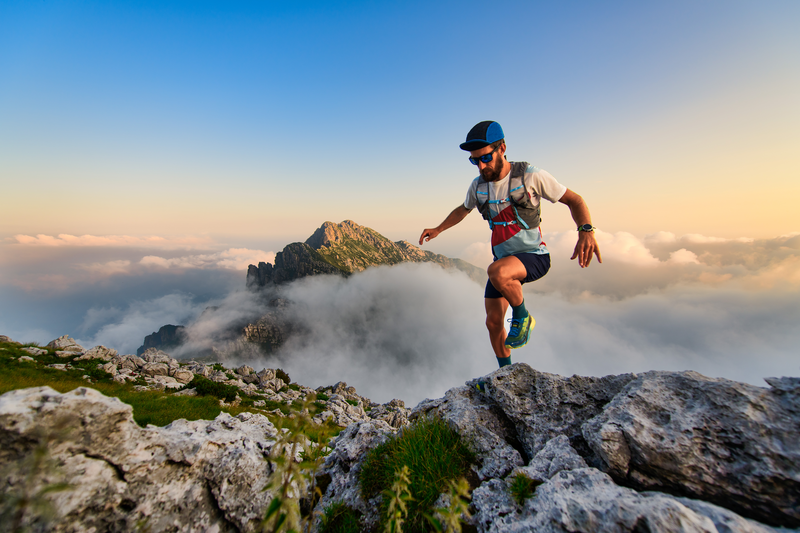
95% of researchers rate our articles as excellent or good
Learn more about the work of our research integrity team to safeguard the quality of each article we publish.
Find out more
ORIGINAL RESEARCH article
Front. Sustain. Food Syst. , 25 October 2023
Sec. Sustainable Food Processing
Volume 7 - 2023 | https://doi.org/10.3389/fsufs.2023.1265091
This article is part of the Research Topic Sustainability and Efficiency of Food Packaging: from Food Preservation to Sale View all 7 articles
Pursuing new packaging that contributes to the safety of meat products, that can also be from renewable sources and that has adequate mechanical properties is a challenge. Therefore, this study attempted to incorporate a layer of polylactic acid (PLA) containing nisin and oregano oil extract (OEO) into biocomposites of cassava bran (CB) and coffee husk (CH) to improve their mechanical properties and enhance their antimicrobial properties. A compression molding process is carried out, the operating conditions for the preparation of the biocomposite were pressure: 40 bar, temperature: 170°C, and time: 2.5 min. The composition of the biocomposite was 80, 18, and 2% of CB, CH, and glycerol respectively, with 30% moisture. The polylactic acid (PLA) layer was added by immersion and contained nisaplin and OEO. PLA layer was evaporated in forced convection oven. Once the active packaging was obtained, inhibition in vitro against Listeria monocytogenes was performed. The packing containing OEO and nisin that showed the highest inhibition on the pathogen was used for stability analysis in pork. In order to measure changes in pork meat quality, the biocomposite with and without coating and with commercial polystyrene packaging were compared. Raw meat muscle was kept at 4° C and was tested for color, pH, moisture, and texture profile analysis (TPA) for 0, 7, 14, and 21 days. The values of the flexural test of the biocomposite were: 11.08 MPa of maximum flexural strength (σF), 1.81% of maximum deformation (εF), and 842.22 MPa of elastic modulus (EM). According to the results of in vitro inhibition, the packaging can contribute to reduce the proliferation of gram-positive microorganisms, such as L. monocytogenes. During storage, the hardness and gumminess of the meat showed significant changes, mainly in the PLA-free biocomposite. Results on pork meat showed that packaging with antimicrobials and PLA can maintain color, texture, moisture, and pH similar to commercial packaging.
The global food market has changed according to social and economic evolution, an example of this is the growth of supermarkets, which require dependent packaging solutions and the extension of the shelf life of food products. Since the last century, the world population has rapidly grown, and economic prosperity in general. According to FAO (2019), approximately 14% of the world’s food produced for human consumption is lost annually, from the harvest to the retail market, which is equivalent to about 400 billion dollars, due to the action of spoilage microorganisms, factors such as oxygen and light that cause oxidation, rancidity and accelerate degradation reactions in food. As a result, new challenges arise to find alternatives that contribute to food preservation.
Many studies are concentrating on testing the stability of traditional preservatives in food packaging systems because they have analyzed the reactions, they may have with the food matrix. However, the current trend of consumers to prefer fresh, chemical-free healthy products must be taken into account. It is also sought that the containers can be recyclable or biodegradable, since traditional synthetic polymers have useful properties, but at the same time, environmental sustainability is not viable, an aspect that makes them remain for thousands of years in the environment, especially in the sea and soil (Mlalila et al., 2018).
