- 1School of Chinese Ethnic Medicine, Guizhou Minzu University, Guiyang, Guizhou, China
- 2Key Laboratory of Guizhou Ethnic Medicine Resource Development and Utilization in Guizhou Minzu University, State Ethnic Affairs Commission, Guiyang, Guizhou, China
- 3Engineering Research Center for Fruit Crops of Guizhou Province, Key Laboratory of Plant Resource Conservation and Germplasm Innovation in Mountainous Region (Ministry of Education), College of Agriculture, Guizhou University, Guiyang, China
The application of hydrogen-rich water (HRW) can extend the shelf life of fruits and vegetables. This study aims to investigate the effects of HRW on the quality of fresh-cut Gastrodia elata during low-temperature (4°C) storage. The results indicate that HRW treatment inhibited the decrease in weight loss and the increase in respiration rate, and also slowed down the decrease in total acidity and total soluble solids. HRW treatment could reduce the generation of superoxide anions and hydrogen peroxide, enhance the activity and expression of antioxidant-related enzymes such as superoxide dismutase, catalase, ascorbate peroxidase, glutathione reductase, monodehydroascorbate reductase, and dehydroascorbate reductase, and increase the content of non-enzymatic antioxidant substances, ascorbic acid and glutathione, while decreasing the activity and expression of peroxidase and polyphenol oxidase. Furthermore, HRW treatment not only inhibited the reduction in ADP and ATP content and energy charge but also attenuated the increase in AMP content. It also slowed down the decrease in the activities of H+-ATPase, succinate dehydrogenase, Ca2+-ATPase, and cytochrome C oxidase. In conclusion, these results demonstrate that HRW treatment enhances the energy levels and effectively mitigates oxidative damage, thereby preserving postharvest quality of fresh-cut G. elata.
Introduction
Gastrodia elata (G. elata), is rich in functional components such as gastrodin, amino acids, and polysaccharides. Its rhizome is a medicinal and edible material (Fan et al., 2022). Fresh G. elata tubers can be stored at room temperature for a maximum of 5–7 days before it begins to deteriorate (Cheng et al., 2020). To preserve the quality of G. elata, it is commonly dried and subjected to sulfur fumigation after harvesting. However, this method has drawbacks such as a decrease in active ingredient content, poor sensory quality, and undesirable taste (Guan et al., 2023).
Reactive oxygen species (ROS) play a crucial role as signaling molecules in plants, participating in the regulation of diverse physiological processes. However, when exposed to environmental stress or during the aging process, excessive accumulation of ROS in plant cells can lead to oxidative damage (Oliveira et al., 2016). It has been demonstrated that the accumulation of ROS is a prominent feature in plant aging (Apel and Hirt, 2004). Therefore, maintaining a redox balance is crucial for delaying plants senescence. The balance of ROS in plant is primarily regulated by antioxidant systems (Ge et al., 2018).
The cellular energy supply is a crucial factor in maintaining the quality of plants after harvest. The postharvest aging and decline in the quality of plants are closely associated with cellular energy metabolism (Li et al., 2021). Previous study has shown that various postharvest treatments could maintain plant quality by enhancing the energy levels (Li et al., 2020b; He et al., 2021; Wang et al., 2021; Yi et al., 2021).
Compared to traditional preservatives and biocides, H2 and hydrogen-rich water (HRW), do not necessitate the addition of harmful chemicals. This reduction in the use of harmful chemicals diminishes potential risks to both the environment and consumers. Furthermore, H2 and HRW leave minimal to no residue on produce, which is advantageous for ensuring both food safety and product quality. Jiang et al. (2021) observed that the application of H2 to Chinese chive significantly improves its shelf life and reduces decay, while preserving its antioxidant capacity and mitigating oxidative damage during storage. In recent studies, in the food field, HRW has found the opportunity to be used in limiting biogenic amine formation in red beet pickles (Alwazeer et al., 2022) and butter (Bulut et al., 2022). Moreover, HRW has been shown to improve postharvest quality to varying degrees in kiwifruit (Hu et al., 2014), fresh-cut Chinese water chestnut (Li et al., 2022a), and Hypsizygus marmoreus (Chen et al., 2017). Although the studies mentioned above have suggested that HRW has the potential to maintain plant quality, delay aging, and improve antioxidant levels by regulating ROS accumulation, nevertheless, the impact of postharvest treatment with HRW on the quality, antioxidant capacity, and energy metabolism of fresh-cut G. elata has yet to be investigated. This study aims to examine the influence of HRW treatment during low-temperature (4°C) storage on the quality, antioxidant capacity, and energy metabolism of fresh-cut G. elata, and to contribute to the understanding of the mechanism by which HRW treatment maintains the quality of fresh-cut G. elata, facilitating the development of safe and convenient postharvest preservation techniques for fresh-cut G. elata.
Materials and methods
Fruit and treatment
Gastrodia elata tubers harvested in 2022 from Zhaotong, Yunnan Province, China (103° 79′ E, 27° 38′ N), were rapidly transported to the laboratory within 3 h. HRW was produced by blowing H2 (99.99%, v/v) into 3 L of purified water with a H2 generator (AYH-300, Ansha Shancheng Science and Technology Co., Ltd., Beijing, China) for 4 h at a rate of 300 mL min−1. The concentration of HRW used was determined using a portable H2 concentration measuring instrument (Trustlex Co, Led, ENH-2000, Japan).
Gastrodia elata that were free from diseases, pests and mechanical damage and of uniform size were selected, washed with deionized water, then soaked in 0, 0.2 mM, 0.4 mM, and 0.8 mM HRW for 10 min, air-dried, and sliced into 1 cm-thick pieces in a sterilized environment. Based on the preliminary results of the pre-experiment, which indicated that the 0.4 mM HRW treatment distinctly suppressed browning compared to the other treatments (data not shown), the subsequent analysis was carried out using the 0.4 mM HRW treatment. Phenotypic observations, physiological and biochemical indexes were performed at 0, 2, 4, 6, 8 and 10 days of 4°C storage, and fresh-cut G. elata was snap-frozen with liquefied nitrogen and stored in a Thermo Scientific™ TSX ultra-low-temperature refrigerator (USA) at −80°C for subsequent experiments. Three biological replicates, each containing four sections, were used for all index determinations.
Determination of weight loss, respiratory rate, total soluble solid (TSS), titratable acid (TA) and malondialdehyde (MDA)
The weight loss rate (%) is calculated as: [(initial fresh-cut G. elata weight- present fresh-cut G. elata weight)] / initial fresh-cut G. elata weight × 100. Respiration rate was determined using a dual-wavelength infrared carbon dioxide analyzer (HM-GX20, Shandong Hengmei Electronic Technology Co., Ltd., China). The changes in CO2 concentration were monitored by sealing four slices of fresh-cut G. elata in a 100 mL sealed canister connected to the analyzer to form a circulating gas system. Measurements were taken at 1-min intervals for 5 consecutive times, and respiration rates were expressed as mg CO2 kg−1 h−1 fresh weight. TSS was measured using an ATAGO-PAL-1 digital refractometer and TA was titrated using NaOH (pH 13.0, 0.1 mol L−1) and expressed as a percentage of TSS and TA. MDA content was determined to the method of Gao et al. (2018), where absorbance was measured at 450, 532 and 600 nm and expressed as mmol kg−1 FW.
Assays for ROS production and the activity of antioxidant scavenging enzymes
Superoxide anion (O2•-) production rate and hydrogen peroxide (H2O2) were measured on a UV spectrophotometer using O2•- and H2O2 Quantification Kits (Beyotime Biotechnology Co. Ltd., Beijing, China), respectively, in accordance with the specific procedures offered by the producer. The rate of O2•- generation and H2O2 content were indicated in mol kg−1 min−1 and mmol kg−1 FW, respectively.
For assessment the activity of antioxidant scavenging enzyme, superoxide dismutase (SOD), ascorbate peroxidase (APX), catalase (CAT), and glutathione reductase (GR) extraction procedures are detailed in Ding et al. (2021). Dehydroascorbate reductase (DHAR) and monodehydroascorbate reductase (MDHAR) were extracted according to the instructions of the kit (Solarbio Life Sciences. Co. Ltd., Beijing, China). SOD activity was established by assessing the capacity to suppress the reductive effect of nitro blue tetrazolium (NBT) in the presence of light Ding et al. (2021). 1 U represents the amount of enzyme required to depress 50% of the photo-reduction reaction of NBT. CAT activity was defined by examining the change in absorbance caused by H2O2 content at 240 nm (Ge et al., 2018). 1 U of CAT activity was defined as the amount of enzyme per minute that caused the decomposition of 1 μmol of H2O2. APX activity was evaluated by the changed absorbance (290 nm) caused by the oxygenation of ascorbic acid (AsA) after the addition of H2O2 (Ge et al., 2018). 1 U of APX activity was the amount of enzyme per minute that caused the oxidation of 1 μmol of AsA. GR activity was measured change in absorbance due to NADPH oxidation in the absence of GSSH (Ge et al., 2018). GR activity of 1 U was calculated as the enzyme amount per minute leading to 1 nmol of NADPH oxidation. The activities of DHAR and MDHAR were identified according to the kit instructions. 1 U of the activity of DHAR was defined as the enzyme amount catalyzing the production of 1 μmol of AsA per second and MDHAR was determined as the enzyme amount per second catalyzing 1 μmol NADPH oxidation. All the above antioxidant scavenging enzyme activities were shown in U kg−1 FW.
