- 1Natural Resources Institute Finland (Luke), Helsinki, Finland
- 2Finnish Institute for Health and Welfare, Helsinki, Finland
Introduction: Diet has a significant impact on the consumer’s climate impact, and a radical global change in the food system is necessary. However, the change needs to be interpreted and adapted to local conditions.
Methods: To support national climate policy, we evaluated current Finnish diet and its four alternatives: “current diet”; “meat to half diet”; “meat to a third diet”; “a diet rich in fish and milk”; and “a vegan diet”. We created the FoodMin model to simultaneously address both climate impacts and nutrient uptake and to combine carbon dioxide (CO2) emissions from soil organic matter (SOM) degradation with dietary climate impact assessments. As a well-established assessment method remains lacking, product group-specific estimates for the CO2 emissions of SOM origin were produced in two different ways, based on long-term observational data or by modelling with the Yasso07. We also examined, using three scenarios, how much the achievement of soil carbon (C) balance in Finnish production could affect the climate impact of the diet.
Results and Discussion: The climate impact of the current diet was 6.0 kg CO2 per person per day, and for alternative diets, the change compared with the current diet was −14%, −20%, −31%, and −39% respectively, for “meat to half” (5.2 kg CO2 eq. per person per day), “meat to third” (4.8), “a diet rich in fish and milk” (4.2), and “the vegan diet” (3.7). SOM-derived CO2 emissions from domestic fields accounted for 18% of the climate impact of the current diet and up to 23% of alternative diets. In terms of the soil C-balance scenarios, all actions together could mean a 3–13% reduction in the climate impact of the diet: the more products of animal origin, the more reduction opportunities in the diet. With the change in diet, these measures could reduce the climate impact of diets by 13–41%. The nutritional value of a “diet rich in fish and milk” was the best. The study revealed that SOM-induced CO2 emissions and SOM-related activities play a very important role in the climate impact of the diet and its mitigation; they cannot be ignored in dietary assessments in addition to direct product choices.
Introduction
Diet has a significant impact on the consumer’s climate impact. Scientific research has shown that switching to a strongly plant-based or vegan diet can significantly reduce the climate impact of diet in developed countries (Hallström et al., 2015; Poore and Nemecek, 2018).
Over the last decade, there have been considerable efforts to evaluate the nutritional aspects and environmental impacts of the national scale diet at the same time (reviews by Jones et al., 2016; Nelson et al., 2016; Payne et al., 2016; Perignon et al., 2016). Despite the methodological and data challenges related to the assessment of dietary sustainability (Jones et al., 2016), the general understanding of the need to reduce global consumption of animal products, particularly meat, has been achieved (Jones et al., 2016; Nelson et al., 2016; Payne et al., 2016; Perignon et al., 2016; Willet et al., 2019; Sun et al., 2022). In addition to environmental benefits, reduced consumption of animal products has been linked to several health benefits (Springmann et al., 2018, 2020; Jarmul et al., 2020). However, based on a review of 16 studies, Payne et al. (2016) concluded that more attention should be paid to an intake of sugar and essential micronutrients in the dietary recommendation for a reduced climate impact of diet, as has already been done in some recent studies.
A recent development has been the launch by the EAT-Lancet Commission of a global reference diet with the goal of a health benefit of dietary change accompanying the global climate targets (Willet et al., 2019). While a radical global scale transformation of the food system is necessary to keep the planet below a climate change of 2 degrees and to feed a growing population, a global reference diet needs to be interpreted and adapted locally so that the diet will “reflect the culture, geography and demography of the population and individual” (Summary report of EAT-Lancet Commission, 2020, p. 10).
When moving toward a more operational level evaluation and, e.g., political guidance, the country-specific circumstances and food culture should therefore be considered. Finland is the most northern agricultural country in the world. Due to natural conditions, e.g., cold winters and disciplined practices, there is a relatively good pest and disease situation in Finland, resulting in specific strengths for domestic crop cultivation, although the climate also limits the range and yields of crops. The current Finnish agriculture is therefore strongly based on cattle production, and diet is accordingly very rich in dairy products. Indeed, dairy products are very important sources of a range of essential nutrients in the Finnish diet (Valsta et al., 2018). Meanwhile, meat consumption (79.2 kg (with bones)/person) in Finland (Luke, 2020) is average compared to other EU countries, moderate compared to, e.g., the United States, and about twice the global average (OECD, 2020). Given that beef and some dairy products such as cheese are among the food products with the highest climate impact per kilogram (Poore and Nemecek, 2018), a large amount of them challenges the sustainability of Finnish agriculture and diet. However, there is a country-specific reason for the strong position and tradition of cattle breeding in Finnish agriculture: it is based on the comparative advantage of grass production, as natural conditions are most suitable for grass cultivation than for more demanding crops (Huhtanen et al., 2013). Finnish cattle production is largely supported by grass-based silage (Huhtanen et al., 2013).
In foods, the climate impact of beef is among the greatest (Poore and Nemecek, 2018), but grass cultivation has the potential for carbon sequestration (Nelson et al., 2016). The IPCC report on climate change and land (IPCC, 2019) also highlights sustainable land management in combating climate change, alongside a balanced diet, regarding possibilities in agriculture and food consumption. In Finland, according to the National Greenhouse Gas inventory, carbon dioxide (CO2) emissions from agricultural soils are higher than all other CO2 equivalent emissions in the agricultural sector (Statistics Finland, 2018). This is mainly due to the cultivation of drained organic soils (Statistics Finland, 2018).
The Life Cycle Assessment (LCA), specifically its impact category indicator Global Warming Potential (GWP), is a widely applied method to assess the climate impacts of foods and diets. However, its current practice insufficiently includes net CO2 balance due to the soil organic matter (SOM) degradation or sequestration in farmland as part of the assessment of climate impact (Goglio et al., 2015; Knudsen et al., 2019). Indeed, methodological guidelines of LCA also give varied guidance on the issue. For example, while the ISO 14040 series (ISO-EN, 2006a,b) addresses the link between food and farmland mainly as an area reservation, i.e., land use or land use change as hectares, a more recent standard for LCA of bio-based products, EN 16760 (EN, 2015), requires the inclusion of all greenhouse gas emissions from soil. On the other hand, an understanding of the dynamics of soil carbon is still emerging (Basile-Doelsch et al., 2020), and is thus also a feasible assessment method for food and agricultural LCA (Gheewala et al., 2020). Soils have high potential to act as a sink or source of carbon, and soil carbon balance depends on the type of crop, agricultural practices used, soil type and properties, soil water content, and climatic conditions (Valkama et al., 2020). Hence, considering that agricultural soils emit CO2 or sequester carbon from the air to a varied extent, the current assessment practice insufficiently catches the variation in emissions and the climate impact of products, as well as the diets based on the products.
This paper deals with these interlinked issues. It examines the potential large-scale dietary change to greater sustainability, and the relationship of this change to nutrient intake from diet and the climate impact of diet, paying attention particularly to soil CO2 emissions in Finnish conditions. The evaluation is based on a comparison between current and modified diets targeted at complying with the national nutrient intake recommendations for both genders and different age groups.
The study aimed to provide information for policymaking on sustainable food policy in Finland, paying special attention to the nutritional and climate impacts of diet.
Materials and methods
Basic methodological choices in life cycle assessment
The climate impacts of the current and alternative diets were compared using the LCA approach. In LCAs, a functional unit (FU) acts as a reference unit to which impacts are allocated and based on which the systems are compared. In this study, two FUs were applied: (1) the average daily diet of a Finnish consumer; and (2) the energy (2,200 kcal) standardised daily diet of an average Finnish consumer. The former is used in most analyses, but the latter only in comparisons between FUs. According to the LCA guidelines (ISO-EN, 2006a,b), the FU should be clearly defined, and in comparative studies like this study, the comparison should only be made on the basis of the same FU. This principle is challenging LCAs of food and diet, because complex nutritional quality should be included in FU as a key feature of foods and diet (Heller et al., 2013; Saarinen et al., 2017; Saarinen, 2018; McAuliffe et al., 2020; McLaren et al., 2021). In this study, nutritional aspects are considered by (1) further specification of nutritional quality for alternative diets, (2) an assessment of the nutritional quality of all comparable diets, and (3) applying additional energy standardised FU.
In LCA studies, it is important to distinguish the FU from the reference flow (RF) (Heller et al., 2013; Saarinen et al., 2017; Saarinen, 2018; McLaren et al., 2021): when the FU serves as the basis of the comparison and reflects the service provided by the product(s) under consideration, the RF instead indicates how many materials, or products, are needed to produce that service (ISO-EN, 2006a,b). In this study, the RF for the first FU applied consists of the foods included in the diets, i.e., food content. Instead, RF for the second FU has not been separately determined and shown in the results, but it conforms to the RF for the first FU so that the ratios of the amounts of foods are the same, but the absolute amounts differ.
The system boundary describes the phases and emission sources included in the assessment. In this study, the system boundary includes food consumed (including the agricultural phase), industrial manufacturing and cooking in households, consumer food waste, and CO2 emissions from soil organic matter (SOM) degradation as described below. Not all the transport or packages are included in the assessment, because they are not expected to differ significantly between diets, and based on previous studies, other phases of the Finnish food system determine climate impacts (Seppälä et al., 2011; Virtanen et al., 2011), particularly agricultural production.
Diet formulation
The current and alternative diets were compared. The current diet was based on data from a regular food consumption FinDiet 2017 survey for Finnish adults (Valsta et al., 2018; Kaartinen et al., 2020). It was supplemented with Finnish data on the consumption of food by children (Hoppu et al., 2008; Kyttälä et al., 2008) and the elderly (Montonen et al., 2008). Because the composition of the current diet was based on surveys known to be associated with underreporting, it was compared with statistical data on food consumption in Finland in 2016 and 2017 (Luke, 2018). On this basis, “a corrected current diet” was also formed, to which the food consumption of some product groups such as cereals (+16–87%), potatoes (+46%), beer (+24%), and sugar (+16%) was added.
Alternative diets for the two genders and different age groups were modified from the current diets so that they followed the Finnish nutrient recommendations (VRN, 2014, 2017; THL, 2019) starting by following the food-based dietary guidelines, but by reducing the meat intake gradually. Final reconciliation with NR was made by fine-tuning product group consumption as needed. Thus, the difference between diets was based on varied consumption level of product groups using the levels of the current diet as a starting point. Consumption levels were adjusted for women and men and for nine age groups separately, following (1) the Food based dietary guidelines (FBDG) and (2) the nutrient-based recommendations (NR) (VRN, 2014).
Finally, the diets were formed based on the population-weighted average consumption of 91 food product groups, which were also aggregated in 14 food product groups. Weighting is based on the shares of genders and age groups in the Finnish population in 2017 (Statistics Finland, 2017). Alternative diets were: (1) a diet with meat reduced by half; (2) a diet with meat reduced by two thirds; (3) a diet rich in fish and milk; and (4) a vegan diet.
