cited, in accordance with accepted academic practice. No use, distribution or reproduction is permitted which does not comply with these terms.
*Correspondence: Héctor Eduardo Hernández-Nuñez, aGVoZXJuYW5kZXpuQHV0LmVkdS5jbw==; Juan Carlos Suárez, anUuc3VhcmV6QHVkbGEuZWR1LmNv