- 1College of Food Science, Southwest University, Chongqing, China
- 2Guizhou Provincial Academy of Agricultural Sciences, Guiyang, China
- 3State Key Laboratory of Southwestern Chinese Medicine Resources, Chengdu University of Traditional Chinese Medicine, Chengdu, China
The objective of the present study was to evaluate structure–activity relationships of four sanshools on antioxidant activities. In vitro, hydroxy-α-sanshool (HAS), hydroxy-β-sanshool (HBS), and hydroxy-γ-sanshool (HRS) had stronger antioxidant capacities than hydroxy-γ-sanshool (RS), attributed to the hydroxyl group. Furthermore, HRS had the greatest oxygen radical absorbance capacities (ORAC) and free radical scavenging (DPPH and ABTS) capacities, attributed to more cis-double bonds. Sanshools could effectively decrease the level of oxidative stress in colonic tissue and HCT-116 cells, including increased enzyme activities of catalase (CAT), superoxide dismutase (SOD), and glutathione peroxidase (GSH-Px) and decreased concentrations of malondialdehyde (MDA). After intragastric administration of sanshool, HRS increased the the level of hepatic Total antioxidant capacity (T-AOC) and colon GSH-Px and SOD. Moreover, sanshools significantly increased Nrf2, HO-1 proteins and decreased Keap1 protein. The protective effect of HRS was much higher than HAS, HBS, and RS, apparently due to its amide and hydroxyl groups. The binding energies of Nrf2 with HAS, HBS, HRS, and RS were −5.17, −5.11, −5.75, and −4.16 kcal/mol, respectively. HRS is more easily combine with Nrf2. In summary, sanshools acted as substitutes for natural antioxidants, in which HRS has the greatest antioxidant activity. This study provided a basis for future research on relationships between structure and activity.
1 Introduction
Prickly ash is listed in the pharmacopeia, with >30 prescriptions (Kono et al., 2020). The primary chemical constituents consist of alkaloids, amides, volatile, and fatty acids (Luo et al., 2022) with diverse functionalities. In African, Zanthoxylum species were used in traditional medicine for sickle cell anemia and malaria (Ouédraogo et al., 2019). In Japan and the United states, the Zanthoxylum-extract medicines are applied in studies on modern clinical trials (Rong et al., 2016). In Chinese, Zanthoxylum species were remedies of vomiting, ascariasis, itching, acute and chronic pain, and diarrhea (Zhang et al., 2021). Sanshools are polyunsaturated amides and are regarded as critical constituents in the biological activities of prickly ash. More than 10 sanshools have been identified since the first sanshool structure (α-sanshool) was reported (Crombie, 1952, 1954). The structure of sanshools was commonly subdivided into two parts, C-terminus and N-terminus (Sun et al., 2020). For example, there are 2–5 C-terminal unsaturated alkyl groups (Huang et al., 2012); unsaturated alkyls of the fatty chain could be oxidized to ketone, hydroxyl, or aldehyde groups. The N-terminal isobutyl could be dehydrogenated to isobutylene, or hydroxylated to 2′-hydroxy-isobutyl (Luo et al., 2022). In addition, differences in the position of oxidizing groups and the presence of cis and trans- double bonds can also generate isomers with distinct biological activities. N-isobutylamide sanshools incite burning oroxensation whereas hydroxylated congeners were reported to be anesthetic (numbing) orosensory recognition (Chruma et al., 2018). Therefore, whether there is difference among the abilities of antioxidant in sanshools with various structure?
Antioxidants are among the most studied topics both in nutrition and food science (Liu Y. et al., 2022; Liu R. et al., 2022). Antioxidants are compounds that can interact with free radicals and suppress chain reaction-related diseases (such as certain cancers, cardiovascular diseases, type 2 diabetes, chronic inflammation, aging, and the maintenance of gut health) (Rice-Evans and Diplock, 1993; Bartoszek and Polak, 2012). Although oxidative stress may be caused from enhanced levels of both reactive oxygen species (ROS) and reactive nitrogen species (RNS), researches on cell responses to sanshools have been focused on the influence of ROS to date. It has been reported (Brewer, 2011) that extracts of prickly ash have strong antioxidant activities that inhibited an overactive peroxidase system and scavenged free radicals in pathological models (Brewer, 2011; Falowo et al., 2014; Deng et al., 2019). These extracts reduced the production of malondialdehyde (MDA) in rat liver and protected primary Sprague Dawley rat hepatocytes against H2O2-induced cytotoxicity by inhibiting depletion of mitochondrial membrane potential and intracellular ROS generation (Zhang et al., 2017). In addition, hydroxy-α-sanshool (HAS) inhibited oxidative stress by reducing MDA production and increasing the activity of antioxidative enzymes (Li et al., 2020; Liu Y. et al., 2022; Liu R. et al., 2022). The above findings suggest that sanshools may activate a more comprehensive and even wider system of antioxidative responses to control redox balance. The nuclear transcription factor NF-E2-related factor 2 (Nrf2) is a helix–loop–helix basic leucine zipper transcription factor and controls the activation of downstream target genes coding for a network of enzymatic antioxidants. Under normal and balanced redox homeostasis, Kelch-like ECH-associated protein 1 (Keap1) binds to Nrf2 and promotes its degradation through the ubiquitin proteasome pathway (Kaspar et al., 2009). The dissociation of Nrf2 and Keap1 was promoted by the increased level of intracellular ROS during oxidizing conditions and Nrf2 escapes subsequent degradation (Ma, 2013). After that, the released Nrf2 is translocated to the nucleus, driving the expression of Nrf2 target genes (Ma, 2013).
Most studies focused on the capacity of scavenging free radicals and signaling pathways associated with antioxidant activity, but the relationship between sanshools with different structure and antioxidant ability was rarely reported and need to be illuminated (Luo et al., 2022). The abundant (>90%) components of sanshools in prickly ash are HAS, HBS, HRS, and RS, which vary in a hydroxyl group, N-alkyl group, and double bond (Figure 1A). Therefore, our objective was to explore the structure–activity relationships of sanshools on antioxidant activities in vitro and vivo. Antioxidant effects of four sanshools (HAS, HBS, HRS, and RS) against oxidative stress were assessed to elucidate structure–activity relationships. The study investigated the levels of oxygen radical absorbance capacity, radical scavenging capacity, mitochondrial membrane potential, intracellular reactive oxygen species, antioxidant activities, and expression of the signaling pathway caused by sanshool. We concluded that sanshools could act as substitutes for natural antioxidants, in which HRS has the highest antioxidant activity.
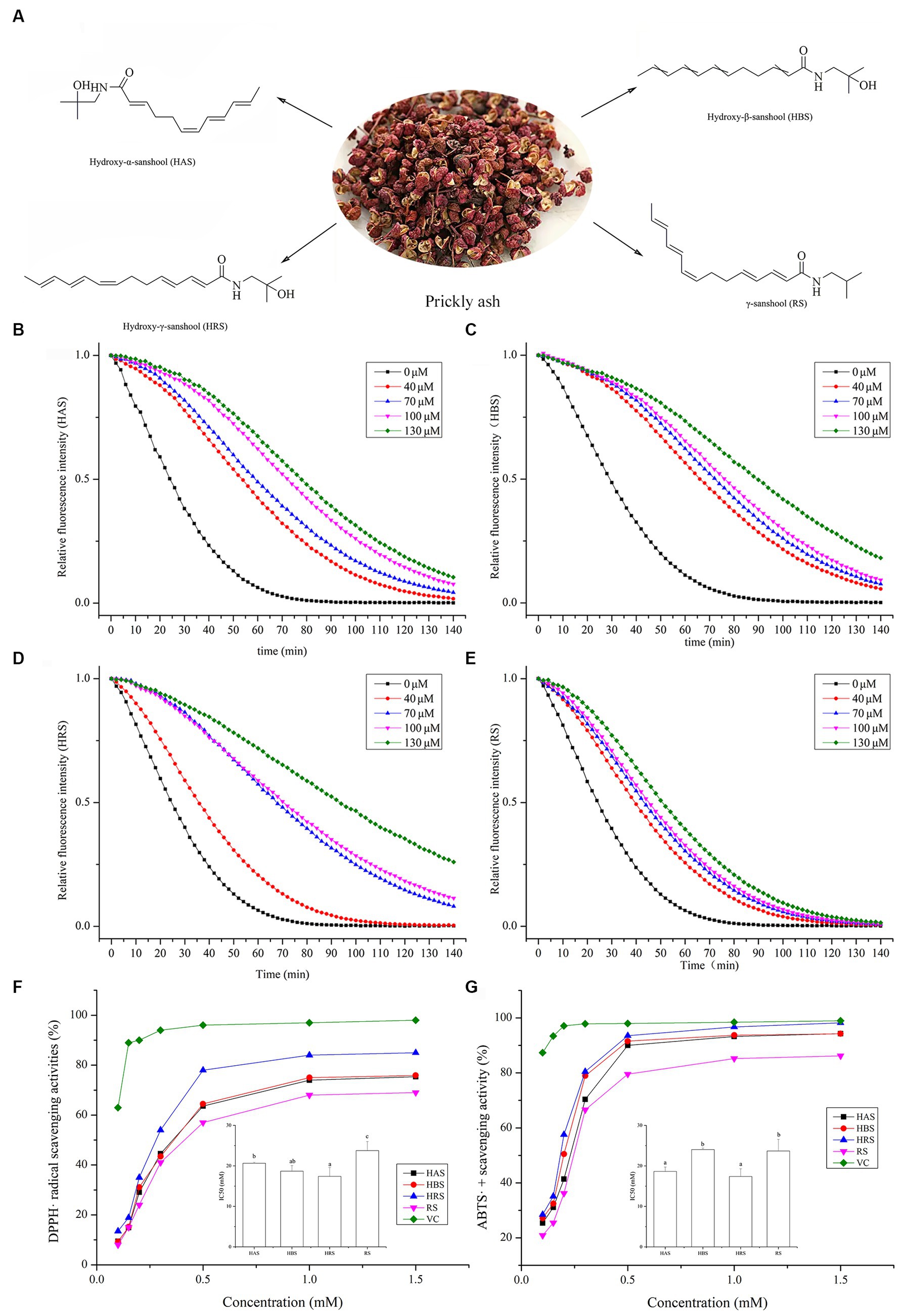
Figure 1. (A) Sanshools from prickly ash: hydroxy-α-sanshool (HAS), hydroxy-β-sanshool (HBS), hydroxy-γ-sanshool (HRS), and γ-sanshool (RS). ORAC measurement on fluorescein consumption induced by peroxyl radicals (ROO•) generated by four sanshools: (B) HAS, (C) HBS, (D) HRS, and (E) RS. (F) DPPH radical scavenging activity. (G) ABTS radical scavenging activity.