Estimation of the climate impacts and nutrient intake of diet
Alternative diets were designed using the Excel-based FoodMin dietary model, which was created in this study. The FoodMin model integrates environmental and nutritional aspects to ensure the climate impacts of diets and nutrient intake from diets can be assessed simultaneously, and nutritionally balanced diets can be designed. The model’s basic structure is presented in Figure 1.
The FoodMin dietary model consists of LCA-based climate impact estimations for 91 food product groups and following the food categorization used in the FinDiet 2017 survey (Valsta et al., 2018; Kaartinen et al., 2020) and Fineli® food composition database (THL, 2019). A more detailed description of the methodology and data used in the FoodMin diet model is presented in the Supplementary material. Climate impact estimates for food product groups were completed with a calculation of carbon dioxide (CO2) emissions from agricultural soils and estimations of consumer biowaste (Figure 1), as described below.
Furthermore, this food product group-based approach was completed with energy use for domestic cooking and other food manufacturing methods and related greenhouse gas emissions (Figure 1; Supplementary Table S2).
A diet’s nutrient intake was calculated based on the nutrient composition of about 800 products within the 91 product groups (THL, 2019); some product groups consisted of just a few products, while others consisted of dozens. For a vegan diet, exceptions to this general principle were made in some product groups to provide adequate and balanced nutrients in accordance with the Finnish nutrition recommendations. The most important exceptions were in fats, where fortified margarines played a central role, and milk substitute products such as oat drink, where only fortified products were included. In addition, in nuts, for example, some products such as macadamia nuts were omitted.
The average value of 35 nutritional factors was calculated for each product group. The minimum and maximum values of those factors were also determined for the product groups by selecting them individually, one nutritional factor at a time, i.e., not based on the lowest or the most nutritious product in the product group. The average nutritional values for product groups are presented in Supplementary Table S3.
The model calculates nutrient intake from every product group and adds them together to form the nutrient intake of the entire diet for two genders, nine age groups, and two different activity levels for adults and the elderly. The model also compares these values with the Finnish NR for genders and nine age groups (Supplementary Table S4). Due to these features of the model, it allows dynamic design and the adjustment of diets.
Method for estimating consumer food waste
Consumer food waste was added to the assessment of the climate impact of diets at the level of product groups as a separate measure. It is notable that food waste and loss from the production chain are included in the LCA-based climate impact estimations for the products groups. The data for consumer food waste estimation were based on the previous household diary study, including data on originally edible food waste (Silvennoinen et al., 2014), and the food waste coefficients were produced as described in the Supplementary material.
Food waste coefficients were produced for 14 food categories: vegetables (9%); potatoes (10%); fruit and berries (10%); bread (7%); other cereals (8%); rice and pasta (8%); milk (3%); cheese (5%); other milk products (4%); pork and beef (6%); poultry (3%); fish (6%); beans (4%); and other (4%). These were used for the appropriate product groups from a total of 91 food groups.
Estimation of carbon dioxide emissions from agricultural soils
In order to integrate SOM-origin CO2 emissions from agricultural soils into the FoodMin model, product group-specific emission estimates (kg CO2 per kg of product) were required. They were produced in a stepwise process.
At first, the change in soil organic carbon (SOC) stock per ha was assessed. As the assessment methods for LCA are not yet well established, the change in soil C stock in mineral soils was estimated in two different ways: based on (1) observed data from the Finnish national soil monitoring network classified according to management and region; and (2) modeling the C stock change using the Yasso07 soil carbon model. Emissions from organic soils (OM > 20%) were estimated as in the national greenhouse gas inventory (Statistics Finland, 2021). The emission factors for organic soils growing fodder grass were 5,700 kg C ha−1 yr.−1 and 7,900 kg C ha−1 yr-1 for annual crops (Hiraishi et al., 2014).
In terms of management practices, fields growing annual or perennial crops more than 80% of the time were classified as “annual” or “perennial,” respectively. The remaining fields were classified as “rotational.” Regionally, the data were divided in 4 different regions – north, east, west, and south – according to the study by Heikkinen et al. (2013).
The change in soil C stock within these management and region combinations were determined based on measured soil C contents between 2009 and 1998. The change in soil C stock was modeled using generalized linear models, as described in Heikkinen et al. (2013).
To convert area-based data to mass-based data (i.e., kg CO2 emissions per kg of different crops), cultivated areas of crop plants (Figure 2) in each group of soil class, management, and regions were determined based on geospatial datasets, The Food Agency’s crop database and Finnish Soil Database (Lilja and Nevalainen, 2006).
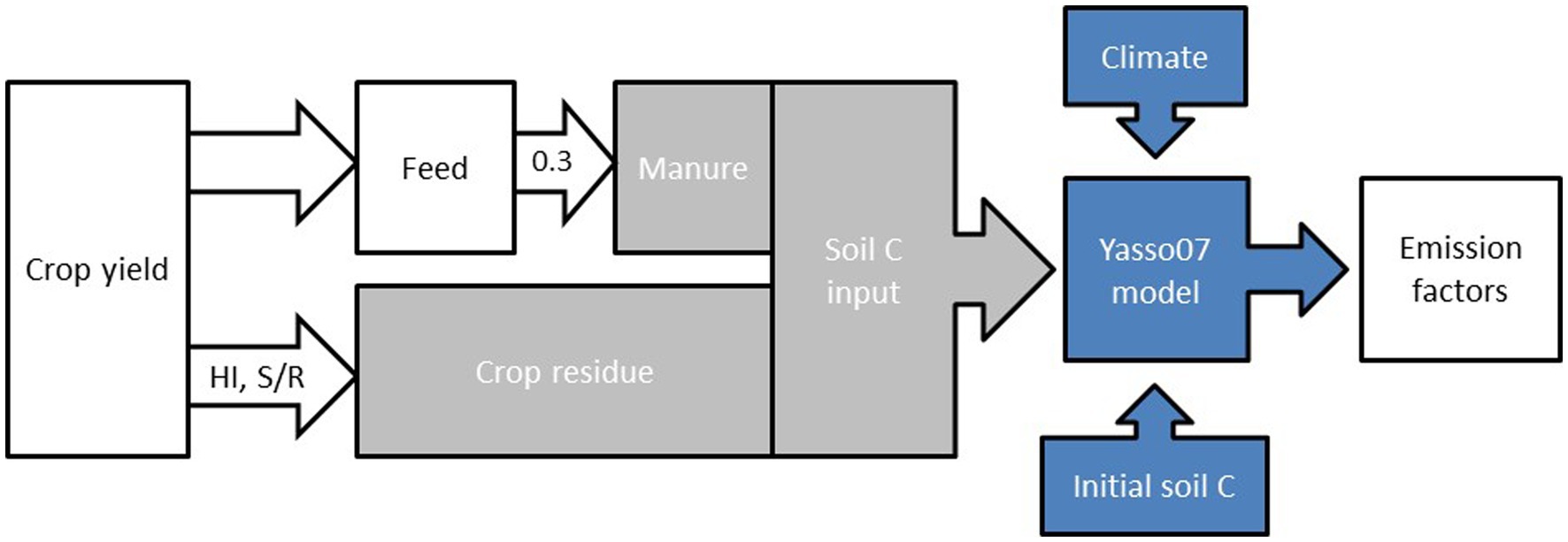
Figure 2. Schematic workflow to calculate crop specific emission factors using Yasso07 soil carbon model. HI, harvest index and S/R, shoot to root ratio.
Modeling was performed using the Yasso07 soil C model, which is a widely used model in various forest, agricultural, and land use change studies (Tuomi et al., 2009). Several countries, including Finland, use the Yasso07 model in national greenhouse gas inventories. Yasso07 predicts the decomposition of organic material based on climatic condition and litter chemical quality. In this study, soil CO2 emissions were calculated following the calculation procedures used in the Finnish GHG inventory (Statistics Finland, 2021) as closely as possible.
The C input of agricultural soils consists mainly of crop residues and manure. In this study, both crop residues and manure-derived carbon inputs were estimated based on crop yield statistics. First, the fraction of crop yield to be used to feed livestock was determined (Table 1). Yields were then converted to crop-residue-derived carbon using the crop-specific harvest indexes (HI), shoot to root ratio (S/R), and root turnover rates. Conversion factors corresponded to those used in the Finnish GHG inventory (Figure 2). Manure-derived carbon was calculated by assuming that 30% of the biomass of feed remained after livestock digestion. The chemical quality of plant residues and manure were taken from the Finnish GHG inventory. Finally, manure- and crop-residue-derived carbon inputs were summed.
Emission factors for each crop plant were determined using the Yasso07 model and the above soil carbon input estimates and climatic data from the Finnish GHG inventory (mean annual temperature, temperature amplitude, and annual precipitation). Model initialisation was done as in the GHG inventory, taking the historical change from forest to cropland into account. For each crop plant, the model was run for 20 years. The crop-specific emission factors were determined as the average change of soil carbon stock during the 20-year modeling period.
Finally, the product-group-specific CO2 emissions from agricultural soils (kg CO2 per kg of a product) were assessed and scaled up to the dietary level using the formulas
where Em (CO2 soil) is product-group-specific kg CO2 emission per kg of a product; S (org) is the crop-type specific share of cultivated organic soils; EF (org) is the crop-type-specific emission factor for organic soils (kg CO2 per ha); EF (min) is the crop-type-specific emission factor for mineral soils (kg CO2 per ha); Y is average yield level (kg/ha); Em (CO2 soil) diet is total kg CO2 emission per diet from domestic soil CO2 emissions. In the calculation of emission estimates for the product groups, the average of the CO2 emissions (per kg) obtained in the two ways described above was used, while the original results were used as extremes of variations.
Crop plants were classified as annuals (cereals, potatoes, and vegetables) and perennials (grass). The emissions were targeted at product groups based on products’ main raw material. The raw material basis was obtained from previous LCA studies of the products, including the product group or the public sources for recipes, or they were based on the supposition that more accurate information was unavailable. For animal products, feed consumption was changed to meat yield (kg) per ha for calculation in the FoodMin model. Data on feed consumption were obtained from previous LCA studies. Data for crop yield and soil type distribution for crops were based on Finnish Soil Database (Lilja and Nevalainen, 2006).
Scenarios on effects of soil carbon balance
Finnish agricultural soils emit a significant amount of CO2, but many activities are currently aimed at significantly increasing the soil C sequestration potential. Some Finnish dairy and meat companies have even expressed their goal of achieving carbon neutrality in their milk and meat products, and soil C sequestration is one of the most important means there. The study used three scenarios to examine how much achieving soil C balance in Finnish production could affect the climate impact at the dietary level. The scenarios were: (1) soil C balance could be achieved in milk and beef production in which emission from organic soils was highlighted; (2) soil C balance could be achieved in mineral soils; and (3) the above conditions are both met. In the scenarios, these emissions were simply set to zero in the FoodMin model.
