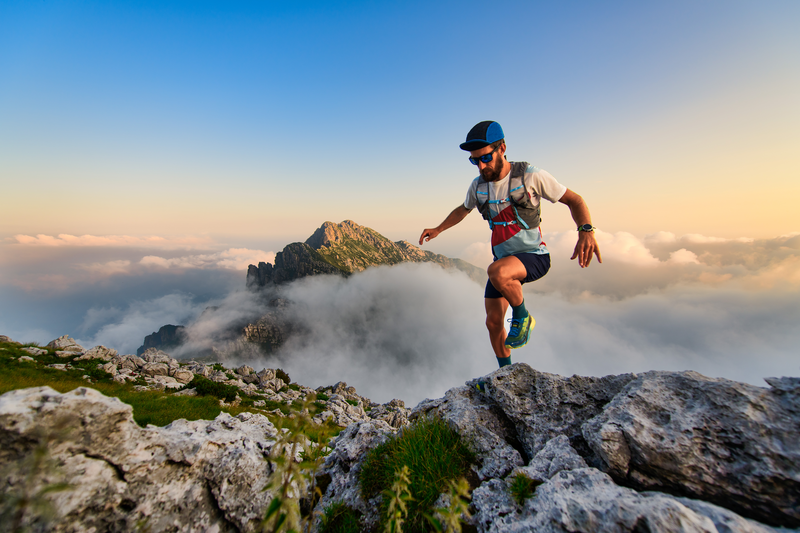
94% of researchers rate our articles as excellent or good
Learn more about the work of our research integrity team to safeguard the quality of each article we publish.
Find out more
ORIGINAL RESEARCH article
Front. Sustain. Food Syst. , 04 January 2024
Sec. Nutrition and Sustainable Diets
Volume 7 - 2023 | https://doi.org/10.3389/fsufs.2023.1282427
Common bean (Phaseolus vulgaris L.) is a crop of high nutritional interest which is widespread throughout the world as a result of which it is considered as a potential crop for ensuring future food security. The effect of organic and conventional farming systems on the nutritional and functional composition of two cultivars of market class fabada (a landrace and a modern elite cultivar) is described. The findings of our study have shown that the common beans from organic farming have a higher content of protein, phenolic compounds, and relevant microelements such as iron and zinc than the seeds from conventional farming trial. However, in conventional crops a higher content of polyunsaturated fatty acids and lower concentrations of phytic acid and raffinose oligosaccharides were also detected. In contrast, no significant differences were detected in the proximate composition of the two genotypes tested, nor in their antioxidant activity or levels of phenolic compounds. The elite cultivar maintains the nutritional characteristics of the landrace from which it derives, suggesting the genomic regions modified in the improvement do not play an important role in their genetic control.
Common bean (Phaseolus vulgaris L.) is one of the most important grain legumes and is cultivated widely throughout the world (FAO, Food and Agriculture Organization of the United Nations, 2023a). Bean seeds are interesting due to their nutritional composition, characterized by a high content of protein, carbohydrates, vitamins, minerals, and the presence of functional compounds such as dietary fiber and phenolic compounds, which exert protective effects against various diseases (Bennink and Rondini, 2008; Câmara et al., 2013; Hayat et al., 2014; Lo Turco et al., 2016; Chávez-Mendoza and Sánchez, 2017). Therefore, this legume is considered a potential crop for ensuring future food security (Parodi et al., 2018; Nadeem et al., 2021), supplying vegetable protein to the increased world population, especially in Africa and developing countries, and mitigating obesity in developed countries due to its low glycemic index. In addition, legume crops have environmental benefits. For instance, legumes have the unique capacity to fix nitrogen in the soil and hence improve soil fertility, while at the same time reducing the need to use conventional inorganic fertilizers and the crop has a small water footprint. For these reasons, legumes have an important role to play in the transition toward more sustainable farming systems that provide economic, environmental, and social benefits.
Agriculture is a significant contributor of greenhouse gas emissions (CO2, methane and nitrous oxide) related to climatic change (Muller et al., 2017). A reduction in agricultural emissions (largely methane and nitrous oxide) could play a significant role in climate change mitigation (Lynch et al., 2021). Organic agriculture is an integrated production management system that promotes and enhances agro-ecosystem health, including biodiversity, biological cycles, and soil biological activity. It emphasizes the use of natural inputs (i.e., minerals and products derived from plants) and the renunciation of synthetic fertilizers and pesticides (FAO, Food and Agriculture Organization of the United Nations, 2023b). This means that organic farming tends to have a limited environmental impact and, owing to the absence of synthetic inputs, organic produce is generally considered to be safer and healthy (Aulakh et al., 2022).
On the other hand, it is well-known that agronomic factors such as the type and fertility of the soil, climatic variables, plant management, and cultivars used have an effect on the nutritional and functional value of common bean seeds (González et al., 2006; Uyanöz et al., 2007; Medina-Velo et al., 2017; Celmeli et al., 2018; Nicoletto et al., 2019; Philipo et al., 2020; Bosmali et al., 2023). However, sometimes there are discrepancies in the findings of different works studying the same aspect, which could be due to the difficulty of reproducing the tests and interactions among the various factors evaluated (genotype, climatic conditions, soil, etc.).
In this sense, although it seems clear that organic farming is a sustainable alternative to conventional farming, and its positive influence on some dry bean phytochemicals has been revealed, such as a higher content of phenolic compounds (Barański et al., 2014; Mastura et al., 2017; Barreto et al., 2021; Bosmali et al., 2023), what is certain that in other cases, the results are still inconclusive, as Barreto et al. (2021) point out. One possible cause of this lack of consistency that should be pointed out is the fact that, on the one hand, there are in fact still very few works that study differences in organic and conventional production and, on the other, the works that do exist generally consist of a comparison of values for commercial samples, and do not take into account the diversity of factors like cultivated variety, environmental conditions, fertilizers, land management, etc. These works, which are undoubtedly of interest from the point of view of product characterization, do not however allow information to be obtained on the direct influence of the farming method (organic vs. conventional) on the nutritional properties of dry beans.
Seeds of common bean show wide phenotypic variation (coat color, color distribution, seed form, and size). On the basis of seed phenotypes, the diverse range of bean have been grouped into different market classes (Voysest, 2000). The market class fabada demonstrates an extreme seed phenotype within the species. Its growth was originally described in Asturias (northern Spain) and currently has a Protected Geographical Indication recognized by the EU, due to the particular quality, reputation and characteristics attributable to the geographical origin (MAPA, Ministerio de Agricultura, Pesca y Alimentación, 2023). However, a detailed description of the seed's chemical composition and nutritional characteristics has not been reported under local farming conditions.
The goal of this work is to describe the nutritional and functional composition of bean seeds of the market class fabada as well as to investigate the effect of organic or conventional farming systems on those parameters. To this end, the proximate composition, total phenolic compounds, antioxidant capacity, sugars, fatty acid profile, phytic acid and mineral content were determined in a landrace and an elite cultivar of this market class.
The homozygous lines A25 and A4804 were used in this study (Supplementary Figure 1). Both lines (genotypes) have an indeterminate climbing growth habit and are classified in the market class fabada (syn. Faba Granja), a well-differentiated seed phenotype within the dry beans, with creamy white, very large seeds (~100 g/100 seeds) that have an oblong shape and a length/width ratio >2.2. Line A25 (cv. Andecha) is an old cultivar obtained through a local landrace selection (Ferreira et al., 2012). Line A4804 derives from A25 through backcrossing programs and it carries various resistance genes: it carries the Co-2 gene located at chromosome Pv11 which confers resistance to anthracnose, the Pm-1 gene, located at chromosome Pv04, conferring resistance to powdery mildew, and the I gene, located at chromosome Pv02, which confers resistance to potyvirus.
