- 1Institute of Nutrition, Mahidol University, Salaya, Thailand
- 2ICAR-Central Coastal Agricultural Research Institute, Goa, India
- 3Sargento Foods, Elkhart Lake, WI, United States
- 4Lactalis USA, Buffalo, NY, United States
- 5Faculty of Agricultural Sciences and Technologies, Nigde Omer Halisdemir University, Nigde, Türkiye
- 6Sustainable AgriFoodtech Innovation and Research (SAFIR), Arras, France
Plant proteins have been considered a potential source of bio-functional peptides, which can be beneficial for human health. The potent antioxidant properties of plant-based peptides protect against oxidative damage and associated diseases. Despite a significant number of studies on the preparation and biological functions of plant-based peptides, only a limited number of peptides are commercially utilized. The systematic studies of the extraction, optimization, isolation, pharmacokinetics, stability, and safety aspects of plant protein-derived peptides (PPDP) are in progress. Also, the molecular mechanism of action and health benefits of bioactive PPDP is still lacking. Hence, this review provides a comprehensive discussion of various plant protein sources to end-product applications of PPDP. In this context, different plant sources explored for functional and bioactive PPDP have been presented. The green biotechnological techniques such as fermentation and enzymatic hydrolysis for extraction of PPDP have been described. The functional and biological properties of PPDP have been revisited. The most important part of the application which includes the stability and bioavailability of PPDP has been discussed. Additionally, the health impact of PPDP administration has been summarized. Lastly, future perspectives and concluding remarks have been documented.
1 Introduction
Plant-based proteins and peptides have been gaining enormous attention in recent years due to the increased demand for food to cover the needs of a rapidly growing world population. In addition to the growing awareness against animal-based food which produces greenhouse gases and impacts the environment and human health. In 2019, the Eat-Lancet Commission suggested a “planetary” diet consisting primarily of plant-based foods (i.e., legumes, peanuts, tree nuts, seeds, and soy), where animal-based foods contribute a relatively small proportion. Such a diet is considered to have a positive impact on both human health and a sustainable environment (Willett et al., 2019). In the same year, the World Health Organization (WHO) and the Food and Agriculture Organization (FAO) published guiding principles for sustainable and healthy diets that promote a wide range of plant-sourced food items such as whole grains, legumes, a variety of fruit and vegetables, and nuts, with moderate amounts of dairy, poultry, eggs, fish, and small amounts of red meat (WHO, 2019). Moreover, recent publications have highlighted the promising health-beneficial potential of plant-based proteins and peptides in the treatment of various diseases and disorders. For example, recent investigations have reported that plant protein-derived peptides (PPDP) can be used in the treatment of diabetes (Patil et al., 2020), memory enhancement (Katayama et al., 2021), and cancer therapy (Vuyyuri et al., 2018). In addition, these valuable natural compounds can exhibit appetite-regulating effects (Kaneko, 2021) and have an efficient role in nutrient and drug delivery (Kumar et al., 2022). These beneficial properties have been attributed to their antioxidant, anti-microbial, and anti-inflammatory activities (Wen et al., 2020; Wong et al., 2020; Mammari et al., 2021; Fan et al., 2022). The aforementioned beneficial biological properties have driven the rapid development of plant-based proteins and peptides in functional food industries, nutraceuticals, and many other industrial applications, such as feed applications, cosmetics, and medicine industries (Wong et al., 2020; Kumar et al., 2022; Sharma et al., 2022).
However, one challenging issue is to retain the biological activities (e.g., antioxidant, anti-microbial, and anti-inflammatory) and functional properties (e.g., emulsifying capacity, water holding capacity, and foaming stability) of PPDP during the extraction and the following processing stages, thus maintaining their health-promoting benefits. Traditional extraction methods using chemicals have been widely applied that could affect both the environment and human health. In recent years, a wide range of alternative green extraction technologies has been practiced including a biotechnological approach (microbial fermentation and enzymatic hydrolysis) and non-thermal techniques (high hydrostatic pressure, supercritical/subcritical, ultrasound, and microwave-assisted extraction methods) (Singh et al., 2022; Rivero-Pino et al., 2023). Given the importance of the field, recently few review articles have been published focusing on isolation and mechanism of action of antioxidant PPDP (Wen et al., 2020), antimicrobial PPDP (Sharma et al., 2022; Rivero-Pino et al., 2023), various bioactivities (Fan et al., 2022), emerging production techniques (Singh et al., 2022), cereal protein-based peptide- production and bioactivities (Gong et al., 2022). The current review scrutinized all aspects of PPDP from sources to end product applications. The article highlighted all potential plant sources for protein extraction and peptide production. The green biotechnological approach including microbial fermentation and enzymatic hydrolysis for PPDP production has been discussed. The most important part of the research application including the functional and biological properties of PPDP have been highlighted. Additionally, critical points of food and nutraceutical industry applications which are the stability and bioavailability of PPDP have been summarized. Furthermore, the health benefits of PPDP including blood sugar control, bone and joint health, brain and cognitive health, and heart health have been explained. Finally, future trends and concluding remarks are presented.
2 Plant protein sources
Various plants including fruits, legumes, cereals, pseudocereals, seeds, nuts, shoots, and leaves, can provide outstanding quality proteins. The currently used plant sources owing to their high protein content are described below.
2.1 Legumes
Legumes are edible pods, seeds, or other parts of the Leguminosae family and possess abundant nutrients. Legumes are considered a significant source of protein; however, their consumption by humans is relatively low, constituting only a minor portion of the typical human diet. On average, individuals worldwide consume approximately 21 g of legumes per day (Semba et al., 2021). With a protein content of 20–45% and a high concentration of the essential amino acid lysine, legumes are a superior source of protein (Ambika et al., 2022). Whereas lupins and soybeans have a greater protein content (38–45%), beans and peas have relatively lower levels (17–20%) (Ambika et al., 2022). However, leguminous proteins are regarded as an incomplete protein supply because they are generally deficient in sulfur-containing amino acids (cysteine, tryptophan, methionine, and cystine), except soy protein (Maphosa and Jideani, 2017). Leguminous proteins contain albumins and globulins, which are classified as vialin and legumin. Most legumes’ main protein component, vialin, has a low concentration of sulfur-containing amino acids (Maphosa and Jideani, 2017).
2.2 Cereals
Cereal proteins are derived from grain sources such as rice, millet, barley, corn, sorghum, wheat, and its bran. Cereals contribute a considerable part of the food pyramid and serve as the primary source of energy. Considering plant proteins, cereal proteins do have a balanced amino acid composition in a rather small amount but are digestible and absorbed by the human body (Cavazos and Gonzalez de Mejia, 2013). The protein content in cereal grains varies from 10 to 15% of dry weight, and a significant portion of the protein content comes from storage proteins (Shewry and Halford, 2002). These storage proteins include prolamins, germains, and globulins. Prolamins are the primary storage proteins in most cereals, except for rice and oats, which have a higher content of globulins (Cavazos and Gonzalez de Mejia, 2013). Rice and rice bran are excellent sources of high-quality, hypoallergenic protein (Amagliani et al., 2017) that can be used in various applications. Rice bran protein hydrolysate contains several biologically active peptides that possess potent antihypertensive (Suwannapan et al., 2020) and antioxidant properties (Phongthai and Rawdkuen, 2020). Sorghum, on the other hand, has a higher protein content, but its quality is low, with digestibility ranging between 30 and 70% based on the variety and how it is grown (Agrawal et al., 2017). Though its protein quality is poor, hydrolysis of sorghum protein by alkaline protease can result in peptides with higher antioxidant and ACE-inhibitory activity (Jia et al., 2019). Likewise, protein from wheat possesses a higher concentration of essential amino acids and also contributes an estimated 30% of the dietary protein intake of an individual (Gong et al., 2022). Wheat gluten contains a significant amount of alanine, which also aids in the elasticity of bakery product formulation as it forms a gluten network (Cian et al., 2015). Similarly, maize protein consists mainly of zein and gluten (Zhou et al., 2013), and has high levels of branched-chain and neutral amino acids, which can be used to create short peptides with high activity (Gong et al., 2022).
2.3 Pseudocereals
There has been a recent surge in interest in using pseudocereals such as amaranth, buckwheat, and quinoa as a source of high-quality protein, unsaturated fatty acids, vitamins, minerals, and fiber. They are also gluten-free, making them suitable for those with celiac disease. Studies have shown that quinoa and amaranth contain high levels of lysine, an essential amino acid that is often lacking in cereals, making them potentially valuable dietary supplements (López et al., 2018). Compared to wheat and maize, amaranth has a lysine level that is twice as high. Moreover, other amino acids such as tryptophan, arginine, and sulfur-containing amino acids are also abundant in amaranth (Malik and Singh, 2022). Buckwheat, which includes Tartary buckwheat and common buckwheat, has an amino acid composition that meets the essential amino acid content requirements set by the FAO and WHO for food proteins. It is considered to be one of the most nutritious grain crops due to its comprehensive amino acid profile and high biological value (Tang et al., 2009; Sytar et al., 2016), as it is rich in sulfur-containing amino acids and other essential amino acids. Specifically, two amino acids (proline and glutamic acid) account for half of the amino acids in buckwheat protein (Malik and Singh, 2022). The protein in buckwheat is mainly made up of albumin and globulin, but the presence of trypsin and other protease inhibitors in buckwheat can result in poor taste and low digestibility of its protein (Zhou et al., 2015) which limits its practical applications.
2.4 Seeds and nuts
Incorporating certain seeds into the diet has become a popular trend to improve the quality of nutrition. The most commonly consumed seeds include chia seeds, flaxseed, pumpkin seeds, hemp seeds, watermelon seeds, and so on. These seeds are abundant sources of protein and other nutrients. Flaxseed, for instance, is an excellent source of high-quality protein and other phytochemicals along with a favorable essential amino acid profile (Kajla et al., 2015; Dahal and Koirala, 2020). Studies in flaxseed protein isolate signify the higher concentration of non-polar amino acid, sulfur-containing amino acid followed by aromatic amino acids and the most dominant amino acid was isoleucine (15.31 g/100 g) and glutamine (13.6 g/100 g) (Sharma and Saini, 2022). However, lysine, cystine, and methionine are the limiting amino acid (Ganorkar and Jain, 2013). Other seeds are also excellent sources of specific amino acids, such as leucine and arginine in watermelon seed, aromatic amino acids, lysine, threonine, tryptophan in paprika seed, and so on. However, these seeds’ protein lacks certain amino acids including lysine in chia seed, isoleucine, and sulfur amino acids in paprika seed (Olivos-Lugo et al., 2010; Nasiru and Oluwasegun, 2019; Cvetkovic et al., 2022). Although nuts and almonds are high in lipids, they also contain a considerable amount of protein. Native Brazilian Savannah almonds like Baru and pequi are excellent complementary sources of protein with complete amino acid profiles (Sá et al., 2020). Pequi almonds, which have a higher content of sulfur amino acids and lysine, the first limiting amino acid, are similar to cashew nuts (Sá et al., 2020).
3 Biotechnological approach for plant protein-derived peptide extraction
The conventional chemical methods (acid/alkaline hydrolysis) are well-known and reviewed many times. This section explained the green biotechnology techniques including microbial fermentation and enzymatic hydrolysis as a sustainable approach for the extraction of PPDP.
3.1 Microbial fermentation
Microbial fermentation of protein-containing foods usually involves proteolysis by protease-producing yeast or bacteria (Figure 1). Throughout bacterial growth, these bacteria secrete proteolytic enzymes that cause dietary proteins to hydrolyze into biopeptides. It is simple to produce biopeptides by microbial fermentation. The chosen microbe is initially bred into an exponential phase in the nutrient medium, which is then isolated and introduced to the food material as a starter culture (Aguilar-Toalá et al., 2017; Daliri et al., 2017). The microbial strain, type of protein source, and fermentation circumstances all have a significant impact on the resulting peptides qualities, types, and amount of release (Görgüç et al., 2020). Bioactive peptides can also be released through fermentation, which is often carried out by different kinds of microbes in a submerged or solid form. The ability to eliminate the production costs of the enzyme (proteases) for hydrolysis is one advantage over enzymatic hydrolysis (Nasri et al., 2022). Yet, in microbial fermentation, proteins are cleaved by microbes and the proteases produced by them, as opposed to enzymatic hydrolysis, which is usually carried with food-grade proteases. Because of this, the choice of microbial strain and any related proteolytic activity will decide the best release of bioactive peptides, regardless of the specificity of the protease used in the enzymatic hydrolysis (Nasri et al., 2022). Lactobacillus species are most frequently used to create protein hydrolysates as indicated in Table 1. To prevent safety issues, the microbe used for the fermentation must be harmless, or at the very least, its deactivation and removal from the finished product must be guaranteed. The biggest drawback of using fermentation as a processing method is the possibility of producing additional substances (such as exopolysaccharides or bacteriocins), which should also be characterized and evaluated for their biological characteristics and safety. Xiao et al. (2018) fermented red bean and produced short peptides. The fermented bean had a strong inhibitory impact on the angiotensin-I converting enzyme (ACE). Wu et al. (2018) fermented whole-grain oats for 72 h using Lactobacillus plantarum B1-6, Rhizopus oryzae, or a combination of both. When both microorganisms were used, the degree of hydrolysis increased. A greater ACE inhibitory action was also present in the fermented oats. The degrading of plants’ rigid cell walls by microbial enzymes, which increases protein digestibility and biopeptide release, has been linked to an increase in bioactivity during fermentation (Di Stefano et al., 2019). Due to its poor repeatability between batches, fermentation is also a less precise process than enzymatic hydrolysis, which hinders the production of a produced well with no batch-to-batch variation.
3.2 Enzymatic hydrolysis
Enzymatic hydrolysis, a sustainable method for the extraction of plant protein-based peptides (Figure 2), is more predictable than fermentation. However, several factors, such as non-protein compounds, interfere with the hydrolysis process, so the initial extraction of crude protein is crucial. For instance, phenolic chemicals can drastically reduce the enzymatic activity of certain enzymes (Ying et al., 2021). When the crude protein is subjected to a specific protease, targeted peptide cleavage can result; however, the selection of a suitable enzyme and enzymatic condition for the targeted peptide is a must. Moreover, in this process, the selected protease, nature of proteins, and ambient hydrolysis conditions considerably affect the yield (Koirala et al., 2023). Peptides might be released via the site-specific action of proteases, which would precisely target peptide bonds based on their active site. Yet, due to the release of hydrophobic residues during enzymatic hydrolysis, some peptides may have a bitter taste (Rivero-Pino et al., 2023). A wide variety of peptide bonds can be broken by the endoprotease subtilisin; however, it preferentially cleaves methionine and aromatic residues (Berraquero-García et al., 2022). This protease releases positively charged peptides into the medium with a preference for arginine or lysine residues. When hydrolyzing proteins using multiple proteases (either simultaneously or sequentially), the pH and temperature of the reaction should be considered, as they will alter the activity of the protease. For instance, trypsin activity can decrease above 47°C, resulting in prolonged hydrolysis and an ineffective release of peptides, while subtilisin exhibits stronger resilience to high temperatures and has been shown to affect the release of peptides (Rivero-Pino et al., 2021).
Enzymatic hydrolysis has been reported to have several advantages over other conventional processes, such as an easy and quick process, a mild production environment, high specificity, predictability, and scalability. Moreover, studies have documented that peptides isolated from enzymatic hydrolysis have enhanced solubility and heat resistance. Specific proteases are utilized for the cleaving of the peptide, which could be derived either from animals, such as pepsin, chymotrypsin, or trypsin, plant enzymes like bromelain, or microorganisms, such as neutrase, alcalase, or flavorzymes. Nevertheless, protease efficiency and proteolysis, as well as the functionality of peptides, depend upon the hydrolysis environment (hydrolysis time, enzyme-substrate ratio, temperature, and pH) (Toldrá et al., 2020; Koirala et al., 2023).
4 Identification and analyses of PPDP
Identification and characterization of bioactive peptides have garnered considerable interest over the past few decades. Quantifying bioactive peptides has proven to be challenging and requires continual analytical breakthroughs (Okoye et al., 2022). However, numerous chromatography techniques, such as membrane ultracentrifugation, high-performance liquid chromatography, electrophoresis, ultrafiltration, precipitation, and others are frequently used to separate and identify bioactive peptides and proteins. Regarding selectivity and recovery, affinity chromatography methods are the most effective downstream processing techniques at the moment (Vollet Marson et al., 2020). Further identification of the peptide can be achieved using mass spectrometry (Moyer et al., 2021) and combination methods (tandem mass spectrometry in combination with LC–MS) (Zhang et al., 2022).
4.1 Liquid chromatography-mass spectrometry
Liquid chromatography-mass spectrometry (LC–MS) is an effective method for the identification and quantification of plant protein-derived peptides (Alves et al., 2019; Wen et al., 2020). Due to its high degree of sensitivity and capability to identify even minute levels of peptides in matrices, LC–MS is the most promising technique for determining the presence of bioactive peptides (Alves et al., 2019). Because of the varied sample types to be examined, it might never be viable to suggest a single standard LC–MS technique for metabolite profiling.