Activities of peroxidase (POD) and polyphenol oxidase (PPO)
In the present investigation, frozen tissues weighing 2.0 g were subjected to extraction using a 50 mM pre-chilled phosphate buffer solution (PBS) with a pH of 7.0, supplemented with 1% polyvinylpyrrolidone (PVP). The supernatant obtained after centrifugation at 4°C, 1000 g for 10 min (Thermo Fisher Scientific Inc., USA) was used as the crude enzyme solution for POD (EC 1.11.1.7) and PPO (EC 1.10.3.1) activity assays. POD activity was determined following the protocol outlined by Li et al. (2022b). Specifically, a reaction mixture of 1 mL was prepared, consisting of 50 mM PBS (pH 7.0), 20 mM hydrogen peroxide (H2O2), 1% guaiacol, and 50 μL of the extracted supernatant. The spectrophotometric monitoring of absorbance changes at 560 nm enabled the quantitative estimation of POD activity, with the definition of one unit of POD being the catalysis of 1 μmol of guaiacol oxidation per minute. Concurrently, PPO activity was assayed using a reaction mixture of 1 mL comprising 50 mM catechol, 50 μL of the extracted supernatant, and 0.1 M PBS at pH 6.8. The assessment of PPO activity was facilitated by monitoring the changes in absorbance at 420 nm, with one unit of PPO defined as the oxidation of 1 nmol of catechol per minute. To standardize the enzymatic activity measurements, all results were expressed as 106 units per kilogram of protein (U kg−1 protein), thereby allowing for meaningful comparison and interpretation of the obtained data. All the above enzyme activities were shown in 106 U kg−1 protein.
Determination of AsA and glutathione (GSH) content
The contents of AsA and GSH content were determined by reference to Dong et al. (2022) and expressed as mg 100 g−1 FW, and mmol 100 g−1 FW, respectively.
Determination of the content of ATP, ADP, AMP, and energy charge
ATP, ADP, and AMP were determined according to the Dong et al. (2022). Initially, a tissue homogenate was prepared by grinding every 3 g of fresh-cut G. elata samples into powder with a freezer mill and adding with 5 mL of perchloric acid (0.6 M) and mixing well. It was then centrifuged at 11,000 × g and 4°C for 30 min. The resulting supernatant was neutralized with 1 M KOH to a pH of approximately 6.8 and then filtered through a 0.45 μm syringe filter (Futecs Co., Ltd., Daejeon, Korea). High performance liquid chromatography (HPLC, Aglient Technology CO. Ltd., USA) was used to determine the content of ATP, ADP and AMP, expressing in g kg−1. The energy charge (EC) was obtained as (ATP +1/2 ADP)/ (ATP + ADP + AMP).
Determination of energy metabolism-related enzymes activity
To assay the activities of cytochrome C oxidase (CCO), succinate dehydrogenase (SDH), H+-ATPase, and Ca2+-ATPase, we used commercial assay kits produced by Nanjing Jiancheng Bioengineering and followed the manufacturer’s protocols. The results are presented in 103 U·kg−1 FW.
Determination of gene expression
Gene expression was determined by quantitative Real-Time PCR (qRT-PCR) analysis. An RNeasy Plant Mini Kit (Takara, Japan) was used to extract RNA from tissue (1.0 g) in strict adherence to the exact procedure. RNA was purified using the manufacturer’s instructions of TRIzol™ Plus RNA Purification Kit (Invitrogen™, Carlsbad, USA) to remove DNA contamination. Synthesis of first-strand cDNA was performed using the Prime-Script™ RT-PCR kit (TaKaRa, Dalian, China). qRT-PCR was performed using the KAPA 1-step qRT-PCR Kit (Bio-rad, California, USA) by CFX384 Touch Real-Time PCR Detection System. The ACTIN gene was used for quantitative normalization. Primers are shown in Supplementary Table S1.
Data analysis
All physiological data were examined in triplicate, and gene expression data were replicated in quadruplicate. The results are presented as the mean ± standard error (SE). To assess significant variations in mean values between the control and HRW treatment groups, we conducted a one-way analysis of variance (ANOVA) using SPSS 19.0 software (SPSS, Inc., Chicago, United States). Subsequently, we analyzed significant differences using Student’s t-test (p < 0.05). For correlation analysis, Spearman’s correlation coefficients were calculated and visualized using the ‘corrplot’ package in R software, with significance levels set at p < 0.05 and p < 0.01.
Results
Effect of HRW on phenotypic observation, weight loss, respiratory rate, TSS, TA, and MDA in fresh-cut Gastrodia elata
As shown in Figure 1A, the control group of fresh-cut G. elata exhibited significant browning on the surface after 6 days of storage at 4°C. However, the HRW treatment effectively inhibited the browning of fresh-cut G. elata (Figure 1A). The weight loss of fresh-cut G. elata increased in both groups throughout the storage period. The HRW-treated group displayed a substantially lower weight loss compared to the control at 8 (p = 0.003)-10 (p = 0.001) days (Figure 1B). After HRW treatment, the respiratory rate of fresh-cut G. elata increased from 0–6 days and then declined. Apart from day 4 (p > 0.05), it showed a notably elevated level compared with the control (Figure 1C). Both the control and the HRW-treated group showed a decreasing trend in TSS and TA throughout the experimental period. However, compared to the control, the HRW effectively delayed the decrease in TSS and TA of fresh-cut G. elata (Figures 1D,E). TA content was notably higher in the HRW-treated group compared to the control group on days 4 (p = 0.002) and 10 (p = 0.004) of storage, respectively, whereas TSS exhibited a significant increase over the control on days 6 (p = 0.004), 8 (p = 0.003), and 10 (p = 0.003). Throughout the storage stage, the content of MDA showed an increasing trend in both the control and treatment group. However, HRW treatment markedly reduced the MDA content at 2 (p = 0.003) and 6 (p = 0.001), 8 (p = 0.001), 10 (p = 0.004) days (Figure 1F).
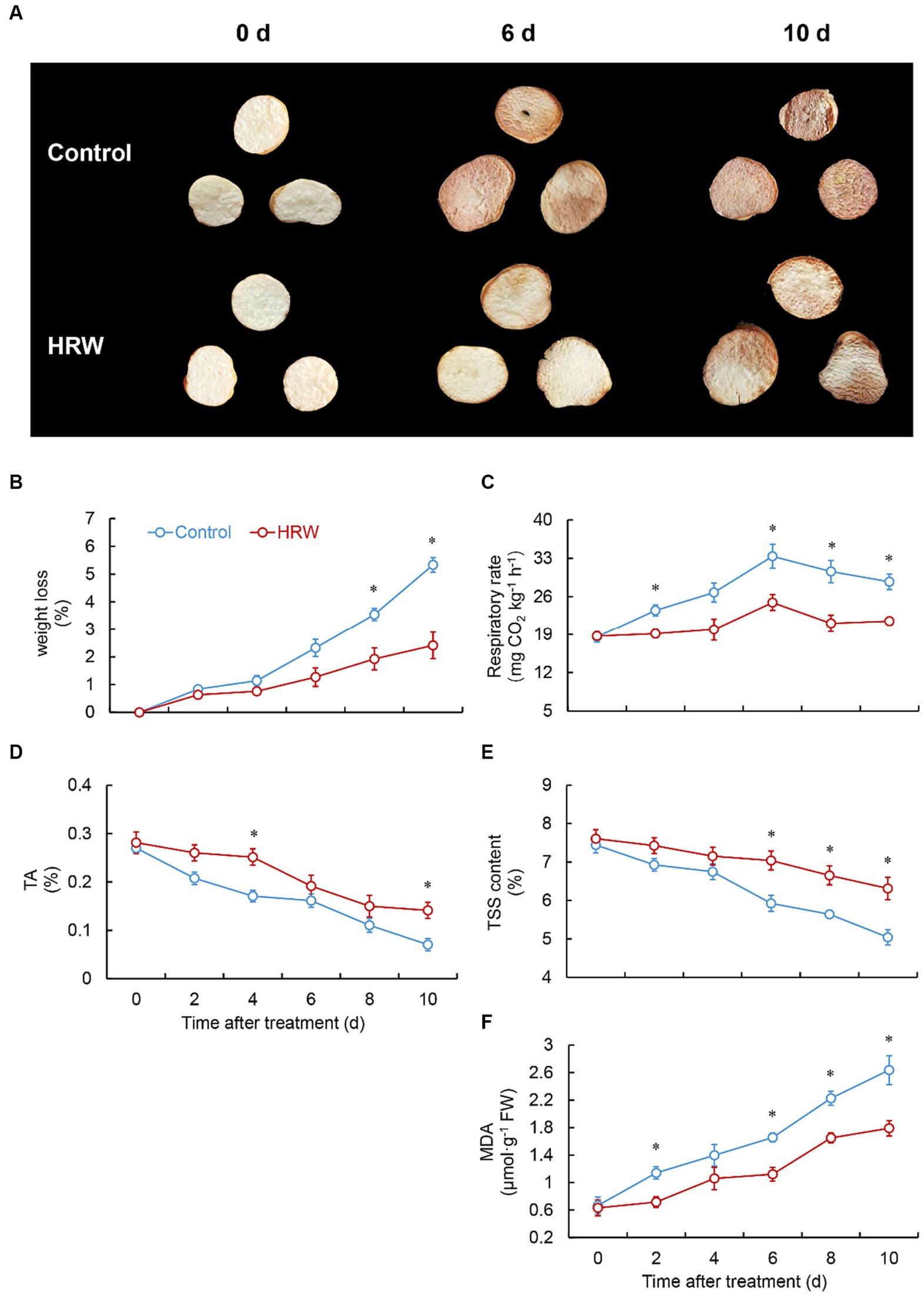
Figure 1. Visual appearance of fresh-cut Gastrodia elata after HRW treatment during storage at 4°C (A), and effect of HRW treatment on the weight loss (B), respiratory rate (C), TSS (D), TA (E), and MDA (F). *Denotes significant difference at the level of p < 0.05. Vertical bars represent the standard errors of the means (±SE).