The crops were grown in Villaviciosa (Asturias, Spain; 3°29′ 01″ N, 5° 26′ 11″ W; elevation 6.5 m) from April to September 2022. A completely randomized design was used in this study comprising four replicates per farming system/crop management type (conventional or organic) and cultivar (A25 and A4804). In the organic farming system, the crop was grown in a field trial of 1 m2 plots which each contained 8–10 plants, while the conventional farming system took place in a tunnel greenhouse with plots of 2 m2 containing 10–14 plants. Both crops were mulched with plastic for weed control. The conventional trial took place in soil that had been intensively used for bean growth for the last 15 years (two cycles per year) on which synthetic fertilizers and pesticides had been used. The organic trial was performed in soil that had been used for a common bean-ryegrass rotation system for the previous 8 years (bean in summer and ryegrass in winter, the ryegrass being incorporated into the soil as green manure), whose only crop management was treatment with organic fertilizers and pesticides.
Analysis of the soil characteristics of the conventional and organic trials were conducted by the company KUDAM (Laboratorio Kudam S.L., Alicante, Spain). Each soil was sampled after harvesting using a shovel at a depth of 40–50 cm. Each soil sample was a mix of 8–10 subsamples spread across the respective plots in order to capture its variability. The main physical-chemical characteristics of the soils are described in Supplementary Table 1.
The dry pods from each plot were manually harvested and shelled. The dry seeds were frozen for 48 h to prevent weevil attack and then kept at room temperature before being processed. Samples of 50 g of seeds per line and plot were ground in a coffee grinder and passed through a standard sieve (number 18, corresponding to a sieve open ring size of 1.00 mm). Each repetition was individually analyzed and the final value per line and per field condition was calculated as the arithmetic mean of the four repetitions.
Moisture was determined by drying at 105°C, ash by incineration at 550°C, crude protein with the Kjeldahl method and total fat with the Shoxlet method, all according to AOAC methods (Horwitz, 2005). Total carbohydrates and energy were indirectly calculated in accordance with FAO, Food and Agriculture Organization of the United Nations (2023c). Total carbohydrate (%) was estimated using the difference method as follows:
and the energy content was calculated as follows:
Fatty acids, previously derivatized to fatty acid methyl esters, were analyzed by GC-MS following Rodríguez-Ruiz et al. (1998). Briefly, samples (100 mg) were placed in test tubes with 1 ml of the methylation mixture (methanol/acetyl chloride, 20:1 v/v) and 0.5 ml hexane and heated at 100°C for 30 min. After cooling to room temperature, 1 ml of distilled water was added to establish two phases. The upper one (hexane) was recovered and injected into an Agilent 7980 chromatograph equipped with an MSD 5975C detector (Palo Alto, CA, United States) and a J&W CP 7420 FAME column (100 m × 250 μm and 0.25-μm i.d). Oven conditions were as follows: 180°C (10 min) rising to 200°C (25 min) at a rate of 1°C/min and then to 240°C (10 min) at a rate of 15°C/min. Analyses were carried out in split mode (1/50) and the carrier gas flow (He) was set at 1 ml/min. The identity of the fatty acid methyl esters was ascertained by comparison with authentic standards. All analyses were carried out in duplicate.
The samples (0.5 g) were digested with 10 ml of concentrated nitric/hydrochloric acid (1/4 v/v) in an Ethos One microwave system (Milestone, Sorisole, Italy). Digestion conditions for the microwave system were as follows: increase in temperature up to 96°C in 3 min, up to 160°C in 10 min, up to 185°C in 3 min, after which temperature was kept constant for 15 min; finally, a cooling stage (30 min) was carried out to 25°C. The resulting solution was transferred to a 20 ml volumetric flask and diluted if necessary. Mineral determination was carried out by inductively coupled plasma mass spectrometry (ICP-MS, Agilent model 7800). The ICP-MS operating conditions were optimized using autotuning functions within the ICP-MS MassHunter software. Quantification of K, P, S, Mg, Ca, Fe, Zn, Cu, Mo, Ni, Mn, B, V, Co, As and Se was performed from an external calibration with 4 internal standards: scandium (Sc), germanium (Ge), iridium (Ir) and rhodium (Rh). All analyses were performed in duplicate.
Verbascose, stachyose, raffinose and sucrose were extracted following Gangola et al. (2014). Briefly, 1.5 g bean flour was extracted with 30 ml of 80% (v/v) ethanol in a shaking bath at 60°C for 45 min. The sample was centrifuged at 12,000 rpm for 10 min, after which the supernatant was collected and the pellet was re-extracted. Supernatants from 3 sequential extractions were pooled, dried at 50°C in a rotary vacuum evaporator, redissolved in 5 ml water and then filtered through a prewashed C18 cartridge and a 0.45 um acetate cellulose membrane. Two extractions were carried out per sample.
The determination of verbascose, stachyose, raffinose and sucrose in the extracts was carried out in an HPLC system (Waters Associates, Milford, MA) equipped with a 510 pump, a 717 plus autosampler, a temperature controller and a 410 refractive index detector. Separations were carried out in an cationic exchange column (Sugar-Pak I, Waters Associates) using an aqueous solution containing 50 mg/L of calcium salt of EDTA as the mobile phase at 0.2 ml/min. The injection volume was 50 μl and the oven temperature 90°C (Manoharlal et al., 2020). The identity of analytes was ascertained by comparison with authentic standards. Quantitation was performed according to an external standard method.
The extraction of phenolic compounds was carried out following the previously optimized method (Madrera and Valles, 2020). Briefly, 1.5 g bean flour were extracted with 30 ml of 46% aqueous ethanol (0.1% perchloric acid), over a period of 10.3 min, in a water bath at 20°C using an ultrasonic homogenizer UP200Ht (Hielscher, Teltow, Germany) equipped with a 2 mm diameter sonotrode at a frequency of 26 kHz. After extraction, the solids were separated by centrifugation and the supernatant was dried in a rotary vacuum evaporator at 40°C, after which the residue was reconstituted with 4 ml of 20% aqueous methanol (0.1% perchloric acid) and filtered through a 0.45 μm polyvinylidene difluoride (PVDF) syringe filter. Two extractions were carried out per sample.
Total phenolic content (TPC) was determined by spectrophotometry using the Folin–Ciocalteu method (Singleton and Rossi, 1965). The reaction was carried out in 10 ml volumetric flasks, to which the various reactants were added in the following order and quantities: 200 μl of phenolic extract, 5 ml of water, 250 μl of Folin–Ciocalteu reagent, 750 μl of 20% sodium carbonate and water to reach the final volume. The absorbance was measured at 700 nm after 30 min at room temperature. Gallic acid was used as standard for the quantification of total phenolic compounds. The results are expressed as μg gallic acid equivalent (GAE)/g. All extracts were analyzed in duplicate.
Reducing power was carried out by the ferric reducing antioxidant power (FRAP) method, following Benzie and Strain (1999). Working FRAP reagent was prepared daily from the following three solutions in the ratio 10:1:1: 300 mM acetate, pH 3.6; 10 mM TPZ (2,4,6-tripyridyl-s-triazine) in 40 mM HCl and 20 mM FeCl3·6H2O. Briefly, 100 μl of appropriately diluted extracts were mixed with 3.0 ml working FRAP reagent in a test tube and the absorbance was read at 593 nm against a reagent blank after 20 min at room temperature. FeSO4·7H2O solutions were used to construct a standard curve and the results were expressed as μmol Fe (II)/g. All extracts were analyzed in triplicate.