According to Margeta et al. (2021) microplastics have been found in various types of marine life such as plankton, marine reptiles, shellfish, lobsters, and crabs, among others; an aspect that generates bioaccumulation in different marine species until reaching humans and also toxic effects due to harmful compounds contained in plastic such as metals, bisphenol A, phthalates or polycyclic aromatic hydrocarbons. Microplastics also affect soil biota because they lead to changes in the microbial community structure and reduce bacterial diversity (Liu et al., 2023). These effects lead to the development of new products focused on more sustainable packaging alternatives with components that can contribute to the extension of the shelf life of food.
Perishable foods, especially meat products, due to their physical and chemical conditions, tend to decompose in less time. For example, one of the main problems associated with the preservation of pork cuts is traditional packaging, due to exposure to oxygen, which causes deterioration of the cuts through the growth of bacteria, fungi, and other microorganisms that decompose the meat, something that occurs mainly in systems that are not airtight; there is also loss of moisture due to exudation of the meat, generating volatile gases inside the packaging that cause undesirable odors and flavors (FAO, 1991; Ajaykumar and Mandal, 2019).
Due to the new challenges in food preservation, commercial and academic interest has focused on the use of biodegradable polymers, added with active agents, to maintain or improve their condition. There are some developments in the area of biodegradable packaging (i.e., packaging made from cassava flour, fique fiber, and plasticizer), but these have a permeable character, and therefore their use for high moisture products is limited, and the costs during their production are not competitive with conventional ones (Cajiao et al., 2016); for this reason, the use of low-cost raw materials such as by-products from agro-industries like coffee and cassava is being sought.
Liu et al. (2009) determined that a coating based on polylactic acid incorporating 2.5% commercial nisin effectively inhibited pathogenic Listeria monocytogenes bacteria in orange juice and liquid egg tests. In their research, Salmieri et al. (2014) evaluated the inhibition of L. monocytogenes in ham by incorporating nisin in PLA-based coatings.
Holcapkova et al. (2018), studied the synergism effect of polyethylene glycol (PEG) in mixtures of polylactic acid (PLA), where polyether acts as the plasticizer, ensuring the long-term antimicrobial activity of bacteriocin nisin. The antibacterial activity of the samples was detected by agar diffusion technique against Micrococcus luteus. The antibacterial properties of the samples were analyzed according to the ISO 22196 standard against Gram-negative (Escherichia coli) and Gram-positive (Staphylococcus aureus, L. monocytogenes) bacterial strains. Nisin is a natural antimicrobial agent produced by Lactococcus lactis and widely used in the food industry, however, its efficacy against L. monocytogenes may decrease due to natural or acquired resistance of this pathogen to nisin, or may also be affected by the complexity of the food environment (Wu et al., 2023). This limitation can be overcome by combining it with other natural compounds such as essential oils that have also shown broad inhibitory power (Anuar et al., 2017; Mir et al., 2018; Requena et al., 2019).
One of the needs of the stakeholders in the pork meat chain is to guarantee optimum packaging that allows the quality of the meat product to be preserved until it reaches the final consumer; in this sense and in response to national demand and international export requirements, the challenge is to design packaging that allows the product to be protected without altering its physical–chemical characteristics; in addition, with environmental trends, packaging must be environmentally friendly. According to our previous studies where the addition of PLA reduces the water absorption in the biocomposite (Muñoz Pabon et al., 2021). The objective of this research proposal was to take advantage of fibrous waste in the elaboration of a biocomposite similar to polystyrene, to test the synergistic effect of a coating based on polylactic acid (PLA) plus nisin and oregano essential oil (OEO), to perform in vitro inhibition tests of L. monocytogenes and to evaluate the stability of pork meat packaged in this biocomposite.
The cassava bran (CB) was supplied by the CLAYUCA Corporation with a moisture of 7.63%, ash 1%, ethereal extract 0.62%, protein 1.9%, fiber 27.2%, and carbohydrates 61.7%, and was sifted on sieve number 60, which corresponds to 250 μm. The coffee husks (CH) were obtained at the TECNICAFE coffee technology park in Colombia, processed in a hammer/blade mill (Penagos, TP8, Colombia), and sifted in a 40 size to a size of less than 425 μm, moisture between 10 and 13%. High molecular weight (120 kDa) polylactic acid (PLA) L (−), supplied by Carguill Dow Polymers LLC (United States) under reference 2002D. Analytical grade methylene chloride, nisaplin (1,000 IU/mg) supplied by Danisco, United States commercial oregano oil, supplied by Now foods, USA. Microorganism for challenge. L. monocytogenes ATCC 51742 were used. Provided by the von Hunbolt cerapium of the Microbiology and applied biotechnology research group (MIBIA) as written in Spanish, of the Universidad del Valle, Colombia.