Effect of HRW on ROS metabolism and POD, PPO activities
As shown in Figures 2A,B, both the control and HRW-treated group exhibited a gradual increase in the O2•- production rate and the content of H2O2. However, in the HRW-treated group, the O2•- production rate was markedly lower than the control at 2 (p = 0.001) and 6 (p = 0.002), 8 (p = 0.002), 10 (p = 0.003) days (Figure 2A). Additionally, the HRW treatment exhibited notably lower levels of H2O2 throughout the entire storage period (Figure 2B). The group treated with HRW displayed a swift rise in SOD activity within 2 day, reaching its maximum by day 4. Subsequently, the activity fluctuated but consistently remained significantly higher than the control for the entire duration of the experiment (Figure 2C). Both HRW treatment and control showed a continuous increase in CAT activity from 0 to 8 days, reaching their maximum levels. However, the HRW-treated group exhibited substantially greater CAT activity than the control at 4 (p = 0.003), 6 (p = 0.002), 8 (p = 0.008) days (Figure 2D). After HRW treatment, fresh-cut G. elata POD and PPO activities remained considerably lower than the control throughout the entire experimental period (Figures 2E,F). The HRW-treated group exhibited a rapid increase in APX activity from 0 to 2 days, followed by fluctuations from 2 to 8 days, and subsequently a decline. Moreover, the HRW-treated group demonstrated considerably higher APX activity than the control at 2 (p = 0.002), 8 (p = 0.001) and 10 (p = 0.001) days. In contrast, the control group showed an increase in APX activity from 0 to 4 days, followed by a decline (Figure 3A). The HRW-treated group exhibited an increase in GR activity throughout the entire stage, except for a slight decrease at 6–8 days, and at 4 (p = 0.001), 6 (p = 0.001), 8 (p = 0.002), 10 (p = 0.0001) days, it was markedly higher than the control (Figure 3B). The HRW-treated group demonstrated a rapid surge in MDHAR activity, peaking between 0 and 4 days, and subsequently experiencing fluctuations. Consistently, throughout the entire storage period, the HRW-treated group exhibited significantly higher MDHAR activity compared to the control, particularly between 2 and 8 days (Figure 3C). The HRW-treated group DHAR activity increased during 0–4 days, followed by fluctuations. In contrast, the control exhibited an increase during 0–2 days, followed by fluctuations and subsequent decline during 2–8 days. Compared to the control, the HRW-treated group showed significant elevation at 4 (p = 0.001) 8 (p = 0.003) and 10 (p = 0.0001) days (Figure 3D).
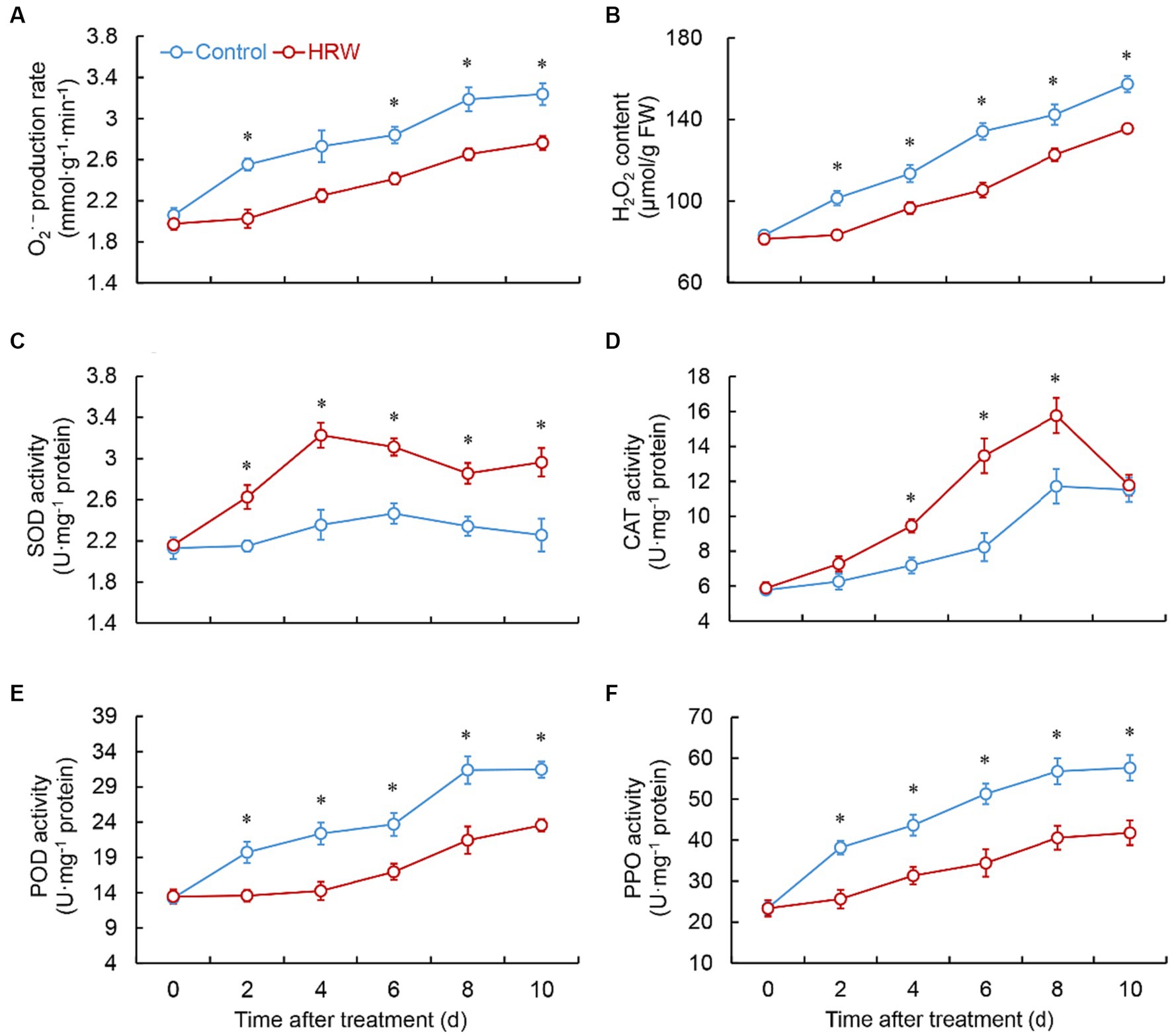
Figure 2. Changes in O2•- production rate (A), H2O2 content (B), and the activity of SOD (C), CAT (D), POD (E) and PPO (F) in fresh-cut Gastrodia elata after HRW treatment during storage at 4°C. *Denotes significant difference at the level of p < 0.05. Vertical bars represent the standard errors of the means (±SE).
