- 1Department of Plant Production and Genetics, Faculty of Agriculture, University of Kurdistan, Sanandaj, Iran
- 2Department of Water Sciences and Engineering, Faculty of Agriculture, University of Kurdistan, Sanandaj, Iran
- 3The University of Queensland, Brisbane, QLD, Australia
- 4Department of Soil Science, Faculty of Agriculture, University of Kurdistan, Sanandaj, Iran
Introduction: Quinoa a crop with high nutritional value has the widespread adaptability to different climates and there is an increased demand for this product in the world. Characterized by roots with extensive penetration capabilities and wide, alternate leaves giving rise to diverse inflorescences, quinoa plants exhibit variable heights ranging from 20 to 300 cm. The duration of their growth phase spans 95 to 125 days’ contingent upon cultivar selection and prevailing climatic conditions.
Materials and methods: In order to reduce chemical fertilizers consumption through using organic fertilizers a two-year study was conducted at the Research Farm of the University of Kurdistan, Iran, during the 2021–2022 growing seasons. In this study the effects of varying levels of irrigation and vermicompost application on soil characteristics and quinoa (Chenopodium quinoa) yield were investigated. The experiments were arranged as split plots within a randomized complete block design. The main factor consisted of four irrigation levels (50%, 75%, 100%, and 125% of quinoa’s water requirement), while the sub-factor encompassed four vermicompost application rates (0, 5, 10, and 15 tons per hectare). The irrigation method employed was a drip-tape irrigation system with a seven-day interval between irrigations, and water quantities were measured using a volumetric meter on the main pipeline.
Results: The results demonstrated that the highest levels of available soil phosphorus (21.83 mg kg−1) and soil ammonium (36.08 mg kg−1) were observed in the treatment receiving 50% of quinoa’s water requirement combined with 15 tons per hectare of vermicompost. Additionally, the application of 15 tons per hectare of vermicompost led to the highest concentrations of soil nitrate (14.16 mg kg−1), available potassium (144.62 mg kg−1), and quinoa seed yield (1784.01 kg ha−1)). Over both years, the greatest activity of alkaline and acid phosphatase enzymes was noted in the treatment receiving 125% of quinoa’s water requirement in combination with 15 tons per hectare of vermicompost. Vermicompost application was found to enhance yield and ameliorate drought stress by enhancing soil physical and chemical properties and improving soil moisture retention.
Introduction
Quinoa (Chenopodium quinoa Willd.) is indigenous to the Andes region, spanning Bolivia, Peru, and Chile (Hemalatha et al., 2016). It features deep, permeable roots, wide, alternating leaves, and blossoms in various colors. Quinoa’s height varies from 20 to 300 cm, and its growing season ranges from 95 to 125 days, contingent on the specific variety and prevailing climatic conditions (Rathore and Kumar, 2021).
Quinoa is an allotetraploid plant with 250 varieties cultivated worldwide and native to the Andean regions of South America, such as Peru, Bolivia, Chile, Argentina, Ecuador, and Colombia (Agarwal et al., 2023). The plant is also grown in Colorado, Washington, Oregon, England, and Scandinavia. Quinoa is gaining much attention because of its resistance to different temperatures and climatic conditions, including low rainfall, temperate regions, marginal lands, high temperature, and poor sandy, alkaline soils, without losing its nutritional value (Galvez et al., 2010; Agarwal et al., 2023; Jan et al., 2023). Owing to its high micronutrient intake, resistance to extreme climatic conditions, dietary benefits, and fewer inputs for production (e.g., chemical fertilizers), quinoa is considered a “future smart food” by the Food and Agriculture Organization of the United Nations (FAO, 2012). The plant consists of leaves and seeds as edible parts, but seeds are gaining more importance regarding their nutritional benefits (Rathore and Kumar, 2021). Quinoa seeds show a high composition of proteins, carbohydrates, and lipids with excellent balance (Pathan and Siddiqui, 2022). Since it supplies all the amino acids, FAO/WHO recommends it for adults as well. Because of quinoa’s high nutritional value, the FAO declared 2013 as the “Year of Quinoa.”
The quinoa yield in Kenya, Europe, Denmark, and Australia has been recorded as 4, 2, 3, and 1 tons per hectare, respectively. FAO has predicted quinoa yield between 3 and 4 tons per hectare under proper crop management (FAO, 2012).
In conventional agricultural practices, the widespread use of chemical fertilizers is customary. Nonetheless, the indiscriminate application of chemical fertilizers poses potential environmental risks, encompassing surface and groundwater pollution, contamination of wetlands, soil pollution, reductions in soil organic matter (SOM), and the introduction of toxic elements from these fertilizers into the human food chain (Mengistu et al., 2017). However, the continuous use of chemical fertilizers is responsible for the decline in soil organic matter (SOM) content as well as the decrease in the quality of agricultural soil. The excessive use of chemical fertilizers hardens the soil, diminishes soil fertility, pollutes the air, water, and soil, and depletes important nutrients and minerals in the soil, therefore posing hazards to the environment. Consequently, organic fertilization has emerged as a viable alternative (Pahalvi et al., 2021).
To attain sustainable agriculture, altering the input application patterns and prioritizing conservation while augmenting SOM content have been identified as pivotal strategies (Adekiya and Agbede, 2017). Organic fertilizers not only supply organic matter and nutrients but also enhance microbial activity, foster biodiversity, and increase microbial populations within the soil. Moreover, they positively influence various soil physicochemical parameters, nutrient cycling, and soil structure (Jabeen and Ahmad, 2019). Organic matter exerts direct effects on crop growth and yield through nutrient provision and indirect effects by modifying soil physical properties like aggregate stability and porosity. Additionally, it enhances the root zone of crops, thereby stimulating crop growth (Ortas and Lal, 2014). One recognized method for soil restoration and improvement is vermicomposting, which is instrumental in enhancing the overall health of degraded soils.
Vermicompost is an environmentally friendly natural manure produced by the decomposition of organic matter through the synergistic interactions between microorganisms and earthworms (Mengistu et al., 2017). It manifests as a peat-like substance characterized by an exceptionally high decomposition rate, notable porosity, superior aeration properties, efficient drainage capabilities, substantial water-holding capacity, and heightened microbial activity. This biological fertilizer stands out for its remarkable water retention capacity, reducing crop water requirements (CWR) by approximately 30–40% (Aksakal et al., 2016). The introduction of vermicompost into the soil fosters the development of additional soil pores, thus enhancing crucial soil aeration for both crop growth and microbial activity (Sangwan et al., 2018). Notably, vermicompost is rich in essential nutrients such as magnesium, calcium, phosphorus, potassium, and nitrate, all readily accessible to crops. Its attributes, including microbial activity, water-holding capacity, drainage, aeration, and high porosity, make it an ideal soil conditioner and amendment. Vermicompost has proven to be a valuable asset for enhancing soil productivity in consecutive cropping cycles. It serves as a potent soil amendment, augmenting soil fertility by elevating nutrient content, cation exchange capacity (CEC), and soil organic matter (SOM), while simultaneously ameliorating soil structure (Chandra, 2015). The depletion of SOM represents a prominent contributor to the erosion of ecosystem resilience and the degradation of ecosystem services (Feller et al., 2012). Vermicompost has promising application prospects as a cost-effective and efficient soil amendment (Tascı and Kuzucu, 2023). In another study, the results showed that the advantages of vermicomposting to reduce nitrogen are that it can stabilize production, increase production, increase farmers’ income, reduce the cost of cultivation, and reduce the use of nitrogen fertilizer in further crops (Thakur et al., 2021).
Furthermore, organic fertilization, including the application of vermicompost, has demonstrated efficacy in suppressing crop diseases, particularly those induced by soil-borne pathogens. It enhances microbial biomass and activity, elevates SOM content, and fortifies soil against erosion (Sangwan et al., 2018). Compared to other organic fertilizers, vermicompost exhibits an exceptional capacity to augment the availability of essential nutrients for crops (Jabeen and Ahmad, 2019) and stimulate enzymatic activity, including protease, amylase, cellulase, and pectinase.