A mixture of CB, CH and glycerol was prepared, the levels of each quantity were CB 50 to 80%, HC 18 to 30%, glycerol 2 to 4% and moisture 30 to 50%. Then the amounts of each component were weighed and mixed for 10 min following the method of Cajiao et al. (2016). Subsequently, balls of the mixture of 80 g each were weighed, to perform the molding in a compression machine (EDAFA, Medellin) the operating conditions time 2 min, pressure of 40 bar were kept fixed and the temperature was 180°C.
The coating was prepared with 1 g PLA, nisin (1 g = 1,000 UI), and different concentrations of oregano oil extract (OEO), the concentration of nisin was established taking into account the studies of Govaris et al. (2010). Three treatments were evaluated, BIO 1: 150 μL OEO + 1 g nisin; BIO 2: 130 μL OEO, and BIO 3: 1 g nisin. To dissolve the PLA resin and the antimicrobial compounds, 15 mL of methylene chloride was added to a beaker and mixed for 30 min, at the end of this time, the obtained mixture was poured over the biocomposite of 1. 5*5 cm and 1.74 ± 0.06 mm thick, the coated material was left at 30°C in a convection oven (model FD 260, brand Binder, Germany), for 4 h until the solvent evaporated, following the method of Jin et al. (2010).
The mechanical properties were evaluated in accordance with the ASTM D790-10 technical standard (American Society for Testing and Materials, 2010) using a 500 N load cell. Samples with dimensions of 127 mm (length), 12.7 mm (width), and 3.2 mm (thickness) were calibrated with a digital Vernier caliper (CD-6 CSX-B Mitutoyo, Brazil). The test was performed with a universal machine (EZ-L Shimadzu, Japan). The samples were placed on two support structures, located under the ends of the samples. A bending force was then applied to the center of the sample until it fractured. Five samples per treatment were evaluated. Prior to testing, the samples were conditioned for 8 days at 50 ± 5% relative moisture and 23 ± 2°C (American Society for Testing and Materials, 2010). Results were obtained for maximum flexural strength (MPa; σf), maximum deformation (%; εf), and elastic modulus (MPa; EM).
Listeria monocytogenes ATCC 51742 strains were stored at −25°C with 30% v/v glycerol. Bacterial cultures in the exponential growth phase were prepared by inoculating the microbial stock suspensions in Trypticase Soy Broth, followed by incubation at 37°C for 12 h, the inocula were adjusted to obtain bacterial suspensions of 105–6 CFU/mL.
In vitro bacterial inhibition of BIO was evaluated using an agar diffusion method as described by Jin and Zhang (2008). Each sample of the biocomposite was cut into 0.6 mm diameter circles, then placed on the surface of a semi-blotted seeding Brain Heart Infusion (BHI) agar plate. The bacterial density was approximately 105–6 CFU/mL of L. monocytogenes. The plates were incubated at 37°C for 24 h. The diameters of the zone of inhibition were measured with a caliper (Mitutoyo Electronic Digital Caliper 500–196-30, Japan) around the sample to determine the antimicrobial activity. Bicomposites without antimicrobial addition were used as controls.
The texture profile analysis test was performed according to Guill (2004), at 27°C temperature with a texture analyzer (EZ-L Shimadzu, Japan), five samples were taken (14 mm cube) parallel to the longitudinal orientation of the muscle fibers of the central part of each portion of meat. Prior to the analysis, the samples were left to equilibrate in the laboratory at 20°C for 3 h. Each sample was immobilized between stainless steel plates and subsequently compressed, perpendicular to the muscle fiber orientation, in two consecutive cycles of 30% compression with 5 s between cycles. The probe was 4 cm in diameter. The test was performed at a speed of 1 mm/s.
From the resulting force-time curve the following parameters were obtained: hardness (N), maximum force to compress the sample; fracturability (N), the force during the first compression at which the material fractures; Cohesion, extent to which the sample could deform before rupture (A2/A1, where A1 is the total energy required for the first compression and A2 the total energy required for the second compression); elasticity (cm) ability of the sample to recover its original shape after deformation force is removed; Gummability (N/cm2), the force required to disintegrate a semi-solid sample to a stable swallowing state (cohesiveness*hardness); chewability (Ncm), the work required to chew a solid sample to a stable state (gumminess*elastic).
Meat color (n = 3) was determined using a Konica Minolta CM-5 spectrophotometer, controlled by SpectraMagic NX software, with D65 illuminant and 10° observation angle. The samples were analyzed in triplicate, taking approximately 5 g of sample. Luminosity (L) and chromaticity parameters a * (−a * = green and + a * = redness) and b * (−b * = blue and + b * = yellow) were measured. To determine other color properties of the films, ΔE (color difference), YI (yellowness index), and WI (whiteness index) values were calculated using the following equations (where the meat in commercial polypropylene packaging was left as standard and time zero):
Inside each packaged sample, the pH was measured using a FC 232D lance tip (HI 99163, HANNA, United States). Prior to measurement, the pH meter was calibrated with pH 4 and 7 buffer solutions and each sample was tested five times. To avoid contamination during each measurement, the samples taken during the different times were different.
