- 1School of Medicine, Foshan University, Foshan, Guangdong, China
- 2Beijing Innovation Center of Food Nutrition and Human Health, Laboratory of Molecular Sensory Science, Beijing Technology and Business University, Beijing, China
- 3Vanced Materials Technology (Zhongshan) Co., Ltd., Guangdong, China
- 4Department of Food Science and Technology, Faculty of Agriculture and Food Science, Ibb University, Ibb, Yemen
Bone protein is a significant secondary product of the meat industry, comprising a substantial quantity of protein. These proteins could be broken down through enzymatic hydrolysis to generate antioxidant peptides. This study aimed to produce antioxidant peptides from bovine bone extract by enzymatic hydrolysis utilizing Flavourzyme and Protamex by optimizing enzyme amounts and time using the Box–Behnken design. The final optimized conditions obtained through the model were as follows: The amount of Flavourzyme was 1,100 U, the amount of Protamex was 2,814 U, and the time was 3.77 (h). Bovine bone extract hydrolysate (BBEH) was purified stepwise using ultrafiltration membranes with molecular cutoffs of 5, 3, and 1 kDa. To assess the antioxidant capacity of the fractions, several methods were used, including radical scavenging activity “1,1-diphenyl-2-picrylhydrazyl (DPPH),” “2,2′-azino-bis (3-ethylbenzothiazoline-6-sulfonic acid) (ABTS),” metal chelating activity (MCA), reducing power (RP), and thiobarbituric acid assay (TBA). The results indicated that the ultrafiltration fraction with a molecular weight of less than 1 kDa showed significant antioxidant activity, with 48, 42, and 50% inhibition rates for DPPH, ABTS, and metal chelating, respectively. Using size exclusion chromatography, the fraction with a molecular weight less than 1 kDa was further separated into five sub-fractions: Frac-I, Frac-II, Frac-III, Frac-IV, and Frac-V. Sub-Frac-III, which exhibits significant DPPH radical scavenging activity (55%) and a reducing power of 0.8 at 700 nm, was separated into six sub-sub-fractions using reversed-phase HPLC (RP-HPLC) based on molecular weight and hydrophobicity. The sub-sub-fraction with the highest value for DPPH radical scavenging activity was sub-Fra-III-6, which exhibited approximately 69.45% activity. The sub-Fra-III-6 was analyzed using LC–MS/MS, which identified two specific peptides: Ala-Pro-Phe with a mass of 333.12 Da and Asp-His-Val with a mass of 369.14 Da. These two peptides are likely the primary peptides that might have a crucial role in antioxidant capacity. It can be concluded that BBEH is a valuable source of natural antioxidants and has the potential to serve as a viable resource in the cultured meat industry.
Introduction
The production of bovine bone is increasing due to the development of the cattle industry in China. China produces approximately 12 million tons of bovine bone every year, according to the National Bureau of Statistics in 2019. The bovine bone is typically used as animal feed or discarded, resulting in significant environmental pollution and the wastage of valuable resources.
Bovine bone collagen comprises most of the essential mineral components the human body needs. Additionally, it contains lipids, protein, water, and ash (Yao et al., 2020). Collagen peptide, primarily found in bovine bone, is a precious nutritional resource. Bone peptides, which are composed of amino acids, possess a range of bioactive properties. These include the prevention of atherosclerosis (Igase et al., 2018), the ability to delay skin aging (Müller et al., 2018), the promotion of osteoblast growth (Elango et al., 2019), and the protection against the harmful effects of free radicals. Additionally, they exhibit immuno-modulatory, antitumor, anti-diabetic, and antimicrobial functions (Rozi et al., 2018). Yang et al. (2019) extracted five antioxidant peptides from tuna bone collagen; of these, two peptides have potential applications in the food industry.
The beef bone extract has a weak flavor and high viscosity, so the use of this extract as a food ingredient is narrow, which limits its usage to comparatively low-value ingredients in sports nutrition or pet foods that are soup- or sauce-based. Currently, the use of enzymes to convert these low-value food materials into valuable functional food is in high demand. During hydrolysis, amino acids and short-chain peptides are released by cleavage of the long-chain protein, resulting in a reduction in the viscosity (Chiang et al., 2019). Short-chain peptides can accelerate protein synthesis, promote the use and absorption of minerals, boost immunological function, and reduce the suppression of free radical generation (Yamamoto et al., 2016). Enzymatic hydrolysis can be used to synthesize peptides that have a high level of purity and are chemically stable.
Several types of protein hydrolysate can be produced by treating protein content with different proteases. This was in line with the previous research that showed that the specificity of protease significantly alters the peptide composition of hydrolysates, and this specificity is closely related to the functional activity of that peptide. According to Wang et al. (2022), protein hydrolysate of skipjack tuna roes produced by using Flavourzyme exhibits the highest DPPH scavenging activity among other enzymes such as pepsin, trypsin, papain, and thermolysin.
The hydrolysis efficiency can be increased by using the double-enzyme method compared to the single-enzyme method due to the different enzyme cleavage sites at every step. The results of the (Zheng et al., 2023) study showed that the DH of hard-shelled mussels was significantly triggered by the type of enzyme. In this study, a combination of endo protease (trypsin) and exo protease (papain) was used to obtain the required level of DH.
Various chronic diseases arise in the human body as a result of an excessive accumulation of free radicals. Oxidative damage arises inside the body as a result of an excess of free radicals. If this damage is not rapidly healed and remains for a long time, it can lead to several diseases, including inflammatory disease, melanoma, and heart disease (Wang et al., 2022).
In addition, cell damage is induced by various toxic peroxy radicals, which are strongly related to chronic human diseases and aging. Antioxidants may reduce oxidation activities induced by free radicals. Various studies reveal that synthetic antioxidants can have an unpredictable level of toxicity in humans and affect the lungs, liver, and spleen (El-Shourbagyand Elzahar, 2014). Increasing attention is diverted toward natural antioxidants due to the limited use of these synthetic chemical antioxidants. One of the antioxidants from natural sources is mainly peptides. Current research focuses on these antioxidant peptides because of their prospective health benefits related to low cost, safety, functional properties, low molecular weight, and high activity. Antioxidant peptides draw great attention due to their potential application in treating diseases, such as immunopathy, neurodegenerative disorders, Alzheimer’s diseases, inflammation, Parkinson’s, and diabetes mellitus. Several studies used peptides as antioxidants. For example, Sierra et al. (2021) found that the peptide Pro-Gly-Tyr (PGY) can scavenge reactive oxygen species (ROS) by enhancing the production of catalase and superoxide dismutase I in HepG2 cells stimulated by 2,2″ azobis (APPH). The GGFDMG peptide can decrease ROS-induced damage by enhancing the amounts of intracellular antioxidative enzymes, which help eliminate ROS.
Another study reveals that AAVPSGASTGIYEALELR can activate the DAF − 16 signaling pathway to mimic the oxidative stress in the cells (Wang et al., 2021).
Short-chain peptides with molecular weight (<1 kDa) are usually bioactive. These peptides usually contain a large percentage of non-polar amino acids (Zhao et al., 2020). According to Wu et al. (2023) bioactive peptides usually contain 3–30 amino acids with molecular weights 500 to 1850 Da and are generated from various protein sources by microbial fermentation, chemical degradation, and enzymatic hydrolysis. Peptide fractionated from monkfish muscle having molecular weight < 1 kDa can increase the antioxidant ability of the liver to prevent non-alcoholic fatty liver disease (NAFLD) progression by the modulation of the AMPK/Nrf2 pathway and intestinal flora (Ren et al., 2022).
As far as we know, limited research has investigated the antioxidant activities of protein hydrolysates and peptides derived from bovine bone extract. This study aimed to optimize the enzyme amounts and time for producing bovine bone extract hydrolysate (BBEH) with antioxidant activity and purify antioxidant peptides from this hydrolysate through a series of purification techniques. Based on the best antioxidant potential activity, these peptides can be a useful source for the cultured meat production industry.
Materials and methods
Sample and reagents
Bovine bone extract (BBE) was provided by Dufengxuan Gusheng Co., Ltd. (Fushun, Liaoning, China). Potassium ferricyanide, TCA (trichloroacetic acid), 2,2-azino-bis(3-ethylbenzthiazoline)-6- sulfonic acid (ABTS), butylated hydroxyanisole (BHA), 2,2-diphenyl-1-picrylhydrazyl (DPPH), ferric chloride, and potassium were purchased from Alfa Aesar (Shanghai, China). Enzymes, including trypsin, Papain, Protamex, and Flavourzyme (500 MG), were purchased from Novozymes (Bagsvaerd, Denmark). Synthetic peptides (95% purity), including Ala-Pro-Phe and Asp-His-Val, were supplied by Scilight-Peptide (Beijing, China). All the chemicals and reagents used were analytical grade. The hydrolysate was produced by protease, including Flavourzyme and Protamex (500 MG) (Novozymes Bagsvaerd, Denmark), trypsin, and papain. The activity of the enzyme was calculated by following the method proposed by Kembhavi et al. (1993). One unit of enzyme activity can be defined as the amount of enzyme used to release 1 gram of tyrosine per minute under optimal conditions.
Experimental design
Based on the results of the single-factor experiment (data not shown), the Box–Behnken design (BBD) was selected for the optimization of enzyme amount and time, and two responses including the degree of hydrolysis and total score were selected for the evaluation of the results and the selection of the best condition. The score was calculated as a total score = 0.5*sensory score + 0.2* umami amino acids +0.2*nucleotide+0.1* < 1 kDa peptide. The independent variables were hydrolysis time (A, h), Flavourzyme (B, U), and Protamex (C, U). The coded and un-coded levels for these variables are listed in Table 1. The response in the form of the total score and degree of hydrolysis (DH) of the experimental design is given in Table 2. A second-order equation was used to explain the relationship between independent and dependent variables.