In addition to these attributes, vermicompost is acknowledged for its ability to enhance various soil physical properties. It promotes improved air and water permeability, culminating in increased total porosity. It effectively enhances soil aggregate stability, reducing penetration resistance and bulk density, thus fostering favorable conditions for crop cultivation (Aksakal et al., 2016). Moreover, vermicompost amplifies the soil’s fertility status, thereby augmenting nutrient availability for crops and enhancing moisture retention capacity (Chandra, 2015). In arid and semi-arid regions, including Iran, the imperative for ensuring food security and sustainable agriculture hinges on the judicious management, conservation, and rational utilization of limited water resources (Irmak et al., 2016). Iran, notably, confronts the fourth highest water stress levels in the Middle East (Liu et al., 2018). Recent years have witnessed an upsurge in water demand, coupled with groundwater depletion and insufficient access to water resources, exacerbating water scarcity concerns in these areas (Dhawan, 2017). With the agricultural sector being the largest consumer of water, it contends with the burgeoning water demands of the industrial and urban sectors, thereby intensifying water competition. Furthermore, it is well established that irrigation plays a pivotal role in augmenting agricultural production. Effective irrigation water management, through the adoption of enhanced techniques, holds the potential to conserve water and enhance water productivity (WP). Embracing high-efficiency irrigation systems such as the drip irrigation system emerges as an efficacious means to elevate water efficiency and curtail water usage in agriculture (Bozkurt Çolak et al., 2021).
The pressurized irrigation system represents a contemporary irrigation approach distinguished by its heightened irrigation application efficiency. When adequately designed, implemented, and managed, the pressurized irrigation system stands poised to significantly augment water productivity (WP) and curtail the extraction of water from primary water sources. A notable innovation in micro-irrigation methods is the drip-tape irrigation system (Liu et al., 2018). In this approach, irrigation water is delivered directly into the root zone of crops. Consequently, surface evaporation rates diminish, deep water penetration is optimized, and crop yields experience an upswing (Irmak et al., 2016). The drip-tape irrigation system boasts several merits, including enhanced water use efficiency (WUE), decreased groundwater pollution (attributable to reduced nitrate leaching), adoption of shorter irrigation intervals, effective salt leaching, uniform water distribution, quantitatively and qualitatively improved crop yields, weed management, judicious administration of fertilizers and pesticides, automation capabilities, and high adaptability (Valentín et al., 2020).
Notably, farmers in Kurdistan province, as well as other regions of Iran, cultivate crops with substantial water and nutrient requirements. The excessive application of irrigation water and chemical fertilizers in these agricultural lands has led to the contamination of groundwater, exemplified by elevated nitrate concentrations, and surface water, characterized by the proliferation of algae in dam lakes. Given the inherent significance of quinoa as a valuable grain and its exceptional adaptability to diverse climatic conditions, conducting a study on quinoa, a low-expectation crop with high nutritional value, serves as a strategic initiative. Such research offers an alternative to water-intensive crops and aims to investigate the potential of vermicompost in enhancing soil quality and fostering quinoa cultivation in Kurdistan Province, Iran.
Materials and methods
Area description
This study was carried out within the Research Field of the University of Kurdistan, located in the Dehgolan Plain, situated at geographical coordinates of 18.35 N latitude and 18.47 E longitude, with an altitude of 1,866 m above mean sea level. The research spanned the years 2021 and 2022. The region in question experiences an average annual precipitation of 350 mm and falls within the classification of a semi-arid Mediterranean climate as determined by the Amber method. The annual average maximum and minimum temperatures in this locale are recorded at 23 and 6.6°C, respectively (Figure 1).
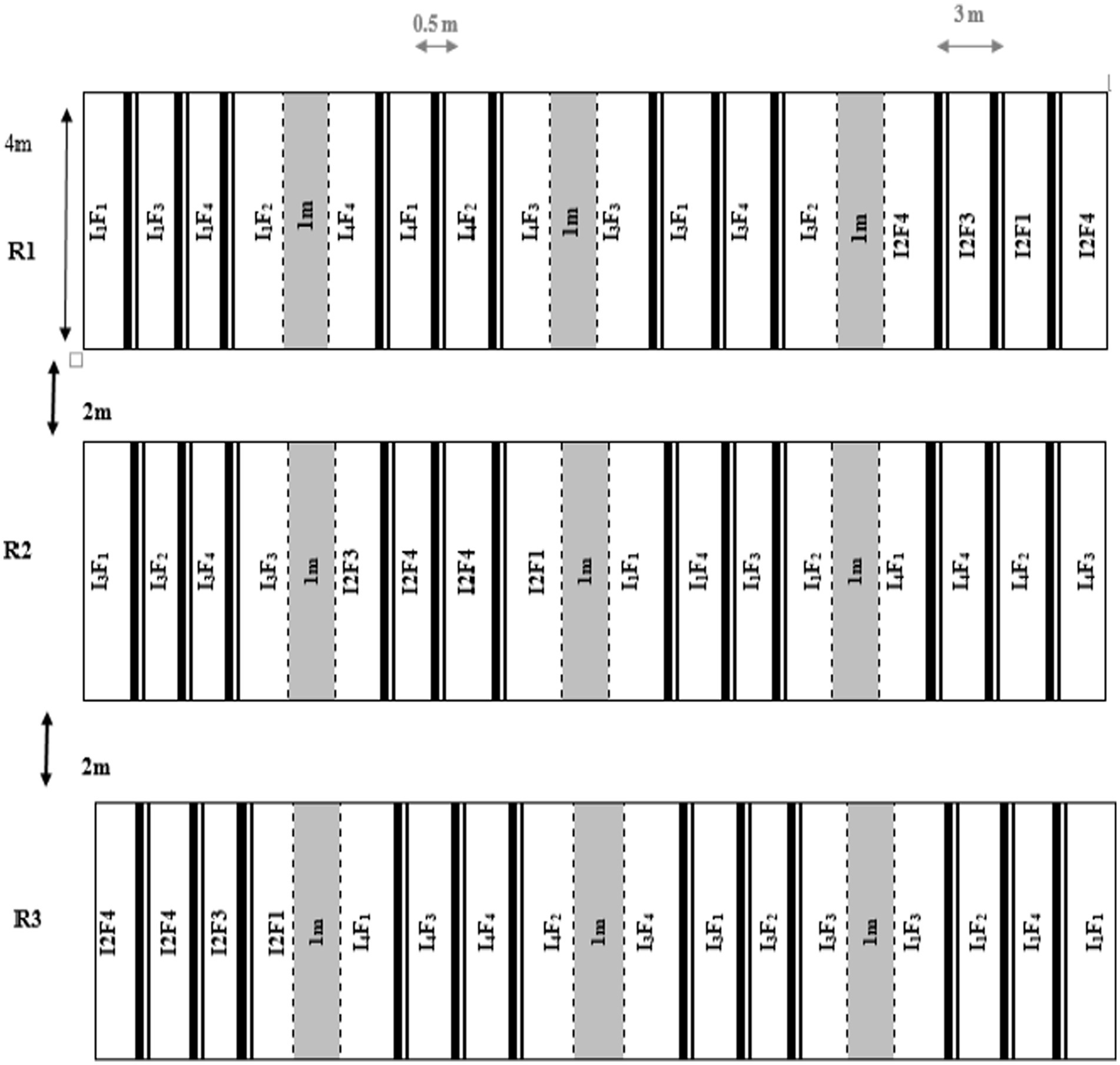
Figure 1. Schematic diagram of the experimental design and locations of treatments and their replications. I1F1, 50% plant water requirement + no use of vermicompost; I1F2, 50% of plant water requirement +5 tons per hectare of vermicompost; I1F3, 50% of plant water requirement +10 tons per hectare of vermicompost; I1F4, 50% of plant water requirement +15 tons per hectare of vermicompost; I2F1, 75% of plant water requirement + no use of vermicompost; I2F2, 75% of plant water requirement +5 tons per hectare of vermicompost; I2F3, 75% of plant water requirement +10 tons per hectare of vermicompost; I2F4, 75% of plant water requirement +15 tons per hectare of vermicompost. I3F1, 100% plant water requirement + no use of vermicompost; I3F2, 100% of plant water requirement +5 tons per hectare of vermicompost; I3F3, 100% of plant water requirement +10 ton/h of vermicompost; I3F4, 100% of plant water requirement +15 tons per hectare of vermicompost. I4F1, 125% plant water requirement + no use of vermicompost; I4F2, 125% of plant water requirement +5 tons per hectare of vermicompost; I4F3, 125% of plant water requirement +10 tons per hectare of vermicompost; I4F4, 125% of plant water requirement +15 tons per hectare of vermicompost.
Properties of soil and vermicompost
Table 1 provides an overview of the pertinent characteristics of the field soil and vermicompost utilized in this study. The absorbable phosphorus content of the soil was extracted following the methodology established by Olsen and Sommers (1990), while absorbable potassium was extracted using ammonium acetate, as per the procedure outlined by Westeman (1990). The concentrations of phosphorus and potassium in the soil were determined using a spectrophotometer (model: Ultraviolet C 292) and a flame photometer (model: Jenway), respectively. Additionally, the assessment of iron absorbability by both soil and modifiers was conducted through the DTPA method, as prescribed by Linday and Norvell (1978).