Results and discussion
Food content and nutritional quality of the diets
The food composition of the diets based on the population-weighted average consumption for 14 food product groups are presented in Table 2, and for 91 product groups in Supplementary Table S5. The food composition of the diet indicates what foods are needed together to implement the FU.
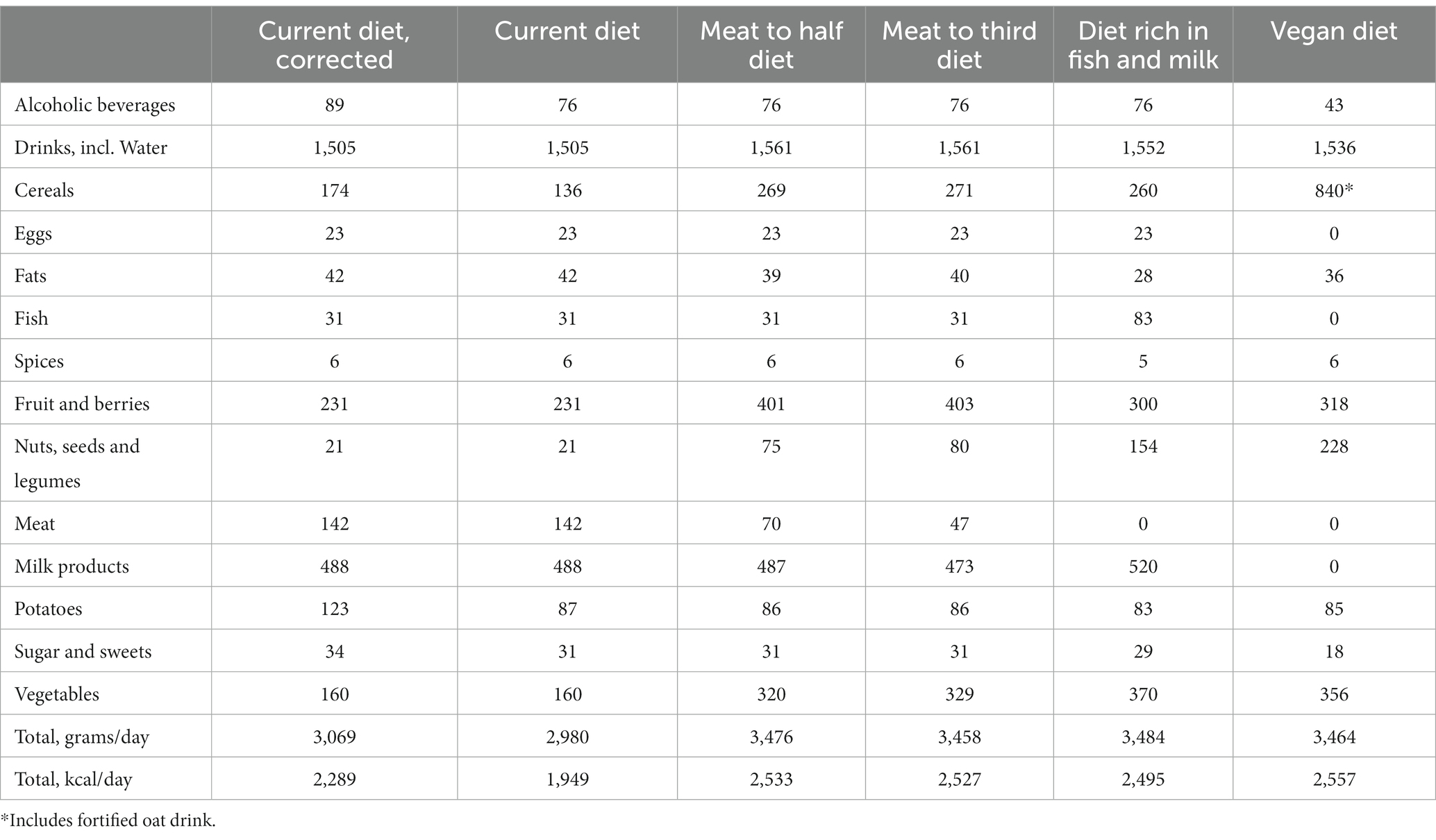
Table 2. Food content of the diets based on population-weighted average consumption for 14 food product groups.
The vegan diet has the most changes in food content compared to the current diet. Even the consumption of alcoholic beverages and sugar and sweets had to be significantly reduced to make room for the nutritious products needed to meet the NR. Additionally, the amount of fats changed little, but there were significant qualitative changes, and, unlike other diets, fortified products had to be strongly favored (e.g., fortified margarines and oat drinks). In diets still containing meat products, even more amounts of fruit and berries needed to be added than in the FBDG to improve the nutritional quality of those diets and meet the nutrient-based recommendations. In all alternative diets, the consumption of cereals, vegetables and nuts, seeds, and legumes significantly increased compared to the current diet, in line with the FBDG.
According to the results, the current diet deviates considerably from the nutrition recommendation (Supplementary Table S6). For adults, this largely accords with the results from the National FinDiet 2017 Survey (Valsta et al., 2018; Kaartinen et al., 2020). A comparison of the results from this study and FinDiet 2017 is shown in Table 3 and further discussed below.
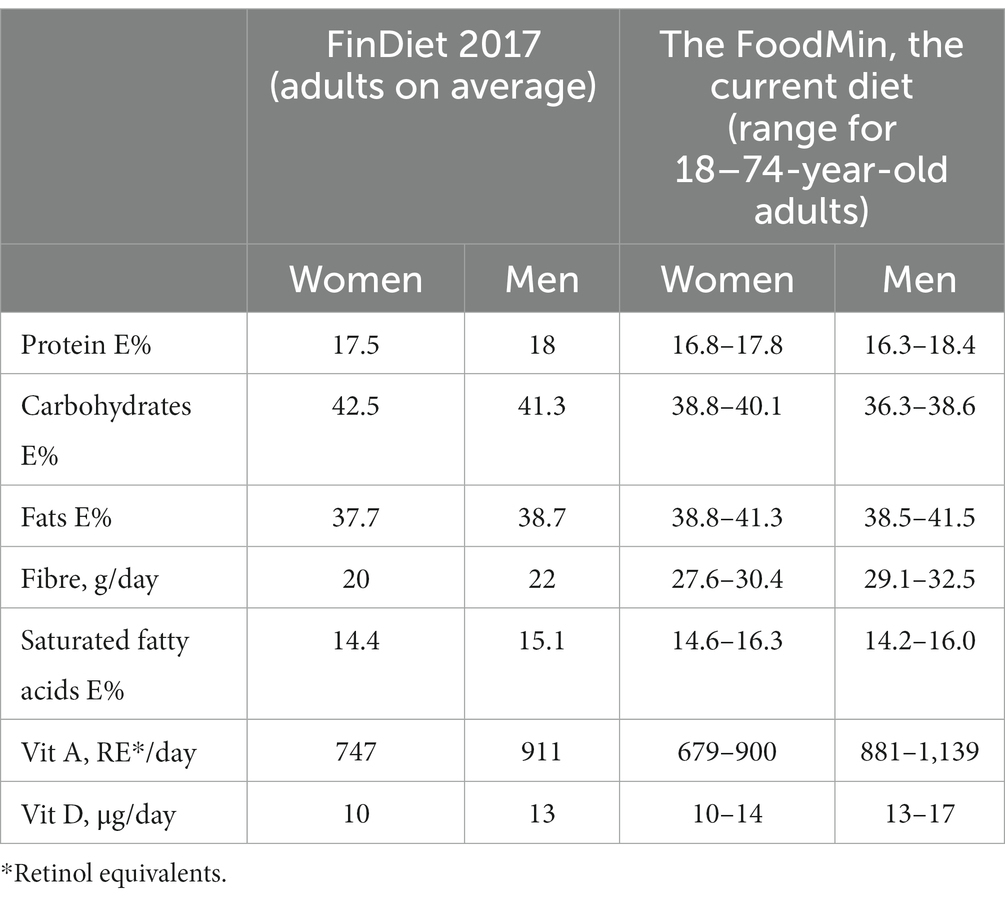
Table 3. An intake of some essential nutrients according to the FinDiet 2017 survey (Valsta et al., 2018) and this study.
Furthermore, despite adjusting food consumption in the alternative diets in relation to the NR, a slight inconsistency regarding some energy nutrients and micronutrients remained in the diets, mostly in the vegan diet and meat to half diet (Supplementary Table S6). In general, these inconsistencies were related to fatty acid composition and vitamin D and iodine intake, both of which are particularly well-known challenges in relation to the Finnish diet (Valsta et al., 2018). It is recommended for all population groups to supplement vitamin D intake with a nutrient supplement at least during the winter (VRN, 2014). Vitamin D supplementation of margarines are also an important source of vitamin D.
In this study, an intake of iodine was insufficient in the vegan diet, as well as in meat to half and meat to a third diets for children under 13. At present, dairy products are the most important ‘natural’ sources of iodine in Finland with the share of over 30% (Valsta et al., 2018), but iodine is usually also added to the salt used in the food industry and for table salt which makes salt the most important source of iodine (40%). Overall, an intake of salt is abundant compared to the recommendations, and much of the intake comes from meat products, but also cereal products, like bread. However, the change in salt intake and thus iodine intake due to a large change in the diet is difficult to predict, especially because such a change can also affect the use of salt in the food industry and for home-cooked foods in plant-based foods. The outcome of this project on iodine intake must therefore also be interpreted with caution.
Interpretation of the quality of a fatty acid composition, in turn, is complicated, among other things, because its recommendations are tied to total energy intake, and they generally are expressed by a range. However, the recommendation (which is based on a range) for unsaturated fatty acids refers to a minimum intake, so exceeding the values is probably not critical. The results showed varying, even contradictory, values in different age groups in different diets (Supplementary Table S6). However, an intake of poly-unsaturated fatty acids (PUFA) generally exceeded the recommendation in the alternative diets while intake of mono-unsaturated fatty acids (MUFA) was lower than is recommended. The recommended intake of DHA was not achieved in the vegan diet because it is obtained only from products of animal origin.
As summary, the nutritional quality of the diet rich in fish and milk was the best. Its only shortcoming was the imbalance in MUFA and PUFA intake (Supplementary Table S6).
In addition, energy intake from diets differed between diets (Table 2) being 20% lower in the current diet and 8% lower in the corrected current diet compared to the alternative diets. Energy intake from alternative diets were according to the recommendations, but under the recommendations from the current diet. It is noteworthy, however, that the current diet was based on survey, which typically involves underreporting about 20% of energy intake. The effect of difference in energy intakes on the interpretation of climate impacts results is discussed below.
Climate impacts of diets
The current diet and its alternatives
According to the results, the climate impact of the current Finnish diet is 6.0 kg CO2 per person per day (Figure 3). This is greater than previous studies (Risku-Norja et al., 2009; Vieux et al., 2018), but without SOM-origin CO2 emissions, it accords with them. SOM-origin CO2 emissions from domestic fields causes 23% of the total climate impact of the current diet, and up to 19% for alternative diets.