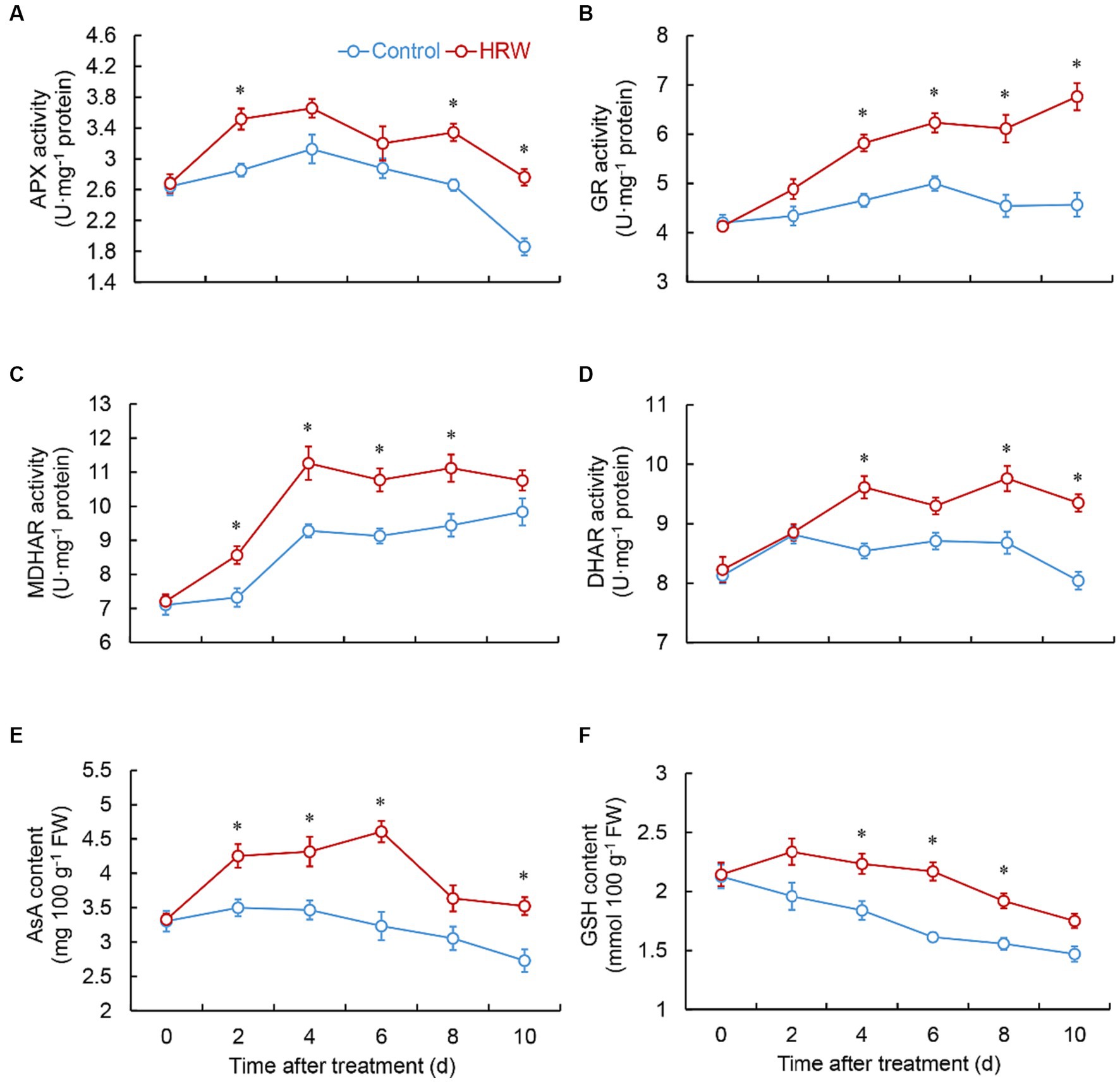
Figure 3. Changes in the activity of APX (A), GR (B), MDHAR (C), DHAR (D), and the content of AsA (E), and GSH (F) in fresh-cut Gastrodia elata after HRW treatment during storage at 4°C. *Denotes significant difference at the level of p < 0.05. Vertical bars represent the standard errors of the means (±SE).
Determination of AsA and GSH content
From day 0 to 2, the control experienced a rise in AsA content, followed by a continuous decrease. Conversely, the HRW-treated group showed an increase in AsA content from 0 to 6 days, followed by a decline. Additionally, the AsA content of the HRW-treated group was significantly higher than that of the control group at days 2 (p = 0.003), 4 (p = 0.005), 6 (p = 0.001) and 10 (p = 0.003) (Figure 3E). The control showed a continuous decrease in GSH content throughout the entire experimental stage. In contrast, the HRW-treated group exhibited a continuous increase from 0 to 2 day, followed by a decline. Moreover, the HRW-treated group demonstrated considerably higher GSH content than the control at 4 (p = 0.004),6 (p = 0.00001) and 8 (p = 0.001) days (Figure 3F).
Determine the content of ATP, ADP, AMP and energy charge
The control exhibited a similar decreasing trend in ATP and ADP levels throughout the entire storage stage. Throughout the entire experimental phase, the HRW treatment consistently exhibited significantly elevated (p < 0.01) levels of ATP compared to the control (Figure 4A). Additionally, the HRW treatment exhibited markedly higher (p < 0.01) ADP levels compared to the control specifically during the 4–10 days (Figure 4B). Both the control and the HRW treatment group displayed a gradual and progressive elevation in AMP levels. In the HRW treatment, the AMP levels remained substantially lower (p < 0.01) than the control (Figure 4C). The HRW treatment group showed an increase in energy charge during the first 2 days, followed by a decrease, and significantly higher (p < 0.001) than the control throughout the experimental period (Figure 4D).
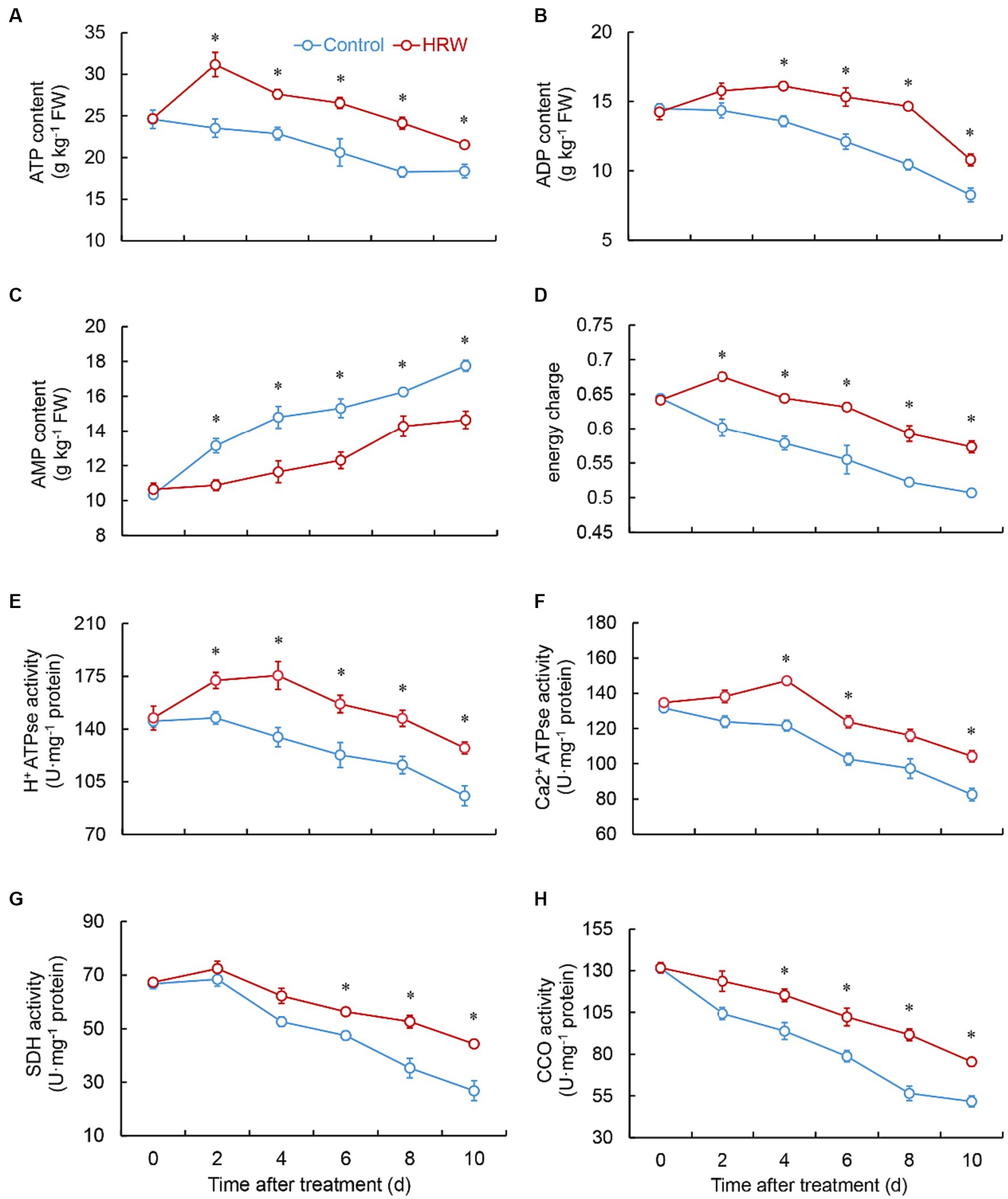
Figure 4. Changes in the content of ATP (A), ADP (B), AMP (C), and energy charge (D), and the activity of H+-ATPase (E), Ca2+-ATPse (F), SDH (G) and CCO (H) in fresh-cut Gastrodia elata after HRW treatment during storage at 4°C. *Denotes significant difference at the level of p < 0.05. Vertical bars represent the standard errors of the means (±SE).
Energy metabolism-related enzymes activity determination
The HRW-treated group exhibited similar trends in H+-ATP and Ga2+-ATP activities. Both activities increased from 0 to 4 days, followed by a decline, and remained greater than the control throughout the experimental stage. In the HRW treatment, the H+-ATP activity was markedly higher (p < 0.01) than the control during the 2–10 days (Figure 4E), while the Ga2+-ATP activity was considerably higher during the 4 (p = 0.001), 6 (p = 0.002) and 10 (p = 0.001) days (Figure 4F). Both the control and the HRW-treated group exhibited a slight rise in SDH activity from 0 to 2 days, followed by a decline. However, the HRW-treated group showed significantly higher SDH activity than the control at 6 (p = 0.002), 8 (p = 0.002) and 10 (p = 0.002) days (Figure 4G). Both the control and the HRW-treated group demonstrated a consistent decline in CCO activity over the entire duration of the experimental stage. However, the HRW-treated group showed markedly higher CCO activity than the control at 4–10 days (p < 0.01) (Figure 4H).