Table 1 unequivocally demonstrates that the soil’s texture is classified as clay loam. The determination of soil texture was accomplished through the hydrometric method. It is worth noting that clay loam soil, while typically rich in nutrients, exhibits two notable drawbacks. In its wet state, it tends to swell, thereby impeding water infiltration rates into the soil. Over time, this compromised drainage condition can hinder crop growth and ultimately reduce yields. Conversely, when clay loam soil dries, it contracts, forming compact clods that can crust the soil surface. These two limitations can be mitigated through the regular incorporation of organic matter, such as vermicompost, which serves to ameliorate these adverse soil conditions.
Qualitative properties of irrigation water
Table 2 provides a comprehensive overview of the physical and chemical properties of the irrigation water. The data in this table indicate that the irrigation water falls within the C2-S1 class. This classification signifies that the irrigation water is non-detrimental to crop growth and yield, rendering it suitable for application in this particular crop.
Experimental design
This research was conducted using a split-plot design based on a randomized complete block design (RCBD) with three replications over the course of two consecutive years. The primary factor consisted of four levels of irrigation (I1, I2, I3, and I4), corresponding to 50, 75, 100, and 125% of the quinoa crop’s water requirement, respectively. The sub-factor encompassed four levels of vermicompost fertilizer (F1, F2, F3, and F4), equating to 0, 5, 10, and 15 tons per hectare (t ha−1), respectively. It’s important to note that no chemical fertilizers were employed in this experiment, with sole reliance on vermicompost.
The dimensions of the sub-plots within the experimental design were set at 4 m in length and 3 m in width. The quinoa variety utilized in this study was Titicaca, selected for its suitability for cultivation in mountainous regions. The experimental site underwent initial plowing using a moldboard plow, reaching a depth of 30 cm. Prior to quinoa seed sowing, a disc implement was employed to break up soil clods and achieve uniform ground leveling. Following the plowing phase and the establishment of the experimental plot layout, vermicompost quantities allocated to each plot were meticulously measured and blended with the field soil to a depth of 20 cm using a manual tiller. Each sub-plot accommodated six rows with a spacing of 50 cm between them. Quinoa seeds were manually sown on the first of July in both years (Figure 1).
Characteristics of the irrigation system
A drip-tape irrigation system served as the chosen method for irrigation. The irrigation interval remained consistent at 7-day intervals, and the drip tapes were spaced at 50-cm intervals within each plot. To monitor the amount of irrigation water, a volumetric meter was installed on the main pipe. Additionally, shut-off valves were strategically positioned at the outset of the irrigation laterals to provide control and regulation over the volume of irrigation water entering each plot. The respective diameters of the main pipe, water supply, and drip tapes were 56, 32, and 15 mm.
To determine the depth of irrigation water, the soil moisture balance method, as outlined by Xu et al. (2016), was employed. In the initial year, prior to each irrigation event, soil water content within the root zone was assessed in the control plot (representing irrigation at 100% of the water requirement with zero tons of vermicompost) through daily measurements using a weighing method. Subsequently, the depth of irrigation water was calculated using Equation (1) as per Xu et al. (2016).
In the second year, automated soil moisture monitoring was implemented through the placement of 12 soil moisture meter sensors at depths of 10, 20, 30, 40, 50, and 60 cm from the soil surface within the control plot. Additionally, soil moisture content at field capacity was measured in the field by creating a 1 m2 plot, following the methodology established by Daillo and Marico (2013).
Measurement of phosphatase
A quantity of 1 g of soil was carefully transferred into a 50-ml Erlenmeyer flask. Subsequently, 25 mL of toluene, along with 4 mL of MUB1 buffer solution (maintaining pH levels at 6.5 for acid phosphatase and pH 11 for alkaline phosphatase), were added to the flask. Additionally, 1 mL of para-nitrophenyl phosphate (PNP2) solution, prepared in the designated buffer, was introduced into the mixture. Following this, the Erlenmeyer flasks were securely sealed, and their contents were meticulously agitated. The prepared samples were then incubated at a temperature of 37°C for a duration of 1 h.
Upon completion of the incubation period, 1 mL of 0.5 M CaCl2 and 4 mL of 0.5 M NaOH were introduced into the flask, and thorough mixing ensued. The resulting mixture was subsequently subjected to filtration through two layers of filter paper. To facilitate the measurement of absorbance, readings were obtained at a wavelength of 400 nm.
For the purpose of establishing controls, 1 mL of PNP solution was incorporated immediately before adding 0.5 M CaCl2, and these steps were repeated with three replicates for each measurement.
The calibration curves
A 1 mL volume of para-nitrophenol standard solution was meticulously dispensed into a 100 mL volumetric flask and subsequently adjusted to the flask’s capacity using distilled water. Following this, increments of 0, 1, 2, 3, 4, and 5 mL from the prepared solution were transferred into six separate 50 mL Erlenmeyer flasks. Each of these aliquots was adjusted to a total volume of 5 mL with the addition of distilled water.
The results were then computed employing the calibration curve to facilitate the determination of correction and the concentration of para-nitrophenol per milliliter.
Para-nitrophenol (μg/g−1 dwth−1) =
where C denotes the measured concentration of para-nitrophenol (micrograms per milliliter of filtered solution), dwt represents the dry weight of 1 g of wet soil, V represents the total volume of soil suspension (ml), sw is the weight of the soil sample (1 g), and t is the heating time.
Measurement of ammonium and nitrate
Ammonium and nitrate concentrations were determined through an extraction method employing a two-molar chloropotassium solution, as outlined by Mulvaney (1996).
Subsequent data analysis was conducted using SAS version 9.1. To compare treatment means, the LSD (Least Significant Difference) test was employed. Additionally, graphical representations of the data were generated using Microsoft Excel.
Results
The combined analysis of variance revealed significant effects of both irrigation and vermicompost on various soil parameters, including available phosphorus, ammonium, acid phosphatase, and soil basal respiration (Table 3). An increase in vermicompost application was associated with elevated levels of nitrate, potassium, and acidic phosphatase in the soil, while higher irrigation levels led to a decrease in ammonium, available potassium, soil electrical conductivity, and available phosphorus (Table 3).
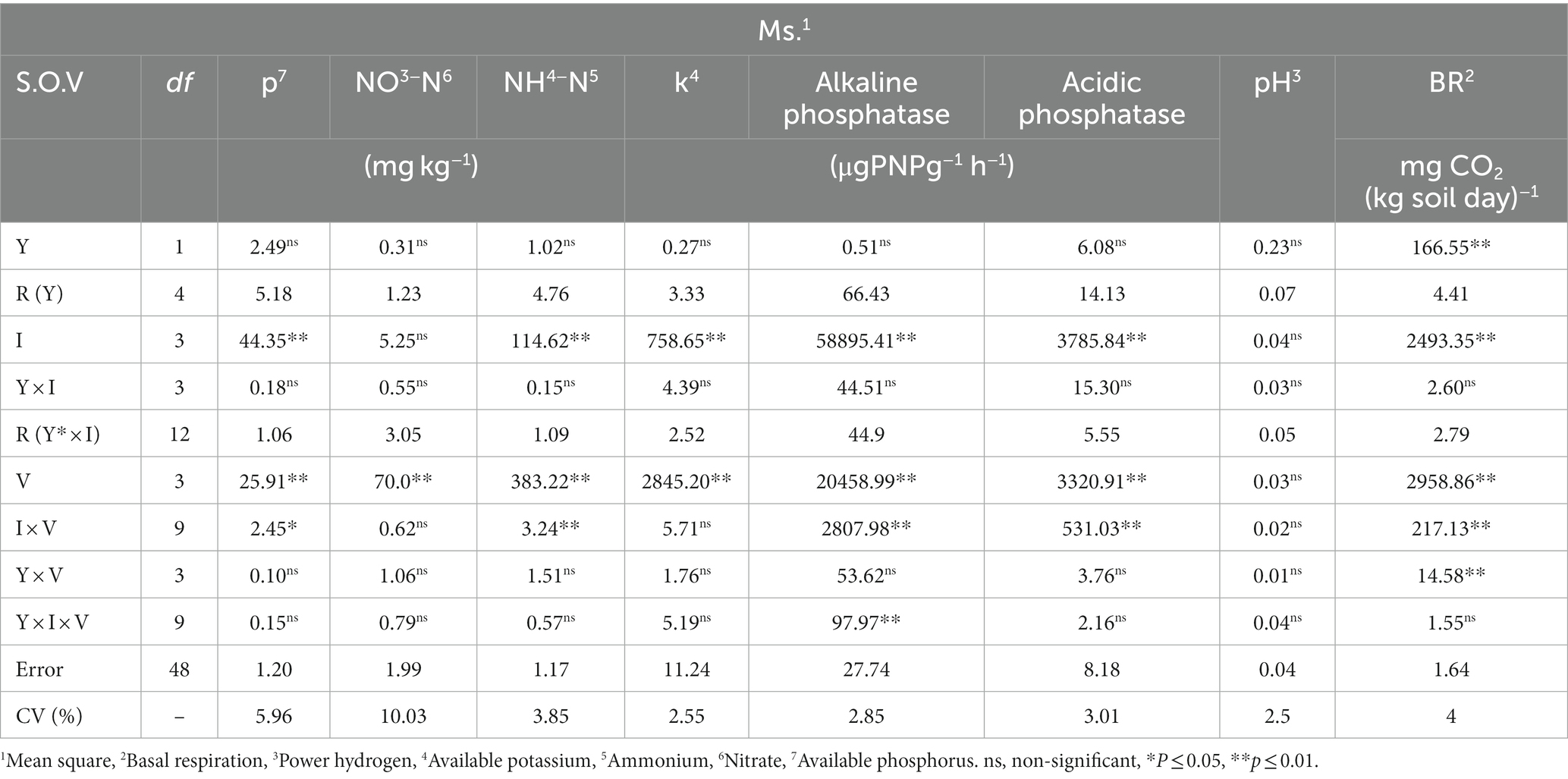
Table 3. Combined analysis of variance results for the soil parameters affected by applying different levels of irrigation and vermicompost in 2 years of experiment.