Radical scavenging activity was determined by using the 2,2-diphenyl-1-picryl-hydrazyl-hydrate (DPPH) method of Diñeiro García et al. (2009). Forty microliter of either appropriately diluted extract (the standard or methanol in the case of the reagent blank) were added to 1.460 ml of DPPH solution (1 × 10−4 M) in methanol. Samples were diluted with methanol to ensure that the readings were in the linear range of the standard curve. Absorbance at 515 nm was measured after 120 min, when the reaction had reached its stable state. The inhibition percentage (IP) was calculated as follows:
where Asample is the absorbance of the solution in its stable state and Ablank is the absorbance of the DPPH solution when methanol was added rather than the sample. Trolox solutions were used to construct a standard curve and the results were expressed as μmol trolox equivalent (TE)/g. All extracts were analyzed in triplicate.
Phytic acid was determined with the K-PHYT test kit (Megazyme International Ltd, Bray, Ireland) in dry beans following the instruction detailed by manufacturer. Briefly, 1 g of bean flour was extracted overnight with 20 ml of 0.66N HCl by shaking vigorously at room temperature. Next, an aliquot was centrifuged at 13,000 rpm for 10 min, following which 0.5 ml of the sobrenatant was taken and neutralized with 0.5 ml of 0.75N NaOH. A phytase was added to the neutralized extract followed by an alkaline phosphatase and trichloroacetic acid to release the phosphorus from the sample. Finally, the total phosphorus and the free phosphorus in the sample were determined by spectrophotometry at an absorbance of 655 nm after derivatization with ascorbic acid and ammonium molybdate to form molybdenum blue. The difference between the free phosphorus and the total phosphorus allowed the estimation of the phytic acid content in the sample (Megazyme, 2023).
Significant differences in the nutritional and functional composition were detected by means of a two-factor ANOVA (genotype and farming system). Pearson's correlation coefficient (r) was computed to estimate correlations between variables. The program used was SPSS version 15.0 (SPSS Inc., Chicago, IL, USA).
From the nutritional point of view, there were no significant differences between genotypes (Table 1), with average values being within those characteristic for the species (Admassu Shimelis and Kumar Rakshit, 2005; De Almeida Costa et al., 2006; Celmeli et al., 2018; Wodajo and Emire, 2022). However, protein, ash, carbohydrate and energy contents did show significant differences with respect to the farming system employed. Specifically, the protein content, one of the main nutritional traits of common beans, was significantly higher (14% on average) in the organic crop, as was ash content (>9%), while carbohydrate content was higher (>5%) in samples from conventional farming.
Table 1. Proximate composition of common beans culivated under organic or conventional conditions (mean ± standard deviation).
Several works have reported differences in nutritional values due to both the farming system and the cultivars tested (Shellie and Hosfield, 1991; González et al., 2006; Kargiotidou et al., 2019; Herrera et al., 2021). Higher protein content has been associated with higher levels of nitrogen in the soil, although the genotype effect seems more important (Leal et al., 2021). In this sense, it should be noted that the organic soil employed in this work presented a higher total nitrogen (Supplementary Table 1), although it is also true that higher protein levels have been also reported in organic crops with lower total nitrogen than the substrate where the equivalent crop is grown under conventional conditions (the fixation of atmospheric nitrogen through symbiosis with rhizobium bacteria and the absence of pesticides perhaps favoring the accumulation of protein in such conditions) (Barreto et al., 2021). Contrary to Barreto et al. (2021), our results show a higher ash content in organic crops, which could be interesting from a culinary point of view because, as Velasco-González et al. (2013) pointed out, higher ash content favors water absorption and reduces the final hardness of the grains.
It is worth noting that it is usual to observe different behaviors between genotypes for the same growing conditions (genotype x environmental interaction), so it is not always possible to extrapolate from published results and it is necessary to replicate works under the specific conditions of interest.
Phenolic compounds are a family of phytochemicals present in dry beans that play an important role in human health because they exhibit antioxidant activity related to antidiabetic, antiobesity, anti-inflammatory, antimutagenic and anticarcinogenic properties (Ganesan and Xu, 2017). Although the most important phenolic compounds, from the functional point of view, are found in the colored seeds as a result of the greater accumulation of flavonols present in their coat, the phenolic components of the cotyledons, basically phenolic acids, constitute a group of important nutraceuticals because of their antioxidant activity (Rodríguez Madrera et al., 2020).
The results obtained (Table 2) showed a similar TPC in both genotypes, which were within the characteristic ranges for this market class (Rodríguez Madrera et al., 2021). However, the accumulation of phenolic compounds in organic crop was 26.6% higher than conventional one. A higher content of polyphenols was reported in black varieties grown in organic farming system (Celmeli et al., 2018). The formation of polyphenols is associated with response to stress and numerous causes have been suggestedsuch as the water level and the temperature (Juszczuk et al., 2004; Nicoletto et al., 2019; Herrera et al., 2021). Although in our trial both crops were subjected to a period of stress due to unusually high temperatures during the seed development stage (Supplementary Table 2), this does not seem to justify the differences in phenolic content between crops. However the characteristics of the soil could be the explanation for the differences detected. Juszczuk et al. (2004) observed that phosphorus deficiency induces a greater production of polyphenols in the P. vulgaris plant, and Oney-Montalvo et al. (2020) detected that in soils with a higher content of nitrogen and organic matter and less phosphorus (Supplementary Table 1), higher levels of phenolics compounds are metabolized in habanero peppers.
Table 2. Total phenolic content and antioxidant activity of common beans culivated under organic or conventional conditions (mean ± standard deviation).
The antioxidant activity of dry beans was evaluated using two complementary methods: the DPPH method, which determines radical scavenging activity, and the FRAP method, which evaluates the reducing power of the extracts. The DPPH method reflected higher antioxidant power in the extracts of seeds grown under organic conditions (70% higher), in accord with Mastura et al. (2017) in P. vulgaris and a review by Barański et al. (2014) in relation to crops/crop-based foods; on the contrary, there were no significant differences in the reducing power of the two conditions. The apparent discrepancies between the results of the two methods can be explained by taking into account that the different phenolic compounds present in the extracts are expressed with different intensity depending on the method employed (Alcalde et al., 2019), which is linked to the fact that the methods used are based on different mechanisms: FRAP is based on single electron transfer and DPPH is based on a mixed mechanism of hydrogen atom transfer and single electron transfer (Pisoschi et al., 2016). In addition, the TPC values determined by the Folin-Ciocalteu method, a method also based on single electron transfer, presented a lower correlation coefficient with the FRAP values (rTPC/FRAP = 0.862) than with the DPPH values (rTPC/DPPH = 0.920), again revealing the differences in the response of the extracted components and the methods used. In this sense, the characterization of the phenolic content and antioxidant activity of a panel of dry beans showed that polyphenol content does not always correlate with reducing power or radical scavenging activity, indicating that using more than one methods seems necessary to adequately define the antioxidant activity of dry beans (Rodríguez Madrera et al., 2021).
Fatty acids (FAs) are functional components of the human diet that influence metabolic diseases such as type 2 diabetes and inflammatory diseases such as arthritis, and cancer (Calder, 2015).
Significant differences were found in some of the factors studied for the 12 FAs detected, of particular note being the differences detected for the four major FAs: palmitic acid, oleic acid, linoleic acid and linolenic acid (Table 3), which represented more than 95% of the total FAs, in accordance with previously reported results for dry beans (Lo Turco et al., 2016; Adewole et al., 2021; Byrdwell and Goldschmidt, 2022).