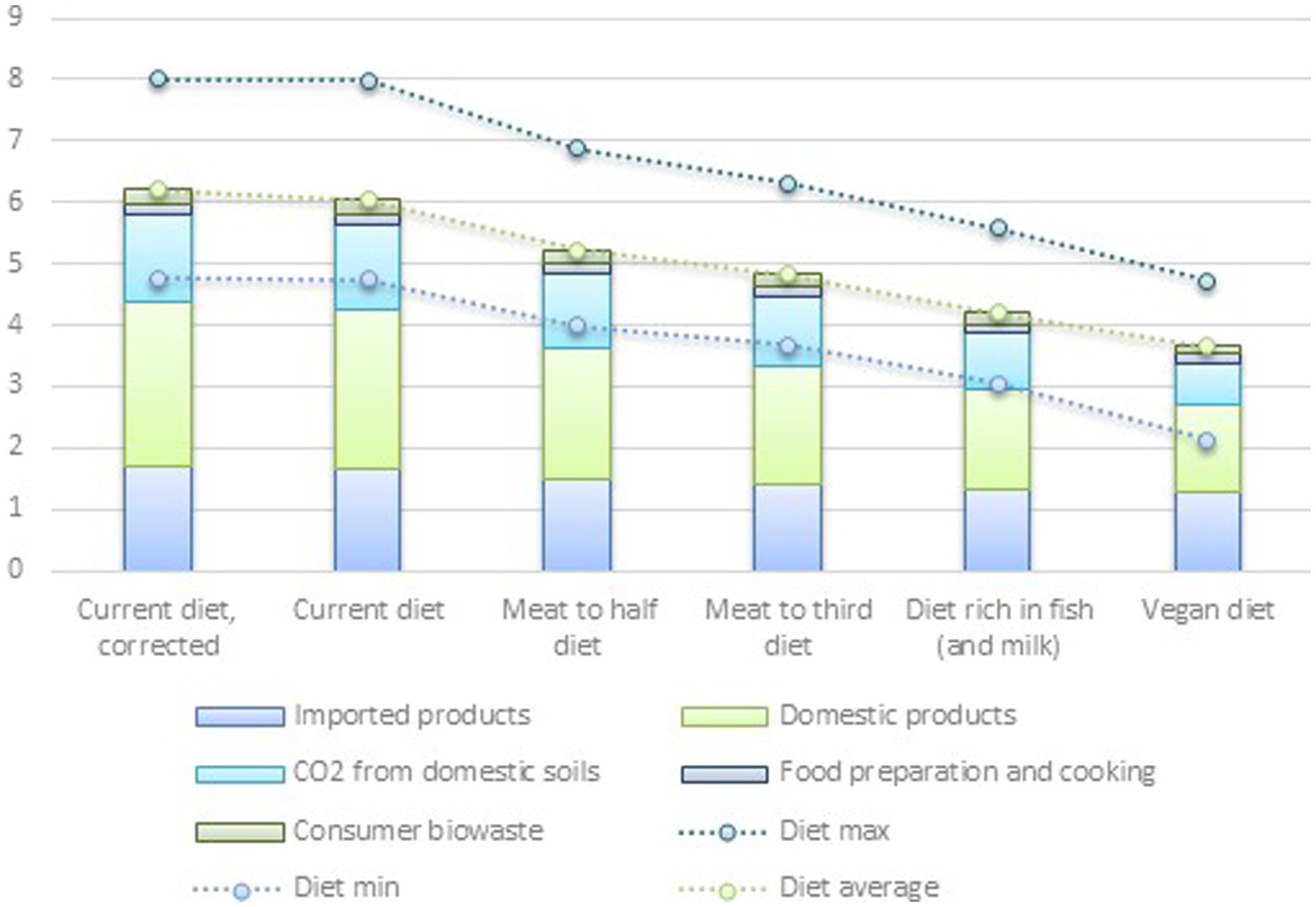
Figure 3. Climate impacts of diets, kg CO2 per person per day, and its potential variation due to product choice and variation in calculation of soil CO2 emission.
There is a decreasing trend in the climate impact of a diet containing fewer animal origin products (Figure 3), which accords well with much of the previous research (review provided by Jarmul et al., 2020). Thus, the biggest change compared to the current diet was obtained with the vegan diet alternative (−39%) (3.7 kg CO2 eq. per person per day). This is a slightly smaller decrease than the global estimate provided by Poore and Nemecek (2018) (−48%) and even smaller than the result from the review provided by Jarmul et al. (2020) (−70%). However, the comparison is hampered by the fact that the methods differ. For example, Jarmul et al. (2020) included articles on the health impact assessment in the review, not nutrient intake assessments as in our project.
For other diet alternatives, the reductions compared to the current diet were 14, 20, and 31%, respectively, for halving the amount of meat (5.2 kg CO2 eq. per person per day), the meat to third (4.8), and the diet rich in fish and milk (4.2) (Figure 3). The climate impact of the corrected current diet was 3% higher than in the current diet (6.2), and the reductions would be slightly greater when alternative diets were compared to the corrected diet instead of the current diet. This difference is due to the underreporting of the consumer food survey, and it seems to have only a small effect on the results. This is explained by the fact that the underreporting focuses on foods with a low climate impact per mass unit.
Although the trend is clear, there is also a very large variation within the diet alternatives (Figure 3). It suggests that product choice within product groups has a large impact on the climate impact of diet, because most of the variation is due to a difference between minimum and maximum climate impact coefficients for the product groups (Supplementary Table S1). This also highlights the importance of diet design in studies on the environmental impacts of diets; the product classification should be sufficiently detailed, and the product range sufficiently broad.
In general, domestic production contributes most to the climate impacts of every diet alternative. The effect of domestic production comprises the production chains of products (domestic products in Figure 3), which accounts for 39–43% of the total climate impact of the diets, and SOM-origin CO2 emission (19–24%). However, there is an increasing trend in the share of imported products, with a decreased amount of animal origin products in the diet (Figure 3). The share of imports is thus highest in the vegan diet. However, it is notable that the absolute amount of the climate impact related to imports does not increase, meaning that the climate impacts of food consumption will not necessarily be outsourced more than today if plant-based food were consumed more.
In this study, originally edible consumer food waste (3–4% as a share of the climate impact) and the manufacturing stage (2–4%) played only a small role in the climate impact of diets. It is noteworthy that waste generated at stages other than the consumer stage is not included in the estimate of food waste and for the consumer stage according to household food waste rates, thus not considering food waste rates in food services. In this study, the climate impacts associated with food waste generated in the production stages are included in the coefficients of domestic and imported products. Food services were not considered separately, because their share of food consumption was low in the food consumption data used. Our results are somewhat in line with or perhaps a little less than in other studies such as Poore and Nemecek (2018), according to which the share of the climate impacts from food loss and waste related to the whole food chain was 13%, and manufacturing 4%. Scherhaufer et al. (2018) estimate the food loss and waste in European food chains (16–22% of the total emissions of consumed food), and Hallström et al. (2021) for Sweden (18% of the dietary climate impact). However, due to wider system boundaries in the assessment of food waste in these other studies a direct comparison of these results with our results is not possible.
Energy standardised diets
When an energy-corrected diet (2,200 kilocalories) was used as the FU, the difference between the current diet and other diets increased due to both the increased impacts of the current diet and the decreased impacts of the other diets (Figure 4). It is noteworthy that the climate impact of the corrected current diet decreased only slightly, because the energy intake of the corrected diet was close to 2,200 kilocalories (Table 2). Meanwhile, the energy intake from the current diet was below 2,200 kilocalories (Table 2), and the energy correction increased the climate impact fairly considerably. However, this scaling up seems incorrect, because the underreporting associated with consumption surveys is not associated with all products equally (Table 2; Supplementary Table S5), as the scaling up assumes. Thus, the climate impact for the corrected current diet is assumed to be closer to the reality than for the energy-corrected current diet. Interestingly, the climate impact for the corrected current diet is quite similar to the current diet without an energy correction.
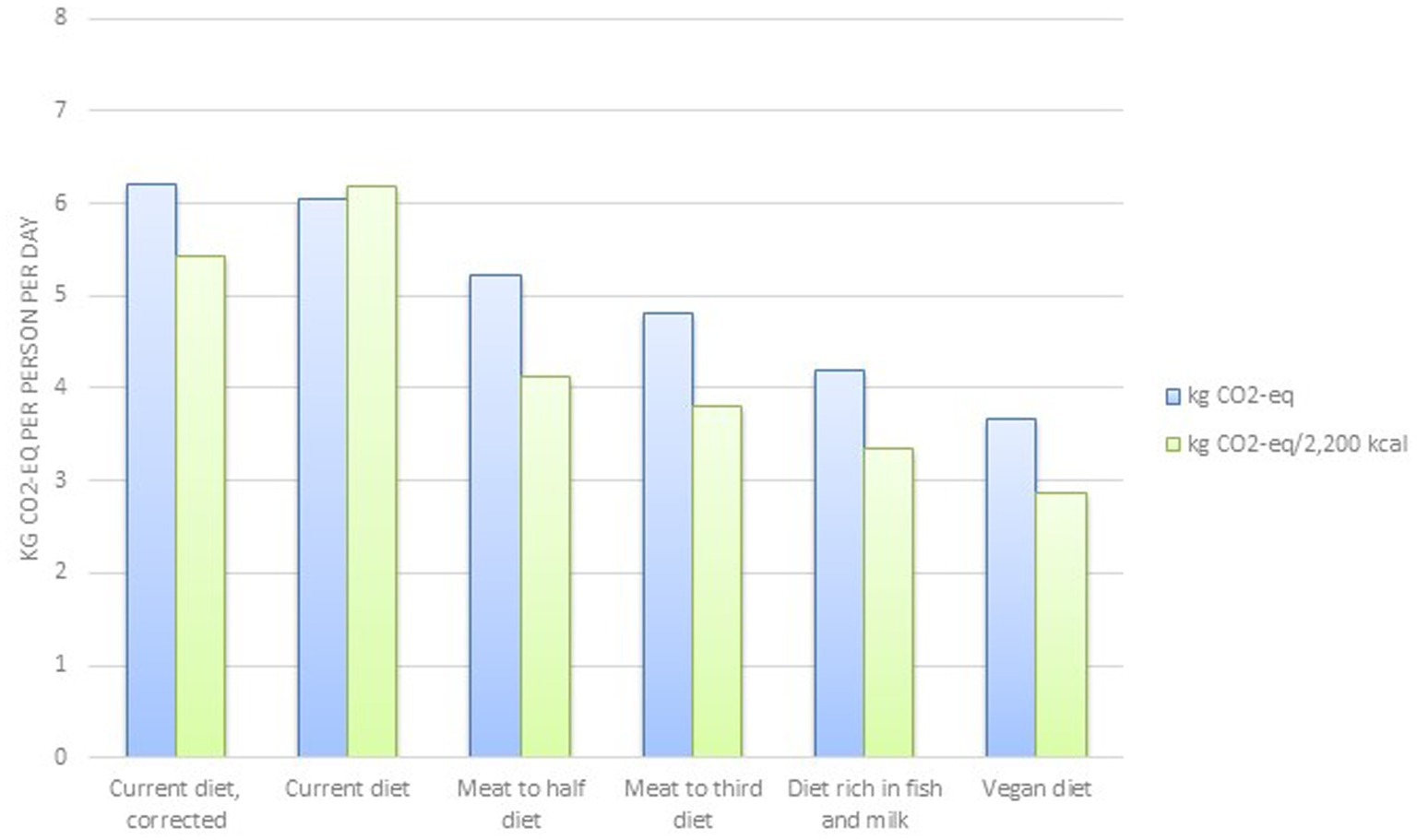
Figure 4. Comparison of the climate impacts of the diets and energy-corrected diets (energy intake standardised to 2,200 kilocalories).