4.2 Ultra-high-performance liquid chromatography–tandem mass spectrometry
It has been demonstrated that UHPLC, which is widely used in peptide identification and isolation, is effective for analyzing complex mixtures in both isocratic and gradient modes (Sorensen et al., 2020; Okoye et al., 2022). The combination of UHPLC and mass spectrometry (MS) gives additional benefits in terms of sensitivity, selectivity, and high throughput for the investigation of peptides. A useful method for both targeted and nontargeted analysis is UHPLC linked to tandem MS (Nováková et al., 2017). The UHPLC-ESI-MS/MS has recently been demonstrated to be a useful tool for the simultaneous determination of plant-based bioactive peptides due to its rapid separation power, high capacity, low solvent use, high resolution, high specificity and selectivity, and multiple ion detection based on selective ion fragmentation (Pandey et al., 2015).
4.3 Matrix-assisted laser desorption/ionization time-of-flight mass spectrometry
The Matrix-Assisted Laser Desorption/Ionization (MALDI) system compares the protein fingerprint with a database of reference spectra using a variety of algorithms built into the system (Welker and Moore, 2011). A TOF analyzer, which counts the mass of intact peptides, is frequently used in conjunction with MALDI. It is referred to as a “soft” ionization technique since MALDI-TOF MS produces little to no fragmentation and allows the detection of molecular ions even in complex mixtures of biopolymers (Susnea et al., 2013). In terms of applications for analyzing biological samples, MALDI-TOF-MS has established itself as a relatively adaptable technology, particularly for proteins and peptides (Alves et al., 2019).
5 Functional properties of PPDP
PPDP is known for improving the quality of food by modifying the oil emulsification, form formation, colloidal stability, solubility, aroma retention, and viscosity of the finished product. The presence of hydrophobic amino acids (tryptophan, tyrosine, and L-phenylalanine) in these peptides regulates the functional characteristics of peptides viz. foaming, emulsification, water holding capacity, and solubility (Table 2; Görgüç et al., 2020). Figure 3 represents the PPDP extraction from various plant sources and their functional and biological properties.
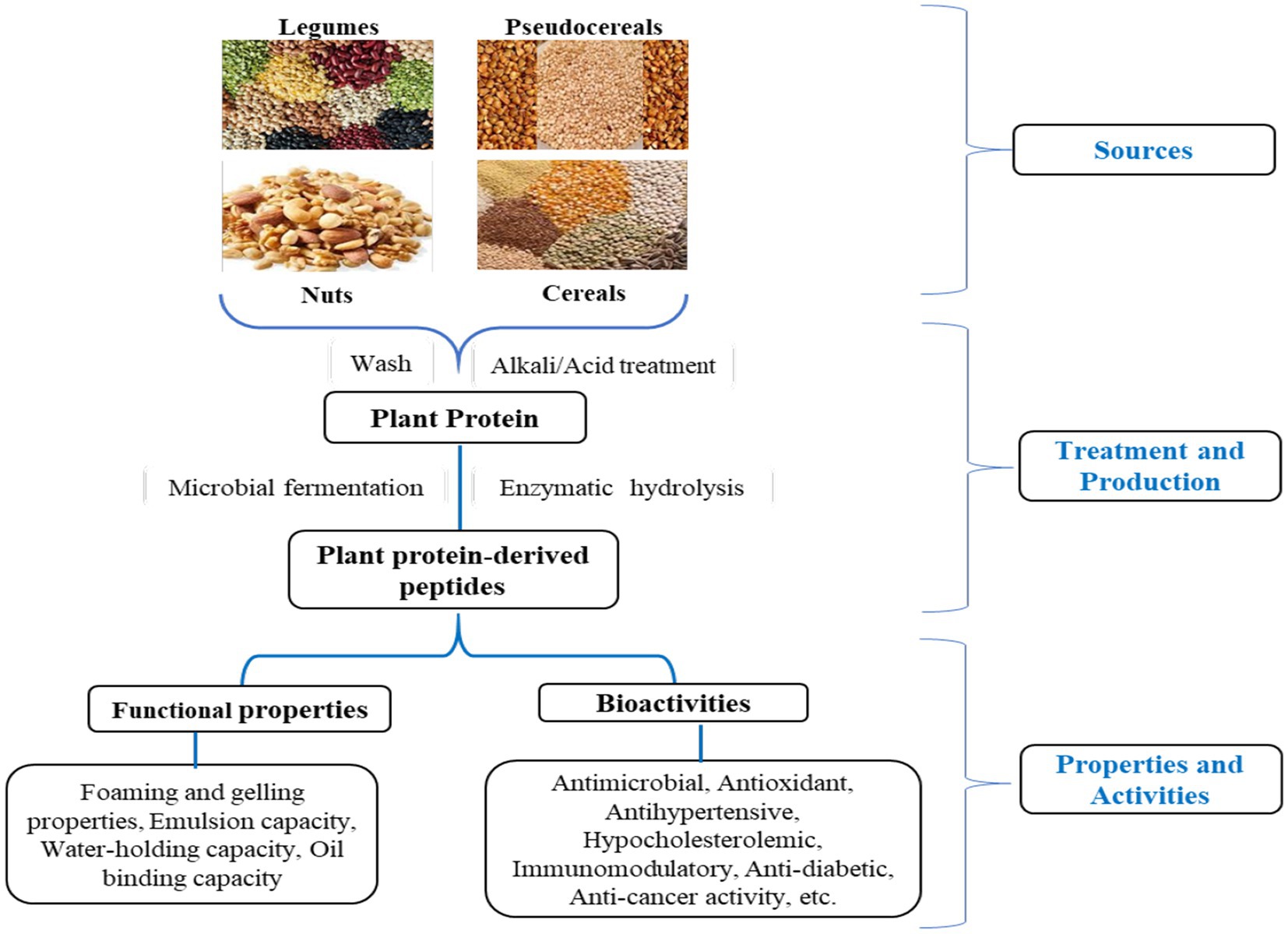
Figure 3. Schematic presentation of plant protein sources, treatment and production, and properties and activities of plant protein derived-peptide.
5.1 Foaming and gelling properties
To enhance the textural and sensorial properties of the final aerated products, such as sponge cakes, ice creams, mousses, and desserts, foaming agents are crucial. Due to their surface-active properties, PPDP shows elevated affinity at the water/air interface, directly related to its amphiphilic structure. Peptides provide good foaming properties and are therefore used as foam stabilizers, altering the textural and structural attributes of food (Hou F. et al., 2017). Previous studies demonstrated that utilizing pre-heat treated enzyme hydrolysates of soybean derivates showed enhanced emulsifying and foaming attributes when compared to other proteins (Liang et al., 2020). Recent studies by Liu et al. (2021) reported that the amphiphilic nature of corn-derived zein peptide resulted in the formation of a nanoparticle-sized spherical network of micellar structure through self-assembly methodology and therefore can be utilized as a foaming and emulsifying agent. The foaming capacity of PPDP is associated with the presence of hydrophobic amino acids, which affect the air-liquid interface, the flexibility of molecular proteins, the solubility and denaturability of the proteins. These mentioned variables comprise molecule migration or transport, infiltration/penetration, and rearrangement at the air-water interface and are thus responsible for forming foam. However, for efficient foaming ability, a peptide must quickly migrate to the air-water interface and must have good unfolding, foaming, and rearranging properties. The hydrophobic nature of peptides is associated with their solubility in lipids, oils, and fats, while hydrophilic amino acids attract more to the aqueous medium (Acquah et al., 2018). Therefore, based on the type of food matrix, it is important to maintain a hydrophobicity/ hydrophilicity balance in the food. Additionally, PPDP has a good emulsion stability index and emulsifying activity index and transforms water-holding capacity by affecting the water-binding capabilities of peptides (Görgüç et al., 2020). The stability of foam is associated with the nature of the film and echoes the level of protein–protein interaction in the matrix (Yi-Shen et al., 2018). A recent study by Tang et al. (2021) demonstrated that incorporating different peptides from various sources such as corn, soy, legumes, and whey can significantly improve the elastic modulus of foam and enhance the overall moisture efficiency of foam when added to the white egg powder.
The capability of PPDP to form gels is another widely known attribute. The formation of gel is a complex process that contains several phases, such as kinetics, including association and dissociation, denaturation, and aggregation. The gel is a colloidal or diluted solid–liquid intermediate that does not have any constant flow. Protein gelation can be triggered by changes in pH, pressure, salt, shearing, the presence of certain solvents, and heat treatment; therefore, the quality and structure of several food products depend on the heat-induced protein gels (Wang and Xiong, 2019). In many foods, the gel component plays a significant role in providing sensory and textural features, which in several cases are a three-dimensional network of water-filled polymers (Ashaolu, 2020). A study by Lopes-da-Silva and Monteiro (2019) applied neutral polysaccharides to soy protein hydrolysates containing bioactive peptides and discovered the gelling characteristics of hydrolyzed soy protein. The findings indicated that the presence of calcium played a critical role in creating calcium ion bridges between peptides that stabilized and promoted gel formation. Kamani et al. (2019) identified that fortifying bioactive peptides from gluten and soy peptide isolates in sausage formulation improved the stability of emulsion and augmented gel-formation attributes by founding a robust structural network.
5.2 Water and fat holding capacity
The oil/fat or water holding capacity is described as the total amount of fat or water absorbed per gram of peptide/protein due to the hydrophobic or hydrophilic nature of peptides or protein in the food matrix when interacting with oil and water. Therefore, water retaining capacity is an important indicator for the prediction of loss of moisture demonstrating whether protein isolates or peptides can be added to certain food products whereas, fat/oil holding capacity signifies the hydrophobic potential of peptides or protein isolates (Ucak et al., 2021). The capability of holding water and oil/fat is one of the crucial attributes for application in food, as this can directly influence the texture and taste of the food products. Görgüç et al. (2020) stated that the interaction of peptides and hydrocarbon chains of lipids occurs by binding to the non-polar side chain of amino acids present on peptides and lipids, thus exhibiting increased oil binding capacity. The factors that influence or affect the water-holding capacity of peptides are the presence of hydrophilic and hydrophobic groups, molecular size, and the profile of amino acids present in the protein/peptides (Nuñez et al., 2021). They have reported that peptides consisting of a high ratio (>75%) of specific amino acids, i.e., Lys, Asn, Thr, Gln, Ser, Tyr, Asp., His, Ser, Arg, and Glu, mostly contribute to the water holding capacity. Smaller peptides are mostly hydrophilic, thus, in comparison to larger-size peptide fragments, lower- molecular-weight peptides showed better water-holding capacity. Jin et al. (2020) reported walnut protein hydrolysate prepared using Alcalsase showed improved hydrophobicity and dispersibility with a reduction in the molecular weight of the hydrolysate. This significantly increased the foaming stability by 42.56%, the emulsifying capacity by 59%, and the overall oil and water holding capacity of the hydrolysates. In the same way, Yang et al. (2017) demonstrated improved functional properties such as oil-holding capacity, water-holding capacity, and high emulsifying capacity of PPDP when pine nut protein was hydrolyzed using Protamex. PPDP can bind to the oil/water interface and therefore can act as a natural emulsifier by forming a layer to protect air or oil droplets and stabilizing the water/oil interface due to the amphiphilic properties of embedded amino acids (Yesiltas et al., 2021). Previous findings stated that PPDP produced from potato protein, walnut protein, faba bean proteins, soybean protein, cereal seeds, and flaxseed proteins, have displayed emulsifying properties (Cui et al., 2020; Görgüç et al., 2020). Yesiltas et al. (2021) demonstrated the impact of protein conformation on the functional properties of PPDP. They mentioned that the β-strand conformation of peptides with shorter lengths showed better emulsifying activity, whereas α-helical conformation exhibited better emulsifying properties when amino acid numbers were sufficient to make a helical structure. In addition to this, Cui et al. (2020) reported that utilizing the high hydrostatic pressure treatment during the processing of peptides from sweet potatoes could enhance the emulsifying activity by inducing the molecular flexibility of the peptide. It is concluded that higher conformational flexibility of protein/peptide can result in easier adsorption and structural rearrangement at the oil/water interface layer, thus enhancing the process of emulsification.
6 Biological activities of PDP
PPDP has demonstrated various biological properties such as antihypertensive (Wang et al., 2019), hypocholesterolemia immunomodulatory, anti-inflammatory (Maestri et al., 2016), antioxidant (Durand et al., 2021), antimicrobial (Tkaczewska, 2020), anti-diabetic (Wang J. et al., 2020), hepatoprotective (Kaneko, 2021), opiate (Maestri et al., 2016), appetite regulatory (Kaneko, 2021), and memory and cognitive enhancement (Katayama et al., 2021), have been attributed to bioactive peptides. The following sections elucidate the biological activities of several plant protein-derived peptides (Table 3).
6.1 Antimicrobial activity
The World Health Organization considers antibiotic resistance to be one of the three most significant worldwide public health issues of the 21st century (Mwangi et al., 2019). Antimicrobial peptides (AMPs) are regarded as a significant treatment option for multidrug-resistant microorganisms. AMPs, commonly known as peptide antibiotics, with a wide spectrum of antimicrobial activity. These peptides play an important role in the host’s innate immunological defense mechanism against external pathogens and have been shown to have a broader antibacterial action (Han et al., 2021). These peptides damage microbial membranes and block protein and nucleic acid production through mechanisms that involve interactions with the microbial cell membrane (Valdez-Miramontes et al., 2021). Electrostatic attraction between positively charged antimicrobial peptides and negatively charged phospholipids could lead to their accumulation at the surface membrane of the pathogen, where they could form channels or pores in the membrane or solubilize the membrane into micellar structures (Lee et al., 2016; Valdez-Miramontes et al., 2021). It is therefore plausible to postulate that the electrostatic interaction between the peptide and bacterial membrane is enhanced through an increase in the net positive charge of antimicrobial peptides, which will increase antibacterial activity (Liscano et al., 2019). Antimicrobial peptides are usually found to be high in certain hydrophobic amino acids (such as phenylalanine, tryptophan, leucine, isoleucine, and valine), as well as certain cationic amino acids with net charges between +2 and + 9 (Yang et al., 2021). Antimicrobial peptides have been isolated and identified from a wide range of plant sources in various studies. Certain antifungal peptides from plant proteins have been tested to be effective against human pathogens (Mani-López et al., 2021). For example, 12 different bioactive peptides identified in soybean meal protein prevented the growth of foodborne pathogens (both gram-positive and gram-negative) (Freitas et al., 2019). Moreover, Heymich et al. (2021) reported novel antimicrobial peptides (Leg 1 and Leg 2) from chickpea protein hydrolyzed by chymotrypsin that are effective against 16 different bacteria, including spoilage-causing, pathogenic, and antibiotic-resistant strains. Likewise, peptide HVLDTPLL, isolated from Moringa oleifera seed protein hydrolysates, demonstrated an inhibitory effect against S. aureus, where the major inhibitory activity was due to the interaction of the peptide with dihydrofolate reductase and DNA gyrase via hydrophobic and hydrogen bond interactions (Zhao et al., 2022). Additionally, for detailed production, isolation, and mechanism of action of PPDP please refer to Rivero-Pino et al. (2023).
6.2 Antioxidant activity
Plant-derived peptides with antioxidant activity are an excellent all-natural way to boost your immune system, prevent tissue damage from reactive oxygen species, and lower your risk of developing chronic conditions. Antioxidant peptides neutralize free radicals in several ways, including by donating protons and/or electrons (Görgüç et al., 2020), blocking lipid peroxidation activities, chelating metal ions, and stimulating the body’s natural antioxidant defense system (Wen et al., 2020). The antioxidant activity of peptides has been linked with the particular amino acids present in the peptide sequence that have excellent radical scavenger properties, including phenylalanine, histidine, cysteine, methionine, lysine, proline, tyrosine, isoleucine, threonine, alanine, tryptophan, and leucine (Fan et al., 2022). In vivo, the regulation of antioxidant pathways (NF-κB, Keap1-Nrf2-ARE, mitogen-activated protein kinase, and so on) is signaled by ROS, and plant protein-derived peptides can regulate these signaling pathways (Lv et al., 2022). For instance, peptides TWLPLPR, KVPPLLY, and YVLLPSPK from walnut protein minimized ROS production and elevated glutathione peroxidase activity (Zhao et al., 2020). Novel peptides from cereals and legumes, including rice bran, maize, wheat, barley, oats, rye, cawpwa, chickpeas, mung beans, and so forth, have been identified earlier. Studies have also discovered novel peptides from other plant-based protein sources, including potatoes (García-Moreno et al., 2021), cowpea (Gómez et al., 2021), amaranth sprouts (Osuna-Ruíz et al., 2023), hemp bran protein (Samaei et al., 2021), and spinach rubisco (Marinaccio et al., 2023). Hemp bran protein-based peptides such as LLY, LLR, IR, TY, and so on are reported to have excellent free radical scavenging activity (Samaei et al., 2021). Thus, plant protein-derived peptides could serve as antioxidants that can prevent cellular damage from ROS and free ions. A concise and scrutinized review of antioxidant peptides derived from plant protein including isolation, purification, identification, and mechanism of action has been published by Wen et al. (2020).
