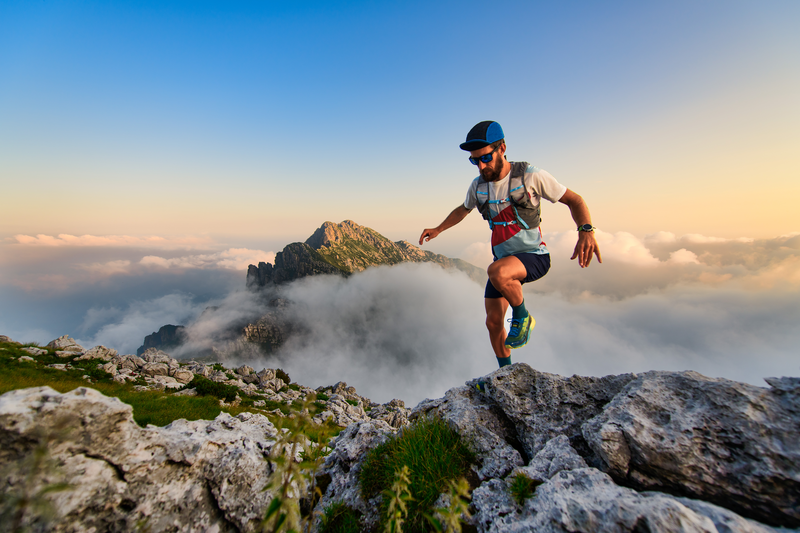
94% of researchers rate our articles as excellent or good
Learn more about the work of our research integrity team to safeguard the quality of each article we publish.
Find out more
REVIEW article
Front. Sustain. Food Syst. , 05 January 2024
Sec. Crop Biology and Sustainability
Volume 7 - 2023 | https://doi.org/10.3389/fsufs.2023.1333544
This article is part of the Research Topic Regulation of Ovule and Seed Development View all 5 articles
The life cycles of plants are characterized by significant alternations between the diploid sporophytic and the haploid gametophytic generations. Meiosis and fertilization are the prerequisites for achieving the alternation of generations. Diploid sporophytes undergo meiosis to produce haploid gametes, and male–female gametes fuse (double fertilization) to revert to the diploid sporophyte. The formation and development of male gametophytes are crucial for the alternation of generations in higher plants. During the long evolution of plants from aquatic to terrestrial, the way of sexual reproduction has undergone subversive innovations. From sperm swimming for fertilization to relying on the precise delivery of pollen tubes to female gametes for the fusion of the male–female gametes, higher requirements are placed on the male gametophytes’ development and fertilization process. The formation of male gametophytes has undergone significant changes to meet the needs for delivery and transportation of the male gametes. With the emergence of more and more evidence, the molecular mechanism underlying male gametophytes’ development, especially the initiation and specialization of germline cells has been better understood. Focusing on the latest studies, we reviewed and elucidated the critical proteins and factors involved in male gametophyte formation, highlighting the decisive role of auxin in archesporial cell specialization and the importance of microspore maturation in pre-mitosis, and analyzed the molecular mechanisms underlying male gametogenesis, with a view to providing insights for further exploration of male gametophytes formation in the future.
• We reviewed the latest advance in male gametophyte formation, highlighting the vital discovery of auxin in the specialization of archesporial cells and the importance of microspore maturation for microgametogenesis.
The formation of viable pollen grains is crucial for the double fertilization of angiosperms. In flowering plants, the ontogeny of anther, especially the formation of male gametophytes, is tightly governed, and the processes are highly conservative and programmed. The understanding of male gametophyte development in most angiosperms on genetic and molecular knowledge comes from the model plants Arabidopsis thaliana (Sanders et al., 1999), Oryza sativa (rice) (Zhang et al., 2011), and Zea mays (maize) (van der Linde and Walbot, 2019). Male gametophyte development refers to a series of programmed developmental processes, including the differentiation of male germ cells, the development of microspores, and the formation of male gametophytes. The development of male gametophytes can be divided into two phases according to the morphological changes of tissue and cells. In Arabidopsis, during phase I, composed of flowering stages 1–7, the anther primordium differentiates and divides into archesporial cells to establish the anther morphology (Sanders et al., 1999). The microspore mother cells undergo meiosis to give rise to haploid microspores. During phase II, containing stages 8–14, the anther grows and extends upward through the filaments. Free microspores undergo two rounds of mitosis to produce mature male gametophytes, and anthers dehisce to release pollen grains. About 70% of angiosperms undergo only one mitosis to form bicellular pollen when their pollen grains are released. In the growing pollen tubes, the reproductive cells undergo a second mitosis to form two sperm cells (Brewbaker, 1967). In the anther ontogeny, the specification of pre-meiotic cell fate in germ cells and the differentiation of somatic cell layers surrounding archesporial cells, are essential for male gametocyte formation and development. The formation of male gametophytes is a complicated process that has been accurately monitored in the spatiotemporal window. They undergo two consecutive processes: microsporogenesis and microgametogenesis. Microsporogenesis is the differentiation and development of archesporial cells into microsporocytes. Subsequently, meiosis occurs, and the microsporocyte gives rise to haploid microspores within the microsporangia (or pollen sac). Depending on the species, microgametogenesis refers to the process during which the microspore undergoes one or two mitotic divisions to develop into bicellular or tri-cellular pollen (Brewbaker, 1967).
We will review these two phases of pollen formation, focusing on discoveries in the formation of male gametophytes. Pollen grains that land on the stigma germinate to generate pollen tubes that grow in the pistil. The pollen tube carries two sperm cells and grows toward the ovule, where it fuses with two female gametes to complete double fertilization. Frequent cell–cell communication between the male (pollen or pollen tube) and the female tissues occurs at various stages, such as pollen adhesion and recognition, pollen germination, pollen tube elongation, and fertilization (Yu et al., 2022). Dresselhaus and Franklin-Tong (2013) gave a comprehensive overview (reviewed in Mol Plant), and we would not mention these sections.
The formation of the male gametophyte begins with the differentiation of stamen primordium generated by the floral meristem. The pollen development pattern of Arabidopsis is shown in Figure 1 (Sanders et al., 1999). At stage 1, the anther primordium derived from the division and differentiation of the floral meristem generally contains three cell layers, from outside to inside, the outer epidermis layer (L1), the middle layer (L2), and the inner layer (L3). At stage 2, cell division and proliferation lead to an increase in each layer of cells and expansion of anther primordia. Subsequentially, L1 cells differentiate and form the epidermis layer. L2 cells rapidly divide and develop into archesporial cells and L2-derived cells at four corners of the anther, where four anther lobes will be formed subsequently. In the center of each anther lobes, archesporial cells are surrounded by the somatic cell layer differentiated by L2-derived cells. L3 cells divide and give rise to vascular and connective tissue. At stage 3, archesporial cells undergo periclinal division to produce the outer primary parietal cells and inner primary sporogenous cells, which belong to germline cells. At stage 4, the periclinal division of primary parietal cells gives rise to outer endothecium and inner secondary parietal cells. The secondary parietal cells divide to form the primary tapetum and the middle layer. The primary sporogenous cells divide periclinally again to generate the secondary sporogenous cells. At stage 5, after the formation of the anther walls, the secondary sporogenous cells divide continuously and differentiate into many microsporocytes (microspore mother cells), filling the entire interior of the microsporangium. The diploid microspore mother cells are interconnected, with a prominent feature of a large nucleus located at the center. The four anther lobes each comprise five-layer cells in a pre-meiotic anther. The cells are microspore mother cells, tapetum, middle layer, endothecium, and epidermis from inside to outside. At stage 6, callose deposition occurs to isolate microsporocytes and the microspore mother cells initiate the process of meiosis to produce four haploid microspores embedded by a unique callose envelope. The tapetum undergoes endomitosis and the cytoplasm becomes dense. The volume of the locules becomes large, the middle layer becomes crushed and degenerated, and the cytoplasm of endothecium and epidermal cells almost disappears. The tapetum is tightly associated with the anther locule (Quilichini et al., 2014). At stage 7, after meiosis is completed, and tetrads of microspores are surrounded and separated by the callose wall. The tapetal cells become two-nucleate as a result of endomitosis, with numerous expanded vacuoles (Quilichini et al., 2014). Remnants of the middle layer are present. At stage 8, the surface of the microspore membrane becomes undulating, and the development of primexine initiates between the callose layer and the microspore membrane. The callose surrounding the microspores is degraded by the callase enzyme synthesized and secreted by the tapetal cells. The precursors of sporopollenin secreted by the tapetal cells accumulate at the top of the undulated microspore membrane, forming the bacula and tectum. Subsequently, the microspores are released from the tetrads (Sanders et al., 1999). The exine of microspores has been attached with sporopollenin, lipase, and glycine-rich proteins. Up to the early tricellular stage of pollen development, a large amount of liquid fills the space surrounding the microspore instead of the space between the tapetum and the microspore, as previously thought (Quilichini et al., 2014).
Figure 1. Schematic diagram of anther development stages in Arabidopsis. Schematic diagram of anther development from Stage 1 to Stage 14 in Arabidopsis (Sanders et al., 1999). E, epidermis (orange); En, endothecium (Pale Mauve); M, middle layer (yellow); PMC, pollen mother cell or microsporocyte (light green); T, tapetum (teal); FM, free microspore (light green); M, microspore (light green); PM, polarized microspores (light green); P, pollen (green). Color coding displays cells are identical types at the same stage or derived cells at different stages.
In angiosperms, the development of male gametophytes is uniform and synchronized before the tetrad stage. The sporophyte portion of the stamen primordia, including four anther locules, the central vascular bundle or connective tissue, and the anther wall, presents a butterfly-shaped feature in the transverse section. The four identical lobes, two adaxial and two abaxial, surround the central connective and vascular tissues. However, the stamen lacks meristem, and all parts of the stamen can be traced back to the floral meristem. In Arabidopsis, the formation of the stamen primordia relies on the division activity of the L1, L2, and L3 layers in the floral meristem. In the subsequent patterning establishment of anther primordium, only L1 and L2 meristem layers contribute to forming anther lobes; L3 participates in developing the vascular bundle in filament and connective tissue at the center of each four-lobed anther. In Arabidopsis, during the cell type specialization of anther lobes, L2 cells undergo the periclinal division to form two-layer cells: the outer primary parietal cells and the internal subsets endowed with the fate of archesporial cells. The primary parietal cells carry out the periclinal division, producing the secondary parietal cells and an external subset that subsequently differentiates into the endothelium. The secondary parietal cells divide again to form two-layer cells that differentiate into the tapetum and middle layer, respectively. Except that the epidermis is derived from L1 cells, the other cells result from the development and specialization of L2 cells. The tapetum, middle layer, endothecium, and epidermis cells comprise the locular wall and surround the inner archesporial cell, which develops into microsporocytes. In Arabidopsis, the specialization of early archesporial cells is closely related to the patterning establishment of the anther primordium as shown in Figure 1. It is of great significance to elucidate and understand the molecular mechanisms underlying the initiation, differentiation, and development of the germline cell, archesporial cells.
To the knowledge, most of the previous studies and discoveries have focused on meiosis. The underlying molecular mechanism initiating the specialization of male germline cells from the somatic cell of the floral meristem is not well understood. The latest studies suggested that auxin may play an important role in determining the fate of AR cells during male gametophyte formation. It is proposed that archesporial cell initiation is governed by numerous external and internal factors, among which the phytohormones are the key controllers. In Arabidopsis, the dynamic distribution of auxin in pre-meiotic anther was consistent with the initiation and development of the germ cells (Zheng et al., 2021). In Arabidopsis, detected by immunostaining and biosensor, the auxin was uniformly distributed in three layers of cells of anther primordium at stage 1. From stage 3, the auxin distribution gradually accumulated to the center and formed an inconspicuous auxin gradient. At stage 5, auxin was concentrated in the microsporocytes (microspore mother cells) and an auxin gradient was gradually established in the anther primordium. Auxin signals could hardly be detected in the outer endothecium and epidermis. The local auxin maximum was detected in germ lineage cells compared with the somatic cells from other layers of the anther lobes. With the development and differentiation of anther primordium, auxin was dynamically converged in the germ lineage cells, such as archesporial cells, primary sporogenous cells, and microsporocytes (Figure 2A). These results collectively demonstrate that the local auxin maximum orchestrates the specification of male germline cells, and the auxin gradient governs the stamen primordium formation.
