- 1Faculty of Agriculture and Life Science, Hirosaki University, Hirosaki, Japan
- 2The United Graduate School of Agricultural Sciences – Iwate University, Morioka, Japan
- 3Graduate School of Fisheries Sciences, Hokkaido University, Hakodate, Japan
- 4School of Pharmaceutical Sciences, Health Sciences University of Hokkaido, Tobetsu-cho Ishikari-gun, Japan
- 5Advanced Research Promotion Center, Health Sciences University of Hokkaido, Tobetsu-cho Ishikari-gun, Japan
Ishimozuku (Sphaerotrichia firma) is one species of edible brown algae in Japan. The lipids contain rich of fucoxanthin and n-3 unsaturated fatty acids. This study analyzed dietary Ishimozuku lipid components and the effects on fatty liver and intestinal metabolite component production in obese model mice. Fatty acid composition and fucoxanthin contents of Ishimozuku lipids were analyzed by gas chromatography (GC) and high performance liquid chromatography (HPLC) analysis. Ishimozuku lipids contained fucoxanthin about 44.71 ± 0.02 mg/g. KK-Ay mice were fed with high-fat diet (Control) and a high-fat diet + 0.5% Ishimozuku lipid diet (Ishimozuku) for 4 weeks. Liver triglyceride contents and serum triglyceride concentrations were significantly lower in the Ishimozuku group than in the Control group. Results show that acetyl-CoA carboxylase (ACC) and stearoyl-CoA desaturase (SCD-1) mRNA expression were suppressed in the livers of Ishimozuku group mice. Furthermore, short chain fatty acid amounts in the cecal contents were greater in the Ishimozuku group mice. These results suggest that dietary Ishimozuku lipid components suppress liver lipid accumulation. Results also indicate Ishimozuku lipid components improve the balance of the intestinal microflora, which is disturbed by a high fat diet, and which promotes short chain fatty acid accumulation in the intestine. That accumulation is related to lipid and glucose metabolism.
1 Introduction
Obesity is considered a major risk factor for type-2 diabetes, hypertension, and hyperlipidemia (Flier, 2004). The incidence of this cluster, which is known as metabolic syndrome, is a worldwide issue (Matsuzawa et al., 1999). Furthermore, Obesity leads to low-grade chronic inflammation, which is the cause of insulin resistance (Fernandez-Real and Ricart, 2003). Non-alcoholic steatohepatitis (NASH), a non-alcoholic fatty liver disease, is a condition by which inflammation and fibrosis occur in the liver in addition to fat accumulation (Musso et al., 2016). It in-duces more severe diseases such as hepatic cirrhosis or liver cancer. Although the mechanisms of NASH progress have not been clarified even today, the “two-hit theory” is generally reported (Tiniakos et al., 2010). According to this theory, fat accumulation in the liver results from factors such as obesity and insulin resistance as a first hit (simple steatosis). Thereafter, simple steatosis progresses to steatohepatitis because of factors such as inflammatory cytokines and oxidative stress as the second hit. Therefore, when developing a therapeutic agent, this mechanism must be considered sufficiently. Investigating the site of the point which agents suppress is particularly important.
Undaria pinnatifida (Japanese Wakame), Saccharina japonica (Japanese Makonbu), and Sargassum fulvellum (Japanese Hondawara) are all known to contain fucoxanthin, which is a specific carotenoid in brown algae (Terasaki et al., 2021a). The structure of fucoxanthin is unique, with an unusual alliance bond and 5,6-monoepoxide in its molecule. According to reports, fucoxanthin has bioactivities that include anti-oxidant, anti-inflammatory, anticancer, anti-obesity, anti-diabetic, and anti-angiogenic activities (Peng et al., 2011). Dietary intake of fucoxanthin induces expression of uncoupling protein 1 and plays an important role in energy expenditure in white adipose tissue (WAT) (Maeda et al., 2005, 2007a). Moreover, it exhibits anti-inflammatory effects in adipose tissue, consequently ameliorating low-grade chronic inflammation induced by obesity (Hosokawa et al., 2010). Therefore, dietary fucoxanthin improves insulin resistance caused by obesity (Zhang et al., 2018). In addition, fucoxanthin has been proven to be the most potent inducer of apoptosis and anti-proliferation in human cancer cells (Kotake-Nara et al., 2001, 2005). Reportedly, fucoxanthin has anti-tumor properties sufficient to prevent carcinogenesis in mice (Terasaki et al., 2021b).
It is particularly interesting that dietary fucoxanthin has been reported to affect gut microbiota. Seaweed lipids containing fucoxanthin induce short-chain fatty acid production in intestines of colorectal cancer model mice (Rausch et al., 2021). Moreover, fucoxanthin has been associated with gut microbiota related to short-chain fatty acid production in colorectal cancer model mice (Terasaki et al., 2021a).