2 Materials and methods
2.1 Materials and chemicals
All four sanshools (HAS, HBS, HRS, and RS; purity ≥98%) were powdery substances and purchased from Chengdu Maidesheng Technology Ltd. (Chengdu, China). Dextran sulfate sodium (DSS, MW 40,000) was obtained from Shanghai Aladdin Biochemical Technology Co., Ltd. (Shanghai, China). All reagents and chemicals were purchased from Sigma-Aldrich (Milan, Italy). Primary antibodies (anti-Nrf2, anti-HO-1, anti-Keap1, anti-actin), and secondary antibodies were obtained from the Beyotime Institute of Biotechnology (Shanghai, China).
2.2 Antioxidant activity analysis of sanshools
2.2.1 Oxygen radical absorbance capacity assay
The ORAC assay was conducted as described by Zhang et al. (2018) with minor modifications (Zhang et al., 2018). Sanshool solution (0, 40, 70, 100, and 130 μM) and fluorescein (0.096 μM) were putted in a black 96-well plate and kept at 37°C for 20 min. Subsequently, 20 μL of AAPH (119 mM) was added and quickly mixed. The fluorescence intensity was recorded every 2 min by a microplate reader (Biotek, Vermont, United States). Excitation and emission wavelength was 485 nm and 535 nm, respectively. The trolox was used as a standard.
2.2.2 DPPḤ radical scavenging capacity assay
The DPPH assay was conducted as described by Blois (1958). Sanshools were prepared in DMSO (50 μL) and 150 μL of DPPH (80 μM) were placed in a 96-well plate. After mixing for 1 h at 25°C, absorbance was recorded at 517 nm. In the control group, the sample was replaced with ascorbic acid. The capability of scavenging DPPH radical was evaluated by the following formula (1):
Where A0 was the absorbance of a sample containing sanshool and DPPH solution; A1 was absorbance without DPPH solution, and A2 was absorbance without sanshool.
2.2.3 ABTS•+ radical scavenging capacity assay
The ABTS•+ radical scavenging capacity assay was conducted by Re et al. (1999). Various concentrations of the sample solution (100 μL) and 100 μL of ABTS solution were mixed. After incubation for 20 min in the dark, absorbance was recorded at wavelength 734 nm. The mixture of ABTS and distilled water was used as the control group. The ABTS radical scavenging capacity of sanshools was evaluated as follows:
Where A0 and A1 were absorbances of the reaction system without and with the sample, respectively.
2.3 Ethics statement and treatment
Male C57BL/6 mice (20–22 g and 6–8 weeks) were purchased from Spife Biotechnology Co., Ltd. (Beijing, China) and reared in an SPF environment with free access to water and food at the ambient temperature of 24 ± 2°C and kept on a dark- and light- cycle, changing every 12 h. The experimental procedures and animal welfare have been approved by the Animal Ethics Committee of Guizhou University (approval no. EAE-GZU-2022-E025), in accordance with the Guide for the Care and Use of laboratory Animals (order no. 1998-55, Ministry of Public Health, China; National Institutes of Health, the United States). Mice were randomly divided into the following six groups: the normal control group, DSS-model group, DSS + HAS group, DSS + HBS group, DSS + HRS group, DSS + RS group. The intragastric dose of sanshool was 2.5 mg/kg, referred to the study of You et al. (2015). UC was induced with 2.5% (wt/vol) DSS dissolved in the drinking water for 8 days. After mice were termination, the liver and colon were collected at −80°C.
2.4 Cell culture and proliferation assay
The cells were treated with H2O2 or (and) sanshools for 24 h and assessed by Cell Counting Kit8 (APE × BIO, TX, United States), according to our previous study (Chen et al., 2022).
2.5 Antioxidant enzyme activities and MDA content
The levels of SOD, CAT, T-AOC, and GSH-Px activities and MDA content in cell or tissue homogenates were measured according to the commercially kits.
2.6 Intracellular reactive oxygen species concentrations
Effects of sanshools on ROS concentrations were determined with a DCFH-DA fluorescent probe assay. For this, 2 × 105 cells/well was seeded in a 6-well plate for 24 h. Then, the cell culture medium was replaced with DCFH-DA (5 μM) solution and incubated for 30 min at 37°C. Images were observed with an inverted fluorescence microscope (TS100, Nikon, Japan). Intracellular ROS concentrations were determined by measurement of DCF fluorescence with a flow cytometer (BD Company, Franklin Lakes, NJ, United States).
2.7 Mitochondrial membrane potential
The HCT-116 cells were grown on a 6-well plate and treated with H2O2 (200 μM) for 4 h and sanshools (30 μM) for another 24 h. After treatment, cells were washed three times with serum-free medium and incubated with JC-1 (MedChenexpress, New Jersey, USA) at 37°C in the dark for 30 min. After cells were washed twice with PBS, fluorescence was assessed with an inverted fluorescence microscope (TS100, Nikon, Japan).
2.8 Protein extraction and western blot
The protein extraction and western blot of treated cells were performed by our previous study (Chen et al., 2022).
2.9 Molecular docking
Molecular docking was performed with AutoDock 4.2. The crystal structure of Nrf2 was obtained from the Protein Data Bank. Sanshools 3D structures were downloaded from PubChem and energetically minimized by the MM2 method with ChemBio3D. We conducted blind docking and considered the whole protein for docking with sanshools to detect potential hits in Nrf2. The grid box used for blinding docking was set to 120 × 120 × 120 Å, with a spacing of 1.0 Å. According to the blind docking result, focused docking was then performed, and the grid box was set to 23 × 23 × 23 Å, with a spacing of 0.35 Å. Both blind and focused docking was conducted for 100 runs with a Genetic Algorithm. The conformation with the lowest predicted binding energy was selected as the proposed binding conformation for sanshool. Results were visually analyzed by PyMol (DeLano Scientific LLC, United States).
2.10 Statistical analyses
All data were expressed as mean ± standard deviation (SD) (n ≥ 3). Student’s t-test and one-way ANOVA were used to detect differences between treatments for two or more than three groups, respectively. All analyses were done with SPSS17 (SPSS, Inc., Chicago, IL, United States), and p < 0.05 was considered significant.
3 Results
3.1 Antioxidant activity of sanshools in vitro
The ORAC values of four sanshools are shown in Figures 1B–E. As fluorescent decay curves of all four sanshools were above that of the control group, all sanshools had a kinetic protective behavior against fluorescent decay induced by AAPH. In addition, these sanshools had excellent linear relationships between sample concentrations and the net areas under the curve (AUC). The ORAC values (μmol Trolox equiv. /g DW) were RS (290.02 ± 13.16) < HBS (625.23 ± 4.7) < HAS (662.61 ± 23.54) < HRS (759.41 ± 8.37). HRS had the highest ORAC values among the other three sanshools in vitro.
All four sanshools had significant DPPH radical scavenging ability and caused dose-dependent inhibitory effects from 0.3 to 1.5 mM, with the inhibitory effect generally stable when the concentration was >0.5 mM (Figure 1F). The 50% inhibitory concentration value of HRS was the lowest among all samples (p < 0.05), implying HRS had stronger DPPH radical scavenging ability than the other three sanshools, but still lower than VC (positive control).
All four sanshools had obvious ABTS radical scavenging ability, in a concentration-dependent manner (Figure 1G). Maximum scavenging abilities of HAS, HBS, HRS, and RS reached 98.18, 97.65, 95.46, and 87.26% of VC, respectively. The IC50 value of HRS on ABTS radical scavenging ability was the lowest (p < 0.05), indicated it had the most potent ABTS radical scavenging activity.
3.2 The treatment of oxidative damage cells
The viability of HCT-116 cells was reduced by adding various concentrations of H2O2 for 24 or 48 h (Figure 2A). Cell survival rates were 53.85 ± 4.25% and 46.38 ± 2.57% of the control group after incubation for 24 and 48 h with 200 μM H2O2. Therefore, treatment with 200 μM H2O2 for 24 h was the oxidative damage model for HCT-116 cells.