The linear regression of optimal response (Y) was calculated by a second-degree polynomial equation (Eq. 1) using the least squares method (Yang et al., 2012). Here, Y is the model calculated response; the variables are time (A), Flavourzyme (B), and Protamex (C); the coefficients of linear regression are a1, a2, and a3; the quadratics are a22 and a33; and the effects of cross product factors on response are a12, a13, and a23. Analysis of variance (ANOVA) was applied to figure out the regression coefficient of the response surface equation and to confirm the RSM. Three important parameters were considered in order to assess the statistical significance: (a) coefficient of variation (CV), (b) F-test, (c) coefficient of determination (R2), and based on the criterion of desirability, optimal processing conditions were generated by numerical and graphical analyses.
Preparation of enzyme hydrolysates of bovine bone extract (BBE)
To hydrolyze bovine bone extract, four different types of enzymes were used (Flavourzyme™, Protamex™, trypsin, papain, and the enzyme combination) and previously used conditions for 4 h. Table 3 demonstrates the optimal conditions for various enzymes. For the adjustment of pH value, after every half hour, NaOH (sodium hydroxide) solution (1 N) or HCL (hydrochloric acid) solution (1 N) was added to the hydrolyzed mixture. The solution was boiled for 10–15 min after hydrolysis to deactivate the enzymes, and the obtained peptide mixture was then centrifuged at 8000 rpm for 15 min. The hydrolyzed samples were kept at 25°C for analysis.
Calculation of degree of hydrolysis (DH)
The DH was calculated using the previous method (Begum et al., 2021). DH is calculated as the ratio of the total nitrogen to the amino acids nitrogen in percentage (%). It is actually the cleavage of peptide bonds by the enzyme. The content of nitrogen in amino acid (AN) was quantified by the formaldehyde titration method, while Kjeldahl using a FOSS KJELTEC 8400 (Foss Co. Ltd., Denmark) was used to calculate the total nitrogen (TN) content.
Antioxidant activity determination of bovine bone extract hydrolysate
DPPH radical scavenging assay
With some modifications, the procedure previously described by Wu et al. (2003) was used to calculate the DPPH activity of the sample. A 1.5 mL of 0.2 mm DPPH in 95% ethanol solution was added to 1.5 mL of sample solution. The solution was then stored at room temperature in darkness for 30 min. Shimadzu UV-2401/2501 spectrophotometer (Molecular Devices, Japan) was used to measure the activity at 517 nm. A decrease in absorption at 517 nm indicates high DPPH free radical scavenging activity. For the control solution, distilled water was used and prepared using the same process.
The equation used to calculate the activity was as follows:
ABTS radical scavenging assay
The ABTS radical scavenging activity of the hydrolysates was calculated using the method described in Re et al. (1999) with minor modifications. A stock solution was prepared by mixing ABTS (7 mM) and potassium persulphate (2.45 mM). This prepared mixture was kept for 16 h at room temperature in the dark. The stock solution absorbance was adjusted to 0.70 ± 0.02 at 734 nm by diluting with distilled water. The diluted ABTS solution (1 mL) was mixed with the sample solution (1 mL) for the analysis and kept for 10 min at room temperature. The absorbance of the sample was measured at 734 nm. For the control solution, instead of a sample, distilled water was used. The following equation was used to calculate the activity:
Metal chelating activity
The efficiency of BBE hydrolysate to chelate Fe+2 (ferrous ions) was calculated as described by the procedure (Decker and Welch, 1990). A total volume of 1.5 mL from each sample of the solution was mixed with a solution containing 0.2 mL of 5 mM ferrozine and 0.1 mL of 2 mM FeCl2. The absorbance was measured at 562 nm after 20 min of incubation of the reaction mixture. A 1 mL of distilled water, instead of the sample, was used as a control solution. The sample exhibited chelating activity was quantified according to the following equation:
Reducing power
A method described by Liu et al. (2010) with minor amendments was used to measure the reducing activity of the hydrolysate. Briefly, 1 mL of the sample was mixed with 1 mL of 0.2 M phosphate buffer (pH 6.6) and 1 mL of a 1% potassium ferricyanide solution. The mixture was incubated for 20 min at 50°C. After incubation, 1 mL of 10% TCA was added to the reaction mixture. A 0.1% ferric chloride solution, 1 mL of sample solution, and 1 mL of distilled water were mixed. The sample was kept at room temperature for 10 min, and 700 nm UV absorbance was used for detection. A high UV value in the reaction mixture showed high activity.
Thiobarbituric acid (TBA) assay
The TBA value was calculated using the method defined by Buege et al. (1978). A 0.5 g sample was homogenized in distilled water and mixed with 2 mL of TBA reagent containing 15% TCA, 0.375% TBA solution, and 0.25 N of hydrochloric acid (HCl). The solution was kept in hot water for 15 min. Upon heating, a pink color formed. The absorption of the solution was measured at a wavelength of 535 nm.
The following formula was used to calculate the TBA value.
The TBA value is used to define the extent of oxidation and is stated as milligrams of malonaldehyde (MA) equivalents per kilogram sample.
Purification of peptides from bovine bone extract hydrolysate
Ultrafiltration
The enzymatic hydrolysate of BBE was fractionated by ultrafiltration using the MWCO membranes of 1,000 Da, 3,000 Da, and 5,000 Da. The BBEH was primarily filtered using a 5,000-Da membrane to obtain fractions (F-I, MW <5,000 Da), and then the 5,000 Da permeate was further filtered using a 3,000-Da membrane into a fraction of retentate (F-II, <3,000 Da). Thereafter, the 3,000-Da permeate was fractionated into a 1,000-Da retentate (F-III, <1,000 Da) permeate. The antioxidant activity of the fraction was calculated using DPPH, ABTS, and metal chelating assays. Those fractions that have higher activity were collected, freeze-dried, and stored at −20°C for further analysis.
Gel filtration chromatography (GFC)
The freeze-dried fraction was re-dissolved in distilled water and further purified using a gel filtration column, Superdex Peptide 10/300GL (10 mm × 300 mm); 2 mL of sample was filtered through a 0.45-μm filter membrane; the column temperature was 25°C with a flow rate of 0.6 mL min−1; and ultra-pure water was used as eluent. The fractions were monitored using a UV detector at 245 nm using a gel filtration chromatography device (AKTA Avant by Amersham Pharmacia Biotech Ltd., Uppsala, Sweden). The antioxidant activity of the fractions was dogged using reducing power and DPPH radical scavenging assays.
Reversed-phase high-performance liquid chromatography (RP-HPLC)
Fraction purified from the gel chromatography process having the highest function was re-dissolved in ultrapure water, and a 0.22-μm filtered membrane was used to filter the solution, which was then loaded onto the Eclipse XDB-C18 column (21.2 × 250 mm, and 10 μm) (Agilent Technologies, CA, USA) with a flow rate of 20 mL min−1. Methanol and ultrapure water were used as a mobile phase by adjusting the pH to 4 using 0.1% formic acid. The fractions were detected using a UV detector at 215 nm. All the peaks were collected separately, and a vacuum rotary evaporator (R-3 HB, BUCHI Labortechnik, Switzerland) was then used to remove water and methanol from the sample. The concentrated samples were used for further analysis.
LC–MS/MS for the identification of antioxidant peptides
The fraction having the highest radical scavenging activity after RP-HPLC purification was dissolved in ultra-pure water to obtain a concentration of 20 mg/mL, and 30 mL of the sample with a flow rate of 0.2 mL/min was loaded onto an Agilent 6,410 LC–MS/MS system to identify the sequence of the peptide. Before mass spectrometry identification, the samples must be separated using the ZORBAX SB-C18 column (4.6 × 250 mm, 5 μm). The scanning mode of mass spectrometry was from 50 m/z to 1,000 m/z. Other conditions were as follows: an ion source, positive ionization mode; temperature, 350°C; fragmentation voltage, 135 V; and capillary voltage, 4,000 V. In the multi-reaction monitoring (MRM) mode, the peptide’s mother ion [M + H]+ is bombarded with 10/15 eV collision energy to form fragment ions. For the analysis, mass spectrophotometry and MS/MS ion fragment B.07.00 software were used in the Agilent Mass Hunter qualitative analysis (Liu et al., 2010).
Statistical analysis
The statistical analysis was performed using SPSS-17 (IBM, NY, USA) and Origin 9.1 software (OriginLab Corporation, Massachusetts, USA). A one-way ANOVA was used to analyze the statistical analysis significance, and in case of significant differences (p < 0.05) among the treatments, Duncan’s multi-range test was carried out. The data were presented as means ± SD (standard deviation).
Results and discussion
Model fitting
The results of the three different variables [time (A), Flavourzyme (B), and Protamex (C)] for the total score and DH are presented in Table 3. The DH of the sample ranged from 6.5 to 14.5. The regression analysis showed that a second-order polynomial equation could be used to illustrate the total score and DH.
DH = +8.70 + 0.66*A + 3.12*B + 0.59*C + 0.26*A*B – 1.48*A*C + 0.20*B*C + 1.29*A2 + 0.26*B2 + 0.38*C2
Total score = 8.74 + 0.53*A + 0.60*B + 0.42*C + 0.30*A*B + 0.20*A*C – 0.050*B*C
−0.55*A2-0.75*B2 – 0.34*C2
where Y represents a1A (time), a2B (Flavourzyme), and a3C (Protamex), which are coded variables. The results of the regression coefficient and ANOVA are presented in Supplementary Table (data not shown). The model coefficient of determination (R2) was 0.96 and 0.98 for response 1 and response 2, respectively. It is suggested that the fitted model could explain 96 and 98% of the total variation. Values demonstrate a high degree of correlation between the predicted values and the observed experimental data.
In addition, the three-dimensional response surface, representing the relationship between the DH and total score and the parameters, was analyzed using RSM (Eq. 1).