For the alternative diets, energy intake was significantly higher than 2,200 kilocalories (Table 2), and the energy correction thus decreased the climate impact. However, these results should be interpreted with great caution, because the nutrient intake from the diets has changed. As the aim is to compare the climate impact of nutritionally adequate diets, the nutrient intake compared with the nutrition recommendation should be ensured before final conclusions.
Soil carbon balance scenarios
According to the results of soil C balance scenarios, the climate impact of diets with animal-based products could be reduced by 7–12% if SOM-origin CO2 emissions from cattle production could be prevented (Figure 5). Instead, achieving C stock balance in mineral soil affected only 2–4% of the climate impact of the diets. Thus, these actions together could mean a 3–13% reduction in the overall climate impact of the diets. The most reductions would be in the diets with the most animal-based products (i.e., the current diet). With a dietary change, these actions could mean a 13–41% reduction in the overall climate impact of the diets.
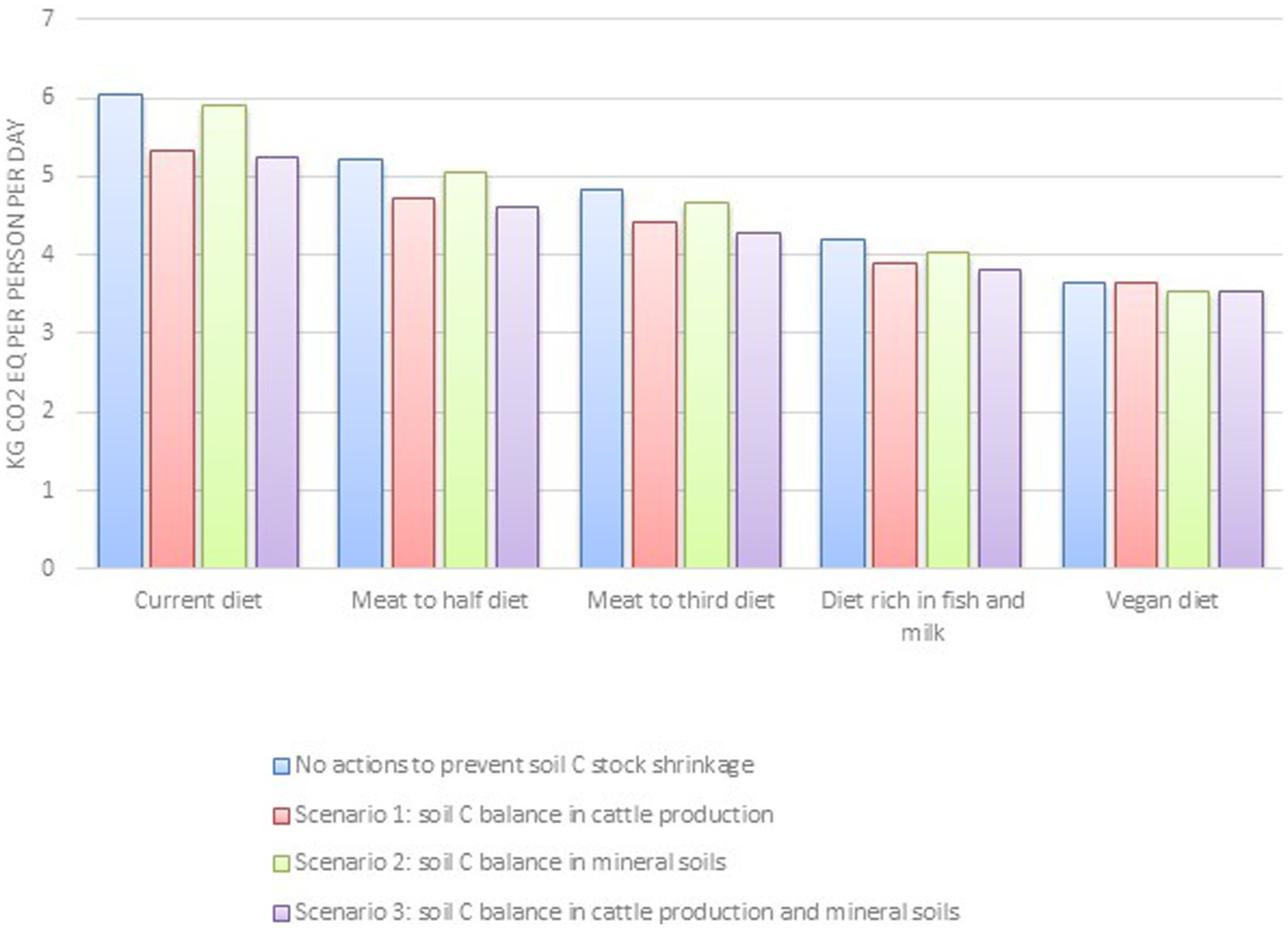
Figure 5. Effects of soil C stock change scenarios on current diet and its alternatives. The most reductions would be in the diets with the most animal-based products (i.e., current diet) as the effect of balancing soil C in cattle production has greater potential than in mineral soils.
Interestingly, if these actions were successfully completed, the climate impacts of a diet rich in fish and milk and the vegan diet would be close to each other (−37% and − 41% respectively), and even the climate impact of the diet with meat to a third (−29%) was close to them. This highlights the importance of soil C as a factor affecting climate change – in this case, as a source of CO2 emissions. Soil as a potential carbon sink was not considered in this study.
We did not take a position on how these changes would be achieved. In practice, however, CO2 emissions of organic soils can only be excluded by total rewetting or by excluding organic soils from the food system in LCA. In mineral soils, carbon balance could be achieved by diversifying the cropping systems, cultivation of cover crops, and application of organic amendments (Heikkinen et al., 2021). However, these actions might have indirect effects on carbon leakage and storage elsewhere that were not considered in this study. Several other measures are also proposed to reduce emissions from cattle production, such as the removal of methane from the air of animal shelters (e.g., Pratt and Tate, 2018).
Uncertainty and limitations
Nutritional quality and food composition of diets
Each product group in the FoodMin model to which consumption data applies includes multiple products; the model is thus based on aggregated consumption data. However, when calculating the nutrient intake, the products of the product groups and their different nutrient contents are considered in the model when producing the average nutrient content of the product groups. This procedure creates uncertainty in assessing the nutrient content of a diet, because it does not directly consider what products consumers have actually consumed.
To assess this uncertainty, an assessment of the nutritional quality of the current diet in the FoodMin model was verified against the results of the FinDiet 2017 Survey (Valsta et al., 2018) (Table 3). The results of the FoodMin model for the adult population (18–74 years) were used in the verification, because the FinDiet 2017 Survey targeted these age groups. According to the results of the comparison, the FoodMin model slightly underestimates the intake of proteins and carbohydrates and overestimate the intake of fats and particularly fibre. It also somewhat overestimates the intake of vitamins A and D. These inconsistencies appear to be due in particular to the assessment of nutrient intake from fats.
Another cause of uncertainty is data quality on food consumption in children, adolescents, and the elderly. It is much lower than in adults, which reduces the results’ reliability. On the other hand, it is important to consider all age groups, even based on weaker data, because there are clear differences in their food use: average food consumption does not apply to all age groups. In addition, both children and the elderly represent vulnerable groups to which special attention should be paid in dietary change. The final age- and gender-group-specific results of this study are beyond the scope of this paper and will be published elsewhere, but the tentative results reveal large variation in the climate impacts of these groups.
Furthermore, the amounts of foods and the energy content of the alternative diets were significantly higher than those of the current diet. How reliable is this? The alternative diets were designed to meet lower limits of nutrient intake according to the Finnish nutrition recommendations. Foods typically include several nutrients in different abundance. In the FoodMin model, diets are designed by manually adjusting the intake of food groups one at a time relative to the nutrient-based recommendation, although the model simultaneously evaluates the nutritional quality and compliance with the recommendations. As a result of the modeling, the intake of common nutrients significantly exceeded the lower recommended limits when ensuring an intake of more rarely appearing nutrients, although not to the extent that would be harmful to health (the upper limit of the recommended intake when applicable). It is therefore possible and even likely that the diets included some extra food. A more systematic diet optimisation in relation to the lower limit of the recommended intake would be possible using linear programming, as has been done in some studies for adults’ diets (e.g., Vieux et al., 2018). However, in this study, data covered both genders and several age groups, and the modeling approach was chosen to support iteration in all these groups simultaneously. The modeling could be refined for the group-specific optimisation in further studies. The approach applied in this study also facilitates an assessment of the impacts of the FBDG on nutrition intake and the environment, and it therefore supports national policymaking on sustainable diets guidance. Springmann et al. (2020) highlighted this issue by showing that national FBDG could be globally both healthier and more environmentally sustainable.
Another reason for the possible extra intake of food in alternative diets is due to the recommendation used. The lower limit of the recommended intake in the Finnish nutrient recommendation refers to the average nutrient requirement plus twice the standard deviation (SD) in the individual person’s nutrient needs, i.e., not the average need. The idea is that when the recommendations are applied in public catering, for example, food meets the nutrient needs of 97% of eaters. For example, the Nordic recommendations refer to average needs, and the reference values (average recommendation, AR) are therefore lower. However, these values cannot be used for average diets without distribution based on individual diets as in this study. In the end, the principle applied in the Finnish recommendations also is safer for public nutrition, but probably results in a slightly higher climate impact for alternative diets.
Climate impact coefficients of products
This study followed a bottom-up approach, meaning that the coefficients for the product groups were based on the life cycle climate impact assessments of products upscaled to present the entire product groups. This creates similar uncertainty to that encountered in assessing the nutrient intake from product groups. In addition, reliable and representative LCAs are available only for a reasonably small number of products, particularly in product groups with a large variation, such as cereals, seafood, and fish, as well as legume based products. Such product groups still exist in the FoodMin model, although it is based on food ingredients, not dishes (the data on which are only used for estimating emissions related to manufacture and cooking). Many of these product groups contain potentially innovative new products that have emerged or will emerge out of a possible dietary change. Little is still known about their climate impact, which causes uncertainty, especially in the assessment of alternative diets.