It was carried out under the NTC 1663 of 2009 standard. Glass capsules were weighed, duly cleaned, and dried, then 5 to 7 g of meat were weighed in the capsules and left in a Binder forced convection oven at 150°C for 4 h, after which they were left in a desiccator for 12 h. After this time, the capsules were weighed with the different meat samples, and the moisture calculation was determined with the following equation:
Where.
m0 = is the mass of the capsule in grams.
m1 = is the mass, in grams, of the capsule containing the test portion before drying.
m2 = is the mass in grams of the capsule containing the test portion after drying
To obtain the biocomposite, a factorial design was carried out with four components: moisture, coffee husk, cassava bran, and glycerol. A main component analysis was carried out to explain the correlation of the compounds of the mixture with the stress, deformation, and modulus. For the analysis of the effect of packaging on L. monocytogenes bacteria, a one-factorial design with 3 concentrations (BIO 1, BIO 2, and BIO 3) was performed. BIO 1 was selected as the most inhibitory to test the effect of the packaging on the meat. Three types of packaging were tested T1: biocomposite without active coating, T2: biocomposite containing oil plus nisin and T3: commercial polystyrene packaging, for 21 days. Experimental values were analyzed using Minitab v 18, 2018.
According to the analysis of variance of the factorial design, it is observed that the four individual factors glycerol, CB, CH, and moisture significantly affected (p < 0.05) the σf and the EM. From the interactions, the σf was influenced by the moisture and CB factors, while EM was not significantly influenced by any interaction. Regarding the εf of the biocomposite, no factor significantly influenced it (p > 0.05).
Figure 1 shows main effects graph, where it can be observed that the factors that had the greatest influence on σf and EM were CB, moisture, and CH.
Figure 1. Main effects graph of CB, glycerol, HC and moisture factors. (A) Maximum flexural strength response α = 0.05; (B) Elastic modulus response α = 0.05; (C) Maximum deformation response α = 0.05.
According to these main effects graph, both bran and coffee husk, humidity and glycerol had an effect on σf and EM, while for maximum deformation the previously mentioned factors had no effect. The starch present in the CB can be plasticized by adding glycerol. When glycerol was added above 2%, the σf and the EM decreased below 10 Mpa y 700 Mpa respectively, while the εf above 1.75%. When plasticizer such as glycerol is added, it increases the flexibility or deformation of the material, due to its low molecular size it can mobilize in the spaces between the molecules of the polymer chains, mainly for its affinity with amylose, this behavior could decrease the strength of the hydrogen bonds driving the movement of the molecules and increasing the flexibility, on the contrary, the maximum flexural strength is produced when the starch-starch hydrogen bonds exceed the starch-plasticizer interactions, i.e., when there is less amount of plasticizer in the mixture (Tarique et al., 2021).
According to Figure 2, a positive correlation is observed between CB and CH with stress and modulus, that is, the higher the percentage of bran and husk, the higher the values of σf and EM. From previous studies (Muñoz Pabon et al., 2021) we know that cassava bran is a raw material by-product of the process of obtaining starch from sour cassava, which due to its high starch composition and in the presence of plasticizers and heat can form a resistant matrix with the husk. According to the proximal analysis of the bran, it contains 61.7% carbohydrates mainly starch, which can interact in the presence of heat with the water molecule to cause starch gelatinization and form a thermoplastic polymeric matrix that influences the flexural properties of the biocomposite reinforced with natural fibers (Bustamante and Mosquera Alcy, 2014).
Likewise, according to the statistical analysis, coffee husk had a significant effect (p < 0.05) on σf and EM. It is observed that the influence of the husk content is lower than that of the bran, an aspect that can be attributed to the intrinsic fragility that the fiber can generate on the biocomposite matrix, that is, to the stress-concentrating effect of the fibers. Therefore, larger particles can cause defects in the polymeric matrix, causing it to fracture (Chaiwong et al., 2019).
It is likely that the interaction of the polymeric matrix is not totally uniform, due to the different sizes that the fiber of the coffee husk material may present (larger particles could be acting as flaws in the brittle polymeric matrix, causing premature rupture). Similar behavior was presented by Sánchez-safont et al. (2018) in rice fiber and other products. Similarly, Marengo et al. (2013) studied that fibers obtained from agro-industrial waste at different humidities significantly influenced the mechanical properties of the material. On the other hand Sánchez-safont et al. (2018), in their study of biocomposites from lignocellulosic wastes, observed an increase in flexural modulus with fiber content.