The response surface plots of the three factors A, B, and C to the DH and the total score plotted according to the regression equation are shown in Figures 1A–C, 2A–C. It is shown in Figure 1A that the Protamex enzyme does not affect the DH while adding Flavourzyme significantly increases the DH. Figure 1B indicates that the increase in time for the hydrolysis causes an increment in the DH. In Figure 1C, the addition of Protamex significantly increases the DH. In Figures 2A–C, it can be seen that the total score significantly increases with an increase in the addition of enzymes and time duration.
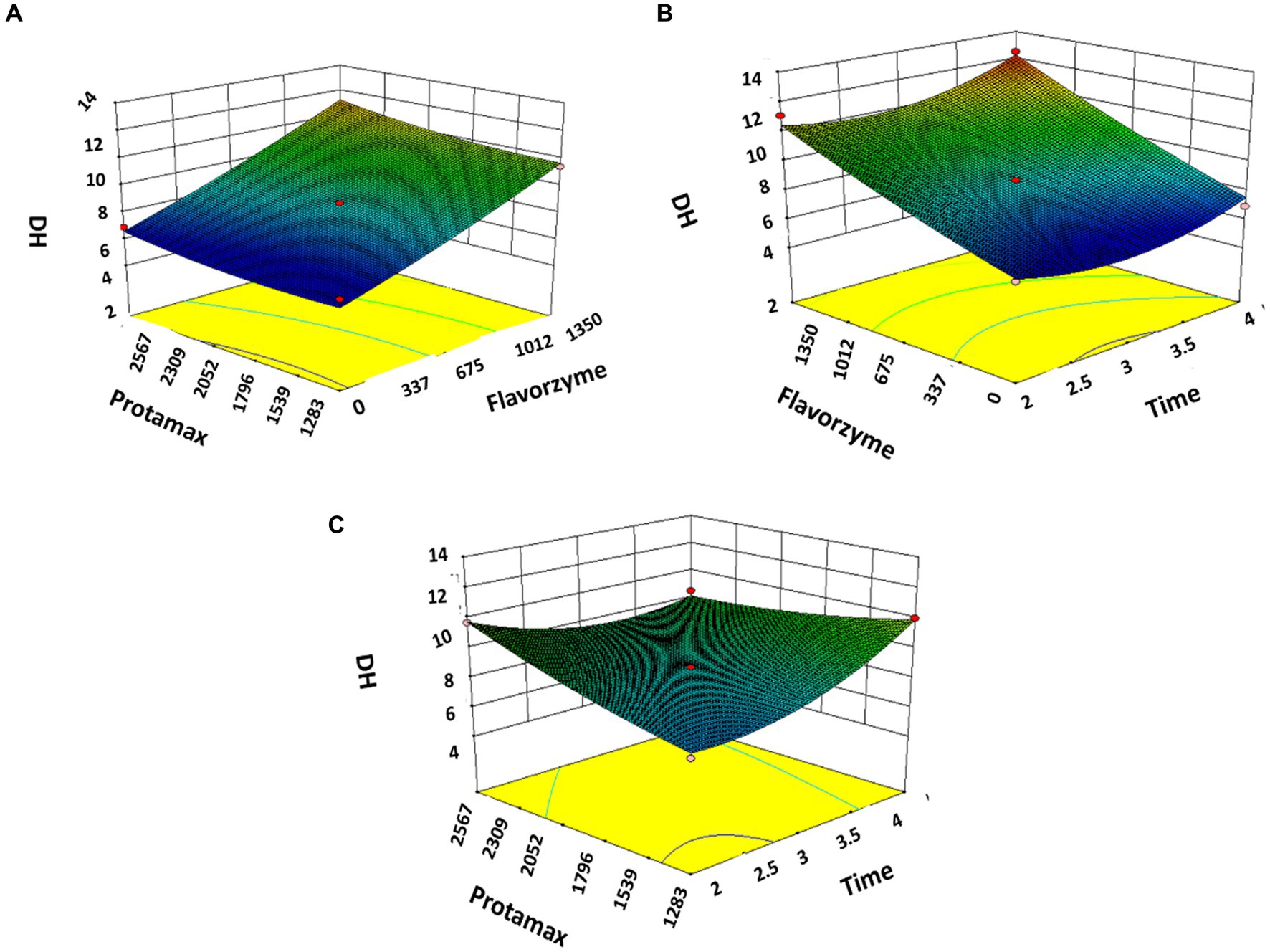
Figure 1. Response surface plots of Flavorzyme, Protamex, and time (hours) on the degree of hydrolysis of BBEH. (A) Response surface plots of Flavorzyme, Protamex to degree of hydrolysis. (B) Response surface plots of Flavorzyme, time to degree of hydrolysis. (C) Response surface plots of Protamex, time to degree of hydrolysis.
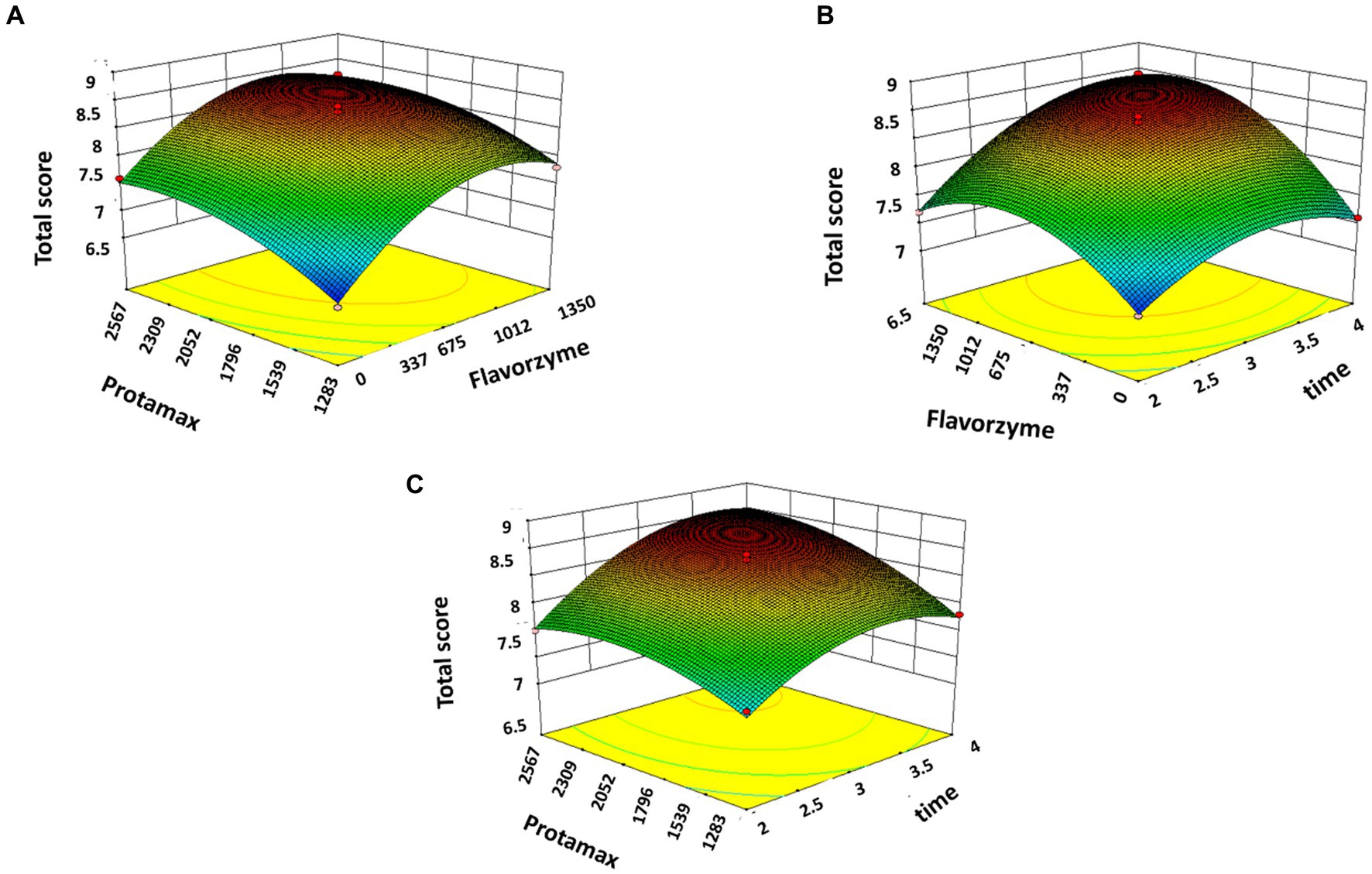
Figure 2. Response surface plots of Flavourzyme, Protamex, and time (hours) on the total score of BBEH. (A) Response surface plots of Flavorzyme, Protamex to total score. (B) Response surface plots of Flavorzyme, time to total score. (C) Response surface plots of Protamex, time to total score.
The final optimized conditions obtained through the model were as follows: the amount of Flavourzyme: 1100 U, the amount of Protamex: 2814 U, and Time: 3.77 (h). In view of operability in the actual experiment, the optimized conditions were altered as follows: extraction time: 4 h, Flavourzyme: 1150 U, and Protamex: 2800 U. Using the optimized conditions, verification experiments were performed to confirm the precision of the response model. The experimental value of the total score is 9.19, and for DH, it is 10.40, which was close to the predicted values of 8.7 and 10.17, indicating that the model was adequate and accurate for predicting the DH and total score.
Enzymatic hydrolysis of bovine bone extract
The bovine bone extract proximate composition was evaluated on a dry weight basis, and the protein content was 40 g/100 g. Thus, we could suppose that the bovine bone extract would be a good sample for obtaining functional peptides by enzymatic hydrolysis. Single-enzyme hydrolysis and a combination of two enzymes (Protamex following Flavourzyme, papain following Flavourzyme, and trypsin following Flavourzyme) were carried out. Protamex, papain, and trypsin have endopeptidase characteristics, but Flavourzyme is an exopeptidase; therefore, the reason why enzymes were combined in order to obtain the best results.