In general, it is currently impossible to estimate or manage all the uncertainty associated with dietary assessments because of the complexity of the issue, and the lack and the potential mismatch of data. The FoodMin model attempts to show this by providing the climate impact results with a range (Figure 3), based on the average, minimum, and maximum climate impact coefficients for the product groups (Figure 3). However, the range represents the hypothetical range of the climate impacts of individual diets rather than the average diet, because it is formed directly based on extremes but not through statistical analysis. It is larger than a range caused by halving or doubling the coefficient of domestic beef (the highest single coefficient in the dataset used) in the current diet (−21 … +32% vs. −11 … +21%), for example, which suggests that it is important to consider the whole product group in the calculation of the coefficients for the product groups. Alternatively, if coefficients of individual products are used to represent the product groups, their choice should be made carefully and the uncertainty due to the heterogeneity of the product group should also be presented.
A significant source of uncertainty is also caused by the origin of products and the climate impact coefficients associated with imported products. Poore and Nemecek (2018, suppl. database, regional data) was used as a main data source for imports, but the coefficients there are quite high in general particularly when the whole range (database) is included, as is done for several product groups in this study except for the clear outliers. Better targeting at real importing countries could have increased reliability, but on the other hand, a limit of the availability of climate impact factors is easily met.
The self-sufficient rates used for products may strongly affect the results, especially as coefficients for imports are reasonably high, as they are in general. For the alternative diets, the assumption of self-sufficiency regarding legumes and legume-based diet is crucial. We assumed that half the consumption of legumes would be imported if the diet became much more plant rich. The current self-sufficient level is much higher (75% for peas), but the selection of legumes is currently quite insufficient for direct human consumption, including only peas and broad beans, and not very many processed products. Anticipating change in the quantity, quality, and domesticity of products is challenging, which is also reflected in the uncertainty of climate impact coefficients.
The self-sufficiency of fish has the potential to have a faster impact. In our dataset, the coefficient for domestic fish per kg is lower (3.87 kg CO2 e per kg file) than for imported fish (4.0). In addition, there are underutilized fish stocks in Finland, the use of which would also be advantageous from a climate perspective. We assumed that in an alternative diet that relied heavily on fish (and dairy), i.e., a diet rich in fish and milk, the proportion of domestic fish would be much greater than it is today (50% vs. 80%), following the guidance in the Finnish nutrition recommendations (VRN, 2014). However, without this assumption, the climate impact of that diet would be only about 1% higher, so in practice, the climate impact of the diet will not be affected.
Soil CO2 emissions
SOM-origin CO2 emissions from domestic fields accounted for 19–24% of the climate impacts of the diets. It is a significant proportion and must be considered when deciding on actions to mitigate climate change. However, the assessment made in this study may even underestimate these emissions, as is seen in Figure 6, where it is compared with the National Greenhouse Gas Inventory estimates.
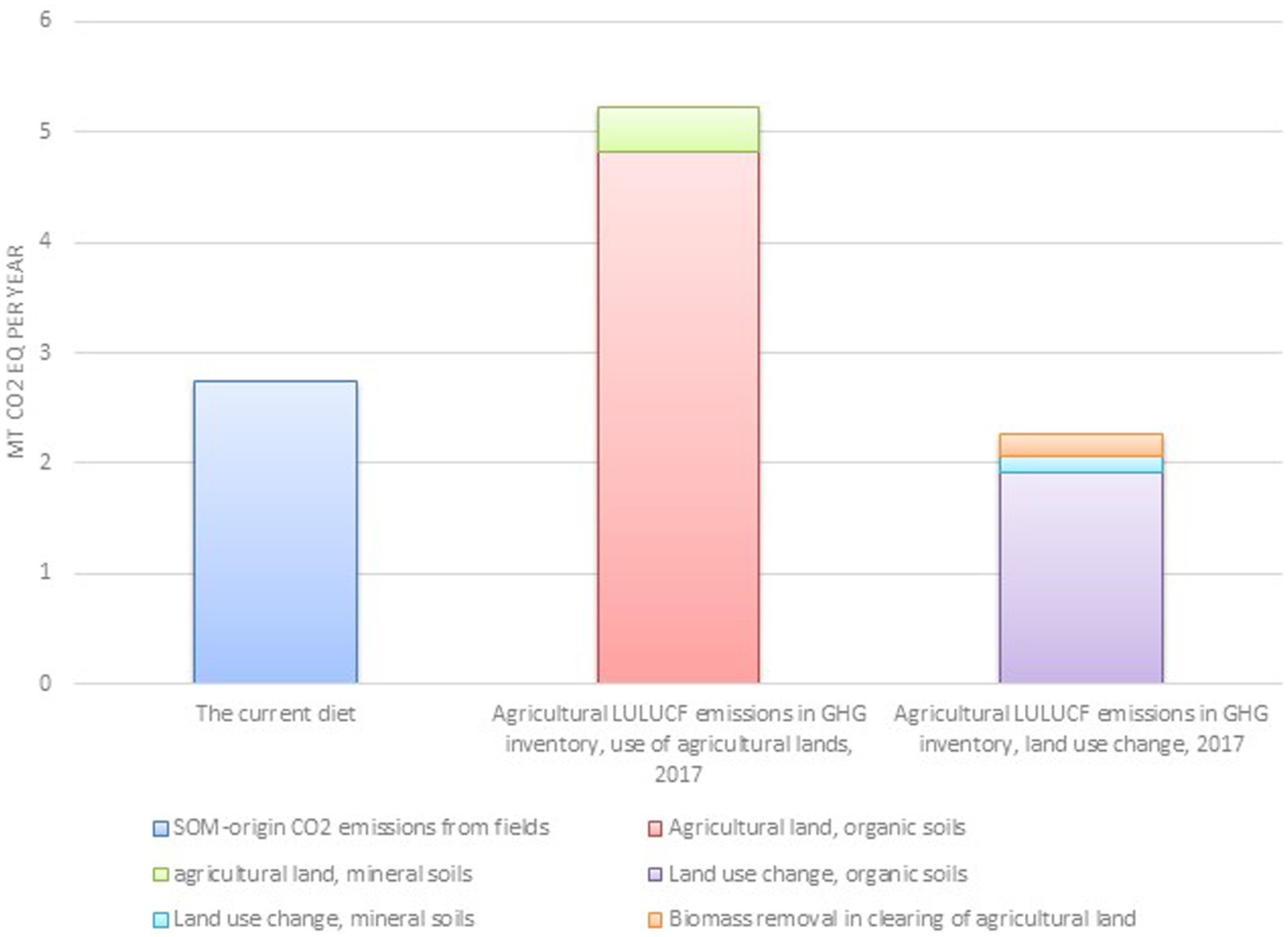
Figure 6. SOM-origin CO2 emissions from domestic fields in the current diet according to the FoodMin model and the National Greenhouse Gas Inventory 2017.
Our approach was intended to capture areas called “cropland remaining cropland” in the National Greenhouse Gas Inventory, which produced foods and feed for domestic consumption and were brought into agricultural use more than 20 years ago. It is unclear why the gap between our estimate and “cropland remaining cropland” is so large (Figure 6). It is partly because of the area needed to produce food for export, but Finland does not export half the production, as Figure 6 suggests. Another explanation for the gap is that the agricultural land in the National Greenhouse Gas Inventory includes some areas outside active farming; there are 200,000 ha more land recorded as agricultural land than in the field register. In our approach, land use for providing food in the diet was instead calculated based on actual food consumption and average yields added by areas recorded as “set aside,” all of which were assumed to be included in crop rotations. Thus, the agricultural land use may be less efficient in practice than in our assumptions.
Emissions from land use change, i.e., “land converted to cropland,” were not included in our assessment, although it has an impact on total emissions in Finland (Figure 6). In addition, SOM-origin CO2 emissions associated with imports were not assessed in the study due to serious data gaps, and because the scope of the study was to support Finland’s national climate policy. However, there are SOM-origin emissions associated with imports, especially if emissions from land use change are included (Sandström et al., 2018). In the case of Finland, these are related especially to broilers, where soy is used to a significant extent as feed, unlike in the production of other meats or milk.
Besides these gaps, there are uncertainties in the assessment of the SOM-origin CO2 emissions due to high spatial variation in SOC and errors related to sampling and analysis (Goidts et al., 2009; Smith et al., 2020). Furthermore, the effects of, e.g., cultivation practices on SOC content are largely site-specific. The estimation of emissions, especially from organic soils, is highly uncertain, as indicated by the wide confidence intervals of the IPCC default emission factors (IPCC, 2014) used in this study.
Due to the uncertainties related to the assessment of CO2 of SOM origin, the share of SOM-origin CO2 emissions in the results can be considered a minimum estimate. Given this, and the fact that mineral soils are potential carbon sinks, SOM-sequestering activities are also very important in reducing the climate impact of the diet. Although we were unable to include the effects of specific measures to increase the soil sink function, they cannot be ignored in dietary assessments in addition to the direct product choices. Different crops and cultivation practices affect SOM in different ways (McDaniel et al., 2014; King and Blesh, 2018; Valkama et al., 2020; Heikkinen et al., 2021), and the effects may not be totally in line with the dietary recommendations, which favor plant-rich or vegan diets based on annual crops and intensive cultivation with typically the greatest loss of SOM. However, a gradual transition to plant-rich diets will eventually release cultivated areas for more cost-effective carbon sequestration activities than those that can be applied on actively cultivated areas, and the contrast with increasing annual cultivation will this not be as severe as might be assumed.
The SOM effects are currently mainly relevant in complementing an assessment of the climate impact in LCA, not a factor that the consumer can use in practice for daily consumption decisions. It may be possible to have labels on “SOM-sequestering” food products in the future, but the recommendations based on health effects and LCA are currently already steering consumption into the right direction. It is clear, that a significant reduction of CO2 emissions from agricultural soils will be expensive, and less easy to achieve from the perspective of farmers than changing from animal to plant protein production, for example. Such a transition cannot therefore only be funded by farmers or be included in food prices but must be supported by the whole of society.
Consumers’ food waste
Research on consumer food waste has been relatively limited, and, e.g., in Europe, harmonized collection has only started (EU, 2019). There is therefore a quite high degree of uncertainty in the food waste coefficients used in this study, especially compared with other studies. However, the fact that there are no comprehensive food waste coefficients for the different product groups introduces even more uncertainty to the result.
This study did not consider food waste in the food service sector, but household-specific food waste coefficients were used for all food consumption. According to the food consumption data used for adults in the study, the vast majority of foods, as much as 80–100%, was consumed at home. On the other hand, public food services are available in Finland for different age groups and different needs. For example, schools offer lunch to all schoolchildren. In addition to the food service provided in connection with social and health services, the use of public food services is also common among people of working age. Food waste generation is much higher in food services than at home. In Finland about 17–20% of food produced in the food service sector was wasted in different subsectors, e.g., schools, day care centres, restaurants, and cafes (Silvennoinen et al., 2015, 2019). This food waste consists, above all, of waste related to serving and waste in the kitchen, while the leftovers produced directly by the consumer in the eating phase are small.