Gene expressions of ROS metabolism of fresh-cut Gastrodia elata
The GeSOD expression in the HRW treatment rapidly increased at 2 days, reached its maximum at 4 days, and remained considerably higher (p < 0.001) than the control from 2 to 8 days (Figure 5A). After HRW treatment, the GeCAT expression remained substantially higher (p < 0.001) than the control (Figure 5B). As shown in Figures 5C,D, HRW treatment substantially reduced the GePOD (p < 0.01) and GePPO (p < 0.001) expressions throughout the entire experimental stage (Figures 5C,D). Based on Figure 5, it is evident that HRW treatment considerably increased the expression of essential enzymes involved in ascorbate-glutathione (AsA-GSH) metabolism (APX, GR, MDHAR and DHAR) to varying extents (Figures 5E–H).
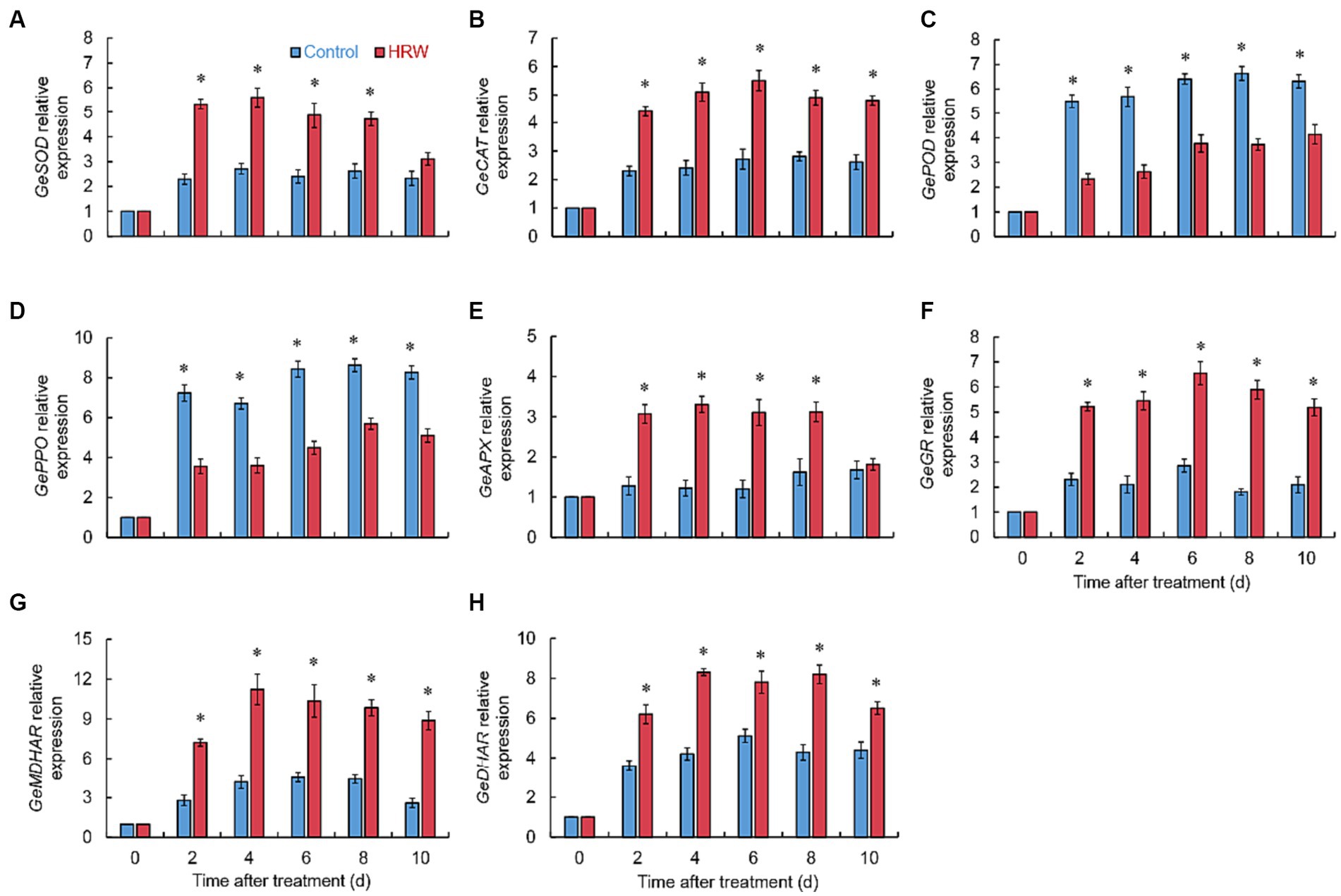
Figure 5. Expression of GeSOD (A), GeCAT (B), GePOD (C), GePPO (D), GeAPX (E), GeGR (F), GeMDHAR (G) and GeDHAR (H) in fresh-cut Gastrodia elata after HRW treatment during storage at 4°C. *Denotes significant difference at the level of p < 0.05. Vertical bars represent the standard errors of the means (±SE).
Discussion
H2 has emerged as a promising agent with profound effects on plants. Its applications span from bolstering plant tissue resilience to various abiotic and biotic stresses, regulating growth, enhancing antioxidant properties, extending shelf life, and mitigating nitrite accumulation during the storage of agricultural products (Wang et al., 2023). As H2 garners attention as the potential “fuel of the 21st century,” it is poised for wider adoption in production and transportation. Such a trend is expected to lead to cost reductions, making H2-based solutions more accessible for agricultural and industrial purposes. Yet, the direct application of gaseous H2 in agriculture presents practical, safety, and cost challenges. In contrast, HRW offers a simple and secure treatment method with promising applications. This approach is not only more environmentally friendly than other growth regulators and preservatives but also more feasible for practical implementation (Hancock et al., 2022).
After harvesting, G. elata undergoes a series of physiological and biochemical reactions, including respiration and transpiration. Excessive postharvest respiration and transpiration rates are important factors leading to plant senescence and quality deterioration (Ge et al., 2019a). Reducing respiratory rate and weight loss are effective measures to maintain postharvest plant quality. Previous studies have confirmed that HRW could maintain the firmness of kiwifruit postharvest and effectively reduce the respiratory rate and weight loss, thereby ensuring the quality of kiwifruit (Hu et al., 2014). Similarly, in the present study, respiration rate and weight loss were significantly reduced in the HRW-treated group, resulting in the maintenance of G. elata quality. In addition, in studies conducted by Chen et al. (2017) on H. marmoreus and An et al. (2021) on pakchoi, MDA serves as a key indicator of membrane lipid oxidation and peroxidation, and its content can reflect the degree of senescence in fresh-cut fruit and vegetables (Jędrzejuk et al., 2018). The findings of this study indicate that HRW treatment effectively decreased the respiratory rate of fresh-cut G. elata, delayed quality deterioration, and significantly decreased the MDA content during storage, and maintains cell membrane integrity. it was found that postharvest treatment with HRW significantly reduced the MDA content and mitigated cell membrane damage. TA and TSS can serve as substrates consumed during plant respiration and are crucial indicators for assessing plant postharvest quality. During the storage period, an excessively rapid respiration rate can quickly deplete TSS and TA (Chen et al., 2019). The results of this study indicate that HRW treatment offers effective protection against the loss of TSS and TA in fresh-cut G. elata. These findings align with the observed reduction in the respiratory rate of fresh-cut G. elata upon HRW treatment. Furthermore, comparable findings were obtained in related studies investigating the postharvest treatment of lychee fruit (Yun et al., 2021) and kiwifruit (Hu et al., 2014) with HRW.
When plant organisms are subjected to biological or non-biological stimuli, they produce a highly reactive class of molecules known as ROS, which include O2•-, H2O2, and hydroxyl radicals. These ROS, at appropriate concentrations, can act as signaling molecules, activating defense genes within the plant to counter external threats (Li et al., 2020a). However, excessive ROS can cause damage to the plant organism, and thus, the plant needs to timely eliminate excess ROS to maintain redox balance. Antioxidant enzymes and antioxidants within the plant can clear excess ROS, thereby protecting the plant organism from oxidative stress damage (Ge et al., 2018). Through this mechanism, the plant organism can adapt to complex and changing environments, maintaining normal physiological function and survival. The findings of this study demonstrate that HRW treatment significantly reduces the generation rate of O2•- and the content of H2O2 during storage period in fresh-cut G. elata. After being treated with HRW, lychee showed a significant decrease in O2•- production rate, accompanied by a notable reduction in H2O2 content compared to the control, thereby maintaining the postharvest quality of lychee (Yun et al., 2021). In studies conducted on kiwifruit (Hu et al., 2014) and pakchoi (An et al., 2021), it was also revealed that HRW has the capability to preserve the quality of fruits and vegetables by expediting the removal of O2•-.