6.3 Antihypertensive activity
Problems related to organ dysfunction can be caused by hypertension, such as ischemic stroke, cardiovascular disease, renal disease, and atherosclerosis (Lee and Hur, 2017). Angiotensin-converting enzyme (ACE) inhibition is thought to be the primary mechanism underlying the blood pressure-lowering effects of several drugs, including those that affect the renin-angiotensin system (Yu et al., 2021). ACE, a dipeptidyl carboxypeptidase, is responsible for converting the decapeptide angiotensin I into the octapeptide angiotensin II. It acts upon sympathetic nerves, blood vessels, and adrenal glands, and increases blood pressure. As a result, inhibiting ACE is targeted for hypertension treatment. High bioavailability and a lack of negative effects make ACE-inhibitory peptides from plant-protein an attractive alternative (Aluko, 2015; Shobako, 2021).
Peptide structure, such as chain length, peptide content, and sequences, has been shown to have a major impact on ACE inhibitory activity. ACE inhibitory peptides are often tiny, ranging in length from 2 to 12 amino acids, and have a hydrophobic or positively charged residue at the end (Kaur et al., 2020; Pavlicevic et al., 2022). The bioactive peptide’s ability to inhibit ACE is enhanced by the presence of an aromatic amino acid at its carboxyl terminus. The vaso-protective action of PPDP is achieved by reducing the concentration of intracellular Ca2+ in smooth muscle cells of blood vessels (McClean et al., 2014), which inhibits membrane channel activity and interferes with certain signaling pathways. They are especially prevalent in pulse-based food products produced by protease digestion. The protease P hydrolysates of soybeans yielded a peptide, VLIVP, with more ACE inhibitory action than the peptides IPP and VPP from milk (Puchalska et al., 2014). Hydrolysate produced by hydrolyzing the peanut flour with Alcalase exhibited strong ACE-inhibition, with the peptide fractions less than 5 kDa displaying greater ACE-inhibition than the crude hydrolysate (Yu et al., 2021). Strong ACE inhibitory action was observed in a peptide ITP obtained from sweet potato protein digest. Mushrooms are high in protein and could be a useful source of antihypertensive peptides. Out of 18 new peptides isolated from oyster mushroom protein hydrolysate, ASPYAFGL was shown to possess the strongest ACE-inhibitory activity (Zhang et al., 2017). Similarly, The IC50 values for inhibiting angiotensin-I converting enzyme activity were determined to be 56 and 120 μg/mL, respectively, for pepsin and pancreatin digest of spinach leaf protein. Oral treatment of either leaf protein digest to spontaneously hypertensive rats (SHR) resulted in antihypertensive effects at dosages of 0.25 and 0.5 μg/kg, respectively (Sosalagere et al., 2022). Combining LC-ESI-Q-TOF and LC-ESI-Q-TRAP allowed for the isolation of antihypertensive peptides in maize (Rizzello et al., 2016). A number of ACE-inhibiting bioactive peptides have been identified from plant foods, including garlic, sesame, broccoli, sea weeds, wheat germ, barley, corn, mushrooms, etc. The antihypertensive peptides have also been obtained from cereals and pulses (Saleh et al., 2016).
6.4 Hypo-cholesterolemic activity
Numerous plant-derived bioactive peptides can reduce the levels of lipids and cholesterol in the blood. The processes through which plant-derived peptides exert their hypo-cholesterolemic action have emerged in recent years. Some of these mechanisms include the regulation of lipid metabolism in the liver (Nagaoka et al., 2021), the activation of the expression of genes encoding low-density lipoprotein (LDL) receptors in the liver (Udenigwe and Aluko, 2012), the interaction of plant-derived bioactive peptides with bile acids and cholesterol in the gut, and their mediated effects on cholesterol receptors and hormones (Maestri et al., 2016). The hydrophobic residues (including tryptophan, tyrosine, and leucine in soystatin) are the most functionally significant in these peptides. Hydrophobic residues can interact with lipids and hydrophobic portions of bile compounds. In contrast, hydrophilic peptides can impede the activity of numerous biosynthetic enzymes. Bioactive peptides generated from the amaranth protein exhibited a reduction of cholesterol levels in the blood by suppressing the actions of pancreatic lipase and cholesterol esterase (Ajayi et al., 2021). Similarly, chia protein hydrolysate-derived peptides with molecular masses below 3 kDa exhibited hypo-cholesterolemic action (Coelho et al., 2018). Hydrolyzed cowpea protein was used by Marques et al. (2015) to isolate and identify the hypo-cholesterolemic peptides GCTLN. Cowpea bioactive peptide was found to reduce cholesterol by inhibiting HMG-CoA reductase activity and decreasing cholesterol micellar solubilization. Hydrophobic amino acids (such as tryptophan, tyrosine, and leucine) were found to be crucial to bind bile acids and cholesterol (Boachie et al., 2018). Similarly, methionine-to-glycine and lysine-to-arginine ratios are often lower in peptides with significant hypo-cholesterolemic action (Fan et al., 2022). Soy protein contains the hypo-cholesterolemic peptides IAVPTGVA, LPYP, and IAVPGEVA, which have been shown to influence cholesterol metabolism in HepG2 cells by inhibiting the pathway of a key enzyme (3-hydroxy-3-methylglutaryl coenzyme A reductase, HMG-CoA reductase) (Aiello et al., 2018). The US FDA’s acceptance of the claim that soy-based foods lessen the risk of cardiovascular disease attests to the fact that a diet rich in soybean protein has long been known to lower the blood content of LDLs (Girgih et al., 2013).
6.5 Immunomodulatory activity
The immunomodulatory peptides are involved in the host’s defense response without interacting with the pathogen. The role of the gastrointestinal system in the immunological response of the body increases the significance of dietary peptides in immunomodulation (Wittkopf et al., 2014). The inflammatory process is at the heart of diseases such as cardiovascular disease as well as several types of cancers, making anti-inflammatory peptides a promising therapeutic target (Chakrabarti et al., 2014). The immunomodulatory action of bioactive peptides is associated with peptide sequence, amino acid composition, charge, hydrophobicity, and molecular weight, according to the scientific literature. A study discovered that immunomodulatory peptides have an increase proportion of positively charged and hydrophobic (aliphatic and aromatic) amino acid residues (Pavlicevic et al., 2022). While a positive charge may enable peptides to operate like chemokines, like that described for defensins, hydrophobicity enables peptides to interact with nonpolar cell membrane components, which ultimately affects signaling pathways (Fan et al., 2022). Soybean lunasin, a peptide consisting of 43 amino acids, was shown to have multiple immunomodulatory activities. These included regulating gene expression in NK cells, inhibiting inflammation by suppressing the NF- κB pathway, and preventing cell transformation induced by carcinogens and viral oncogenes (Singh et al., 2014). Similarly, another study concluded that 46 out of 51 soybean protein hydrolysate-derived peptides demonstrated immunomodulatory properties (Wen et al., 2021). As inflammation also plays a significant role in a wide variety of human disorders, inflammation-associated immunomodulatory action of plant-derived protein hydrolysates or peptides has also garnered a lot of interest recently. The anti-inflammatory peptides IIGGAL, FLPPVTSMQ, and PPYLSP were all discovered and extracted from zein hydrolysate (Liang Q. et al., 2018).
6.6 Other biological activities
Many plant-based bioactive peptides have vital physiological functions beyond those already stated, including anti-diabetic, and anticancer properties, hepatoprotective, appetite management, and metal-binding activities. Peptides from several plants such as walnuts, rice bran, soybeans, quinoa, rapeseed, and flaxseed have been reported to have anti-diabetic potential. Based on its inhibitory impact on α-amylase and α-glucosidase, a new anti-diabetic peptide (LPLLR) was isolated from walnut protein hydrolysates and characterized (Wang J. et al., 2020). LPLLR’s antidiabetic mechanism involved boosting glucose uptake, glycogen synthesis, and lowering gluconeogenesis by activating the IRS-1/PI3K/Akt and AMPK insulin signal pathways.
Cancer cells due to their ability to resist growth-inhibiting signals are known for their ability to proliferate and evade apoptosis. Bodily immune boost, necrosis initiation, and the suppression of angiogenesis are some of the potential mechanisms through which bioactive peptides achieve their anti-proliferative actions (Sharma et al., 2019). Bioactive peptides not only alert the immune system but also encourage it to display and express tumor cell surface antigens (Ortiz-Martinez et al., 2014). A recent study used Thermolysin to extract a peptide (LLPSY) from olive seeds. The peptide LLPSY has an IC50 value of 23.6 μg/mL and a molecular weight of 591.33 Da. It was discovered that the peptide has potent anticancer properties (Vásquez-Villanueva et al., 2018). A potent hepatoprotective toward diethylnitrosamine/carbon tetrachloride (CCl4)-induced injury in rats was recently found to be a combination of peptide and exopolysaccharide from Pleurotus ostreatus (Abdel-Monem et al., 2020). Napin is one of the main storage proteins in canola flowers, and its subtilisin digest yielded the bioactive tripeptide Arg-Ile-Tyr (RIY). After oral treatment, RIY inhibited CCK-mediated decreases in food consumption and stomach emptying (Kaneko, 2021).
7 Stability and bioavailability of PDP
The functional and biological activity of PPDP can be influenced by food processing parameters, other food constituents, and the gastrointestinal atmosphere. The sequence of peptides could be modified through different food processing conditions involving alkali treatment, acid treatment, dehydrating, pasteurization, fermentation, and interfering with other food constituents (Piskov et al., 2020). Additionally, some physical and chemical properties of plant peptides, like isoelectric point, hydrophobicity, and hydrophilicity, can influence the application of peptides in the food industry (Sahni et al., 2020). Also, the interaction between PPDP and food constituents needs to be considered in food and nutraceutical formulation. So, it is essential to assess the influence of different conditions (temperature, pH, and other food ingredients) on the bioavailability and bioactivity of plant protein-derived peptides.
7.1 Food processing parameters and stability
7.1.1 Temperature
It was noticed that the antioxidant activity of millet bran peptides was relatively stable at diverse levels of pasteurization involving heating at 63°C for 30 min, 69°C for 30 min, 75°C for 10 min, 80°C for 25 s, and 100°C for 12 s. Furthermore, it was confirmed that peptides like RGLLLPSMSNAP, CFMTY, and CTGTPYC presented constant ABTS+ radical scavenging activity at 100°C for 15 min. However, the ABTS+ radical scavenging activities of CTGTPYC and CFMTY were significantly decreased at 100°C for 30 min (Mirzaei et al., 2020). The peptide structure might cumulate at high temperatures, which has a major effect on peptide solubility. The results confirmed that heating at 100°C for more than 30 min could reduce the solubility of the peptide. Although the solubility of these peptides (RGLLLPSMSNAP, CFMTY, and CTGTPYC) decreased by heating at 100°C for 30 min, it was not significant (Mirzaei et al., 2020). However, Xu et al. (2022) reported that heating at 100°C for 45–60 min significantly reduced the solubility of PPDP. Reduced solubility on heating could be the major reason behind the poor functional and bioactive properties of these peptides due to heat-induced conformational changes.
7.1.2 pH
pH is another parameter that can influence the stability of plant peptides. Plant proteins can be digested in the gastrointestinal tract of humans due to the presence of many enzymes or microbial fermentation and converted into a lot of free amino acids and small peptides. The imitation of this digestion method is a much more beneficial way to determine the stability of bioactive plant peptides. Furthermore, the antioxidant activity of the peptide CFMTY at pH 6.0 did not significantly differ from other pH levels. A parallel result was perceived for other peptides like CTGTPYC, signifying that the antioxidant activity of these peptides was stable at diverse pH levels, while the RGLLLPSMSNAP peptide represented a lower ABTS+ radical scavenging activity at pH 10.0 as compared to other pH levels (Xu et al., 2022). Generally, it was concluded that the antioxidant activity of these above-mentioned peptides was comparatively stable at pH values of 2.0–8.0. The stability of PPDP at various pH ranges depends on the amino acid composition of that peptide.
7.1.3 Other additives
Salt and sugar are regular ingredients in food product development. Their addition to food can also influence the functional properties of nutraceuticals or functional ingredients such as PPDP. This can occur by modifying the polarity of the peptide environment or influencing the biochemical reactions of sugar and peptides at extreme heating (Wong et al., 2019). It was observed that there was no significant difference in the ABTS+ radical scavenging activity of the peptides RGLLLPSMSNAP, CFMTY, and CTGTPYC before and after the usage of sugar (2–6 mg/mL) (Xu et al., 2022). A similar result was noticed for salt (2–4 mg/mL) usage. However, at a salt concentration of 6 mg/mL, the ABTS+ scavenging activities of these peptides significantly decreased, suggesting a high concentration of salt can relatively decrease their antioxidant activity (Xu et al., 2022). Besides this, the phenolic compounds in fruits and vegetables are well known for their binding affinity with proteins or peptides. This protein or peptide phenolic conjugate can influence functional and biological properties either by enhancing the flavor and bioactivity or producing an astringent/bitter compound and reduced bioactivity (Pérez-Gregorio et al., 2020). For detailed molecular interaction between the phenolic compound and protein, their gastrointestinal digestion and health benefits, please refer to Pérez-Gregorio et al. (2020).
7.2 Bioavailability
Bioactive peptides have to control the digestive degradation in the gastrointestinal track to be utilized for physical and biological functions in the body (Ling et al., 2018). After the imitated gastrointestinal digestion of bioactive peptides, it was concluded that the antioxidant activity of CTGTPYC and CFMTY was decreased through gastrointestinal digestion. Consequently, both of these peptides were comparatively resilient to digestion, while RGLLLPSMSNAP was unstable through gastrointestinal digestion. This outcome recommended that RGLLLPSMSNAP be applied for food preservation except for oral administration (Xu et al., 2022). Preceding studies also established that peptides with lengthy chains of amino acids were more vulnerable to digestion in the gastrointestinal track than peptides of short length. This is because long-chain peptides generally have more sites that could be cleaved using digestive enzymes (Mirzaei et al., 2020). The assumption that peptides could not be entirely digested is plausible. Even if they are cleaved, peptides are transferred through the intestine to the blood flow, due to which many studies have manifested the in vitro trans-epithelial transference of peptides. In general, cell line studies are used to demonstrate the bioavailability of the peptides because of their related structural features and comparable reproducibility (Wen et al., 2020). For instance, it was documented that the bioactivity of soybean protein hydrolysate varied during imitated gastrointestinal digestion and transference through the epithelium. The antioxidant activities of soybean protein hydrolysate-gastrointestinal before and after intestinal cancer cell models and cell absorption were examined. It was observed that after intestinal cancer cells and cell absorption of PPDP, the antioxidant activities decreased (Zhang et al., 2018). The bioavailability of wheat germ peptides was also examined after transferring through intestinal cancer cells, and it was confirmed that all peptides had worthy absorption properties (Zhang et al., 2019). However, more clinical investigation on the bioavailability of PPDP is required, which could be useful for the peptide’s application in the development and advancement of functional foods.
Certain bioactive peptides are liable for degradation as they are moderately fragile and can degrade in the gastrointestinal environment, so they could not perform their biological activities very well (Wang et al., 2021). However, such issues can be resolved through either chemical modification and/or encapsulation in a nanocarrier-based delivery system. Peptide encapsulation protects the functional and bioactive properties of peptides from adverse environments throughout their handling, storage, and consumption (Koirala et al., 2023). For instance, walnut plant peptides encapsulated in a liposome carrier had greater antioxidant activity and stability against environmental stress compared to those without encapsulated peptides (Wang et al., 2022). There is another strategy known as peptide cyclization, which produces tolerance in plant peptides against high temperatures and gastrointestinal environments. Peptides on cyclization possess greater stability as compared to linear plant peptides, and such peptides are commonly molded with an N-terminal amide link that forms the ring configuration with a C-terminal carboxylic acid. Consequently, the C-terminal and N-terminal ends of the peptide chain were concealed, eluding the degradation by protease enzymes that specially identify terminal amino and carboxylic acids (Zhang C. et al., 2020).
8 Health benefits of PPDP
Currently, bioactive peptides produced from both animal and plant-sourced proteins are getting medical attention because of increasing scientific proof of their health-improving capacities (Figure 4). Bioactive peptides isolated from plants, animals, marine life, and advanced terrestrial entities have a wide range of health benefits and are comparatively eco-friendly and safe (Zaky et al., 2022; Koirala et al., 2023). Specifically, plant or botanical foodstuffs such as rice, wheat, soybean, soy, sorghum, pumpkin, hemp, amaranth, millet, and so on are potential sources of bioactive peptides. Plant protein-derived peptides have potent antioxidant, antimicrobial, antidiabetic, antihypertensive, antitumor, immunomodulatory, and antidementia properties (Majid and Poornima Priyadarshini, 2020; Yuan et al., 2022). The bioactivities of peptides are influenced by the composition of amino acids, hydrophobicity, sequence configuration, length, and charge of the peptides (Chalamaiah et al., 2019).