Figure 2. Development of anther primordium and specialization of AR Cells pre-meiosis. (A) With the specialization and development of AR cells in Arabidopsis, auxin gradually accumulates into pollen mother cells (Zheng et al., 2021). (B) The signaling pathways about the specification and initiation of AR cells in Arabidopsis. (C) In rice and maize, PPC cells surrounding AR cells maintain their somatic cell niche.
TRYPTOPHAN AMINOTRANSFERASE OF ARABIDOPSIS 1 (TAA1) and TRYPTOPHAN AMINOTRANSFERASE RELATED2 (TAR2) of Arabidopsis are involved in tryptophan-dependent auxin biosynthesis (Stepanova et al., 2008; Ma et al., 2014). In Arabidopsis, TAA1 and TAR2 are initially expressed in three layers of cells of the floral meristem. With the specialization of the anther primordium, they gradually concentrate in the germline cells, microspore mother cells, at stage 5. The loss function of TAA1/TAR2 showed dysfunction in the initiation of anther primordium. In the abaxial locules of the taa1 tar2-2 weak allele mutant, microsporocytes displayed malformations, and the somatic layer was disordered. Most of the cells in adaxial locules were highly vacuolated. It was suggested that the expression pattern of TAA/TAR2 and auxin’s dynamic distribution were highly consistent and closely correlated with the specialization of the anther primordium. Arabidopsis transcription factor SPOROCYTELESS (SPL) (Yang et al., 1999) is one of the early regulators that is responsible for the differentiation of microspore mother cells and the determinant of sporogenous identity. AGAMOUS directly interacts with the CArG-box-like motif in the 3′ region of the SPL to regulate its expression in Arabidopsis (Ito et al., 2004). However, SPL was downregulated in the taa1 tar2-2 double mutant. The ectopic expression of SPL in archesporial cells partially rescued the sterile phenotype of the taa1 tar2-2 mutant (Zheng et al., 2021). The transcripts of TAA1/TAR2 were over-accumulated in spl mutants. The above results indicated that TAA1/TAR2 directly participates in the local auxin biosynthesis and accumulation, leading to activating SPL expression, which plays a critical role in the initiation and specialization of archesporial cells (Figure 2B). Taken together, SPL/NZZ serves as an adaptor-like transcriptional repressor to precisely inhibit the biosynthesis of auxin through a feedback pathway (Yuan and Sundaresan, 2015; Zhang et al., 2015; Zheng et al., 2021).
Van der Linde and Walbot (2019) speculated that the identity determination of archesporial cells might depend on environmental conditions rather than phytohormones after initiating the development of the stamen primordium (van der Linde and Walbot, 2019). Kelliher and Walbot (2012) found that archesporial cells could be differentiated from hypoxia-treated L1 cells (Kelliher and Walbot, 2012). MALE STERILE CONVERTED ANTHER1 (MSCA1) in Maize, termed glutaredoxin, participates in coordinating hypoxic homeostasis, which triggers the specialization and initiation of archesporial cells in anther lobes (Kelliher and Walbot, 2012). Maize MULTIPLE ARCHESPORIAL CELLS1 (MAC1), encoding a small secreted peptide, is initially expressed throughout the anther lobes and the central connective tissue before AR cells specification. After differentiation, the expression of MAC1 is significantly increasing and is limited to AR and AR-derived cells (Wang et al., 2012). MULTIPLE SPOROCYTE1 (MSP1) is expressed in cells surrounding microsporocytes (Microspore mother cells, MMC) rather than in microsporocytes (male germ lineage cells). MAC1 secreted from archesporial cells or MMC, is recognized by the receptor (MSP1) on the cell membrane of neighboring somatic cells (van der Linde et al., 2018), inhibiting the self-specification toward MMC fate and promoting the establishment and self-renew of somatic cell niche. The loss function of MAC1 resulted in generating excessive archesporial cells and missing the endothelium and the secondary parietal cells (Wang et al., 2012). These data indicated that the MSP1 of somatic cells recognized MAC1 secreted from archesporial cells (or MMC) and mediated cell–cell communication to maintain the specific identity of somatic cells (Figure 2C). Maize MSP1 and MAC1 were proposed to be homologs of rice OsMSP1 (Nonomura et al., 2003) and TDL1A (TAPETUM DETERMINANT1-like 1A) (Zhao et al., 2008), respectively. Similarly, they are homologs of Arabidopsis EMS1/EXS1 (EXCESS MALE SPOROCYTES1/EXTRA SPOROGENOUS CELLS) (Zhao et al., 2002) and TPD1 (TAPETUM DETERMINANT1) (Yang et al., 2003), respectively. Oryza sativa MSP1, as the receptor of MMC-secreted-OsTDL1A, is specifically expressed in somatic cells neighboring MMCs, preventing the excessive somatic cells from differentiating into MMCs (Nonomura et al., 2003; Zhao et al., 2008). OsMSP1-OsTDL1A orchestrates the precise differentiation between MMCs and their neighbors, maintaining the identity specification of somatic cells neighboring MMCs and suppressing the over-proliferation of MMCs (Figure 2C). Analysis of miRNA data in wild-type rice, msp1, and ostdl1a showed that MIR2275 was not expressed in the mutants, resulting in the depletion of 24 nt-phasiRNAs derived from PHAS loci (Phased small interfering RNA (phasiRNA) generating loci, PHAS loci) (Fei et al., 2016). The spatiotemporal profile of Argonaute expression (OsAGO1d, OsAGO2b, and OsAGO18) positively correlated with the stage-specific accumulation of phasiRNA, which is essential for specifying cell fate in the early anther at the appropriate spatiotemporal windows (Fei et al., 2016; Dhaka et al., 2020). These data suggested that MIR2275 and AGOs function downstream of OsMSP1-OsTDL1A to maintain the specific cell identities of anther primordium through small RNA-mediated gene silencing at the pre-meiosis stage. On the other hand, this small RNA-mediated gene silencing was a prerequisite for switching from a mitotic to a meiotic program in MMCs. Rice MEIOSIS ARRESTED AT LEPTOTENE1(MEL1), one of the members of the AGO family, is specifically expressed in MMCs and involved in repressing the somatic cell fate via a siRNA-mediated gene silencing (Nonomura et al., 2007). It is unclear whether the intercellular movement of small interfering RNA (siRNA) contributes to determining the cell identities of anther primordium.
Similarly, the TPD1-EMS1/EXS receptor-ligand pairs regulate the specialization of germ cells and somatic cells during anther pattern development in Arabidopsis. The tpd1, ems1/exs, and serk1 serk2 mutants exhibited the defect of the tapetum and the over-proliferation of MMCs (Zhao et al., 2002; Yang et al., 2003; Albrecht et al., 2005; Colcombet et al., 2005; Chen W. et al., 2019). The EMS1/EXS receptor, localized at the secondary parietal cells, recognizes TPD1 secreted by microsporocytes to be activated, and its kinase activity is enhanced by the trans-phosphorylation with SERK1/SERK2 coreceptors, specifying the tapetum cell fate and limiting the over-production of microsporocytes (Chen W. et al., 2019; Gou and Li, 2020).
In Arabidopsis, ERECTA (ER)/ERECTA-LIKE1 (ERL1)/ERL2 (Hord et al., 2008), BARELY ANY MERISTEM 1/2 (BAM1/2) (Hord et al., 2006), and RECEPTOR-LIKE PROTEIN KINASE2 (RPK2) (Mizuno et al., 2007) have been proposed to participate in the initiation and specification of anther primordium. CLAVATA3-insensitive receptor kinases (CIKs) (Cui et al., 2018) serve as co-receptors and are involved in early cell fate specification of anther primordium orchestrated by BAM1/2 and RPK2. Mitogen-activated protein kinase 3/6 (MPK3/6) (Hord et al., 2008) might function downstream of leucine-rich receptor kinases and co-receptors. It phosphorylates SPL to trigger the formation of archesporial cells and primary parietal cells (Zhao et al., 2017; Cui et al., 2018). In the loss function of the BRASSINAZOLE-RESISTANT1 family bzr-h (bzr hextuple, bzr-h) mutant, the anther primordium had only the L1 layer, failed to generate archesporial cells and hardly detected the expression of SPL (Chen L. G. et al., 2019). However, in the quintuple mutant of the BES1 (BZRs) family mutant, bes1-1 bzr1-1 beh1-1 beh3-1 beh4-1 (qui-1), the increased number of microspore mother cells squeezed or encroached on the space of the tapetum, resulting in the defective or missed tapetal cell layer (Chen W. et al., 2019). These data support that BZRs transcription factors participate in the anther primordium’s cell fate specialization (Figure 2B). So far, the small peptides or ligands, which are perceived by BAM11/2-ER/ERL1/ERL2-RPK2-CIKs receptor/co-receptor complex to coordinate the cell fate specification of the anther primordium, are still mysteries.
Meiosis plays a vital role in maintaining genomic stability and genetic diversity for sexually reproductive organisms (Mercier et al., 2015). Two critical events, meiosis and mitosis, take place in male gametogenesis. Meiosis occurs after the primary sporogenous cells in the anther locules develop into microsporocytes. During microsporogenesis, the diploid microsporocytes undergo one meiosis to generate four haploid microspores. It requires the cell division machinery to be reprogrammed at the entry into meiosis and be paralyzed at the termination of meiosis. Each microspore contains half of the genetic content of microsporocytes, which is attributed to a single duplication of the genome followed by two rounds of chromosome separation in meiosis. Genetic exchange and recombination mainly occur in the first division of meiosis (meiosis I) (Mercier et al., 2015). The prophase I can be divided into leptotene, zygotene, pachytene, diplotene, and diakinesis, according to the dynamic morphology of chromosomes. Nelms and Walbot (2019) used single-cell sequencing and found that during the leptotene and pre-zygotene of meiosis, the transcriptome of microsporocytes underwent two sharp transitions in Zea mays male gametogenesis. These two transcriptome re-organizations are consistent with the meiotic cytological process. During zygotene, programmed double-strand breaks (DSBs) and the homologous chromosome pairing appear, and the synaptonemal complex (SC) forms (Imai et al., 2021). Homologous recombination repairs a subset of DSBs to form the crossovers (CO) that result in the reciprocal exchanges of genetic information between the homologous non-sister chromatids, creating new allelic combinations, and generating genetic diversity (Mercier et al., 2015). However, recombination does not occur randomly on chromosomes, and the numbers of COs on each chromosome are limited. Of course, the prerequisite for recombination is the production of DNA double-strand breaks (DSBs) (Willems and De Veylder, 2022). Mutations of meiosis-related genes and cytokinesis-associated genes will lead to abnormal microspore formation, which ultimately results in pollen abortion.