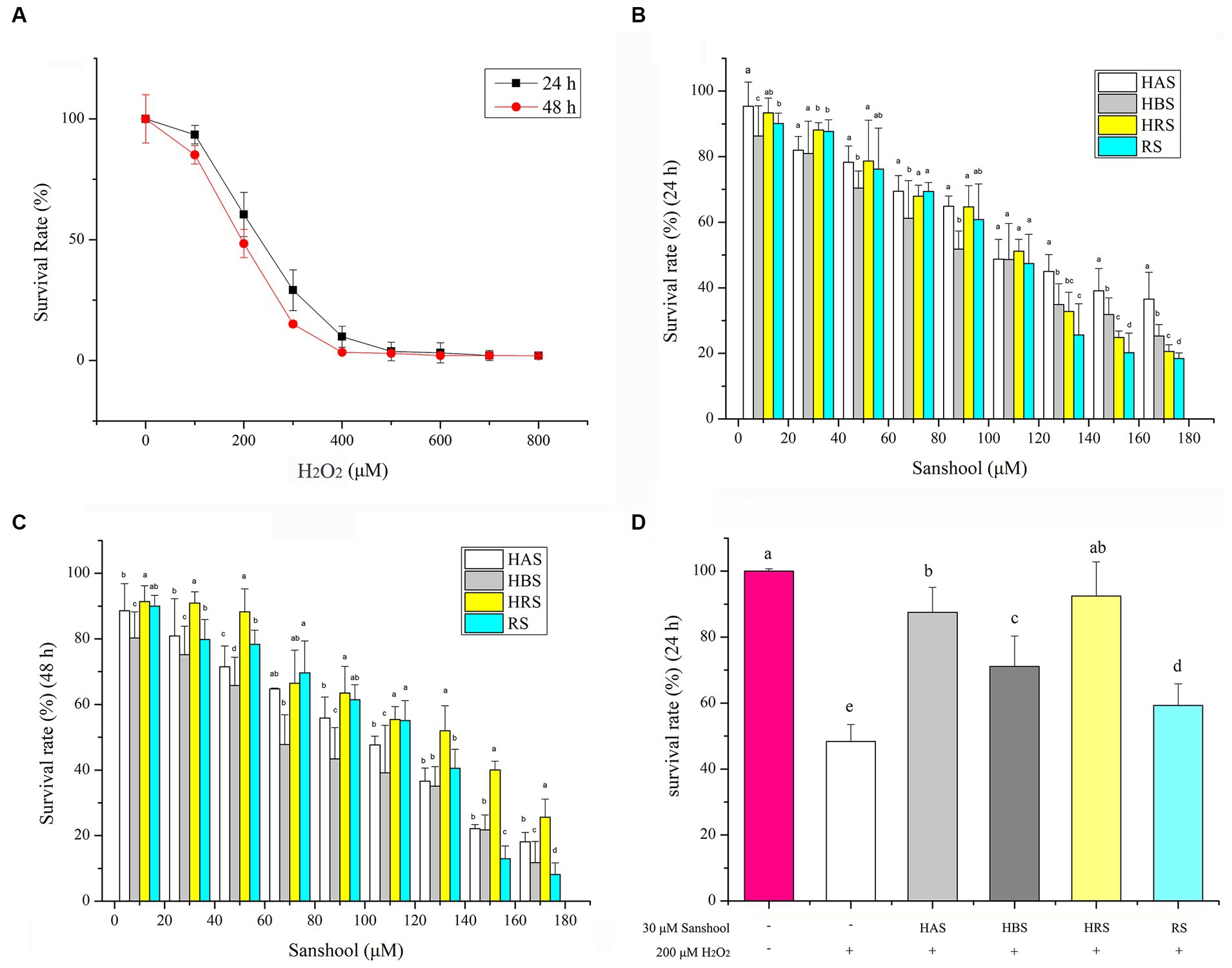
Figure 2. (A) Cell viability of HCT-116 cells after treatment with 200 μM H2O2 for 24 and 48 h. Cell viability of HCT-116 cells after treatment with sanshools for 24 h (B) and 48 h (C). (D) Cell viability of HCT-116 cells after treatment with sanshools and 200 μM H2O2 for 24 h. All data were expressed as means ± SD from three replicated experiments. a-dWithin a cluster, columns without a common superscript differed (p < 0.05).
To determine the ability of sanshools to ameliorate the effects of H2O2-induced oxidative stress in HCT-116 cells, a CCK8 assay was conducted after cells were treated with each sanshool for 24 or 48 h. The cell inhibition rate was <20%, even when the concentration of sanshool was as high as 30 μM for 24 h (Figures 2B,C), indicating minimal toxicity. Therefore, 30 μM sanshool was used to determine its ameliorating effects of H2O2-induced oxidative stress. Cells were treated with 200 μM H2O2 for 4 h and thereafter with sanshools for 24 h (Figure 2D). Compared to the untreated group, treatment with 200 μM H2O2 significantly decreased cell survival. However, after 24 h, each of the four sanshools had reversed H2O2-induced injury, with a survival rate of 87.48 ± 7.58, 73.08 ± 9.25, 92.47 ± 10.36, and 60.27 ± 6.53% for HAS, HBS, HRS, and RS, respectively, indicating that sanshools at concentrations <30 μM protected HCT-116 cells from oxidative damage. As 30 μM sanshool had obvious protective effects and minimal cell cytotoxicity with treatment for 24 or 48 h.
3.3 The mitochondrial membranes potential and accumulation of ROS in H2O2-induced HCT-116 cells
In our study, the effect of sanshools on protecting cells against H2O2-induced oxidative stress was examined by a JC-1 probe. In healthy mitochondria, JC-1 complexes emit red fluorescence (Figure 3A), whereas, in dysfunctional mitochondria, the complexes have green fluorescence. The use of H2O2 led to a significant increase in the intensity of green fluorescence in cells when compared to the untreated group. This suggests that H2O2 resulted in damage to the mitochondria. However, 30 μM sanshools decreased the number of cells with green fluorescence and increased red fluorescence intensity, indicated that sanshools protected HCT-116 cells from H2O2-induced mitochondrial dysfunction. The red fluorescence intensity of RS was lower than that of HAS, HRS, and HBS, showing that sanshool with hydroxy group have better effect on mitochondria damage inhibition.
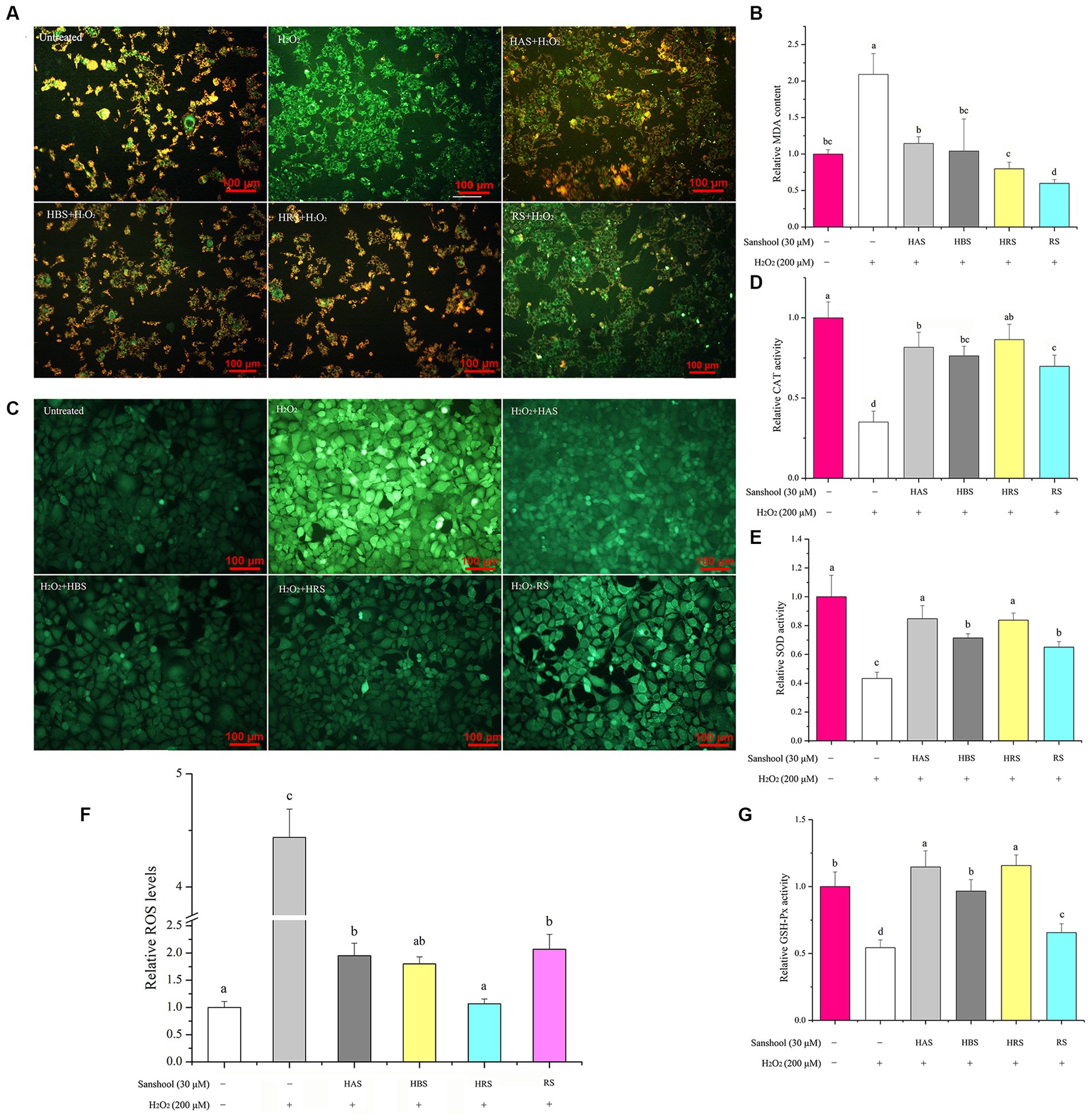
Figure 3. (A) The level of mitochondrial membrane potential of HCT-116 cells after treatment with 200 μM H2O2 for 24 h. Effects of 30 μM sanshools on changes in relative MDA concentration (B) and enzyme activities of CAT (D), SOD (E), and GSH-Px (G) in HCT-116 cells. (C,F) The level of ROS concentrations in HCT-116 cells after treatment with 200 μM H2O2 for 24 h.