Table 3. Fatty acid profile (expressed as percentage of total fatty acids) of common beans culivated under organic or conventional conditions (mean ± standard deviation).
Palmitic acid was the only FA that did not show significant differences with the farming system used, while oleic, linoleic and linolenic acids, which demonstrated the greatest changes from a quantitative point of view, all showed significant differences for both genotype and farming system, as well as their interaction. Both genotypes of dry bean presented higher oleic acid contents in organic farming, the linolenic acid content was higher in conventional crops and linoleic acid was more abundant in conventional farming for the A4804 genotype and in organic farming for A25.
On the other hand, the content of saturated fatty acids and unsaturated fatty acids did not show significant differences between farming systems (Supplementary Figure 2). However, there was a greater accumulation of polyunsaturated fatty acids (PUFA) than mono-unsaturated fatty acids (MUFA) in the conventional crop (Figure 1). These facts could be explained by the desaturation of FAs by fatty acid desaturases (FADs), a highly substrate specific group of enzyme present in plants (Dong et al., 2016). The results obtained show that in conventional farming there was greater activity of FAD2 and FAD6, both ω-6 desaturases that synthesize linoleic acid (18:2) from oleic acid (18:1), and FAD3, FAD7 and FAD8, both ω-3 desaturases that convert linoleic acid (18:2) into linolenic acid (18:3) (Dong et al., 2016). In addition, from a nutritional point of view, the presence of linoleic acid (ω-6) and linolenic acid (ω-3) is of special interest, given that the human body is unable to synthesize these PUFAs and thus they are referred to as essential FAs. However, overconsumption of ω-6 PUFAs with a concomitant low intake of ω-3 PUFAs is strongly associated with the pathogenesis of many modern diet-related chronic diseases, currently health recommendations are to reduce the current ω-6/ω-3 ratio from 20–50/1 to 4–5/1 (Mariamenatu and Abdu, 2021). In this sense, although the fat content of dry beans is low, the higher levels of ω-3 and the ω-6/ω-3 ratios of < 1 detected in the common beans (Figure 2) could contribute to decreasing this consumption ratio.
Figure 1. Sum of mono-unsaturated fatty acids (MUFA) and polyunsaturated (PUFA) fatty acids in common beans grown under organic and conventional conditions.
The human diet needs to include relatively small amounts of the minerals that cannot be synthesized by the body. Minerals fulfill numerous functions: they are part of all body fluids, participate in the maintenance of osmotic pressure, are involved in the formation of bones and tissues as well as in nerve impulse transmission, and act as cofactors with various enzymes, among other functions. The lack of certain elements such as Fe, Zn, and P is the cause of important nutrition problems (malnutrition) and, on occasions, this can become a health public problem (Latham, 1997). Minerals can be classified according to the concentrations required by the body into macrominerals (quantities >100 mg/day, Ca, P, Na and Cl), microminerals (concentrations < 100 mg/day, Fe, Cu, Co, K, Mg, I, Zn, Mn, Mo, F, Cr, Se, and S) and ultra-trace minerals (concentrations < 1 mg/day, B, Si, As, and Ni) (Soetan et al., 2010).
The analysis of the common bean samples showed values for the different minerals within the range of those reported by other authors for the species (Guzmán-Maldonado et al., 2003; Ribeiro et al., 2012; Dutta et al., 2016; Espinoza-García et al., 2016; Kahraman et al., 2017; Celmeli et al., 2018; Palčić et al., 2018; Gunjača et al., 2021). In all cases (Table 4), K was the most abundant mineral component, constituting between 1.5 and 1.7% by weight of the seeds. Other major elements, in decreasing order, were P (0.43%−0.54%), S (0.20%−0.27%), Mg (0.15%−0.17%), and Ca (0.08%−0.15%). Seven minerals were found with values higher than mg/kg: Fe (50.68–66.94 mg/kg), Zn (25.17–39.69 mg/kg), Cu (8.52–11.25 mg/kg), Mo (4.35–5.74 mg/kg), Ni (0.75–1.78 mg/kg), Mn (11.39–14.06 mg/kg), and B (5.89–6.85 mg/kg) and five other were lower than mg/kg: V (80.24–97.4 μg/kg), Co 34.59–90.06 μg/kg), As (29.61–33.29 μg/kg), and Se (35.97–82.59 μg/kg). The 16 elements analyzed showed significant differences for at least some of the factors studied (genotype, farming system). Regarding the differences detected between genotypes, it is worth highlighting the higher fixation of Se (cofactor of antioxidant enzymes) in the A25 genotype (average: 69 μg/kg), 75% higher than was fixed by A4804 (average: 39.1 μg/kg). Celmeli et al. (2018) have described two–three-fold differences in levels of Se between genotypes.
Table 4. Mineral content in common beans culivated under organic or conventional conditions (mean ± standard deviation).
A significant interaction was detected between genotype by farming system with respect to the content of 10 of the analyzed elements (Table 4). It is important to note that only Mo levels showed opposing trends with respect to farming system depending on the genotype. For the remaining minerals where the farming system factor was significant, both genotypes behaved in the same way: P, S, V, Mn, Co, Ni, As, B, K, Ca, Fe, Zn were more abundant in dry beans grown according to organic practices (with increases that ranged between 2% for K and 83% for Ca) and the conventional crops accumulated higher levels of Mg and Cu (8% and 16% respectively). Although with small differences, Akbaba et al. (2012) obtained similar results, with higher levels of Ca, Fe, Mn, P, Zn, K, Mg in haricot beans samples grown under an organic farming regime and no detected differences for Cu and S. However, Uyanöz et al. (2007) detected lower Fe content in organically grown dry beans (cattle manure) compared to the control (conventional) crop and another substrate fertilized with ammonium sulfate, and they detected no differences for Cu, Mn, Zn, P, Ca, K, or Mg. Barreto et al. (2021) detected significant differences in levels of K, Cu, Mn, Ca, Na, and Zn between organic and non-organic crops, although for some elements the detected variations differed depending on the growing region (environment effect). In this sense, it should be noted that the genotype x environment interaction in experimental designs such as that used in the current work and the those mentioned above has considerable weight, one study finding that the sum of squares of the genotype x environment interaction represented more than 26% of variation for Fe and Zn (Philipo et al., 2020), and this could well be the cause of the discrepancies between the results in different trials.
In this respect, the large number of parameters that have a significant influence on the mineral content of dry bean should be noted. These include available phosphorus, iron, silt %, sand %, exchangeable potassium, clay and total soil nitrogen (Philipo et al., 2020), and sometimes these factors demonstrating opposite effects within the same trial, which makes it difficult to interpret the results and extrapolate the conclusions beyond the scope of a specific experimental design. In other words, the very high number of factors that seem to play an important, and sometimes opposite, role when considering general factors such as environmental conditions, including parameters with various effects and origins that are sometimes random (soil, weather conditions), could be the cause of the interactions detected in the studies and also of the discrepancies that are sometimes observed between results.
In addition, a significant (p < 0.01) and positive correlation was detected between the available mineral content of the soils and the seeds harvested (Table 4, Supplementary Table 1) for Cu (r = 0.63), Fe (r = 0.85), Mn (r = 0.93), Mg (r = 0.78), Ca (r = 0.95), along with a negative effect for B (r = −0.93), P (r = −0.70) and Zn (r = −0.83), while no significant correlation was detected for K content. Plant intrinsic and extrinsic factors have been suggested to be the cause of the lack of correlation between the mineral content of beans and the soils in which they are grown (Barreto et al., 2021).
