Corrigendum: Mechanism of enhanced freshness formulation in optimizing antioxidant retention of gold kiwifruit (Actinidia chinensis) harvested at two maturity stages
- 1Discipline of Horticultural Science, School of Agricultural, Earth and Environmental Sciences, University of KwaZulu-Natal, Scottsville, South Africa
- 2Plant Science Laboratory, Cranfield University, Bedfordshire, United Kingdom
- 3Department of Food Systems and Development, Faculty of Natural and Agricultural Sciences, University of Free State, Bloemfontein, South Africa
Kiwifruit exhibits a climacteric ripening pattern and has as an extremely perishable nature. Considering that high perishability leads to a loss in antioxidants and overall nutritional quality. This study aimed to examine the efficacy of enhanced freshness formulation (EFF), a hexanal-based formulation containing antioxidants such as geraniol, α-tocopherol and ascorbic acid, on maintaining the bioactive compounds of gold kiwifruit (cv. ‘Y368’) harvested at two maturity stages. Kiwifruits were treated with three treatments, namely, control (untreated fruit), 0.01 and 0.02% (v/v) EFF. Fruits were treated with 8 weeks of cold storage at 0°C and 90% relative humidity, then transferred to 20°C for 8 days. Three bioactive compounds (ascorbic acid, total phenolics and flavonoids), antioxidant capacities using DPPH and FRAP assays, polyphenol oxidase, ascorbate oxidase, phenylalanine and tyrosine ammonia lyase enzyme activities were evaluated. The results showed that EFF significantly (p < 0.05) influenced bioactive compounds, antioxidant capacities and the activity of enzymes involved in the synthesis and oxidation of bioactive compounds. The maturity stage significantly influenced the content of bioactive compounds. Later harvested kiwifruit had greater content of bioactive compounds, compared to earlier harvested kiwifruit. The total phenolic content was 0.77, 1.09 and 1.22 mg GAE g−1 FW for control, 0.01 and 0.02% EFF, respectively. The FRAP antioxidant concentration was 0.76, 0.91 and 0.96 μmol Fe (II) g−1 FW for control, 0.01 and 0.02% EFF. The findings illustrate the capacity of EFF to optimize bioactive compounds and storability of kiwifruit during postharvest storage.
Introduction
The action of bioactivities including ascorbic acid, carotenoids and various flavanones and polyphenols contribute to the health beneficial properties of kiwifruit (Pérez-Burillo et al., 2018). Gold (yellow-fleshed) kiwifruit (Actinidia chinensis) possesses significant nutritional value and an array of bioactive compounds which make it a good source of antioxidants. The high nutritional value of kiwifruit has resulted in an increasing global demand for kiwifruits (Ma et al., 2017). According to the Post-Harvest Innovation (PHI) Programme, it takes approximately 25 days to export South African fresh produce to Europe. However, deterioration of logistical infrastructure and shortage of refrigerated containers causes congestion and shipping delays at South Africa’s ports (Brodie, 2022). In addition to this, the delivery of kiwifruit exported by sea is delayed by at least three weeks, due to delays that transpire at the European port (Eichstaedt, 2022).
Delays experienced along the supply chain result in extended storage duration of kiwifruit. These challenges present a problem since several kiwifruit cultivars have an extremely perishable nature due to their high metabolic activity (Asiche et al., 2017). This leads to a significant loss in bioactive compounds of kiwifruit (Huang et al., 2017; Cha et al., 2019; Kumarihami et al., 2020). The kiwifruit industry highly relies on cold storage, which facilitates in prolonging the storage of kiwifruit (Shin et al., 2018; Cha et al., 2019; Xia et al., 2020). However, cold storage alone is not enough to optimize quality if the storage duration is prolonged. Findings from previous research have illustrated that prolonged storage under cold conditions (0–5°C) results in a significant loss of bioactive compounds of kiwifruit (Goffi et al., 2019; Jeong et al., 2020; Xia et al., 2020; Choi et al., 2022). The importance of incorporating postharvest treatments with cold storage to reduce the loss of bioactive compounds is well established in kiwifruit.
High demand from European markets necessitates South African growers and exporters to develop strategies for preserving kiwifruit during extended storage periods, aiming to meet the increasing demand for high-quality kiwifruit with health-promoting characteristics. Fruit maturity is an essential factor that influences the concentration of bioactive compounds and the postharvest performance of kiwifruit (Deepthi and Sekhar, 2015; Lee et al., 2015; Nkonyane et al., 2022). Literature has demonstrated that kiwifruit should be harvested when the total soluble solid content is at a minimum of 6.2 °Brix, in order to optimize fruit quality during storage (Burdon et al., 2016). Mahlaba et al. (2021) also determined that a minimum harvest and export value should be ≥6.2 °Brix for gold kiwifruit produced in South Africa. Thus, harvesting at the optimum maturity stage minimizes the loss of bioactive compounds. In spite of this, South African gold kiwifruits destined for export, are not harvested at a singular maturity stage but harvested at the mid (≥ 6.2 °Brix) and late (≥ 8.5 °Brix) maturity stages (Mahlaba et al., 2021; Nkonyane et al., 2022). However, these authors demonstrated that late harvested kiwifruit experience a substantial decrease in quality during storage. Therefore, it is essential to employ postharvest treatments that can maintain bioactive compounds of kiwifruit harvested at various maturity stages.
Past research has investigated the impact of various postharvest treatments such as 1-methylcyclopropene (Lim et al., 2016; Xu et al., 2021; Zhang et al., 2021), edible coatings (Allegra et al., 2016; Hu et al., 2019; Kumarihami et al., 2022), ozone (Goffi et al., 2019; Wang et al., 2022), UV-C (Hu et al., 2022) and heat treatment (Shahkoomahally and Ramezanian, 2015; Chiabrando et al., 2018) on the maintenance of bioactive compounds in kiwifruit. Notably, these studies predominantly focused on kiwifruit harvested at only one maturity stage. To the best of our ability, we have not found studies investigating the efficacy of postharvest treatments on optimizing bioactive compounds of gold kiwifruit harvested at different maturity stages.
Considering this gap in literature and the growing demand for high-quality kiwifruit, it is essential to explore a postharvest treatment that can optimize the bioactive compounds of kiwifruit harvested at various maturity stages. Hexanal is a naturally occurring volatile compound, specifically a six-carbon aldehyde formed from linoleic acid via the lipoxygenase pathway during lipid peroxidation in plants (Paliyath and Padmanabhan, 2018). Enhanced freshness formulation (EFF) is a formulation that comprises of 1% hexanal, 1% geraniol, 1% α-tocopherol and 1% ascorbic acid (Cheema et al., 2014). EFF has the capacity to optimize phenolics and ascorbic acid of various fruit (Cheema et al., 2014; Gill et al., 2016; Jincy et al., 2017). Hexanal’s capacity to reduce the loss in bioactive compounds could serve as a potential tool of optimizing quality and storability of South African grown gold kiwifruit. South African gold kiwifruits are harvested at both the mid and late maturity stage. However, literature has illustrated that late harvested experience a substantial decrease in quality. Considering that the export of South African grown gold kiwifruit is expected to rise given the growing demand from international markets, this makes it necessary to devise a postharvest strategy that can maintain optimum quality of kiwifruit harvested at both maturity stages.
Therefore, the current study was conducted to evaluate the efficacy of EFF to optimize bioactive compounds (phenolic and flavonoid compounds, ascorbic acid and carotenoids) of gold kiwifruit (cv. ‘Y368’) harvested at two maturity stages. Furthermore, the activity of enzymes, phenylalanine ammonia-lyase, tyrosine ammonia-lyase, polyphenol oxidase and ascorbate oxidase, involved in the synthesis and oxidation of these bioactive compounds (phenolic and flavonoid compounds, ascorbic acid and carotenoids) were investigated.
Materials and methods
Materials
Kiwifruit (Actinidia chinensis) cv. “Y368” were harvested from Roselands farm, a commercial kiwifruit farm located in the Richmond area (Latitude: 29.9033°S, Longitude: 30.2397°E), KwaZulu-Natal Province, South Africa. Kiwifruits were harvested when the total soluble solid content was greater than 6.2 °Brix (Mahlaba et al., 2021), at two maturity stages namely, maturity stage one (M1) and maturity stage two (M2) with 7 and 9 °Brix, respectively. Harvested fruit were immediately transported in a ventilated vehicle to the Postharvest Laboratory of the University of KwaZulu-Natal, where kiwifruit without blemishes, decay or physical damage were graded and selected for uniformity in size, then assigned to the respective postharvest treatments. All chemicals used to conduct the experiments in this study were purchased from Sigma-Aldrich, South Africa.
Preparation of postharvest treatments and storage
The enhanced freshness formulation (EFF) was prepared by making a stock formulation comprising of 1% (v/v) hexanal, 1% (v/v) geraniol, 1% (w/v) α-tocopherol, 1% (w/v) ascorbic acid, 0.1% (w/v) cinnamic acid and 10% (v/v) Tween 20 dissolved in ethanol (10% v/v) (Cheema et al., 2014). A 0.01 and 0.02% (v/v) hexanal concentrations were prepared by mixing the stock solution in 100 L and 50 L of distilled water, respectively. Kiwifruit were immersed in 0.01 and 0.02% (v/v) EFF solution for 2.5 min, then air dried at room temperature (Cheema et al., 2014). Control fruit were left untreated. Each treatment consisted of three replicates (n = 3); each replicate had three fruit per storage interval for each maturity stage. The kiwifruits were stored for 8 weeks in a cold room with temperature set at 0°C and relative humidity at 90%, then transferred to 20°C for one week to stimulate shelf life.