To determine the effects of sanshools on redox status of H2O2-induced cell, intracellular ROS concentrations were measured by determining fluorescent probes 2′, 7′- dichlorodihydrofluorescein diacetate (DCFH-DA). After exposure to 200 μM H2O2, the fluorescence intensity of DCF in HCT-116 cells increased sharply, compared to the control group (Figure 3C), indicating that ROS generation was significantly stimulated by H2O2 treatment. However, treatment with HAS, HBS, HRS, and RS for 24 h decreased ROS generation, respectively, indicating that four sanshools may be beneficial for inhibiting the generation of ROS. The results of the study revealed that HRS had a higher ability to eliminate ROS compared to other sanshools, as indicated in Figure 3F. This suggests that HRS possesses more potent ROS scavenging abilities. However, it was also observed that the ROS level in the HRS group was still higher than that of the untreated group. There was no significant difference between 30 μM HAS and HBS on scavenging effects of intracellular ROS, whereas non-hydroxylated RS had poorer inhibitory activities than HAS, HBS, and HRS, indicating that the hydroxyl group has an important role in inhibitory activity against H2O2-stimulated ROS production.
3.4 Antioxidant enzyme activity and MDA concentration
Treatment with H2O2 increased MDA concentrations compared to the untreated group (p < 0.05; Figure 3B). All four sanshools mitigated MDA production induced by H2O2, with RS having the best inhibition. After exposure to 200 μM H2O2, the activities of CAT (Figure 3D), GSH-Px (Figure 3G), and SOD (Figure 3E) were reduced by 64.95, 45.70, and 56.74%, respectively. However, compared with the group treated with 200 μM H2O2 (p < 0.05), sanshools increased the activity of SOD (from 44.19 ± 3.03% to 97.67 ± 5.34%). It was noticeable that HAS and HRS exhibited a significantly higher level of SOD activity compared to HBS and RS., indicating sanshool with cis-double bond had improvement effect in SOD enzyme activity of HCT-116 cells. Similarly, the four sanshools showed excellent enhancement ability of CAT activity in the given concentration (30 μM). The CAT activity enhancement abilities of HRS, HAS, HBS, and RS were measured and found to have increased by 147.03 ± 8.27, 132.90, 117.68%, and 99.27 ± 7.36%, respectively. The values of HRS, HAS, and HBS were significantly higher than RS (p < 0.05), indicating that they have the ability to increase CAT activity. Compared to the H2O2 damaged group, the results of relative activities of GSH-Px indicated that all four sanshools exhibited significant enhancement effect and they were increased from 20.81 ± 1.62% to 111.05 ± 14.64% in HCT-116 cells (p < 0.05). The relative value of GSH-Px enzymes of four sanshools samples were different and followed the order of RS < HBS < HAS < HRS, but no significant difference were observed between HAS and HBS, indicating that HAS and HRS exhibited the most potent ability of enhancing GSH-Px activity.
According to the obove analysis, the ability of sanshools to resist oxidative stress was supported. The analysis of oxidative-related indicators were conducted, including T-AOC, MDA, GSH-Px, and SOD in the hepitic and colon tissures of mice (Figure 4). The level of MDA in DSS-induced colitis was obviously elevated, whereas the activities of GSH-PX, SOD, and T-AOC were markedly reduced. After intragastric administration of sanshool, HRS increased the the level of hepatic T-AOC and colon GSH-Px and SOD. HBS incereased the level of colon T-AOC and GSH-PX. RS significantly increased the colon T-AOC. Meanwhile, all sanshools decresed colon MDA content. It is noteworthy that sanshools exhibited efficacy in mitigating DSS-induced oxidative stress in the colon of mice.
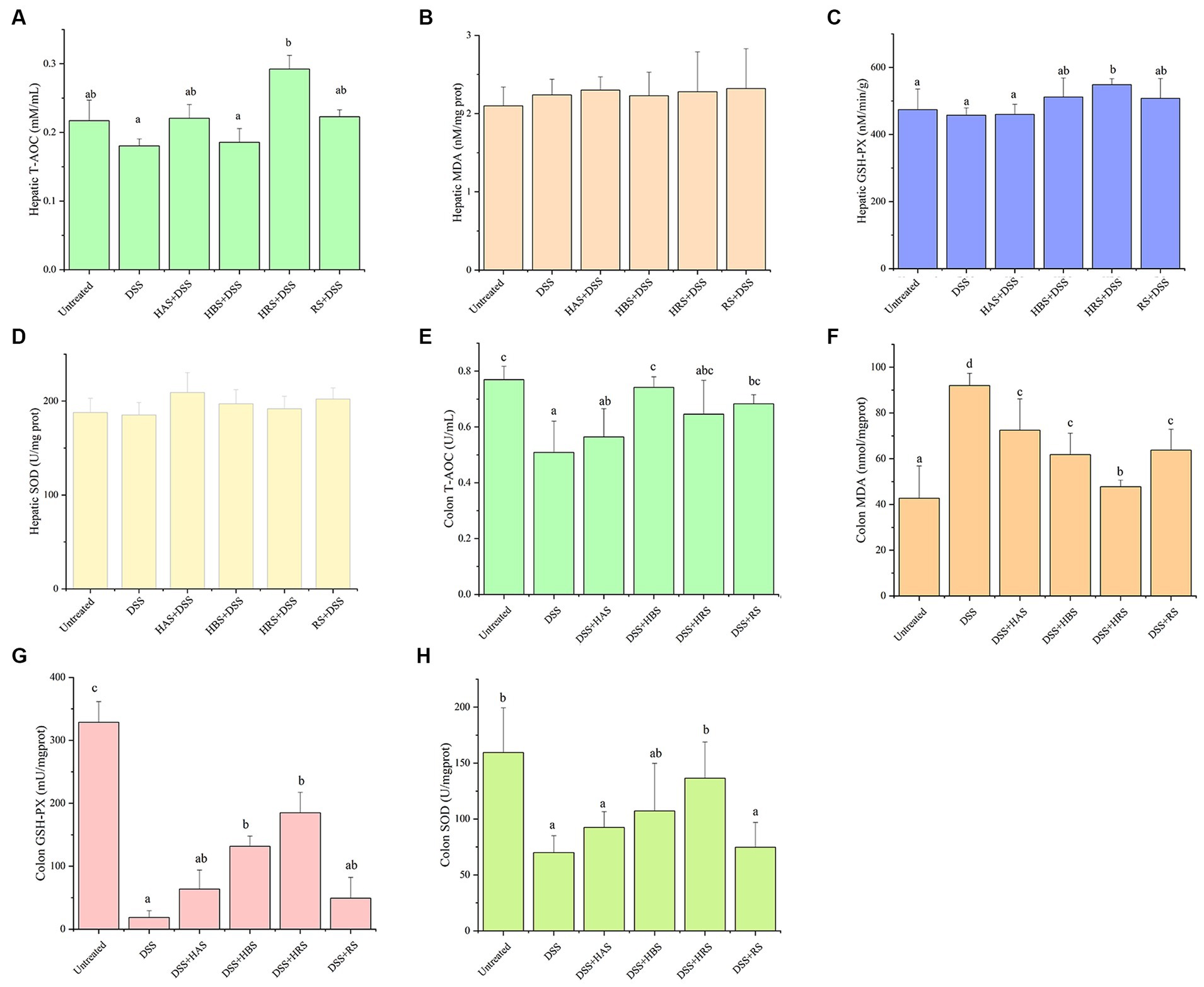
Figure 4. Antioxidant effects of sanshools in DSS-induced colitis mice. The level of T-AOC (A), MDA concentration (B) and enzyme activities of GSH-Px (C), and SOD (D) in liver tissue. The level of T-AOC (E), MDA concentration (F) and enzyme activities of GSH-Px (G), and SOD (H) in colon tissue. All data were expressed as means ± SD from three replicated experiments. a-dWithin a cluster, columns without a common superscript differed (p < 0.05).
3.5 Western-blot analysis of Keap1-Nrf2 pathway-related protein
The levels of Nrf2 and HO-1 in cells were lower in the H2O2-damaged group than in the untreated group. On the other hand, the relative protein expression of keap1 was higher in the H2O2-damaged group. All four sanshools attenuated H2O2-induced acute cell injury by up regulating protein expression of Nrf2 and HO-1 and down regulating transcription factors (Keap1) in the Keap1/Nrf2 signaling pathway (Figures 5A,B). Obviously, the protein expressions of Nrf2 in the group treated with HAS, HBS, and HRS was higher than RS, and HRS possess significant enhancement on the protein expression of HO-1 than other sanshools. In the aspect of inhibition expression Keap1, HRS and HBS exhibited significant inhibition effect than HAS and RS. Similarly, in the colon of mice, the Nrf2 and HO-1 were lower expressed in the group with DSS, while Keap1 was highly expressed (Figures 5C,D). Nobably, when the mice were treated with sanshool, the adverse biological progress was inhibited.