Degree of hydrolysis (DH) calculation
The antioxidant activity of the hydrolysates is related to their DH. The percentage of peptide bonds cleaved by the enzyme is defined as DH. Hydrolysates with higher DH are supposed to contain more short-chain peptides of low molecular weight. Therefore, hydrolysate with higher DH retains stronger antioxidant activity (Zhuang et al., 2013).
Specific enzymes can be used for the hydrolysis of protein, which can upgrade the antioxidant activity of hydrolysates (Wali et al., 2020). The molecular weight of the protein hydrolysate, amino acid composition, and resulting bioactivity can be affected by the selection of enzyme, hydrolysis conditions, protein substrate, and degree of hydrolysis (Balti et al., 2011). The DH and time relation of the samples hydrolyzed with Protamex, Flavourzyme, papain, and trypsin are shown in Table 4 and Figure 3. It can be seen in the table that DH gradually increased with time; the increase in DH is slight and stabilized. When the hydrolysis time reaches up to 4 h, the DH rate decreases, which can be related to the fact that as time increases, the number of peptide bonds available for hydrolysis decreases.
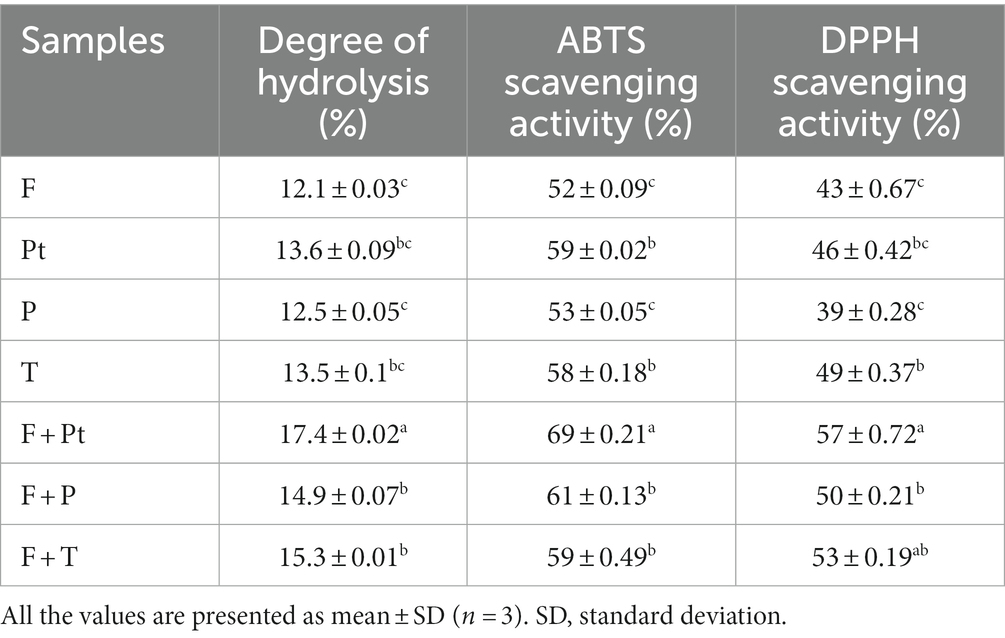
Table 4. Degree of hydrolysis, ABTS scavenging activity, and DPPH scavenging activity of each hydrolyzed sample (4 h of hydrolysis).
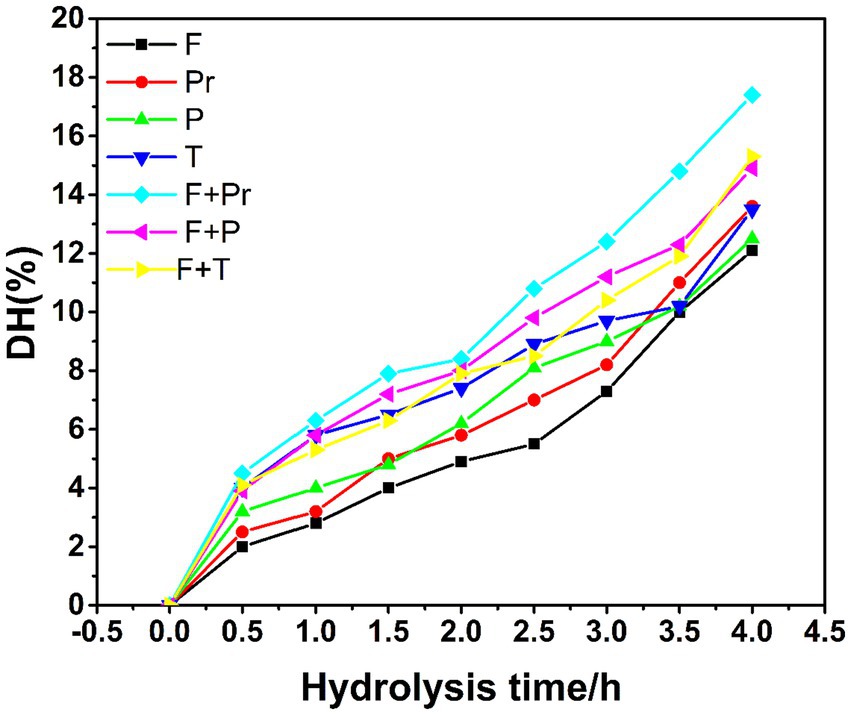
Figure 3. Degree of hydrolysis of BBE with different enzymes (F hydrolysate with Flavourzyme™, Pr hydrolysate with Protamex™, P hydrolysate with papain, T hydrolysate with trypsin, F+Pr hydrolysate with Flavourzyme™ and Protamex™, F+P hydrolysate with Flavourzyme™ and papain, F+T hydrolysate with Flavourzyme™ and trypsin).
Reaction time and type of enzyme greatly influence the DH. The results (Figure 3) indicated that for all the enzymes, DH increased with time. At 4 h of digestion time, DH associated with Flavourzyme and Protamex combination was significantly higher as compared to the other enzyme digestion. The highest DH (17.4%) for the hydrolysis of BBE was produced by Flavourzyme and Protamex combination treatment for 4 h. Moreover, in terms of radical scavenging activity, the DPPH (57%) and ABTS (69%) radical scavenging activity were higher at DH (17.4%). According to Zheng et al. (2023), enzymatic hydrolysate of hard-shelled mussels is produced using a two-step enzymatic procedure: endo-proteases (alcalase, neutrase, trypsin, and pepsin) and exogenous proteases (papain, Flavourzyme, and bromelain), which showed improved antioxidant activity. A combination of endoprotease and exoprotease action is wide in nature and can hydrolyze more of the peptide bonds of BBE (Begum et al., 2021). Therefore, the higher DH is associated with Flavourzyme + Protamex treatment because these two enzyme combinations are more effective in preparing BBE hydrolysates.
Antioxidant peptides purification
BBEH fractionation by ultrafiltration
After enzyme hydrolysis, the short-chain peptides will be obtained. Peptides have some functional activity that the parent protein does not have (Wali et al., 2020). Due to differences in mechanisms of antioxidant assays, experimental conditions, and limited standardized protocols, it is recommended to use more than one assay for the evaluation of the antioxidant potential of the materials. Antioxidant activity is closely related to the peptide length, so it is not easy to evaluate which amino acid residue plays a dynamic role in antioxidant activity (Feng et al., 2018). For the purification and identification of peptides of different molecular weights, the ultrafiltration method was selected. The BBEH was fractionated by ultrafiltration with molecular weight cutoff (MWCO) membranes with a concentration of 1 g/10 mL in distilled water to obtain three fractions, which were named F-I (MW 5–3 kDa), F-II (3–1 kDa), and F-III (<1 kDa), respectively. The molecular weight (MW) of peptides has a vital role in antioxidant activity. Low molecular weight hydrolysate peptides generally have high antioxidant activity compared to large molecular weight peptides (Xing et al., 2018). Previously, many researchers investigated the relationship between bioactivity and MW through fractionation by ultrafiltration, and it has been stated that higher activity is directly associated with the lower MW (Zhuang et al., 2013). As shown in Figure 4 and Table 5, all the fractions showed radical scavenging capacities at 5 mg/mL concentration, among which the strongest scavenging ability was observed in F-III in terms of DPPH radical, metal chelating, and ABTS radical, while both F-I and F-II showed the low DPPH radical scavenging activity and metal chelating activity. In terms of TBA and reducing power ability (Table 5), the activity increased as MW decreased. However, F-III was comparatively rich in low MW peptides. In the study reported in Wang et al. (2022), skipjack tuna roes hydrolysate was separated (50.0 mg/mL) into different components using the MWCO ultrafiltration membranes of 1, 3, and 5 kDa, respectively. Four resulting components, defined as TRPH-I (MW < 1 kDa), TRPH-II (1 < MW < 3 kDa), TRPH-III (3 < MW < 5 kDa), and TRPH-IV (MW > 5 kDa), were collected. TRPH-I fraction having a molecular weight (<1 kDa) having the highest DPPH• scavenging activity (55.23 ± 0.8%). Other studies also confirmed that the protein hydrolysate antioxidant ability is negatively influenced by the molecular weight of the peptides. Our study is also in line with other studies that low molecular weight fraction of hydrolysate from tuna dark muscle, skate cartilage, Antarctic krill, and miiuy croaker muscle had higher activities (He et al., 2019; Wang et al., 2021). Ultrafiltration fraction from Monkfish (Lophius litulon) Swim Bladders having molecular weight of <1 kDa showed significantly higher DPPH (51.57 ± 1.45%) and HO·scavenging rates (76.96 ± 2.40%) as compared to the higher molecular weight fraction (Sheng et al., 2023).