There are three main RFOs present in legumes: raffinose (Gal(a1–6)Glc(a1–2b)Fruf), stachyose (Gal(a1–6)Gal(a1–6)Glc(a1–2b)Fruf) and verbascose (Gal(a1–6)Gal(a1–6)Gal(a1–6)Glc(a1–2b)Fruf). Stacchyose and the disaccaharyde sucrose (Glc(a1–2b)Fruf) are the principal soluble sugars in dry bean.
In all the samples analyzed, sucrose was the major sugar, followed by stachyose, raffinose and verbascose (Table 5). In general, these values are within the range of those reported in different varieties of dry beans (Naivikul and D'Appolonia, 1978; McPhee et al., 2002; Admassu Shimelis and Kumar Rakshit, 2005; Nicoletto et al., 2019; Kotha et al., 2020).
Table 5. Sugars (mg/kg) in common beans culivated under organic or conventional conditions (mean ± standard deviation).
In accordance with other authors, the genotype is, together with the location of growth, a main cause of differences in the content of RFO and sucrose (Admassu Shimelis and Kumar Rakshit, 2005). In our study, the genotype factor was significant for the content of sucrose, stacchyose and raffinose, the levels of the three saccharides being higher in the A25 genotype than in the A4804. Moreover, the farming system was also significant with respect to raffinose and sucrose levels, both saccharides being more abundant (28 and 47% respectively) in organic crops. Consequently, the sum of ROFs and total sugars were significantly higher (p < 0.01) in the assays with A25 (Supplementary Figure 3), with a difference of 70% between the total sugar content in A4804 conventional (28.2 g/kg) and that of A25 organic (47.9 g/kg). A higher sugar content in organic crops has also been detected for green beans (El-Nafad et al., 2022) and courgette (Kopczyńska et al., 2021).
Kotha et al. (2020) proposed an MD (mono-saccharides and disaccharides) to RFO ratio, as a direct reference to the flatulence problems related to dry bean consumption, flatulence problems being higher the higher the MD/RFO ratio. According to this criterion, in our assay, organic crops (ratio = 1.37) could cause fewer problems than conventional crops (ratio = 0.98).
Phytic acid (PA, myo-inositol hexakisphosphate) is a form of phosphorus and energy reserves in plants, especially in legumes. The powerful chelating capacity of the six phosphate groups of this molecule, together with the absence of phytases in monogastric animals, has led to phytic acid being considered one of the main antinutrients in dry beans. However, this high capacity to form complexes has also been associated with possible health benefits such as reducing the risk of heart disease and cancer, and inhibiting the formation of kidney stones (Feizollahi et al., 2021).
All the samples in this trial showed a PA content in the range 10.3–13.2 mg/g (Figure 3), in line with values reported in different genotypes of this species and similar to other legumes (Sinkovič et al., 2022). In addition, both the genotype (p < 0.05) and the farming system (p < 0.01) were significant with respect to the PA content, with differences of up to 33% found between the trials A25 conventional and A4804 organic. Caproni et al. (2020) showed the influence of the genotype factor in a study of the PA content in a collection of 192 European genotypes of common beans, with values between 4.8–19.9 mg/g, and Wang et al. (2017) reported a phytic acid range between 8.6–17.1 mg/g in 20 cultivars, along with significant differences due to growing location and farming year.
Figure 3. Phytic acid (mg/g) and molar ratios in common beans grown under organic and conventional conditions. PA, phytic acid.
The consideration of PA as an antinutrient in food can be evaluated, in addition to its absolute content, in terms of its concentration relative to the minerals on which it acts as a chelating agent. For this reason, in practice, the molar ratios of phytate:mineral are used as an indicator of the bioavailability of the minerals, this being higher the lower the ratio. Thus, as an example, maximum molar ratios of 15 have been suggested for PA:Zn and 1 for PA:Fe (Hurrell, 2004; Umeta et al., 2005). In line with data reported for raw and cooked common beans (Castro-Alba et al., 2019; Hummel et al., 2020; Jepleting et al., 2022; Wafula et al., 2022), the estimated values for the molar ratios of the tested samples in this study were in all cases higher than the recommended values (Figure 3). However, PA:Zn and PA:Fe molar ratios showed significant differences depending on the genotype (p < 0.01), and, furthermore, the farming system did not influence the PA:Zn ratio although for the PA:Fe ratio, a different trend was found depending on the genotype (significant interaction) (p < 0.01), with a lower ratio in conventional farming for the A4804 genotype and in organic farming for the A25 genotype.
The results indicate that the content of different nutritional and functional parameters of the common bean market class fabada is dependent on the farming system employed and that the appropriate management of the practices can favor the production of crops with a better value from a food point of view. In this sense, our findings show a higher content of protein, phenolic compounds and important microelements such as Fe and Zn in organic crops, while conventional crops have a higher content of polyunsaturated fatty acids and lower concentrations of phytic acid and raffinose oligosaccharides. In contrast, no significant differences were detected in the proximate composition of the two genotypes tested, nor in their antioxidant activity or total phenolic compounds, and although significant differences were detected for some parameters such as several minerals and fatty acids, these differences do not seem relevant from a nutritional point of view. Therefore, it can be established that in general the elite genotype (A4804) retains the nutritional characteristics of the traditional genotype (A25) from which it derived. However, the interaction detected between the genotype and the farming system make it difficult to generalize about optimal management practices and should be adapted to the particularities of each growing region and environmental conditions.
The original contributions presented in the study are included in the article/Supplementary material, further inquiries can be directed to the corresponding author.
RR: Conceptualization, Formal analysis, Investigation, Methodology, Writing—original draft. AC: Formal analysis, Project administration, Resources, Validation, Visualization, Writing—review & editing. JF: Formal analysis, Funding acquisition, Resources, Validation, Visualization, Writing—review & editing.
The author(s) declare financial support was received for the research, authorship, and/or publication of this article. This work was supported in part by grant PID 2021-123919 funded by MCIN/AEI/10.13039/501100011033 and by ERDF A way of making Europe and by grant IDI/2021/000104 (GRUPIN: PCTI 2021–2023) funded by the Government of Principado de Asturias and by ERDF A way of making Europe.
The authors declare that the research was conducted in the absence of any commercial or financial relationships that could be construed as a potential conflict of interest.
All claims expressed in this article are solely those of the authors and do not necessarily represent those of their affiliated organizations, or those of the publisher, the editors and the reviewers. Any product that may be evaluated in this article, or claim that may be made by its manufacturer, is not guaranteed or endorsed by the publisher.