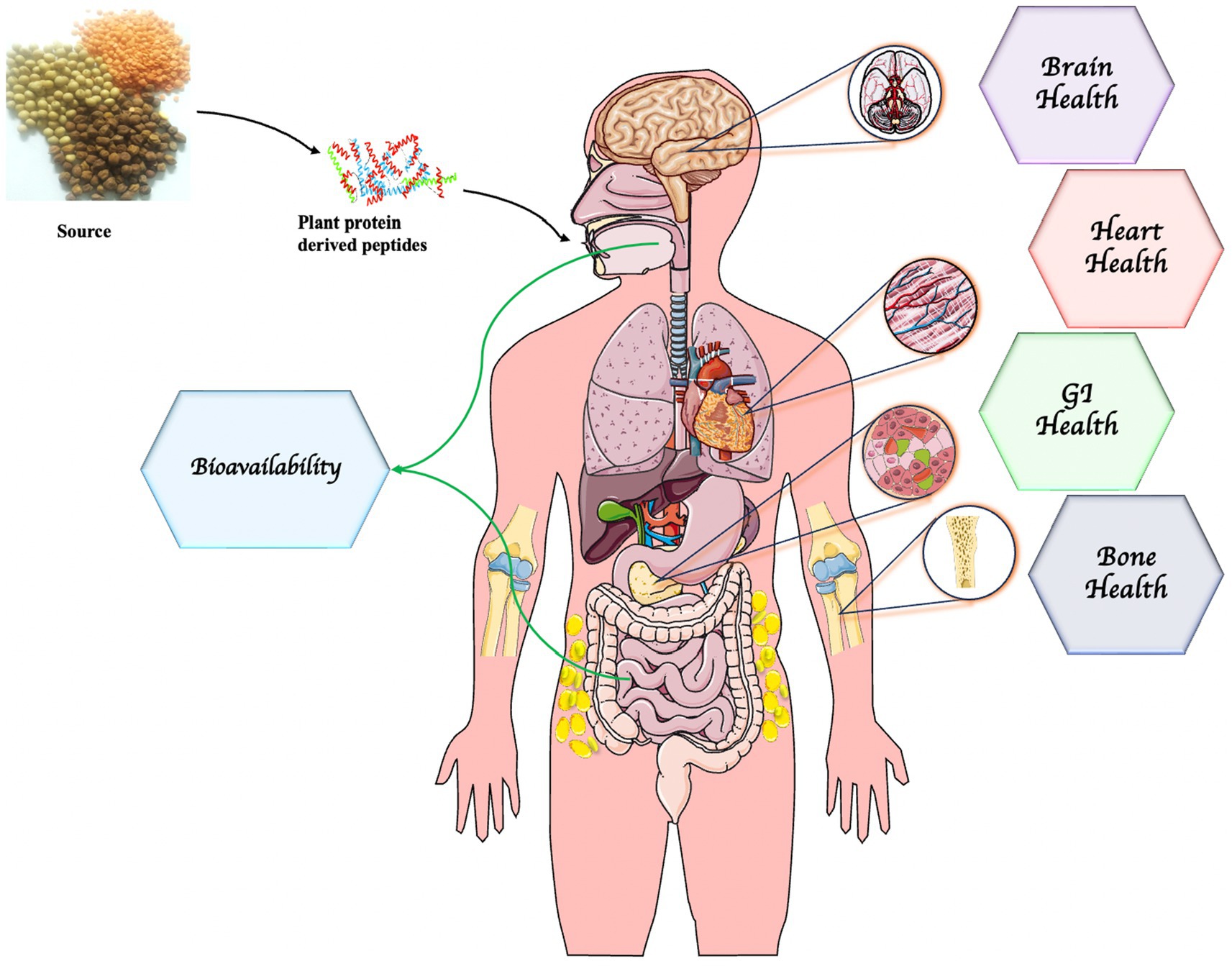
Figure 4. Health promoting ability of plant protein-derived peptides. Parts of the figure were drawn by using pictures from Servier Medical Art. Servier Medical Art by Servier is licensed under a Creative Commons Attribution 3.0 Unported License (https://creativecommons.org/licenses/by/3.0/).
8.1 Blood sugar control
Diabetes mellitus, an assembly of metabolic diseases described by insistent hyperglycemia, is a severe and long-lasting illness that affects the lives and health of people all over the world (Saeedi et al., 2019). There are two major categories of diabetes: type 1, which is also known as insulin-dependent diabetes mellitus, and type 2, which is known as non-insulin-dependent diabetes mellitus. In type 1 diabetes mellitus, pancreatic cells cannot produce enough insulin because of a genetic syndrome or an autoimmune reaction. Type 2 diabetes mellitus, which affects 90% of overall diabetic patients, occurs because of the collective influence of insulin resistance and inadequate secretion of insulin (Axelsson et al., 2017). Type 2 diabetes is also diligently linked to obesity; the occurrence and harshness of diabetes are mostly evident in obese people. Unrestrained hyperglycemia harms the vascular system and upsurges the threat of heart disease, kidney damage, and retinal sickness. Some plant peptides such as quinoa seeds, cumin seeds, walnuts, black beans, soybeans, lupin beans, etc. reported to cure diabetes mellitus (Gong et al., 2022). These seeds help to cure high-risk patients of diabetes, obesity, and dyslipidemia, because of their rich protein and nutrient content, gluten-free eminence, and therapeutic properties (Bakhtavar and Afzal, 2020). The major enzymes that are involved in controlling blood sugar levels are dipeptidyl peptidase-IV (DPP-IV), α-glucosidase, and α-amylase. Plant peptides that can stop the actions of these enzymes are efficient approaches for the controlling and managing of type 2 diabetes. For instance, a few α-amylase inhibitory peptides have been detected in cumin seeds (Siow and Gan, 2016; Siow et al., 2017) and Pinto bean (Ngoh and Gan, 2018). Walnut protein-derived peptide LPLLR also presented inhibitory activity for α-amylase and improved resistance of insulin against HepG2 cells (Wang J. et al., 2020). The peptides FVVAEQAGNEEGFE and INEGSLLLPH with a molecular weight of 3.5–7 kDa, obtained from the fermentation of beans had α-amylase inhibitory activity (Jakubczyk et al., 2017). Peptides obtained from Lupin bean (IAVPTGVA, LTFPGSAED) have been confirmed to stop DPP-IV activity (Lammi et al., 2018). As well Quinoa plant peptides including DKKYPK, GEHGSDGNV, and IQAEGGL, have strong DPP-IV, and inhibitory activities of α-amylase and α-glucosidase (Vilcacundo et al., 2017).
In humans, consumption of soybean peptides has been confirmed to decrease blood sugar levels (Kashima et al., 2016). Additionally, it was experienced through a model of diabetic mice that consumption of Soymorphin-5 (YPFVV) peptide obtained from the soybean β-conglycinin with water, can decrease blood sugar levels in mice. It has been proposed that the reduction in blood sugar level was related to the stimulation of β-oxidation and the upsurge in energy intake through PPARα and adiponectin (Yamada et al., 2012). As glucose is not capable of crossing the membrane lipid bilayer, glucose movement through SGLT and GLUT transporters occurs. SGLT transporters are used for the positive movement of glucose using energy and GLUT transporters use the submissive movement of glucose which is devoid of energy (Patil et al., 2020). So, glucose transporters like SGLT and GLUT are significant targets for the adjustment of diabetes. Peptides obtained from Black beans (AKSPLF, FEELN, ATNPLF, and LSVSVL) decreased the receiving of glucose in Caco-2 cells. Feeding of Black bean peptides showed a reduction in postprandial glucose in rats (Mojica et al., 2017). Likewise, α-glucosidase inhibition activity of soya protein peptides, WLRL, SWLRL, and LLPLPVLK was observed, and the IC50 was 165.29, 182.05, and 237.43 μmol/L, respectively. Moreover, specific amino acids (Leu and Pro) in peptides have potent α-glucosidase inhibitory activity, and the mode of action is either solely or synergistically (Wang et al., 2019).
8.2 Bone and joint health
Several pathways control the bone systems, foremost the collapse of older bone tissues through osteoclast genesis and then the formation of new bone tissues by osteoblast genesis (Okagu et al., 2022). Uncontrollable bone-related complications like osteoporosis, osteoarthritis, rheumatoid arthritis, bone cancer, and many other bone disorders are instigated by an interruption in these pathways (Barnsley et al., 2021). Although oxidative strain has been related to the development of osteoblasts, vital for bone health, extreme oxidative strain stimulates osteoclast discrepancy while conquering osteoblast genesis (Galliera et al., 2021). Correspondingly, inflammation, which is essential for the remodeling of bones, inclines the bone to compulsive stress and becomes chronic, as observed in postmenopausal women and older men (Xie et al., 2019) because of lesser total antioxidant capability and greater inflammation as compared to healthy people (Epsley et al., 2021). So, targeting oxidative imbalance and inflammation could prevent and/or treat bone diseases. An innovative approach for the hindrance and handling of bone disorders is the usage of nutraceuticals, including bioactive peptides, phytochemicals, polysaccharides, and other phyto-derivatives. Plant peptides that are potentially used to constrain those pathways involved in the origin of bone disorders include proteins from lupine and soybean products (Millan-Linares et al., 2018). Peptides from plants have been used to improve bone health through enhancing mineralization, calcium absorption, and osteoblast discrepancy. These osteomodulatory peptides also stop the degradation of bone by constraining differentiation and persistence of osteoclast while enhancing osteoclast apoptosis (Hou T. et al., 2017). Some studies examined plant-derived peptides for useful impact on bone health. For instance, a peptide GPETAFLR from lupine proved to stop the generation of pro-inflammatory cytokines while enhancing the emission of anti-inflammatory cytokines. GPETAFLR peptide also presented anti-osteoclast genesis by downregulation of gene countenance of pro osteoclast genesis like tartrate-resistant acid phosphatase (TRAP), osteoclast-associated receptor, whereas upregulating the gene countenance of osteo protegerin protein, which stops osteoclast genesis (Millan-Linares et al., 2018, 2019).
8.3 Brain health
Bioactive peptides have been used to cure many chronic disorders, including central nervous system diseases like Parkinson’s, dementia, and Alzheimer’s (Perlikowska, 2021). The blood–brain barrier maintains the central nervous system by permitting the careful permeation of constituents between the blood and parenchyma of the brain, which involves mostly microvascular endothelial cells, astrocytes, and pericytes (Xiong et al., 2021). Tight connections are necessary on the endothelial cell membrane to sustain the function of the blood–brain barrier. Some important proteins that are involved in the tight connections framework known as zonula occludin-1, occluding, and Claudin-5 are used to control the paracellular pathway by joining nearby endothelial cells (Greene et al., 2020). It was noticed in previous studies that deposition of both Aβ and inflammation disturb the structure and function of tight connections to upsurge the native penetrability of the blood–brain barrier, which causes many nervous system syndromes, including vascular dementia, multiple sclerosis, Alzheimer’s disease, and traumatic brain injury. Over 90 percent of Alzheimer’s disease patients demonstrate disruption of the blood–brain barrier in the Para hippocampal gyrus and hippocampus, which leads to neuronal damage (Muszyński et al., 2017). So, it was perceived that disruption of the blood–brain barrier could be an initial biomarker for Alzheimer’s disease. There were some studies done on the shielding of the blood–brain barrier using plant peptides. Peptides from walnuts have been broadly studied for having neuroprotective aspects that impact memory and learning. Literature suggests that walnut peptides are exceptional antioxidants with the capability to improve mental insufficiency in mice (Ren et al., 2018). Successively, walnut peptides were also used to shield neurons by rummaging reactive oxygen species, encouraging cell autophagy, and guarding mitochondrial functions (Yang et al., 2022). Implementation of plant peptides from fermented rice and soybean protein hydrolysate presented inhibition of memory damage in mice models with dementia. This outcome was reliant on the stimulation of neurotrophic factors like NGF, BDNF, and NT-3 through the instigation of the transcription factor CREB (Shimizu et al., 2018; Corpuz et al., 2019). It was noticed that the bioactivity of walnut peptides showed improved efficacy when used with tea polyphenols as compared to when used alone, signifying improved penetration on the blood–brain barrier (Sheng et al., 2020). Soybean peptides have been used to lessen age-linked mental weakness; their anti-inflammatory and antioxidant activities have useful effects on Alzheimer’s and Parkinson’s disease. Additionally, soy-deprestatin peptide has antidepressant effects merely when used orally, not intraperitoneally (Mori et al., 2018).
8.4 Heart health
Hypertension is a worldwide problem and is one of the major inclining reasons for cardiovascular disease (Quiroga et al., 2017). Renin and angiotensin-converting enzyme inhibitors are mainly used to control blood pressure in the human body. Bestowing European commendations for the management of hypertension, functional foods manufactured from plant peptides are superb aspirants to inhibit angiotensin-converting enzyme and renin (Quiroga et al., 2017). High content of the ACE inhibitory peptide (ASPYAFGL) was isolated from five different species of mushrooms, including straw, enoki, wood ear, oyster, and shiitake mushrooms (Zhang et al., 2017). The inhibition of angiotensin-converting enzyme by plant peptides has been established to decrease high blood pressure and minimize or stop cardiovascular syndrome (Saleh et al., 2016). These ACE-inhibitory plant peptides have a short sequence of amino acids, and they consist of positively charged deposits at the C-terminal (Kaur et al., 2020). The C-terminal of plant peptides contains an aromatic amino acid, which enhances their ability to block the angiotensin-converting enzyme (Maestri et al., 2016). Several ACE inhibitory peptides have been derived from plants, including wheat germ, corn, barley, sesame, garlic, mushrooms, sea weeds, broccoli, and so forth. Thrombosis is also a major cause of cardiovascular diseases; therefore, in recent years, natural food constituents that have anti-thrombogenic characteristics have remained a concern. Amaranth plant peptides have strong antithrombotic activity (Sabbione et al., 2018). Albumins and glutelins derived from the amaranth plant were known as inhibitors of thrombus formation (Sabbione et al., 2015). Buckwheat peptides in addition to amaranth, also have antithrombotic action (Sabbione et al., 2016). It was found that albumin and glutelin from buckwheat peptides showed antiplatelet activity as well (Yu et al., 2016).
8.5 Other
Antimicrobial peptides are naturally found in all living organisms and are used for resistance against several pathogens like bacteria, viruses, fungi, and nematodes. Antimicrobial peptides generally have a lower molecular weight, a positive net charge, and a high amount of hydrophobic amino acid remnants (Huan et al., 2020). These are found in plants as a vital part of inherent immunity, acting like a former line of protection against many pathogenic infections. Antimicrobial peptides are widely occurring in plants, and because of their identification in each part of plants, they are instantly accessible at any infection site, while others are merely available in particular situations (Roudi et al., 2017; Li et al., 2021). Antimicrobial peptides derived from plants have been considered in medication as potential therapeutic mediators for infection control. These peptides are useful in wound healing procedures due to their role in modulating immune reactions. Additionally, these are also efficient for monitoring cell propagation using anti-tumor activities (Roudi et al., 2017).
Cancer is known as a major cause of death in the established world. Though the causes of cancer are complicated and change among diverse populations, it is widely recognized that bad eating habits are significant in generating cancer. So dietary changes are becoming a potent adjunct to the conventional treatment of cancer (Kanarek et al., 2020). Excitingly, some natural foods are used to guard against cancer development. Some pseudo-cereals like buckwheat, amaranth, and quinoa are recognized to have both medicinal and functional food properties, with the presence of bioactive peptides that inhibit carcinogenesis or have anti-cancer activities (Graf et al., 2015). Inflammation is produced through immune reactions in our body, which shield us from injured cells, viruses, and some other harmful stimulation. There are two types of inflammation: one is short-lived, known as acute inflammation, and the other is systemic inflammation which remains for months or years, known as chronic inflammation. Chronic inflammation might be the cause of major metabolic disorders involving diabetes, coronary artery disease, Alzheimer’s disease, and many others (Knight-Sepulveda et al., 2015). It was reported that whole quinoa protein and amaranth protein had anti-inflammatory properties. Sixteen bioactive peptides from amaranth protein peptides were further recognized as having the best anti-inflammatory capacity (Moronta et al., 2016; Shi et al., 2019). Aging is a natural biological phenomenon that becomes a communal source of anxiety for people of various ages. There can be an accumulation of Metabolic waste as a result of functional modifications and metabolic imbalance; oxidative pressure produced due to dysfunction of mitochondria and incomplete cell division are known as potential reasons for driving age (Santos and Sinha, 2021). The anxiety of aging has prompted continuous research for medicines and bioactive compounds to postpone aging, so plant peptides have gained much attention because of their outstanding anti-aging activity. For instance, buckwheat-derived polypeptides like trypsin inhibitors have been presented as anti-aging mediators (Li et al., 2019, 2020).