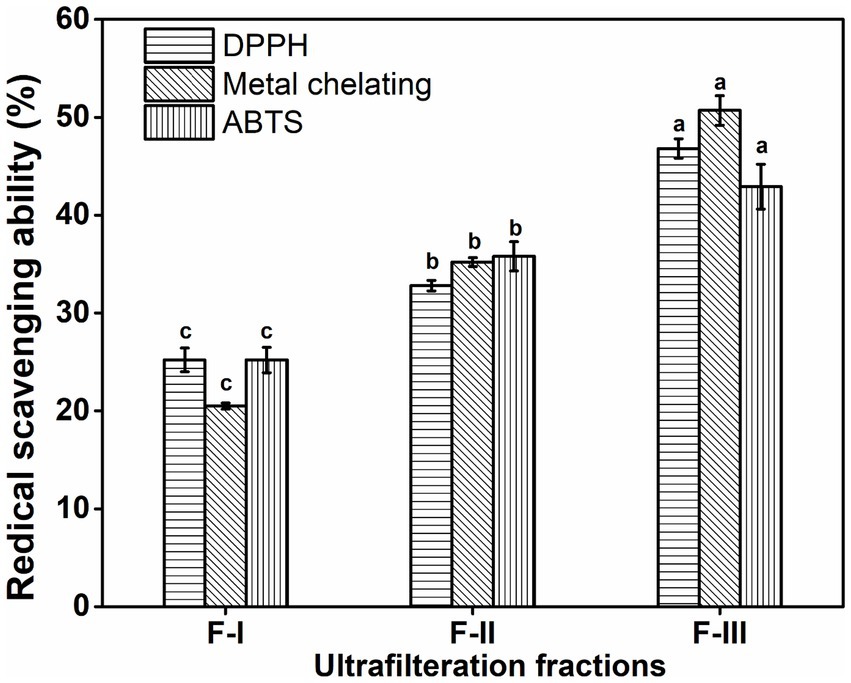
Figure 4. Radical scavenging abilities of fractions isolated by ultrafiltration at 5 mg/mL. All the values were mean ± SD (n = 3). SD, standard deviation (p > 0.05).
These findings were comparable to the other researchers’ findings showing that the antioxidant activity of the hydrolysates is strongly dependent on the MW distribution. Hydrolysate and low MW peptides can interact with free radicals and control or slow the process of oxidation (Guo et al., 2015). Therefore, fractions under 1 kDa were selected for further antioxidant ability analysis.
Gel permeation chromatography
Enzymatic hydrolysis can produce a wide range of free amino acids and smaller peptides. Peptide activity is highly affected by the molecular size of the peptides. The most frequent method used to separate bioactive peptides from protein hydrolysate is gel filtration. These changes in protein structure can affect antioxidant activity (Kou et al., 2013). This method also separates colloids from low molecular weight solutions and removes salt from the protein mixture (Najafian and Babji, 2014). Fraction F-III obtained from ultrafiltration exhibited higher antioxidant activity; then, it was further separated into several fractions using gel filtration chromatography at the concentration of 1 mg/mL, among which three fractions, Frac-I, Frac-II, and Frac-III, showed the highest antioxidant activities. All fractions separated showed antioxidant activities. The results of DPPH radical activity, reducing power, and gel permeation chromatography results of F-III of BBEH are shown in Figure 5.
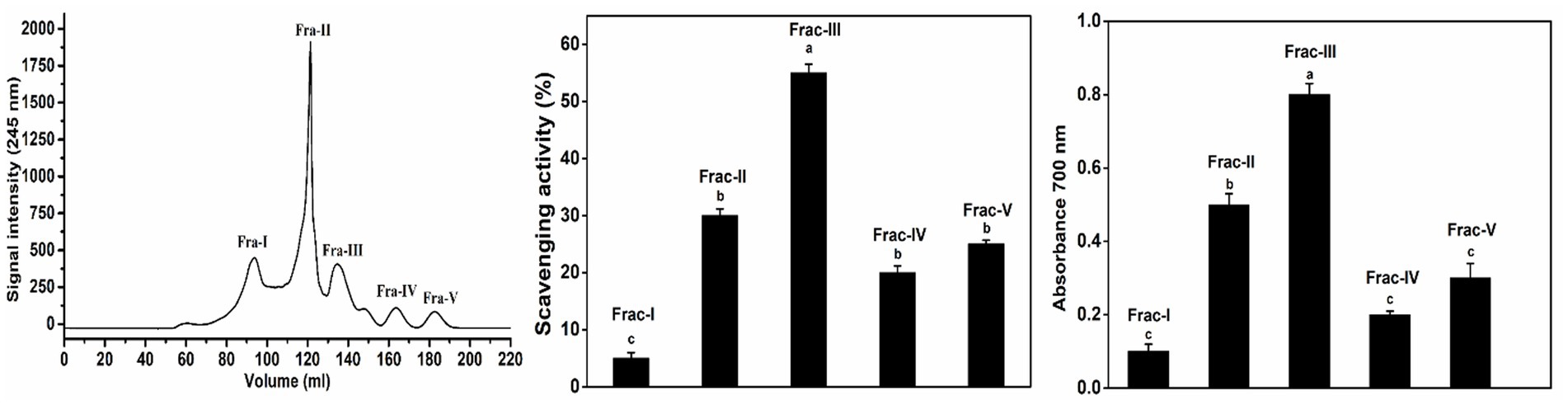
Figure 5. Gel filtration chromatography of F-III on a Superdex Peptide 10/300GL (10 mm × 300 mm). Elution profile of bovine bone extract hydrolysate (left) and the DPPH radical-scavenging activities and reducing the power of the separated fractions (right).
According to the scavenging ability shown in Figure 5, the Frac-III had the highest scavenging rate of 55.06% among all fractions, and the Frac-IV had the lowest radical scavenging activity of 20%. According to Cai et al. (2022), skipjack tuna cardiac arterial bulbs enzyme hydrolysate ultrafiltration fraction was sub-fractionated using a Sephadex G-25 column, and two fractions GPC-I and GPC-II were obtained. DPPH and HO scavenging rates of GPC-II were 76.71 ± 1.66% and 70.45 ± 1.84%, respectively. The results showed that peptides that elute later showed higher antioxidant activity. According to Wang et al. (2022), the DPPH• activity of IC-iii was lower than IC-ii, but its MW was higher than that of IC-iii (p < 0.05). These findings suggest that the activity of peptides depends not only on peptide molecular size but also on other factors such as the sequence of amino acids and composition (Sila and Bougatef, 2016). Antioxidant peptide from Antarctic krill hydrolysate was identified using stepwise chromatography, and the gel chromatography results showed that DPPH• (48.19 ± 2.51%) and HO• (40.52 ± 2.03%) scavenging abilities of AK-II-1 were significantly higher than those of other fractions at the concentration of 1.0 mg protein/mL (p < 0.05) (Wang et al., 2021).
Various bioactive compounds have a direct association with reducing power and antioxidant activity. Therefore, the reducing test was probed to calculate the activity of all the fractions. The absorbance of Frac-III at 700 nm was 0.8, followed by Frac-II (0.52). Xing et al. (2018) reported that short-chain peptides with an MW of <1,400 Da purified from mackerel hydrolysate had an improved ability to scavenge free radicals. Other studies also showed higher hydroxyl radical scavenging activity of lower MW fractions than larger MW fractions (Bah et al., 2016; Mohammadi et al., 2016). Consequently, the Frac-III revealed the highest antioxidant activity, so it was selected for further analysis.
Separation of Frac-III by RP-HPLC
The hydrophilic and hydrophobic characteristics of peptides play a vital role in the retention time to elute in the RP-HPLC column; also, the ratio of polar and non-polar solvents adjusts the retention time. The peptide separation with RP-HPLC takes advantage of the molecular polarity differences in the tested solution. RP-HPLC is considered a popular method for the purification of peptides from protein hydrolysates (Li et al., 2017).
A purified fraction from gel filtration that exhibited the highest antioxidant activity was purified using RP-HPLC; due to the advantages of high efficiency of the column, improved resolution, and sensitivity, it was suggested to be the last step in the purification of the peptides.
According to Zhang et al. (2014), polar peptides (hydrophilic) eluted first than non-polar (hydrophobic peptides). Figure 6 and Table 6 present the scavenging activity and elution fractions from Frac-III. The gel filtration fraction was further separated into six fractions (fractions 1 to 6), as shown in Figure 6. As compared to the other fractions, the activity at the concentration of 500 μg/mL of the sixth peak of the RP-HPLC fractions had highest DPPH scavenging ability (69.45%). The sixth fraction was eluted at 15.23 min, and the retention time was longer than the other five peaks. It has been proposed that peptide fractions separated by hydrophobic chromatography column have a large elution time, suggesting that antioxidant peptides from BBEH retain strong hydrophobic characteristics. Myofibrillar protein hydrolysates were fractionated using RP-HPLC into four fractions. Fraction 4 having an elution time of 12:63 min showed high DPPH radical scavenging activity as compared to other fractions. Owing to the large elution time in the hydrophobic column, it has been suggested that peptides from the sample retain strong hydrophobic properties. The antioxidant activity of the purified peptides was 2.97-fold greater than the sample without separation (Najafian and Babji, 2014). Sheep plasma protein hydrolysate was purified using semi-preparation RP-HPLC; based on the retention time, nine fractions were collected. The DPPH radical scavenging ability and FRAP antioxidant capacity were evaluated, and among all fractions, F1and F2 exhibited significantly higher DPPH and FRAP activity (97.28%), (97.10%), and (64.58–89.58%), respectively. Numerous studies determine the biological activity of protein hydrolysates; the size of peptides is closely related to the activity; and the most active fraction in these studies contributing to activity was <1 kDa fraction. However, various other factors such as the structure of these peptides and richness in hydrophobic and aromatic amino acids also responsible for activity (Hou et al., 2019). These results were consistent with Megías et al. (2004) and Zhao et al. (2010). The structural characteristics of peak 6 were further identified for the conformation of hydrophobic amino acids present.