The opposite result is observed with moisture and glycerol, which have a negative correlation with stress and modulus, but a positive correlation with deformation. By increasing the water and glycerol content in the mixture, they serve as plasticizers that decrease the σf and the flexural modulus, that is, a decrease in the strength of the material. This is because, by increasing the water and glycerol content, the molecules available in the medium associated with the hydrophilic components (starch and fiber) form hydrogen bridges, favoring the loss of strength properties, as they promote molecular mobility within the structure. According to some researchers, water acts as a plasticizer, reducing the interactions and proximity between the polymeric chains (amylose/amylopectin) of starch, consequently, low mechanical stresses of the material are obtained, and deformation increases (Mello and Mali, 2014; Navia et al., 2015). Considering these results and previous studies by Muñoz-Pabon et al. (2022), the formulation used to develop the biocomposite was CB 80%, CH 18%, glycerol 2%, and a moisture of 30%. After validation, the results of the biocomposite were σf =11.08 MPa, εf = 1.81% EM = 842. 22 MPa. Also from previous studies, polystyrene presented a flexural stress of 0.8 Mpa. The results of the new biocomposite are similar to those found by Sarsari et al. (2016); in that study, they employed walnut shell and starch.
As seen in Table 1 the three concentrations of antimicrobials have a significant effect on the inhibition of L. monocytogenes bacteria (p < 0.05). BIO 1 presents a greater inhibition diameter, while BIO 2 and BIO 3 have no significant differences.
From preliminary studies (Muñoz-Pabon et al., 2022), it was known that the addition of nisin had no inhibitory effect on Gram-negative bacteria (Salmonella and E.coli), similar results were obtained by Li et al. (2012) and dos Santos et al. (2018), because the cell wall membrane of Gram-negative bacteria, contains lipids, proteins, and lipopolysaccharides, which provide effective protection against biocides such as nisin (Jin and Zhang, 2008), which is a bacteriocin that is biologically active proteins, or protein complexes that have exclusively bactericidal action on Gram-positive bacteria and related species (Moreno et al., 2000).
As shown in Table 1, BIO 1 combined with OEO and nisin had a greater inhibitory effect on the pathogenic bacteria studied, possibly due to the synergistic effect of the antimicrobials. The mechanism of action of plant extracts and essential oils has not yet been fully established, however, studies indicate that compounds such as eugenol and carvacrol, present in oregano oil may inhibit the ATPase enzyme responsible for cell energy generation leading to cell death, furthermore they may act in the permeabilization or disruption of the cytoplasmic membrane, allowing the flow of nonspecific compounds or the release of cytoplasmic contents, respectively (Zhang et al., 2015), the synergistic effect provided by the nisin on the L. monocytogenes is due to the fact that the cell membranes of Gram-positive bacteria, such as the L. monocytogenes, contain large amounts of negatively charged lipids (phosphatidylglycerol and cardiolipin), which could attach to the c-terminus of nisin through electrostatic interactions generating pores in the target cell furthermore nisin blocks the transcription of functional genes, including the stress gene regulator B (sigB), gadD2 and prominent biomarkers targeting the chaperonin (groEL; Wu et al., 2023). BIO 2 added with OEO also presented antimicrobial activity on L. monocytogenes, because OEO contains carvacrol and thymol mainly, the latter can disintegrate the outer membrane of microorganisms and therefore facilitate the release of K+ and ATP, carvacrol is another component that has antimicrobial activity, it can modify the fatty acid profiles and the structure of the cell membrane, as well as influence the proton motive force and the synthesis of flagellin and, therefore, reduce bacterial motility (Yousefi et al., 2020). BIO 3, which only presents nisin, also had an inhibitory effect on L. monocytogenes, because nisin is an antimicrobial peptide classified as a class I bacteriocin, composed of 34 amino acids with a molecular weight of 3.35 kDa that has been widely used in the food industry for its effect on gram-positive bacteria such as the pathogen in our study (Gharsallaoui et al., 2016).
The texture is a predominant element in the acceptability and quality of meat products. The results of TPA parameters of fresh pork meat packed in three types of packaging during storage 0, 7, 14, and 21 days refrigerated at 4°C are presented in Table 2.
The peak of fracturability was not observed in any of the treatments. Both cohesiveness and adhesiveness increase over time for all three treatments, an increase in these values reflects harsh and unattractive products (Trabelsi et al., 2019). The use of the three types of packaging and storage time affected (p < 0.05) the toughness value. The highest toughness value was presented in T1 samples at day 21, while the lowest was observed at T3. There were no significant differences between meat packaged at T2 and T3, possibly due to the fact that the coating allows the meat juices to be retained, as does the commercial packaging. After 21 days of storage, the toughness value of the sample in T3 was 1.46 N compared to 2.11 and 1.55 N for T1 and T2, respectively. The higher toughness of meat packed at T1, can possibly be attributed to moisture loss during storage since this packaging is fibrous attuned to the water present in the meat. Toughness during storage may increase due to oxidative damage to proteins, which has an impact on their solubility, leading to aggregation and formation of cross-linked complexes, carbonyls, accumulation of methmioglobin, and reduction of sulfhydryl groups (Liu and Wang, 2005; Ganhão et al., 2010). In addition, chewiness was significantly higher in the T1-packed meat treatment, suggesting that loss of juices during storage in this packaging negatively influences meat tenderness. The gumminess of the meat is also higher in T1, i.e., in this packaging there is evidence of greater damage to the protein structure of the meat, making it sticky and gummy. The gumminess of the meat is also greater in T1, i.e., in this packaging there is greater damage to the structure of the meat proteins, making it sticky and gummy, while the meat packaged in T2 remains less adhesive, less gummy, i.e., it does not lose its textural characteristics during the 21 days of storage, an aspect that shows the advantages of the PLA coating on the quality of the meat in terms of texture.