Total phenolic content
The total phenolic content was determined using a method described by Singleton et al. (1999). Kiwifruit pulp (1 g) was homogenized with 5 mL of 80% methanol. Thereafter, 2.6 mL of distilled water and 200 μL of Folin–Ciocalteu’s phenol reagent was added to 200 μL of the methanolic extracts. After 6 min, 2 mL of 7% (w/v) sodium carbonate was added to the reaction mixture. The absorbance was measured at 750 nm after 90 min using a Shimadzu UV spectrophotometer (Model UV-1800 240 V, Kyoto, Japan). The total phenolic content was expressed as mg gallic acid equivalents GAE g−1 fresh weight (FW) of kiwifruit.
Total flavonoid content
Distilled water (3.2 mL) and 150 μL of 5% (w/v) sodium nitrite was added to 500 μL of methanolic extract. After 5 min, 150 μL of 10% (w/v) aluminum chloride was added. After 6 min, 1 mL of 1 M sodium hydroxide was added and the absorbance recorded at 510 nm. The total flavonoid content was expressed as mg catechin equivalents CE g−1 fresh FW of kiwifruit.
Ascorbic acid
A method described by Malik and Singh (2005) and modified by Goffi et al. (2019), was used to determine the ascorbic acid content of kiwifruit. Ascorbic acid was extracted from 1 g of kiwifruit pulp using 4 mL of 16% (v/v) metaphosphoric acid, containing 0.18% (w/v) disodium ethylenediaminetetraacetic acid and centrifuged at 1100 × g for 10 min at 4°C. The assay mixture contained 200 μL supernatant, Folin’s reagent (1: 5 v/v) and 0.3% (v/v) metaphosphoric acid in a final volume of 2 mL. The absorbance was measured at 760 nm, using ascorbic acid as standard and expressed as mg g−1 FW.
Total carotenoids
Total carotenoid content was determined using a method adapted from Yan et al. (2015) and modified by Xia et al. (2020). Five milli liter of acetone containing 0.1% butylated hydroxytoluene was used to extract carotenoids from 1 g of kiwifruit pulp. After centrifugation at 10,000 × g for 10 min at 4°C, the supernatant was filtered through the Whatman filter paper No. 42. The total carotenoid content was determined by measuring the absorbance of the extract at 450 nm and expressed as μg g−1 FW.
DPPH radical-scavenging activity
DPPH was assessed using a protocol described by Brand-Williams et al. (1995). Thus, 6 × 10−5 mol/L DPPH was dissolved in methanol (1 L) and 3.9 mL of this solution was added to 100 μL of methanolic extracts. After 30 min at room temperature, the absorbance of the reaction mixture was measured at 517 nm. The DPPH radical scavenging activity was quantified using Eq. (1) and expressed as percentage DPPH radical-scavenging activity (DPPH %)
Ferric-reducing antioxidant power
The FRAP was assessed using a previously reported protocol (Benzie and Strain, 1996). The FRAP working solution was prepared daily by mixing 300 mM acetate buffer (pH 3.6), 10 mM TPTZ solution in 40 mM HCl, and 20 mM FeCl3 solution at a ratio of 10:1:1. The freshly solution was warmed at 30°C before usage. Then, 100 μL of each kiwifruit pulp extract was mixed with 3 mL of FRAP solution and incubated at 37°C for 4 min. Thereafter, the absorbance was recorded at 593 nm. A calibration curve was prepared using ferrous sulfate. The findings were expressed as μM g−1 FW.
Extracts for phenylalanine ammonia lyase and tyrosine ammonia lyase
The enzymatic activity of PAL (EC 4.3.1.5) and TAL (EC 4.3.1.25) was evaluated using a method described by Khan et al. (2003). Extraction was carried out by homogenizing 1 g of kiwifruit pulp with 10 mL of ice-cool Tris–HCl buffer (50 mM, pH 8.5) comprising of 5% (w/v) polyvinylpolypyrrolidone (PVPP) and 14.4 mM β-mercaptoethanol. After centrifugation at 10000 g at 4°C for 20 min, the enzyme activity of PAL and TAL was quantified using the recovered supernatant. Bradford assay Bradford (1976) was used to determine the protein concentration.
Phenylalanine ammonia lyase
The activity of PAL was quantified using an assay mixture containing 800 μL of Tris–HCl buffer (0.5 mM, pH 8.0), 100 μL 6 μM of L-phenylalanine, and 100 μL of enzyme extract. After incubation at 40°C for an hour, the reaction was stopped by adding 100 μL of 5 N HCl to the mixture. The enzyme activity was quantified using the absorbance measured at 290 nm. The activity was expressed as U g−1 of protein, where a unit of enzyme activity was defined as the amount of enzyme that produces 1 nmole of cinnamic acid min−1.
Tyrosine ammonia lyase
The activity of TAL was quantified using an assay mixture containing 800 μL of Tris–HCl buffer (0.5 mM, pH 8.0), 100 μL of 5.5 μM of L-tyrosine and 100 μL of enzyme extract. After incubation at 40°C for an hour, the reaction was stopped by adding 100 μL of 5 N HCl to the mixture. The enzyme activity was quantified using the absorbance measured at 333 nm. The activity was expressed as U g−1 of protein, where a unit of enzyme activity was defined as the amount of enzyme that produces 1 nmole of coumaric acid min−1.
Ascorbate oxidase
The enzyme activity of ascorbate oxidase (EC 1.10.3.3) was assayed using a previously described protocol by Jiang et al. (2018). AO was extracted by homogenizing 1 g of pulp with 3 mL potassium phosphate buffer (50 mM, pH 7.5) comprising of 1 mL of potassium phosphate buffer (100 mM, pH 6.8), 0.1 mM ethylenediaminetetraacetic acid (EDTA), 0.3% (w/v) Triton X-100, 4% (w/v) polyvinyl-polypyrrolidone (PVPP), and 0.1 mM ascorbic acid. After centrifugation at 16000 g for 15 min, the absorbance was measured. The enzyme activity was expressed as U g−1 of protein and defined as the amount of enzyme causing an increase of 0.01 in the absorbance per min at 265 nm at 25°C. The Bradford assay Bradford (1976) was used to determine the protein concentration.
Polyphenol oxidase activity
A protocol by Adiletta et al. (2019) was used to assay PPO (EC 1.14.18.1) activity. One gram of pulp was homogenized in sodium phosphate buffer (100 mM, pH 6.4) comprising of 0.05 g of PVPP. After centrifugation at 10,000 g for 30 min at 4°C, 100 μL of the enzyme extract was added to a buffered substrate (500 mM catechol in 100 mM sodium phosphate buffer, pH 6.4) and the increase in absorbance was monitored at 398 nm. PPO activity was expressed as U g−1 of protein. The Bradford assay Bradford (1976) was used to determine the protein concentration.
Statistical analysis
All experiments were performed using a completely randomized factorial design. The experiment comprised of three factors: treatments, fruit maturity stage and storage period. Statistical analysis was performed in R software version 4.3.1 (R Core Team, 2023). Data were expressed as mean values ± SE and were subjected to analysis of variance (ANOVA) with a 5% level of significance. A Pearson correlation test was used to correlate the measured parameters.
Results and discussion
Total phenolic and flavonoid content
The interaction among treatment, maturity stage, and storage time had no significant (p > 0.05) effect on the TPC. The results illustrate that treatments significantly influenced (p < 0.001) the TPC (Figure 1A), with treated fruit registering substantially greater TPC compared to the control. The TPC was 0.88, 1.17 and 1.29 mg GAE g−1 FW for the control, 0.01 and the 0.02% EFF treatment level, respectively. The interaction between treatment and storage time had no significant effect (p > 0.05) on TPC. However, the interaction between maturity stage and storage time (Figure 1B) had a significant (p < 0.001) effect on TPC, with M2 registering significantly higher TPC than M1. Kiwifruit at both maturity stages exhibited an increase in TPC, reaching maximum values of 1.21, and 1.58 mg GAE g−1 FW for M1 and M2, respectively, at six weeks of storage. At the end of storage, TPC for M1 and M2 was 1.06 and 1.19 mg GAE g−1 FW, respectively.