DNA topoisomerase VI (Topo VI), a highly conservative heterotetramer composed of two TopVIA and two TopVIB subunits (Robert et al., 2016), alters DNA topology structure via catalyzing transient DNA double-strand breaks to form the cleavage complex, guiding other intact DNA strands to pass. Three Spo11/TopVIA homologs and one TopVIB homolog have been studied in Arabidopsis. AtSPO11-1 interacts with AtSOP11-2 to form a heterodimer catalytic core for breaking double-strand DNA, functioning in meiotic recombination (Grelon et al., 2001; Hartung et al., 2007). Arabidopsis MEIOTIC TOPOISOMERASE VIB-LIKE (MTOPVIB) stabilizes the catalytic structure and participates in ATP binding and hydrolysis (Fu et al., 2016; Robert et al., 2016; Vrielynck et al., 2016). AtSOP11-1/2 and TOPVIB are indispensable for relaxing DNA super-helixes, inducing the formation of DNA double-strand breaks and playing essential roles in meiotic recombination (Fu et al., 2016; Robert et al., 2016; Vrielynck et al., 2016). Five Spo11/TopVIA homologs and one TopVIB have been identified in Oryza sativa. In the Ossop11-1, Ossop11-2, Ossop11-4, AtmtopVIB, ZmmtopVIB, and OsmtopVIB mutants, the pairing of homologous chromosomes was blocked, and univalents were randomly arranged in the nuclei of microsporocytes rather than aligned at the metaphase plate. With the asymmetrical separation of chromosomes, aneuploid chromosomes were generated in the aneuploid mono-microspores (Grelon et al., 2001; Hartung et al., 2007; An et al., 2011; Fu et al., 2016; Vrielynck et al., 2016). OsAM1, encoding coiled-coil domain protein, is critical for the leptotene-zygotene transition during rice meiosis. The Osam1 mutant displayed dysfunction in sister chromatid cohesion, homologous recombination, and telomere bouquet formation. The meiosis was arrested at the leptotene stage (Zhang et al., 2015). These findings collectively suggest that topoisomerase and other components involved in DNA replication, recombination, and repair machinery play crucial functions in the meiotic progress (Table 1).
In meiosis, DSBs are induced to occur naturally, and repaired by Nonhomologous End joining (NHEJ), homologous Recombination (HR), and Microhomology mediated End joining (MMEJ). The homologous recombination depends on homologous sequences, including chain invasion of homologous chromatids, and synthesis based on homologous templates, then leading to gene conversion or crossovers formation. Most DSBs are repaired via sister chromatids without the formation of crossovers; crossover occurrence only accounts for an extremely low proportion of DSB repairs (Mercier et al., 2015). Single-strand binding proteins, RecA and DMC1(Disrupted Meiotic cDNA1)–RAD51 (Radiation sensitive 51), mediate the inter invasion of homologous chains to form the crossovers for repairing DSBs (Tarsounas et al., 2004). RAD51D has previously been verified to be critical for the repair of double-strand breaks in mammals (Tarsounas et al., 1999). Rice OsRAD51D combines telomere to inhibit non-homologous linkage and promote homologous pairing and recombination. In the Osrad51d mutants, non-homologous pairing and interaction occurred in the repair of double-strand breaks during meiosis, leading to male sterility (Zhang et al., 2020). Arabidopsis HIGH CROSSOVER RATE1 (HCR1) encoding PROTEIN PHOSPHATASE X1 is a member of the PP4 (Ser/Thr-specific phosphoprotein phosphatase) complex (Nageswaran et al., 2021). HCR1 interacted with crossover-associated protein HEI10 (homologous of human enhancer of invasion 10), PTD (homologous of Par Ting Dancer), MSH5 (homologous of MutSγ), and MLH1(homologous of MutLγ) (Mercier et al., 2015; Pyatnitskaya et al., 2019; Nageswaran et al., 2021; Willems and De Veylder, 2022). HCR1 also interacted with chromosome axis protein, DSB factor, and recombinant enzyme in the Y2H assay (Nageswaran et al., 2021). The loss of HCR1 resulted in enormously increasing numbers of crossovers and significantly decreased the interference intensity of distal euchromatic regions (Nageswaran et al., 2021). It was suggested that the HCR1/PPX1–PP4 phosphatase complex might antagonize or equibalance the function of pre-recombinant kinase to limit the number of crossovers in Arabidopsis. However, the loss of ABERRANT GAMETOGENESIS1 (OsAGG1) led to the reduction of crossovers in meiosis (Chang et al., 2020). A similar phenotype was found in the mutants involved in crossover formation, including ZMM loss-of-function mutants, Osmsh4, Osmsh5, Oszip4, Osrad17, and Oshei10 (Ishimaru et al., 2005; Zhang et al., 2014; Wang et al., 2016; Hu et al., 2018). Rice OsAGG1 could interact with ZMM-associated proteins, including OsHEI10, OsZIP4, and OsMSH5, which were involved in the formation of class I crossover (Chang et al., 2020). In rice, loss of DESYNAPSIS1 (DSNP1), an E3 ubiquitin ligase, generated aberrant synaptonemal complex-like polymers. Loss of ZMM class proteins, including HEI10, ZIP4, and MER3, were reduced for distribution on meiotic chromosomes, resulting in a decrease in the formation of crossovers (Ren et al., 2021). These indicated that DSNP1 inhibited the formation of the synaptonemal complex-like polymer to stabilize homologous chromosome pairing and promote the formation of crossovers (COs) during meiosis (Ren et al., 2021). DSNP1 and OsAGG1 were involved in forming class I COs in rice. How DSNP1 and OsAGG1 coordinate and jointly promote the formation of COs is required to further explore.
Gemini Pollen 3 (GEM3)/AUGMIN subunit 6 (AUG6-1), encoding a conservative component of the AUGMIN complex, mediated AUGMIN-dependent microtubule nucleation (Oh et al., 2016). In the gem3 mutant, the bivalents were scattered at different locations rather than aligned at the metaphase plate. The organization of microtubule nucleation in meiotic spindle formation was defective, resulting in spindle position or structural abnormality (Oh et al., 2016). In soybean, loss of MS1/GmNACK2a resulted in an insufficient phragmoplast expansion to influence the cell plate formation. MS1 interacted with GmMAPKKK to activate microtubule-associated protein 65 (GmMAP65) via the MAPK cascade signaling pathway, promoting the dissolution of overlapping microtubules and recruitment of Golgi-derived vesicles toward the middle region of the phragmoplast to form the new cell plates (Fang et al., 2021). Arabidopsis Callose Defective Microspore1(CDM1), a CCCH-type zinc finger protein, is required for cytokinesis division of meiocytes and plays an important role in the regulation of callose metabolism during microsporogenesis (Lu et al., 2014; Pandey et al., 2021). The assembly of the cytoskeleton and cytokinesis associated with the formation of the cell plate might affect both the meiosis and mitosis processes.
After meiosis, the haploid microspores terminate the progress of meiosis, which involves the synergies of multiple complexes. In Arabidopsis, the previously discovered SUPPRESSOR WITH MORPHOGENETIC EFFECTS ON GENITALIA7 (SMG7) and THREE DIVISIONMUTANT1 (TDM1)/ MS5/POLLENLESS3 play essential functions in the termination and exit of meiosis (Bulankova et al., 2010; Cifuentes et al., 2016). TDM1 is incorporated into PROCESSING BODY (P-bodies) via interaction with SMG7. Similarly, the main translation initiation complex, elF4F, is recruited by TMD1 into TDM1-containing P-bodies to inhibit the translation (Cairo et al., 2022). A suppressor screen to reveal that the inactivity of cyclin-dependent kinase D;3 (CDKD;3) would inhibit the multiple cycles of nuclear division in smg7 mutant (Tanasa et al., 2023). A centromeric histone H3 (CENH3) mutation promotes meiotic exit and partially restores fertility in SMG7-deficient Arabidopsis (Capitao et al., 2021). The reduced level of CENH3 alleviates the SMG-defective phenotype, which displayed excessive chromosome aggregation and spindle assembly, exiting meiosis. Collectively, temporary inhibition of translation governed by TDM1-SMG7-elF4F-containing P bodies downregulates the level of cyclin-dependent kinases and CENH3 and promotes the termination of meiotic processes in Arabidopsis.
Microgametogenesis means the microspore undergoes mitosis to produce the bicellular or tri-cellular pollen grains depending on the species (Shin et al., 2021). In Arabidopsis, the first asymmetric division gives rise to a vegetative and a generative cell with different morphological characteristics and cell fate. The generative cell is enclosed within the vegetative cell, forming a cell-in-celled structure. Subsequently, the generative cell goes through symmetric mitosis to produce two sperm cells with the identity properties. The schematic diagram of Arabidopsis pollen development is shown in Figure 1. At stage 9, the small vesicles aggregate to form vacuoles and surround the central nucleus in free microspores (Sanders et al., 1999). The emergence of baculae and tecta marks the initiation of the microspore exine (Quilichini et al., 2014). The intine wall appears between the exine and the microspore plasma membrane. At stage 10, within the microspore, small vesicles coalesce into a large central vacuole that squeezes the nucleus to the edge. Microspores are highly polarized. The exine wall of the microspore is gradually formed. The sporopollenin polymers consist of a mixture of unbranched amylose monomers and branched amylopectin monomers (Ariizumi and Toriyama, 2011; Lallemand et al., 2013; Ghelli et al., 2023). These sporopollenin precursors are synthesized by ER-related enzymes or cytoplasmic enzymes in the tapetum, secreted into the locules through the secretory pathways or ABC transporter localized on the plasma membrane, reaching the surface of microspores, and finally assembling into the exine wall of microspores (Huang et al., 2013). The cytoplasmic contents fill the entire intracellular space enclosed by the microspore wall. At stage 11, the microspores undergo an asymmetric mitotic division to produce bi-cellular pollen, a smaller germ cell engulfed in the cytoplasm of a larger vegetative cell, forming unique cell-in-celled structures. The bicellular pollen contains many small vesicles, and the large vegetative cell is rich in subcellular organelles such as lipid droplets, mitochondria, and endoplasmic reticulum. The large vacuoles are broken into many small vesicles in the vegetative cell (Bai et al., 2023). The darkly stained organelles appear in tapetum cells, which are likely elaioplasts and tapetosomes in size and shape. The tapetum becomes thin and remains disintegrated during the bicellular pollen. At stage 12, the generative cell undergoes the second mitosis to form two identical sperm cells housed in the vegetative cell. Arabidopsis NOT-LIKE-DAD (NLD) is a phospholipase that is localized to the endo-membrane of vegetative cells to enclose the two sperm cells (Gilles et al., 2021). The nucleus of the vegetative cell keeps close physical contact with two sperm cells for the delivery of sperm cells during fertilization (Lalanne and Twell, 2002; McCue et al., 2011). The tapetal remnants derived from the rupture and degeneration of tapetal cells (mainly composed of lipids and proteins) are gradually deposited on the pollen surface, facilitating the formation of the pollen coat (Quilichini et al., 2015). At stage 13, the pollen coat deposition is completed, and the pollen grains mature after dehydration. Vegetative cells store lipids and carbohydrates during pollen maturation in order to supply the germination and growth of the pollen tube. The anther dehisces and the septal cells rupture to release tri-cellular pollen grains (Zhao and Ma, 2000). The stamens begin to senesce at stage 14, and the anther structures shrink (Sanders et al., 1999).
At pre-mitosis of Arabidopsis, the development and maturity of mononuclear microspores are vital for the microgametogenesis initiated by subsequent mitosis. In Arabidopsis, from the initiation of meiosis, the apoplastic gating structure at the tapetum-middle layer interface appears, which promotes the tapetum and the developing pollen forming the symplastic isolation, separating from other sporophytic tissues (Truskina et al., 2022). The pollen wall is composed of the exine layer and the intine layer. Its formation is an extremely complex process involving various biological events regulated by genes from sporophytes and gametophytes (Ariizumi and Toriyama, 2011). The exine layer is mainly composed of sporopollenin, and its formation is accompanied by the maturation of microspores, containing the development and degradation of the callose wall, the biosynthesis and transport of sporopollenin in the tapetum, the programmed cell death of the tapetum, and the deposition of pollen coat (Shi et al., 2015). The formation mechanism of the inner layer is different from that of the exine layer. The main components of the inner layer are pectin and cellulose, which are synthesized in the microspore and transported to the surface for the assembly of the intine of the microspore wall (Ariizumi and Toriyama, 2011). The active expression of Arabidopsis genes in the generative cells regulates the formation of inner layers (Figure 3).