SOD is an important antioxidant enzyme present in plant cells. It has the ability to dismutate O2•- into O2 and H2O2. However, excessive levels of H2O2 can also cause damage to cells. Therefore, CAT present in plants can degrade H2O2 into harmless H2O and O2 (Ge et al., 2019b). The results of this study demonstrate that HRW treatment effectively boosts both the activities and expressions of SOD and CAT in fresh-cut G. elata. An et al. (2021) demonstrated that HRW combined with vacuum pre-cooling treatment could enhance activities of SOD and CAT in pakchoi, thereby maintaining its antioxidant capacity and delaying the aging process (An et al., 2021). According to the findings of Li et al. (2022a), HRW treatment was found to enhance the antioxidant capacity of fresh-cut Chinese water chestnut, thereby preserving its postharvest quality. HRW was found to significantly increase the SOD activity throughout the entire storage period (Li et al., 2022a). In addition, similar results were observed in the study of HRW treatment on Brassica campestris spp. chinensis L. seedlings, as reported by Wei et al. (2021). Therefore, we hypothesize that HRW stabilizes the levels of H2O2 and O2•- by stimulating the activities of SOD and CAT, thereby preventing excessive ROS from causing cellular damage. Phenolic compounds, under the oxidation action of PPO and POD, form quinone polymerization products and brown pigments, leading to rapid browning of fruits and vegetables after being freshly cut (Richard-Forget and Gauillard, 1997). The findings of this study demonstrate that HRW treatment effectively mitigates the activities and expressions of POD and PPO during the storage duration of fresh-cut G. elata, effectively alleviating browning. Similarly, Zhang et al. (2023) found that H2 fumigation inhibited POD and PPO activities, thereby suppressing postharvest browning of Lanzhou Lily. Furthermore, the PPD activity of HRW-treated buds was significantly reduced, contributing to the mitigation of bud browning (Hu et al., 2021).
In plants, the ascorbate-glutathione (AsA-GSH) cycle plays a crucial role in the ROS clearance system. APX serves as a pivotal enzyme within the AsA-GSH cycle, which efficiently converts H2O2 to H2O and O2 in the presence of AsA (Wei et al., 2019). Through this mechanism, plants can effectively eliminate excessive ROS, protect cells from oxidative stress damage, and maintain normal cellular physiological functions. Furthermore, AsA and GSH are effective antioxidants that require the involvement of reductases such as DHAR and GR to maintain their stability and continuity. Additionally, monodehydroascorbate can be converted to AsA under the action of MDHAR, while GR facilitates the transformation of oxidized glutathione into GSH, thereby amplifying the antioxidant potential of the AsA-GSH cycle (Ramos-Martinez, 2017). The study revealed that HRW treatment effectively increased the activities and expressions of AsA-GSH-associated enzymes, such as APX, GR, MDHAR, and DHAR, to different extents. This effectively increases the levels of AsA and GSH during storage, providing fresh-cut G. elata with antioxidant capacity and maintaining storage quality. Yun et al. (2021) conducted a study and found that HRW treatment significantly enhances the activity of AsA-GSH cycle-related enzymes, including APX, GR, and MDHAR, as well as the levels of AsA and GSH during lychee fruit storage. This improvement in antioxidant capacity through HRW treatment helps maintain fruit quality (Yun et al., 2021). Moreover, consistent findings in pakchoi corroborated that HRW treatment augmented antioxidant capacity and effectively postponed postharvest aging. These effects are associated with the GR activity and the AsA content (An et al., 2021). Chen et al. (2017) conducted a study and found that HRW treatment in H. marmoreus led to higher activities of APX and GR, ensuring the maintenance of a high level of antioxidant capacity (Chen et al., 2017). The findings above indicate that HRW treatment has the potential to stimulate the activities and expressions of enzymes involved in the AsA-GSH cycle, such as APX, GR, MDHAR, and DHAR, leading to elevated levels of AsA and GSH. This helps in the clearance of excessive ROS generated during the storage of fresh-cut G. elata, thus maintaining ROS balance.
ATP serves as the principal energy currency necessary to support vital life processes. Multiple studies have shown that the energy generated through energy metabolism has a significant impact on quality, aging process, and resistance to abiotic stress in plant (Shu et al., 2020). The integrity of cell membranes is a crucial factor in maintaining normal cellular function. However, when plants lack ATP, they are more prone to membrane damage in the face of stress, which further leads to increased injury by ROS to the membrane. Conversely, high levels of energy metabolism can maintain postharvest membrane integrity and improve fruit quality (Ge et al., 2019a). Therefore, maintaining an appropriate level of energy is vital for preserving cell membrane integrity and plant quality. This study found that HRW treatment effectively enhances the energy status of fresh-cut G. elata, thereby improving the efficiency of the antioxidant system. Previous research has documented that elevated energy levels can improve plant quality and effectively retard the process of aging. According to the study conducted by Li et al. (2020b), the application of benzothiadiazole was found to elevate ATP levels through mitochondrial energy metabolism. This enhancement in ATP levels is suggested to play a significant role in enhancing the resistance of apple fruit during storage. Research has indicated that pre-treatment with α-lipoic acid maintains elevated intracellular ATP levels, effectively enhancing the antioxidant capacity of lychee fruit and delaying peel browning (He et al., 2021). Furthermore, postharvest treatment with methyl jasmonate can enhance the cold resistance of peach fruit by activating energy metabolism to maintain high levels of ATP (Jin et al., 2013).
Sustaining elevated levels of energy metabolism necessitates the concerted activity of various enzymes within the mitochondria. Key enzymes such as H+-ATPase, Ca2+-ATPase, SDH, and CCO play indispensable roles in the oxidative phosphorylation and ATP synthesis processes in plants (Jin et al., 2014). These enzymes, through their synergistic action, promote ATP synthesis within the mitochondria and maintain the high energy levels required by plant cells. The present study observed a notable augmentation in the activities of H+-ATPase, Ca2+-ATPase, SDH, and CCO in fresh-cut G. elata following HRW treatment. These findings provide further evidence for the effective improvement of energy status by HRW. In our previous study on Rosa sterilis, it was found that post-harvest treatment with HRW significantly enhances the activities of H+-ATPase, Ca2+-ATPase, SDH, and CCO. This indirectly improved the antioxidant capacity of R. sterilis (Dong et al., 2022). NO can induce the activity of energy metabolism-related enzymes (H+-ATPase, Ca2+-ATPase, SDH, and CCO) during cold storage, thereby maintaining a high-energy state and enhancing the cold resistance of banana fruits (Wang et al., 2015). Ge et al. (2019a) have demonstrated that postharvest treatment with trisodium phosphate is closely associated with the maintenance of apple fruit quality and the activity of H+-ATPase, Ca2+-ATPase, SDH, and CCO. Furthermore, we conducted correlation analyses of all measured parameters in HRW-treated fresh-cut G. elata to examine the relationship among phenotypic indexes, ROS and energy metabolism (Supplementary Figure S1). The results revealed significant positive correlations between the weight loss rate, respiration rate, MAD, O2•- production rate, H2O2 content, and the activity and gene expression of PPO and POD. This suggests that HRW treatment effectively inhibits browning and preserves quality in correlation with the inhibition of these indices. Conversely, the weight loss rate, respiration rate, O2•- production rate, and H2O2 content displayed significant negative correlations with the activity and gene expression related to both AsA-GSH and energy metabolism. This result implies that HRW may mitigate oxidative damage resulting from ROS by enhancing energy metabolism and bolstering antioxidant capacity, ultimately contributing to the maintenance of fresh-cut G. elata quality.
However, it is important to acknowledge that current research predominantly resides within the laboratory realm. To validate the true potential and safety of HRW, further investigations are needed, transitioning from the laboratory scale to pilot studies and assessing long-term application effects on agricultural products. This scrutiny is particularly crucial to ascertain whether HRW could exert any biotoxic effects on these products. Despite its promise, the use of H2 in its various forms faces certain challenges. H2 ‘s propensity to escape rapidly in air, low solubility in water, and limited retention capacity are significant hurdles. Consequently, it is imperative to embark on comprehensive research endeavors to develop more rational and efficient strategies for harnessing HRW ‘s potential in applications.
Conclusion
In summary, this study demonstrates that during low temperature storage, HRW treatment enhanced the antioxidant enzyme activities in fresh-cut G. elata and effectively inhibited the activities of PPO and POD, thus maintaining its postharvest quality. Additionally, through HRW treatment, adequate energy levels are sustained, ensuring the proper functioning of the antioxidant system and maintaining the equilibrium of ROS. The potential mechanisms underlying the maintenance of postharvest quality in fresh-cut G. elata by HRW treatment are illustrated in Figure 6.