Available soil phosphorus
The interactive effects of year × irrigation × vermicompost were not statistically significant. The available soil phosphorus demonstrated sensitivity to the interaction between different levels of irrigation and vermicompost application. Specifically, an increase in soil water content resulted in a reduction of available soil phosphorus, whereas higher vermicompost levels led to increased soil phosphorus content (Figure 2).
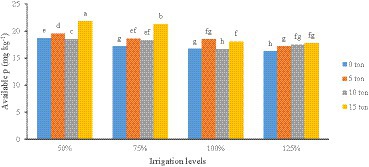
Figure 2. The effect of different levels of irrigation (50, 75, 100, and 125% of the plant’s water requirement) and vermicompost (0, 5, 10, and 15 tons per hectare) on available soil phosphorus in 2 years of experiment. The same letters indicate statistically insignificant differences (p < 0.05).
Soil nitrate
Over the 2021–2022 period, increasing vermicompost levels significantly raised soil nitrate content. The combined effects of irrigation × vermicompost and year × irrigation × vermicompost interactions were not statistically significant. Soil nitrogen content ranged from 12.02 mg kg−1 in treatments without vermicompost to 16.14 mg kg−1 when 15 tons per hectare of vermicompost was applied. No significant differences were observed between the use of 5 and 10 tons per hectare of vermicompost (Figure 3).
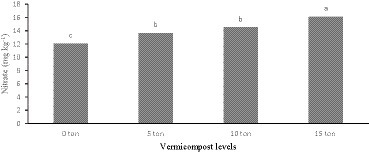
Figure 3. The effect of applying different levels of vermicompost (0, 5, 10, and 15 tons per hectare) on soil nitrate in 2 years of experiment. The same letters indicate statistically insignificant differences (p < 0.05).
Soil ammonium content
The addition of varying proportions of vermicompost to quinoa-growing soil led to an increase in soil ammonium content. The triple interaction effect of year × irrigation × vermicompost was not statistically significant. Soil ammonium content in water stress treatments exceeded that in treatments with adequate water for the crop. The highest soil ammonium levels were observed in the treatment receiving 50% of the plant’s water requirement and 15 tons per hectare of vermicompost (Figure 4).
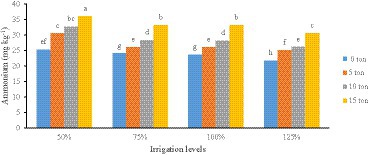
Figure 4. The effect of different levels of irrigation (50, 75, 100, and 125% of the plant’s water requirement) and vermicompost (0, 5, 10, and 15 tons per hectare) on soil ammonium content in 2 years of experiment. The same letters indicate statistically insignificant differences (p < 0.05).
Available potassium
The results highlighted that available soil potassium content was significantly affected by irrigation and vermicompost application (p < 0.001). However, the interaction effect of irrigation × vermicompost was not statistically significant (p < 0.001 and p < 0.05). Soil potassium content ranged from 137.54 mg kg−1 in the 50% irrigation stress treatment to 118.97 mg kg−1 in the treatment without vermicompost (Table 4).
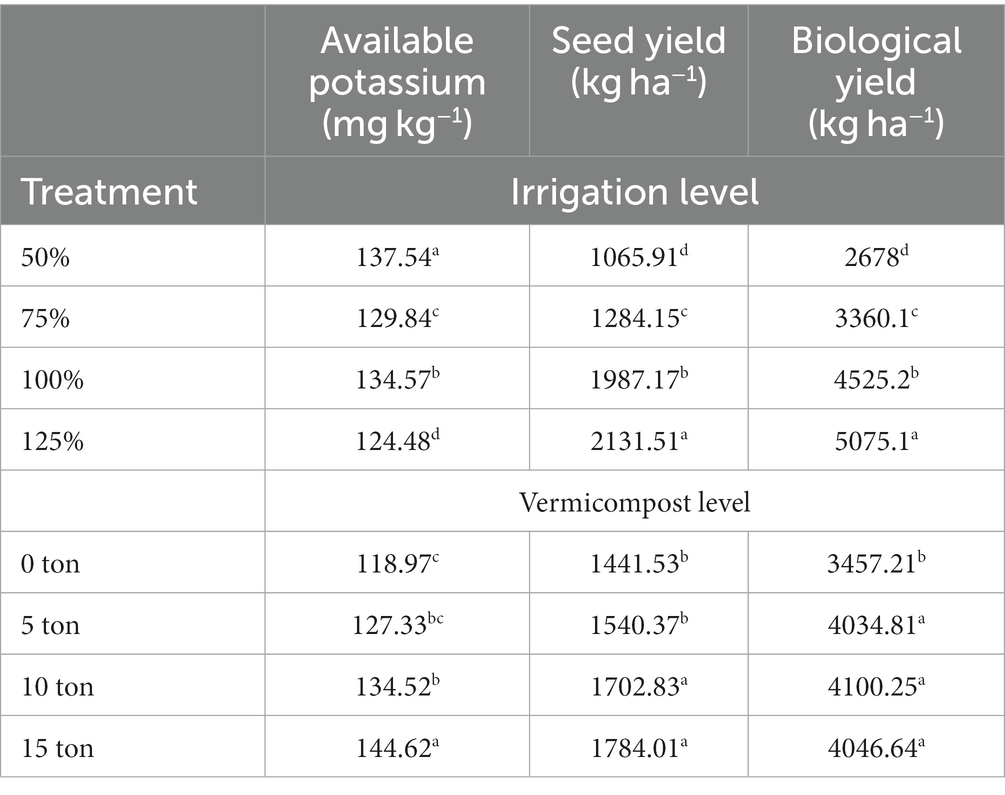
Table 4. Means comparing irrigation and vermicompost effects on the soil potassium concentration, seed yield, and biological yield of quinoa in 2 years of experiment.
Alkaline phosphatase
Figure 5 illustrates alkaline phosphatase activity, with the highest enzyme activity observed at the highest irrigation level (125% of water requirement) and the application of 15 t ha−1 of vermicompost in both years of the experiment. Increasing vermicompost in water stress treatments (50 and 75% of the water requirement) did not significantly affect alkaline phosphatase activity during 2021–2022. However, in treatments with ample water supply for the plants, increased vermicompost significantly enhanced enzyme activity (Figure 5).