Figure 3. The signaling pathways involved in the exine formation of pollen. The signaling pathways are involved in the exine formation of pollen in Arabidopsis, which includes the degradation of callose and microspore pectin walls, the deposition of fibrillar materials, the biosynthesis and transport of sporopollenin, and the adhesion of pollen coat proteins (Shi et al., 2021).
In angiosperms, the types and degradation of tapetum exhibit a high degree of diversity, which endows them with various mechanisms for the formation of the exine layer. In Arabidopsis, pollen coat and sporopollenin are secreted by tapetal cells and adhere to the surface of microspores, mediating the exine layer biogenesis. Callose synthesis and punctual degradation are essential for the exine formation of microspore mother cells, microspores, and pollen. On the other hand, the remnants of the degenerated tapetal cells (mainly composed of lipids and proteins) are deposited on the microspores’ surface. They are involved in the formation of the cuticle and the exine layers. During microsporogenesis, microspores are surrounded and separated by the callose wall to form the tetrad structure, the tapetal cells subsequently synthesize and secrete callase (β-1,3-D-glucanase) complex to degrade the callose wall, leading to the release of microspores. It has been previously discovered that rice callose synthase GSL5 (GLUCAN SYNTHASE-LIKE5) (Shi et al., 2015) and UGP1 (UDP-GLUCOSE PYROPHOSPHORYLASE 1) (Chen et al., 2007) is predominately expressed in the meiotic MMCs and participate in the callose deposition of microsporocytes. Arabidopsis CALS5 (CALLOSE SYNTHASE 5) is required for callose formation around developing microspores, which is critical for the exine formation during microgametogenesis (Dong et al., 2005). Arabidopsis KOM (KOMPEITO), localized to the Golgi apparatus, is a member of atypical rhomboid-serine protease that lacked activity. KOM plays an essential role in the regulation of the activity and function of CALS5 in Arabidopsis (Kanaoka et al., 2022). Arabidopsis CALLOSE DEFECTIVE MICROSPORE1 (CDM1) promotes the biogenesis of the exine by regulating the expression of callose synthesis genes (CALS5, CALS12) and callase-related genes (MYB80 and A6). Arabidopsis MYB80/MS188 controls the secretion of the callase by promoting the expression of the A6 in the tapetum (Zhang et al., 2007). MYB80 coordinates the development between the tapetum and microspore, mediating the programmed cell death in the tapetum. The precise coordination of the timing between the degeneration of the tapetum and the development of microspores is critical for male gametogenesis.
In rice, the ETERNAL TAPETUM 1 (EAT1) transcription factor regulates the programmed cell death of the tapetum by activating the aspartic protease of OsAP25 (ASPARTOC PROTEASES 25) and OsAP37 in rice (Niu et al., 2013). Two bHLH transcription factors, TDR (TAPETUM DEGENERATION RETARDATION) and TIP2/bHLH142 (TDR INTERACTING PROTEIN2) could combine and function upstream of EAT1 (Ko et al., 2021). EAT1 was recently found to interact with OsUDT1 (UNDEVELOPED TAPETUM1). The loss of UDT1 resulted in lagging the tapetal degradation and the abortion of microspores (Jung et al., 2005; Ko et al., 2021). During early meiosis, UDT1 directly interacts with TIP2 to form a heterodimer, activating the expression of EAT1 which is enhanced by the dimerization complex of EAT1/UDT1. UDT1 indirectly regulates the expression of TIP2/bHLH142 in the tapetum and meiocytes and plays a role in the meiosis of microspores and the PCD of the tapetum (Ko et al., 2021). However, it has previously been proved that TDR and TIP2 act upstream of EAT1. There should be a feedback cycle pathway between UDT1-EAT1 and TDR-TIP2, it needs solid experimental data to be supported. On the other hand, GIBBERELLIN MYB GENE (GAMYB) specifically binds to the MYB motif in the promoter of TIP2/bHLH142 to regulate the expression of bHLH142 in the tapetum. TDR combines the E-box near the MYB motif in the bHLH142 promoter to repress the module of GAMYB-regulated bHLH142 (Ko et al., 2021). Rice UDT1 and PTC1 (PERSISTENT TAPETAL CELL1) activate GDSL esterase/lipase RMS2 (RICE MALE STERILE 2) to degrade lipids and promote the anther cuticles formation and lipid deposition on the exine layer of microspores (Zhao et al., 2020). These data indicate that TIP2/bHLH142 functions as the hinge, which integrates and orchestrates the UDT1-EAT1 and GAMYB-TDR pathways in Oryza sativa. Finally, they coordinate the meiotic process of microsporocytes, the programmed cell death of the tapetum, and lipid transport, achieving microspore maturity and microsporogenesis.
In Arabidopsis, transcription factor AMS (ABORTED MICROSPORE) regulates membrane transporters IEF (IMPERFECTIVE EXINE FORMATION) and ABCG26 (ATP-BINDING CASSETTE TRANSPORTER G26) that are involved in the transport of sporopollenin/tetraketide sporopollenin precursors, promoting the formation of the exine layer in two parallel pathways (Choi et al., 2011; Lou et al., 2018; Wang et al., 2021). TEX2 (THIN EXINE2) is a nucleotide sugar transporter that transports the sporopollenin precursors for the formation of the primexine and the assembly of the exine structures (Wang and Dobritsa, 2021) in Arabidopsis. Fatty Acid Export 1 (FAX1) exports fatty acids from the tapetum to regulate ROS homeostasis to activate the DYT1-TDF1-AMS-MS188 signaling pathway in the tapetum and is involved in the exine biogenesis of microspores in Arabidopsis (Zhu et al., 2020). Arabidopsis QUARTET 3 (QRT3) encodes a polygalacturonase that plays a critical role in the degradation of the tetrad pectin wall (Shi et al., 2021). In Arabidopsis, the coat protein complex II (COPII) composed of five cytoplasmic components for the transportation of vesicles mediates the anterograde trafficking from the endoplasmic reticulum (ER) to the Golgi apparatus. Sar1, a small G protein, is activated by the guanine nucleotide exchange factor SEC12 anchored in the endoplasmic reticulum. Subsequently, Sar1 assembles Sar1–SEC23–SEC24 by interacting with SEC23 and integrates SEC13-SEC31 via the interaction between SEC23 and SEC31 (Movafeghi et al., 1999; Saito-Nakano and Nakano, 2000; Lederkremer et al., 2001; Futai et al., 2004). Arabidopsis Sar1B/C is involved in the targeted delivery of sporopollenin to the outer surface of microspores (Liang et al., 2020). SEC31A and SEC31B are coat proteins found in COPII vesicles, which promote the formation of larger central vacuoles in microspores (Liu X. et al., 2021). Arabidopsis Hyp-galactosyltransferase (GALT2-GALT9) activates the Hyp-O-galactosylation of Hyp-arabinogalactan (AG). Highly glycosylated arabinogalactan proteins (AGPs) play a crucial role in male gametogenesis. In the galt2galt5galt7galt8galt9 (Hyp-galactosyltransferase) quintuple mutant, mature microspores displayed membrane blebbing, and many lytic vacuoles and their pollen exine and inner membrane layers showed malformations (Kaur et al., 2022). Arabidopsis βVPE (β VACUOLAR PROCESSING ENZYME) is directly activated by MYB2 to play critical roles in the maturation of CEP1, RD19A, and RD19C proteases (Guo et al., 2022). The loss of βVPE resulted in little fibrillar materials attaching to the exine of the microspores, and microspores were shrunken and degraded (Cheng et al., 2020). These data indicate that proteins, which regulate or participate in the secretory/transportation of sporopollenin and lipids, play essential roles in exine biogenesis and pollen coat formation. Proteins involved in the tapetum development and apoptosis are important for the maturity of microspores or pollen (Figure 3).
The pattern formation of the intine is established during the microspore development stage, later than the initiation of the exine layer, and is mainly regulated by the male gametophyte genes. The intine layer of rice pollen is initiated by microspores after releasing it at the later tetrad stage and is predominantly completed at the end of the binuclear pollen stage. Similarly, Arabidopsis thaliana Microgametophyte Defective Mutant 1(AtNMDM1) interacts with AtNMDM2 in pollen nuclei to activate the expression of pollen intine-related Arabinogalactan proteins (AGPs) and pectin methylesterases (PEM), mediating cellulose distribution, and promoting intine layer formation (Mi et al., 2022). These data indicate that the genes derived from germ cells regulate the intine development and the vesicle transport of microspores, and are also crucial for microspore maturity.
Microspore maturation also contains the generation and transport of vesicles within the microspore. In Arabidopsis, the adaptor protein (AP) complex is the major coat protein that connects membrane proteins with the clathrin molecules that form the lipid vesicle coat. Arabidopsis AP1G2 is an adaptor protein complex 1 (AP-1) γ subunit and interacts with PICALM5A/B and PICALM9A/B, mediating endocytosis in transporting insoluble enzymes and membrane proteins from the trans-Golgi network (TGN) to endosomes and lysosomes. In the ap1g2–1+/− mutant, nearly half of the microspores failed to undergo mitosis due to defective polarization (Zhou et al., 2022). Arabidopsis importin β, KETCH1 (karyopherin enabling transport of cytoplasmic HYL1), is located in the nucleus of the reproductive cells at unicellular and bicellular pollen (Xiong et al., 2020). It specifically interacts with RPL27a and other RPs (ribosome proteins) to protect RPs from 26S proteasome-mediated degradation and maintain the nuclear accumulation of RPs. It plays a vital role in ribosome biogenesis and translational efficiency during microspore maturity in Arabidopsis (Xiong et al., 2020). Intracellular membrane fusion undergoes dynamic assembly and disassembly of soluble N-ethylmaleimide-sensitive factor (NSF) attachment protein (SNAP) receptors (SNAREs). Assembly and disassembly of SNARE complexes are essential for maintaining microspore membrane homeostasis (Ma et al., 2021; Rui et al., 2021; Liu X. et al., 2021; Tan et al., 2022). Arabidopsis SEC17/18 promotes the formation of large central vacuoles after the disassembly of the SNAREs (Liu F. et al., 2021). In Arabidopsis asnap-1/+ mutant, the cytoplasmic contents of the mononuclear microspore were leaked, and the internal tissue was disintegrated (Liu F. et al., 2021). The inability of microspores to maturity is prevented from undergoing mitosis. During the development of the microspore, any proteins that participate in its assembly, disassembly, transportation of vesicles, and ribosomal biogenesis will affect the maturity of the microspore, resulting in developmental stagnation before mitosis.
In Arabidopsis, a mature mononuclear microspore characterized by a thick cell wall and uniform dense cytoplasm has a larger nucleus located at the center with small growing vesicles surrounding the central nucleus. Subsequently, the microspore’s volume becomes larger with growth, the scattered small vacuoles in the cytoplasm gather to form a larger vacuole, and the nucleus gradually migrates to the location of the germinal pore at the reproductive pole. The highly polarized microspore undergoes an asymmetric mitosis I (pollen mitosis I, PM I), producing a larger vegetative cell and a smaller generative cell. In approximately 30% of angiosperms, including Arabidopsis, the reproductive cells undergo direct division to produce tri-cellular pollen. In approximately 70% of angiosperms, the division of germ cells is suspended, and pollen remains in the bicellular morphology until germination. In the growing pollen tube, reproductive cells undergo mitosis to form mature tri-cellular pollen (Brewbaker, 1967). Therefore, mitosis is an important process and a distinct mechanism for microgametogenesis.