9 Conclusion and future perspective
Plant-based proteins and peptides are extensively studied for their functional and biological properties. This review attempted to provide a comprehensive summarization of the plant protein sources to end-product applications. A wide range of peptides are isolated from novel plant protein sources, where scientists are inclined to isolate the peptides with potent biological activities. The biotechnological approach including microbial fermentation and enzymatic hydrolysis are reported to be green non-thermal techniques. However, a large number of studies are yet to be conducted to obtain such peptides from novel plant protein sources. Herein, enzymatic hydrolysis is the most preferred method, as target-specific peptides can be harvested and can enhance the yield of such peptides. Nevertheless, the selection of the enzyme is crucial to the adoption of this approach. Furthermore, PPDP is reported for various functional properties such as water-holding properties, foaming, and gelling. Yet, the application of such peptides in the food and pharmaceutical industries is critical, and further research into the application of such functional peptides is essential. Stability and interaction with the food matrix, on the other hand, are the issues of concern. Recent advancements in encapsulation and nanotechnologies could have resolved these issues; however, only limited research is available in the area. Apart from this, peptides isolated from plant proteins showed excellent biological and disease-healing functions. Specifically, peptides from plant sources are effective in preventing and/or treating chronic diseases such as hypertension, diabetes, cardiovascular-related issues, etc., However, more human clinical studies are warranted to confirm the health benefits of PPDP.
Hence future research should focus on the effective extraction, isolation, and purification at large scale. Further, optimization and standardization of the system are crucial to obtaining a consistent product. When considering the applications of PPDP as a functional food ingredient, the stability and the interaction of PPDP with other food components must be assessed to ensure the final benefit of PPDP. The sensory properties of isolated PPDP or final product should be considered. Also, to retain the bioactivity of PPDP, a targeted controlled delivery system should be developed using a nanotechnology approach or chemical modification. In addition, a clear understanding of the molecular mechanism in vivo and the pharmacokinetics of PPDP should be determined. Last but not least, new approaches including molecular docking and in-silicon prediction should be implemented to identify target receptors or binding sites thereby evaluating structure–activity relationships.
Author contributions
NN: Conceptualization, Project administration, Supervision, Validation, Writing – original draft, Writing – review & editing. AK: Data curation, Formal analysis, Writing – original draft. KS: Data curation, Formal analysis, Writing – original draft. NA: Data curation, Writing – original draft. KSB: Data curation, Investigation, Writing – original draft. İU: Data curation, Investigation, Visualization, Writing – original draft. MA: Data curation, Formal analysis, Writing – original draft. AH: Data curation, Writing – original draft, Writing – review & editing. AT: Data curation, Writing – original draft.
Funding
The author(s) declare financial support was received for the research, authorship, and/or publication of this article. This research work was supported by the Mahidol University, Thailand.
Conflict of interest
KS and KB were employed by Sargento Foods. NA was employed by Lactalis USA.
The remaining authors declare that the research was conducted in the absence of any commercial or financial relationships that could be construed as a potential conflict of interest.
Publisher’s note
All claims expressed in this article are solely those of the authors and do not necessarily represent those of their affiliated organizations, or those of the publisher, the editors and the reviewers. Any product that may be evaluated in this article, or claim that may be made by its manufacturer, is not guaranteed or endorsed by the publisher.
References
Abdel-Monem, N. M., El-Saadani, M. A., Daba, A. S., Saleh, S. R., and Aleem, E. (2020). Exopolysaccharide-peptide complex from oyster mushroom (Pleurotus ostreatus) protects against hepatotoxicity in rats. Biochem. Biophys. Rep. 24:100852. doi: 10.1016/j.bbrep.2020.100852
Acquah, C., Di Stefano, E., and Udenigwe, C. C. (2018). Role of hydrophobicity in food peptide functionality and bioactivity. J. Food Bioact. 4, 88–98. doi: 10.31665/JFB.2018.4164
Agrawal, H., Joshi, R., and Gupta, M. (2017). Isolation and characterisation of enzymatic hydrolysed peptides with antioxidant activities from green tender sorghum. LWT 84, 608–616. doi: 10.1016/j.lwt.2017.06.036
Aguilar-Toalá, J., Santiago-López, L., Peres, C., Peres, C., Garcia, H., Vallejo-Cordoba, B., et al. (2017). Assessment of multifunctional activity of bioactive peptides derived from fermented milk by specific Lactobacillus plantarum strains. J. Dairy Sci. 100, 65–75. doi: 10.3168/jds.2016-11846
Aiello, G., Ferruzza, S., Ranaldi, G., Sambuy, Y., Arnoldi, A., Vistoli, G., et al. (2018). Behavior of three hypocholesterolemic peptides from soy protein in an intestinal model based on differentiated CaCo-2 cell. J. Funct. Foods 45, 363–370. doi: 10.1016/j.jff.2018.04.023
Ajayi, F. F., Mudgil, P., Gan, C.-Y., and Maqsood, S. (2021). Identification and characterization of cholesterol esterase and lipase inhibitory peptides from amaranth protein hydrolysates. Food Chem X 12:100165. doi: 10.1016/j.fochx.2021.100165
Aluko, R. E. (2015). Structure and function of plant protein-derived antihypertensive peptides. Curr. Opin. Food Sci. 4, 44–50. doi: 10.1016/j.cofs.2015.05.002
Alves, T. O., D’Almeida, C. T., Scherf, K. A., and Ferreira, M. S. (2019). Modern approaches in the identification and quantification of immunogenic peptides in cereals by LC-MS/MS. Front. Plant Sci. 10:1470. doi: 10.3389/fpls.2019.01470
Amagliani, L., O'Regan, J., Kelly, A. L., and O'Mahony, J. A. (2017). The composition, extraction, functionality and applications of rice proteins: a review. Trends Food Sci. Technol. 64, 1–12. doi: 10.1016/j.tifs.2017.01.008
Ambika, A., Aski, M. S., Gayacharan,, Hamwieh, A., Talukdar, A., Kumar Gupta, S., et al. (2022). Unraveling origin, history, genetics, and strategies for accelerated domestication and diversification of food legumes. Front. Genet. 13:932430. doi: 10.3389/fgene.2022.932430
Ashaolu, T. J. (2020). Applications of soy protein hydrolysates in the emerging functional foods: a review. Int. J. Food Sci. Technol. 55, 421–428. doi: 10.1111/ijfs.14380
Axelsson, A. S., Mahdi, T., Nenonen, H. A., Singh, T., Hänzelmann, S., Wendt, A., et al. (2017). Sox5 regulates beta-cell phenotype and is reduced in type 2 diabetes. Nat. Commun. 8:15652. doi: 10.1038/ncomms15652
Ayyash, M., Johnson, S. K., Liu, S.-Q., Mesmari, N., Dahmani, S., Al Dhaheri, A. S., et al. (2019). In vitro investigation of bioactivities of solid-state fermented lupin, quinoa and wheat using Lactobacillus spp. Food Chem. 275, 50–58. doi: 10.1016/j.foodchem.2018.09.031
Bakhtavar, M. A., and Afzal, I. (2020). Climate smart dry chain technology for safe storage of quinoa seeds. Sci. Rep. 10:12554. doi: 10.1038/s41598-020-69190-w
Barnsley, J., Buckland, G., Chan, P. E., Ong, A., Ramos, A. S., Baxter, M., et al. (2021). Pathophysiology and treatment of osteoporosis: challenges for clinical practice in older people. Aging Clin. Exp. Res. 33, 759–773. doi: 10.1007/s40520-021-01817-y
Berraquero-García, C., Almécija, M. C., Guadix, E. M., and Pérez-Gálvez, R. (2022). Valorisation of blood protein from livestock to produce haem iron-fortified hydrolysates with antioxidant activity. Int. J. Food Sci. Technol. 57, 2479–2486. doi: 10.1111/ijfs.15616
Boachie, R., Yao, S., and Udenigwe, C. C. (2018). Molecular mechanisms of cholesterol-lowering peptides derived from food proteins. Curr. Opin. Food Sci. 20, 58–63. doi: 10.1016/j.cofs.2018.03.006
Cavazos, A., and Gonzalez de Mejia, E. (2013). Identification of bioactive peptides from cereal storage proteins and their potential role in prevention of chronic diseases. Compr. Rev. Food Sci. Food Saf. 12, 364–380. doi: 10.1111/1541-4337.12017
Chakrabarti, S., Jahandideh, F., and Wu, J. (2014). Food-derived bioactive peptides on inflammation and oxidative stress. Biomed. Res. Int. 2014, 1–11. doi: 10.1155/2014/608979
Chalamaiah, M., Ulug, S. K., Hong, H., and Wu, J. (2019). Regulatory requirements of bioactive peptides (protein hydrolysates) from food proteins. J. Funct. Foods 58, 123–129. doi: 10.1016/j.jff.2019.04.050
Cian, R. E., Vioque, J., and Drago, S. R. (2015). Structure–mechanism relationship of antioxidant and ACE I inhibitory peptides from wheat gluten hydrolysate fractionated by pH. Food Res. Int. 69, 216–223. doi: 10.1016/j.foodres.2014.12.036
Coelho, M. S., Soares-Freitas, R. A. M., Arêas, J. A. G., Gandra, E. A., and Salas-Mellado, M. D. L. M. (2018). Peptides from chia present antibacterial activity and inhibit cholesterol synthesis. Plant Foods Hum. Nutr. 73, 101–107. doi: 10.1007/s11130-018-0668-z
Corpuz, H. M., Fujii, H., Nakamura, S., and Katayama, S. (2019). Fermented rice peptides attenuate scopolamine-induced memory impairment in mice by regulating neurotrophic signaling pathways in the hippocampus. Brain Res. 1720:146322. doi: 10.1016/j.brainres.2019.146322
Cui, Q., Zhang, A., Li, R., Wang, X., Sun, L., and Jiang, L. J. F. B. (2020). Ultrasonic treatment affects emulsifying properties and molecular flexibility of soybean protein isolate-glucose conjugates. Food Biosci. 38:100747. doi: 10.1016/j.fbio.2020.100747
Cvetkovic, T., Ranilovic, J., and Jokic, S. (2022). Quality of pepper seed by-products: a review. Food Secur. 11:748. doi: 10.3390/foods11050748
Dahal, M., and Koirala, P. (2020). Effect of roasting on bioactive and Antinutritional components of flaxseed (Linum usitatissimum). J. Food Nutr. Disord. 9:278.
Daliri, E. B.-M., Lee, B. H., and Oh, D. H. (2017). Current perspectives on antihypertensive probiotics. Probiot. Antimicrob. Proteins 9, 91–101. doi: 10.1007/s12602-016-9241-y
Dhayakaran, R., Neethirajan, S., and Weng, X. (2016). Investigation of the antimicrobial activity of soy peptides by developing a high throughput drug screening assay. Biochem. Biophys. Rep. 6, 149–157. doi: 10.1016/j.bbrep.2016.04.001
Di Stefano, E., Tsopmo, A., Oliviero, T., Fogliano, V., and Udenigwe, C. C. (2019). Bioprocessing of common pulses changed seed microstructures, and improved dipeptidyl peptidase-IV and α-glucosidase inhibitory activities. Sci. Rep. 9:15308. doi: 10.1038/s41598-019-51547-5
Durand, E., Beaubier, S., Ilic, I., Kapel, R., and Villeneuve, P. (2021). Production and antioxidant capacity of bioactive peptides from plant biomass to counteract lipid oxidation. Curr. Res. Food Sci. 4, 365–397. doi: 10.1016/j.crfs.2021.05.006
Epsley, S., Tadros, S., Farid, A., Kargilis, D., Mehta, S., and Rajapakse, C. S. (2021). The effect of inflammation on bone. Front. Physiol. 11:511799. doi: 10.3389/fphys.2020.511799
Fan, H., Liu, H., Zhang, Y., Zhang, S., Liu, T., and Wang, D. (2022). Review on plant-derived bioactive peptides: biological activities, mechanism of action and utilizations in food development. J. Future Foods 2, 143–159. doi: 10.1016/j.jfutfo.2022.03.003
Felix, M., Cermeño, M., Romero, A., and FitzGerald, R. J. (2019). Characterisation of the bioactive properties and microstructure of chickpea protein-based oil in water emulsions. Food Res. Int. 121, 577–585. doi: 10.1016/j.foodres.2018.12.022
Fernández-López, J., Lucas-González, R., Viuda-Martos, M., Sayas-Barberá, E., Navarro, C., Haros, C. M., et al. (2019). Chia (Salvia hispanica L.) products as ingredients for reformulating frankfurters: effects on quality properties and shelf-life. Meat. Sci. 156, 139–145. doi: 10.1016/j.meatsci.2019.05.028
Fideler, J., Johanningsmeier, S. D., Ekelöf, M., and Muddiman, D. C. (2019). Discovery and quantification of bioactive peptides in fermented cucumber by direct analysis IR-MALDESI mass spectrometry and LC-QQQ-MS. Food Chem. 271, 715–723. doi: 10.1016/j.foodchem.2018.07.187
Freitas, C. S., Vericimo, M. A., da Silva, M. L., da Costa, G. C. V., Pereira, P. R., Paschoalin, V. M. F., et al. (2019). Encrypted antimicrobial and antitumoral peptides recovered from a protein-rich soybean (Glycine max) by-product. J. Funct. Foods 54, 187–198. doi: 10.1016/j.jff.2019.01.024
Galliera, E., Massaccesi, L., Banfi, G., De Vecchi, E., Ragone, V., and Corsi Romanelli, M. M. (2021). Effect of oxidative stress on bone remodeling in Periprosthetic Osteolysis. Clin. Rev. Bone Miner. Metab. 19, 14–23. doi: 10.1007/s12018-021-09278-7
García-Moreno, P. J., Yang, J., Gregersen, S., Jones, N. C., Berton-Carabin, C. C., Sagis, L. M., et al. (2021). The structure, viscoelasticity and charge of potato peptides adsorbed at the oil-water interface determine the physicochemical stability of fish oil-in-water emulsions. Food Hydrocoll. 115:106605. doi: 10.1016/j.foodhyd.2021.106605
Girgih, A., Myrie, S., Aluko, R., and Jones, P. (2013). Is category ‘A’ status assigned to soy protein and coronary heart disease risk reduction health claim by the United States Food and Drug Administration still justifiable? Trends Food Sci. Technol. 30, 121–132. doi: 10.1016/j.tifs.2012.12.003
Girgih, A. T., He, R., Malomo, S., Offengenden, M., Wu, J., and Aluko, R. E. (2014). Structural and functional characterization of hemp seed (Cannabis sativa L.) protein-derived antioxidant and antihypertensive peptides. J. Funct. Foods 6, 384–394. doi: 10.1016/j.jff.2013.11.005
Gómez, A., Gay, C., Tironi, V., and Avanza, M. V. (2021). Structural and antioxidant properties of cowpea protein hydrolysates. Food Biosci. 41:101074. doi: 10.1016/j.fbio.2021.101074
Gong, X., An, Q., Le, L., Geng, F., Jiang, L., Yan, J., et al. (2022). Prospects of cereal protein-derived bioactive peptides: sources, bioactivities diversity, and production. Crit. Rev. Food Sci. Nutr. 62, 2855–2871. doi: 10.1080/10408398.2020.1860897
Görgüç, A., Gençdağ, E., and Yılmaz, F. M. (2020). Bioactive peptides derived from plant origin by-products: biological activities and techno-functional utilizations in food developments–a review. Food Res. Int. 136:109504. doi: 10.1016/j.foodres.2020.109504
Graf, B. L., Rojas-Silva, P., Rojo, L. E., Delatorre-Herrera, J., Baldeón, M. E., and Raskin, I. (2015). Innovations in health value and functional food development of quinoa (Chenopodium quinoa Willd.). Compr. Rev. Food Sci. Food Saf. 14, 431–445. doi: 10.1111/1541-4337.12135
Greene, C., Hanley, N., and Campbell, M. (2020). Blood-brain barrier associated tight junction disruption is a hallmark feature of major psychiatric disorders. Transl. Psychiatry 10:373. doi: 10.1038/s41398-020-01054-3
Han, Y., Zhang, M., Lai, R., and Zhang, Z. (2021). Chemical modifications to increase the therapeutic potential of antimicrobial peptides. Peptides 146:170666. doi: 10.1016/j.peptides.2021.170666
Heymich, M.-L., Friedlein, U., Trollmann, M., Schwaiger, K., Böckmann, R. A., and Pischetsrieder, M. (2021). Generation of antimicrobial peptides Leg1 and Leg2 from chickpea storage protein, active against food spoilage bacteria and foodborne pathogens. Food Chem. 347:128917. doi: 10.1016/j.foodchem.2020.128917
Hou, F., Ding, W., Qu, W., Oladejo, A. O., Xiong, F., Zhang, W., et al. (2017). Alkali solution extraction of rice residue protein isolates: influence of alkali concentration on protein functional, structural properties and lysinoalanine formation. Food Chem. 218, 207–215. doi: 10.1016/j.foodchem.2016.09.064
Hou, T., Liu, Y., Kolba, N., Guo, D., and He, H. (2017). Desalted duck egg white peptides promote calcium uptake and modulate bone formation in the retinoic acid-induced bone loss rat and Caco-2 cell model. Nutrients 9:490. doi: 10.3390/nu9050490
Huan, Y., Kong, Q., Mou, H., and Yi, H. (2020). Antimicrobial peptides: classification, design, application and research progress in multiple fields. Front. Microbiol. 11:582779. doi: 10.3389/fmicb.2020.582779
Jakubczyk, A. (2018). Effect of addition of fermented bean seed flour on the content of bioactive components and nutraceutical potential of wheat wafers. LWT 98, 245–251. doi: 10.1016/j.lwt.2018.08.052
Jakubczyk, A., Karaś, M., Złotek, U., and Szymanowska, U. (2017). Identification of potential inhibitory peptides of enzymes involved in the metabolic syndrome obtained by simulated gastrointestinal digestion of fermented bean (Phaseolus vulgaris L.) seeds. Food Res. Int. 100, 489–496. doi: 10.1016/j.foodres.2017.07.046
Jia, J.-Q., Miao, N., Du, J.-J., and Wu, Q.-Y. (2019). Angiotensin-I converting enzyme inhibitory peptides from sweet Sorghum grain protein: optimisation of hydrolysis conditions and hydrolysate characterization. J. Chem. Soc. Pak. 41:175. doi: 10.52568/000723/JCSP/41.01.2019
Jin, F., Wang, Y., Tang, H., Regenstein, J. M., and Wang, F. (2020). Limited hydrolysis of dehulled walnut (Juglans regia L.) proteins using trypsin: functional properties and structural characteristics. LWT 133:110035. doi: 10.1016/j.lwt.2020.110035
Kajla, P., Sharma, A., and Sood, D. R. (2015). Flaxseed-a potential functional food source. J. Food Sci. Technol. 52, 1857–1871. doi: 10.1007/s13197-014-1293-y
Kamani, M. H., Meera, M. S., Bhaskar, N., and Modi, V. K. (2019). Partial and total replacement of meat by plant-based proteins in chicken sausage: evaluation of mechanical, physico-chemical and sensory characteristics. J. Food Sci. Technol. 56, 2660–2669. doi: 10.1007/s13197-019-03754-1
Kanarek, N., Petrova, B., and Sabatini, D. M. (2020). Dietary modifications for enhanced cancer therapy. Nature 579, 507–517. doi: 10.1038/s41586-020-2124-0
Kaneko, K. (2021). Appetite regulation by plant-derived bioactive peptides for promoting health. Peptides 144:170608. doi: 10.1016/j.peptides.2021.170608
Kashima, H., Uemoto, S., Eguchi, K., Endo, M. Y., Miura, A., Kobayashi, T., et al. (2016). Effect of soy protein isolate preload on postprandial glycemic control in healthy humans. Nutrition 32, 965–969. doi: 10.1016/j.nut.2016.02.014
Katayama, S., Corpuz, H. M., and Nakamura, S. (2021). Potential of plant-derived peptides for the improvement of memory and cognitive function. Peptides 142:170571. doi: 10.1016/j.peptides.2021.170571
Kaur, N., Sharma, P., Jaimni, S., Kehinde, B. A., and Kaur, S. (2020). Recent developments in purification techniques and industrial applications for whey valorization: a review. Chem. Eng. Commun. 207, 123–138. doi: 10.1080/00986445.2019.1573169
Kaushik, P., Dowling, K., McKnight, S., Barrow, C. J., Wang, B., and Adhikari, B. (2016). Preparation, characterization and functional properties of flax seed protein isolate. Food Chem. 197, 212–220. doi: 10.1016/j.foodchem.2015.09.106
Knight-Sepulveda, K., Kais, S., Santaolalla, R., and Abreu, M. T. (2015). Diet and inflammatory bowel disease. Gastroent. Hepat. 11, 511–520.