Color is an important quality attribute that affects consumer acceptance and can provide information about food freshness. The values of the parameters are presented in Table 3.
The L* values (clarity indicator coordinate) of all samples are affected by storage time at 4°C under aerobic conditions and by the type of packaging (p < 0. 05), likewise the values of b* (yellow trend indicator) are influenced by time and packaging type (p < 0.05), while the values of a* (red trend indicator) are affected by time (p< 0.05) but not by packaging type (p > 0.05). The packaging type * time interactions had no significant effect on parameters a*, b* and L* (p > 0.05).
This finding is in accordance with the results of Chouliara et al. (2007), obtained on color parameters of chicken meat added with oregano oil; similarly Soysal et al. (2015) reported that the type of packaging added with nisin and other antimicrobials have no influence on color. According to the literature, the a* value has a correlation with the chemical status of the pigment myoglobin (Mancini and Hunt, 2005). The decrease in a* value during storage probably indicates discoloration associated with the oxidation of the iron atom within the heme group in oxymyoglobin (red) or deoxymyoglobin to metmyoglobin (brown; Wallace et al., 1982).
In this study, the a* values at day 21 of the samples packed at T2 and T3 are statistically similar compared to T1 packing, also the color change ΔE of T2 is the lowest 0.77, this may be attributed to the antioxidant effect of AO (Lagouri et al., 1993) and nisin which may slightly delay the discoloration to methmioglobin; similar behavior found in some studies (Georgantelis et al., 2007; Siripatrawan and Noipha, 2012).
L* values decreased and b*, increased for all three treatments during the 21 days of testing, an aspect that was evident in YI and WI values. This indicates discoloration of the meat when stored at 4°C, the color of the samples was grayish (a decrease in clarity and a decrease in redness), these changes were probably due to lipid oxidation and microbial decay (Siripatrawan and Noipha, 2012).
An accumulation of hydrogen peroxide produced by lactic acid bacteria during storage is possibly one of the main factors responsible for the discoloration of control samples.
The peroxide produced may cause greening due to oxidation of the heme pigments to form collodylglobin or further decay of the porphyrins to bile pigments (Seideman et al., 1984). According to Tukey’s test for L* parameter, T1 and T3 have no significant difference, compared to meat packed at T2, this change may be due to the antioxidant effect of oregano oil and nisin, which can retain more myoglobin oxidation; similar results were obtained by Siripatrawan and Noipha (2012), in pork samples coated with green tea extract. Chouliara et al. (2007) also reported a tendency to decrease the L* parameter in chicken meat samples packed with oregano oil; it should also be taken into account that the presence of oxygen makes the meat more susceptible to the oxidation process (Mexis et al., 2012). The color differentials related to the type of packaging, the ΔE during the 21 days range from 0.77 to 7.30, according to the ISO 12647-2 standard, it is established that ΔE values lower than 5 are hardly appreciable by the human eye. According to the results, the highest ΔE was found in the T3 package, possibly due to the fact that it does not contain any color-preserving additive.
As shown in Figure 3, the pH values of the pork samples were not influenced (p > 0.05) by the packaging treatments, neither by the packaging*time interaction. But were influenced by the storage time (p < 0.05). During the first 8 days, the meat has values that can be classified as normal; as storage time passes, pH values above 6.2 suggest that the meat can be classified as dark, tough, and dry (Manalo and Gabriel, 2020). This aspect correlates with the increase in YI and the loss of clarity WI.
Figure 3. pH values of raw meat packaged during 21 days. Values are ± standard deviation of five replicates. Data in the same column or row with letters indicate a significant difference (p < 0.05), between storage time.
In the T1 sample, pH increased from 6.11 to 8.3 after 21 days of storage likewise T2 shows an increase in pH from 6.09 to 8.15, for T3, pH increased from 5.82 to 8.28. Other researchers also reported an increase in pH of antimicrobial coated meat during refrigerated storage (Fang et al., 2018); probably due to the aging process where part of the meat proteins are hydrolyzed by endogenous proteases, such as calpains or microbial enzymes into volatile basic nitrogen molecules including ammonia and primary, secondary and tertiary amines, alkaline reacting compounds (Fan et al., 2009; Smaoui et al., 2016).