Gametophyte proteins are essential for vesicle transport, organelle reprogramming, microspore polarization, asymmetric mitotic division, and cytokinesis (Table 2). Arabidopsis MYB81 is a microspore-specific member of the GAMYB transcription factor family and is specifically expressed in the nucleus of mononuclear microspores. In the myb81 mutant, the mononuclear microspore with the large central vacuole is arrested at the microspores polarization stage and fails to enter PM I (Oh et al., 2020). Arabidopsis VACUOLELESS GAMETOPHYTES (VLG) is a cysteine/histidine-rich zinc finger protein and interacts with proteins in multivesicular bodies or pre-vacuolar compartments, mediating a large central vacuolar fusion before microspore polarization (D'Ippolito et al., 2017). Arabidopsis RANs, the small GTPases, play fundamental roles in pollen mitosis I. Both the dominant negative and constitutively active variants of RAN1 can lead to the developmental stagnation of microgametogenesis at PM I (Qin et al., 2022). SEC6, an exocyst subunit of octameric tethered complexes, is involved in vesicle trafficking to promote cell plate formation in pollen mitosis I in Arabidopsis (Tan et al., 2022). The mutation of SEC6 failed to form the cell plate during the mitosis I and caused the accumulation of vesicles in the cytoplasm. The cytokinesis-specific syntaxin KNOLLE failed in being recruited to the cell plate, but was localized in the cytosol (Tan et al., 2022). RAN1 and SEC might facilitate tethering the vesicles before fusion and participate in cell plate formation during cytokinesis in Arabidopsis. The C-terminal R-SNARE domain of Arabidopsis Tomosyn protein (TOMOSYN, AtTMS) bound to SYP1s (SYP111, SYP124, SYP125, SYP131, and SYP132) which are the members of Qa-SNAREs, activating the vesicle secretory and negatively regulating SNARE-dependent membrane fusion (Li et al., 2019). Syntaxin of Plants 31/32 (SYP31/SYP32) interacts with COG3 (Conserved Oligomeric Golgi, COG) localized on Golgi, guiding the transport of SYP31/32 within the Golgi. They might maintain the anterograde and retrograde transport in Golgi-to-ER and be involved in cell plate formation and the deposition of the intine during pollen mitosis I (PM I) in Arabidopsis (Rui et al., 2021). R-SNARE protein YKT61 interacts with many SNAREs derived from different endomembrane components, including the cell plate-associated KNOLLE during PM I (Ma et al., 2021), which is involved in vesicular trafficking to maintain the biogenesis and dynamics of endomembrane compartments in Arabidopsis (Rui et al., 2021). However, in phospholipid metabolism, the production of phosphatidylinositol 3,5-bisphosphate [PI (3,5) P2] is regulated by FAB1A/B/C/D, PI (3,5) P2-converting kinase, and Arabidopsis VAC14 which is a homolog of the yeast and metazoan VAC14s that are responsible for the production of PI (3,5) P2. The mutations of FAB1A/B/C/D and VAC14 resulted in the inability of the central vacuoles to fission into small vesicles after pollen mitosis I in Arabidopsis. These demonstrated that phospholipids, as membrane components, play a crucial role in the dynamic vacuolar organization (Zhang et al., 2018). Arabidopsis PM I is accompanied by the dynamic patterns of vacuolar organization, involving the formation of cell plates, the maintenance of the endomembrane system, and the intra-Golgi trafficking. The deposition of callose is critical for the de novo cell plate formation in mitotic cell division and pollen maturity. In cotton, callose synthase gene GhCalS4/GhCalS8 is generally repressed by transcriptional factor GhWRKY15. After mitosis, pollen-specific SKS-like protein (PSP231) interacted with the RNA-binding protein GhRBPL1 to disarm the repression, promoting pollen maturity (Li et al., 2020). Arabidopsis transcription factor TFIIB-related protein BRP4 mediated the subunit 6 of the Origin Recognition Complex (ORC6) to participate in regulating the PM I cell cycle process (Qin et al., 2014).
During pollen mitosis II (PM II), the generative cell encysted in the vegetative cell undergoes symmetric mitosis to produce two identical sperm cells, forming tricellular pollen in Arabidopsis. Arabidopsis H + -ATPase (AHA) localized on the plasma membrane facilitates H+ efflux depending on the ATP hydrolysis and is essential for maintaining pH homeostasis during PM II (Robertson et al., 2004). CAF-1 (CHROMATIN ASSEMBLY FACTOR 1), a histone chaperone, interacts with replisome and mediates the formation of H3-H4 tetramers through DNA replication-dependent chromatin assembly in Arabidopsis (Chen et al., 2008). Arabidopsis DUO POLLEN1(DUO1) and DUO3 have redundant and distinct molecular mechanisms for regulating generative cell mitosis and sperm cell specification (Brownfield et al., 2009b). The loss of DUO1 and DUO3 led to the failure of germ cells to enter the M phase of cell cycles after undergoing S-phase DNA replication. AtCycB1;1, the G2/M checkpoint protein in the cell-cycle progression, was defective in the duo1 mutant to block generative-cell division. However, another mechanism was suggested to block the generative cell entry into the M phase since AtCycB1;1 was detected in the duo3 mutant. A-type cyclin-dependent kinase;1 (CDKA;1) acts as a crucial regulator of both the G1/S and the G2/M phase transitions of cell cycles, and also plays vital roles in PM II (Brownfield et al., 2009a).
During organismal development, two distinct molecular mechanisms underlying epigenetics coordinate developmental and environmental cues to determine cell fate. They regulate chromatin structure by DNA methylation and histone modification to control gene output and N6A mRNA modification that regulates mRNA metabolism. Epigenetics regulates the cell fate determination of generative and vegetative cells via DNA methylation and covalent modification of histones (Huang and Sun, 2021; Huang and Sun, 2022). Arabidopsis RELATIVE OF EARLY FLOWERING 6 (REF6), a specific lysine(K)-H3K27me3 demethylase, recognizes and binds CTCTGYTY motif, negatively regulating DNA non-CG methylation and playing essential roles in epigenetic reprogramming during male gametogenesis (Huang and Sun, 2021; Pan et al., 2022). When the LAT52 promoter drove it to erase H3K27me3 methylation modifications in the vegetative cell of transgenic Arabidopsis, leading to chromatin remodeling and triggering the identity transition from the vegetative cell to the germline one (He et al., 2022; Pan et al., 2022). It suggests that the H3K27me3 paradigm is critical for determining the identity of vegetative cells during male gametogenesis. Quantitative proteomic analysis of vegetative nuclei (VNs) and germ cell nuclei (GNs) derived from germinated lily pollen have identified 29 transcription factors (TFs) and ten cell fate determinants. The 29 TFs derived from VN are mainly involved in the development of pollen tubes. Ten germ cell-specific TFs primarily participate in cell fate differentiation and male gametogenesis (You et al., 2021). In Arabidopsis pollen grains, the transposable elements (TEs) of sperm cells are methylated to disfunction by the mechanism of RNA-directed DNA methylation (Martinez et al., 2016). The mobile siRNAs derived from pollen vegetative nuclei enhance the transposon methylation of the reproductive cells, resulting in TEs silencing of offspring in an epigenetic inheritance manner (Long et al., 2021). Three excellent reviews highlight how the cell cycle has influenced DNA methylation dynamics during male gametogenesis and shed light on the epigenetic regulation of cell fate (Huang and Sun, 2021; Liu and Wang, 2021; Papareddy and Nodine, 2021).
Male gametophyte development in angiosperm is a complex and continuous biological process. Each critical step is independent and closely related to each other. In the past few years, many functional proteins required for the male developmental program have been found, including those that functioned in microspore and tapetal development, meiosis and mitosis, the dynamic state of callose and vacuole, the specification of cell fate, and the differentiation of cell layers. The proteins involved in male gametophyte formation are classified and reviewed here. However, the molecular mechanism underlying this progress and the regulatory network is required for further elucidation. With gene editing technologies and high-throughput approaches, more and more functional proteins will be discovered during male gametogenesis. In the future, we should integrate molecular biology, biochemistry, and multi-omics techniques to find and elucidate many functional proteins in male gametophyte development. The next challenge is exploring the systematic and high-throughput approaches to construct a more rigorous regulatory network diagram for male gametophytic development.
D-LC: Investigation, Visualization, Writing – original draft. C-XX: Investigation, Resources, Visualization, Writing – original draft. PW: Investigation, Writing – review & editing. T-YG: Funding acquisition, Investigation, Writing – review & editing. BW: Writing – review & editing. T-YY: Conceptualization, Data curation, Funding acquisition, Investigation, Project administration, Resources, Supervision, Visualization, Writing – original draft, Writing – review & editing.
The author(s) declare financial support was received for the research, authorship, and/or publication of this article. This work was supported by the Natural Science Foundation of Shandong (ZR2022MC217), the Yantai University Top Talent Project (2220011), and the Graduate Research Innovation Foundation (GGIFYTU2354).
The authors declare that the research was conducted in the absence of any commercial or financial relationships that could be construed as a potential conflict of interest.
All claims expressed in this article are solely those of the authors and do not necessarily represent those of their affiliated organizations, or those of the publisher, the editors and the reviewers. Any product that may be evaluated in this article, or claim that may be made by its manufacturer, is not guaranteed or endorsed by the publisher.