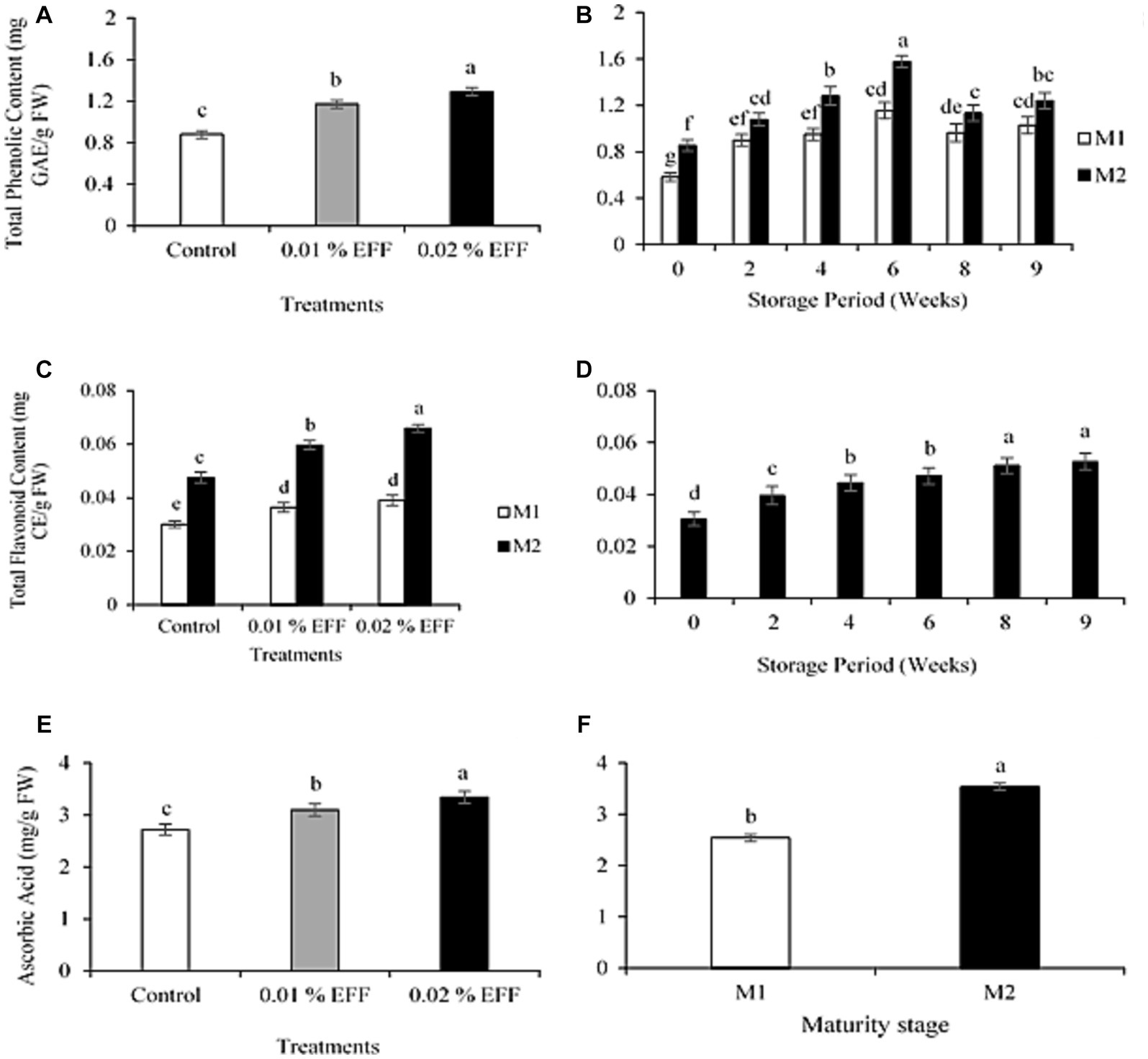
Figure 1. Effect of treatments (A) and the effect of interaction between maturity stage and storage time (B) on the total phenolic content of kiwifruit. Effect of interaction between treatment and maturity stage (C) and the effect of storage time (D) on the total flavonoid content of kiwifruit. Effect of treatments (E) an maturity stage (F) on ascorbic acid content of kiwi fruit. Values are the means ± SE (n = 3).
The interaction among treatment, maturity stage and storage time had no significant (p > 0.05) effect on the TFC. The interaction between treatment and storage time had no significant effect (p > 0.05) on TFC. However, the combined effect of treatment and maturity stage (Figure 1C) significantly (p < 0.001) influenced the TFC. Treated fruit exhibited significantly higher TFC compared to the control. Maturity stage influenced the TFC, with M2 exhibiting significantly greater TFC relative to M1. Significant differences among treated fruit were only observed at M2, with 0.02% EFF registering higher TFC. At M1, no significant differences among treated fruit were observed. In addition, the TFC significantly (p < 0.001) increased throughout the storage period (Figure 1D).
The findings of this study illustrated that storage duration affects phenolic content of Y368 kiwifruit. This is characterized by a significant decrease in TPC after six weeks of storage. These findings corroborate those of Lim and Eom (2018), Goffi et al. (2019), and Jeong et al. (2020), who demonstrated that TPC in kiwifruit decreased during cold storage. Furthermore, Gullo et al. (2016) and Nkonyane et al. (2022), attained comparable findings to the present study. These authors illustrated that kiwifruit exhibited an increase in TPC when transferred to ambient conditions. Results obtained from this study demonstrated that EFF-treated kiwifruit had substantially higher TPC and TFC during storage. The findings attained are in alignment to those reported by Gill et al. (2016), Sharma et al. (2023), and Öz et al. (2023), who demonstrated that EFF effectively maintained higher phenolic content of guava, jujube and persimmon fruit, respectively. Secondary metabolites such as phenolic and flavonoid compounds are crucial for the maintenance of fruit quality, as they delay senescence induced by oxidative degradation, by functioning as antioxidants that scavenge free radicals (Gulcin, 2020). Therefore, the efficacy of EFF to enhance TPC and TFC may contribute to optimized quality and storability of kiwifruit during storage.
Ascorbic acid
The interaction among treatment, maturity stage and storage time had no significant (p > 0.05) effect on AA. The interaction between treatment and storage time had no significant effect (p > 0.05) on AA. The treatments had a significant effect (p < 0.001) on AA content (Figure 1E), with the 0.02% treated fruit registering higher AA content, followed by 0.01% treated fruit. AA content for the control, 0.01 and 0.02% EFF treatment level was 2.72, 3.12 and 3.34 mg g−1 FW. These findings indicate that EFF treatment application effectively retained AA content throughout the storage period. Our results corroborate those reported by Cheema et al. (2014), Gill et al. (2016), Jincy et al. (2017), Silué et al. (2022) and Sharma et al. (2023) who illustrated that EFF retained ascorbic acid of tomato, guava, mango and jujube fruit, respectively. The maturity stage had a significant effect (p < 0.001) on AA content (Figure 1F), with M2 (3.53 mg g−1 FW) exhibiting a greater AA content than M1 (2.54 mg g−1 FW).
Findings from the present study are in alignment with those of Lim and Eom (2018), who illustrated that AA content increased with an advancement in maturity. In plants, AA preserves cell integrity by reducing hydrogen peroxide and reacting rapidly with radical species (Dumanović et al., 2021). Thus, it provides DNA and lipids with protection from oxidative damage (Meitha et al., 2020). The loss of AA in kiwifruit during storage is estimated to be between 50 and 70% (Sharma et al., 2015). AA undergoes oxidative loss as it is converted to dehydroascorbic acid by the enzymatic action of ascorbate oxidase (Chatzopoulou et al., 2020). EFF has been reported to delay the loss of AA in guava fruit by reducing the oxidation process (Gill et al., 2016). Therefore, the findings from the present study show that EFF has the potential to preserve the nutritional value of kiwifruit by delaying the loss of AA during storage.
Total carotenoids
The interaction among treatment, maturity stage and storage time had no significant (p > 0.05) effect on TC. Treated kiwifruit exhibited significantly (p < 0.01) lower TC, compared to the control (Figure 2A). The TC was registered at 5, 4.78 and 4.71 μg g−1 FW for the control, 0.01 and 0.02% treatment level, respectively. The interaction between treatment and storage time had no significant effect (p > 0.05) on TC. The interaction between maturity stage and storage period significantly (p < 0.05) influenced the TC content (Figure 2B). The TC significantly decreased after four weeks of storage for kiwifruit harvested at M1. TC significantly decreased after two weeks for kiwifruit harvested at M2. Throughout the storage period, kiwifruit harvested at M2 exhibited greater TC relative to M1.