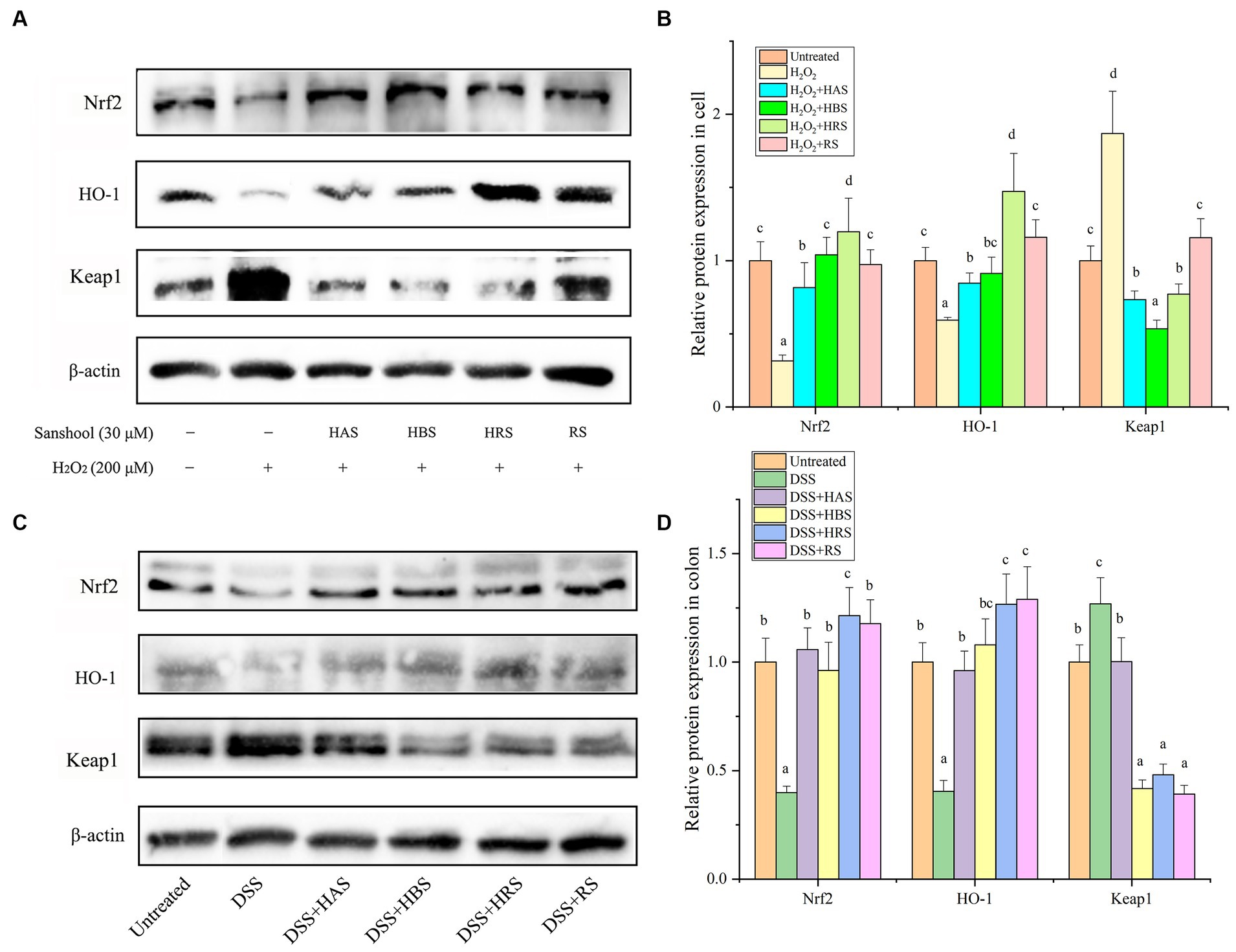
Figure 5. Sanshools activated Keap1-Nrf2 pathway. (A) Western blot analysis of Nrf2, HO-1, and Keap1 in HCT-116 cells. (B) The quantitative data of panel (A). (C) Western blots analysis of Nrf2, HO-1, and Keap1 in mice colon tissues. (D) The quantitative data of panel (C). All data were expressed as means ± SD from three replicated experiments. a-cWithin a cluster, columns without a common superscript differed (p < 0.05).
To explore whether sanshools could alleviate oxidative injury by promoting Nrf2 into the nucleus and characterizing potential binding sites among various sanshools and Nrf2, the protein expression of Nrf2 in cell nuclear and cytoplasmic and molecular docking were studied. Expression of Nrf2 protein was extremely low in the nucleus of the damaged group, whereas HBS, HRS, and RS significantly promoted translocation of Nrf2 protein into the nucleus, while no statistical significance was observed between HAS and H2O2 group. In the cytoplasm, Nrf2 content was decreased in the H2O2 damaged group, whereas sanshools treatment increased expression of Nrf2, implying it promoted Nrf2 protein translocation into the nucleus (Figures 6A–C). The binding energies of Nrf2 with HAS, HBS, HRS, and RS were −5.17, −5.11, −5.75, and −4.16 kcal/mol, respectively (Figures 6D–G). In addition, the amino acid residues GLY-325, ARG-326, and GLN-563 of Nrf2 and HRS formed four hydrogen bond interactions, whereas only one hydrogen bond was formed between amino acid residue THR-560 of Nrf2 and RS, showing that HRS is more easily combine with Nrf2. The above results confirm that sanshools have a strong binding effect with Nrf2, suggesting that HRS may be a potential activator of Nrf2.
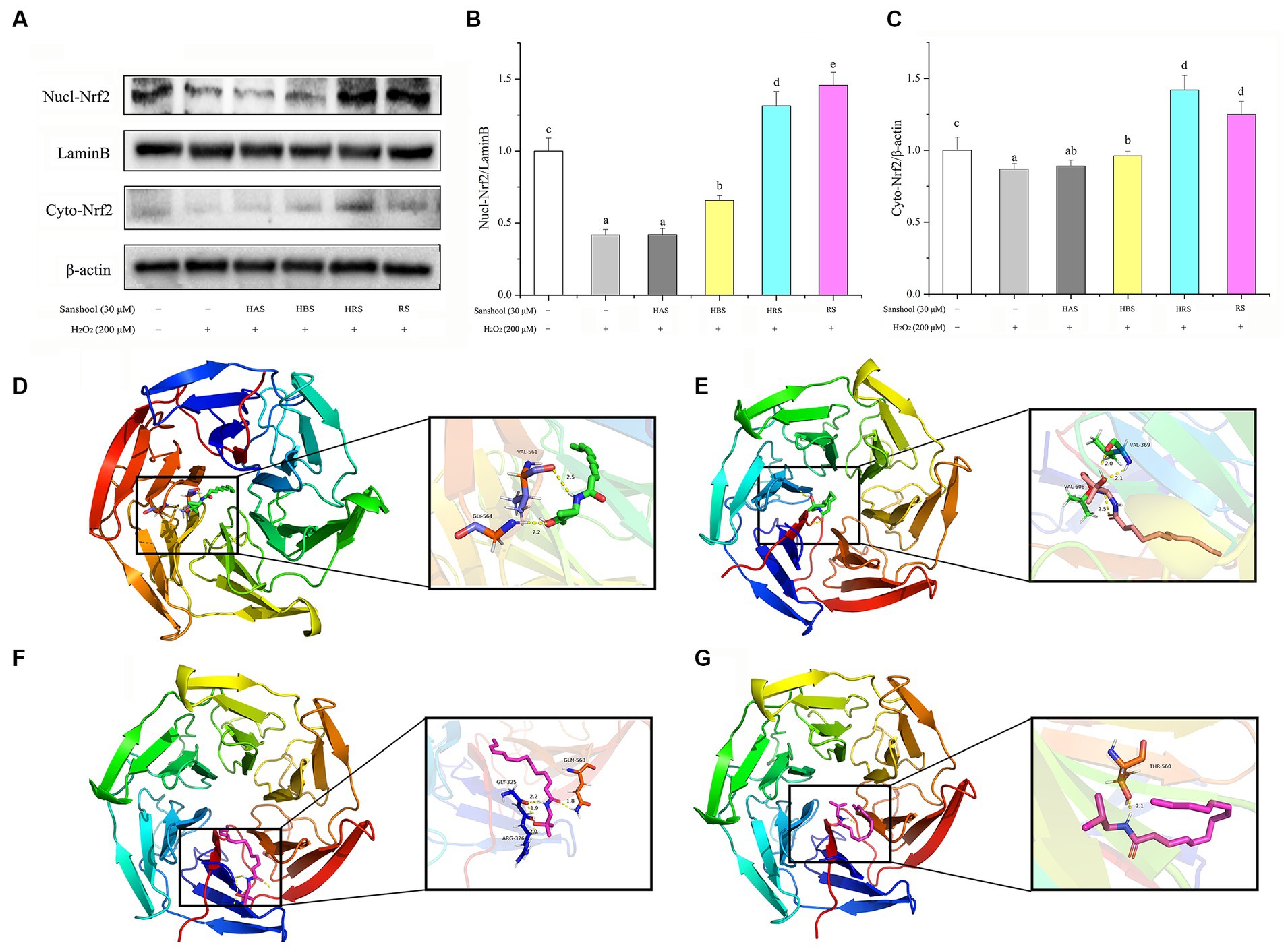
Figure 6. Nrf2 could be used as an important target for sanshool in the treatment of oxidative stress. (A) Western blot analysis of nuclear and cytoplasmic Nrf2 in HCT-116 cells treated with 30 μM sanshools for 24 h. (B) The quantitative data of nuclear Nrf2. (C) The quantitative data of cytoplasmic Nrf2. The molecular docking simulation: (D) HAS and Nrf2, (E) HBS and Nrf2, (F) HRS and Nrf2, and (G) RS and Nrf2. Hydrogen bonds are shown as dotted lines. All data were expressed as means ± SD from three replicated experiments. a-eWithin a cluster, columns without a common superscript differed (p < 0.05).
4 Discussion
Sanshools and hydroxy-sanshools are the predominant class of long-chained polyunsaturated amides which are characterized by the presence of amide bond (−C(=O) NH−). These alkylamides are from the same family and are responsible for the tingling, numbing, and buzzing mouth sensation. Apart from utilization as an attractive flavoring, the favorable effects of prickly ash have been extensively documented and presented pre-clinic or clinical potentiality in human health promotion. Antioxidant activities have been attested in extracts of prickly ash based on the DPPH or other radical assays (Li et al., 2020). Except the Zanthoxylum genus, alkylamides also present in Brassicaceae, Convolvulaceae, Euphorbiaceae, Piperaceae, Poaceae, and Solanaceae. These compounds are consisted with saturated or unsaturated fatty acid chain and cyclic, aliphatic, or aromatic amine moiety, all possessing the antioxidant effects (Elufioye et al., 2020). Having that in mind, we aimed to elucidate the effect of HAS, HBS, HRS, and RS in eradicating free radicals, ameliorating intestinal injury and cytoprotective against H2O2-induced oxidative stress to further illustrate structure–activity relationships of sanshools.