Koirala, P., Dahal, M., Rai, S., Dhakal, M., Nirmal, N. P., Maqsood, S., et al. (2023). Dairy milk protein–derived bioactive peptides: avengers against metabolic syndrome. Curr. Nutr. Rep. 12, 308–326. doi: 10.1007/s13668-023-00472-1
Kontani, N., Omae, R., Kagebayashi, T., Kaneko, K., Yamada, Y., Mizushige, T., et al. (2014). Characterization of Ile-his-Arg-Phe, a novel rice-derived vasorelaxing peptide with hypotensive and anorexigenic activities. Mol. Nutr. Food Res. 58, 359–364. doi: 10.1002/mnfr.201300334
Kumar, M., Tomar, M., Punia, S., Dhakane-Lad, J., Dhumal, S., Changan, S., et al. (2022). Plant-based proteins and their multifaceted industrial applications. LWT 154:112620. doi: 10.1016/J.LWT.2021.112620
Lammi, C., Bollati, C., Ferruzza, S., Ranaldi, G., Sambuy, Y., and Arnoldi, A. (2018). Soybean- and Lupin-derived peptides inhibit DPP-IV activity on in situ human intestinal Caco-2 cells and ex vivo human serum. Nutrients 10:1082. doi: 10.3390/nu10081082
Lee, S. Y., and Hur, S. J. (2017). Antihypertensive peptides from animal products, marine organisms, and plants. Food Chem. 228, 506–517. doi: 10.1016/j.foodchem.2017.02.039
Lee, T.-H., Hall, N., and Aguilar, M. I. (2016). Antimicrobial peptide structure and mechanism of action: a focus on the role of membrane structure. Curr. Top. Med. Chem. 16, 25–39. doi: 10.2174/1568026615666150703121700
Liang, G., Chen, W., Qie, X., Zeng, M., Qin, F., He, Z., et al. (2020). Modification of soy protein isolates using combined pre-heat treatment and controlled enzymatic hydrolysis for improving foaming properties. Food Hydrocoll. 105:105764. doi: 10.1016/j.foodhyd.2020.105764
Liang, Q., Chalamaiah, M., Ren, X., Ma, H., and Wu, J. (2018). Identification of new anti-inflammatory peptides from zein hydrolysate after simulated gastrointestinal digestion and transport in Caco-2 cells. J. Agric. Food Chem. 66, 1114–1120. doi: 10.1021/acs.jafc.7b04562
Liang, Y., Lin, Q., Huang, P., Wang, Y., Li, J., Zhang, L., et al. (2018). Rice bioactive peptide binding with TLR4 to overcome H(2)O(2)-induced injury in human umbilical vein endothelial cells through NF-κB signaling. J. Agric. Food Chem. 66, 440–448. doi: 10.1021/acs.jafc.7b04036
Li, C., Li, W., Zhang, Y., and Simpson, B. K. (2020). Comparison of physicochemical properties of recombinant buckwheat trypsin inhibitor (rBTI) and soybean trypsin inhibitor (SBTI). Protein Expr. Purif. 171:105614. doi: 10.1016/j.pep.2020.105614
Li, J., Cui, X., Ma, X., Li, C., and Wang, Z. (2019). Recombinant buckwheat trypsin inhibitor improves the protein and mitochondria homeostasis in Caenorhabditis elegans model of aging and age-related disease. Gerontology 65, 513–523. doi: 10.1159/000500156
Li, J., Hu, S., Jian, W., Xie, C., and Yang, X. (2021). Plant antimicrobial peptides: structures, functions, and applications. Bot. Stud. 62:5. doi: 10.1186/s40529-021-00312-x
Ling, Y., Liping, S., and Yongliang, Z. (2018). Preparation and identification of novel inhibitory angiotensin-I-converting enzyme peptides from tilapia skin gelatin hydrolysates: inhibition kinetics and molecular docking. Food Funct. 9, 5251–5259. doi: 10.1039/C8FO00569A
Liscano, Y., Salamanca, C. H., Vargas, L., Cantor, S., Laverde-Rojas, V., and Oñate-Garzón, J. (2019). Increases in hydrophilicity and charge on the polar face of alyteserin 1c helix change its selectivity towards gram-positive bacteria. Antibiotics 8:238. doi: 10.3390/antibiotics8040238
Liu, C., Guo, Y., Zhao, F., Qin, H., Lu, H., Fang, L., et al. (2019). Potential mechanisms mediating the protective effects of a peptide from walnut (Juglans mandshurica maxim.) against hydrogen peroxide induced neurotoxicity in PC12 cells [10.1039/C8FO02557F]. Food Funct. 10, 3491–3501. doi: 10.1039/C8FO02557F
Liu, M., Wang, F., Pu, C., Tang, W., and Sun, Q. (2021). Nanoencapsulation of lutein within lipid-based delivery systems: characterization and comparison of zein peptide stabilized nano-emulsion, solid lipid nanoparticle, and nano-structured lipid carrier. Food Chem. 358:129840. doi: 10.1016/j.foodchem.2021.129840
Liu, Y., Chen, D., You, Y., Zeng, S., Li, Y., Tang, Q., et al. (2016). Nutritional composition of boletus mushrooms from Southwest China and their antihyperglycemic and antioxidant activities. Food Chem. 211, 83–91. doi: 10.1016/j.foodchem.2016.05.032
Lopes-da-Silva, J. A., and Monteiro, S. R. (2019). Gelling and emulsifying properties of soy protein hydrolysates in the presence of a neutral polysaccharide. Food Chem. 294, 216–223. doi: 10.1016/j.foodchem.2019.05.039
López, D. N., Galante, M., Robson, M., Boeris, V., and Spelzini, D. (2018). Amaranth, quinoa and chia protein isolates: physicochemical and structural properties. Int. J. Biol. Macromol. 109, 152–159. doi: 10.1016/j.ijbiomac.2017.12.080
Lu, X., Zhang, L., Sun, Q., Song, G., and Huang, J. (2019). Extraction, identification and structure-activity relationship of antioxidant peptides from sesame (Sesamum indicum L.) protein hydrolysate. Food Res. Int. 116, 707–716. doi: 10.1016/j.foodres.2018.09.001
Lv, R., Dong, Y., Bao, Z., Zhang, S., Lin, S., and Sun, N. (2022). Advances in the activity evaluation and cellular regulation pathways of food-derived antioxidant peptides. Trends Food Sci. Technol. 122, 171–186. doi: 10.1016/j.tifs.2022.02.026
Maestri, E., Marmiroli, M., and Marmiroli, N. (2016). Bioactive peptides in plant-derived foodstuffs. J. Proteome 147, 140–155. doi: 10.1016/j.jprot.2016.03.048
Majid, A., and Poornima Priyadarshini, C. G. (2020). Millet derived bioactive peptides: a review on their functional properties and health benefits. Crit. Rev. Food Sci. Nutr. 60, 3342–3351. doi: 10.1080/10408398.2019.1686342
Malik, A. M., and Singh, A. (2022). Pseudocereals proteins- a comprehensive review on its isolation, composition and quality evaluation techniques. Food Chem. Adv. 1:100001. doi: 10.1016/j.focha.2021.100001
Mammari, N., Krier, Y., Albert, Q., Devocelle, M., and Varbanov, M.On Behalf of the OEMONOM (2021). Plant-derived antimicrobial peptides as potential antiviral agents in systemic viral infections. Pharmaceuticals (Basel) 14:774. doi: 10.3390/ph14080774
Mani-López, E., Palou, E., and López-Malo, A. (2021). Legume proteins, peptides, water extracts, and crude protein extracts as antifungals for food applications. Trends Food Sci. Technol. 112, 16–24. doi: 10.1016/j.tifs.2021.03.035
Maphosa, Y., and Jideani, V. A. (2017). “The role of legumes in human nutrition” in Functional food-improve health through adequate food. ed. M. C. Hueda. InTech, 13.
Marinaccio, L., Zengin, G., Pieretti, S., Minosi, P., Szucs, E., Benyhe, S., et al. (2023). Food-inspired peptides from spinach rubisco endowed with antioxidant, antinociceptive and anti-inflammatory properties. Food Chem. X 18:100640. doi: 10.1016/j.fochx.2023.100640
Marques, M. R., Fontanari, G. G., Pimenta, D. C., Soares-Freitas, R. M., and Arêas, J. A. G. (2015). Proteolytic hydrolysis of cowpea proteins is able to release peptides with hypocholesterolemic activity. Food Res. Int. 77, 43–48. doi: 10.1016/j.foodres.2015.04.020
McClean, S., Beggs, L. B., and Welch, R. W. (2014). Antimicrobial activity of antihypertensive food-derived peptides and selected alanine analogues. Food Chem. 146, 443–447. doi: 10.1016/j.foodchem.2013.09.094
Millan-Linares, M. C., Lemus-Conejo, A., Yust, M. M., Pedroche, J., Carrillo-Vico, A., Millan, F., et al. (2018). GPETAFLR, a novel bioactive peptide from Lupinus angustifolius L. protein hydrolysate, reduces osteoclastogenesis. J. Funct. Foods 47, 299–303. doi: 10.1016/j.jff.2018.05.069
Millan-Linares, M. C., Toscano, R., Lemus-Conejo, A., Martin, M. E., Pedroche, J., Millan, F., et al. (2019). GPETAFLR, a biopeptide from Lupinus angustifolius L., protects against oxidative and inflammatory damage in retinal pigment epithelium cells. J. Food Biochem. 43:e12995. doi: 10.1111/jfbc.12995
Mirzaei, M., Mirdamadi, S., Safavi, M., and Soleymanzadeh, N. (2020). The stability of antioxidant and ACE-inhibitory peptides as influenced by peptide sequences. LWT 130:109710. doi: 10.1016/j.lwt.2020.109710
Moayedi, A., Mora, L., Aristoy, M. C., Safari, M., Hashemi, M., and Toldrá, F. (2018). Peptidomic analysis of antioxidant and ACE-inhibitory peptides obtained from tomato waste proteins fermented using Bacillus subtilis. Food Chem. 250, 180–187. doi: 10.1016/j.foodchem.2018.01.033
Mojica, L., Gonzalez de Mejia, E., Granados-Silvestre, M. Á., and Menjivar, M. (2017). Evaluation of the hypoglycemic potential of a black bean hydrolyzed protein isolate and its pure peptides using in silico, in vitro and in vivo approaches. J. Funct. Foods 31, 274–286. doi: 10.1016/j.jff.2017.02.006
Mori, Y., Asakura, S., Yamamoto, A., Odagiri, S., Yamada, D., Sekiguchi, M., et al. (2018). Characterization of soy-deprestatin, a novel orally active decapeptide that exerts antidepressant-like effects via gut-brain communication. FASEB J. 32, 568–575. doi: 10.1096/fj.201700333RR
Moronta, J., Smaldini, P. L., Docena, G. H., and Añón, M. C. (2016). Peptides of amaranth were targeted as containing sequences with potential anti-inflammatory properties. J. Funct. Foods 21, 463–473. doi: 10.1016/j.jff.2015.12.022
Moyer, T. B., Brechbill, A. M., and Hicks, L. M. (2021). Mass spectrometric identification of antimicrobial peptides from medicinal seeds. Molecules 26:7304. doi: 10.3390/molecules26237304
Muszyński, P., Kulczyńska-Przybik, A., Borawska, R., Litman-Zawadzka, A., Słowik, A., Klimkowicz-Mrowiec, A., et al. (2017). The relationship between markers of inflammation and degeneration in the central nervous system and the blood-brain barrier impairment in Alzheimer's disease. J. Alzheimers Dis. 59, 903–912. doi: 10.3233/jad-170220
Mwangi, J., Hao, X., Lai, R., and Zhang, Z.-Y. (2019). Antimicrobial peptides: new hope in the war against multidrug resistance. Zool. Res. 40, 488–505. doi: 10.24272/j.issn.2095-8137.2019.062
Nagaoka, S., Takeuchi, A., and Banno, A. (2021). Plant-derived peptides improving lipid and glucose metabolism. Peptides 142:170577. doi: 10.1016/j.peptides.2021.170577
Nasiru, A., and Oluwasegun, A. (2019). Phytochemical, nutritional and amino acid composition of Citrullus lanatus (cucurbitaceae) seeds cultivated in south-South Nigeria. J. Pharmacogn. Phytochem. 8, 3738–3740.