The Supplementary Material for this article can be found online at: https://www.frontiersin.org/articles/10.3389/fsufs.2023.1282427/full#supplementary-material
Adewole, E., Ojo, A., Oludoro, O., and Osasona, I. (2021). Fatty acid profiles of phaseolus species. Food Res. 5, 131–135. doi: 10.26656/fr.2017.5(5).439
Admassu Shimelis, E., and Kumar Rakshit, S. (2005). Antinutritional factors and in vitro protein digestibility of improved haricot bean (Phaseolus vulgaris L.) varieties grown in Ethiopia. Int. J. Food Sci. Nutr. 56, 377–387. doi: 10.1080/09637480500512930
Akbaba, U., Sahin, Y., and Türkez, H. (2012). Comparison of element contents in haricot beans grown under organic and conventional farming regimes for human nutrition and health. Acta Sci. Pol. Hortorum Cultus 11, 117–125. doi: 10.3906/fiz-1107-12
Alcalde, B., Granados, M., and Saurina, J. (2019). Exploring the antioxidant features of polyphenols by spectroscopic and electrochemical methods. Antioxidants 8, 523. doi: 10.3390/antiox8110523
Aulakh, C. S., Sharma, S., Thakur, M., and Kaur, P. (2022). A review of the influences of organic farming on soil quality, crop productivity and produce quality. J. Plant Nutr. 45, 1884–1905. doi: 10.1080/01904167.2022.2027976
Barański, M., Srednicka-Tober, D., Volakakis, N., Seal, C., Sanderson, R., Stewart, G. B., et al. (2014). Higher antioxidant and lower cadmium concentrations and lower incidence of pesticide residues in organically grown crops: a systematic literature review and meta-analyses. Br. J. Nutr. 112, 794–811. doi: 10.1017/S0007114514001366
Barreto, N. M. B., Pimenta, N. G., Braz, B. F., Freire, A. S., Santelli, R. E., Oliveira, A. C., et al. (2021). Organic black beans (Phaseolus vulgaris L.) from Rio de Janeiro State, Brazil, present more phenolic compounds and better nutritional profile than nonorganic. Foods 10, 900. doi: 10.3390/foods10040900
Bennink, M., and Rondini, E. (2008). Beans and Health: A Comprehensive Review. Frazee, MN: The Bean Institute.
Benzie, I. F. F., and Strain, J. J. (1999). Ferric reducing/antioxidant power assay: direct measure of total antioxidant biological fluids and modified version for simultaneous measurement of total antioxidant power and ascorbic acid concentration. Method Enzymol. 299, 15–27. doi: 10.1016/S0076-6879(99)99005-5
Bosmali, I., Giannenas, I., Christophoridou, S., Ganos, C. G., Papadopoulos, A., Papathanasiou, F., et al. (2023). Microclimate and genotype impact on nutritional and antinutritional quality of locally adapted landraces of common bean (Phaseolus vulgaris L.). Foods 12, 1119. doi: 10.3390/foods12061119
Byrdwell, W. C., and Goldschmidt, R. J. (2022). Fatty acids of ten commonly consumed pulses. Molecules 27, 7260. doi: 10.3390/molecules27217260
Calder, P. C. (2015). Functional roles of fatty acids and their effects on human health. J. Parenter. Enteral. Nutr. 39, 18S−32S. doi: 10.1177/0148607115595980
Câmara, C., Urrea, C., and Schlegel, V. (2013). Pinto beans (Phaseolus vulgaris L.) as a functional food: implications on human health. Agriculture 3, 90–111. doi: 10.3390/agriculture3010090
Caproni, L., Raggi, L., Talsma, E. F., Wenzl, P., and Negri, V. (2020). European landrace diversity for common bean biofortification: a genome-wide association study. Sci. Rep. 10, 19775. doi: 10.1038/s41598-020-76417-3
Castro-Alba, V., Lazarte, C. E., Bergenståhl, B., and Granfeldt, Y. (2019). Phytate, iron, zinc, and calcium content of common bolivian foods and their estimated mineral bioavailability. Food Sci. Nutr. 7, 2854–2865. doi: 10.1002/fsn3.1127
Celmeli, T., Sari, H., Canci, H., Sari, D., Adak, A., Eker, T., et al. (2018). The nutritional content of common bean (Phaseolus vulgaris L.) landraces in comparison to modern varieties. Agronomy 8, 166. doi: 10.3390/agronomy8090166
Chávez-Mendoza, C., and Sánchez, E. (2017). Bioactive compounds from mexican varieties of the common bean (Phaseolus vulgaris): implications for health. Molecules 22, 1360. doi: 10.3390/molecules22081360
De Almeida Costa, G. E., da Silva Queiroz-Monici, K., Pissini Machado Reis, S. M., and de Oliveira, A. C. (2006). Chemical composition, dietary fibre and resistant starch contents of raw and cooked pea, common bean, chickpea and lentil legumes. Food Chem. 94, 327–330. doi: 10.1016/j.foodchem.2004.11.020
Diñeiro García, Y., Suárez Valles, B., and Picinelli Lobo, A. (2009). Phenolic and antioxidant composition of by-products from the cider industry. Food Chem. 117, 731–738. doi: 10.1016/j.foodchem.2009.04.049
Dong, C.-J., Cao, N., Zhang, Z.-G., and Shang, Q.-M. (2016). Characterization of the fatty acid desaturase genes in cucumber: structure, phylogeny, and expression patterns. PLoS ONE 11, e0149917. doi: 10.1371/journal.pone.0149917
Dutta, S. K., Chatterjee, D., Sarkar, D., Singh, S. B., Boopathi, T., Kuotsu, R., et al. (2016). Common Bean (Phaseolus vulgaris L., Fabaceae), landraces of Lushai Hills in India: nutrients and antioxidants source for the farmers. Indian J. Tradit. Knowl. 15, 313–320.
El-Nafad, R., El-Bastawesy, A., Ghoniem, G., and El-Refai, A. (2022). Effect of blanching process and frozen storage on bioactive compounds and some quality parameters of organic and conventional green bean. J. Food Dairy Sci. 13, 125–131. doi: 10.21608/jfds.2022.150884.1069
Espinoza-García, N., Martínez-Martínez, R., Chávez-Servia, J. L., Vera-Guzmán, A. M., Carrillo-Rodríguez, J. C., Heredia-García, E., et al. (2016). Contenido de minerales en semilla de poblaciones nativas de frijol común (Phaseolus vulgaris L.). Rev. Fitotec. Mex. 39, 215–223. doi: 10.35196/rfm.2016.3.215-223
FAO Food Agriculture Organization of the United Nations. (2023a). FAOSTAT. Crops and Livestock Products. Available online at: https://www.fao.org/faostat/en/#data/QCL (accessed July 16, 2023).
FAO Food Agriculture Organization of the United Nations. (2023b). Organinc Agriculture. Available online at: https://www.fao.org/organicag/oa-faq/oa-faq1/en (accessed July 16, 2023).
FAO Food Agriculture Organization of the United Nations. (2023c). Food Energy - Methods of Analysis and Conversion Factors. FAO Food and Nutrition Paper 77. Rome: FAO. Available online at: https://www.fao.org/3/y5022e/y5022e00.htm (accessed July 16, 2023).
Feizollahi, E., Mirmahdi, R. S., Zoghi, A., Zijlstra, R. T., Roopesh, M. S., Vasanthan, T., et al. (2021). Review of the beneficial and anti-nutritional qualities of phytic acid, and procedures for removing it from food products. Food Res. Int. 143, 110284. doi: 10.1016/j.foodres.2021.110284
Ferreira, J. J., Campa, A., Pérez-Vega, E., Rodríguez-Suárez, C., and Giraldez, R. (2012). Introgression and pyramiding into common bean market class fabada of genes conferring resistance to anthracnose and potyvirus. Theor. Appl. Genet. 124, 777–788. doi: 10.1007/s00122-011-1746-x
Ganesan, K., and Xu, B. (2017). Polyphenol-rich dry common beans (Phaseolus vulgaris L.) and their health benefits. Int. J. Mol. Sci. 18, 2331. doi: 10.3390/ijms18112331
Gangola, M. P., Jaiswal, S., Khedikar, Y. P., and Chibbar, R. N. A. (2014). Reliable and rapid method for soluble sugars and RFO analysis in chickpea using HPAEC–PAD and its comparison with HPLC–RI. Food Chem. 154, 127–133. doi: 10.1016/j.foodchem.2013.12.085
González, A. M., Monteagudo, A. B., Casquero, P. A., De Ron, A. M., and Santalla, M. (2006). Genetic variation and environmental effects on agronomical and commercial quality traits in the main European market classes of dry bean. Field Crops Res. 95, 336–347. doi: 10.1016/j.fcr.2005.04.004
Gunjača, J., Carović-Stanko, K., Lazarević, B., Vidak, M., Petek, M., Liber, Z., et al. (2021). Genome-wide association studies of mineral content in common bean. Front. Plant Sci. 12, 636484. doi: 10.3389/fpls.2021.636484
Guzmán-Maldonado, S. H., Martínez, O., Acosta-Gallegos, J. A., Guevara-Lara, F., and Paredes-López, O. (2003). Putative quantitative trait loci for physical and chemical components of common bean. Crop Sci. 43, 1029–1035. doi: 10.2135/cropsci2003.1029
Hayat, I., Ahmad, A., Masud, T., Ahmed, A., and Bashir, S. (2014). Nutritional and health perspectives of beans (Phaseolus vulgaris L.): an overview. Crit. Rev. Food Sci. Nutr. 54, 580–592. doi: 10.1080/10408398.2011.596639
Herrera, M. D., Reynoso-Camacho, R., Melero-Meraz, V., Guzmán-Maldonado, S. H., and Acosta-Gallegos, J. A. (2021). Impact of soil moisture on common bean (Phaseolus vulgaris L.) phytochemicals. J. Food Compos. Anal. 99, 103883. doi: 10.1016/j.jfca.2021.103883
Horwitz, W. (2005). Official Methods of Analysis, 18th edn, ed. G. W. Latimer: AOAC International, Gaithersburg, USA.