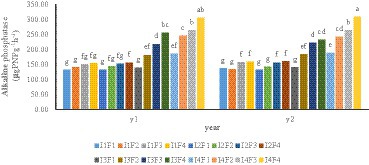
Figure 5. The effect of different levels of year and irrigation (50, 75, 100, and 125% of the plant’s water requirement) and vermicompost (0, 5, 10, and 15 tons per hectare) on alkaline phosphatase in 2 years of experiment. I1F1, 50% plant water requirement + no use of vermicompost; I1F2, 50% of plant water requirement +5 tons per hectare of vermicompost; I1F3, 50% of plant water requirement +10 tons per hectare of vermicompost; I1F4, 50% of plant water requirement +15 tons per hectare of vermicompost; I2F1, 75% of plant water requirement + no use of vermicompost; I2F2, 75% of plant water requirement +5 tons per hectare of vermicompost; I2F3, 75% of plant water requirement +10 tons per hectare of vermicompost; I2F4, 75% of plant water requirement +15 tons per hectare of vermicompost. I3F1, 100% plant water requirement + no use of vermicompost; I3F2, 100% of plant water requirement +5 tons per hectare of vermicompost; I3F3, 100% of plant water requirement +10 tons per hectare of vermicompost; I3F4, 100% of plant water requirement +15 tons per hectare of vermicompost. I4F1, 125% plant water requirement + no use of vermicompost; I4F2, 125% of plant water requirement +5 tons per hectare of vermicompost; I4F3, 125% of plant water requirement +10 tons per hectare of vermicompost; I4F4, 125% of plant water requirement +15 tons per hectare of vermicompost. The same letters indicate statistically insignificant differences (p < 0.05).
Acidic phosphatase
While the interaction effects of year × irrigation, year × vermicompost, and year × irrigation × vermicompost were not statistically significant, the effect of vermicompost × irrigation showed significance (p < 0.001) (Table 3). Across all irrigation treatments, acid phosphatase activity increased with the addition of vermicompost (Figure 6).
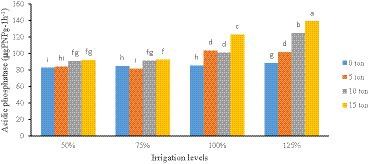
Figure 6. The effect of different levels of irrigation (50, 75, 100, and 125% of the plant’s water requirement) and vermicompost (0, 5, 10, and 15 tons per hectare) on soil acidic phosphatase in 2 years of experiment. The same letters indicate statistically insignificant differences (p < 0.05).
pH
Experimental factors did not exert a significant influence on soil pH (p < 0.001 and p < 0.05). Specifically, plots receiving vermicompost showed no significant differences in pH across all irrigation levels (Table 3).
Basal respiration
Both irrigation and vermicompost treatments significantly impacted soil basal respiration during the 2021 and 2022 growth periods (p < 0.001). Notably, the triple interaction effect of year × irrigation × vermicompost was not statistically significant. Basal respiration ranged from 17.95 mg CO2 (kg soil day)−1 for treatments without vermicompost and under 50% of plant water requirement stress to 59.09 mg CO2 (kg soil day)−1 for treatments with 15 tons of vermicompost and 125% water requirement. Soil basal respiration increased with higher vermicompost applications across all irrigation levels (Figure 7).
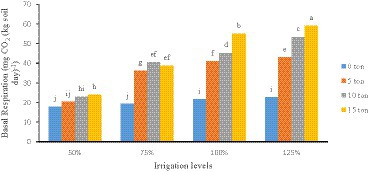
Figure 7. The effect of different levels of irrigation (50, 75, 100, and 125% of the plant’s water requirement) and vermicompost (0, 5, 10, and 15 tons per hectare) on soil basal respiration in 2 years of experiment. The same letters indicate statistically insignificant differences (p < 0.05).
Seed yield
Statistical analysis demonstrated that seed yield was significantly influenced by both irrigation and vermicompost levels (p < 0.001). The interaction of irrigation × vermicompost was not statistically significant for quinoa seed yield (p < 0.001 and p < 0.05). The highest seed yield of 2,131.51 kg ha−1 was achieved when quinoa plants were irrigated with 125% of their water requirement. Moreover, the maximum quinoa seed yield of 1,884.01 kg ha−1 was recorded with the application of 15 t ha−1 of vermicompost (Table 5). The utilization of vermicompost significantly increased grain yield compared to scenarios without vermicompost across all irrigation levels.
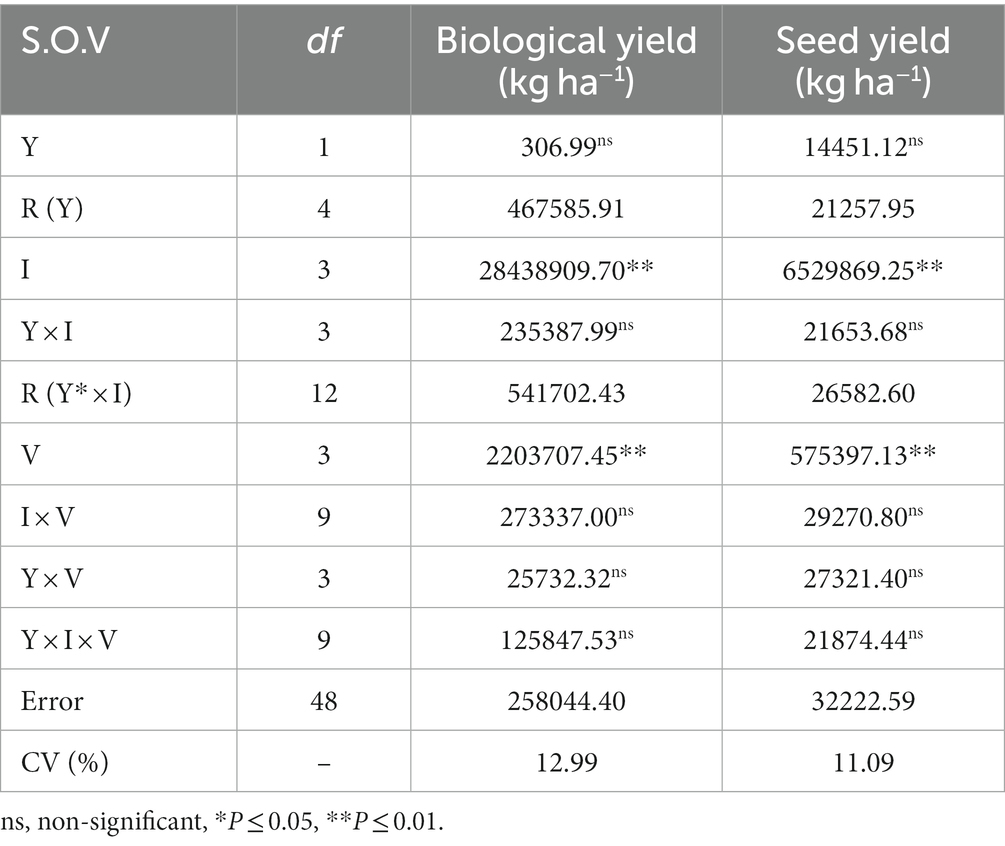
Table 5. Combined analysis of variance results for the biological and seed yield affected by applying different levels of irrigation and vermicompost in 2 years of experiment
Biological yield
The results of the analysis of variance (ANOVA) revealed that the biological yield of quinoa was significantly influenced by the effects of irrigation and vermicompost (p < 0.001). Notably, the double interaction effect of irrigation × vermicompost and the triple interaction effect of year × irrigation × vermicompost were not statistically significant.
Discussion
The application of vermicompost significantly influenced the available phosphorus content of the soil. This outcome is consistent with previous research highlighting the positive effects of vermicompost on soil nutrient enhancement (Demir, 2019). The gradual release of phosphorus from vermicompost, coupled with microbial activity in the soil, contributes to sustained phosphorus input (Arancon et al., 2006). During the breakdown of vermicompost, organic matter decomposition leads to the release of humic acid, converting previously unavailable soil phosphate into accessible forms (Demir, 2019). Additionally, vermicompost enhances soil phosphorus availability by activating microorganisms and increasing phosphatase activity (Azarmi et al., 2008). Several mechanisms underpin the beneficial effects of organic fertilizers, such as vermicompost, on available soil phosphorus, including the production of carbonic acid during organic matter decomposition, the formation of easily plant-absorbable humic phosphorus compounds, the displacement of adsorbed phosphates with phosphate ion release, organic compound competition with phosphate ions for adsorption sites on calcium carbonate particles, and organic matter coating of clay and iron/aluminum oxide surfaces (Jami et al., 2019). Previous studies corroborate our findings, highlighting vermicompost’s ability to increase available soil phosphorus (Sabijon and Sudaria, 2018; Baghbani-Arani et al., 2021). Irrigation water increase led to a decrease in available soil phosphorus, likely due to increased nutrient uptake by crops and phosphorus leaching beyond the reach of plant roots (Salmanzadeh et al., 2017). Our results align with these previous studies (Abdrabbo et al., 2015; Salmanzadeh et al., 2017).