Analysis of antioxidant peptide sequences and peptide synthesis
For peptide sequence identification, mass spectrometric approaches have been widely used due to their high sensitivity (Hu et al., 2019). To identify the peptides that were mainly accountable for the activity, peak-6 obtained through RP-HPLC was further identified using LC–MS/MS. The peptides were found with m/z 333.12 (M + H)+ and 369.14 (M + H)+, and the sequences of peptides were Ala-Pro-Phe and Asp-His-Val, respectively (Figures 7A,B). Previous research showed that the antioxidant activity of peptides depends on structure, molecular weight, sequence, and amino acid composition (Xia et al., 2020). Peptide solubility in lipids can be enhanced by hydrophobic peptides, which enables accessibility to hydrophobic radical species. Non-polar amino acids (Met, Ala, Pro, Val, Leu, and Trp) played a vital role in the free radical scavenging activity. It was identified previously that the majority of peptides exhibit antioxidant activity by having one or more hydrophobic amino acids (Samaranayaka and Li-Chan, 2011; Zhu et al., 2013). Peptide consists of 2–20 amino acids that can exert biological effects by crossing the intestinal barrier (Shahidi and Zhong, 2008). Other researchers also reported that molecular weight distribution has an important role in the antioxidant activity of the hydrolysates. Low molecular weight hydrolysates and peptides possess high antioxidant activity (Guo et al., 2015).
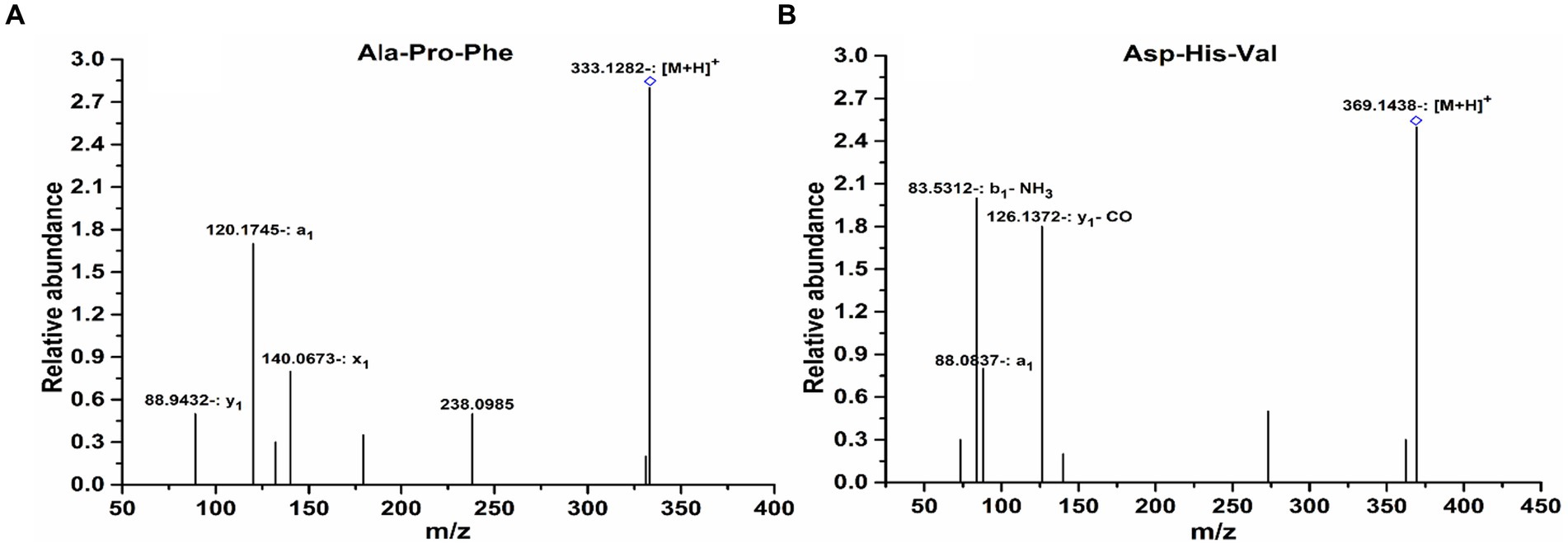
Figure 7. Identification of the molecular weight and amino acid sequence of the purified peptide using the LC–MS/MS spectrum. (A) Ala-Pro-Phe. (B) Asp-His-Val.
The identified peptide (Ala-Pro-Phe) shown in Figure 7A consists of hydrophobic and aromatic amino acids. Previous research revealed increased radical scavenging ability in peptides having aromatic and hydrophobic amino acids (Sarmadi and Ismail, 2010).
The identified peptides were synthesized, on the basis of their amino acid sequence. The antioxidant activity was investigated using the DPPḤ and TBA assays. As shown in Table 7, both the synthesized peptides exhibit antioxidant activity, and the peptide (Ala-Pro-Phe) showed the highest DPPH radical scavenging activity and TBA value IC50 (0.158 mg/mL) and 1.82 mg malonaldehyde/kg.
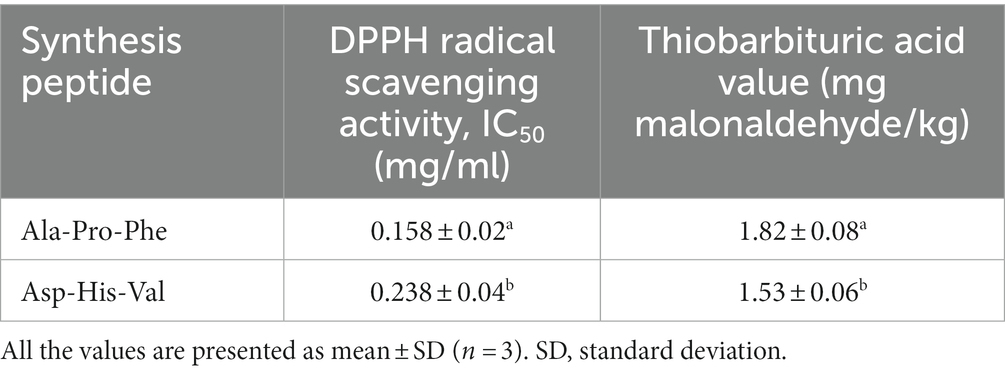
Table 7. Antioxidant activities of the synthesized peptides from bovine bone extract hydrolysate (BBEH).
Protein hydrolysates are abundant in non-polar (hydrophobic) amino acids (i.e., Leu, Ala, Val, Ile, Phe, Tyr, Pro, Lys, and Met) and conform to antioxidant capacity because of the presence of a large number of electrons that can quench free radicals by donating their electrons (Rong et al., 2013; Zhang et al., 2014). The identified peptide (Asp-His-Val) shown in Figure 7B contains histidine. Histidine-containing peptides have been reported to have antioxidant capacity. Histidine can donate electrons via resonance structure and convert radicals to stable molecules (Park et al., 2001). The acidic amino acids present, such as Asp (aspartic acid) in the peptide sequence, are important for the antioxidant activity because of the carboxyl group in their side chain (Suetsuna et al., 2000). Short-chain peptides were previously separated and identified, e.g., hydrolysates of corn gluten (375.46, 488.64, and 522.64 Da), hydrolysates of sweet potato protein (640–930 Da), and hydrolysates of Zizyphus jujube protein (678.36 and482.27 Da) (Wongsrangsap and Chukiatsiri, 2021). According to the literature, aspartic acid possesses free radical quenching activity, due to the existence of free electrons, while the presence of an imidazole ring in histidine structure acts as a proton donor, indole and pyrrolidine rings in proline donate hydrogen via hydroxyl group. In our study, the identified peptide also consists of these amino acids. A favorable hydrophobic microenvironment for peptides can be produced by hydrophobic amino acids such as phenylalanine and valine (Ma et al., 2018). Peptides isolated from pea protein hydrolysate have 3–7 amino acids and molecular weights of 933.06, 507.59, and 429.47 Da. The ratio of hydrophobic amino acids in the peptide structure was 33.33, 50, and 28.57%, respectively, which may be helpful by providing H+ and acting as an antioxidant source (Zhao and Liu, 2023).
Conclusion
In the current study, we have fractionated and characterized two antioxidant peptides from BBEH (bovine bone extract hydrolysate) using various enzymes for hydrolysis (Flavourzyme and Protamex) for the first time. The results show that BBEH is effective for chelating ferrous ions and scavenging free radicals. Peptides with various molecular weights (MW) and amino acid sequences were purified after a series of steps, and their antioxidant capacities were assessed. The results indicated that ultrafiltration Fra-III infatuated high free radical scavenging activity and was further purified into 6 fractions by RP-HPLC. The MW and amino acid sequence of the purified peptide were identified as Ala-Pro-Phe (333.12 Da) and Asp-His-Val (369.14 Da). Two newly purified peptides can be used for free radical scavenging and as natural antioxidants to prevent food oxidation. These results could play the foundation of the practical utilization of BBEH peptides as functional food ingredients and provide the basis for the application of BBEH antioxidant peptides in pharmaceuticals. The potential application of this finding might provide a novel strategy for using antioxidant peptides in health-promoting products and can replace synthetic antioxidants. However, further in vivo studies are required to examine the antioxidant capacities of these two purified peptides; moreover, studies should be done to determine structure–activity, physiological functions relationship, and application of the identified peptides.
Data availability statement
The original contributions presented in the study are included in the article/Supplementary material, further inquiries can be directed to the corresponding authors.