Earlier reports of some studies have described anti-obesity and anti-diabetes effects of dietary Wakame lipids in obesity-model mice. Wakame, consisting of brown algae, contains fucoxanthin. Moreover, brown algae, including Wakame, contain n-3 polyunsaturated fatty acids in marine products such as eicosapentaenoic acid (EPA) and stearidonic acid. These fatty acids reportedly improve arteriosclerosis and NASH (Scorletti et al., 2014). Dietary EPA and docosahexaenoic acid (DHA) improve the gut microbiome related to metabolism in diabetic model mice (Zhuang et al., 2021). Therefore, algae lipids contribute to prevention of these obesity-related disorders.
In Japan, Mozuku is well-known and familiar edible brown algae. The volume of Okinawa-mozuku (Cladosiphon okamuranus) distribution has become ~90% of the market in Japan. That amount includes aquaculture techniques, which have supported stable production. By contrast, Ishimozuku (Sphaerotrichia firma) is an edible brown algae harvested from Hokkaido to Kyushu in Japan. The production is low and it is all-natural. Its morphology resembles that of this Okinawa-mozuku (Cladosiphon okamuranus), but the species are different. Earlier reports have also described that Ishimozuku has higher fucoxanthin and polyphenol contents than those of Okinawa-mozuku (Maeda et al., 2018). For that reason, Ishimozuku shows higher anti-oxidant activity than Okinawa-mozuku.
This study evaluated the effects of lipid components of Ishimozuku on suppressing accumulation of fatty liver and hyperlipidemia. High fat diets containing Ishimozuku lipids were administered to obese model KK-Ay mice for 4 weeks. Subsequently, suppressive effects of hepatic lipid accumulation and gene expression of lipid synthesize was analyzed. Furthermore, as an effect on gut microbiota, changes in the productivity of short-chain fatty acids in the intestinal tract were evaluated.
2 Materials and methods
2.1 Samples and reagents
Ishimozuku was harvested in Fukaura town in Aomori, Japan. Plant samples were collected in 2019 during the summer. Ishimozuku lipid components were extracted using acetone. Then 4 kg wet weight algae were washed by water and were wiped off using a paper towel. After the lipid components were extracted using 4 L acetone for 12 h, they were filtrated using paper filter, and evaporated by rotary evaporator. About 10 g Ishimozuku lipids were obtained. All chemicals, which were guaranteed to be of reagent or tissue-culture grade, were from Sigma (MO, USA) or Wako Pure Chemical Industries Ltd. (Osaka, Japan).
2.2 Fucoxanthin contents and fatty acid composition of Ishimozuku lipids
Fucoxanthin contents was analyzed by HPLC as previously reported (Maeda et al., 2018). The fatty acid composition of the Ishimozuku lipids was determined using a gas chromatograph (GC, GC-14B; Shimadzu Corp., Kyoto, Japan) equipped with an capillary column (Omegawax® 320; Merck KGaA, Darmstadt, Germany), as described in an earlier report (Maeda et al., 2007b).
2.3 Animal diet and care
Obesity-model mice (KK-Ay mice, male, 4 weeks old) were purchased from CLEA Japan, Inc. (Tokyo, Japan) and housed them in an air-controlled room (temperature, 23 ± 1°C) with a 12/12-h light/dark cycle. Mice were given free access to standard diets and tap water without any restrictions. After a week of adaptation, mice were divided control group (n = 8) and Ishimozuke group (n = 8), then fed an experimental diet for 4 weeks. The diet was made in accordance with AIN-93G's recommendations. The diet compositions are presented in Table 1. The mice were killed after they were anesthetized with isoflurane. Serum preparation for biochemical analysis was done after blood was collected from the abdominal aorta. The removal of liver, kidney, spleen, brown adipose tissue (BAT), and epididymal, mesenteric, inguinal, perirenal and retroperitoneal white adipose tissue (WAT) were carried out quickly, weighed, and frozen with liquid nitrogen. It was then stored at −70°C until analysis. To study mRNA expression, a small part of both the liver and epididymal WAT was stored in RNA LaterTM solution (Thermo Fisher Scientific K.K., Tokyo, Japan) at −70°C. An automatic analyzer (Olympus AU5431; Olympus Corporation, Tokyo, Japan) was used to analyze serum components (GOT, GPT, triglyceride) at the Japan Medical Laboratory (Osaka, Japan). The NIPRO Stat Strip XP2 (Nipro Corp., Osaka, Japan) was used to analyze blood glucose concentrations.
2.4 Measurement of hepatic lipid weights
Lipids were extracted according to the method explained by Folch et al. (1957). The 50 mg liver was homogenized using 2 mL chloroform: methanol (2:1 = v/v) and 2 mL water. Then it was centrifuged by 2000 rpm for 10 min. Next, the supernatant was transferred, 2 mL of chloroform : methanol (2:1 = v/v) was added to the residue again for extraction. Then, the supernatants were combined. The solvent was removed using an evaporator to obtain liver lipids.
Triglyceride E-test Wako (Fujifilm Wako Pure Chemical Corp., Osaka, Japan) was used to measure liver triglyceride. After 10 μL of 10 mg/mL dimethyl sulfoxide (0.1% Tween-20) liver lipids were plated in a 96 well plate, 200 μL of the enzyme reagent was added to each well. Then the reaction was conducted at 37°C for 15 min. The absorbance was measured at a main wavelength of 595 nm and at a sub-wavelength of 690 nm (Varioskan LUX; Thermo Fisher Scientific K.K., Tokyo Japan).