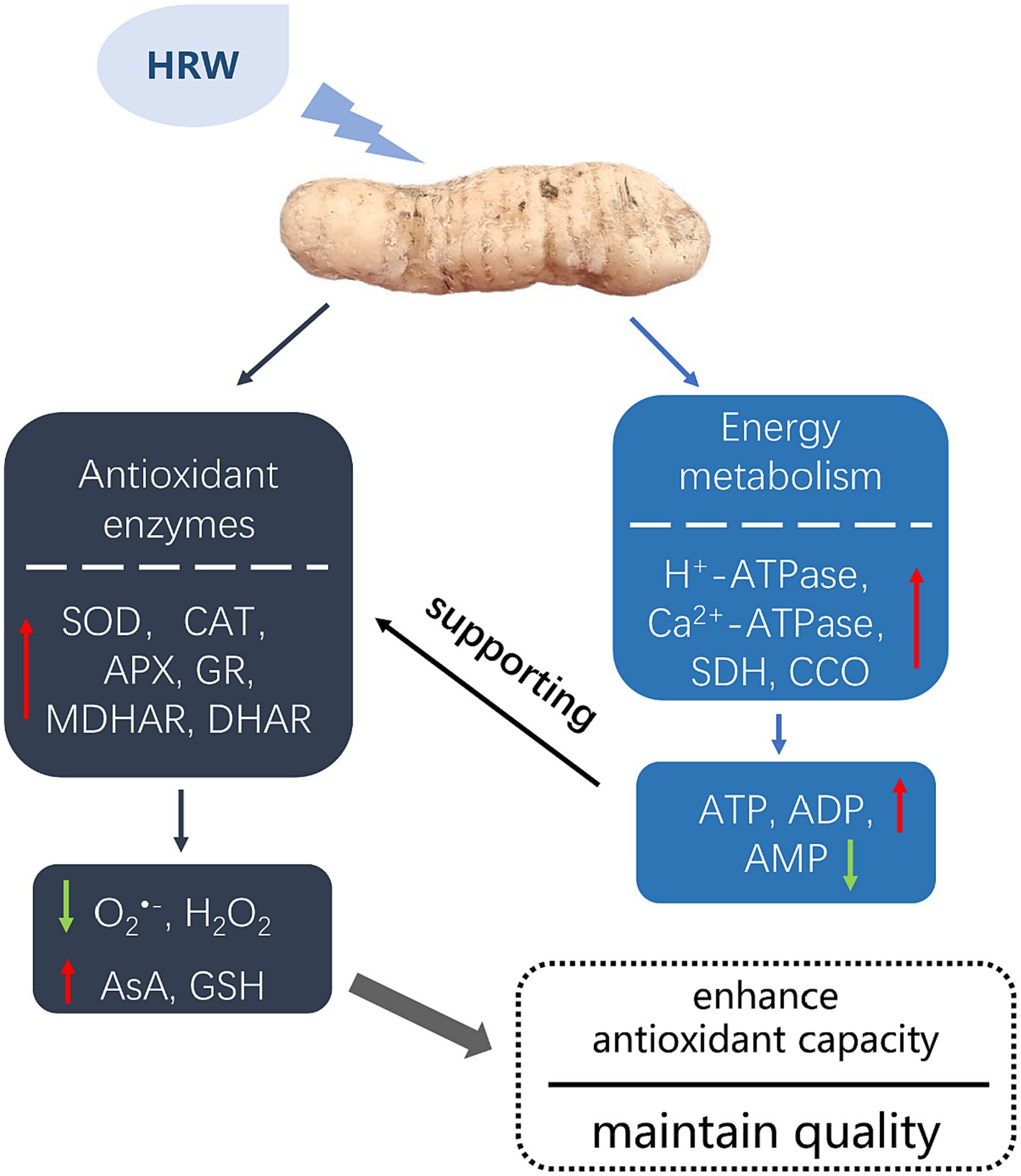
Figure 6. A speculation mechanism to explain the role of HRW in mainting quality in fresh-cut Gastrodia elata.
In our forthcoming research, we will investigate the potential molecular mechanisms of HRW action on quality maintenance in fresh-cut G. elata and lay the theoretical foundation for further applications. Additionally, we recognize the persisting challenges in deploying HRW in agriculture, including the rapid dispersion of H2 in the atmosphere and its limited solubility in water. Hence, further investigations are warranted to optimize the rational and efficient utilization of hydrogen in applications.
Data availability statement
The raw data supporting the conclusions of this article will be made available by the authors, without undue reservation.
Author contributions
BD: Conceptualization, Funding acquisition, Writing – original draft, Writing – review & editing. DZ: Investigation, Methodology, Writing – review & editing. QY: Resources, Software, Writing – review & editing. FD: Software, Writing – review & editing, Formal analysis. YC: Formal analysis, Investigation, Writing – review & editing. XD: Conceptualization, Funding acquisition, Writing – review & editing, Writing – original draft, Data curation.
Funding
The author(s) declare financial support was received for the research, authorship, and/or publication of this article. This study was supported by the Guizhou Provincial Science and Technology Projects [Qian G. Z. K. J. (2021) 172]; National Natural Science Foundation of China (32260795, 32002103); Guizhou Provincial Science and Technology Research Project ‘Open Competition Mechanism’ [Qian Jiao. Ji. (2023) 001]; Guangdong Basic and Applied Basic Research Foundation (2020A1515110092).
Conflict of interest
The authors declare that the research was conducted in the absence of any commercial or financial relationships that could be construed as a potential conflict of interest.
Publisher’s note
All claims expressed in this article are solely those of the authors and do not necessarily represent those of their affiliated organizations, or those of the publisher, the editors and the reviewers. Any product that may be evaluated in this article, or claim that may be made by its manufacturer, is not guaranteed or endorsed by the publisher.
Supplementary material
The Supplementary material for this article can be found online at: https://www.frontiersin.org/articles/10.3389/fsufs.2023.1274701/full#supplementary-material
References
Alwazeer, D., Bulut, M., and Çelebi, Y. (2022). Hydrogen-rich water can restrict the formation of biogenic amines in red beet pickles. Fermentation 8:741. doi: 10.3390/fermentation8120741
An, R., Luo, S., Zhou, H., Zhang, Y., Zhang, L., Hu, H., et al. (2021). Effects of hydrogen-rich water combined with vacuum precooling on the senescence and antioxidant capacity of pakchoi (Brassica rapa subsp. Chinensis). Sci. Hortic. 289:110469. doi: 10.1016/j.scienta.2021.110469
Apel, K., and Hirt, H. (2004). Reactive oxygen species: metabolism, oxidative stress, and signal transduction. Annu. Rev. Plant Biol. 55, 373–399. doi: 10.1146/annurev.arplant.55.031903.141701
Bulut, M., Sezer, Y. Ç., Ceylan, M. M., Alwazeer, D., and Koyuncu, M. (2022). Hydrogen-rich water can reduce the formation of biogenic amines in butter. Food Chem. 384:132613. doi: 10.1016/j.foodchem.2022.132613
Chen, Y., Ge, Y., Zhao, J., Wei, M., Li, C., Hou, J., et al. (2019). Postharvest sodium nitroprusside treatment maintains storage quality of apple fruit by regulating sucrose metabolism. Postharvest Biol. Tec. 154, 115–120. doi: 10.1016/j.postharvbio.2019.04.024
Chen, H., Zhang, J., Hao, H., Feng, Z., Chen, M., Wang, H., et al. (2017). Hydrogen-rich water increases postharvest quality by enhancing antioxidant capacity in Hypsizygus marmoreus. AMB Express 7, 221–210. doi: 10.1186/s13568-017-0496-9
Cheng, B., Tan, C., Tang, X., Chu, Z., Min, J., and Luo, Y. (2020). Study on quality influence of hot air drying and heat pump drying of Xiaocaoba Gastrodia elata. Journal Phys. 1601:042009. doi: 10.1088/1742-6596/1601/4/042009
Ding, X., Zhu, X., Zheng, W., Li, F., Xiao, S., and Duan, X. (2021). BTH treatment delays the senescence of postharvest pitaya fruit in relation to enhancing antioxidant system and phenylpropanoid pathway. Foods 10:846. doi: 10.3390/foods10040846
Dong, B., Zhu, D., Yao, Q., Tang, H., and Ding, X. (2022). Hydrogen-rich water treatment maintains the quality of Rosa sterilis fruit by regulating antioxidant capacity and energy metabolism. LWT-Food Sci. Technol. 161:113361. doi: 10.1016/j.lwt.2022.113361
Fan, S., Tian, W., Wang, Q., Shangguan, C., Liu, X., Zhang, X., et al. (2022). Evaluation of the changes in active substances and their effects on intestinal microflora during simulated digestion of Gastrodia elata. LWT-Food Sci. Technol. 169:113924. doi: 10.1016/j.lwt.2022.113924
Gao, H., Lu, Z., Yang, Y., Wang, D., Yang, T., Cao, M., et al. (2018). Melatonin treatment reduces chilling injury in peach fruit through its regulation of membrane fatty acid contents and phenolic metabolism. Food Chem. 245, 659–666. doi: 10.1016/j.foodchem.2017.10.008
Ge, Y., Chen, Y., Li, C., Wei, M., Li, X., Li, S., et al. (2019a). Effect of trisodium phosphate dipping treatment on the quality and energy metabolism of apples. Food Chem. 274, 324–329. doi: 10.1016/j.foodchem.2018.08.142
Ge, Y., Duan, B., Li, C., Tang, Q., Li, X., Wei, M., et al. (2018). γ-Aminobutyric acid delays senescence of blueberry fruit by regulation of reactive oxygen species metabolism and phenylpropanoid pathway. Sci. Hortic. 240, 303–309. doi: 10.1016/j.scienta.2018.06.044
Ge, Y., Li, X., Li, C., Tang, Q., Duan, B., Cheng, Y., et al. (2019b). Effect of sodium nitroprusside on antioxidative enzymes and the phenylpropanoid pathway in blueberry fruit. Food Chem. 295, 607–612. doi: 10.1016/j.foodchem.2019.05.160
Guan, J., Chen, Z., Guo, L., Cui, X., Xu, T., Wan, F., et al. (2023). Evaluate how steaming and sulfur fumigation change the microstructure, physicochemical properties and in vitro digestibility of Gastrodia elata Bl. starch. Front. Nutr. 9:1087453. doi: 10.3389/fnut.2022.1087453
Hancock, J. T., Russell, G., and Stratakos, A. C. (2022). Molecular hydrogen: the postharvest use in fruits, vegetables and the floriculture industry. Appl. Sci. 12:10448. doi: 10.3390/app122010448
He, M., Wu, Y., Hong, M., Yun, Z., Li, T., and Jiang, Y. (2021). α-Lipoic acid treatment alleviates postharvest pericarp browning of litchi fruit by regulating antioxidant ability and energy metabolism. Postharvest Biol. Tec. 180:111629. doi: 10.1016/j.postharvbio.2021.111629
Hu, H., Li, P., Wang, Y., and Gu, R. (2014). Hydrogen-rich water delays postharvest ripening and senescence of kiwifruit. Food Chem. 156, 100–109. doi: 10.1016/j.foodchem.2014.01.067