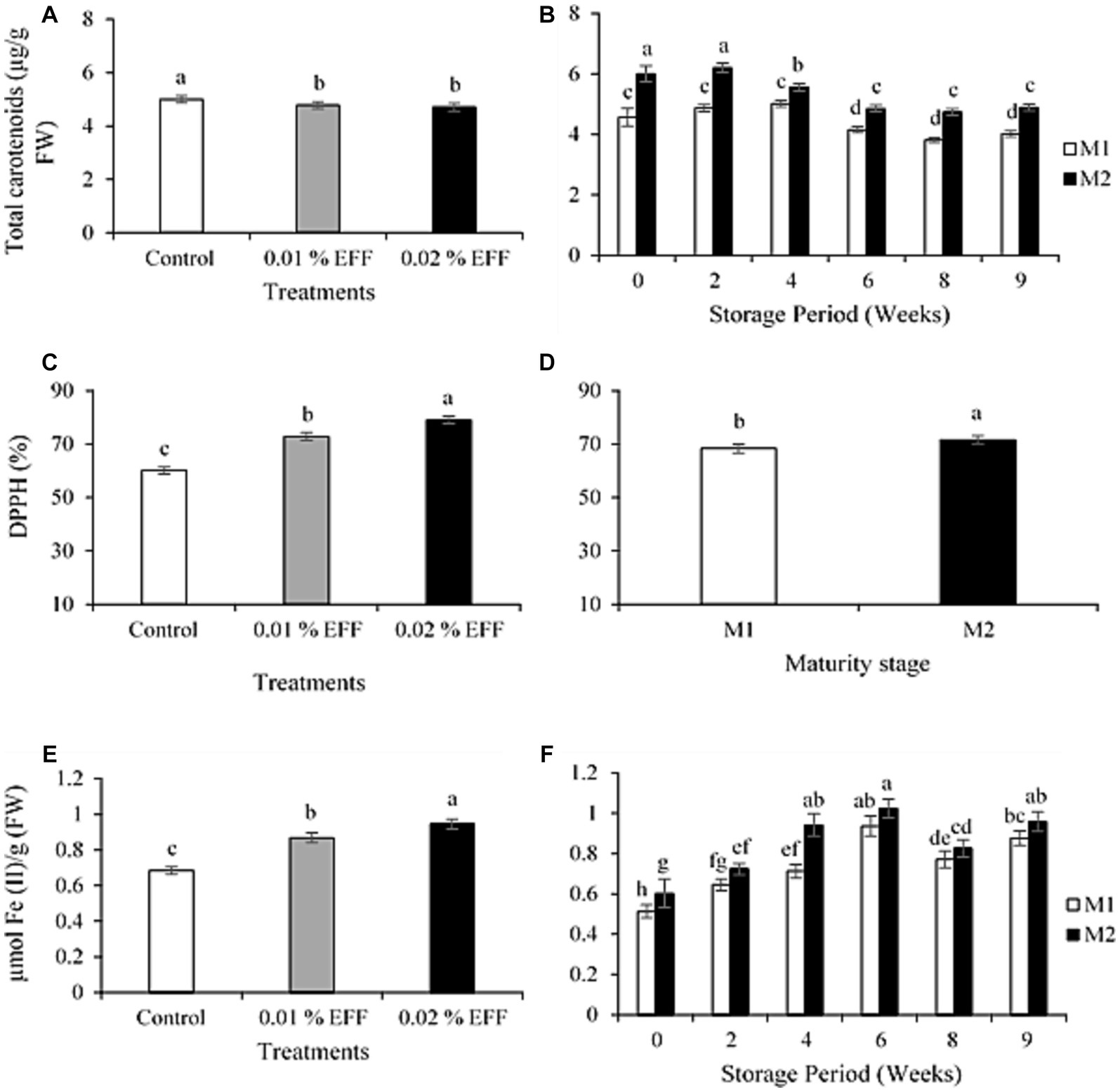
Figure 2. Effect of treatments (A) and the effect of interaction between maturity stage and storage time (B) on the total carotenoid content of kiwifruit. Effect of treatments (C) and maturity stage (D) on DPPH scavenging activity of kiwifruit. Effect of treatments (E) and the effect of interaction between maturity stage and storage time (F) on FRAP of kiwi fruit. Values are the means ± SE (n = 3).
The antioxidant properties of carotenoids provide protection from certain kinds of cancer. EFF treated fruit exhibited less carotenoid content relative to the untreated kiwifruit. Similarly, Tiwari and Paliyath (2011) and Dek et al. (2018) showed that EFF lowered the accumulation of carotenoids in tomato fruit. This may be indicative of better-quality maintenance due to a slower rate of ripening (Cheema et al., 2018). Storage temperature has a strong influence on carotenoid metabolism (Matsumoto et al., 2009). Exposing kiwifruit to low temperatures slows down the accumulation of carotenoids, whereas high temperatures enhance the accumulation of carotenoids (Xia et al., 2020). Low storage temperatures upregulate the expression of cleavage dioxygenase and 9-cis-epoxycarotenoid dioxygenase involved in the degradation of carotenoids, resulting in a decrease in carotenoid content (Xia et al., 2020).
Antioxidant capacity
The interaction between treatment, maturity stage and storage time had no significant (p > 0.05) effect on DPPH. The interaction between treatment and storage time had no significant effect (p > 0.05) on DPPH. Treatments had a significant effect (p < 0.001) on DPPH scavenging activity (Figure 2C). Treated fruit had higher DPPH scavenging activity relative to the control. The maturity stage had a significant (p < 0.01) effect on DPPH scavenging activity (Figure 2D). Kiwifruit harvested at M2 exhibited greater DPPH scavenging activity compared to kiwifruit harvested at M1. The interaction among treatment, maturity stage and storage time had no significant (p > 0.05) effect on the Ferric Reducing Antioxidant Power (FRAP). The interaction between treatment and storage time had no significant effect (p > 0.05) on FRAP. Treatments had a significant effect (p < 0.001) on FRAP (Figure 2E). The control, 0.01 and 0.02% EFF treatment level had 0.68, 0.87 and 0.95 μmol Fe (II) g−1 FW, respectively. The interaction between maturity stage and storage time had a significant effect (p < 0.05) on the FRAP (Figure 2F), with M2 registering significantly greater FRAP relative to M1. The FRAP significantly increased and decreased during cold storage, followed by an increase during shelf life. The findings are comparable with those attained by Jincy et al. (2017) and Öz et al. (2023), where hexanal treated mango and persimmon fruit had higher antioxidant capacity.
Bioactive compounds such as ascorbic acid, polyphenols and flavonoids in plants have a significant influence on the antioxidant capacity (He et al., 2019). Thus, DPPH and FRAP assays were performed to assess the antioxidant capacity of these bioactive compounds. EFF treatment application substantially (p < 0.05) increased the antioxidant capacities, which is in accordance with results attained by Jincy et al. (2017), for EFF-treated mango fruit. Therefore, the enhanced antioxidant capacity exhibited by EFF-treated kiwifruit may be owed to a higher content of bioactive compounds in treated fruit (Figure 1). Furthermore, the findings of this study show that the maturity stage significantly influenced the antioxidant capacity of kiwifruit (Figures 2D,F). Kiwifruit harvested at M2 exhibited greater antioxidant capacity than kiwifruit harvested at M1. Considering that bioactive compounds influence antioxidant capacity, these findings indicate that the higher antioxidant capacities of kiwifruit harvested at M2 is attributed to their higher concentration of bioactive compounds (Figure 1). These results are in alignment with those of Lim and Eom (2018), who demonstrated that kiwifruit harvested at later maturity stages exhibited a greater concentration of bioactive compounds. The findings obtained in the current study, indicate that EFF treatment effectively optimized the bioactive compounds and antioxidant capacity in kiwifruit at both maturity stages.
Phenylalanine ammonia-lyase and tyrosine ammonia-lyase
The interaction among treatment, maturity stage and storage time had no significant (p > 0.05) effect on PAL activity. The interaction between treatment and storage time had no significant effect (p > 0.05) on PAL. Treatments significantly (p < 0.001) influenced the PAL enzyme activity (Figure 3A). The control, 0.01 and 0.02% EFF treatment level registered 1.11, 1.32 and 1.41 U g−1 FW, respectively. The maturity stage had a significant (p < 0.001) effect on PAL activity (Figure 3B), with kiwifruit harvested at M2 (1.46 U g−1 FW) exhibiting greater PAL activity compared to kiwifruit harvested at M1 (1.08 U g−1 FW). The interaction among treatment, maturity stage and storage time had no significant (p > 0.05) effect on TAL. The interaction between treatment and storage time had no significant effect (p > 0.05) on TAL. The treatments significantly (p < 0.001) influenced TAL activity (Figure 3C), with treated fruit exhibiting higher TAL enzyme activity relative to the control. A significant difference was not observed among treated fruit. The interaction between maturity stage and storage period had a significant effect (p < 0.001) on the TAL activity (Figure 3D).