Author contributions
NB: Investigation, Methodology, Software, Writing – original draft, Writing – review & editing. QK: Writing – review & editing. SA-D: Conceptualization, Data curation, Formal analysis, Methodology, Software, Writing – original draft, Writing – review & editing. DLu: Conceptualization, Formal analysis, Validation, Investigation, Writing – review & editing. FY: Conceptualization, Data curation, Formal analysis, Investigation, Methodology, Writing – review & editing. JL: Conceptualization, Formal analysis, Methodology, Writing – review & editing. DW: Conceptualization, Data curation, Formal analysis, Validation, Writing – review & editing. RL: Conceptualization, Formal analysis, Methodology, Validation, Writing – review & editing. JW: Conceptualization, Data curation, Methodology, Validation. DLi: Formal analysis, Funding acquisition, Project administration, Resources, Supervision, Writing – review & editing. HS: Formal analysis, Funding acquisition, Project administration, Resources, Supervision, Writing – review & editing.
Funding
The author(s) declare financial support was received for the research, authorship, and/or publication of this article. This study was financially supported by the National Natural Science Foundation of China (Grant Nos. 82270413 and 81870307), the Natural Science Foundation of Guangdong Province of China (Grant Nos. 2022A1515011368 and 2023A1515011581), the Key Projects of Department of Education of Guangdong Province of China (Grant Nos. 2022ZDZX2057 and 2022ZXKC474), and the Guangzhou basic research program of 2023 (Grant No. 2023A04J0708).
Conflict of interest
QK was employed by Vanced Materials Technology (Zhongshan) Co., Ltd.
The remaining authors declare that the research was conducted in the absence of any commercial or financial relationships that could be construed as a potential conflict of interest.
Publisher’s note
All claims expressed in this article are solely those of the authors and do not necessarily represent those of their affiliated organizations, or those of the publisher, the editors and the reviewers. Any product that may be evaluated in this article, or claim that may be made by its manufacturer, is not guaranteed or endorsed by the publisher.
Supplementary material
The Supplementary material for this article can be found online at: https://www.frontiersin.org/articles/10.3389/fsufs.2023.1345833/full#supplementary-material
Abbreviations
BBE, Bovine bone extract; BBEH, Bovine bone extract hydrolysate; DH, Degree of hydrolysis; DPPH, 1,1, diphenyl-2-picrylhydrazl; ABTS, 2,2′-azino-bis (3-ethylbenzothiazoline-6-sulfonic acid); MCA, Metal chelating activity; RP, Reducing power; TBA, Thiobarbituric acid assay; UF, Ultrafiltration; RP-HPLC, Reversed-phase high-performance liquid chromatography; GFC, Gel filtration chromatography; LC/MS/MS, Liquid chromatography–mass spectrometry; TCA, Trichloroacetic acid; BHA, Butylated hydroxyanisole; NaOH, Sodium hydroxide; HCL, Hydrochloric acid; AN, Amino nitrogen; TN, Total nitrogen.
References
Bah, C., Bekhit, A., McConnell, M., and Carne, A. (2016). Generation of bioactive peptide hydrolysates from cattle plasma using plant and fungal proteases. Food Chem. 213, 98–107. doi: 10.1016/j.foodchem.2016.06.065
Balti, R., Bougatef, A., El Hadj Ali, N., Ktari, N., Jellouli, K., Nedjar-Arroume, N., et al. (2011). Comparative study on biochemical properties and Antioxidative activity of cuttlefish (Sepia officinalis) protein hydrolysates produced by Alcalase and Bacillus licheniformis NH1 proteases. J. Amino Acids 2011:107179. doi: 10.4061/2011/107179
Begum, N., Raza, A., Song, H., Iftikhar, M., Zhang, Y., Zhang, L., et al. (2021). Fractionation and identification of flavor peptides from bovine bone extract after enzymatic hydrolysis and Maillard reaction by consecutive chromatography. J. Food Proc. Preserv. 45:e15778. doi: 10.1111/jfpp.15778
Buege, J., Aust, S., Fleischer, S., and Packer, L. (1978). Microsomal lipid peroxidation. Methods Enzymol. 52, 302–310.
Cai, W.-W., Hu, X.-M., Wang, Y.-M., Chi, C.-F., and Wang, B. (2022). Bioactive peptides from skipjack tuna cardiac arterial bulbs: preparation, identification, antioxidant activity, and stability against thermal, pH, and simulated gastrointestinal digestion treatments. Mar. Drugs 20:626. doi: 10.3390/md20100626
Chiang, J. H., Loveday, S. M., Hardacre, A. K., and Parker, M. E. (2019). Effects of enzymatic hydrolysis treatments on the physicochemical properties of beef bone extract using endo- and exoproteases. Int. J. Food Sci. Technol. 54, 111–120. doi: 10.1111/ijfs.13911
Decker, E. A., and Welch, B. (1990). Role of ferritin as a lipid oxidation catalyst in muscle food. J. Agric. Food Chem. 38, 674–677. doi: 10.1021/jf00093a019
Elango, J., Robinson, J., Zhang, J., Bao, B., Ma, N., de Val, J., et al. (2019). Collagen peptide upregulates osteoblastogenesis from bone marrow mesenchymal stem cells through MAPK- Runx2. Cells :446:8. doi: 10.3390/cells8050446
El-Shourbagy, G., and Elzahar, K. (2014). Oxidative stability of ghee as affected by natural antioxidants extracted from food processing wastes. Ann. Agric. Sci. 59, 213–220. doi: 10.1016/j.aoas.2014.11.008
Feng, Y., Ruan, G.-R., Jin, F., Xu, J., and Wang, F.-J. (2018). Purification, identification, and synthesis of five novel antioxidant peptides from Chinese chestnut (Castanea mollissima Blume) protein hydrolysates. LWT 92, 40–46. doi: 10.1016/j.lwt.2018.01.006
Guo, P., Qi, S., Chuanhe, Z., and Wang, Q. (2015). Purification and identification of antioxidant peptides from Chinese cherry (Prunus pseudocerasus Lindl.) seeds. J. Funct. Foods 19, 394–403. doi: 10.1016/j.jff.2015.09.003
He, Y., Pan, X., Chi, C.-F., Sun, K.-L., and Wang, B. (2019). Ten new pentapeptides from protein hydrolysate of miiuy croaker (Miichthys miiuy) muscle: preparation, identification, and antioxidant activity evaluation. LWT 105, 1–8. doi: 10.1016/j.lwt.2019.01.054
Hou, C., Wu, L., Wang, Z., Saguer, E., and Zhang, D. (2019). Purification and identification of antioxidant alcalase-derived peptides from sheep plasma proteins. Antioxidants 8:592. doi: 10.3390/antiox8120592
Hu, X., Yang, X., Wu, Q., Li, L., Wu, Y., Chen, S., et al. (2019). Purification and identification of antioxidant peptides from schizochytrium limacinum hydrolysates by consecutive chromatography and electrospray ionization-mass spectrometry. Molecules 24:3004. doi: 10.3390/molecules24163004
Igase, M., Kohara, K., Okada, Y., Ochi, M., Igase, K., Inoue, N., et al. (2018). A double-blind, placebo-controlled, randomised clinical study of the effect of pork collagen peptide supplementation on atherosclerosis in healthy older individuals. Biosci. Biotechnol. Biochem. 82, 893–895. doi: 10.1080/09168451.2018.1434406
Kembhavi, A. A., Kulkarni, A., and Pant, A. (1993). Salt-tolerant and thermostable alkaline protease from Bacillus subtilis NCIM no. 64. Appl. Biochem. Biotechnol. 38, 83–92. doi: 10.1007/BF02916414
Kou, X., Gao, J., Xue, Z., Zhang, Z., Wang, H., and Wang, X. (2013). Purification and identification of antioxidant peptides from chickpea (Cicer arietinum L.) albumin hydrolysates. LWT Food Sci. Technol. 50, 591–598. doi: 10.1016/j.lwt.2012.08.002
Li, X.-R., Chi, C.-F., Li, L., and Wang, B. (2017). Purification and identification of antioxidant peptides from protein hydrolysate of scalloped hammerhead (Sphyrna lewini) cartilage. Mar. Drugs 15:61. doi: 10.3390/md15030061
Liu, P., Huang, M., Song, S., Hayat, K., Zhang, X., Xia, S., et al. (2010). Sensory characteristics and antioxidant activities of Maillard reaction products from soy protein hydrolysates with different molecular weight distribution. Food Bioproc. Technol. 5, 1–15. doi: 10.1007/s11947-010-0440-3
Ma, Y., Wu, Y., and Li, L. (2018). Relationship between primary structure or spatial conformation and functional activity of antioxidant peptides from Pinctada fucata. Food Chem. 264, 108–117
Megías, C., Yust, M., Pedroche, J., Lquari, H., Girón-Calle, J., Alaiz, M., et al. (2004). Purification of an ACE inhibitory peptide after hydrolysis of sunflower (Helianthus annuus L.) protein isolates. J. Agric. Food Chem. 52, 1928–1932. doi: 10.1021/jf034707r
Mohammadi, R., Mohammadifar, M. A., Mortazavian, A. M., Rouhi, M., Ghasemi, J. B., and Delshadian, Z. (2016). Extraction optimization of pepsin-soluble collagen from eggshell membrane by response surface methodology (RSM). Food Chem. 190, 186–193. doi: 10.1016/j.foodchem.2015.05.073