2.5 Quantitative real-time PCR analysis
The QuickGene RNA tissue kit S II (Kurabo Industries Ltd., Osaka, Japan) was used to extract total RNA from samples that had been treated with RNA later, according to the manufacturer's protocol. cDNA was generated from total RNA by following the manufacturer's protocol with a High-Capacity cDNA Reverse Transcription Kit provided by Thermo Fisher Scientific K.K., Tokyo, Japan. The AriaMx Real Time PCR system, which is owned by Agilent Technologies Japan, Ltd. in Tokyo, Japan, was used for real-time quantitative PCR analysis. The PCR solution comprised SYBER Green supermix, each primer (10 μM) and the cDNA sample. PCR cycling conditions were 95°C for 1 min and 40 cycles of 95°C for 15 s, 60°C for 1 min. The Primer sequences for ACC, ACL, SCD-1 and TNF-α were the following: ACC-F 5'-tgcctatgaactcaacagcg-3', ACC-R 5'-ctgtttagcgtggggatgtt-3'; ACL-F 5'-atcatggactcctacggcag−3', ACL-R 5'-gcttgggcttcatttctgttt−3'; SCD-1-F 5'-agaaacatgcgtagacggatca-3', SCD-1-R 5'-agaaacatgcgtagacggatca-3'; TNF-α-F : 5'-tccaggcggtgcctatgt-3'; TNF-α-R : 5'-gcccctgccacaagca-3'; and GAPDH-F 5'-catggccttccgtgttccta-3', GAPDH-R 5'-gcggcacgtcagatcca-3'.
2.6 Analysis of metabolic parameters in cecal contents
After the cecum was suspended in cold saline, and weighed, the 0.1 g of cecal content mixed with 500 μL distilled water was mixed with a vortex mixer for 5 min. Then it was centrifuged 1000 rpm for 5 min. The pH of supernatant was analyzed using a compact pH meter LAQA twin (Horiba Advanced Techno, Co., Ltd., Kyoto, Japan).
The labeling reagents for long-chain and short-chain fatty acid analysis (YMC Co., Ltd., Kyoto, Japan) were utilized to analyze the contents of short chain fatty acids in cecum in accordance with the manufacturer's protocol. In a water bath (60°C), 1 mL of cecal content extract was mixed with 200 μL of reagent A and reagent B for 20 min. Subsequently, after mixing with 200 μL of reagent C and incubating at 60°C for 15 min, it was mixed with 4 mL of reagent D and 5 mL of hexane and centrifuged at 3000 rpm for 5 min. Nitrogen gas was used to correct and evaporate the supernatant. The 200 μL of methanol was used to dilute the residue and store it at −20°C until it is analyzed by HPLC. The labeling reagent was analyzed using an ACQUITY UPLC H-Class PLUS system (Nihon Waters K.K., Tokyo, Japan). The reverse-phase column used was a 2.1 mm × 100 mm column (ACQUITY UPLC HSS T3 1.8 μm; Nihon Waters K.K., Tokyo, Japan). The mobile phases were 0.1% formic acid water (solvent A) and 0.1% formic acid acetonitrile (solvent B). Linear gradient elution was performed as time (t min), (t, A%): (0 min, 100%), (3 min, 70%), (7 min, 60%), (10 min, 60%), and (10.1 min, 100%); with a 0.3 mL/min flow rate, 5 μL injection volume, at 400 and 210 nm wavelengths, with 30°C column temperature. The standard curve prepared for each standard reagent was used to calculate the concentrations of lactic acid, acetic acid, propionic acid, and butyric acid.
2.7 Analysis of metabolic parameters in cecal contents
Results expressed as mean ± standard error (SE) are presented hereinafter. Statistical analyses between multiple groups were determined using ANOVA. Statistically significant differences were estimated using Fisher's PLSD test. Significant difference was inferred for p < 0.05. Statistical analyses were conducted using software (Stat View-J ver. 5.0; SAS Institute Inc., IL, USA).
3 Results
3.1 Fucoxanthin content and fatty acid composition of Ishimozuku lipids
Fucoxanthin contents of Ishimozuku lipid extract were analyzed using UHPLC. Results show that fucoxanthin contents were 44.71 ± 0.02 mg/g. Table 2 presents the fatty acid composition of the Ishimozuku lipid extracts. The percentages of saturated fatty acids were 44.01%. Monounsaturated fatty acids were 14.96%. Polyunsaturated fatty acids were 36.10%. The major fatty acids were palmitic acid (C16: 0, 29.64%), oleic acid (C18: 1n-9, 13.82%), and linoleic acid (C18: 2n-6, 10.43%). In addition, there were contents of stearidonic acid (C18: 4n-3, 6.04%) and eicosapentaenoic acid (C20: 5n-3, 6.97%).