Organic fertilizers, particularly vermicomposts, contain substantial nutrient percentages, especially nitrogen, which contributes to increased soil nitrate and ammonium levels. This elevation improves soil quality and enhances plant nitrogen accessibility (Abd–Elrahman et al., 2022). While Demir (2019) reported non-significant changes in soil nitrate levels due to irrigation, vermicompost additions increased soil nitrate, consistent with our findings. Soil ammonium content was higher under water stress conditions, with an inverse relationship observed with increasing irrigation levels. Water stress conditions limit water uptake by plant roots, leading to reduced photosynthesis, transpiration, and nutrient uptake (Nyawade et al., 2021). Organic fertilizers promote root network expansion, enhancing nutrient uptake and water access under such conditions (Bistgani et al., 2018). The co-occurrence of water deficit and osmotic stress due to reduced soil matrix potential can result in ionic imbalances and nutrient deficiencies (Nyawade et al., 2021). Organic fertilizers may mitigate these effects by extending root networks, improving nutrient uptake, and creating more favorable conditions for water uptake. Thus, soil potassium content was higher in stress treatments compared to well-irrigated conditions. The substantial potassium content in the applied vermicompost may have contributed to increased soil potassium levels post-harvest. Organic acids present in organic fertilizers also play a role in enhancing soil element concentrations, including potassium (Adak et al., 2014). These results concur with previous research (Demir, 2019; Rani et al., 2020).
Soil enzyme activity serves as a valuable indicator of microbial activity, soil biochemical reactions, and overall soil quality, given its correlation with other soil quality parameters. Acid and alkaline phosphatase activities are closely associated with soil organic matter content (Zhang et al., 2018). Adding organic fertilizers, including vermicompost, can augment enzyme activity. Enzyme activity is also highly sensitive to soil moisture levels, with increased humidity promoting enzyme activity (Hu and Cao, 2007). Increased phosphatase enzyme activity may be attributed to enhanced microbial populations resulting from vermicompost application, the presence of phosphate-dissolving bacteria, organic matter supply, and subsequent microbial sustenance (Zhao et al., 2019). Organic matter type significantly impacts soil enzyme activity, with high organic carbon, nitrogen, and phosphorus contents stimulating microbial activity and thus increasing enzyme activity. Organic matter may stabilize and protect enzyme molecules from various stressors (Zhao et al., 2019).
Contrary to some studies, our results did not demonstrate significant changes in soil pH due to organic fertilizer application (Parthasarathi et al., 2008). The absence of pH alteration may be attributed to carbonic acid and organic acid production during organic matter decomposition, which can counteract any pH shifts caused by organic matter addition. Our results showed that vermicompost improved soil basal respiration and some enzyme activities. The enhancement of these soil microbial indicators, following greater application rates, strongly indicated the benefit of vermicompost for soil microorganisms and their functioning. Vermicompost has been shown to provide a wide diversity of carbon and nutrient substrates for soil microorganisms (Lv et al., 2020). Soil respiration is regulated by two factors: the respiratory quotient and the total biomass of soil microorganisms. We postulated that vermicompost would increase the respiratory quotient by supplying soil microorganisms with available carbon and nutrients. This is because many microorganisms in the soil are dormant, and they await favorable conditions to become active (Lavelle et al., 1992).
Soil respiration, a reflection of biological activity, is a critical indicator of soil health. Organic materials augment soil fertility, storage capacity, and porosity, enhancing nutrient release over time and promoting plant accessibility. Thus, organic fertilizers increase both nutrient supply and water availability, fostering greater biomass production (Singer et al., 2007). Increased soil microbial and enzyme activity resulting from organic matter and nutrient addition can lead to higher soil respiration rates (Hale et al., 2021). Water content reductions in soil can limit root water uptake, subsequently diminishing photosynthesis and transpiration, while active mobilization systems curtail energy conservation. These combined factors result in a substantial reduction in nutrient uptake capacity (Bistgani et al., 2018). In water deficit conditions, plants face both water and osmotic stress due to reduced soil matrix potential, leading to ionic imbalances and nutrient deficiency (Nyawade et al., 2021). Organic fertilizers like vermicompost extend root networks, enhancing nutrient uptake and water access. As a result, soil potassium content is higher under stress conditions compared to well-irrigated ones (Jbawi et al., 2018).
In our study, we found that vermicompost and mineral fertilizer had a positive effect on increasing the concentration of nitrogen and phosphorus. This is because vermicompost contributes to the release of nutrients, acting as an improver of soil properties and providing nutrients to the soil. Nitrogen and phosphorus play a role in increasing the effectiveness of plants in carrying out the process of carbon metabolism and enhancing nutrient absorption. Additionally, potassium regulates the osmotic activity of plant cells and controls the opening and closing of stomata (Kadam et al., 2018). Vermicompost contributes to the balance in plant absorption, promoting the availability of phosphorus in the soil. This is due to the presence of an adequate quantity of mineral phosphorus that is readily absorbed by the plant. Furthermore, vermicompost plays a role in enhancing soil properties and contains essential elements such as phosphorus and potassium, in addition to its secretion of organic acids. These organic acids work to reduce the degree of soil interaction and mineral analysis while releasing elements such as potassium and phosphorous (Chandra, 2015). Vermicompost’s positive impact can be attributed to its nutrient-rich composition, which promotes the availability of macro- and microelements, especially under optimal moisture conditions for crops. High humic substance content in vermicompost compounds enhances nutrient supply, particularly zinc and iron, bolstering plant metabolism and yield (Kadam et al., 2018). Organic fertilizers significantly improve soil physicochemical properties, and vermicompost has been shown to boost crop yield and growth across various soil types (El-Gamal et al., 2020).
The accumulation of photosynthetic materials and a decline in relative growth rate, driven by insufficient water availability, contribute to reduced dry matter production, possibly explaining the decrease in biological yield under moisture stress conditions (Jbawi et al., 2018). Organic materials increase soil fertility, storage capacity, and porosity, releasing nutrients over time for plant uptake. Consequently, organic fertilizers enhance nutrient availability while improving water access, ultimately promoting biomass production (Singer et al., 2007). Vermicompost’s influence on root growth, coupled with nutrient supply, may contribute to increased potassium and nitrogen absorption. Moreover, the high nutrient and organic matter content in vermicompost enhances soil cation exchange capacity, improving nutrient access for crops. Growth-promoting hormones present in vermicompost further support crop growth and biological yield. Various necessary plant nutrients like nitrogen, phosphorus, and potassium and various plant growth substances like auxins, gibberellins, cytokinins, and humic acids are naturally present in vermicompost in a form that can be easily absorbed by plants. As a result, the yield and quality of crops are improved. Additionally, vermicompost promotes root growth, which facilitates efficient water and nutrient absorption from the soil. This is attributed to the presence of hormone substances in vermicompost (Nauman et al., 2020). The results of this study support the hypothesis that the application of vermicompost organic manures can improve the productivity and quality of Quinoa. Vermicompost application was associated with increased biological yield in our study, aligning with previous findings (Wesseling et al., 2019).
Conclusion
The findings of this study underscore the substantial benefits of vermicompost application in enhancing soil nutrient levels. Specifically, the highest concentrations of available phosphorus, potassium, nitrate, and ammonium were observed in plots treated with 15 tons per hectare of vermicompost. Notably, soil elements were more abundant in drought stress treatments compared to well-irrigated conditions and those receiving 125% of the crop’s water requirement. This outcome can be attributed to reduced nutrient absorption by crops under drought stress, accompanied by decreased leaching of elements from the soil. Consequently, soil element concentrations were higher in drought stress treatments, where water availability for crops was limited.
Furthermore, increased vermicompost usage contributed to elevated organic matter and carbon content in the soil, providing a conducive substrate for heightened soil basal respiration. Soil microbial activity and basal respiration were particularly enhanced in treatments with adequate water supply, where organic carbon decomposition was more pronounced.
Phosphatase enzyme activity displayed an upward trend with increasing irrigation levels and vermicompost application. The highest seed yield, amounting to 2,131.51 kg ha−1, and biological yield, reaching 5,075.10 kg ha−1, were achieved in treatments providing 125% of the crop’s water requirement. This underscores the efficacy of vermicompost as a nutrient-rich substrate, gradually releasing elements to support crop growth and yield. Additionally, vermicompost application alleviated the impact of drought stress by improving soil physical and chemical properties, thereby enhancing soil moisture retention.
Considering the prevailing deficiency of organic matter in the majority of Iran’s agricultural soils, we recommend the widespread use of fertilizers like vermicompost. Such practices can not only ameliorate soil physical and chemical characteristics but also augment crop yield and soil nutrient concentrations, contributing to sustainable agricultural practices in the region.
Data availability statement
The original contributions presented in the study are included in the article/supplementary material, further inquiries can be directed to the corresponding authors.