Nasri, R., Abdelhedi, O., Nasri, M., and Jridi, M. (2022). Fermented protein hydrolysates: biological activities and applications. Curr. Opin. Food Sci. 43, 120–127. doi: 10.1016/j.cofs.2021.11.006
Ngoh, Y.-Y., and Gan, C.-Y. (2018). Identification of Pinto bean peptides with inhibitory effects on α-amylase and angiotensin converting enzyme (ACE) activities using an integrated bioinformatics-assisted approach. Food Chem. 267, 124–131. doi: 10.1016/j.foodchem.2017.04.166
Nováková, L., Svoboda, P., and Pavlík, J. (2017). “Chapter 29 - ultra-high performance liquid chromatography” in Liquid chromatography. eds. S. Fanali, P. R. Haddad, C. F. Poole, and M.-L. Riekkola. 2nd ed (Elsevier), 719–769.
Nuñez, S. M., Cárdenas, C., Valencia, P., Masip, Y., Pinto, M., and Almonacid, S. (2021). Water-holding capacity of enzymatic protein hydrolysates: a study on the synergistic effects of peptide fractions. LWT 152:112357. doi: 10.1016/j.lwt.2021.112357
Okagu, I. U., Aham, E. C., Ezeorba, T. P. C., Ndefo, J. C., Aguchem, R. N., and Udenigwe, C. C. (2022). Osteo-modulatory dietary proteins and peptides: a concise review. J. Food Biochem. 46:e14365. doi: 10.1111/jfbc.14365
Okoye, C. O., Ezeorba, T. P. C., Okeke, E. S., and Okagu, I. U. (2022). Recent findings on the isolation, identification and quantification of bioactive peptides. Appl. Food Res. 2:100065. doi: 10.1016/j.afres.2022.100065
Olivos-Lugo, B. L., Valdivia-López, M. Á., and Tecante, A. (2010). Thermal and physicochemical properties and nutritional value of the protein fraction of Mexican chia seed (Salvia hispanica L.). Food Sci. Technol. Int. 16, 89–96. doi: 10.1177/1082013209353087
Ortiz-Martinez, M., Winkler, R., and García-Lara, S. (2014). Preventive and therapeutic potential of peptides from cereals against cancer. J. Proteome 111, 165–183. doi: 10.1016/j.jprot.2014.03.044
Osuna-Ruíz, I., Ledezma, A. K. D., Martínez-Montaño, E., Salazar-Leyva, J. A., Tirado, V. A. R., and García, I. B. (2023). Enhancement of in-vitro antioxidant properties and growth of amaranth seed sprouts treated with seaweed extracts. J. Appl. Phycol. 35, 471–481. doi: 10.1007/s10811-022-02872-2
Pandey, R., Rameshkumar, K. B., and Kumar, B. (2015). Ultra high performance liquid chromatography tandem mass spectrometry method for the simultaneous determination of multiple bioactive constituents in fruit extracts of Myristica fragrans and its marketed polyherbal formulations using a polarity switching technique. J. Sep. Sci. 38, 1277–1285. doi: 10.1002/jssc.201401297
Patil, S. P., Goswami, A., Kalia, K., and Kate, A. S. (2020). Plant-derived bioactive peptides: a treatment to cure diabetes. Int. J. Pept. Res. Ther. 26, 955–968. doi: 10.1007/s10989-019-09899-z
Pavlicevic, M., Marmiroli, N., and Maestri, E. (2022). Immunomodulatory peptides—a promising source for novel functional food production and drug discovery. Peptides 148:170696. doi: 10.1016/j.peptides.2021.170696
Pérez-Gregorio, R., Soares, S., Mateus, N., and de Freitas, V. (2020). Bioactive peptides and dietary polyphenols: two sides of the same coin. Molecules 25:3443. doi: 10.3390/molecules25153443
Perlikowska, R. (2021). Whether short peptides are good candidates for future neuroprotective therapeutics? Peptides 140:170528. doi: 10.1016/j.peptides.2021.170528
Phongthai, S., Lim, S.-T., and Rawdkuen, S. (2016). Optimization of microwave-assisted extraction of rice bran protein and its hydrolysates properties. J. Cereal Sci. 70, 146–154. doi: 10.1016/j.jcs.2016.06.001
Phongthai, S., and Rawdkuen, S. (2020). Fractionation and characterization of antioxidant peptides from rice bran protein hydrolysates stimulated by in vitro gastrointestinal digestion. Cereal Chem. 97, 316–325. doi: 10.1002/cche.10247
Pihlanto, A., Johansson, T., and Mäkinen, S. (2012). Inhibition of angiotensin I-converting enzyme and lipid peroxidation by fermented rapeseed and flaxseed meal. Eng. Life Sci. 12, 450–456. doi: 10.1002/elsc.201100137
Piskov, S., Timchenko, L., Grimm, W.-D., Rzhepakovsky, I., Avanesyan, S., Sizonenko, M., et al. (2020). Effects of various drying methods on some physico-chemical properties and the antioxidant profile and ACE inhibition activity of oyster mushrooms (Pleurotus ostreatus). Food Secur. 9:160. doi: 10.3390/foods9020160
Puchalska, P., García, M. C., and Marina, M. L. (2014). Development of a capillary high performance liquid chromatography–ion trap-mass spectrometry method for the determination of VLIVP antihypertensive peptide in soybean crops. J. Chromatogr. A 1338, 85–91. doi: 10.1016/j.chroma.2014.02.059
Quiroga, A. V., Aphalo, P., Nardo, A. E., and Añón, M. C. (2017). In vitro modulation of renin–angiotensin system enzymes by Amaranth (Amaranthus hypochondriacus) protein-derived peptides: alternative mechanisms Different from ACE inhibition. J. Agric. Food Chem. 65, 7415–7423. doi: 10.1021/acs.jafc.7b02240
Ren, D., Zhao, F., Liu, C., Wang, J., Guo, Y., Liu, J., et al. (2018). Antioxidant hydrolyzed peptides from Manchurian walnut (Juglans mandshurica maxim.) attenuate scopolamine-induced memory impairment in mice. J. Sci. Food Agric. 98, 5142–5152. doi: 10.1002/jsfa.9060
Rivero-Pino, F., Espejo-Carpio, F. J., and Guadix, E. M. (2021). Unravelling the α-glucosidase inhibitory properties of chickpea protein by enzymatic hydrolysis and in silico analysis. Food Biosci. 44:101328. doi: 10.1016/j.fbio.2021.101328
Rivero-Pino, F., Leon, M. J., Millan-Linares, M. C., and Montserrat-de la Paz, S. (2023). Antimicrobial plant-derived peptides obtained by enzymatic hydrolysis and fermentation as components to improve current food systems. Trends Food Sci. Technol. 135, 32–42. doi: 10.1016/j.tifs.2023.03.005
Rizzello, C. G., Tagliazucchi, D., Babini, E., Rutella, G. S., Saa, D. L. T., and Gianotti, A. (2016). Bioactive peptides from vegetable food matrices: Research trends and novel biotechnologies for synthesis and recovery. J. Funct. Foods, 27, 549–569. doi: 10.1016/j.jff.2016.09.023
Roudi, R., Syn, N. L., and Roudbary, M. (2017). Antimicrobial peptides as biologic and immunotherapeutic agents against Cancer: a comprehensive overview. Front. Immunol. 8:1320. doi: 10.3389/fimmu.2017.01320
Sá, A. G. A., Moreno, Y. M. F., and Carciofi, B. A. M. (2020). Plant proteins as high-quality nutritional source for human diet. Trends Food Sci. Technol. 97, 170–184. doi: 10.1016/j.tifs.2020.01.011
Sabbione, A. C., Luna-Vital, D., Scilingo, A., Añón, M. C., and González de Mejía, E. (2018). Amaranth peptides decreased the activity and expression of cellular tissue factor on LPS activated THP-1 human monocytes. Food Funct. 9, 3823–3834. doi: 10.1039/c8fo00323h
Sabbione, A. C., Rinaldi, G., Añón, M. C., and Scilingo, A. A. (2016). Antithrombotic effects of Amaranthus hypochondriacus proteins in rats. Plant Foods Hum. Nutr. 71, 19–27. doi: 10.1007/s11130-015-0517-2
Sabbione, A. C., Scilingo, A., and Añón, M. C. (2015). Potential antithrombotic activity detected in amaranth proteins and its hydrolysates. LWT Food Sci. Technol. 60, 171–177. doi: 10.1016/j.lwt.2014.07.015
Saeedi, P., Petersohn, I., Salpea, P., Malanda, B., Karuranga, S., Unwin, N., et al. (2019). Global and regional diabetes prevalence estimates for 2019 and projections for 2030 and 2045: results from the international diabetes federation diabetes atlas, 9th edition. Diabetes Res. Clin. Pract. 157:107843. doi: 10.1016/j.diabres.2019.107843
Sahni, P., Sharma, S., and Surasani, V. K. R. (2020). Influence of processing and pH on amino acid profile, morphology, electrophoretic pattern, bioactive potential and functional characteristics of alfalfa protein isolates. Food Chem. 333:127503. doi: 10.1016/j.foodchem.2020.127503
Saleh, A. S., Zhang, Q., and Shen, Q. (2016). Recent research in antihypertensive activity of food protein-derived hydrolyzates and peptides. Crit. Rev. Food Sci. Nutr. 56, 760–787. doi: 10.1080/10408398.2012.724478
Samaei, S. P., Martini, S., Tagliazucchi, D., Gianotti, A., and Babini, E. (2021). Antioxidant and angiotensin I-converting enzyme (ACE) inhibitory peptides obtained from Alcalase protein hydrolysate fractions of hemp (Cannabis sativa L.) Bran. J. Agric. Food Chemi. 69, 9220–9228. doi: 10.1021/acs.jafc.1c01487
Santos, A. L., and Sinha, S. (2021). Obesity and aging: molecular mechanisms and therapeutic approaches. Ageing Res. Rev. 67:101268. doi: 10.1016/j.arr.2021.101268
Semba, R. D., Ramsing, R., Rahman, N., Kraemer, K., and Bloem, M. W. (2021). Legumes as a sustainable source of protein in human diets. Glob. Food Sec. 28:100520. doi: 10.1016/j.gfs.2021.100520
Shapira, E., Brodsky, B., Proscura, E., Nyska, A., Erlanger-Rosengarten, A., and Wormser, U. (2010). Amelioration of experimental autoimmune encephalitis by novel peptides: involvement of T regulatory cells. J. Autoimmun. 35, 98–106. doi: 10.1016/j.jaut.2010.03.004
Sharma, M., and Saini, C. S. (2022). Amino acid composition, nutritional profiling, mineral content and physicochemical properties of protein isolate from flaxseeds (Linum usitatissimum). J. Food Meas. Charact. 16, 829–839. doi: 10.1007/s11694-021-01221-0
Sharma, P., Kaur, J., Sharma, G., and Kashyap, P. (2022). Plant derived antimicrobial peptides: mechanism of target, isolation techniques, sources and pharmaceutical applications. J. Food Biochem. 46:e14348. doi: 10.1111/jfbc.14348
Sharma, P., Kehinde, B. A., Kaur, S., and Vyas, P. (2019). Application of edible coatings on fresh and minimally processed fruits: a review. Nutri. Food Sci. 49, 713–738. doi: 10.1108/NFS-08-2018-0246
Sheng, J., Yang, X., Liu, Q., Luo, H., Yin, X., Liang, M., et al. (2020). Coadministration with tea polyphenols enhances the neuroprotective effect of defatted walnut meal hydrolysate against scopolamine-induced learning and memory deficits in mice. J. Agric. Food Chem. 68, 751–758. doi: 10.1021/acs.jafc.9b05081
Shewry, P. R., and Halford, N. G. (2002). Cereal seed storage proteins: structures, properties and role in grain utilization. J. Exp. Bot. 53, 947–958. doi: 10.1093/jexbot/53.370.947
Shimizu, A., Mitani, T., Tanaka, S., Fujii, H., Maebuchi, M., Amiya, Y., et al. (2018). Soybean-derived Glycine-arginine dipeptide administration promotes neurotrophic factor expression in the mouse brain. J. Agric. Food Chem. 66, 7935–7941. doi: 10.1021/acs.jafc.8b01581
Shi, Z., Hao, Y., Teng, C., Yao, Y., and Ren, G. (2019). Functional properties and adipogenesis inhibitory activity of protein hydrolysates from quinoa (Chenopodium quinoa Willd.). Food Sci. Nutr. 7, 2103–2112. doi: 10.1002/fsn3.1052
Shobako, N. (2021). Hypotensive peptides derived from plant proteins. Peptides 142:170573. doi: 10.1016/j.peptides.2021.170573
Singh, B. P., Bangar, S. P., Alblooshi, M., Ajayi, F. F., Mudgil, P., and Maqsood, S. (2022). Plant-derived proteins as a sustainable source of bioactive peptides: recent research updates on emerging production methods, bioactivities, and potential application. Crit. Rev. Food Sci. Nutr. 63, 9539–9560. doi: 10.1080/10408398.2022.2067120
Singh, B. P., Vij, S., and Hati, S. (2014). Functional significance of bioactive peptides derived from soybean. Peptides 54, 171–179. doi: 10.1016/j.peptides.2014.01.022
Siow, H.-L., and Gan, C.-Y. (2016). Extraction, identification, and structure–activity relationship of antioxidative and α-amylase inhibitory peptides from cumin seeds (Cuminum cyminum). J. Funct. Foods 22, 1–12. doi: 10.1016/j.jff.2016.01.011
Siow, H.-L., Lim, T. S., and Gan, C.-Y. (2017). Development of a workflow for screening and identification of α-amylase inhibitory peptides from food source using an integrated bioinformatics-phage display approach: case study – cumin seed. Food Chem. 214, 67–76. doi: 10.1016/j.foodchem.2016.07.069
So, K. H., Suzuki, Y., Yonekura, S., Suzuki, Y., Lee, C. H., Kim, S. W., et al. (2015). Soluble extract of soybean fermented with aspergillus oryzae GB107 inhibits fat accumulation in cultured 3T3-L1 adipocytes. Nutr. Res. Pract. 9, 439–444. doi: 10.4162/nrp.2015.9.4.439
Sonklin, C., Alashi, M. A., Laohakunjit, N., Kerdchoechuen, O., and Aluko, R. E. (2020). Identification of antihypertensive peptides from mung bean protein hydrolysate and their effects in spontaneously hypertensive rats. J. Funct. Foods 64:103635. doi: 10.1016/j.jff.2019.103635
Sorensen, M. J., Anderson, B. G., and Kennedy, R. T. (2020). Liquid chromatography above 20,000 PSI. TrAC Trends Anal. Chem. 124:115810. doi: 10.1016/j.trac.2020.115810
Sosalagere, C., Adesegun Kehinde, B., and Sharma, P. (2022). Isolation and functionalities of bioactive peptides from fruits and vegetables: A reviews. Food Chem. 366:130494. doi: 10.1016/j.foodchem.2021.130494
Susnea, I., Bernevic, B., Wicke, M., Ma, L., Liu, S., Schellander, K., et al. (2013). “Application of MALDI-TOF-mass spectrometry to proteome analysis using stain-free gel electrophoresis” in Applications of MALDI-TOF spectroscopy. eds. Z. Cai and S. Liu (Berlin Heidelberg: Springer), 37–54.