3.2 Body weight gain, food intake, and tissue weight
The final body weight did not show any significant (P>0.05) difference (Control group, 42.4 ± 0.6 g; Ishimozuku group, 42.7 ± 0.8 g) and food intake (Control group, 164.4 ± 2.2 g; Ishimozuku group, 169.1 ± 4.5 g). Tissue weights found at the end of experimental diet feeding period are presented in Table 3. No significant difference (P > 0.05) was found between liver, spleen, kidney, or cecum weights, on between WAT weights.
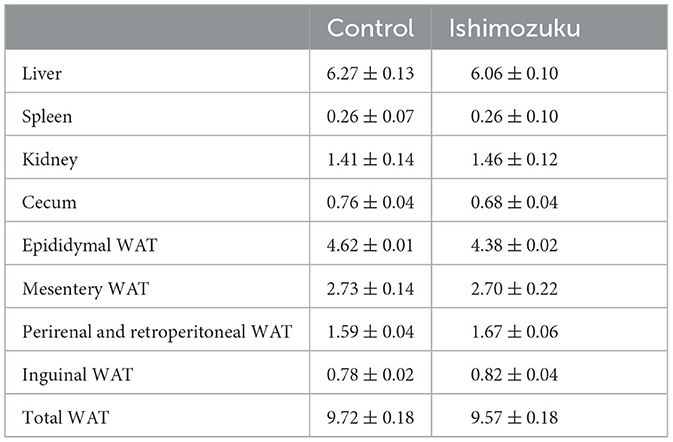
Table 3. Tissue weights of KK-Ay mice fed experiment diets (g/100 g body weight; WAT, white adipose tissue).
3.3 Plasma parameter and blood glucose concentration
Table 4 shows plasma lipid parameters. Triglycerides were significantly lower in the Ishimozuku group (p < 0.05) than in the Control group. Glutamic-oxaloacetic transaminase (GOT) and glutamic-oxaloacetic transaminase (GPT) are indicators of liver inflammation. They were unaffected. Blood glucose concentrations did not show significant different between groups.
3.4 Hepatic lipid content
Figures 1A, B respectively portray the lipid and triacylglyceride contents of livers. Lipid contents of the Ishimozuku group tended to be lower than those of the Control group. Furthermore, the Ishimozuku group showed significantly more ameliorated triglyceride accumulation than the Control group.
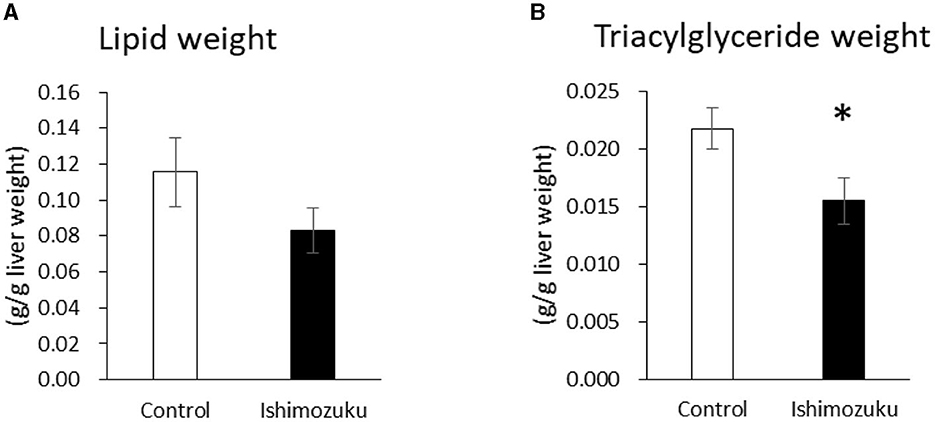
Figure 1. Lipid and triglyceride contents in the liver: (A) lipid weight and (B) triglyceride contents. *p < 0.05 vs. Control.
3.5 Fecal weight and lipid content
Table 5 presents the fecal weight and lipid contents. The Ishimozuku group tended to show increased fecal weight and fecal triglyceride contents, but no significant difference was found.
3.6 Gene expression related to fatty acid synthesis and inflammation in the liver and WAT
The mRNA expressions associated with the synthesis of fatty acids in the liver were analyzed using real-time quantitative RT-PCR. Acetyl-CoA carboxylase (ACC) and ATP-citrate lyase (ACL) are rate limiting enzymes of fatty acid synthesis (Softic et al., 2016). After converting citrate to acetyl-CoA to acetyl Co A, ACL converts acetyl Co A to malonyl CoA. ACC mRNA expression was found to be suppressed significantly (P < 0.05) in the Ishimozuku group compared with the Control group (Figure 2A).