To fully explore relationships between sanshool structure and antioxidant abilities, ORAC, DPPH, and ABTS assays were used to assess antioxidant abilities of all four sanshools. As expected, all sanshools have abilities of scavenging free radicals. The radical scavenging activity of sanshools is due to the hydrogen-donating ability and the presence of other H-donating groups (hydroxy and amide) (Xiao et al., 2022). Based on previous studies, the hydroxyl group is the main antioxidant site of many antioxidants, including polyphenols, phytic acid, catechin, phospholipids, and tocopherols (Son and Lewis, 2002). In this study, RS had the lowest antioxidant activity, due to its structure lacked the hydroxy which present in the other three sanshools. Antioxidative activity depends not only on the number of hydroxyl groups but also on the double-bond structure (Son and Lewis, 2002). Although double bonds involved in scavenging peroxyl radicals are contentious, antioxidant activities of HAS and HBS were lower than HRS which has more double bonds, implying that the double bond of sanshool was involved in the neutralization of peroxyl radicals. Similarly, it was also confirmed that cannabinoids with more double bonds than non-olivetolic had higher antioxidant activity (Dawidowicz et al., 2021). In addition, the antioxidant ability of HAS with cis-double bond was higher than HBS with trans-double bond. The previous study showed that substitution of a natural trans-double bond with a cis-double bond increased the oxidative ability of sphingomyelin by up to eight-fold, indicating that the trans-double bond is not essential for its antioxidant effects. Furthermore, a trans-double bond tends to be less reactive chemically than a cis-double bond, and the trans structure may also be less susceptible to free-radical reactions (Subbaiah et al., 2009). Perhaps this accounts for cis-isomer HAS with greater antioxidant activity than trans-isomer HBS, despite an identical molecular formula. Four sanshools prepared from the same species of prickly ash had different structures, which altered configurations and hydrogen-donating abilities (Cerdá et al., 2004; Mladenović et al., 2011). The IC50 value of HAS and HBS showed different effect on DPPḤand ABTS·scavenging activities, indicated that cis-isomer HAS exhibited more superior antioxidant activities on hydrosoluble free radical (ABTS·). Based on an assessment of antioxidant activities summarized above, we concluded that HRS had the best antioxidant activity, indicating it has the potential as a natural antioxidant and free radical scavenger. The above results confirmed that sanshool have the antioxidant ability, which were similar to previous studies (Liu et al., 2021; Peng et al., 2021). In another research, the sanshool possessed the synergistic antioxidant effects when it was mixed with other components (cichoric acid and caffeic acid derivative in Echinacea pallida) (Dalby-Brown et al., 2005). Relationships between the structure and antioxidant activities of sanshools are inherently complex, but our study provided novel insights.
Our results revealed that four sanshools (0–30 μM) have minimal toxicity on cell inhibition rate. Several studies showed that the sanshools extracted from leaves, fruits, bark, and roots of prickly ash exerted anti-proliferative effects. Extracts from the fruit inhibited cell proliferation in three human cancer cell lines (DLD-1, Caco-2, and HepG2) through JNK-dependent autophagic and no inhibition was observed in MCF-7, WiDr, and A549 (Nozaki et al., 2016). The growth of neurofibromatosis type 1 (NF-1)-deficient cancer xenografts in mice was blocked by the extracts of prickly ash through the PAK1/cyclin D1 pathway (Hirokawa et al., 2006). The 10 amides isolated from Nepalese Z. armatum were tested in a p53- and NF1-defective tumor cell line and only hydroxy-α-sanshool has no negatively impacting cell viability (Devkota et al., 2013). In our previous study, hydroxy-γ-sanshool inhibited cell proliferation of HCT-116 (IC50 = 88.01 μM) by activating P53 and Caspase 8 and had no cytotoxic effect to normal cells (IC50 = 481.52 μM). Therefore, the sanshools were not universally the key components which was used to inhibit the cell proliferation. In other word, the lower concentration of sanshools did not affect HCT-116 cell viability. In addition, H2O2 is widely used as an inducer of oxidative stress in vitro, generating hydroxyl radicals that cause serious damage to cells and attack biomolecules, ultimately leading to apoptosis or necrosis. In the present study, 200 μM H2O2 decreased cell viability and increased green fluorescence intensity in mitochondria of HCT-116 cells; however, treatment with sanshools reversed the decrease of cell viability and increased red fluorescence intensity. These results indicate that low-dose sanshools could protect HCT-116 cells from death induced by H2O2. Redox-active naphthoquinones linked with amide had superior activity in ATP rescue, which was maintained by the mitochondrial electron transport chain to increase the cell viability. Therefore, the protective effects of sanshools against mitochondrial dysfunction and cell death may have been due to the amide on the alkyl chain. Red fluorescence intensity in the RS group was lower than the other three sanshools, attributed to the hydroxyl group reducing oxygen entering the mitochondria and increasing ATP utilization or oxygen consumption, and attenuation of the formation of superoxide anions (Xiao et al., 2022). Although structures of HRS, HBS, and HAS differed, there was no significant difference in their protective effect on mitochondria membrane potential. Therefore, sanshools significantly reduced H2O2-induced cell death and mitochondrial dysfunction, with amide and hydroxyl groups implicated in maintaining mitochondrial membrane potential.
The increase in ROS level affects cell homeostasis, functions, and structures, resulting in oxidative stress. According to our findings, when HCT-116 cells were exposed to H2O2, there was an accumulation of intracellular ROS. However, this was improved with the administration of 30 μM sanshools. The protective effects of sanshools in the present research were partly mediated by ROS scavenging, given that sanshools treatment reduced the formation of MDA in colon tissues and cells, and changed the CAT activity of HCT-116. Similar results were obtained in previous research that the production of MDA in rat liver was reduced by the water extract of Szechuan pepper (Lu and Lu, 1995). Another recent research reported that HAS isolated from Zanthoxylum bungeanum regulates liver oxidative stress in hyperlipidemic rats by decreasing the levels of MDA (Li et al., 2020). Thus, due to their antioxidant abilities, direct action on ROS scavenging is one of the main protection mechanisms by sanshools. Accumulating evidence has demonstrated that there is an additional mechanism by which sanshools could reduce oxidative stress: indirect action on regulation of antioxidant enzyme. HBS was purified from Z. piperitum and can dose-dependently upregulates Gpx1 or Sod2 expression at 1.4–1.7 fold, protecting endogenous compounds from oxidative stress on mouse embryonic fibroblasts (Tomita et al., 2022). In the brain of D-gal/AlCl3 treated mice, the activities of SOD, CAT, and GSH-Px were improved by HAS at the doses of 2.5 and 5 mg/kg, while the MDA level was decreased (Liu Y. et al., 2022; Liu R. et al., 2022). SOD, CAT, and GSH-Px are three pivotal enzymes involved in ROS detoxification and suppress or prevent the production of free radicals (Ighodaro and Akinloye, 2018). Activities of these three key enzymes can be impaired and are usually accompanied by increased concentrations of malondialdehyde (MDA) (a marker for the degree of cellular oxidative damage) content under oxidative stress conditions (Nahar et al., 2016). In this study, all four sanshools significantly increased the activities of SOD, CAT, and GSH-Px in HCT-116 cells, similar to a previous report (Li et al., 2020). That HAS was superior to HBS in improving antioxidant enzyme activity was attributed to the spatial structure maintenance of the cis-double bond (Guerrero et al., 2012). In addition, the hydroxylation pattern on the core scaffold of compounds was reported to be closely associated with their affinity for antioxidant enzymes (Zhao et al., 2020) and that was also confirmed in natural flavonoids with abundant types (Zhang et al., 2019). Although RS induced the least improvement in antioxidant enzyme activities (CAT and GSH-Px) among the four sanshools, it also had the best inhibition of MDA production induced by H2O2. MDA was chemically reactive due to its carbonylic functions, whereas the potential OH group seemed not to react with carbonylic groups of MDA (Tsikas, 2017).
The accumulation of free radicals can disturb the physiological homeostasis. According to the studies, several signaling pathways could be rescued or accentuated by sanshool on the process of scavenging free radical, such as TLR4/NFκβ (Hong et al., 2017), MAPK (Wang et al., 2017), Nrf2 (Liu Y. et al., 2022; Liu R. et al., 2022), and PI3K/AKT (Li et al., 2020) signaling pathway. In our study, the Nrf2 pathway was activated in vitro and in vivo. Normally, Nrf2 is localized in the cytoplasm combined by Keap1. However, in the presence of oxidative stress, Nrf2 is released to the cell nucleus and activates many downstream antioxidant proteins such as heme oxygenase-1 (HO-1) to maintain the cell microenvironment homeostasis. In present western blot assay, all four sanshools had different protective effects against oxidant damage by activating the Keap1/Nrf2 signaling pathway, with the variation potentially due to several factors (Ma, 2013). Firstly, these four sanshools have different structures. Secondly, differences between HAS and HBS could be that the steric effect perturbs planarity and the ability to delocalize electrons. Thirdly, protein expression is regulated not only by the antioxidant abilities of four sanshools but also by the complex coordination of intracellular or in body microenvironments (Zhang et al., 2001). The protective of all four sanshools against oxidant damage was by activating the Keap1/Nrf2 pathway, with HRS having the most profound effects on the expression of proteins. Through molecular docking predictions, we found that there are various binding sites and several hydrogen bonds between Nrf2 and four sanshools. The results also showed that sanshool can effectively promote the shift of Nrf2 into the nucleus of the cell model, effectively alleviating oxidative stress. Erythritol bound with Nrf2 promoting a movement of Nrf2 protein into the nucleus to alleviate nonalcoholic fatty liver disease (Jin et al., 2021), although its binding energy (−2.55 kcal/mol) was inferior to the interaction between sanshools and Nrf2. The above results confirmed that sanshools have a strong binding effect with Nrf2; implying sanshools promote the activation of Nrf2. Conversely, different binding sites were formed by Nrf2 protein with sanshools, indicating differences among structures in binding energy and degree of Nrf2 activation, which affects the ability to mitigate H2O2-induced damage.