Hummel, M., Talsma, E. F., Taleon, V., Londoño, L., Brychkova, G., Gallego, S., et al. (2020). Iron, zinc and phytic acid retention of biofortified, low phytic acid, and conventional bean varieties when preparing common household recipes. Nutrients 12, 658. doi: 10.3390/nu12030658
Hurrell (2004). Phytic acid degradation as a means of improving iron absorption. Int. J. Vitam. Nutr. Res. 74, 445–452. doi: 10.1024/0300-9831.74.6.445
Jepleting, N., Sila, D. N., and Orina, I. N. (2022). Nutritional composition and antinutrient to mineral molar ratios of selected improved common beans grown in Kenya. Curr. Res. Nutr. Food Sci. 10, 1230–1239. doi: 10.12944/CRNFSJ.10.3.35
Juszczuk, I. M., Wiktorowska, A., Malusá, E., and Rychter, A. M. (2004). Changes in the concentration of phenolic compounds and exudation induced by phosphate deficiency in bean plants (Phaseolus vulgaris L.). Plant Soil 267, 41–49. doi: 10.1007/s11104-005-2569-9
Kahraman, A., Kahraman, A., and Onder, M. (2017). Accumulation of heavy metals in dry beans sown on different dates. J. Elem. 1, 2018. doi: 10.5601/jelem.2017.22.2.1308
Kargiotidou, A., Papathanasiou, F., Baxevanos, D., Vlachostergios, D. N., Stefanou, S., Papadopoulos, I., et al. (2019). Yield and stability for agronomic and seed quality traits of common bean genotypes under mediterranean conditions. Legum. Res. 42, 308–313. doi: 10.18805/LR-437
Kopczyńska, K., Srednicka-Tober, D., Hallmann, E., Wilczak, J., Wasiak-Zys, G., Wyszyński, Z., et al. (2021). Bioactive compounds, sugars, and sensory attributes of organic and conventionally produced courgette (Cucurbita Pepo). Foods 10, 2475. doi: 10.3390/foods10102475
Kotha, R. R., Finley, J. W., and Luthria, D. L. (2020). Determination of soluble mono, di, and oligosaccharide content in 23 dry beans (Phaseolus vulgaris L.). J. Agric. Food Chem. 68, 6412–6419. doi: 10.1021/acs.jafc.0c00713
Leal, F. T., Bettiol, J. V. T., Filla, V. A., Coelho, A. P., Mingotte, F. L. C., Lemos, L. B., et al. (2021). Grain quality of common bean (Phaseolus vulgaris L.) cultivars under low and high nitrogen dose. Rev. Fac. Cienc. Agrar. Univ. Nac. Cuyo. 53, 118–127. doi: 10.48162/rev.39.012
Lo Turco, V., Potortì, A. G., Rando, R., Ravenda, P., Dugo, G., and Di Bella, G. (2016). Functional properties and fatty acids profile of different beans varieties. Nat. Prod. Res. 30, 2243–2248. doi: 10.1080/14786419.2016.1154056
Lynch, J., Cain, M., Frame, D., and Pierrehumbert, R. (2021). Agriculture's contribution to climate change and role in mitigation is distinct from predominantly fossil CO2-emitting sectors. Front. Sustain. Food Syst. 4, 518039. doi: 10.3389/fsufs.2020.518039
Madrera, R. R., and Valles, B. S. (2020). Development and validation of ultrasound assisted extraction (UAE) and HPLC-DAD method for determination of polyphenols in dry beans (Phaseolus vulgaris). J. Food Compos. Anal. 85, 103334. doi: 10.1016/j.jfca.2019.103334
Manoharlal, R., Saiprasad, G. V. S., and Madhavakrishna, K. (2020). Evaluation of mono-, di-, and oligo-saccharydes of staple Indian grain flours. J. Microb. Biotech. Food Sci. 9, 799–804. doi: 10.15414/jmbfs.2020.9.4.799-804
MAPA Ministerio de Agricultura, Pesca y Alimentación. (2023). I.G.P. Faba Asturiana. Available online at: https://www.mapa.gob.es/es/alimentacion/temas/calidad-diferenciada/dop-igp/legumbres/IGP_fabastur.aspx (accessed July 16, 2023).
Mariamenatu, A. H., and Abdu, E. M. (2021). Overconsumption of omega-6 polyunsaturated fatty acids (PUFAs) versus deficiency of omega-3 PUFAs in modern-day diets: the disturbing factor for their “balanced antagonistic metabolic functions” in the human body. J. Lipids 2021, 8848161. doi: 10.1155/2021/8848161
Mastura, H., Hasnah, H., and Dang, T. N. (2017). Total phenolic content and antioxidant capacity of beans: organic vs inorganic. Int. Food Res. J. 24, 510–517.
McPhee, K. E., Zemetra, R. S., Brown, J., and Myers, J. R. (2002). Genetic analysis of the raffinose family oligosaccharides in common bean. J. Am. Soc. Hortic. Sci. 127, 376–382. doi: 10.21273/JASHS.127.3.376
Medina-Velo, I. A., Dominguez, O. E., Ochoa, L., Barrios, A. C., Hernández-Viezcas, J. A., White, J. C., et al. (2017). Nutritional quality of bean seeds harvested from plants grown in different soils amended with coated and uncoated zinc oxide nanomaterials. Environ. Sci. Nano 4, 2336–2347. doi: 10.1039/C7EN00495H
Megazyme (2023). Phytic acid (phytate)/Total Phosphorus. Assay Procedure. Available online at: https://www.megazyme.com/documents/Assay_Protocol/K-PHYT_DATA.pdf (accessed July 16, 2023).
Muller, A., Schader, C., El-Hage Scialabba, N., Brüggemann, J., Isensee, A., Erb, K.-H., et al. (2017). Strategies for feeding the world more sustainably with organic agriculture. Nat. Commun. 8, 1290. doi: 10.1038/s41467-017-01410-w
Nadeem, M. A., Yeken, M. Z., Shahid, M. Q., Habyarimana, E., Yilmaz, H., Alsaleh, A., et al. (2021). Common bean as a potential crop for future food security: an overview of past, current and future contributions in genomics, transcriptomics, transgenics and proteomics. Biotechnol. Biotechnol. Equip. 35, 759–787. doi: 10.1080/13102818.2021.1920462
Naivikul, O., and D'Appolonia, B. L. (1978). Comparion of legume and wheat flour carbohydrates. I. Sugar analysis. Cereal. Chem. 55, 913–918.