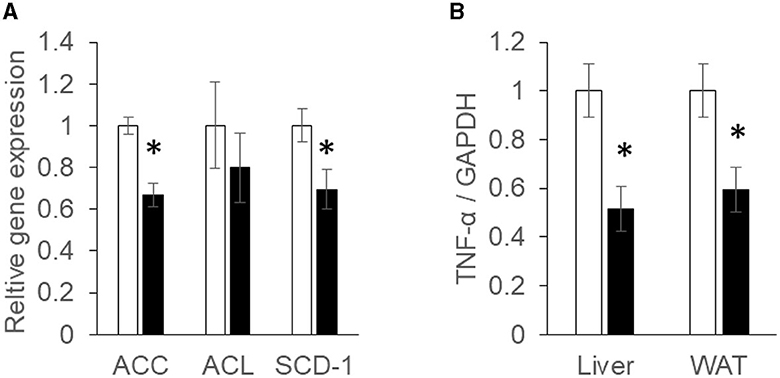
Figure 2. mRNA expression concentration of fatty acid synthesis and inflammation: (A) acetyl-CoA carboxylase (ACC), ATP-citrate lyase (ACL), and stearoyl-CoA desaturase (SCD-1) mRNA expression in the liver; (B) tumor necrosis factor α (TNF-α) mRNA expression concentration in the liver and white adipose tissue (WAT). Black bar represents the Control group. White bar represents the Ishimozuku group. *p < 0.05 vs. Control.
Stearoyl-CoA desaturase (SCD-1), an enzyme related to fatty acid metabolism, is responsible for forming unsaturated fatty acid. SCD-1 expression is up-regulated in the fatty liver. As a result, synthetic functions of oleic acid or palmitoleic acid are promoted (Ntambi et al., 2002). SCD-1 mRNA expression was significantly (p < 0.05) down-regulated in Ishimozuku groups (Figure 2A).
Tumor necrosis factor α (TNF-α) is a cytokine related to inflammation (Fernandez-Real and Ricart, 2003). After TNF-a is secreted from adipocytes in an obesity condition, it induces insulin resistance or low chronic inflammation in the body. TNF-α mRNA expression was found to be suppressed significantly more in the Ishimozuku group of WAT and liver tissue than in the Control group (Figure 2B).
3.7 Short chain fatty acid composition of cecal contents
Table 6 shows short chain fatty acid compositions of cecal contents. The Ishimozuku group mice were found to have significantly increased propionic acid and butyric acid contents compared to those of the Control group. Furthermore, pH of the cecal content was lower in the Ishimozuku group than in the Control group (Figure 3).
4 Discussion
This study analyzed anti-obesity effects of Ishimozuku lipid contents in obesity-model mice (KK-Ay). Results show that hepatic TG contents and serum TG concentration were suppressed significantly more in the Ishimozuku group than in the Control group (Table 4, Figure 1B). The mechanism of action was related to suppression of mRNA expression of ACC and ACL, which is a rate-limiting enzyme for fatty acid synthesis in the liver (Figure 2A). Moreover, the Ishimozuku group showed low mRNA expression of TNF-α in WAT and SCD-1 in the liver (Figure 2A). No significant difference was found in the lipid contents of feces. Results suggest that suppressing effects of absorption and promotion of excretion of dietary lipids in the intestine did not contribute to it (Table 5). This result suggests that chronic inflammation caused by obesity was ameliorated in WAT and liver.
Similar effects to those shown by this result have been reported for dietary brown algae lipids in animal experiments. For example, feeding with contained fucoxanthin diets (0.5 mg and 2.0 mg/g) suppressed serum TG concentration and hepatic TG contents (Woo et al., 2009, 2010). Fucoxanthin contents found through the present study in the Ishimozuku group were 0.225 mg/g of diets, which was a slightly low level. Ishimozuku lipids contained EPA of about 6.97 ± 0.41% and stearidonic acid (C18: 4n-3) of about 6.04 ± 0.09% (Table 2). EPA is a typical n-3 polyunsaturated fatty acid that is abundant in marine products. Dietary fish oil high in EPA reportedly has suppressive effects of serum TG concentration and hepatic lipid concentration in animal and clinical studies (Dyerberg et al., 1975; Saini and Keum, 2018). Fatty acids contained in seaweed lipids exist mainly in the form of glycolipids. Presumably, the glycolipid-bound EPA contained in Ishimozuku lipids showed inhibitory effects on fatty acid biosynthesis.
The relationship between intestinal microflora and various diseases has been clarified by previous research (Kau et al., 2011). Acetic acid, butyric acid, propionic acid, and lactic acid are among the compounds these microbes secrete in large amounts (Shimizu et al., 2019). Short-chain fatty acids are responsible for maintaining acidic conditions in the intestinal tract and inhibiting the growth of harmful intestinal microbiota. Reportedly, they also reduce the risk of colorectal cancer in the large intestine (Ohigashi et al., 2013). Furthermore, these short chain fatty acids are involved in the regulation of appetite, lipid metabolism, and glucose homeostasis (Shimizu et al., 2019). Short-chain fatty acid receptors are present in the intestinal tract (Bouter et al., 2017). G-protein-coupled receptors GPR43 and GPR41 have been reported as major short-chain fatty acid receptors. Actually, GPR43, which is present in adipose tissue, promotes lipid metabolism (Kimura et al., 2013). GPR41 is present in the sympathetic nerve, where it promotes energy consumption by sympathetic nerve activation (Samuel et al., 2008). Also, GPR41 regulates the secretion of an intestinal hormone (PYY), which suppresses appetite (Degen et al., 2005).