Müller, T. D., Clemmensen, C., Finan, B., DiMarchi, R. D., and Tschöp, M. H. (2018). Anti-obesity therapy: from rainbow pills to polyagonists. Pharmacol. Rev. 70, 712–746. doi: 10.1124/pr.117.014803
Najafian, L., and Babji, A. (2014). Isolation, purification and identification of three novel antioxidative peptides from patin (Pangasius sutchi) myofibrillar protein hydrolysates. LWT Food Sci. Technol. 60, 452–461. doi: 10.1016/j.lwt.2014.07.046
Park, J.-N., Fukumoto, Y., Fujita, E., Tanaka, T., Washio, T., Otsuka, S., et al. (2001). Chemical composition of fish sauces produced in Southeast and East Asian countries. J. Food Comp. Analy. 14, 113–125
Re, R., Pellegrini, N., Proteggente, A., Pannala, A., Yang, M., and Rice-Evans, C. (1999). Antioxidant activity applying an improved ABTS radical cation decolorization assay. Free Radic. Biol. Med. 26, 1231–1237. doi: 10.1016/S0891-5849(98)00315-3
Ren, Z., Yang, F., Yao, S., Bi, L., Jiang, G., Huang, J., et al. (2022). Effects of low molecular weight peptides from monkfish (Lophius litulon) roe on immune response in immunosuppressed mice. Front. Nutr. 9:929105. doi: 10.3389/fnut.2022.929105
Rong, H., Girgih, A., Malomo, S., Ju, X., and Aluko, R. (2013). Antioxidant activities of enzymatic rapeseed protein hydrolysates and the membrane ultrafiltration fractions. J. Funct. Foods 5, 219–227. doi: 10.1016/j.jff.2012.10.008
Rozi, P., Maimaiti, P., Abuduwaili, A., and Wali, A. (2018). Isolation and evaluation of bioactive protein and peptide from domestic animals’ bone marrow. Molecules 23:1673. doi: 10.3390/molecules23071673
Samaranayaka, A., and Li-Chan, E. (2011). Food-derived peptidic antioxidants: a review of their production, assessment, and potential applications. J. Funct. Foods 3, 229–254. doi: 10.1016/j.jff.2011.05.006
Sarmadi, B. H., and Ismail, A. (2010). Antioxidative peptides from food proteins: a review. Peptides 31, 1949–1956. doi: 10.1016/j.peptides.2010.06.020
Shahidi, F., and Zhong, Y. (2008). Bioactive peptides. J. AOAC Int. 91, 914–931. doi: 10.1093/jaoac/91.4.914
Sheng, Y., Wang, W.-Y., Wu, M.-F., Wang, Y.-M., Zhu, W.-Y., Chi, C.-F., et al. (2023). Eighteen novel bioactive peptides from monkfish (Lophius litulon) swim bladders: production, identification, antioxidant activity, and stability. Mar. Drugs 21:169. doi: 10.3390/md21030169
Sierra, L., Fan, H., Zapata, J., and Wu, J. (2021). Antioxidant peptides derived from hydrolysates of red tilapia (Oreochromis sp.) scale. LWT 146:111631. doi: 10.1016/j.lwt.2021.111631
Sila, A., and Bougatef, A. (2016). Antioxidant peptides from marine by-products: isolation, identification and application in food systems. A review. J. Funct. Foods 21, 10–26. doi: 10.1016/j.jff.2015.11.007
Suetsuna, K., Ukeda, H., and Ochi, H. (2000). Isolation and characterization of free radical scavenging activities peptides derived from casein. J. Nutr. Biochem. 11, 128–131. doi: 10.1016/S0955-2863(99)00083-2
Wali, A., Yanhua, G., Ishimov, U., Yili, A., Aisa, H., and Salikhov, S. (2020). Isolation and identification of three novel antioxidant peptides from the Bactrian camel milk hydrolysates. Int. J. Pept. Res. Ther. 26, 641–650. doi: 10.1007/s10989-019-09871-x
Wang, J., Wang, Y.-M., Li, L.-Y., Chi, C.-F., and Wang, B. (2022). Twelve antioxidant peptides from protein hydrolysate of skipjack tuna (Katsuwonus pelamis) roe prepared by flavourzyme: purification, sequence identification, and activity evaluation. Front. Nutr. 8:813780. doi: 10.3389/fnut.2021.813780
Wang, Y.-Z., Zhao, Y.-Q., Wang, Y.-M., Zhao, W.-H., Wang, P., Chi, C.-F., et al. (2021). Antioxidant peptides from Antarctic krill (Euphausia superba) hydrolysate: preparation, identification and cytoprotection on H2O2-induced oxidative stress. J. Funct. Foods 86:104701. doi: 10.1016/j.jff.2021.104701
Wongsrangsap, N., and Chukiatsiri, S. (2021). Purification and identification of novel antioxidant peptides from enzymatically hydrolysed Samia ricini pupae. Molecules 26:2588. doi: 10.3390/molecules26092588
Wu, H.-C., Chen, H.-M., and Shiau, C.-Y. (2003). Free amino acid and peptide as related to antioxidant properties in protein hydrolysates of mackerel (Scomber austriasicus). Food Res. Int. 36, 949–957. doi: 10.1016/S0963-9969(03)00104-2
Wu, M.-F., Xi, Q.-H., Sheng, Y., Wang, Y.-M., Wang, W.-Y., Chi, C.-F., et al. (2023). Antioxidant peptides from monkfish swim bladders: ameliorating NAFLD in vitro by suppressing lipid accumulation and oxidative stress via regulating AMPK/Nrf2 pathway. Mar. Drugs 21:360. doi: 10.3390/md21060360
Xia, J., Song, H., Huang, K., Li, S., and Guan, X. (2020). Purification and characterization of antioxidant peptides from enzymatic hydrolysate of mungbean protein. J. Food Sci. 85, 1735–1741. doi: 10.1111/1750-3841.15139
Xing, L., Liu, R., Gao, X., Zheng, J., Wang, C., Zhou, G. H., et al. (2018). The proteomics homology of antioxidant peptides extracted from dry-cured Xuanwei and Jinhua ham. Food Chem. 266, 420–426. doi: 10.1016/j.foodchem.2018.06.034
Yamamoto, S., Deguchi, K., Onuma, M., Numata, N., and Sakai, Y. (2016). Absorption and urinary excretion of peptides after collagen tripeptide ingestion in humans. Biol. Pharm. Bull. 39, 428–434. doi: 10.1248/bpb.b15-00624
Yang, C., Song, H., and Chen, F. (2012). Response surface methodology for meat‐like odorants from Maillard reaction with glutathione I: The optimization analysis and the general pathway exploration, J Food Sci. 77, C966–C974.
Yang, X. R., Zhao, Y. Q., Qiu, Y. T., and Chi, C. (2019). F.: preparation and characterization of gelatin and antioxidant peptides from gelatin hydrolysate of skipjack tuna (Katsuwonus pelamis) bone stimulated by in vitro gastrointestinal. Digestion 17:78. doi: 10.3390/md17020078
Yao, Y., Wang, M., Liu, Y., Han, L., and Liu, X. (2020). Insights into the improvement of the enzymatic hydrolysis of bovine bone protein using lipase pretreatment. Food Chem. 302:125199. doi: 10.1016/j.foodchem.2019.125199
Zhang, M., Mu, T. H., and Sun, M.-J. (2014). Purification and identification of antioxidant peptides from sweet potato protein hydrolysates by Alcalase. J. Funct. Foods 7, 191–200. doi: 10.1016/j.jff.2014.02.012
Zhao, D., and Liu, X. (2023). Purification, identification and evaluation of antioxidant peptides from pea protein hydrolysates. Molecules 28:2952. doi: 10.3390/molecules28072952
Zhao, M., Regenstein, J., and Ren, J. (2010). Changes in the antioxidant activity of loach (Misgurnus anguillicaudatus) protein hydrolysates during a simulated gastrointestinal digestion. Food Chem. 120, 810–816. doi: 10.1016/j.foodchem.2009.11.018
Zhao, Y., Zhao, Q., and Lu, Q. (2020). Purification, structural analysis, and stability of antioxidant peptides from purple wheat bran. BMC Chem. 14:58. doi: 10.1186/s13065-020-00708-z
Zheng, S.-L., Wang, Y.-Z., Zhao, Y.-Q., Chi, C.-F., Zhu, W.-Y., and Wang, B. (2023). High Fischer ratio oligopeptides from hard-shelled mussel: preparation and hepatoprotective effect against acetaminophen-induced liver injury in mice. Food Biosci. 53:102638. doi: 10.1016/j.fbio.2023.102638
Zhu, C., Zhang, W., Zhou, G. H., Xu, X.-L., Kang, Z., and Yin, Y. (2013). Isolation and identification of antioxidant peptides from Jinhua ham. J. Agric. Food Chem. 61, 1265–1271. doi: 10.1021/jf3044764
Keywords: cultured meat, enzymatic hydrolysate, ultrafiltration, antioxidant capacity, chromatography, hydrophobicity, peptide
Citation: Begum N, Khan QU, Al-Dalali S, Lu D, Yang F, Li J, Wu D, Li R, Wang J, Liu D and Song H (2024) Process optimization and identification of antioxidant peptides from enzymatic hydrolysate of bovine bone extract, a potential source in cultured meat. Front. Sustain. Food Syst. 7:1345833. doi: 10.3389/fsufs.2023.1345833
Edited by:
Khurshid Ahmad, Yeungnam University, Republic of KoreaReviewed by:
Bin Wang, Zhejiang Ocean University, ChinaKhashayar Sarabandi, Research Institute of Food Science and Technology (RIFST), Iran
Aphichart Karnchanatat, Chulalongkorn University, Thailand
Ahmed A. Zaky, National Research Centre, Egypt
Copyright © 2024 Begum, Khan, Al-Dalali, Lu, Yang, Li, Wu, Li, Wang, Liu and Song. This is an open-access article distributed under the terms of the Creative Commons Attribution License (CC BY). The use, distribution or reproduction in other forums is permitted, provided the original author(s) and the copyright owner(s) are credited and that the original publication in this journal is cited, in accordance with accepted academic practice. No use, distribution or reproduction is permitted which does not comply with these terms.
*Correspondence: Dahai Liu, seansean2014@126.com; Huanlu Song, songhl@th.btbu.edu.cn