Author contributions
DS: Methodology, Resources, Software, Writing – original draft.GH: Data curation, Methodology, Supervision, Writing – original draft, Writing – review & editing. PF: Methodology, Software, Supervision, Writing – review & editing. HK: Data curation, Formal analysis, Writing – review & editing. ZS: Data curation, Investigation, Writing – review & editing.
Funding
The author(s) declare that no financial support was received for the research, authorship, and/or publication of this article.
Conflict of interest
The authors declare that the research was conducted in the absence of any commercial or financial relationships that could be construed as a potential conflict of interest.
Publisher’s note
All claims expressed in this article are solely those of the authors and do not necessarily represent those of their affiliated organizations, or those of the publisher, the editors and the reviewers. Any product that may be evaluated in this article, or claim that may be made by its manufacturer, is not guaranteed or endorsed by the publisher.
Footnotes
References
Abd–Elrahman, S. H., Saudy, H. S., el–Fattah, D. A. A., and Hashem, F. A.-E. (2022). Effect of irrigation water and organic fertilizer on reducing nitrate accumulation and boosting lettuce productivity. J. Soil Sci. Plant Nutr. 22, 2144–2155. doi: 10.1007/s42729-022-00799-8
Abdrabbo, M. A. A., Hashem, F. A., Abul-Soud, M. A., and Abd-Elrahman, S. H. (2015). Sustainable production of cabbage using different irrigation levels and fertilizer types affecting some soil chemical characteristics. Int. J. Plant Soil Sci. 8, 1–13. doi: 10.9734/IJPSS/2015/17590
Adak, T., Singha, A., Kumar, K., Shukla, S. K., Singh, A., and Kumar Singh, V. (2014). Soil organic carbon, dehydrogenase activity, nutrient availability and leaf nutrient content as affected by organic and inorganic source of nutrient in mango orchard soil. J. Soil Sci. Plant Nutr. 14, 394–406. doi: 10.4067/S0718-95162014005000031
Adekiya, A. O., and Agbede, T. M. (2017). Effect of methods and time of poultry manure application on soil and leaf nutrient concentrations, growth and fruit yield of tomato (Lycopersicon esculentum mill). J. Saudi Soc. Agric. Sci. 16, 383–388. doi: 10.1016/j.jssas.2016.01.006
Agarwal, A., Rizwana, T., Tripathi, A. D., Kumar, T., Sharma, K. P., and Patel, S. K. S. (2023). Nutritional and functional new perspectives and potential health benefits of quinoa and chia seeds. Antioxidants 12:1413. doi: 10.3390/antiox12071413
Aksakal, E. L., Sari, S., and Angin, I. (2016). Effects of vermicompost application on soil aggregation and certain physical properties. Land Degrad. Dev. 27, 983–995. doi: 10.1002/ldr.2350
Arancon, N. Q., Edwards, C. A., and Bierman, P. (2006). Influences of vermicomposts on field strawberries: part 2. Effects on soil microbiological and chemical properties. Bioresour. Technol. 97, 831–840. doi: 10.1016/j.biortech.2005.04.016
Azarmi, R., Giglou, M. T., and Taleshmikail, R. D. (2008). Influence of vermicompost on soil chemical and physical properties in tomato (Lycopersicum esculentum) field. Afr. J. Biotechnol. 7:8.
Baghbani-Arani, A., Modarres-Sanavy, S. A. M., and Poureisa, M. (2021). Improvement the soil physicochemical properties and fenugreek growth using zeolite and vermicompost under water deficit conditions. J. Soil Sci. Plant Nutr. 21, 1213–1228. doi: 10.1007/s42729-021-00452-w
Bistgani, Z. E., Siadat, S. A., Bakhshandeh, A., Pirbalouti, A. G., Hashemi, M., Maggi, F., et al. (2018). Application of combined fertilizers improves biomass, essential oil yield, aroma profile, and antioxidant properties of Thymus daenensis Celak. Ind. Crop. Prod. 121, 434–440. doi: 10.1016/j.indcrop.2018.05.048
Bozkurt Çolak, Y., Yazar, A., Alghory, A., and Tekin, S. (2021). Evaluation of crop water stress index and leaf water potential for differentially irrigated quinoa with surface and subsurface drip systems. Irrig. Sci. 39, 81–100. doi: 10.1007/s00271-020-00681-4
Chandra, G. (2015). Influence of vermicompost on growth, yield and processing quality of potato varieties. MS thesis faculty of agriculture Sher- e-Bangla Agriculture University, Dhaka.
Daillo, D., and Marico, A. (2013). Field capacity (FC) and permanent wilty point (PWP) of clay soils developed on quaternary alluvium in Niger river loop (Mali). Int. J. Eng. Res. Ind. Appl. 3, 1085–1089.
Demir, Z. (2019). Effects of vermicompost on soil physicochemical properties and lettuce (Lactuca sativa Var. Crispa) yield in greenhouse under different soil water regimes. Commun. Soil Sci. Plant Anal. 50, 2151–2168. doi: 10.1080/00103624.2019.1654508
Dhawan, V. (2017). “Water and agriculture in India” in Background paper for the South Asia expert panel during the Global Forum for Food and Agriculture, vol. 28, 80–85.
El-Gamal, B. A., Abu El-Fotoh, H. M., and Hamed, M. A. (2020). Impact of organic and bio-fertilizers on soil health and production of quinoa and soybean. Middle East. J. Agric. Res. 9, 828–847. doi: 10.36632/mejar/2020.9.4.65
FAO (2012). Available at: https://www.irc.fao.org/en/about-faoiyq.
Feller, C., Blanchart, E., Bernoux, M., Lal, R., and Manlay, R. (2012). Soil fertility concepts over the past two centuries: the importance attributed to soil organic matter in developed and developing countries. Arch. Agron. Soil Sci. 58, S3–S21. doi: 10.1080/03650340.2012.693598
Galvez, A. V., Miranda, M., Vergara, J., Uribe, E., Puente, L., and Martinez, E. A. (2010). Nutrition facts and functional potential of quinoa (Chenopodium quinoa Willd.), an ancient Andean grain: a review. J. Sci. Food Agric. 90, 2541–2547. doi: 10.1002/jsfa.4158
Hale, L., Curtis, D., Azeem, M., Montgomery, J., Crowley, D. E., and McGiffen, M. E. Jr. (2021). Influence of compost and biochar on soil biological properties under turfgrass supplied deficit irrigation. Appl. Soil Ecol. 168:104134. doi: 10.1016/j.apsoil.2021.104134
Hemalatha, P., Bomzan, D. P., Rao, B. S., and Sreerama, Y. N. (2016). Distribution of phenolic antioxidants in whole and milled fractions of quinoa and their inhibitory effects on α-amylase and α-glucosidase activities. Food Chem. 199, 330–338. doi: 10.1016/j.foodchem.2015.12.025
Hu, C., and Cao, Z. (2007). Size and activity of the soil microbial biomass and soil enzyme activity in long-term field experiments. World J. Agric. Sci. 3, 63–70.
Irmak, S., Djaman, K., and Rudnick, D. R. (2016). Effect of full and limited irrigation amount and frequency on subsurface drip-irrigated maize evapotranspiration, yield, water use efficiency and yield response factors. Irrig. Sci. 34, 271–286. doi: 10.1007/s00271-016-0502-z
Jabeen, N., and Ahmad, R. (2019). Growth response and nitrogen metabolism of sunflower (Helianthus annuus L.) to vermicompost and biogas slurry under salinity stress. J. Plant Nutr. 40, 104–114. doi: 10.1080/01904167.2016.1201495
Jami, M. G., Baghbani-Arani, A., Karami Borz-Abad, R., and Saadatkhah, A. (2019). Towards improving the vegetative and qualitative traits of sunflower using amending soil (zeolite and manure farmyard) under water deficit stress. Commun. Soil Sci. Plant Anal. 50, 2227–2237. doi: 10.1080/00103624.2019.1651329
Jan, N., Hussain, S. Z., Naseer, B., and Bhat, T. A. (2023). Amaranth and quinoa as potential nutraceuticals: a review of anti-nutritional factors, health benefits and their applications in food, medicinal and cosmetic sectors. Food Chem X 18:100687. doi: 10.1016/j.fochx.2023.100687
Jbawi, E. A., Danoura, R., and Yaacoub, A. (2018). Effect of deficit irrigation and manure fertilizer on improving growth and yield of quinoa in Syria. Open Acc. J. Agric. Res. OAJAR-100007, 01.