By contrast, earlier reports indicate that type 2 diabetes, which is related to obesity, changes the intestinal microflora, especially decreasing the rate of short chain fatty acid production (Karlsson et al., 2013; Sato et al., 2014). Results show that the intestinal tract becomes alkaline, and that the host metabolism is downregulated, thereby increasing appetite. In these experiments, down-regulation of pH of the cecal contents was confirmed in the Ishimozuku group (Figure 3). In connection with that, contents of propionic acid and butyric acid were increased in the cecal contents (Table 6). This result suggests that short chain fatty acids in the gut stimulate GPR41 in the sympathetic nerve, might have caused lipid metabolism by stimulating sympathetic nerves in the Ishimozuku group.
Gut bacteria ferment dietary fiber to produce short-chain fatty acids. Therefore, a diet that is high in fiber increases short-chain fatty acid production. However, in this experiment, dietary algae lipid components promoted short chain fatty acid-production. Dietary fucoxanthin changes the gut microflora in colon cancer model mice (Terasaki et al., 2021a). It was reported Lachnospiraceae, which convert polysaccharide into short chain fatty acids, were increased in fed with fucoxanthin diets group. High fat diets are a risk factor affecting the incidence of colon cancer. Other reports suggest that dietary algae lipids containing fucoxanthin promote short chain fatty acid production (Sun et al., 2020). One report describes that dietary DHA, an n-3 polyunsaturated fatty acid, promote Bifidobacterium, Lactobacillus, and SCFA-producing species in gut microbiota, and upregulate short chain fatty acid production (Zhuang et al., 2021). Collectively, these results suggest that algae lipids increase the proportion of gut microbiota related to short chain fatty acid production and regulate lipid and glucose metabolism.
5 Conclusions
Dietary Ishimozuku lipid attenuated fatty liver and serum triglyceride concentrations in obesity-model mice. Results show that it promoted short chain fatty acid production in the intestine. Those short chain fatty acids are related to lipid metabolism. These results suggest that fucoxanthin contained in Ishimozuku lipids is useful as a food ingredient for controlling obesity-related diseases.
Data availability statement
The original contributions presented in the study are included in the article/supplementary material. The raw data were generated at Hirosaki University. Derived data supporting the findings of this study are available from the corresponding author HM on request.
Ethics statement
The animal studies were approved by the Animal Research Committee of Hirosaki University (approval number A11002). The studies were conducted in accordance with the local legislation and institutional requirements. Written informed consent was obtained from the owners for the participation of their animals in this study.
Author contributions
MS: Conceptualization, Data curation, Methodology, Writing—original draft, Writing—review & editing. SF: Conceptualization, Funding acquisition, Supervision, Validation, Writing—review & editing. MT: Supervision, Validation, Writing—review & editing. HM: Funding acquisition, Supervision, Writing—original draft, Writing—review & editing.
Funding
The author(s) declare financial support was received for the research, authorship, and/or publication of this article. This work was supported by the Hirosaki University Research Project 1 (Innovation Creation / Human Resource Development Project).
Conflict of interest
The authors declare that the research was conducted in the absence of any commercial or financial relationships that could be construed as a potential conflict of interest.
Publisher's note
All claims expressed in this article are solely those of the authors and do not necessarily represent those of their affiliated organizations, or those of the publisher, the editors and the reviewers. Any product that may be evaluated in this article, or claim that may be made by its manufacturer, is not guaranteed or endorsed by the publisher.
References
Bouter, K. E., van Raalte, D. H., Groen, A. K., and Nieuwdorp, M. (2017). Role of the gut microbiome in the pathogenesis of obesity and obesity-related metabolic dysfunction. Gastroenterology 152, 1671–1678. doi: 10.1053/j.gastro.2016.12.048