5 Conclusion
This study has confirmed the favorable effects of sanshools on efficiently inhibiting the oxidative stress. It has been shown that four sanshools scavenged ROS, increased the activity of antioxidant enzymes and decreased MDA concentration. Expression of Nrf2 protein and its downstream antioxidant protein HO-1 were positively increased, whereas Keap1 protein was decreased by sanshools. In particular, HRS had the best antioxidant activity, due to the number of cis-double bond, amido bond, and hydroxy group. To summarize, sanshools, especially HRS, had substantial antioxidant activities. This study provided new information regarding the structure–activity relationships of sanshools that may lead to new antioxidants.
Data availability statement
The original contributions presented in the study are included in the article/supplementary materials, further inquiries can be directed to the corresponding author.
Ethics statement
The animal study was approved by approval no. EAE-GZU-2022-E025. The study was conducted in accordance with the local legislation and institutional requirements.
Author contributions
ZC: Data curation, Formal analysis, Investigation, Methodology, Writing – original draft. LT: Validation. LL: Funding acquisition, Investigation. HW: Formal analysis, Methodology. XF: Funding acquisition, Validation. XL: Conceptualization, Funding acquisition, Project administration, Resources, Writing – reviewing & editing. CR: Funding acquisition, Resources.
Funding
The author(s) declare financial support was received for the research, authorship, and/or publication of this article. This work was supported by the Guizhou Province Science and Technology Plan Project (grant no. QKHZC [2023] general 478), the Natural Science Foundation of Guizhou Province (grant no. ZK [2021] general 165) and the Open Research Fund of State Key Laboratory of Southwestern Chinese Medicine Resources (grant no. SKLTCM2022026).
Conflict of interest
The authors declare that the research was conducted in the absence of any commercial or financial relationships that could be construed as a potential conflict of interest.
Publisher’s note
All claims expressed in this article are solely those of the authors and do not necessarily represent those of their affiliated organizations, or those of the publisher, the editors and the reviewers. Any product that may be evaluated in this article, or claim that may be made by its manufacturer, is not guaranteed or endorsed by the publisher.
References
Bartoszek, M., and Polak, J. (2012). An electron paramagnetic resonance study of antioxidant properties of alcoholic beverages. Food Chem. 132, 2089–2093. doi: 10.1016/j.foodchem.2011.12.060
Blois, M. S. (1958). Antioxidant determinations by the use of a stable free radical. Nature 181, 1199–1200. doi: 10.1038/1811199a0
Brewer, M. S. (2011). Natural antioxidants: sources, compounds, mechanisms of action, and potential applications. Compr. Rev. Food Sci. Food Safety 10, 221–247. doi: 10.1111/j.1541-4337.2011.00156.x
Cerdá, B., Espín, J. C., Parra, S., Martínez, P., and Tomás-Barberán, F. A. (2004). The potent in vitro antioxidant ellagitannins from pomegranate juice are metabolised into bioavailable but poor antioxidant hydroxy–6H–dibenzopyran–6–one derivatives by the colonic microflora of healthy humans. Eur. J. Nutr. 43, 205–220. doi: 10.1007/s00394-004-0461-7
Chen, Z., Lulin, T., Xin, F., Abdel-Nasser, S., Zunguo, L., and Xiong, L. (2022). Hydroxy-γ-sanshool from Zanthoxylum bungeanum (prickly ash) induces apoptosis of human colorectal cancer cell by activating P53 and caspase 8. Front. Nutr. 9:914638. doi: 10.3389/fnut.2022.914638
Chruma, J. J., Cullen, D. J., Bowman, L., and Toy, P. H. (2018). Polyunsaturated fatty acid amides from the Zanthoxylum genus–from culinary curiosities to probes for chemical biology. Nat. Prod. Rep. 35, 54–74. doi: 10.1039/C7NP00044H
Crombie, L. (1952). 574. Amides of vegetable origin. Part I. Stereoisomeric N-iso butylundeca-1: 7-diene-1-carboxyamides and the structure of herculin. J. Chem. Soc. Resumed, 2997–3008. doi: 10.1039/jr9520002997
Crombie, L. (1954). Isolation and structure of an N-isoButyldienediynamide from Pellitory (Anacyclus pyrethrum DC.). Nature 174, 832–833. doi: 10.1038/174832a0
Dalby-Brown, L., Barsett, H., Landbo, A. R., Meyer, A. S., and Mølgaard, P. (2005). Synergistic Antioxidative effects of alkamides, Caffeic acid derivatives, and polysaccharide fractions from Echinacea purpurea on in vitro oxidation of human low-density lipoproteins. J. Agric. Food. Chem. 53, 9413–9423. doi: 10.1021/jf0502395
Dawidowicz, A. L., Olszowy-Tomczyk, M., and Typek, R. (2021). CBG, CBD, Δ9-THC, CBN, CBGA, CBDA and Δ9-THCA as antioxidant agents and their intervention abilities in antioxidant action. Fitoterapia 152:104915. doi: 10.1016/j.fitote.2021.104915
Deng, S., Rong, H., Tu, H., Zheng, B., Mu, X., Zhu, L., et al. (2019). Molecular basis of neurophysiological and antioxidant roles of Szechuan pepper. Biomed. Pharmacother. 112:108696. doi: 10.1016/j.biopha.2019.108696
Devkota, K. P., Wilson, J., Henrich, C. J., McMahon, J. B., Reilly, K. M., and Beutler, J. A. (2013). Isobutylhydroxyamides from the pericarp of Nepalese Zanthoxylum armatum inhibit NF1-defective tumor cell line growth. J. Nat. Prod. 76, 59–63. doi: 10.1021/np300696g
Elufioye, T. O., Habtemariam, S., and Adejare, A. (2020). Chemistry and pharmacology of Alkylamides from natural origin. Rev. Bras 30, 622–640. doi: 10.1007/s43450-020-00095-5
Falowo, A. B., Fayemi, P. O., and Muchenje, V. (2014). Natural antioxidants against lipid–protein oxidative deterioration in meat and meat products: a review. Food Res. Int. 64, 171–181. doi: 10.1016/j.foodres.2014.06.022
Guerrero, L., Castillo, J., Quiñones, M., Garcia-Vallvé, S., Arola, L., Pujadas, G., et al. (2012). Inhibition of angiotensin-converting enzyme activity by flavonoids: structure-activity relationship studies. PLoS One 7:e49493. doi: 10.1371/journal.pone.0049493
Hirokawa, Y., Nheu, T., Grimm, K., Mautner, V., Maeda, S., Yoshida, M., et al. (2006). Sichuan pepper extracts block the PAK1/cyclin D1 pathway and the growth of NF1-deficient cancer xenograft in mice. Cancer Biol. Ther. 5, 305–309. doi: 10.4161/cbt.5.3.2404
Hong, L., Jing, W., Qing, W., Anxiang, S., Mei, X., Qin, L., et al. (2017). Inhibitory effect of Zanthoxylum bungeanum essential oil (ZBEO) on Escherichia coli and intestinal dysfunction. Food Funct. 8, 1569–1576. doi: 10.1039/C6FO01739H
Huang, S., Zhao, L., Zhou, X. L., Ying, M., Wang, C. J., and Weng, J. (2012). New alkylamides from pericarps of Zanthoxylum bungeanum. Chin. Chem. Lett. 23, 1247–1250. doi: 10.1016/j.cclet.2012.09.022
Ighodaro, O. M., and Akinloye, O. A. (2018). First line defence antioxidants-superoxide dismutase (SOD), catalase (CAT) and glutathione peroxidase (GPX): their fundamental role in the entire antioxidant defence grid. Alex. J. Med. 54, 287–293. doi: 10.1016/j.ajme.2017.09.001
Jin, M., Wei, Y., Yu, H., Ma, X., Yan, S., Zhao, L., et al. (2021). Erythritol improves nonalcoholic fatty liver disease by activating Nrf2 antioxidant capacity. J. Agric. Food Chem. 69, 13080–13092. doi: 10.1021/acs.jafc.1c05213
Kaspar, J. W., Niture, S. K., and Jaiswal, A. K. (2009). Nrf2:INrf2 (Keap1) signaling in oxidative stress. Free Radic. Biol. Med. 47, 1304–1309. doi: 10.1016/j.freeradbiomed.2009.07.035
Kono, R., Nomura, S., Okuno, Y., Kagiya, T., Nakamura, M., Utsunomiya, H., et al. (2020). Two Japanese pepper (Zanthoxylum piperitum) fruit-derived compounds attenuate IgE-mediated allergic response in vitro and in vivo via inhibition of mast cell degranulation. Eur. J. Pharmacol. 885:173435. doi: 10.1016/j.ejphar.2020.173435
Li, R., Zhang, Q., Liu, J., Sun, J., He, L., Duan, H., et al. (2020). Hydroxy-α-sanshool possesses protective potentials on H2O2-stimulated PC12 cells by suppression of oxidative stress-induced apoptosis through regulation of PI3K/Akt signal pathway. Oxidative Med. Cell. Longev. 2020:3481758. doi: 10.1155/2020/3481758
Liu, Y., Meng, X., Sun, L., Pei, K., Chen, L., Zhang, S., et al. (2022). Protective effects of hydroxy-α-sanshool from the pericarp of Zanthoxylum bungeanum maxim. On D-galactose/AlCl3-induced Alzheimer's disease-like mice via Nrf2/HO-1 signaling pathways. Eur. J. Pharmacol. 914:174691. doi: 10.1016/j.ejphar.2021.174691
Liu, R., Xu, Y., Zhang, T., Gong, M., Liu, R., Chang, M., et al. (2022). Interactions between liposoluble antioxidants: a critical review. Food Res. Int. 155:111104. doi: 10.1016/j.foodres.2022.111104
Liu, Y., Zhang, Y., Wei, X., Wu, D., Dai, J., Liu, S., et al. (2021). Effect of radio frequency-assisted hot-air drying on drying kinetics and quality of Sichuan pepper (Zanthoxylum bungeanum maxim.). Lwt 147:111572. doi: 10.1016/j.lwt.2021.111572
Lu, C. Q., and Lu, X. Y. (1995). Study on antioxidant and antibacterial effects of different kinds of Zanthoxylum bungeanum. J. Chin. Mater. Med. 20, 752–753.