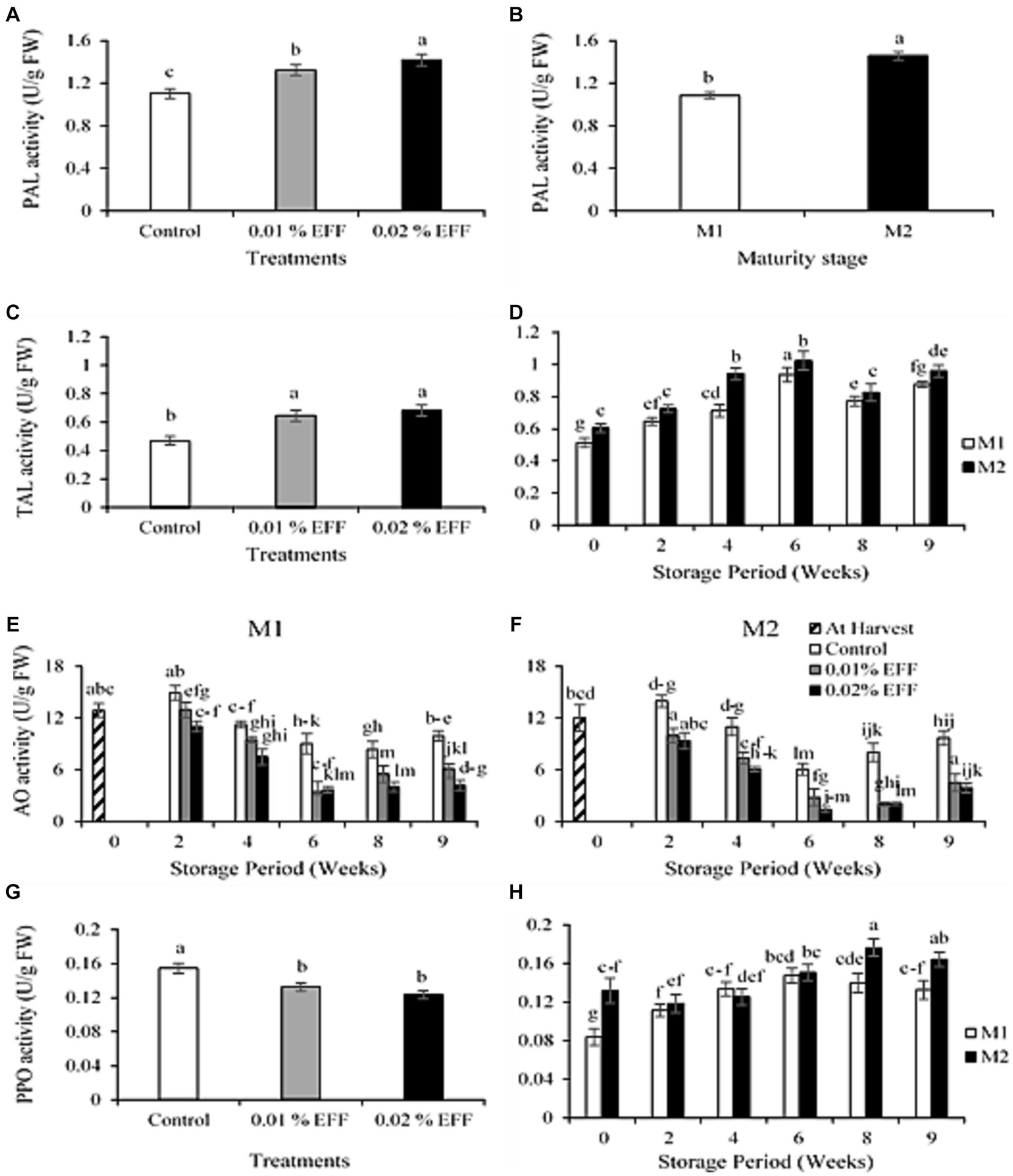
Figure 3. Effect of treatments (A) and maturity stage (B) on PAL activity of kiwifruit. Effect of treatments (C) and the effect of interaction between maturity stage and storage time (D) on TAL activity of kiwifruit. The effect of interaction among treatments, maturity stage and storage time on AO activity of kiwifruit harvested at M1 (E) and M2 (F). Effect of treatments (G) and the effect of interaction between maturity stage and storage time (H) on PPO activity of kiwi fruit. Values are the means ± SE (n = 3).
Results obtained from this study are in accordance with those reported by Bai et al. (2014) and Dhakshinamoorthy et al. (2020), who illustrated that hexanal treatment enhanced the PAL activity of oriental sweet melon and banana fruit, respectively. To the best of our ability, we have not found research studies reporting on the effect of hexanal or EFF on TAL enzyme activity. The phenylpropanoid biosynthesis pathway is a metabolic pathway in plants that utilities phenylalanine and in some instances tyrosine to synthesize secondary metabolites (de Barros Rates and Cesarino, 2023). This pathway is governed by the enzymatic action of PAL, which is involved in the synthesis of phenolic compounds such as phenolic acids and flavonoids (Barros et al., 2015; Bian et al., 2020). Similar to PAL, TAL is an enzyme in the phenylpropanoid biosynthesis pathway involved in the conversion of tyrosine to p-coumarate, and it also facilitates the production of secondary metabolites (Yadav et al., 2020).
The findings in this study suggest that EFF influenced the phenylpropanoid biosynthesis pathway by enhancing PAL and TAL enzyme activities in treated kiwifruit. Considering that PAL and TAL participate in the synthesis of phenolic compounds, the higher TPC and TFC (Figures 1A,C) in EFF-treated kiwifruit, may be attributed to the ability of EFF to enhance PAL and TAL enzyme activities. Previous research studies have shown that hexanal upregulates the expression of genes that encode for PAL enzyme activity (Padmanabhan et al., 2020; Hyun et al., 2022). Thus, we hypothesize that the enhanced enzyme activity of PAL in EFF-treated kiwifruit may be due to the upregulation of PAL genes in response to EFF treatment. However, further research investigating the response of gene expression to EFF-treated kiwifruit is warranted to strengthen this argument.
Ascorbate oxidase
The interaction between treatment, maturity stage and storage time had a significant (p < 0.001) effect on AO enzyme activity (Figures 3E,F). The AO enzyme activity decreased at both maturity stages during storage. However, storing the kiwifruit at 20°C after cold storage, led to an increase in AO activity. Kiwifruit harvested at M1 had higher AO activity (control = 9.93 U g−1 FW, 0.01% EFF = 6.11 U g−1 FW, 0.02% EFF = 4.21 U g−1 FW) in comparison to kiwifruit harvested at M2 (control = 9.69 U g−1 FW, 0.01% EFF = 4.50 U g−1 FW, 0.02% EFF = 3.91 U g−1 FW) at week nine of storage. The treatments significantly (p < 0.001) influenced the AO activity, with the treated fruit exhibiting significantly lower AO enzyme activity compared to the control. Considering that AO is involved in the degradation of ascorbic acid (Chatzopoulou et al., 2020), the efficacy of EFF to optimize retention of ascorbic acid (Figure 1E) may be attributed to its capacity to suppress the enzymatic activity of AO. A qRT-PCR analysis conducted by El Kayal et al. (2017) demonstrated that EFF treatment application downregulated the gene expression of AO in strawberry fruit. Thus, we hypothesize that the mechanism in which EFF suppresses the enzymatic activity of AO is due to its ability to downregulate the gene expression of AO, which in turn, leads to a reduction in the oxidation of ascorbic acid.
Polyphenol oxidase activity
The interaction among treatment, maturity stage and storage time had no significant (p > 0.05) effect on PPO enzyme activity. The interaction between treatment and storage time had no significant effect (p > 0.05) on PPO. The treatments significantly (p < 0.001) influenced the PPO activity (Figure 3G). The control had the highest PPO activity, followed by the 0.01 and 0.02% treatment level. The interaction between maturity stage and storage period had a significant effect (p < 0.01) on the PPO enzyme activity (Figure 3H). Kiwifruit harvested at M2 exhibited significantly higher PPO activity than those harvested at M1. PPO was 0.13 and 0.16 U g−1 FW for M1 and M2 at week 9 of storage, respectively.
The findings of the present study are in accordance with those reported by Kaur et al. (2019) and Sharma et al. (2023), who demonstrated that EFF suppressed the PPO enzyme activity in oriental sweet melons, grape berries and jujube fruit, respectively. PPO is a terminal oxidase that utilizes polyphenols as substrates, which leads to internal browning of fruit. Furthermore, the enzymatic activity of PPO can be inhibited by aliphatic alcohols (Valero et al., 1990). Past research has demonstrated that hexanal in fruit tissues is converted to hexanol which is an aliphatic alcohol (Sharma et al., 2008). The lower PPO enzyme activity exhibited in treated fruit may be attributed to the conversion of hexanal to hexanol in kiwifruit tissues after EFF treatment application. To the best of our abilities, we have not found studies investigating the capability of hexanol to inhibit PPO enzyme activity. Therefore, further research must be conducted to examine if the hexanol volatile compound has an inhibitory effect on the enzymatic activity of PPO.
Pearson correlation coefficient
A correlation analysis was done to examine the relationships between bioactive compounds on antioxidant capacity and the enzymes (Figure 4). The DPPH scavenging activity exhibited a significant and positive correlation with TPC (r = 0.67; p < 0.001), TFC (r = 0.62; p < 0.001) and AA (r = 0.38; p < 0.001). FRAP showed a significant and positive correlation with TPC (r = 0.81; p < 0.001), TFC (r = 0.67; p < 0.001) and AA (r = 0.50; p < 0.001). The correlation between PAL activity, TPC and TFC was 0.78 (p < 0.001) and 0.76 (p < 0.001), respectively. This indicates that TPC and TFC is strongly correlated with PAL enzyme activity. TAL activity exhibited a strong positive correlation with TPC (r = 0.71; p < 0.001) and a weak correlation with TFC (r = 0.39; p < 0.001). AA content and AO enzyme activity exhibited a significant but moderately negative correlation (r = −0.44; p < 0.001).