Degen, L., Oesch, S., Casanova, M., Graf, S., Ketterer, S., Drewe, J., et al. (2005). Effect of peptide YY3-36 on food intake in humans. Gastroenterology 129, 1430–1436. doi: 10.1053/j.gastro.2005.09.001
Dyerberg, J., Bang, H. O., and Hjørne, N. (1975). Fatty acid composition of the plasma lipids in Greenland Eskimos. Am. J. Clin. Nutr. 28, 958–966. doi: 10.1093/ajcn/28.9.958
Fernandez-Real, J. M., and Ricart, W. (2003). Insulin resistance and chronic cardiovascular inflammatory syndrome. Endocr. Rev. 24, 278–301. doi: 10.1210/er.2002-0010
Flier, J. S. (2004). Obesity wars: molecular progress confronts an expanding epidemic. Cell 116, 337–350. doi: 10.1016/S0092-8674(03)01081-X
Folch, J., Lees, M., and Stanley, G. H. S. (1957). A simple method for the isolation and purification of total lipides from animal tissues. J. Biol. Chem. 226, 497–509. doi: 10.1016/S0021-9258(18)64849-5
Hosokawa, M., Miyashita, T., Nishikawa, S., Emi, S., Tsukui, T., Beppu, F., et al. (2010). Fucoxanthin regulates adipocytokine mRNA expression in white adipose tissue of diabetic/obese KK-Ay mice. Arch. Biochem. Biophys. 504, 17–25. doi: 10.1016/j.abb.2010.05.031
Karlsson, F. H., Tremaroli, V., Nookaew, I., Bergström, G., Behre, C. J., Fagerberg, B., et al. (2013). Gut metagenome in European women with normal, impaired and diabetic glucose control. Nature 498, 99–103. doi: 10.1038/nature12198
Kau, A. L., Ahern, P. P., Griffin, N. W., Goodman, A. L., and Gordon, J. I. (2011). Human nutrition, the gut microbiome and the immune system. Nature 474, 327–336. doi: 10.1038/nature10213
Kimura, I., Ozawa, K., Inoue, D., Imamura, T., Kimura, K., Maeda, T., et al. (2013). The gut microbiota suppresses insulin-mediated fat accumulation via the short-chain fatty acid receptor GPR43. Nat. Commun. 4, 1829. doi: 10.1038/ncomms2852
Kotake-Nara, E., Kushiro, M., Zhang, H., Sugawara, T., Miyashita, K., Nagao, A., et al. (2001). Carotenoids affect proliferation of human prostate cancer cells. J. Nutr. 131, 3303–3306. doi: 10.1093/jn/131.12.3303
Kotake-Nara, E., Terasaki, M., and Nagao, A. (2005). Characterization of apoptosis induced by fucoxanthin in human promyelocytic leukemia cells. Biosci. Biotechnol. Biochem. 69, 224–227. doi: 10.1271/bbb.69.224
Maeda, H., Fukuda, S., Izumi, H., and Saga, N. (2018). Anti-oxidant and fucoxanthin contents of brown alga ishimozuku (Sphaerotrichia divaricata) from the West Coast of Aomori, Japan. Mar. Drugs. 16, 255. doi: 10.3390/md16080255
Maeda, H., Hosokawa, M., Sashima, T., Funayama, K., and Miyashita, K. (2005). Fucoxanthin from edible seaweed, Undaria pinnatifida, shows antiobesity effect through UCP1 expression in white adipose tissues. Biochem. Biophys. Res. Commun. 332, 392–397. doi: 10.1016/j.bbrc.2005.05.002
Maeda, H., Hosokawa, M., Sashima, T., Funayama, K., and Miyashita, K. (2007b). Effect of medium-chain triacylglycerols on anti-obesity effect of fucoxanthin. J. Oleo. Sci. 56, 615–621. doi: 10.5650/jos.56.615
Maeda, H., Hosokawa, M., Sashima, T., and Miyashita, K. (2007a). Dietary combination of fucoxanthin and fish oil attenuates the weight gain of white adipose tissue and decreases blood glucose in obese/diabetic KK-Ay mice. J. Agric. Food Chem. 55, 7701–7706. doi: 10.1021/jf071569n
Matsuzawa, Y., Funahashi, T., and Nakamura, T. (1999). Molecular mechanism of metabolic syndrome X: contribution of adipocytokines adipocyte-derived bioactive substances. Ann. N.Y. Acad. Sci. 892, 146–154. doi: 10.1111/j.1749-6632.1999.tb07793.x
Musso, G., Cassader, M., and Gambino, R. (2016). Non-alcoholic steatohepatitis: emerging molecular targets and therapeutic strategies. Nat. Rev. Drug Disc. 15, 249–274. doi: 10.1038/nrd.2015.3
Ntambi, J. M., Miyazaki, M., Stoehr, J. P., Lan, H., Kendziorski, C. M., Yandell, B. S., et al. (2002). Loss of stearoyl-CoA desaturase-1 function protects mice against adiposity. Proc. Natl. Acad. Sci. U.S.A. 99, 11482–11486. doi: 10.1073/pnas.132384699
Ohigashi, S., Sudo, K., Kobayashi, D., and Takahashi, O. (2013). Changes of the intestinal microbiota, short chain fatty acids, and fecal pH in patients with colorectal cancer. Dig. Dis. Sci. 58, 1717–1726. doi: 10.1007/s10620-012-2526-4
Peng, J., Yuan, J. P., Wu, C. F., and Wang, J. H. (2011). Fucoxanthin, a marine carotenoid present in brown seaweeds and diatoms: metabolism and bioactivities relevant to human health. Mar. Drugs 9, 1806–1828. doi: 10.3390/md9101806
Rausch, J., Gillespie, S., Orchard, T., Tan, A., and McDaniel, J. C. (2021). Systematic review of marine-derived omega-3 fatty acid supplementation effects on leptin, adiponectin, and the leptin-to-adiponectin ratio. Nutr. Res. 85, 135–152. doi: 10.1016/j.nutres.2020.11.002