Suwannapan, O., Wachirattanapongmetee, K., Thawornchinsombut, S., and Katekaew, S. (2020). Angiotensin-I-converting enzyme (ACE)-inhibitory peptides from Thai jasmine rice bran protein hydrolysates. Int. J. Food Sci. Technol. 55, 2441–2450. doi: 10.1111/ijfs.14495
Sytar, O., Brestic, M., Zivcak, M., and Phan Tran, L.-S. (2016). The contribution of buckwheat genetic resources to health and dietary diversity. Curr. Genomics 17, 193–206. doi: 10.2174/1389202917666160202215425
Tang, C.-H., Peng, J., Zhen, D.-W., and Chen, Z. (2009). Physicochemical and antioxidant properties of buckwheat (Fagopyrum esculentum Moench) protein hydrolysates. Food Chem. 115, 672–678. doi: 10.1016/j.foodchem.2008.12.068
Tang, T., Du, H., Tang, S., Jiang, Y., Tu, Y., Hu, M., et al. (2021). Effects of incorporating different kinds of peptides on the foaming properties of egg white powder. Innov. Food Sci. Emerg. Technol. 72:102742. doi: 10.1016/j.ifset.2021.102742
Taniguchi, M., Aida, R., Saito, K., Ochiai, A., Takesono, S., Saitoh, E., et al. (2019). Identification and characterization of multifunctional cationic peptides from traditional Japanese fermented soybean Natto extracts. J. Biosci. Bioeng. 127, 472–478. doi: 10.1016/j.jbiosc.2018.09.016
Tkaczewska, J. (2020). Peptides and protein hydrolysates as food preservatives and bioactive components of edible films and coatings-a review. Trends Food Sci. Technol. 106, 298–311. doi: 10.1016/j.tifs.2020.10.022
Toldrá, F., Gallego, M., Reig, M., Aristoy, M.-C., and Mora, L. (2020). Recent Progress in enzymatic release of peptides in foods of animal origin and assessment of bioactivity. J. Agric. Food Chem. 68, 12842–12855. doi: 10.1021/acs.jafc.9b08297
Ucak, I., Afreen, M., Montesano, D., Carrillo, C., Tomasevic, I., Simal-Gandara, J., et al. (2021). Functional and bioactive properties of peptides derived from marine side streams. Mar. Drugs 19:71. doi: 10.3390/md19020071
Udenigwe, C. C., and Aluko, R. E. (2012). Food protein-derived bioactive peptides: production, processing, and potential health benefits. J. Food Sci. 77, R11–R24. doi: 10.1111/j.1750-3841.2011.02455.x
Valdez-Miramontes, C., De Haro-Acosta, J., Aréchiga-Flores, C., Verdiguel-Fernández, L., and Rivas-Santiago, B. (2021). Antimicrobial peptides in domestic animals and their applications in veterinary medicine. Peptides 142:170576. doi: 10.1016/j.peptides.2021.170576
Vásquez-Villanueva, R., Muñoz-Moreno, L., Carmena, M. J., Marina, M. L., and García, M. C. (2018). In vitro antitumor and hypotensive activity of peptides from olive seeds. J. Funct. Foods 42, 177–184. doi: 10.1016/j.jff.2017.12.062
Vilcacundo, R., Martínez-Villaluenga, C., and Hernández-Ledesma, B. (2017). Release of dipeptidyl peptidase IV, α-amylase and α-glucosidase inhibitory peptides from quinoa (Chenopodium quinoa Willd.) during in vitro simulated gastrointestinal digestion. J. Funct. Foods 35, 531–539. doi: 10.1016/j.jff.2017.06.024
Vollet Marson, G., Belleville, M.-P., Lacour, S., and Dupas Hubinger, M. (2020). Membrane fractionation of protein hydrolysates from by-products: recovery of valuable compounds from spent yeasts. Membranes 11:23. doi: 10.3390/membranes11010023
Vuyyuri, S. B., Shidal, C., and Davis, K. R. (2018). Development of the plant-derived peptide lunasin as an anticancer agent. Curr. Opin. Pharmacol. 41, 27–33. doi: 10.1016/j.coph.2018.04.006
Wang, J., Wu, T., Fang, L., Liu, C., Liu, X., Li, H., et al. (2020). Anti-diabetic effect by walnut (Juglans mandshurica maxim.)-derived peptide LPLLR through inhibiting α-glucosidase and α-amylase, and alleviating insulin resistance of hepatic HepG2 cells. J. Funct. Foods 69:103944. doi: 10.1016/j.jff.2020.103944
Wang, K., Luo, Q., Hong, H., Liu, H., and Luo, Y. (2021). Novel antioxidant and ACE inhibitory peptide identified from Arthrospira platensis protein and stability against thermal/pH treatments and simulated gastrointestinal digestion. Food Res. Int. 139:109908. doi: 10.1016/j.foodres.2020.109908
Wang, K., Niu, M., Song, D., Liu, Y., Wu, Y., Zhao, J., et al. (2020). Evaluation of biochemical and antioxidant dynamics during the co-fermentation of dehusked barley with Rhizopus oryzae and Lactobacillus plantarum. J. Food Biochem. 44:e13106. doi: 10.1111/jfbc.13106
Wang, M., Amakye, W. K., Gong, C., Ren, Z., Yuan, E., and Ren, J. (2022). Effect of oral and intraperitoneal administration of walnut-derived pentapeptide PW5 on cognitive impairments in APP(SWE)/PS1(ΔE9) mice. Free Radic. Biol. Med. 180, 191–197. doi: 10.1016/j.freeradbiomed.2022.01.003
Wang, Q., and Xiong, Y. L. (2019). Processing, nutrition, and functionality of hempseed protein: a review. Compr. Rev. Food Sci. Food Saf. 18, 936–952. doi: 10.1111/1541-4337.12450
Wang, R., Zhao, H., Pan, X., Orfila, C., Lu, W., and Ma, Y. (2019). Preparation of bioactive peptides with antidiabetic, antihypertensive, and antioxidant activities and identification of α-glucosidase inhibitory peptides from soy protein. Food Sci. Nutr 7, 1848–1856. doi: 10.1002/fsn3.1038
Wei, C.-K., Thakur, K., Liu, D.-H., Zhang, J.-G., and Wei, Z.-J. (2018). Enzymatic hydrolysis of flaxseed (Linum usitatissimum L.) protein and sensory characterization of Maillard reaction products. Food Chem. 263, 186–193. doi: 10.1016/j.foodchem.2018.04.120
Welker, M., and Moore, E. R. B. (2011). Applications of whole-cell matrix-assisted laser-desorption/ionization time-of-flight mass spectrometry in systematic microbiology. Syst. Appl. Microbiol. 34, 2–11. doi: 10.1016/j.syapm.2010.11.013
Wen, C., Zhang, J., Zhang, H., Duan, Y., and Ma, H. (2020). Plant protein-derived antioxidant peptides: isolation, identification, mechanism of action and application in food systems: a review. Trends Food Sci. Technol. 105, 308–322. doi: 10.1016/j.tifs.2020.09.019
Wen, L., Jiang, Y., Zhou, X., Bi, H., and Yang, B. (2021). Structure identification of soybean peptides and their immunomodulatory activity. Food Chem. 359:129970. doi: 10.1016/j.foodchem.2021.129970
Willett, W., Rockström, J., Loken, B., Springmann, M., Lang, T., Vermeulen, S., et al. (2019). Food in the Anthropocene: the EAT–lancet commission on healthy diets from sustainable food systems. Lancet 393, 447–492. doi: 10.1016/S0140-6736(18)31788-4
Wittkopf, N., Neurath, M. F., and Becker, C. (2014). Immune-epithelial crosstalk at the intestinal surface. J. Gastroenterol. 49, 375–387. doi: 10.1007/s00535-013-0929-4
Wong, F.-C., Xiao, J., Ong, M. G. L., Pang, M.-J., Wong, S.-J., Teh, L.-K., et al. (2019). Identification and characterization of antioxidant peptides from hydrolysate of blue-spotted stingray and their stability against thermal, pH and simulated gastrointestinal digestion treatments. Food Chem. 271, 614–622. doi: 10.1016/j.foodchem.2018.07.206
Wong, F.-C., Xiao, J., Wang, S., Ee, K.-Y., and Chai, T.-T. (2020). Advances on the antioxidant peptides from edible plant sources. Trends Food Sci. Technol. 99, 44–57. doi: 10.1016/j.tifs.2020.02.012
Wu, H., Rui, X., Li, W., Xiao, Y., Zhou, J., and Dong, M. (2018). Whole-grain oats (Avena sativa L.) as a carrier of lactic acid bacteria and a supplement rich in angiotensin I-converting enzyme inhibitory peptides through solid-state fermentation. Food Funct. 9, 2270–2281. doi: 10.1039/C7FO01578J
Wu, W., Li, B., Hou, H., Zhang, H., and Zhao, X. (2017). Isolation and identification of calcium-chelating peptides from Pacific cod skin gelatin and their binding properties with calcium. Food Funct. 8, 4441–4448. doi: 10.1039/C7FO01014A
Xiao, Y., Sun, M., Zhang, Q., Chen, Y., Miao, J., Rui, X., et al. (2018). Effects of Cordyceps militaris (L.) Fr. Fermentation on the nutritional, physicochemical, functional properties and angiotensin I converting enzyme inhibitory activity of red bean (Phaseolus angularis [Willd.] WF Wight.) flour 55, 1244–1255. doi: 10.1007/s13197-018-3035-z,
Xie, Y., Pan, M., Gao, Y., Zhang, L., Ge, W., and Tang, P. (2019). Dose-dependent roles of aspirin and other non-steroidal anti-inflammatory drugs in abnormal bone remodeling and skeletal regeneration. Cell Biosci. 9:103. doi: 10.1186/s13578-019-0369-9
Xiong, R., Zhou, X. G., Tang, Y., Wu, J. M., Sun, Y. S., Teng, J. F., et al. (2021). Lychee seed polyphenol protects the blood-brain barrier through inhibiting Aβ(25-35)-induced NLRP3 inflammasome activation via the AMPK/mTOR/ULK1-mediated autophagy in bEnd.3 cells and APP/PS1 mice. Phytother. Res. 35, 954–973. doi: 10.1002/ptr.6849
Xu, B., Wang, X., Zheng, Y., Li, Y., Guo, M., and Yan, Z. (2022). Novel antioxidant peptides identified in millet bran glutelin-2 hydrolysates: purification, in silico characterization and security prediction, and stability profiles under different food processing conditions. LWT 164:113634. doi: 10.1016/j.lwt.2022.113634
Yamada, Y., Muraki, A., Oie, M., Kanegawa, N., Oda, A., Sawashi, Y., et al. (2012). Soymorphin-5, a soy-derived μ-opioid peptide, decreases glucose and triglyceride levels through activating adiponectin and PPARα systems in diabetic KKAy mice. Am. J. Physiol. Endocrinol. Metab. 302, E433–E440. doi: 10.1152/ajpendo.00161.2011
Yang, F.-J., Xu, C., Huang, M.-C., Qian, Y., Cai, X.-X., Xuan, C., et al. (2021). Molecular characteristics and structure–activity relationships of food-derived bioactive peptides. J. Integr. Agric. 20, 2313–2332. doi: 10.1016/S2095-3119(20)63463-3
Yang, J., Fang, L., Lu, H., Liu, C., Wang, J., Wu, D., et al. (2022). Walnut-derived peptide enhances Mitophagy via JNK-mediated PINK1 activation to reduce oxidative stress in HT-22 cells. J. Agric. Food Chem. 70, 2630–2642. doi: 10.1021/acs.jafc.2c00005
Yang, R., Li, X., Lin, S., Zhang, Z., and Chen, F. (2017). Identification of novel peptides from 3 to 10 kDa pine nut (Pinus koraiensis) meal protein, with an exploration of the relationship between their antioxidant activities and secondary structure. Food Chem. 219, 311–320. doi: 10.1016/j.foodchem.2016.09.163
Yesiltas, B., Gregersen, S., Lægsgaard, L., Brinch, M. L., Olsen, T. H., Marcatili, P., et al. (2021). Emulsifier peptides derived from seaweed, methanotrophic bacteria, and potato proteins identified by quantitative proteomics and bioinformatics. Food Chem. 362:130217. doi: 10.1016/j.foodchem.2021.130217
Ying, X., Agyei, D., Udenigwe, C., Adhikari, B., and Wang, B. (2021). Manufacturing of plant-based bioactive peptides using enzymatic methods to meet health and sustainability targets of the sustainable development goals. Front. Sustain. Food Syst. 5:769028. doi: 10.3389/fsufs.2021.769028
Yi-Shen, Z., Shuai, S., and FitzGerald, R. (2018). Mung bean proteins and peptides: nutritional, functional and bioactive properties. Food Nutr. Res. 62:10.29219/fnr.v62.1290. doi: 10.29219/fnr.v62.1290
Yuan, H., Luo, Z., Ban, Z., Reiter, R. J., Ma, Q., Liang, Z., et al. (2022). Bioactive peptides of plant origin: distribution, functionality, and evidence of benefits in food and health. Food Funct. 13, 3133–3158. doi: 10.1039/D1FO04077D
Yu, G., Wang, F., Zhang, B., and Fan, J. (2016). In vitro inhibition of platelet aggregation by peptides derived from oat (Avena sativa L.), highland barley (Hordeum vulgare Linn. Var. nudum Hook. F.), and buckwheat (Fagopyrum esculentum Moench) proteins. Food Chem. 194, 577–586. doi: 10.1016/j.foodchem.2015.08.058
Yu, J., Mikiashvili, N., Bonku, R., and Smith, I. N. (2021). Allergenicity, antioxidant activity and ACE-inhibitory activity of protease hydrolyzed peanut flour. Food Chem. 360:129992. doi: 10.1016/j.foodchem.2021.129992
Zaky, A. A., Simal-Gandara, J., Eun, J.-B., Shim, J.-H., and Abd El-Aty, A. (2022). Bioactivities, applications, safety, and health benefits of bioactive peptides from food and by-products: a review. Front. Nutr. 8:815640. doi: 10.3389/fnut.2021.815640
Zanoni, C., Aiello, G., Arnoldi, A., and Lammi, C. (2017). Hempseed peptides exert Hypocholesterolemic effects with a statin-like mechanism. J. Agric. Food Chem. 65, 8829–8838. doi: 10.1021/acs.jafc.7b02742
Zhang, C., Bai, X., Dedkova, L. M., and Hecht, S. M. (2020). Protein synthesis with conformationally constrained cyclic dipeptides. Bioorg. Med. Chem. 28:115780. doi: 10.1016/j.bmc.2020.115780
Zhang, J., Wen, C., Li, C., Duan, Y., Zhang, H., and Ma, H. (2019). Antioxidant peptide fractions isolated from wheat germ protein with subcritical water extraction and its transport across Caco-2 cells. J. Food Sci. 84, 2139–2146. doi: 10.1111/1750-3841.14720
Zhang, P., Chang, C., Liu, H., Li, B., Yan, Q., and Jiang, Z. (2020). Identification of novel angiotensin I-converting enzyme (ACE) inhibitory peptides from wheat gluten hydrolysate by the protease of Pseudomonas aeruginosa. J. Funct. Foods 65:103751. doi: 10.1016/j.jff.2019.103751
Zhang, P., Roytrakul, S., and Sutheerawattananonda, M. (2017). Production and purification of glucosamine and angiotensin-I converting enzyme (ACE) inhibitory peptides from mushroom hydrolysates. J. Funct. Foods 36, 72–83. doi: 10.1016/j.jff.2017.06.049
Zhang, Q., Tong, X., Qi, B., Wang, Z., Li, Y., Sui, X., et al. (2018). Changes in antioxidant activity of Alcalase-hydrolyzed soybean hydrolysate under simulated gastrointestinal digestion and transepithelial transport. J. Funct. Foods 42, 298–305. doi: 10.1016/j.jff.2018.01.017
Zhang, Y., Liu, M., Wang, S., Kang, C., Zhang, M., and Li, Y. (2022). Identification and quantification of fox meat in meat products by liquid chromatography–tandem mass spectrometry. Food Chem. 372:131336. doi: 10.1016/j.foodchem.2021.131336
Zhao, F., Wang, J., Lu, H., Fang, L., Qin, H., Liu, C., et al. (2020). Neuroprotection by walnut-derived peptides through autophagy promotion via Akt/mTOR signaling pathway against oxidative stress in PC12 cells. J. Agric. Food Chem. 68, 3638–3648. doi: 10.1021/acs.jafc.9b08252
Zhao, Q., He, L., Wang, X., Ding, X., Li, L., Tian, Y., et al. (2022). Characterization of a novel antimicrobial peptide isolated from Moringa oleifera seed protein hydrolysates and its membrane damaging effects on Staphylococcus aureus. J. Agric. Food Chem. 70, 6123–6133. doi: 10.1021/acs.jafc.2c01335
Zheng, Y., Li, Y., Zhang, Y., Zhang, R., Zhang, Y., and Zhao, S. (2015). Effects of limited enzymatic hydrolysis, pH, ionic strength and temperature on physicochemical and functional properties of palm (Elaeis guineensis Jacq.) kernel expeller protein. J. Food Sci. Technol. 52, 6940–6952. doi: 10.1007/s13197-015-1839-7
Zhou, C., Ma, H., Ding, Q., Lin, L., Yu, X., Luo, L., et al. (2013). Ultrasonic pretreatment of corn gluten meal proteins and neutrase: effect on protein conformation and preparation of ACE (angiotensin converting enzyme) inhibitory peptides. Food Bioprod. Process. 91, 665–671. doi: 10.1016/j.fbp.2013.06.003
Keywords: plant protein, peptide, bioactivities, bioavailability, recycle, good health and wellbeing
Citation: Nirmal N, Khanashyam AC, Shah K, Awasti N, Sajith Babu K, Ucak İ, Afreen M, Hassoun A and Tuanthong A (2024) Plant protein-derived peptides: frontiers in sustainable food system and applications. Front. Sustain. Food Syst. 8:1292297. doi: 10.3389/fsufs.2024.1292297
Edited by:
Ahmad Ali, University of Mumbai, IndiaReviewed by:
Gengjun Chen, Kansas State University, United StatesPamela Jha, SVKM's Narsee Monjee Institute of Management Studies, India
Copyright © 2024 Nirmal, Khanashyam, Shah, Awasti, Sajith Babu, Ucak, Afreen, Hassoun and Tuanthong. This is an open-access article distributed under the terms of the Creative Commons Attribution License (CC BY). The use, distribution or reproduction in other forums is permitted, provided the original author(s) and the copyright owner(s) are credited and that the original publication in this journal is cited, in accordance with accepted academic practice. No use, distribution or reproduction is permitted which does not comply with these terms.
*Correspondence: Nilesh Nirmal, nilesh.nir@mahidol.ac.th