Food waste from food services should be included in further research, although the share of food waste in the climate impact of the diet is still expected to remain low. A more precise targeting of food waste at different foods would also be desirable in further studies.
Conclusion
The study supported the conclusion of various other studies on the need for a radical dietary change to reduce the climate impact of diet in developed countries such as Finland. Consumption of meat should decrease significantly, even by up to a third. At the same time, the consumption of various other foods should increase to ensure adequate nutrient intake. A diet rich in fish that also contained milk and dairy products proved especially beneficial for nutrition and the climate combined.
The study revealed a need to combine the CO2 emissions of SOM decomposition with the climate impact assessment of diets in addition to the consideration of direct product choices. According to the results based on such a dietary assessment, determined actions to improve agricultural practices to sequester and maintain carbon in the soil is equally important in both diets, including animal-sourced foods and vegan diets. With this in mind, the content of the sustainable diet can be more flexible and more in line with the current food culture.
The study also showed that domestic products and SOM-origin CO2 emissions, determine the climate impact of the Finnish diet, both in the current diet and its alternatives. With the transition to a vegan diet, the share of imported products in the total climate impact will increase, but the amount will remain largely unchanged. The outsourcing of emissions would therefore not increase.
The diet evaluation model created in the study, the FoodMin model, was found to be quite reliable in terms of assessing the intake of nutrients, at least by adults, and the climate impact of the Finnish diet. It also succeeded in supplementing the dietary assessment with the SOM-origin CO2 emissions associated with domestically produced products, which are not currently included in the life cycle values of foods.
However, in further research, it is important to decrease the uncertainty of dietary modeling in various ways. The integration of advances in agro-environmental research into dietary modeling is a key development path, including the issue of soil carbon stock and the extension to other environmental impacts, among others. Further research into the environmental impact of new innovative products used as alternatives to meat and dairy products is also needed, because they are largely lacking. Another key development path is to improve data on food consumption in non-adult age groups so that the nutritional effects of dietary changes on sensitive groups can be more reliably assessed.
Data availability statement
The original contributions presented in the study are included in the article/Supplementary material, further inquiries can be directed to the corresponding author/s.
Ethics statement
Ethical review and approval was not required for the study on human participants in accordance with the local legislation and institutional requirements.
Author contributions
MS organized the manuscript, supervised the writing of the manuscript and provided the material on the construction and application of the diet model. JH provided the material on soil carbon modeling. EK provided a statistical analysis of the soil data. HH provided the food waste coefficients. KS contributed to the interpretation of food waste results. LV supervised the interpretation and writing of nutritional results. MS, JH, VK, HH, KS, and KL participated in the writing and editing of the manuscript. All authors contributed to the article and approved the submitted version.
Funding
The study was funded by the Finnish Government Office as part of a joint analysis, assessment, and research activities (VNK/1773/48/2017). The joint analysis, assessment, and research activities coordinated by the Government generate information that supports decision making, working practices, and management by knowledge. The finalizing the manuscript was funded by Academy of Finland (JUSTFOOD project, 327634 and 327370/19.6.2019). The data collection of the FinDiet 2017 Survey was partially funded by the European Food Safety Authority (EFSA), contract OC/EFSA/DATA/2015/03 CT 01 (EU Menu, Lot 2, Finland/Adults).
Acknowledgments
We thank Heli Tapanainen from the Finnish National Institute for Health and Welfare for processing food consumption data of FinDiet 2017 survey into the format required in this study.
Conflict of interest
The authors declare that the research was conducted in the absence of any commercial or financial relationships that could be construed as a potential conflict of interest.
Publisher’s note
All claims expressed in this article are solely those of the authors and do not necessarily represent those of their affiliated organizations, or those of the publisher, the editors and the reviewers. Any product that may be evaluated in this article, or claim that may be made by its manufacturer, is not guaranteed or endorsed by the publisher.
Author disclaimer
The publication is produced by the authors and their organizations and not by EFSA and only represents the views of the authors and not EFSA’s position.
Supplementary material
The Supplementary material for this article can be found online at: https://www.frontiersin.org/articles/10.3389/fsufs.2023.904570/full#supplementary-material
References
Basile-Doelsch, I., Balesdent, J., and Pellerin, S. (2020). Reviews and syntheses: the mechanisms underlying carbon storage in soil. Biogeosciences 17, 5223–5242. doi: 10.5194/bg-17-5223-2020
EU (2019) Commission Delegated Decision (EU) 2019/1597 of 3 May 2019 supplementing Directive 2008/98/EC of the European Parliament and of the Council as regards a common methodology and minimum quality requirements for the uniform measurement of levels of food waste (Text with EEA relevance.) Document 32019D1597. EUR-Lex. Available at: https://eur-lex.europa.eu/eli/dec_del/2019/1597/oj
Gheewala, S. H., Jungbluth, N., Notarnicola, B., Ridoutt, B., and van der Werf, H. (2020). No simple menu for sustainable food production and consumption. Int. J. Life Cycle Assess. 25, 1175–1182. doi: 10.1007/s11367-020-01783-z
Goglio, P., Smith, W. N., Grant, B. B., Desjardins, R. L., McConkey, B. G., Campbell, C. A., et al. (2015). Accounting for soil carbon changes in agricultural life cycle assessment (LCA): a review. J. Clean. Prod. 104, 23–39. doi: 10.1016/j.jclepro.2015.05.040
Goidts, E., Van Wesemael, B., and Crucifix, M. (2009). Magnitude and sources of uncertainties in soil organic carbon (SOC) stock assessments at various scales. Eur. J. Soil Sci. 60, 723–739. doi: 10.1111/j.1365-2389.2009.01157.x
Hallström, E., Bajzelj, B., Håkansson, N., Sjons, J., Åkesson, A., Wolk, A., et al. (2021). Dietary climate impact: contribution of foods and dietary patterns by gender and age in a Swedish population. J. Clean. Prod. 306:127189. doi: 10.1016/j.jclepro.2021.127189
Hallström, E., Carlsson-Kanyama, A., and Börjesson, P. (2015). Environmental impact of dietary change: a systematic review. J. Clean. Prod. 91, 1–11. doi: 10.1016/j.jclepro.2014.12.008
Heikkinen, J., Ketoja, E., Nuutinen, V., and Regina, K. (2013). Declining trend of carbon in Finnish cropland soils in 1974-2009. Glob. Chang. Biol. 19, 1456–1469. doi: 10.1111/gcb.12137
Heikkinen, J., Ketoja, E., Seppänen, L., Luostarinen, S., Fritze, H., Pennanen, T., et al. (2021). Chemical composition controls the decomposition of organic amendments and influences the microbial community structure in agricultural soils. Carbon Manag. 12, 359–376. doi: 10.1080/17583004.2021.1947386
Heller, M. C., Keoleian, G. A., and Willett, W. C. (2013). Toward a life cycle-based, diet-level framework for food environmental impact and nutritional quality assessment: a critical review. Environ. Sci. Technol. 47, 12632–12647. doi: 10.1021/es4025113
Hiraishi, T., Krug, T., Tanabe, K., Srivastava, N., Baasansuren, J., Fukuda, M., et al., (2014). 2013 supplement to the 2006 IPCC guidelines for national greenhouse gas inventories: Wetlands. IPCC, Switzerland.
Hoppu, U., Kujala, J., Lehtisalo, J., Tapanainen, H., and Pietinen, P. (toim.) (2008). Yläkoululaisten ravitsemus ja hyvinvointi. Lähtötilanne lukuvuonna 2007–2008 toteutetun interventiotutkimuksen tulokset. (nutrition and well-being of secondary school children. Baseline results of an intervention survey carried out in the 2007/08 school year) (In Finnish) Kansanterveyslaitoksen julkaisuja B 30/2008. Kansanterveyslaitos, Helsinki.
Huhtanen, P., Jaakkola, S., and Nousiainen, J. (2013). An overview of silage research in Finland: from ensiling innovation to advances in dairy cow feeding. Agric. Food Sci. 22, 35–56. doi: 10.23986/afsci.6632
IPCC (2014) Supplement to the 2006 IPCC guidelines for National Greenhouse gas Inventories: wetlands, T. Hiraishi, T. Krug, K. Tanabe, N. Srivastava, J. Baasansuren, and M. Fukuda, et al., IPCC, Switzerland
IPCC (2019) in Climate change and land: an IPCC special report on climate change, desertification, land degradation, sustainable land management, food security, and greenhouse gas fluxes in terrestrial ecosystems. eds. P. R. Shukla, J. Skea, E. C. Buendia, V. Masson-Delmotte, and H.-O. Pörtner, Roberts, P. Zhai, R. Slade, S. Connors, R. van Diemen, M. Ferrat, E. Haughey, S. Luz, S. Neogi, M. Pathak, J. Petzold, J. Portugal Pereira, P. Vyas, E. Huntley, K. Kissick, M. Belkacemi, and J. Malley.
ISO-EN (2006a). 14040:2006 Environmental management. Life Cycle Assessment. Principles and framework. 20 p. ISO/TC 207/SC 5.
ISO-EN (2006b). 14044:2006 Life Cycle Assessment. Requirements and guidelines. 46 p. ISO/TC 207/SC 5.
Jarmul, S., Dangour, A., Green, R., Liew, Z., Haines, A., and Scheelbeek, P. (2020). Climate change mitigation through dietary change: a systematic review of empirical and modelling studies on the environmental footprints and health effects of 'sustainable diets'. Environ. Res. Lett. 15:123014. doi: 10.1088/1748-9326/abc2f7
Jones, A. D., Hoey, L., Blesh, J., Miller, L., Green, A., and Shapiro, L. F. (2016). A systematic review of the measurement of sustainable diets. Adv. Nutr. 7, 641–664. doi: 10.3945/an.115.011015
Kaartinen, N., Tapanainen, H., Reinivuo, H., Pakkala, H., Aalto, S., Raulio, S., et al. (2020). The Finnish National Dietary Survey in adults and elderly (FinDiet 2017). EFSA Sup. Pub. 17:1914E. doi: 10.2903/sp.efsa.2020.EN-1914
King, A. E., and Blesh, J. (2018). Crop rotations for increased soil carbon: perenniality as a guiding principle. Ecol. Appl. 28, 249–261. doi: 10.1002/eap.1648
Knudsen, M. T., Dorca-Preda, T., Djomo, S. M., Peña, N., Padel, S., Smith, L. G., et al. (2019). The importance of including soil carbon changes, Ecotoxicity and biodiversity impacts in environmental life cycle assessments of organic and conventional Milk in Western Europe. J. Clean. Prod. 215, 433–443. doi: 10.1016/j.jclepro.2018.12.273
Kyttälä, P., Ovaskainen, M., Kronberg-Kippilä, C., Erkkola, M., Tapanainen, H., Tuokkola, J., et al., (2008). Lapsen ruokavalio ennen kouluikää. (A child's diet before school age) (In Finnish) Kansanterveyslaitoksen julkaisuja B 32/2008. Kansanterveyslaitos, Helsinki.