Although pH increases during storage time relatively lower pH values s up to day 21 of samples packed at T1 and T3 could be the result of a higher number of growing lactic acid bacteria (LAB) compared to samples packed at T2, as oregano oil exhibits an antimicrobial effect against numerous bacteria, including LAB (Hasapidou and Savvaidis, 2011).
Table 4 shows that moisture content was influenced by the type of packaging, storage time, and the interaction of these factors (p < 0.05). There were no significant differences between T3 and T2. This behavior is consistent with that mentioned in the measurement of the previous parameters such as texture, color, and pH, where the coating allows the meat to maintain its characteristics similar to meat packed in polystyrene.
The low moisture content of the meat packed in T1 is due to drip loss, i.e., water and water-soluble proteins leaked out during storage, given that T1 has greater water adsorption, an aspect that was corroborated in this study. This phenomenon, visually, is not very attractive, resulting in drier, less shiny, and tougher meat, additionally, nutrients and soluble proteins decrease, an aspect that represents a significant economic loss for the industrial process (Bedane et al., 2018).
In T2 and T3 packaging, drip loss is lower, with higher moisture retention and less damage to proteins, resulting in more tender meat samples.
The amount of bran and husk significantly affected the bending stress and modulus of the biocomposite material, but not the deformation. The addition of glycerol decreased the maximum flexural strength and increased the maximum deformation of the biocomposite, which is why only 2% was added. The addition of bran and husk increases maximum flexural strength and elastic modulus.
According to the in vitro analysis the Bio 1 material containing OEO and nisin has a greater synergistic effect compared to the biocomposite with only nisin or OEO on the growth of L. monocytogenes. The potential use of OEO and nisin in antimicrobial food packaging can be suggested. According to color parameters, meat packaged in commercial polystyrene packaging or with our active biocomposite are statistically similar. For all treatments, changes in optical properties were evident from day 21. The texture of the meat packaged in our new active biocomposite presented statistically similar characteristics to the commercial packaging, due to the fact that the PLA layer maintains the meat juices during the days of storage. According to quality tests performed on pork meat, the active biocomposite in contact with one side of the product did not prolong shelf-life parameters; however, it can be a substitute for polystyrene. Future work is to evaluate the stability, quality and safety of perishable foods at different temperatures over time.
The raw data supporting the conclusions of this article will be made available by the authors, without undue reservation.
KM-P: Conceptualization, Data curation, Formal analysis, Investigation, Methodology, Software, Validation, Writing – original draft, Writing – review & editing. AA: Conceptualization, Project – administration, Supervision, Validation, Writing – review & editing. HC: Funding acquisition, Investigation, Supervision, Writing – review & editing. JC: Investigation, Methodology, Resources, Supervision, Writing – review & editing. JS: Investigation, Methodology, Resources, Supervision.
The author(s) declare that no financial support was received for the research, authorship, and/or publication of this article.
The authors gratefully acknowledge Parque tecnologico del cafe for providing hulk coffee. We would like to thank the corporation CLAYUCA for providing cassava brand and the Universidad del Cauca for providing the equipment used in the research.
The authors declare that the research was conducted in the absence of any commercial or financial relationships that could be construed as a potential conflict of interest.
All claims expressed in this article are solely those of the authors and do not necessarily represent those of their affiliated organizations, or those of the publisher, the editors and the reviewers. Any product that may be evaluated in this article, or claim that may be made by its manufacturer, is not guaranteed or endorsed by the publisher.
Ajaykumar, V. J., and Mandal, P. K. (2019). Modern concept and detection of spoilage in meat and meat products, meat quality analysis: advanced evaluation methods, techniques, and technologies, Netherlands: Elsevier Inc.
American Society for Testing and Materials. (2010). ASTM D 790-10: Standard Test Methods for Flexural Properties of Unreinforced and Reinforced Plastics and Electrical Insulating Materials. West Conshohocken: ASTM International.
Anuar, H., Nur Fatin Izzati, A. B., Sharifah Nurul Inani, S. M., Siti Nur E’zzati, M. A., Siti Munirah Salimah, A. B., Ali, F. B., et al. (2017). Impregnation of cinnamon essential oil into plasticised Polylactic acid biocomposite film for active food packaging. J. Packaging Technol. Res. 1, 149–156. doi: 10.1007/s41783-017-0022-1
Bedane, T. F., Altin, O., Erol, B., Marra, F., and Erdogdu, F. (2018). Thawing of frozen food products in a staggered through-field electrode radio frequency system: a case study for frozen chicken breast meat with effects on drip loss and texture. Innov. Food Sci. Emerg. Technol. 50, 139–147. doi: 10.1016/j.ifset.2018.09.001
Bustamante, L. E., and Mosquera Alcy, V. C. H. (2014). Propiedades mecánicas de matrices hechas de harina pregelatinizada de yuca variedad Mbra 383 y fique Cambridge, UK: Elsevier Trends Journals.