Kadam, V. P., Devi, K. S., Hussain, S. A., and Devi, M. U. (2018). Growth, yield attributes, yield and economics of quinoa (Chenopodium quinoa Willd.) as influenced by variable irrigation water supply through drip and surface methods. Int. J. Curr. Microbiol. Appl. Sci. 7, 3428–3438. doi: 10.20546/ijcmas.2018.707.398
Lavelle, P., Melendez, G., Pashanasi, B., and Schaefer, R. (1992). Nitrogen mineralization and reorganization in casts of the geophagous tropical earthworm Pontoscolex corethrurus (Glossoscolecidae). Biol. Fertil. Soils 14, 49–53. doi: 10.1007/BF00336302
Linday, W. L., and Norvell, W. A. (1978). Development of a DTPA soil test for zinc, Iron, manganese, and copper. Soil Sci. Soc. Am. J. 42, 421–428. doi: 10.2136/sssaj1978.03615995004200030009x
Liu, X., Li, S., He, P., Zhang, P., and Duan, Y. (2018). Yield and nutrient gap analysis for potato in Northwest China. J. Agric. Sci. 156, 971–979. doi: 10.1017/S0021859618000916
Lv, M., Li, J., Zhang, W., Zhou, B., Dai, J., and Zhang, C. (2020). Microbial activity was greater in soils added with herb residue vermicompost than chemical fertilizer. Soil Ecol. Lett. 2, 209–219. doi: 10.1007/s42832-020-0034-6
Mengistu, T., Gebrekidan, H., Kibret, K., Woldetsadik, K., Shimelis, B., and Yadav, H. (2017). The integrated use of excreta-based vermicompost and inorganic NP fertilizer on tomato (Solanum lycopersicum L.) fruit yield, quality and soil fertility. Int. J. Recycl. Org. Waste Agric. 6, 63–77. doi: 10.1007/s40093-017-0153-y
Mulvaney, R. L. (1996). “Nitrogen-inorganic forms” in Methods of soil analysis. Part 2. Chemical properties eds. D. L. Sparks, A. L. Page, P. A. Siddiq, M. Helmke, R. H. Loeppert, and P. N. Soltanpour, et al. (Madison, WI: Soil Science Society of America), 1123–1184.
Nauman, A., Khan, M. N., Ashraf, M. S., Ijaz, S., Saeed-ur-Rehman, H., Abdullah, M., et al. (2020). Influence of different organic manures and their combinations on productivity and quality of bread wheat. J. Soil Sci. Plant Nutr. 12, 145–161.
Nyawade, S. O., Gitari, H. I., Karanja, N. N., Gachene, C. K., Schulte-Geldermann, E., and Parker, M. L. (2021). Yield and evapotranspiration characteristics of potato-legume intercropping simulated using a dual coefficient approach in a tropical highland. Field Crop Res. 274:108327. doi: 10.1016/j.fcr.2021.108327
Olsen, S. R., and Sommers, L. E. (1990). “Phosphorus” in Method of soil analysis. Part 2. 2nd agron Monoger. ed. A. L. Page (Madison, WI: ASA), 403–431.
Ortas, I., and Lal, R. (2014). Long-term fertilization effect on agronomic yield and soil organic carbon under semi-arid Mediterranean region. Am. J. Exp. Agric. 4, 1086–1102. doi: 10.9734/AJEA/2014/8670
Pahalvi, H. N., Rafiya, L., Rashid, S., Nisar, B., and Kamili, A. N. (2021). Chemical fertilizers and their impact on soil health. Microb. Biofert. 2, 1–20. doi: 10.1007/978-3-030-61010-4_1
Parthasarathi, K., Balamurugan, M., and Ranganathan, L. S. (2008). Influence of vermicompost on the physico-chemical and biological properties in different types of soil along with yield and quality of the pulse crop-blackgram. J. Environ. Health Sci. Eng. 5, 51–58.
Pathan, S., and Siddiqui, R. A. (2022). Nutritional composition and bioactive components in quinoa (Chenopodium quinoa Willd.) greens: a review. Nutrients 14:558. doi: 10.3390/nu14030558
Rani, P., Batra, V. K., Bhatia, A. K., and Sain, V. (2020). Effect of water deficit and fertigation on nutrients uptake and soil fertility of drip irrigated onion (Allium cepa L.) in semi-arid region of India. J. Plant Nutr. 44, 765–772. doi: 10.1080/01904167.2020.1860220
Rathore, S., and Kumar, R. (2021). Vermicompost fertilization and pinching improves the growth, yield, and quality of super food (Chenopodium quinoa Willd.) in the western Himalaya. Acta Physiol. Plant. 43, 234–251. doi: 10.1007/s11738-020-03184-z
Sabijon, J., and Sudaria, M. A. (2018). Effect of vermicompost amendment and nitrogen levels on soil characteristics and growth and yield of tomato (Solanum lycopersicum cv. Diamante max). Int. J. Agric. For. Life Sci. 2, 145–153.
Salmanzadeh, M., Schipper, L. A., Balks, M. R., Hartland, A., Mudge, P. L., and Littler, R. (2017). The effect of irrigation on cadmium, uranium, and phosphorus contents in agricultural soils. Agric. Ecosyst. Environ. 247, 84–90. doi: 10.1016/j.agee.2017.06.028
Sangwan, P., Kaushik, C. P., and Garg, V. K. (2018). Feasibility of utilization of horse dung spiked filter cake in vermicomposters using exotic earthworm Eisenia foetida. Bioresour. Technol. 99, 2442–2448. doi: 10.1016/j.biortech.2007.04.061
Singer, J. W., Logsdon, S. D., and Meek, D. W. (2007). Tillage and compost effects on corn growth, nutrient accumulation, and grain yield. Agron. J. 99, 80–87. doi: 10.2134/agronj2006.0118
Tascı, F. G., and Kuzucu, C. O. (2023). The effects of vermicompost and green manure use on yield and economic factors in broccoli. Horticulturae 9:406. doi: 10.3390/horticulturae9030406
Thakur, N., Manjeet, G. K., and Kumar Jain, P. (2021). The impact of vermicompost application on yield of maize (Zea mays). Int. J. Res. Agron. 4, 11–13. doi: 10.33545/2618060X.2021.v4.i1a.43
Valentín, F., Nortes, P. A., Domínguez, A., Sánchez, J. M., Intrigliolo, D. S., Alarcón, J. J., et al. (2020). Comparing evapotranspiration and yield performance of maize under sprinkler, superficial and subsurface drip irrigation in a semi-arid environment. Irrig. Sci. 38, 105–115. doi: 10.1007/s00271-019-00657-z
Wesseling, J. G., Stoof, C. R., Ritsema, C. J., Oostindie, K., and Dekker, L. W. (2019). The effect of soil texture and organic amendment on the hydrological behaviour of coarse-textured soils. Soil Use Manag. 25, 274–283. doi: 10.1111/j.1475-2743.2009.00224.x
Xu, X., He, P., Pampolino, M. F., Li, Y., Liu, S., Xie, J., et al. (2016). Narrowing yield gaps and increasing nutrient use efficiencies using the nutrient expert system for maize in Northeast China. Field Crop Res. 194, 75–82. doi: 10.1016/j.fcr.2016.05.005
Zhang, S., Qin, Y., Zhu, J., and Hou, J. (2018). Over 14% efficiency in polymer solar cells enabled by a chlorinated polymer donor. Adv. Mater. 30:e1800868. doi: 10.1002/adma.201800868
Keywords: irrigation, quinoa, organic fertilizer, available phosphorus, vermicompost
Citation: Sanandaji DS, Heidari G, Fathi P, Khodaverdiloo H and Sharifi Z (2024) Soil properties and quinoa yield as affected by irrigation and vermicompost application under a drip-tape irrigation system. Front. Sustain. Food Syst. 8:1277934. doi: 10.3389/fsufs.2024.1277934
Edited by:
Ashish Kumar Gupta, Indian Council of Agricultural Research, IndiaReviewed by:
Dr Sandeep Kumar Sihag, Indian Agricultural Research Institute (ICAR), IndiaShiveshwar Pratap Singh, Rajendra Prasad Central Agricultural University, India
Nicole Nunoo, University of Georgia, United States
Copyright © 2024 Sanandaji, Heidari, Fathi, Khodaverdiloo and Sharifi. This is an open-access article distributed under the terms of the Creative Commons Attribution License (CC BY). The use, distribution or reproduction in other forums is permitted, provided the original author(s) and the copyright owner(s) are credited and that the original publication in this journal is cited, in accordance with accepted academic practice. No use, distribution or reproduction is permitted which does not comply with these terms.
*Correspondence: Gholamreza Heidari, g.heidari@uok.ac.ir; Parviz Fathi, p.fathi@uok.ac.ir