Albrecht, C., Russinova, E., Hecht, V., Baaijens, E., and de Vries, S. (2005). The Arabidopsis thaliana SOMATIC EMBRYOGENESIS RECEPTOR-LIKE KINASES1 and 2 control male sporogenesis. Plant Cell 17, 3337–3349. doi: 10.1105/tpc.105.036814
An, X. J., Deng, Z. Y., and Wang, T. (2011). OsSpo11-4, a rice homologue of the archaeal TopVIA protein, mediates double-strand DNA cleavage and interacts with OsTopVIB. PLoS One 6:e20327. doi: 10.1371/journal.pone.0020327
Ariizumi, T., and Toriyama, K. (2011). Genetic regulation of sporopollenin synthesis and pollen exine development. Annu. Rev. Plant Biol. 62, 437–460. doi: 10.1146/annurev-arplant-042809-112312
Bai, M., Gao, H., Yang, Y., and Wu, H. (2023). Changes in the content of pollen total lipid and TAG in Arabidopsis thaliana DGAT1 mutant as11. AoB Plants 15, 1–16. doi: 10.1093/aobpla/plad012
Brewbaker, J. L. (1967). The distribution and phylogenetic significance of binucleate and trinucleate pollen grains in the angiosperms. Am. J. Bot. 54, 1069–1083. doi: 10.1002/j.1537-2197.1967.tb10735.x
Brownfield, L., Hafidh, S., Borg, M., Sidorova, A., Mori, T., and Twell, D. (2009a). A plant germline-specific integrator of sperm specification and cell cycle progression. PLoS Genet. 5:e1000430. doi: 10.1371/journal.pgen.1000430
Brownfield, L., Hafidh, S., Durbarry, A., Khatab, H., Sidorova, A., Doerner, P., et al. (2009b). Arabidopsis DUO POLLEN3 is a key regulator of male germline development and embryogenesis. Plant Cell 21, 1940–1956. doi: 10.1105/tpc.109.066373
Bulankova, P., Riehs-Kearnan, N., Nowack, M. K., Schnittger, A., and Riha, K. (2010). Meiotic progression in Arabidopsis is governed by complex regulatory interactions between SMG7, TDM1, and the meiosis I-specific cyclin TAM. Plant Cell 22, 3791–3803. doi: 10.1105/tpc.110.078378
Cairo, A., Vargova, A., Shukla, N., Capitao, C., Mikulkova, P., Valuchova, S., et al. (2022). Meiotic exit in Arabidopsis is driven by P-body-mediated inhibition of translation. Science 377, 629–634. doi: 10.1126/science.abo0904
Capitao, C., Tanasa, S., Fulnecek, J., Raxwal, V. K., Akimcheva, S., Bulankova, P., et al. (2021). A CENH3 mutation promotes meiotic exit and restores fertility in SMG7-deficient Arabidopsis. PLoS Genet. 17:e1009779. doi: 10.1371/journal.pgen.1009779
Chang, Z., Xu, C., Huang, X., Yan, W., Qiu, S., Yuan, S., et al. (2020). The plant-specific ABERRANT GAMETOGENESIS 1 gene is essential for meiosis in rice. J. Exp. Bot. 71, 204–218. doi: 10.1093/jxb/erz441
Chen, L. G., Gao, Z., Zhao, Z., Liu, X., Li, Y., Zhang, Y., et al. (2019). BZR1 Family Transcription Factors Function Redundantly and Indispensably in BR Signaling but Exhibit BRI1-Independent Function in Regulating Anther Development in Arabidopsis. Mol. Plant 12, 1408–1415. doi: 10.1016/j.molp.2019.06.006
Chen, W., Lv, M., Wang, Y., Wang, P. A., Cui, Y., Li, M., et al. (2019). BES1 is activated by EMS1-TPD1-SERK1/2-mediated signaling to control tapetum development in Arabidopsis thaliana. Nat. Commun. 10:4164. doi: 10.1038/s41467-019-12118-4
Chen, Z., Tan, J. L., Ingouff, M., Sundaresan, V., and Berger, F. (2008). Chromatin assembly factor 1 regulates the cell cycle but not cell fate during male gametogenesis in Arabidopsis thaliana. Development 135, 65–73. doi: 10.1242/dev.010108
Chen, R., Zhao, X., Shao, Z., Wei, Z., Wang, Y., Zhu, L., et al. (2007). Rice UDP-glucose pyrophosphorylase1 is essential for pollen callose deposition and its cosuppression results in a new type of thermosensitive genic male sterility. Plant Cell 19, 847–861. doi: 10.1105/tpc.106.044123
Cheng, Z., Guo, X., Zhang, J., Liu, Y., Wang, B., Li, H., et al. (2020). betaVPE is involved in tapetal degradation and pollen development by activating proprotease maturation in Arabidopsis thaliana. J. Exp. Bot. 71, 1943–1955. doi: 10.1093/jxb/erz560
Choi, H., Jin, J. Y., Choi, S., Hwang, J. U., Kim, Y. Y., Suh, M. C., et al. (2011). An ABCG/WBC-type ABC transporter is essential for transport of sporopollenin precursors for exine formation in developing pollen. Plant J. 65, 181–193. doi: 10.1111/j.1365-313X.2010.04412.x
Cifuentes, M., Jolivet, S., Cromer, L., Harashima, H., Bulankova, P., Renne, C., et al. (2016). TDM1 Regulation Determines the Number of Meiotic Divisions. PLoS Genet. 12:e1005856. doi: 10.1371/journal.pgen.1005856
Colcombet, J., Boisson-Dernier, A., Ros-Palau, R., Vera, C. E., and Schroeder, J. I. (2005). Arabidopsis SOMATIC EMBRYOGENESIS RECEPTOR KINASES1 and 2 are essential for tapetum development and microspore maturation. Plant Cell 17, 3350–3361. doi: 10.1105/tpc.105.036731
Cui, Y., Hu, C., Zhu, Y., Cheng, K., Li, X., Wei, Z., et al. (2018). CIK Receptor Kinases Determine Cell Fate Specification during Early Anther Development in Arabidopsis. Plant Cell 30, 2383–2401. doi: 10.1105/tpc.17.00586
Dhaka, N., Sharma, S., Vashisht, I., Kandpal, M., Sharma, M. K., and Sharma, R. (2020). Small RNA profiling from meiotic and post-meiotic anthers reveals prospective miRNA-target modules for engineering male fertility in sorghum. Genomics 112, 1598–1610. doi: 10.1016/j.ygeno.2019.09.009
D'Ippolito, S., Arias, L. A., Casalongue, C. A., Pagnussat, G. C., and Fiol, D. F. (2017). The DC1-domain protein VACUOLELESS GAMETOPHYTES is essential for development of female and male gametophytes in Arabidopsis. Plant J. 90, 261–275. doi: 10.1111/tpj.13486
Dong, X., Hong, Z., Sivaramakrishnan, M., Mahfouz, M., and Verma, D. P. (2005). Callose synthase (CalS5) is required for exine formation during microgametogenesis and for pollen viability in Arabidopsis. Plant J. 42, 315–328. doi: 10.1111/j.1365-313X.2005.02379.x
Dresselhaus, T., and Franklin-Tong, N. (2013). Male-female crosstalk during pollen germination, tube growth and guidance, and double fertilization. Mol. Plant 6, 1018–1036. doi: 10.1093/mp/sst061
Fang, X., Sun, X., Yang, X., Li, Q., Lin, C., Xu, J., et al. (2021). MS1 is essential for male fertility by regulating the microsporocyte cell plate expansion in soybean. Sci. China Life Sci. 64, 1533–1545. doi: 10.1007/s11427-021-1973-0
Fei, Q., Yang, L., Liang, W., Zhang, D., and Meyers, B. C. (2016). Dynamic changes of small RNAs in rice spikelet development reveal specialized reproductive phasiRNA pathways. J. Exp. Bot. 67, 6037–6049. doi: 10.1093/jxb/erw361
Fu, M., Wang, C., Xue, F., Higgins, J., Chen, M., Zhang, D., et al. (2016). The DNA Topoisomerase VI-B Subunit OsMTOPVIB Is Essential for Meiotic Recombination Initiation in Rice. Mol. Plant 9, 1539–1541. doi: 10.1016/j.molp.2016.07.006
Futai, E., Hamamoto, S., Orci, L., and Schekman, R. (2004). GTP/GDP exchange by Sec12p enables COPII vesicle bud formation on synthetic liposomes. EMBO J. 23, 4146–4155. doi: 10.1038/sj.emboj.7600428
Ghelli, R., Brunetti, P., Marzi, D., Cecchetti, V., Costantini, M., Lanzoni-Rossi, M., et al. (2023). The full-length Auxin Response Factor 8 isoform ARF8.1 controls pollen cell wall formation and directly regulates TDF1, AMS and MS188 expression. Plant J. 113, 851–865. doi: 10.1111/tpj.16089
Gilles, L. M., Calhau, A. R. M., La Padula, V., Jacquier, N. M. A., Lionnet, C., Martinant, J. P., et al. (2021). Lipid anchoring and electrostatic interactions target NOT-LIKE-DAD to pollen endo-plasma membrane. J. Cell Biol. 220:e202010077. doi: 10.1083/jcb.202010077
Gou, X., and Li, J. (2020). Paired Receptor and Coreceptor Kinases Perceive Extracellular Signals to Control Plant Development. Plant Physiol. 182, 1667–1681. doi: 10.1104/pp.19.01343
Grelon, M., Vezon, D., Gendrot, G., and Pelletier, G. (2001). AtSPO11-1 is necessary for efficient meiotic recombination in plants. EMBO J. 20, 589–600. doi: 10.1093/emboj/20.3.589
Guo, X., Li, L., Liu, X., Zhang, C., Yao, X., Xun, Z., et al. (2022). MYB2 Is Important for Tapetal PCD and Pollen Development by Directly Activating Protease Expression in Arabidopsis. Int. J. Mol. Sci. 23:3563. doi: 10.3390/ijms23073563
Hartung, F., Wurz-Wildersinn, R., Fuchs, J., Schubert, I., Suer, S., and Puchta, H. (2007). The catalytically active tyrosine residues of both SPO11-1 and SPO11-2 are required for meiotic double-strand break induction in Arabidopsis. Plant Cell 19, 3090–3099. doi: 10.1105/tpc.107.054817
He, K., Mei, H., Zhu, J., Qiu, Q., Cao, X., and Deng, X. (2022). The histone H3K27 demethylase REF6/JMJ12 promotes thermomorphogenesis in Arabidopsis. Natl. Sci. Rev. 9:nwab213. doi: 10.1093/nsr/nwab213
Hord, C. L., Chen, C., Deyoung, B. J., Clark, S. E., and Ma, H. (2006). The BAM1/BAM2 receptor-like kinases are important regulators of Arabidopsis early anther development. Plant Cell 18, 1667–1680. doi: 10.1105/tpc.105.036871
Hord, C. L., Sun, Y. J., Pillitteri, L. J., Torii, K. U., Wang, H., Zhang, S., et al. (2008). Regulation of Arabidopsis early anther development by the mitogen-activated protein kinases, MPK3 and MPK6, and the ERECTA and related receptor-like kinases. Mol. Plant 1, 645–658. doi: 10.1093/mp/ssn029
Hu, Q., Zhang, C., Xue, Z., Ma, L., Liu, W., Shen, Y., et al. (2018). OsRAD17 Is Required for Meiotic Double-Strand Break Repair and Plays a Redundant Role With OsZIP4 in Synaptonemal Complex Assembly. Front. Plant Sci. 9:1236. doi: 10.3389/fpls.2018.01236
Huang, M. D., Chen, T. L., and Huang, A. H. (2013). Abundant type III lipid transfer proteins in Arabidopsis tapetum are secreted to the locule and become a constituent of the pollen exine. Plant Physiol. 163, 1218–1229. doi: 10.1104/pp.113.225706
Huang, X., and Sun, M.-X. (2021). Epigenetic regulation and intercellular communication during male gametophyte development. J. Plant Physiol. 258-259:153391. doi: 10.1016/j.jplph.2021.153391
Huang, X., and Sun, M. X. (2022). H3K27 methylation regulates the fate of two cell lineages in male gametophytes. Plant Cell 34, 2989–3005. doi: 10.1093/plcell/koac136
Imai, Y., Saito, K., Takemoto, K., Velilla, F., Kawasaki, T., Ishiguro, K., et al. (2021). Sycp1 Is Not Required for Subtelomeric DNA Double-Strand Breaks but Is Required for Homologous Alignment in Zebrafish Spermatocytes. Front. Cell Dev. Biol. 9:664377. doi: 10.3389/fcell.2021.664377
Ishimaru, Y., Suzuki, M., Kobayashi, T., Takahashi, M., Nakanishi, H., Mori, S., et al. (2005). OsZIP4, a novel zinc-regulated zinc transporter in rice. J. Exp. Bot. 56, 3207–3214. doi: 10.1093/jxb/eri317
Ito, T., Wellmer, F., Yu, H., Das, P., Ito, N., Alves-Ferreira, M., et al. (2004). The homeotic protein AGAMOUS controls microsporogenesis by regulation of SPOROCYTELESS. Nature 430, 356–360. doi: 10.1038/nature02733
Jung, K. H., Han, M. J., Lee, Y. S., Kim, Y. W., Hwang, I., Kim, M. J., et al. (2005). Rice Undeveloped Tapetum1 is a major regulator of early tapetum development. Plant Cell 17, 2705–2722. doi: 10.1105/tpc.105.034090
Kanaoka, M. M., Shimizu, K. K., Xie, B., Urban, S., Freeman, M., Hong, Z., et al. (2022). KOMPEITO, an Atypical Arabidopsis Rhomboid-Related Gene, Is Required for Callose Accumulation and Pollen Wall Development. Int. J. Mol. Sci. 23:5959. doi: 10.3390/ijms23115959
Kaur, D., Moreira, D., Coimbra, S., and Showalter, A. M. (2022). Hydroxyproline-O-Galactosyltransferases Synthesizing Type II Arabinogalactans Are Essential for Male Gametophytic Development in Arabidopsis. Front. Plant Sci. 13:935413. doi: 10.3389/fpls.2022.935413
Kelliher, T., and Walbot, V. (2012). Hypoxia triggers meiotic fate acquisition in maize. Science 337, 345–348. doi: 10.1126/science.1220080