Nicoletto, C., Zanin, G., Sambo, P., and Dalla Costa, L. (2019). Quality assessment of typical common bean genotypes cultivated in temperate climate conditions and different growth locations. Sci. Hortic. 256, 108599. doi: 10.1016/j.scienta.2019.108599
Oney-Montalvo, J., Uc-Varguez, A., Ramírez-Rivera, E., Ramírez-Sucre, M., and Rodríguez-Buenfil, I. (2020). Influence of soil composition on the profile and content of polyphenols in habanero peppers (Capsicum chinense Jacq.). Agronomy 10, 1234. doi: 10.3390/agronomy10091234
Palčić, I., KaraŽija, T., Petek, M., Lazarević, B., Herak Custić, M., Gunjača, J., et al. (2018). Relationship between origin and nutrient content of croatian common bean landraces. J. Cent. Eur. Agric. 19, 490–502. doi: 10.5513/JCEA01/19.3.2103
Parodi, A., Leip, A., De Boer, I. J. M., Slegers, P. M., Ziegler, F., Temme, E. H. M., et al. (2018). The potential of future foods for sustainable and healthy diets. Nat. Sustain. 1, 782–789. doi: 10.1038/s41893-018-0189-7
Philipo, M., Ndakidemi, P. A., and Mbega, E. R. (2020). Environmental and genotypes influence on seed iron and zinc levels of landraces and improved varieties of common bean (Phaseolus vulgaris L.) in Tanzania. Ecol. Genet. Genom. 15, 100056. doi: 10.1016/j.egg.2020.100056
Pisoschi, A. M., Pop, A., Cimpeanu, C., and Predoi, G. (2016). Antioxidant capacity determination in plants and plant-derived products: a review. Oxid. Med. Cell. Longev. 2016, 1–36. doi: 10.1155/2016/9130976
Ribeiro, N. D., Maziero, S. M., Prigol, M., Nogueira, C. W., Rosa, D. P., and Possobom, M. T. D. F. (2012). Mineral concentrations in the embryo and seed coat of common bean cultivars. J. Food Compos. Anal. 226, 89–95. doi: 10.1016/j.jfca.2012.03.003
Rodríguez Madrera, R., Campa Negrillo, A., Suárez Valles, B., and Ferreira Fernández, J. J. (2020). Characterization of extractable phenolic profile of common bean seeds (Phaseolus vulgaris L.) in a Spanish diversity panel. Food Res. Int. 138, 109713. doi: 10.1016/j.foodres.2020.109713
Rodríguez Madrera, R., Campa Negrillo, A., Suárez Valles, B., and Ferreira Fernández, J. J. (2021). Phenolic content and antioxidant activity in seeds of common bean (Phaseolus vulgaris L.). Foods 10, 864. doi: 10.3390/foods10040864
Rodríguez-Ruiz, J., Belarbi, E.-H., García Sanchez, J. L., and López Alonso, D. (1998). Rapid simultaneous lipid extraction and transesterification for fatty acid analyses. Biotechnol. Tech. 12, 689–691. doi: 10.1023/A:1008812904017
Shellie, K. C., and Hosfield, G. L. (1991). Genotype × environmental effects on food quality of common bean: resource-efficient testing procedures. J. Am. Soc. Hortic. Sci. 116, 732–736. doi: 10.21273/JASHS.116.4.732
Singleton, V. L., and Rossi, A. (1965). Colorimetry of total phenolics with phosphomolybdicphosphotungstic acid reagents. Am. J. Enol. Vitic. 6, 144–158. doi: 10.5344/ajev.1965.16.3.144
Sinkovič, L., Pipan, B., Šibul, F., Nemeš, I., Tepić Horecki, A., Meglič, V., et al. (2022). Nutrients, phytic acid and bioactive compounds in marketable pulses. Plants 12, 170. doi: 10.3390/plants12010170
Soetan, K. O., Olaiya, C. O., and Oyewole, O. E. (2010). The importance of mineral elements for humans, domestic animals and plants: a review. Afr. J. Food Sci. 4, 200–222.
Umeta, M., West, C. E., and Fufa, H. (2005). Content of zinc, iron, calcium and their absorption inhibitors in foods commonly consumed in Ethiopia. J. Food Compos. Anal. 18, 803–817. doi: 10.1016/j.jfca.2004.09.008
Uyanöz, R., Akbulut, M., Çetin, Ü., and Gültepe, N. (2007). Effects of microbial inoculation, organic and chemical fertilizer on yield and physicochemical and cookability properties of bean (Phaseolus vulgaris L.) seeds. Philipp. Agric. Sci. 90, 168–172.
Velasco-González, O., Martín-Martínez, E. S., Aguilar-Méndez, M., and Pajarito-Ravelero, A. (2013). Propiedades físicas y químicas del grano de diferentes variedades de frijol (Phaseolus vulgaris L.). Bioagro 25, 161–166.
Voysest, O. (2000). Mejoramiento Genético de frijol (Phaseolus vulgaris L.). Legado de Variedades de América Latina 1930–1999. Cali: CIAT.
Wafula, E. N., Onduso, M., Wainaina, I. N., Buvé, C., Kinyanjui, P. K., Githiri, S. M., et al. (2022). Antinutrient to mineral molar ratios of raw common beans and their rapid prediction using near-infrared spectroscopy. Food Chem. 368, 130773. doi: 10.1016/j.foodchem.2021.130773
Wang, N., Hou, A., Santos, J., and Maximiuk, L. (2017). Effects of cultivar, growing location, and year on physicochemical and cooking characteristics of dry beans (Phaseolus vulgaris). Cereal Chem. J. 94, 128–134. doi: 10.1094/CCHEM-04-16-0124-FI
Keywords: anti-nutritional factors, elite cultivar, landrace, mineral content, nutritional value, organic farming, Phaseolus vulgaris, phenolic compounds
Citation: Rodríguez Madrera R, Campa Negrillo A and Ferreira Fernández JJ (2024) Modulation of the nutritional and functional values of common bean by farming system: organic vs. conventional. Front. Sustain. Food Syst. 7:1282427. doi: 10.3389/fsufs.2023.1282427
Received: 24 August 2023; Accepted: 04 December 2023;
Published: 04 January 2024.
Edited by:
Uday Chand Jha, Indian Institute of Pulses Research (ICAR), IndiaReviewed by:
Joana Machado, Universidade Católica Portuguesa, PortugalCopyright © 2024 Rodríguez Madrera, Campa Negrillo and Ferreira Fernández. This is an open-access article distributed under the terms of the Creative Commons Attribution License (CC BY). The use, distribution or reproduction in other forums is permitted, provided the original author(s) and the copyright owner(s) are credited and that the original publication in this journal is cited, in accordance with accepted academic practice. No use, distribution or reproduction is permitted which does not comply with these terms.
*Correspondence: Roberto Rodríguez Madrera, cnJvZHJpZ3VlekBzZXJpZGEub3Jn
Disclaimer: All claims expressed in this article are solely those of the authors and do not necessarily represent those of their affiliated organizations, or those of the publisher, the editors and the reviewers. Any product that may be evaluated in this article or claim that may be made by its manufacturer is not guaranteed or endorsed by the publisher.
Research integrity at Frontiers
Learn more about the work of our research integrity team to safeguard the quality of each article we publish.