Saini, R. K., and Keum, Y. S. (2018). Omega-3 and omega-6 polyunsaturated fatty acids: dietary sources, metabolism, and significance – A review. Life Sci. 203, 255–267. doi: 10.1016/j.lfs.2018.04.049
Samuel, B. S., Shaito, A., Motoike, T., Rey, F. E., Backhed, F., Manchester, J. K., et al. (2008). Effects of the gut microbiota on host adiposity are modulated by the short-chain fatty-acid binding G protein-coupled receptor, Gpr41. Proc. Natl. Acad. Sci. U. S. A. 105, 16767–11672. doi: 10.1073/pnas.0808567105
Sato, J., Kanazawa, A., Ikeda, F., Yoshihara, T., Goto, H., Abe, H., et al. (2014). Gut dysbiosis and detection of “live gut bacteria” in blood of Japanese patients with type 2 diabetes. Diab. Car. 37, 2343–2350. doi: 10.2337/dc13-2817
Scorletti, E., Bhatia, L., McCormick, K. G., Clough, G. F., Nash, K., Hodson, L., et al. (2014). Effects of purified eicosapentaenoic and docosahexaenoic acids in nonalcoholic fatty liver disease: results from the Welcome study. Hepatology 60, 1211–1221. doi: 10.1002/hep.27289
Shimizu, H., Ohue-Kitano, R., and Kimura, I. (2019). Regulation of host energy metabolism by gut microbiota-derived short-chain fatty acids. Glycative Stress Res. 6, 181–191. doi: 10.24659/gsr.6.3_181
Softic, S., Cohen, D. E., and Kahn, C. R. (2016). Role of dietary fructose and hepatic de novo lipogenesis in fatty liver disease. Dig. Dis. Sci. 61, 1282–1293. doi: 10.1007/s10620-016-4054-0
Sun, X., Zhao, H., Liu, Z., Sun, X., Zhang, D., Wang, S., et al. (2020). Modulation of gut microbiota by fucoxanthin during alleviation of obesity in high-fat diet-fed mice. J. Agric. Food Chem. 68, 5118–5128. doi: 10.1021/acs.jafc.0c01467
Terasaki, M., Kubota, A., Kojima, H., Maeda, H., Miyashita, K., Kawagoe, C., et al. (2021a). Fucoxanthin and colorectal cancer prevention. Cancers 13, 2379. doi: 10.3390/cancers13102379
Terasaki, M., Uehara, O., Ogasa, S., Sano, T., Kubota, A., Kojma, H., et al. (2021b). Alteration of fecal microbiota by fucoxanthin results in prevention of colorectal cancer in AOM/DSS-treated mice. Carcinogenesis 42, 210–219. doi: 10.1093/carcin/bgaa100
Tiniakos, D. G., Vos, M. B., and Brunt, E. M. (2010). Nonalcoholic fatty liver disease: pathology and pathogenesis. Annu. Rev. Pathol. 5, 145–171. doi: 10.1146/annurev-pathol-121808-102132
Woo, M. N., Jeon, S. M., Kim, H. J., Lee, M. K., Shin, S. K., Shin, Y. C., et al. (2010). Fucoxanthin supplementation improves plasma and hepatic lipid metabolism and blood glucose concentration in high-fat fed C57BL/6N mice. Chem. Biol. Interact. 186, 316–322. doi: 10.1016/j.cbi.2010.05.006
Woo, M. N., Jeon, S. M., Shin, Y. C., Lee, M. K., Kang, M. A., and Choi, M. S. (2009). Anti-obese property of fucoxanthin is partly mediated by altering lipid-regulating enzymes and uncoupling proteins of visceral adipose tissue in mice. Mol. Nutr. Food Res. 53, 1603–1611. doi: 10.1002/mnfr.200900079
Zhang, Y., Xu, W., Huang, X., Zhao, Y., Ren, Q., Hong, Z., et al. (2018). Fucoxanthin ameliorates hyperglycemia, hyperlipidemia and insulin resistance in diabetic mice partially through IRS-1/PI3K/Akt and AMPK pathways. J. Funct. Foods 48 515–524. doi: 10.1016/j.jff.2018.07.048
Keywords: fucoxanthin, obese, fatty liver, algae, short chain fatty acid, Ishimozuku, microflora
Citation: Shibata M, Fukuda S, Terasaki M and Maeda H (2024) Ishimozuku (Sphaerotrichia firma) lipids containing fucoxanthin suppress fatty liver and improve short chain fatty acid production in obese model mice. Front. Sustain. Food Syst. 7:1331061. doi: 10.3389/fsufs.2023.1331061
Received: 31 October 2023; Accepted: 28 December 2023;
Published: 11 January 2024.
Edited by:
SHI Wenzheng, Shanghai Ocean University, ChinaReviewed by:
A. K. M. Azad Shah, Bangabandhu Sheikh Mujibur Rahman Agricultural University, BangladeshXuanri Shen, Hainan Tropical Ocean University, China
Copyright © 2024 Shibata, Fukuda, Terasaki and Maeda. This is an open-access article distributed under the terms of the Creative Commons Attribution License (CC BY). The use, distribution or reproduction in other forums is permitted, provided the original author(s) and the copyright owner(s) are credited and that the original publication in this journal is cited, in accordance with accepted academic practice. No use, distribution or reproduction is permitted which does not comply with these terms.
*Correspondence: Hayato Maeda, hayatosp@hirosaki-u.ac.jp