Ko, S. S., Li, M. J., Ho, Y. C., Yu, C. P., Yang, T. T., Lin, Y. J., et al. (2021). Rice transcription factor GAMYB modulates bHLH142 and is homeostatically regulated by TDR during anther tapetal and pollen development. J. Exp. Bot. 72, 4888–4903. doi: 10.1093/jxb/erab190
Lalanne, E., and Twell, D. (2002). Genetic control of male germ unit organization in Arabidopsis. Plant Physiol. 129, 865–875. doi: 10.1104/pp.003301
Lallemand, B., Erhardt, M., Heitz, T., and Legrand, M. (2013). Sporopollenin biosynthetic enzymes interact and constitute a metabolon localized to the endoplasmic reticulum of tapetum cells. Plant Physiol. 162, 616–625. doi: 10.1104/pp.112.213124
Lederkremer, G. Z., Cheng, Y., Petre, B. M., Vogan, E., Springer, S., Schekman, R., et al. (2001). Structure of the Sec23p/24p and Sec13p/31p complexes of COPII. Proc. Natl. Acad. Sci. U. S. A. 98, 10704–10709. doi: 10.1073/pnas.191359398
Li, B., Li, Y., Liu, F., Tan, X., Rui, Q., Tong, Y., et al. (2019). Overexpressed Tomosyn Binds Syntaxins and Blocks Secretion during Pollen Development. Plant Physiol. 181, 1114–1126. doi: 10.1104/pp.19.00965
Li, Y., Li, L., Wang, Y., Wang, Y. C., Wang, N. N., Lu, R., et al. (2020). Pollen-Specific Protein PSP231 Activates Callose Synthesis to Govern Male Gametogenesis and Pollen Germination. Plant Physiol. 184, 1024–1041. doi: 10.1104/pp.20.00297
Liang, X., Li, S. W., Gong, L. M., Li, S., and Zhang, Y. (2020). COPII Components Sar1b and Sar1c Play Distinct Yet Interchangeable Roles in Pollen Development. Plant Physiol. 183, 974–985. doi: 10.1104/pp.20.00159
Liu, F., Li, J. P., Li, L. S., Liu, Q., Li, S. W., Song, M. L., et al. (2021). The canonical alpha-SNAP is essential for gametophytic development in Arabidopsis. PLoS Genet. 17:e1009505. doi: 10.1371/journal.pgen.1009505
Liu, X., Tong, M., Zhang, A., Liu, M., Zhao, B., Liu, Z., et al. (2021). COPII genes SEC31A/B are essential for gametogenesis and interchangeable in pollen development in Arabidopsis. Plant J. 105, 1600–1614. doi: 10.1111/tpj.15136
Liu, L., and Wang, T. (2021). Male gametophyte development in flowering plants: A story of quarantine and sacrifice. J. Plant Physiol. 258-259:153365. doi: 10.1016/j.jplph.2021.153365
Long, J., Walker, J., She, W., Aldridge, B., Gao, H., Deans, S., et al. (2021). Nurse cell--derived small RNAs define paternal epigenetic inheritance in Arabidopsis. Science 373:eabh0556. doi: 10.1126/science.abh0556
Lou, Y., Zhou, H. S., Han, Y., Zeng, Q. Y., Zhu, J., and Yang, Z. N. (2018). Positive regulation of AMS by TDF1 and the formation of a TDF1-AMS complex are required for anther development in Arabidopsis thaliana. New Phytol. 217, 378–391. doi: 10.1111/nph.14790
Lu, P., Chai, M., Yang, J., Ning, G., Wang, G., and Ma, H. (2014). The Arabidopsis CALLOSE DEFECTIVE MICROSPORE1 gene is required for male fertility through regulating callose metabolism during microsporogenesis. Plant Physiol. 164, 1893–1904. doi: 10.1104/pp.113.233387
Ma, T., Li, E., Li, L. S., Li, S., and Zhang, Y. (2021). The Arabidopsis R-SNARE protein YKT61 is essential for gametophyte development. J. Integr. Plant Biol. 63, 676–694. doi: 10.1111/jipb.13017
Ma, W., Li, J., Qu, B., He, X., Zhao, X., Li, B., et al. (2014). Auxin biosynthetic gene TAR2 is involved in low nitrogen mediated reprogramming of root architecture in Arabidopsis. Plant J. 78, 70–79. doi: 10.1111/tpj.12448
Martinez, G., Panda, K., Kohler, C., and Slotkin, R. K. (2016). Silencing in sperm cells is directed by RNA movement from the surrounding nurse cell. Nat Plants 2:16030. doi: 10.1038/nplants.2016.30
McCue, A. D., Cresti, M., Feijó, J. A., and Slotkin, R. K. (2011). Cytoplasmic connection of sperm cells to the pollen vegetative cell nucleus: potential roles of the male germ unit revisited. J. Exp. Bot. 62, 1621–1631. doi: 10.1093/jxb/err032
Mercier, R., Mezard, C., Jenczewski, E., Macaisne, N., and Grelon, M. (2015). The molecular biology of meiosis in plants. Annu. Rev. Plant Biol. 66, 297–327. doi: 10.1146/annurev-arplant-050213-035923
Mi, L., Mo, A., Yang, J., Liu, H., Ren, D., Chen, W., et al. (2022). Arabidopsis novel microgametophyte defective mutant 1 is required for pollen viability via influencing intine development in Arabidopsis. Front. Plant Sci. 13:814870. doi: 10.3389/fpls.2022.814870
Mizuno, S., Osakabe, Y., Maruyama, K., Ito, T., Osakabe, K., Sato, T., et al. (2007). Receptor-like protein kinase 2 (RPK 2) is a novel factor controlling anther development in Arabidopsis thaliana. Plant J. 50, 751–766. doi: 10.1111/j.1365-313X.2007.03083.x
Movafeghi, A., Happel, N., Pimpl, P., Tai, G. H., and Robinson, D. G. (1999). Arabidopsis Sec21p and Sec23p homologs. Probable coat proteins of plant COP-coated vesicles. Plant Physiol. 119, 1437–1446. doi: 10.1104/pp.119.4.1437
Nageswaran, D. C., Kim, J., Lambing, C., Kim, J., Park, J., Kim, E. J., et al. (2021). HIGH CROSSOVER RATE1 encodes PROTEIN PHOSPHATASE X1 and restricts meiotic crossovers in Arabidopsis. Nat Plants 7, 452–467. doi: 10.1038/s41477-021-00889-y
Nelms, B., and Walbot, V. (2019). Defining the developmental program leading to meiosis in maize. Science 364, 52–56. doi: 10.1126/science.aav6428
Niu, N., Liang, W., Yang, X., Jin, W., Wilson, Z. A., Hu, J., et al. (2013). EAT1 promotes tapetal cell death by regulating aspartic proteases during male reproductive development in rice. Nat. Commun. 4:1445. doi: 10.1038/ncomms2396
Nonomura, K., Miyoshi, K., Eiguchi, M., Suzuki, T., Miyao, A., Hirochika, H., et al. (2003). The MSP1 gene is necessary to restrict the number of cells entering into male and female sporogenesis and to initiate anther wall formation in rice. Plant Cell 15, 1728–1739. doi: 10.1105/tpc.012401
Nonomura, K., Morohoshi, A., Nakano, M., Eiguchi, M., Miyao, A., Hirochika, H., et al. (2007). A germ cell specific gene of the ARGONAUTE family is essential for the progression of premeiotic mitosis and meiosis during sporogenesis in rice. Plant Cell 19, 2583–2594. doi: 10.1105/tpc.107.053199
Oh, S. A., Hoai, T. N. T., Park, H. J., Zhao, M., Twell, D., Honys, D., et al. (2020). MYB81, a microspore-specific GAMYB transcription factor, promotes pollen mitosis I and cell lineage formation in Arabidopsis. Plant J. 101, 590–603. doi: 10.1111/tpj.14564
Oh, S. A., Jeon, J., Park, H. J., Grini, P. E., Twell, D., and Park, S. K. (2016). Analysis of gemini pollen 3 mutant suggests a broad function of AUGMIN in microtubule organization during sexual reproduction in Arabidopsis. Plant J. 87, 188–201. doi: 10.1111/tpj.13192
Pan, J., Zhang, H., Zhan, Z., Zhao, T., and Jiang, D. (2022). A REF6-dependent H3K27me3-depleted state facilitates gene activation during germination in Arabidopsis. J. Genet. Genom. 50, 178–191. doi: 10.1016/j.jgg.2022.09.001
Pandey, S., Moradi, A. B., Dovzhenko, O., Touraev, A., Palme, K., and Welsch, R. (2021). Molecular Control of Sporophyte-Gametophyte Ontogeny and Transition in Plants. Front. Plant Sci. 12:789789. doi: 10.3389/fpls.2021.789789
Papareddy, R. K., and Nodine, M. D. (2021). Plant Epigenetics: Propelling DNA Methylation Variation across the Cell Cycle. Curr. Biol. 31, R129–R131. doi: 10.1016/j.cub.2020.11.049
Pyatnitskaya, A., Borde, V., and De Muyt, A. (2019). Crossing and zipping: molecular duties of the ZMM proteins in meiosis. Chromosoma 128, 181–198. doi: 10.1007/s00412-019-00714-8
Qin, Z., Wu, Y. N., Sun, T. T., Ma, T., Xu, M., Pang, C., et al. (2022). Arabidopsis RAN GTPases are critical for mitosis during male and female gametogenesis. FEBS Lett. 596, 1892–1903. doi: 10.1002/1873-3468.14422
Qin, Z., Zhang, X., Zhang, X., Xin, W., Li, J., and Hu, Y. (2014). The Arabidopsis transcription factor IIB-related protein BRP4 is involved in the regulation of mitotic cell-cycle progression during male gametogenesis. J. Exp. Bot. 65, 2521–2531. doi: 10.1093/jxb/eru140
Quilichini, T. D., Douglas, C. J., and Samuels, A. L. (2014). New views of tapetum ultrastructure and pollen exine development in Arabidopsis thaliana. Ann. Bot. 114, 1189–1201. doi: 10.1093/aob/mcu042
Quilichini, T. D., Grienenberger, E., and Douglas, C. J. (2015). The biosynthesis, composition and assembly of the outer pollen wall: A tough case to crack. Phytochemistry 113, 170–182. doi: 10.1016/j.phytochem.2014.05.002
Ren, L., Zhao, T., Zhao, Y., Du, G., Yang, S., Mu, N., et al. (2021). The E3 ubiquitin ligase DESYNAPSIS1 regulates synapsis and recombination in rice meiosis. Cell Rep. 37:109941. doi: 10.1016/j.celrep.2021.109941
Robert, T., Nore, A., Brun, C., Maffre, C., Crimi, B., Bourbon, H. M., et al. (2016). The TopoVIB-Like protein family is required for meiotic DNA double-strand break formation. Science 351, 943–949. doi: 10.1126/science.aad5309
Robertson, W. R., Clark, K., Young, J. C., and Sussman, M. R. (2004). An Arabidopsis thaliana plasma membrane proton pump is essential for pollen development. Genetics 168, 1677–1687. doi: 10.1534/genetics.104.032326
Rui, Q., Tan, X., Liu, F., Li, Y., Liu, X., Li, B., et al. (2021). Syntaxin of plants31 (SYP31) and SYP32 is essential for Golgi morphology maintenance and pollen development. Plant Physiol. 186, 330–343. doi: 10.1093/plphys/kiab049
Saito-Nakano, Y., and Nakano, A. (2000). Sed4p functions as a positive regulator of Sar1p probably through inhibition of the GTPase activation by Sec23p. Genes Cells 5, 1039–1048. doi: 10.1046/j.1365-2443.2000.00391.x
Sanders, P. M., Bui, A. Q., Weterings, K., McIntire, K. N., Hsu, Y.-C., Lee, P. Y., et al. (1999). Anther developmental defects in Arabidopsis thaliana male-sterile mutants. Sex. Plant Reprod. 11, 297–322. doi: 10.1007/s004970050158
Shi, Q. S., Lou, Y., Shen, S. Y., Wang, S. H., Zhou, L., Wang, J. J., et al. (2021). A cellular mechanism underlying the restoration of thermo/photoperiod-sensitive genic male sterility. Mol. Plant 14, 2104–2114. doi: 10.1016/j.molp.2021.08.019
Shi, X., Sun, X., Zhang, Z., Feng, D., Zhang, Q., Han, L., et al. (2015). GLUCAN SYNTHASE-LIKE 5 (GSL5) plays an essential role in male fertility by regulating callose metabolism during microsporogenesis in rice. Plant Cell Physiol. 56, 497–509. doi: 10.1093/pcp/pcu193
Shin, J. M., Yuan, L., Ohme-Takagi, M., and Kawashima, T. (2021). Cellular dynamics of double fertilization and early embryogenesis in flowering plants. J. Exp. Zool. B Mol. Dev. Evol. 336, 642–651. doi: 10.1002/jez.b.22981
Stepanova, A. N., Robertson-Hoyt, J., Yun, J., Benavente, L. M., Xie, D. Y., Dolezal, K., et al. (2008). TAA1-Mediated Auxin Biosynthesis Is Essential for Hormone Crosstalk and Plant Development. Cells 133, 177–191. doi: 10.1016/j.cell.2008.01.047
Tan, X., Xu, H., Ye, J., Wang, J., Liu, W., Liu, F., et al. (2022). Arabidopsis exocyst subunit SEC6 is involved in cell plate formation during Microgametogenesis. Biochem. Biophys. Res. Commun. 598, 100–106. doi: 10.1016/j.bbrc.2022.01.092
Tanasa, S., Shukla, N., Cairo, A., Ganji, R. S., Mikulkova, P., Valuchova, S., et al. (2023). A complex role of Arabidopsis CDKD;3 in meiotic progression and cytokinesis. Plant Direct 7:e477. doi: 10.1002/pld3.477
Tarsounas, M., Morita, T., Pearlman, R. E., and Moens, P. B. (1999). RAD51 and DMC1 form mixed complexes associated with mouse meiotic chromosome cores and synaptonemal complexes. J. Cell Biol. 147, 207–219.