Luo, J., Ke, J., Hou, X., Li, S., Luo, Q., Wu, H., et al. (2022). Composition, structure and flavor mechanism of numbing substances in Chinese prickly ash in the genus Zanthoxylum: a review. Food Chem. 373:131454. doi: 10.1016/j.foodchem.2021.131454
Ma, Q. (2013). Role of nrf2 in oxidative stress and toxicity. Annu. Rev. Pharmacol. 53, 401–426. doi: 10.1146/annurev-pharmtox-011112-140320
Mladenović, M., Mihailović, M., Bogojević, D., Matić, S., Nićiforović, N., Mihailović, V., et al. (2011). In vitro antioxidant activity of selected 4-hydroxy-chromene-2-one derivatives—SAR, QSAR and DFT studies. Int. J. Mol. Sci. 12, 2822–2841. doi: 10.3390/ijms12052822
Nahar, K., Hasanuzzaman, M., Rahman, A., Alam, M., Mahmud, J., Suzuki, T., et al. (2016). Polyamines confer salt tolerance in mung bean (Vigna radiata L.) by reducing sodium uptake, improving nutrient homeostasis, antioxidant defense, and methylglyoxal detoxification systems. Front. Plant Sci. 7:1104. doi: 10.3389/fpls.2016.01104
Nozaki, R., Kono, T., Bochimoto, H., Watanabe, T., Oketani, K., Sakamaki, Y., et al. (2016). Zanthoxylum fruit extract from Japanese pepper promotes autophagic cell death in cancer cells. Oncotarget 7, 70437–70446. doi: 10.18632/oncotarget.11926
Ouédraogo, L., Fuchs, D., Schaefer, H., and Kiendrebeogo, M. (2019). Morphological and molecular characterization of Zanthoxylum zanthoxyloides (Rutaceae) from Burkina Faso. Plants-Basel 8:353. doi: 10.3390/plants8090353
Peng, Q., Lu, Y., Mo, R., and He, Q. (2021). Antioxidant and nitrite-scavenging activities of Zanthoxylum bungeanum maxim. And Capsicum annuum L.: a synergistic, additive or antagonistic effect of the extracts? Eur. Food Res. Technol. 247, 2877–2885. doi: 10.1007/s00217-021-03845-4
Re, R., Pellegrini, N., Proteggente, A., Pannala, A., Yang, M., and Rice-Evans, C. (1999). Antioxidant activity applying an improved ABTS radical cation decolorization assay. Free Radic. Biol. Med. 26, 1231–1237. doi: 10.1016/S0891-5849(98)00315-3
Rice-Evans, C. A., and Diplock, A. T. (1993). Current status of antioxidant therapy. Free Radic. Biol. Med. 15, 77–96. doi: 10.1016/0891-5849(93)90127-G
Rong, R., Cui, M. Y., Zhang, Q. L., Zhang, M. Y., Yu, Y. M., Zhou, X. Y., et al. (2016). Anesthetic constituents of Zanthoxylum bungeanum maxim.: a pharmacokinetic study. J. Sep. Sci. 39, 2728–2735. doi: 10.1002/jssc.201600295
Son, S., and Lewis, B. A. (2002). Free radical scavenging and Antioxidative activity of Caffeic acid amide and Ester analogues: structure−activity relationship. J. Agric. Food Chem. 50, 468–472. doi: 10.1021/jf010830b
Subbaiah, P. V., Sircar, D., Lankalapalli, R. S., and Bittman, R. (2009). Effect of double bond geometry in sphingosine base on the antioxidant function of sphingomyelin. Arch. Biochem. Biophys. 481, 72–79. doi: 10.1016/j.abb.2008.10.005
Sun, X., Zhang, D., Zhao, L., Shi, B., Xiao, J., Liu, X., et al. (2020). Antagonistic interaction of phenols and alkaloids in Sichuan pepper (Zanthoxylum bungeanum) pericarp. Ind. Crop. Prod. 152:112551. doi: 10.1016/j.indcrop.2020.112551
Tomita, T., Kawano, Y., Kassai, M., Onda, H., Nakajima, Y., and Miyazaki, K. (2022). Hydroxy-β-sanshool isolated from Zanthoxylum piperitum (Japanese pepper) shortens the period of the circadian clock. Food Funct. 13, 9407–9418. doi: 10.1039/D2FO01036D
Tsikas, D. (2017). Assessment of lipid peroxidation by measuring malondialdehyde (MDA) and relatives in biological samples: analytical and biological challenges. Anal. Biochem. 524, 13–30. doi: 10.1016/j.ab.2016.10.021
Wang, Y., Zhong, L., Liu, X., and Zhu, Y. Z. (2017). ZYZ-772 prevents cardiomyocyte injury by suppressing Nox4-derived ROS production and apoptosis. Molecules 22:331. doi: 10.3390/molecules22020331
Xiao, B., Li, Y., Lin, Y., Lin, J., Zhang, L., Wu, D., et al. (2022). Eicosapentaenoic acid (EPA) exhibits antioxidant activity via mitochondrial modulation. Food Chem. 373:131389. doi: 10.1016/j.foodchem.2021.131389
You, Y., Ren, T., Zhang, S., Shirima, G. G., Cheng, Y., and Liu, X. (2015). Hypoglycemic effects of Zanthoxylum alkylamides by enhancing glucose metabolism and ameliorating pancreatic dysfunction in streptozotocin-induced diabetic rats. Food Funct. 6, 3144–3154. doi: 10.1039/C5FO00432B
Zhang, D. P., Lu, Y. M., Wang, Y. Z., Duan, C. Q., and Yan, H. Y. (2001). Acid invertase is predominantly localized to cell walls of both the practically symplasmically isolated sieve element/companion cell complex and parenchyma cells in developing apple fruits. Plant Cell Environ. 24, 691–702. doi: 10.1046/j.0016-8025.2001.00720.x
Zhang, D., Sun, X., Battino, M., Wei, X., Shi, J., Zhao, L., et al. (2021). A comparative overview on chili pepper (capsicum genus) and Sichuan pepper (zanthoxylum genus): from pungent spices to pharma-foods. Trends Food Sci. Technol. 117, 148–162. doi: 10.1016/j.tifs.2021.03.004
Zhang, Q., Tong, X., Sui, X., Wang, Z., Qi, B., Li, Y., et al. (2018). Antioxidant activity and protective effects of Alcalase-hydrolyzed soybean hydrolysate in human intestinal epithelial Caco-2 cells. Food Res. Int. 111, 256–264. doi: 10.1016/j.foodres.2018.05.046
Zhang, H., Zhou, X., Wong, M. H. Y., Man, K. Y., Pin, W. K., Yeung, J. H. K., et al. (2017). Sichuan pepper attenuates H2O2-induced apoptosis via antioxidant activity and up-regulating heme oxygenase-1 gene expression in primary rat hepatocytes. J. Food Biochem. 41:e12403. doi: 10.1111/jfbc.12403
Zhang, J., Zou, X., Zhai, X., Huang, X., Jiang, C., and Holmes, M. (2019). Preparation of an intelligent pH film based on biodegradable polymers and roselle anthocyanins for monitoring pork freshness. Food Chem. 272, 306–312. doi: 10.1016/j.foodchem.2018.08.041
Zhao, J., Huang, L., Sun, C., Zhao, D., and Tang, H. (2020). Studies on the structure-activity relationship and interaction mechanism of flavonoids and xanthine oxidase through enzyme kinetics, spectroscopy methods and molecular simulations. Food Chem. 323:126807. doi: 10.1016/j.foodchem.2020.126807
Keywords: antioxidant activity, Nrf2, oxidative stress, sanshool, structure–activity relationship
Citation: Chen Z, Tan L, Li L, Wang H, Feng X, Liu X and Rao C (2024) Sanshools from Zanthoxylum genus and their antioxidant properties in vitro and in vivo. Front. Sustain. Food Syst. 8:1256568. doi: 10.3389/fsufs.2024.1256568
Edited by:
Cristobal N. Aguilar, Autonomous University of Coahuila, MexicoReviewed by:
Cristian Torres-León, Autonomous University of Coahuila, MexicoLeonardo Sepúlveda, Autonomous University of Coahuila, Mexico
Copyright © 2024 Chen, Tan, Li, Wang, Feng, Liu and Rao. This is an open-access article distributed under the terms of the Creative Commons Attribution License (CC BY). The use, distribution or reproduction in other forums is permitted, provided the original author(s) and the copyright owner(s) are credited and that the original publication in this journal is cited, in accordance with accepted academic practice. No use, distribution or reproduction is permitted which does not comply with these terms.
*Correspondence: Xiong Liu, bGl1eGlvbmc4NDhAaG90bWFpbC5jb20=