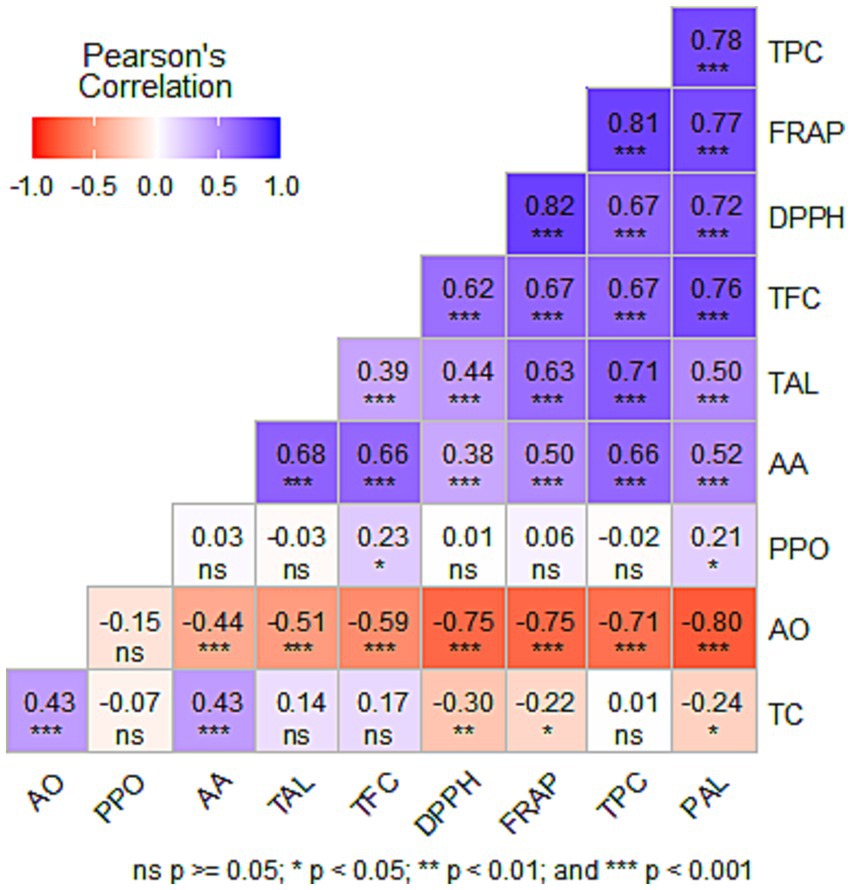
Figure 4. Pearson correlation matrix between total phenolic content (TPC), total flavonoid content (TFC), ascorbic acid (AA), total carotenoids (TC), DPPH, Ferric-Reducing Antioxidant Power (FRAP), phenylalanine ammonia lyase (PAL), tyrosine ammonia lyase (TAL), ascorbate oxidase (AO), and polyphenol oxidase (PPO). The correlation coefficients are proportional to the color intensity. Positive correlation is displayed in blue and negative in red color. n.s. = not significant.
The results from the correlation analysis show that bioactive compounds have a direct influence on the antioxidant capacity of kiwifruit, which is in alignment with findings reported by He et al. (2019). Furthermore, the correlation analysis indicates that the accumulation of phenolic compounds is associated with the enzymatic activity of PAL and TAL. These findings are corroborated by those reported by Khan et al. (2003). Furthermore, the inverse relationship between AA and AO suggests that AO has an influence in the degradation of AA (Jiang et al., 2018). These findings indicate that the bioactive compounds in kiwifruit can be optimized by enhancing the activities of enzymes involved in the synthesis of phenolic compounds and suppressing the enzyme (AO) involved in the degradation of AA. Therefore, the efficacy of EFF to optimize the bioactive compounds in kiwifruit, could be attributed to its capacity to enhance and suppress the activity of enzymes involved in the synthesis and oxidation of these bioactive compounds, which in turn improve kiwifruit quality and storability.
Conclusion
The findings from the present study showed that EFF effectively optimized the antioxidant capacity in kiwifruit by regulating the action of enzymes involved in both the synthesis and oxidation of bioactive compounds. Therefore, it can be concluded that EFF has the potential to optimize the functional quality of South African grown gold kiwifruit, harvested at the mid and late maturity stage. The present study primarily examined the effect of EFF on the activity of enzymes involved in both the synthesis and oxidation of bioactive compounds. However, transcriptome analysis is needed in order to gain an in-depth understanding of the underlying mechanism on how EFF influences these enzymes. Therefore, further research using RNA sequencing is warranted to provide a comprehensive understanding of the mechanism in which EFF optimizes the synthesis of bioactive compounds. Furthermore, assessing the inhibitory effects of the hexanol volatile compound against PPO activity, may elucidate how hexanal application suppresses the action of this enzyme.
Data availability statement
The original contributions presented in the study are included in the article/supplementary material, further inquiries can be directed to the corresponding author.
Author contributions
SM: Conceptualization, Data curation, Formal analysis, Investigation, Methodology, Software, Writing – original draft. LM: Conceptualization, Funding acquisition, Supervision, Validation, Visualization, Writing – review & editing. ST: Conceptualization, Data curation, Supervision, Validation, Writing – review & editing. AM: Conceptualization, Supervision, Writing – review & editing, Project administration.
Funding
The author(s) declare financial support was received for the research, authorship, and/or publication of this article. This work was supported for the study was provided by the National Research Foundation of South Africa (NRF) Grant number: SFH220123657394.
Acknowledgments
The authors are grateful to Thokozani Nkosi and Babalwa Nyide for providing technical assistance.
Conflict of interest
The authors declare that the research was conducted in the absence of any commercial or financial relationships that could be construed as a potential conflict of interest.
Publisher’s note
All claims expressed in this article are solely those of the authors and do not necessarily represent those of their affiliated organizations, or those of the publisher, the editors and the reviewers. Any product that may be evaluated in this article, or claim that may be made by its manufacturer, is not guaranteed or endorsed by the publisher.
References
Adiletta, G., Petriccione, M., Liguori, L., Zampella, L., Mastrobuoni, F., and Di Matteo, M. (2019). Overall quality and antioxidant enzymes of ready-to-eat 'Purple Queen' pomegranate arils during cold storage. Postharvest Biol. Technol. 155, 20–28. doi: 10.1016/j.postharvbio.2019.05.008
Allegra, A., Inglese, P., Sortino, G., Settanni, L., Todaro, A., and Liguori, G. (2016). The influence of Opuntia ficus-indica mucilage edible coating on the quality of ‘Hayward’ kiwifruit slices. Postharvest Biol. Technol. 120, 45–51. doi: 10.1016/j.postharvbio.2016.05.011
Asiche, W. O., Mitalo, O. W., Kasahara, Y., Tosa, Y., Mworia, E. G., Ushijima, K., et al. (2017). Effect of storage temperature on fruit ripening in three kiwifruit cultivars. Horticult. J. 86, 403–410. doi: 10.2503/hortj.OKD-028
Bai, X. H., Teng, L. H., Lu, D. Q., and Qi, H. Y. (2014). Co-treatment of EFF and 1-MCP for enhancing the shelf-life and aroma volatile compounds of oriental sweet melons (Cucumis melo var. makuwa Makino). J. Integr. Agric. 13, 217–227. doi: 10.1016/S2095-3119(13)60372-X
Barros, J., Serk, H., Granlund, I., and Pesquet, E. (2015). The cell biology of lignification in higher plants. Ann. Bot. 115, 1053–1074. doi: 10.1093/aob/mcv046
Benzie, I. F. F., and Strain, J. J. (1996). The ferric reducing ability of plasma (FRAP) as a measure of “antioxidant power”: the FRAP assay. Anal. Biochem. 239, 70–76. doi: 10.1006/abio.1996.0292
Bian, X. H., Li, W., Niu, C. F., Wei, W., Hu, Y., Han, J. Q., et al. (2020). A class B heat shock factor selected for during soybean domestication contributes to salt tolerance by promoting flavonoid biosynthesis. New Phytol. 225, 268–283. doi: 10.1111/nph.16104
Bradford, M. M. (1976). A rapid and sensitive method for the quantitation of microgram quantities of protein utilizing the principle of protein-dye binding. Anal. Biochem. 72, 248–254. doi: 10.1016/0003-2697(76)90527-3
Brand-Williams, W., Cuvelier, M. E., and Berset, C. (1995). Use of a free-radical method to evaluate antioxidant activity. LWT Food Sci. Technol. 28, 25–30. doi: 10.1016/S0023-6438(95)80008-5
Brodie, L. (2022). South African port challenges are constraining South Africa’s fruit exports. Available at: https://www.linkedin.com/pulse/south-african-port-challenges-constraining-africas-fruit-#:~:text=South (accessed April 16, 2022).