Tarsounas, M., Munoz, P., Claas, A., Smiraldo, P. G., Pittman, D. L., Blasco, M. A., et al. (2004). Telomere maintenance requires the RAD51D recombination/repair protein. Cells 117, 337–347. doi: 10.1016/s0092-8674(04)00337-x
Truskina, J., Boeuf, S., Renard, J., Andersen, T. G., Geldner, N., and Ingram, G. (2022). Anther development in Arabidopsis thaliana involves symplastic isolation and apoplastic gating of the tapetum-middle layer interface. Development 149:dev200596. doi: 10.1242/dev.200596
van der Linde, K., Timofejeva, L., Egger, R. L., Ilau, B., Hammond, R., Teng, C., et al. (2018). Pathogen Trojan Horse Delivers Bioactive Host Protein to Alter Maize Anther Cell Behavior in Situ. Plant Cell 30, 528–542. doi: 10.1105/tpc.17.00238
van der Linde, K., and Walbot, V. (2019). Pre-meiotic anther development. Curr. Top. Dev. Biol. 131, 239–256. doi: 10.1016/bs.ctdb.2018.11.001
Vrielynck, N., Chambon, A., Vezon, D., Pereira, L., Chelysheva, L., De Muyt, A., et al. (2016). A DNA topoisomerase VI-like complex initiates meiotic recombination. Science 351, 939–943. doi: 10.1126/science.aad5196
Wang, R., and Dobritsa, A. A. (2021). Loss of THIN EXINE2 disrupts multiple processes in the mechanism of pollen exine formation. Plant Physiol. 187, 133–157. doi: 10.1093/plphys/kiab244
Wang, H., Hu, Q., Tang, D., Liu, X., Du, G., Shen, Y., et al. (2016). OsDMC1 Is Not Required for Homologous Pairing in Rice Meiosis. Plant Physiol. 171, 230–241. doi: 10.1104/pp.16.00167
Wang, C. J., Nan, G. L., Kelliher, T., Timofejeva, L., Vernoud, V., Golubovskaya, I. N., et al. (2012). Maize multiple archesporial cells 1 (mac1), an ortholog of rice TDL1A, modulates cell proliferation and identity in early anther development. Development 139, 2594–2603. doi: 10.1242/dev.077891
Wang, K., Zhao, X., Pang, C., Zhou, S., Qian, X., Tang, N., et al. (2021). IMPERFECTIVE EXINE FORMATION (IEF) is required for exine formation and male fertility in Arabidopsis. Plant Mol. Biol. 105, 625–635. doi: 10.1007/s11103-020-01114-8
Willems, A., and De Veylder, L. (2022). The Plant Anaphase-Promoting Complex/Cyclosome. Annu. Rev. Cell Dev. Biol. 38, 25–48. doi: 10.1146/annurev-cellbio-120420-092421
Xiong, F., Duan, C. Y., Liu, H. H., Wu, J. H., Zhang, Z. H., Li, S., et al. (2020). Arabidopsis KETCH1 Is Critical for the Nuclear Accumulation of Ribosomal Proteins and Gametogenesis. Plant Cell 32, 1270–1284. doi: 10.1105/tpc.19.00791
Yang, S. L., Xie, L. F., Mao, H. Z., Puah, C. S., Yang, W. C., Jiang, L., et al. (2003). Tapetum determinant1 is required for cell specialization in the Arabidopsis anther. Plant Cell 15, 2792–2804. doi: 10.1105/tpc.016618
Yang, W. C., Ye, D., Xu, J., and Sundaresan, V. (1999). The SPOROCYTELESS gene of Arabidopsis is required for initiation of sporogenesis and encodes a novel nuclear protein. Genes Dev. 13, 2108–2117. doi: 10.1101/gad.13.16.2108
You, C., Zhang, Y., Yang, S., Wang, X., Yao, W., Jin, W., et al. (2021). Proteomic Analysis of Generative and Vegetative Nuclei Reveals Molecular Characteristics of Pollen Cell Differentiation in Lily. Front. Plant Sci. 12:641517. doi: 10.3389/fpls.2021.641517
Yu, T. Y., Xu, C. X., Li, W. J., and Wang, B. (2022). Peptides/receptors signaling during plant fertilization. Front. Plant Sci. 13:1090836. doi: 10.3389/fpls.2022.1090836
Yuan, L., and Sundaresan, V. (2015). Spore formation in plants: sporocyteless and more. Cell Res. 25, 7–8. doi: 10.1038/cr.2014.164
Zhang, W. T., Li, E., Guo, Y. K., Yu, S. X., Wan, Z. Y., Ma, T., et al. (2018). Arabidopsis VAC14 Is Critical for Pollen Development through Mediating Vacuolar Organization. Plant Physiol. 177, 1529–1538. doi: 10.1104/pp.18.00495
Zhang, D., Luo, X., and Zhu, L. (2011). Cytological analysis and genetic control of rice anther development. J. Genet. Genomics 38, 379–390. doi: 10.1016/j.jgg.2011.08.001
Zhang, F., Shen, Y., Miao, C., Cao, Y., Shi, W., Du, G., et al. (2020). OsRAD51D promotes homologous pairing and recombination by preventing nonhomologous interactions in rice meiosis. New Phytol. 227, 824–839. doi: 10.1111/nph.16595
Zhang, L., Tang, D., Luo, Q., Chen, X., Wang, H., Li, Y., et al. (2014). Crossover formation during rice meiosis relies on interaction of OsMSH4 and OsMSH5. Genetics 198, 1447–1456. doi: 10.1534/genetics.114.168732
Zhang, B., Xu, M., Bian, S., Hou, L., Tang, D., Li, Y., et al. (2015). Global Identification of Genes Specific for Rice Meiosis. PLoS One 10:e0137399. doi: 10.1371/journal.pone.0137399
Zhang, Z. B., Zhu, J., Gao, J. F., Wang, C., Li, H., Li, H., et al. (2007). Transcription factor AtMYB103 is required for anther development by regulating tapetum development, callose dissolution and exine formation in Arabidopsis. Plant J. 52, 528–538. doi: 10.1111/j.1365-313X.2007.03254.x
Zhao, X., de Palma, J., Oane, R., Gamuyao, R., Luo, M., Chaudhury, A., et al. (2008). OsTDL1A binds to the LRR domain of rice receptor kinase MSP1, and is required to limit sporocyte numbers. Plant J. 54, 375–387. doi: 10.1111/j.1365-313X.2008.03426.x
Zhao, J., Long, T., Wang, Y., Tong, X., Tang, J., Li, J., et al. (2020). RMS2 Encoding a GDSL Lipase Mediates Lipid Homeostasis in Anthers to Determine Rice Male Fertility. Plant Physiol. 182, 2047–2064. doi: 10.1104/pp.19.01487
Zhao, D. Z., and Ma, H. (2000). Male fertility: A case of enzyme identity. Curr. Biol. 10, R904–R907. doi: 10.1016/s0960-9822(00)00848-4
Zhao, D. Z., Wang, G. F., Speal, B., and Ma, H. (2002). The excess microsporocytes1 gene encodes a putative leucine-rich repeat receptor protein kinase that controls somatic and reproductive cell fates in the Arabidopsis anther. Genes Dev. 16, 2021–2031. doi: 10.1101/gad.997902
Zhao, F., Zheng, Y. F., Zeng, T., Sun, R., Yang, J. Y., Li, Y., et al. (2017). Phosphorylation of SPOROCYTELESS/NOZZLE by the MPK3/6 Kinase Is Required for Anther Development. Plant Physiol. 173, 2265–2277. doi: 10.1104/pp.16.01765
Zheng, Y., Wang, D., Ye, S., Chen, W., Li, G., Xu, Z., et al. (2021). Auxin guides germ-cell specification in Arabidopsis anthers. Proc. Natl. Acad. Sci. U. S. A. 118:e2101492118. doi: 10.1073/pnas.2101492118
Zhou, Y., Fang, W., Pang, Z., Chen, L. Y., Cai, H., Ain, N. U., et al. (2022). AP1G2 Affects Mitotic Cycles of Female and Male Gametophytes in Arabidopsis. Front. Plant Sci. 13:924417. doi: 10.3389/fpls.2022.924417
Keywords: male gametogenesis, meiosis, microgametogenesis, microsporogenesis, mitosis
Citation: Cui D-L, Xu C-X, Wang P, Gao T-Y, Wang B and Yu T-Y (2024) Male gametogenesis in flowering plants. Front. Sustain. Food Syst. 7:1333544. doi: 10.3389/fsufs.2023.1333544
Received: 05 November 2023; Accepted: 07 December 2023;
Published: 05 January 2024.
Edited by:
Prakash Babu Adhikari, Nagoya University, JapanReviewed by:
Jorge Lora, Spanish National Research Council (CSIC), SpainCopyright © 2024 Cui, Xu, Wang, Gao, Wang and Yu. This is an open-access article distributed under the terms of the Creative Commons Attribution License (CC BY). The use, distribution or reproduction in other forums is permitted, provided the original author(s) and the copyright owner(s) are credited and that the original publication in this journal is cited, in accordance with accepted academic practice. No use, distribution or reproduction is permitted which does not comply with these terms.
*Correspondence: Tian-Ying Yu, dHl5dUB5dHUuZWR1LmNu
†These authors have contributed equally to this work
Disclaimer: All claims expressed in this article are solely those of the authors and do not necessarily represent those of their affiliated organizations, or those of the publisher, the editors and the reviewers. Any product that may be evaluated in this article or claim that may be made by its manufacturer is not guaranteed or endorsed by the publisher.
Research integrity at Frontiers
Learn more about the work of our research integrity team to safeguard the quality of each article we publish.