Hu, H., Li, P., and Shen, W. (2021). Preharvest application of hydrogen-rich water not only affects daylily bud yield but also contributes to the alleviation of bud browning. Sci. Hortic. 287:110267. doi: 10.1016/j.scienta.2021.110267
Jędrzejuk, A., Rabiza-Świder, J., Skutnik, E., and Łukaszewska, A. (2018). Growing conditions and preservatives affect longevity, soluble protein, H2O2 and MDA contents, activity of antioxidant enzymes and DNA degradation in cut lilacs. Sci. Hortic. 228, 122–131. doi: 10.1016/j.scienta.2017.10.026
Jiang, K., Kuang, Y., Feng, L., Liu, Y., Wang, S., Du, H., et al. (2021). Molecular hydrogen maintains the storage quality of Chinese chive through improving antioxidant capacity. Plan. Theory 10:1095. doi: 10.3390/plants10061095
Jin, P., Zhu, H., Wang, J., Chen, J., Wang, X., and Zheng, Y. (2013). Effect of methyl jasmonate on energy metabolism in peach fruit during chilling stress. J. Sci. Food Agr. 93, 1827–1832. doi: 10.1002/jsfa.5973
Jin, P., Zhu, H., Wang, L., Shan, T., and Zheng, Y. (2014). Oxalic acid alleviates chilling injury in peach fruit by regulating energy metabolism and fatty acid contents. Food Chem. 161, 87–93. doi: 10.1016/j.foodchem.2014.03.103
Li, D., Wang, D., Fang, Y., Li, L., Lin, X., Xu, Y., et al. (2021). A novel phase change coolant promoted quality attributes and glutamate accumulation in postharvest shiitake mushrooms involved in energy metabolism. Food Chem. 351:129227. doi: 10.1016/j.foodchem.2021.129227
Li, F., Hu, Y., Shan, Y., Liu, J., Ding, X., Duan, X., et al. (2022a). Hydrogen-rich water maintains the color quality of fresh-cut Chinese water chestnut. Postharvest Biol. Tec. 183:111743. doi: 10.1016/j.postharvbio.2021.111743
Li, S., Jiang, H., Wang, Y., Lyu, L., Prusky, D., Ji, Y., et al. (2020b). Effect of benzothiadiazole treatment on improving the mitochondrial energy metabolism involved in induced resistance of apple fruit during postharvest storage. Food Chem. 302:125288. doi: 10.1016/j.foodchem.2019.125288
Li, X., Li, C., Cheng, Y., Hou, J., Zhang, J., and Ge, Y. (2020a). Postharvest application of acibenzolar-S-methyl delays the senescence of pear fruit by regulating reactive oxygen species and fatty acid metabolism. J. Agric. Food Chem. 68, 4991–4999. doi: 10.1021/acs.jafc.0c01031
Li, Z., Li, B., Li, M., Fu, X., Zhao, X., Min, D., et al. (2022b). Hot air pretreatment alleviates browning of fresh-cut pitaya fruit by regulating phenylpropanoid pathway and ascorbate-glutathione cycle. Postharvest Biol. Tec. 190:111954. doi: 10.1016/j.postharvbio.2022.111954
Oliveira, M. D. M., Varanda, C. M. R., and Félix, M. R. F. (2016). Induced resistance during the interaction pathogen x plant and the use of resistance inducers. Phytochem. Lett. 15, 152–158. doi: 10.1016/j.phytol.2015.12.011
Ramos-Martinez, J. I. (2017). The regulation of the pentose phosphate pathway: remember Krebs. Arch. Biochem. Biophys. 614, 50–52. doi: 10.1016/j.abb.2016.12.012
Richard-Forget, F. C., and Gauillard, F. A. (1997). Oxidation of chlorogenic acid, catechins, and 4-methylcatechol in model solutions by combinations of pear (Pyrus communis cv. Williams) polyphenol oxidase and peroxidase: a possible involvement of peroxidase in enzymatic browning. J. Agric. Food Chem. 45, 2472–2476. doi: 10.1021/jf970042f
Shu, C., Zhang, W., Zhao, H., Cao, J., and Jiang, W. (2020). Chlorogenic acid treatment alleviates the adverse physiological responses of vibration injury in apple fruit through the regulation of energy metabolism. Postharvest Biol. Tec. 159:110997. doi: 10.1016/j.postharvbio.2019.110997
Wang, H., Cheng, X., Wu, C., Fan, G., Li, T., and Dong, C. (2021). Retardation of postharvest softening of blueberry fruit by methyl jasmonate is correlated with altered cell wall modification and energy metabolism. Sci. Hortic. 276:109752. doi: 10.1016/j.scienta.2020.109752
Wang, R., Yang, X., Chen, X., Zhang, X., Chi, Y., Zhang, D., et al. (2023). A critical review for hydrogen application in agriculture: recent advances and perspectives. Crit. Rev. Environ. Sci. Technol. 1-17, 1–17. doi: 10.1080/10643389.2023.2232253
Wang, Y., Luo, Z., Khan, Z. U., Mao, L., and Ying, T. (2015). Effect of nitric oxide on energy metabolism in postharvest banana fruit in response to chilling stress. Postharvest Biol. Tec. 108, 21–27. doi: 10.1016/j.postharvbio.2015.05.007
Wei, M., Ge, Y., Li, C., Han, X., Qin, S., Chen, Y., et al. (2019). G6PDH regulated NADPH production and reactive oxygen species metabolism to enhance disease resistance against blue mold in apple fruit by acibenzolar-S-methyl. Postharvest Biol. Tec. 148, 228–235. doi: 10.1016/j.postharvbio.2018.05.017
Wei, X., Chen, J., Chen, H., Wu, X., Tian, J., Su, N., et al. (2021). Hydrogen-rich water ameliorates the toxicity induced by ca (NO 3) 2 excess through enhancing antioxidant capacities and re-establishing nitrate homeostasis in Brassica campestris spp. chinensis L. seedlings. Acta Physiol. Plant. 43, 1–12. doi: 10.1007/s11738-021-03220-6
Yi, M., Kong, J., and Yu, Z. (2021). Effect of heat treatment on the quality and energy metabolism in “Golden delicious” apple fruit. J. Food Biochem. 45:e13759. doi: 10.1111/jfbc.13759
Yun, Z., Gao, H., Chen, X., Chen, Z., Zhang, Z., Li, T., et al. (2021). Effects of hydrogen water treatment on antioxidant system of litchi fruit during the pericarp browning. Food Chem. 336:127618. doi: 10.1016/j.foodchem.2020.127618
Keywords: hydrogen-rich water, ROS metabolism, energy metabolism, fresh-cut, Gastrodia elata
Citation: Dong B, Zhu D, Yao Q, Da F, Chen Y and Ding X (2023) Hydrogen-rich water treatment improves fresh-cut Gastrodia elata quality by regulating reactive oxygen species metabolism and energy metabolism during low temperature storage. Front. Sustain. Food Syst. 7:1274701. doi: 10.3389/fsufs.2023.1274701
Edited by:
Duried Alwazeer, Iğdır Üniversitesi, TürkiyeReviewed by:
Menekşe Bulut, Innovative Food Technologies Development, Application and Research Center, TürkiyeGrace Russell, University of the West of England, United Kingdom
Bayram Yurt, Bingöl University, Türkiye
Copyright © 2023 Dong, Zhu, Yao, Da, Chen and Ding. This is an open-access article distributed under the terms of the Creative Commons Attribution License (CC BY). The use, distribution or reproduction in other forums is permitted, provided the original author(s) and the copyright owner(s) are credited and that the original publication in this journal is cited, in accordance with accepted academic practice. No use, distribution or reproduction is permitted which does not comply with these terms.
*Correspondence: Boyu Dong, a3Vyb3JvMTk4NkAxNjMuY29t; Xiaochun Ding, ZGluZ3hjMTExQHNjYmcuYWMuY24=