Burdon, J., Pidakala, P., Martin, P., Billing, D., and Boldingh, H. (2016). Fruit maturation and the soluble solids harvest index for 'Hayward' kiwifruit. Sci. Hortic. 213, 193–198. doi: 10.1016/j.scienta.2016.10.027
Cha, G. H., Kumarihami, H. P. C., Kim, H. L., Kwack, Y. B., and Kim, J. G. (2019). Storage temperature influences fruit ripening and changes in organic acids of kiwifruit treated with exogenous ethylene. Horticult. Sci. Technol. 37, 618–629. doi: 10.7235/HORT.20190062
Chatzopoulou, F., Sanmartin, M., Mellidou, I., Pateraki, I., Koukounaras, A., Tanou, G., et al. (2020). Silencing of ascorbate oxidase results in reduced growth, altered ascorbic acid levels and ripening pattern in melon fruit. Plant Physiol. Biochem. 156, 291–303. doi: 10.1016/j.plaphy.2020.08.040
Cheema, A., Padmanabhan, P., Amer, A., Parry, M. J., Lim, L.-T., Subramanian, J., et al. (2018). Postharvest hexanal vapor treatment delays ripening and enhances shelf life of greenhouse grown sweet bell pepper (Capsicum annum L.). Postharvest Biol. Technol. 136, 80–89. doi: 10.1016/j.postharvbio.2017.10.006
Cheema, A., Padmanabhan, P., Subramanian, J., Blom, T., and Paliyath, G. (2014). Improving quality of greenhouse tomato (Solanum lycopersicum L.) by pre- and postharvest applications of hexanal-containing formulations. Postharvest Biol. Technol. 95, 13–19. doi: 10.1016/j.postharvbio.2014.03.012
Chiabrando, V., Peano, C., and Giacalone, G. (2018). Influence of hot water treatments on postharvest physicochemical characteristics of Hayward and Jintao kiwifruit slices. J. Food Process. Preserv. 42:e13563. doi: 10.1111/jfpp.13563
Choi, H. R., Baek, M. W., Tilahun, S., and Jeong, C. S. (2022). Long-term cold storage affects metabolites, antioxidant activities, and ripening and stress-related genes of kiwifruit cultivars. Postharvest Biol. Technol. 189:111912. doi: 10.1016/j.postharvbio.2022.111912
de Barros Rates, A., and Cesarino, I. (2023). Pour some sugar on me: the diverse functions of phenylpropanoid glycosylation. J. Plant Physiol. 291:154138. doi: 10.1016/j.jplph.2023.154138
Deepthi, V. P., and Sekhar, R. C. (2015). Postharvest physiological and biochemical changes in guava (cv. Lucknow-49) fruits harvested at two stages of maturity during low-temperature storage. International Journal of Processing and Post-Harvest Technology. 6, 128–143. doi: 10.15740/HAS/IJPPHT/6.2/128-143
Dek, M. S. P., Padmanabhan, P., Subramanian, J., and Paliyath, G. (2018). Inhibition of tomato fruit ripening by 1-MCP, wortmannin and hexanal is associated with a decrease in transcript levels of phospholipase D and other ripening related genes. Postharvest Biol. Technol. 140, 50–59. doi: 10.1016/j.postharvbio.2018.02.009
Dhakshinamoorthy, D., Sundaresan, S., Iyadurai, A., Subramanian, K. S., Janavi, G. J., Paliyath, G., et al. (2020). Hexanal vapor induced resistance against major postharvest pathogens of Banana (Musa acuminata L.). Plant Pathol. J. 36, 133–147. doi: 10.5423/PPJ.OA.03.2019.0072
Dumanović, J., Nepovimova, E., Natić, M., Kuča, K., and Jaćević, V. (2021). The significance of reactive oxygen species and antioxidant defense system in plants: A concise overview. Front. Plant Sci. 11:552969. doi: 10.3389/fpls.2020.552969
Eichstaedt, F. (2022). Gold kiwi fruits cultivated in South Africa. Available at: https://www.freshplaza.com/europe/article/9416123/gold-kiwi-fruits-cultivated-in-south-africa/ (Accessed April 17, 2022).
El Kayal, W., El-Sharkawy, I., Dowling, C., Paliyath, G., Sullivan, J. A., and Subramanian, J. (2017). Effect of preharvest application of hexanal and growth regulators in enhancing shelf life and regulation of membrane-associated genes in strawberry. Can. J. Plant Sci. 97, 1109–1120. doi: 10.1139/cjps-2016-0351
Gill, K. S., Dhaliwal, H. S., Mahajan, B. V. C., Paliyath, G., and Boora, R. S. (2016). Enhancing postharvest shelf life and quality of guava (Psidium guajava L.) cv. Allahabad Safeda by pre-harvest application of hexanal containing aqueous formulation. Postharvest Biol. Technol. 112, 224–232. doi: 10.1016/j.postharvbio.2015.09.010
Goffi, V., Zampella, L., Forniti, R., Petriccione, M., and Botondi, R. (2019). Effects of ozone postharvest treatment on physicochemical and qualitative traits of Actinidia chinensis ‘Soreli’during cold storage. J. Sci. Food Agric. 99, 5654–5661. doi: 10.1002/jsfa.9823
Gulcin, İ. (2020). Antioxidants and antioxidant methods: an updated overview. Arch. Toxicol. 94, 651–715. doi: 10.1007/s00204-020-02689-3
Gullo, G., Dattola, A., Liguori, G., Vonella, V., Zappia, R., and Inglese, P. (2016). Evaluation of fruit quality and antioxidant activity of kiwifruit during ripening and after storage. J. Berry Res. 6, 25–35. doi: 10.3233/JBR-150111
Huang, Z., Li, J., Zhang, J., Gao, Y., and Hui, G. (2017). Physicochemical properties enhancement of Chinese kiwi fruit (Actinidia chinensis Planch) via chitosan coating enriched with salicylic acid treatment. J. Food Meas. Charact. 11, 184–191. doi: 10.1007/s11694-016-9385-1
He, X., Fang, J., Chen, X., Zhao, Z., Li, Y., Meng, Y., et al. (2019). Actinidia chinensis planch.: A review of chemistry and pharmacology. Front. Pharmacol. 10:1236. doi: 10.3389/fphar.2019.01236
Hu, X., Chen, Y., Wu, X., Liu, W., Jing, X., Liu, Y., et al. (2022). Combination of calcium lactate impregnation with UV-C irradiation maintains quality and improves antioxidant capacity of fresh-cut kiwifruit slices. Food Chem. 14:100329. doi: 10.1016/j.fochx.2022.100329
Hu, H., Zhou, H., and Li, P. (2019). Lacquer wax coating improves the sensory and quality attributes of kiwifruit during ambient storage. Sci. Hortic. 244, 31–41. doi: 10.1016/j.scienta.2018.09.026
Hyun, J., Lee, J. G., Yang, K. Y., Lim, S., and Lee, E. J. (2022). Postharvest fumigation of (E)-2-hexenal on kiwifruit (Actinidia chinensis cv. ‘Haegeum’) enhances resistance to Botrytis cinerea. Postharvest Biology and Technology. 187:111854. doi: 10.1016/j.postharvbio.2022.111854
Jeong, H. R., Cho, H. S., Cho, Y. S., and Kim, D. O. (2020). Changes in phenolics, soluble solids, vitamin C, and antioxidant capacity of various cultivars of hardy kiwifruits during cold storage. Food Sci. Biotechnol. 29, 1763–1770. doi: 10.1007/s10068-020-00822-7
Jiang, Z. Y., Zhong, Y., Zheng, J., Ali, M., Liu, G.-D., and Zheng, X.-L. (2018). L-ascorbic acid metabolism in an ascorbate-rich kiwifruit (Actinidia. Eriantha Benth.) cv.‘White’during postharvest. Plant Physiol. Biochem. 124, 20–28. doi: 10.1016/j.plaphy.2018.01.005
Jincy, M., Djanaguiraman, M., Jeyakumar, P., Subramanian, K. S., Jayasankar, S., and Paliyath, G. (2017). Inhibition of phospholipase D enzyme activity through hexanal leads to delayed mango (Mangifera indica L.) fruit ripening through changes in oxidants and antioxidant enzymes activity. Sci. Hortic. 218, 316–325. doi: 10.1016/j.scienta.2017.02.026
Kaur, S., Arora, N., Gill, K. B. S., Sharma, S., and Gill, M. (2019). Hexanal formulation reduces rachis browning and postharvest losses in table grapes cv. ‘flame seedless’. Sci. Hortic. 248, 265–273. doi: 10.1016/j.scienta.2019.01.011
Khan, W., Prithiviraj, B., and Smith, D. L. (2003). Chitosan and chitin oligomers increase phenylalanine ammonia-lyase and tyrosine ammonia-lyase activities in soybean leaves. J. Plant Physiol. 160, 859–863. doi: 10.1078/0176-1617-00905
Kumarihami, H. M. P. C., Cha, G. H., Kim, J. G., Kim, H. U., Lee, M., Kwack, Y. B., et al. (2020). Effect of preharvest ca-chitosan application on postharvest quality of 'garmrok' kiwifruit during cold storage. Hortic. Sci. Technol. 38, 239–248. doi: 10.7235/HORT.20200023
Kumarihami, H. P. C., Kim, Y.-H., Kwack, Y.-B., Kim, J., and Kim, J. G. (2022). Application of chitosan as edible coating to enhance storability and fruit quality of kiwifruit: A review. Sci. Hortic. 292:110647. doi: 10.1016/j.scienta.2021.110647