Lilja, H., and Nevalainen, R. (2006). Chapter 5 developing a digital soil map for Finland. Dev. Soil Sci. 31, 67–603. doi: 10.1016/S0166-2481(06)31005-7
Luke (2018). Balance sheet for food commodities 2017, preliminary and 2016 final figures. Available at: https://stat.luke.fi/en/balance-sheet-food-commodities-2017-preliminary-and-2016-final-figures_en.
Luke (2020). Balance sheet for food commodities 2020, preliminary and 2019 final figures. Available at: https://stat.luke.fi/en/balance-sheet-food-commodities-2020-preliminary-and-2019-final-figures_en
McAuliffe, G. A., Takahashi, T., and Lee, M. R. F. (2020). Applications of nutritional functional units in commodity-level life cycle assessment (LCA) of Agri-food systems. Int. J. Life Cycle Assess. 25, 208–221. doi: 10.1007/s11367-019-01679-7
McDaniel, M. D., Tiemann, L. K., and Grandy, A. S. (2014). Does agricultural crop diversity enhance soil microbial biomass and organic matter dynamics? A meta-analysis. Ecol. Appl. 24, 560–570. doi: 10.1890/13-0616.1
McLaren, S., Berardy, A., Henderson, A., Holden, N., Huppertz, T., Jolliet, O., et al. (2021). Integration of environment and nutrition in life cycle assessment of food items: opportunities and challenges. Rome, FAO.
Montonen, J., Männistö, S., Sarkkola, C., Järvinen, R., Hakala, P., Sääksjärvi, K., et al. (2008). Ravinnonsaannin väestöryhmittäiset erot. Terveys 2000 -tutkimus. Differences in food intake by population group. Publications of the National Public Health Institute B 38/2008. National Public Health Institute, Finland. Helsinki.
Nelson, M. E., Hamm, M. W., Hu, F. B., Abrams, S. A., and Griffin, T. S. (2016). Alignment of healthy dietary patterns and environmental sustainability: a systematic review. Adv. Nutr. 7, 1005–1025. doi: 10.3945/an.116.012567
OECD (2020). Meat consumption. OECD Data. Available at: https://data.oecd.org/agroutput/meat-consumption.htm#indicator-chart
Payne, C., Scarborough, P., and Cobiac, L. (2016). Do low-carbon-emission diets lead to higher nutritional quality and positive health outcomes? A systematic review of the literature. Public Health Nutr. 19, 2654–2661. doi: 10.1017/S1368980016000495
Perignon, M., Masset, G., Ferrari, G., Barré, T., Vieux, F., Maillot, M., et al. (2016). How low can dietary greenhouse gas emissions be reduced without impairing nutritional adequacy, affordability and acceptability of the diet? A modelling study to guide sustainable food choices. Public Health Nutr. 19, 2662–2674. doi: 10.1017/S1368980016000653
Poore, J., and Nemecek, T. (2018). Reducing Food’s environmental impacts through producers and consumers. Science 360, 987–992. doi: 10.1126/science.aaq0216
Pratt, C., and Tate, K. (2018). Mitigating methane: emerging technologies to combat climate Change’s second leading contributor. Environ. Sci. Technol. 52, 6084–6097. doi: 10.1021/acs.est.7b04711
Risku-Norja, H., Kurppa, S., and Helenius, J. (2009). Dietary choices and greenhouse gas emissions – assessment of impact of vegetarian and organic options at national scale. Progress in industrial ecology – an. Int. J. 6, 4, 340–354. doi: 10.1504/PIE.2009.032323
Saarinen, M. (2018). Including nutrition in the life cycle assessment of food products. University of Helsinki. Department of Agricultural Sciences. Publications 47: 100 p. Diss.: Helsingin yliopisto, 2018.
Saarinen, M., Fogelholm, M., Tahvonen, R., and Kurppa, S. (2017). Taking nutrition into account within the life cycle assessment of food products. J. Clean. Prod. 149, 828–844. doi: 10.1016/j.jclepro.2017.02.062
Sandström, V., Valin, H., Krisztin, T., Havlík, P., Herrero, M., and Kastner, T. (2018). The role of trade in the greenhouse gas footprints of EU diets. Glob. Food Sec. 19, 48–55. doi: 10.1016/j.gfs.2018.08.007
Scherhaufer, S., Moates, G., Hartikainen, H., Waldron, K., and Obersteiner, G. (2018). Environmental impacts of food waste in Europe. Waste Manag. 77, 98–113. doi: 10.1016/j.wasman.2018.04.038
Seppälä, J., Mäenpää, I., Koskela, S., Mattila, T., Nissinen, A., Katajajuuri, J.-M., et al. (2011). An assessment of greenhouse gas emissions and material flows caused by the Finnish economy using the ENVIMAT model. J. Clean. Prod. 19, 1833–1841. doi: 10.1016/j.jclepro.2011.04.021
Silvennoinen, K., Heikkilä, L., Katajajuuri, J.-M., and Reinikainen, A. (2015). Food waste volume and origin: case studies in the Finnish food service sector. Waste Manag. 46, 140–145. doi: 10.1016/j.wasman.2015.09.010
Silvennoinen, K., Katajajuuri, J.-M., Hartikainen, H., Heikkilä, L., and Reinikainen, A. (2014). Food waste volume and composition in Finnish households. Br. Food J. 116, 1058–1068. doi: 10.1108/BFJ-12-2012-0311
Silvennoinen, K., Nisonen, S., and Pietiläinen, O. (2019). Food waste case study and monitoring developing in Finnish food services. Waste Manag. 97, 97–104. doi: 10.1016/j.wasman.2019.07.028
Smith, P., Soussana, J. F., Angers, D., Schipper, L., Chenu, C., Rasse, D. P., et al. (2020). How to measure, report and verify soil carbon change to realize the potential of soil carbon sequestration for atmospheric greenhouse gas removal. Glob. Chang. Biol. 26, 219–241. doi: 10.1111/gcb.14815
Springmann, M., Spajic, L., Clark, M. A., Poore, J., Herforth, A., Webb, P., et al. (2020). The healthiness and sustainability of national and global food based dietary guidelines: modelling study. BMJ 370:m2322. doi: 10.1136/bmj.m2322
Springmann, M., Wiebe, K., Mason-D'Croz, D., Sulser, T. B., Rayner, M., and Scarborough, P. (2018). Health and nutritional aspects of sustainable diet strategies and their association with environmental impacts: a global modelling analysis with country-level detail. Lancet Planet Health 2, e451–e461. doi: 10.1016/S2542-5196(18)30206-7
Statistics Finland (2017). Väestörakenne (Demographic structure). Available at: http://tilastokeskus.fi/til/vaerak/index.html
Statistics Finland (2018). Greenhouse gas emissions in Finland 1990 to 2016. National Inventory Report under the UNFCCC and the Kyoto Protocol. Statistics Finland.
Statistics Finland (2021) Greenhouse gas emissions in Finland 1990-2019. National Inventory Report under the UNFCCC and the Kyoto protocol. 580 pp. Statistical Finland. Available at: https://unfccc.int/documents/271571
Summary report of EAT-Lancet Commission. (2020). Healthy diets from sustainable food systems. Food, planet, health. Available at: https://eatforum.org/eat-lancet-commission/eat-lancet-commission-summary-report/ (Accessed January 3, 2022).
Sun, Z., Scherer, L., Tukker, A., Spawn-Lee, S. A., Bruckner, M., Gibbs, H. K., et al. (2022). Dietary change in high-income nations alone can lead to substantial double climate dividend. Nat. Food 3, 29–37. doi: 10.1038/s43016-021-00431-5
THL (2019). Eating together - food recommendations for families with children. Second updated edition 2019. 128 p. Helsinki. ISBN: 978-952-343-264-2 (online version).
Tuomi, M., Thum, T., Järvinen, H., Fronzek, S., Berg, B., Harmon, M., et al. (2009). Leaf litter decomposition—estimates of global variability based on Yasso07 model. Ecol. Model. 220, 3362–3371. doi: 10.1016/j.ecolmodel.2009.05.016
Valkama, E., Kunypiyaeva, G., Zhapayev, R., et al. (2020). Can conservation agriculture increase soil carbon sequestration? A modelling approach. Geoderma 369:114298. doi: 10.1016/j.geoderma.2020.114298
Valsta, L., Kaartinen, N., Tapanainen, H., Männistö, S., and Sääksjärvi, K. (2018). Ravitsemus Suomessa - FinRavinto 2017 -tutkimus. Nutirtion in Finland – FinDiet 2017 Survey (in Finnish) Raportti 12/2018. Terveyden ja hyvinvoinnin laitos, Helsinki.
Vieux, F., Perinon, M., Gazan, R., and Darmon, N. (2018). Dietary changes needed to improve diet sustainability: are they similar across Europe? Eur. J. Clin. Nutr. 72, 951–960. doi: 10.1038/s41430-017-0080-z
Virtanen, Y., Kurppa, S., Saarinen, M., Katajajuuri, J. M., Usva, K., Mäenpää, I., et al. (2011). Carbon footprint of food–approaches from national input–output statistics and a LCA of a food portion. J. Clean. Prod. 19, 1849–1856. doi: 10.1016/j.jclepro.2011.07.001
VRN (2014). Terveyttä ruoasta - Suomalaiset ravitsemussuositukset 2014. (health from food – the Finnish nutrition recommendations) (In Finnish) Valtion ravitsemusneuvottelukunta, Helsinki.
VRN (2017). Eating and learning together - – recommendations for school meals. National nutrition council, Finland. ISBN: 978-952-302-844-9 (online).
Keywords: sustainable diet, food consumption, nutrition, food transition, carbon storage, carbon stock, food waste, climate policy
Citation: Saarinen M, Heikkinen J, Ketoja E, Kyttä V, Hartikainen H, Silvennoinen K, Valsta L and Lång K (2023) Soil carbon plays a role in the climate impact of diet and its mitigation: the Finnish case. Front. Sustain. Food Syst. 7:904570. doi: 10.3389/fsufs.2023.904570
Edited by:
Uma Tiwari, Technological University Dublin, IrelandReviewed by:
Sumita Ghosh, University of Technology Sydney, AustraliaRachel Mazac, Stockholm University, Sweden
Copyright © 2023 Saarinen, Heikkinen, Ketoja, Kyttä, Hartikainen, Silvennoinen, Valsta and Lång. This is an open-access article distributed under the terms of the Creative Commons Attribution License (CC BY). The use, distribution or reproduction in other forums is permitted, provided the original author(s) and the copyright owner(s) are credited and that the original publication in this journal is cited, in accordance with accepted academic practice. No use, distribution or reproduction is permitted which does not comply with these terms.
*Correspondence: Merja Saarinen, bWVyamEuc2FhcmluZW5AbHVrZS5maQ==