Lee, I., Im, S., Jin, C. R., Heo, H. J., Cho, Y. S., Baik, M. Y., et al. (2015). Effect of maturity stage at harvest on antioxidant capacity and total phenolics in kiwifruits (Actinidia spp.) grown in Korea. Hortic. Environ. Biotechnol. 56, 841–848. doi: 10.1007/s13580-015-1085-y
Lim, S., Han, S. H., Kim, J., Lee, H. J., Lee, J. G., and Lee, E. J. (2016). Inhibition of hardy kiwifruit (Actinidia aruguta) ripening by 1-methylcyclopropene during cold storage and anticancer properties of the fruit extract. Food Chem. 190, 150–157. doi: 10.1016/j.foodchem.2015.05.085
Lim, Y. J., and Eom, S. H. (2018). Kiwifruit cultivar ‘Halla gold‘ functional component changes during preharvest fruit maturation and postharvest storage. Sci. Hortic. 234, 134–139. doi: 10.1016/j.scienta.2018.02.036
Ma, T., Sun, X., Zhao, J., You, Y., Lei, Y., Gao, G., et al. (2017). Nutrient compositions and antioxidant capacity of kiwifruit (Actinidia) and their relationship with flesh colour and commercial value. Food Chem. 218, 294–304. doi: 10.1016/j.foodchem.2016.09.081
Mahlaba, N., Tesfay, S., Dodd, M., Magwaza, L., Mditshwa, A., and Ngobese, N. (2021). Determination of optimal maturity indices for south African golden kiwifruit cultivars. Paper presented at the X International Symposium on Kiwifruit 1332, 335–342. doi: 10.17660/ActaHortic.2022.1332.44
Malik, A. U., and Singh, Z. (2005). Pre-storage application of polyamines improves shelf-life and fruit quality of mango. J. Hortic. Sci. Biotechnol. 80, 363–369. doi: 10.1080/14620316.2005.11511945
Matsumoto, H., Ikoma, Y., Kato, M., Nakajima, N., and Hasegawa, Y. (2009). Effect of postharvest temperature and ethylene on carotenoid accumulation in the flavedo and juice sacs of Satsuma mandarin (Citrus unshiu Marc.) fruit. J. Agric. Food Chem. 57, 4724–4732. doi: 10.1021/jf9005998
Meitha, K., Pramesti, Y., and Suhandono, S. (2020). Reactive oxygen species and antioxidants in postharvest vegetables and fruits. Int. J. Food Sci. 2020, 1–11. doi: 10.1155/2020/8817778
Nkonyane, M., Tesfay, S., Dodd, M., Magwaza, L., Mditshwa, A., and Ngobese, N. (2022). Effect of harvest maturities on phytochemical composition of golden kiwifruit grown under south African conditions. X Int. Symp. Kiwifruit 1332, 359–364. doi: 10.17660/ActaHortic.2022.1332.47
Öz, A. T., Eryol, B., and Ali, M. A. (2023). Postharvest hexanal application delays senescence and maintains quality in persimmon fruit during low temperature storage. J. Sci. Food Agric. 103, 7653–7663. doi: 10.1002/jsfa.12847
Padmanabhan, P., Cheema, A. S., Todd, J. F., Lim, L. T., and Paliyath, G. (2020). Ripening responses, fruit quality and phospholipase D gene expression in bell peppers exposed to hexanal vapor. Postharvest Biol. Technol. 170:111317. doi: 10.1016/j.postharvbio.2020.111317
Paliyath, G., and Padmanabhan, P. (2018). Preharvest and postharvest technologies based on hexanal: an overview. Postharvest Biol. Nanotechnol., 89–101. doi: 10.1002/9781119289470.ch4
Pérez-Burillo, S., Oliveras, M., Quesada, J., Rufián-Henares, J., and Pastoriza, S. (2018). Relationship between composition and bioactivity of persimmon and kiwifruit. Food Res. Int. 105, 461–472. doi: 10.1016/j.foodres.2017.11.022
R Core Team (2023). R: A language and environment for statistical computing (Version 4.3.1). Vienna, Austria: R Foundation for Statistical Computing. Available at: https://www.R-project.org.
Shahkoomahally, S., and Ramezanian, A. (2015). Hot water combined with calcium treatment improves physical and physicochemical attributes of kiwifruit (Actinidia deliciosa cv. Hayward) during storage. HortScience 50, 412–415. doi: 10.21273/HORTSCI.50.3.412
Sharma, A., Bons, H. K., Jawandha, S. K., and Chung, S. W. (2023). Effect of hexanal treatment on fruit qualities and antioxidant activities on′ Umran′ Indian jujube fruit during cold storage. bioRxiv. doi: 10.1101/2023.04.06.535963,535963
Sharma, R., Jhalegar, M. J., Jha, S., and Rana, V. (2015). Genotypic variation in total phenolics, antioxidant activity, enzymatic activity and quality attributes among kiwifruit cultivars. J. Plant Biochem. Biotechnol. 24, 114–119. doi: 10.1007/s13562-013-0242-6
Sharma, M., Sitbon, C., Paliyath, G., and Subramanian, J. (2008). “Changes in nutritional quality of fruits and vegetables during storage” in Postharvest biology and Technology of Fruits, vegetables and flowers. eds. G. Paliyath, D. P. Murr, A. K. Handa, and S. Lurie (New York: John Wiley & Sons)
Shin, M. H., Kwack, Y. B., Kim, Y. H., and Kim, J. G. (2018). Storage temperature affects the ripening characteristics of ‘Garmrok’, ‘Hayward’, ‘Goldone’, and ‘Jecy gold’ kiwifruit treated with exogenous ethylene. Hortic. Sci. Technol. 36, 730–740. doi: 10.12972/kjhst.20180072
Silué, Y., Nindjin, C., Cissé, M., Kouamé, K. A., Mbéguié-A-Mbéguié, D., Lopez-Lauri, F., et al. (2022). Hexanal application reduces postharvest losses of mango (Mangifera indica L. variety “Kent”) over cold storage whilst maintaining fruit quality. Postharvest Biol. Technol. 189:111930. doi: 10.1016/j.postharvbio.2022.111930
Singleton, V. L., Orthofer, R., and Lamuela-Raventós, R. M. (1999). [14] analysis of total phenols and other oxidation substrates and antioxidants by means of folin-ciocalteu reagent. Oxid. Antioxid. A. 299, 152–178. doi: 10.1016/S0076-6879(99)99017-1
Tiwari, K., and Paliyath, G. (2011). Microarray analysis of ripening-regulated gene expression and its modulation by 1-MCP and hexanal. Plant Physiol. Biochem. 49, 329–340. doi: 10.1016/j.plaphy.2011.01.007
Valero, E., Varon, R., and Garcia-Carmona, F. (1990). Inhibition of grape polyphenol oxidase by several natural aliphatic alcohols. J. Agric. Food Chem. 38, 1097–1100. doi: 10.1021/jf00094a042
Wang, Y., Li, Y., Yang, S., Wu, Z., and Shen, Y. (2022). Gaseous ozone treatment prolongs the shelf-life of fresh-cut kiwifruit by maintaining its ascorbic acid content. LWT 172:114196. doi: 10.1016/j.lwt.2022.114196
Xia, H., Wang, X., Su, W. Y., Jiang, L. J., Lin, L. J., Deng, Q. X., et al. (2020). Changes in the carotenoids profile of two yellow-fleshed kiwifruit cultivars during storage. Postharvest Biol. Technol. 164:111162. doi: 10.1016/j.postharvbio.2020.111162
Xu, D., Zhou, F., Gu, S., Feng, K., Hu, W., Zhang, J., et al. (2021). 1-Methylcyclopropene maintains the postharvest quality of hardy kiwifruit (Actinidia aruguta). J. Food Meas. Character. 15, 3036–3044. doi: 10.1007/s11694-021-00893-y
Yadav, V., Wang, Z., Wei, C., Amo, A., Ahmed, B., Yang, X., et al. (2020). Phenylpropanoid pathway engineering: an emerging approach towards plant defense. Pathogens 9:312. doi: 10.3390/pathogens9040312
Yan, J., Cai, Z., Shen, Z., Zhang, B., Ma, R., and Yu, M. (2015). Extraction and analytical methods of carotenoids in fruit of yellow flesh peach. J. Fruit Sci. 32, 1267–1274.
Keywords: hexanal, postharvest quality, maturation, antioxidant capacity, gold kiwifruit
Citation: Mthembu SSL, Magwaza LS, Tesfay SZ and Mditshwa A (2023) Mechanism of enhanced freshness formulation in optimizing antioxidant retention of gold kiwifruit (Actinidia chinensis) harvested at two maturity stages. Front. Sustain. Food Syst. 7:1286677. doi: 10.3389/fsufs.2023.1286677
Edited by:
Muhammad Faisal Manzoor, Foshan University, ChinaReviewed by:
Waseem Khalid, Government College University, PakistanMaría Emma García Pastor, Miguel Hernández University of Elche, Spain
Copyright © 2023 Mthembu, Magwaza, Tesfay and Mditshwa. This is an open-access article distributed under the terms of the Creative Commons Attribution License (CC BY). The use, distribution or reproduction in other forums is permitted, provided the original author(s) and the copyright owner(s) are credited and that the original publication in this journal is cited, in accordance with accepted academic practice. No use, distribution or reproduction is permitted which does not comply with these terms.
*Correspondence: Asanda Mditshwa, mditshwaa@ukzn.ac.za