Cajiao, E. S., Bustamante, L. E., Cerón, A. R., and Villada, H. S. (2016). Efecto de la Gelatinización de Harina de Yuca sobre las Propiedades Mecánicas Efecto de la Gelatinización de Harina de Yuca sobre las Propiedades Mecánicas, Térmicas y Microestructurales de una Matriz Moldeada por Compresión Effect of Cassava Flour Gelati. Información Tecnológica 27, 53–62. doi: 10.4067/S0718-07642016000400006
Chaiwong, W., Samoh, N., Eksomtramage, T., and Kaewtatip, K. (2019). Surface-treated oil palm empty fruit bunch fiber improved tensile strength and water resistance of wheat gluten-based bioplastic. Compos. Part B 176:107331. doi: 10.1016/j.compositesb.2019.107331
Chouliara, E., Karatapanis, A., Savvaidis, I. N., and Kontominas, M. G. (2007). Combined effect of oregano essential oil and modified atmosphere packaging on shelf-life extension of fresh chicken breast meat, stored at 4 °C. Food Microbiol. 24, 607–617. doi: 10.1016/j.fm.2006.12.005
dos Santos, C. A., dos Santos, G. R., Soeiro, V. S., dos Santos, J. R., Rebelo, M. A., Chaud, M. V., et al. (2018). Bacterial nanocellulose membranes combined with nisin: a strategy to prevent microbial growth. Cellulose 25, 6681–6689. doi: 10.1007/s10570-018-2010-1
Fan, W., Sun, J., Chen, Y., Qiu, J., Zhang, Y., and Chi, Y. (2009). Effects of chitosan coating on quality and shelf life of silver carp during frozen storage. Food Chem. 115, 66–70. doi: 10.1016/j.foodchem.2008.11.060
Fang, Z., Lin, D., Warner, R. D., and Ha, M. (2018). Effect of gallic acid/chitosan coating on fresh pork quality in modified atmosphere packaging. Food Chem. 260, 90–96. doi: 10.1016/j.foodchem.2018.04.005
FAO (1991). Guidelines for slaughtering meat cutting and further processing. FAO Anim. Prod. Health Pap. 91, 67–71.
FAO (2019). Food loss and waste. Available at: https://www.fao.org/nutrition/capacity-development/food-loss-and-waste/en/ (Accessed: 20 May 2020).
Ganhão, R., Morcuende, D., and Estévez, M. (2010). Protein oxidation in emulsified cooked burger patties with added fruit extracts: influence on colour and texture deterioration during chill storage. Meat Sci. 85, 402–409. doi: 10.1016/j.meatsci.2010.02.008
Georgantelis, D., Blekas, G., Katikou, P., Ambrosiadis, I., and Fletouris, D. J. (2007). Effect of rosemary extract, chitosan and α-tocopherol on lipid oxidation and colour stability during frozen storage of beef burgers. Meat Sci. 75, 256–264. doi: 10.1016/j.meatsci.2006.07.018
Gharsallaoui, A., Oulahal, N., Joly, C., and Degraeve, P. (2016). Nisin as a food preservative: part 1: physicochemical properties, antimicrobial activity, and Main uses. Crit. Rev. Food Sci. Nutr. 56, 1262–1274. doi: 10.1080/10408398.2013.763765
Govaris, A., Solomakos, N., Pexara, A., and Chatzopoulou, P. S. (2010). The antimicrobial effect of oregano essential oil, nisin and their combination against Salmonella Enteritidis in minced sheep meat during refrigerated storage. Int. J. Food Microbiol. 137, 175–180. doi: 10.1016/j.ijfoodmicro.2009.12.017
Guill, M. D. (2004). Texture profile analysis of meat products treated with commercial liquid smoke flavourings. Food Control 15, 457–461. doi: 10.1016/S0956-7135(03)00130-0
Hasapidou, A., and Savvaidis, I. N. (2011). The effects of modified atmosphere packaging, EDTA and oregano oil on the quality of chicken liver meat. Food Res. Int. 44, 2751–2756. doi: 10.1016/j.foodres.2011.06.011
Holcapkova, P., Hurajova, A., Kucharczyk, P., Bazant, P., Plachy, T., Miskolczi, N., et al. (2018). Effect of polyethylene glycol plasticizer on long-term antibacterial activity and the release profile of bacteriocin nisin from polylactide blends. Polym. Adv. Technol. 29, 2253–2263. doi: 10.1002/pat.4336
Jin, T., and Zhang, H. (2008). Biodegradable polylactic acid polymer with nisin for use in antimicrobial food packaging. J. Food Sci. 73, M127–M134. doi: 10.1111/j.1750-3841.2008.00681.x
Jin, T., Zhang, H., and Boyd, G. (2010). Incorporation of preservatives in polylactic acid films for inactivating Escherichia coli O157: H7 and extending microbiological shelf life of strawberry puree. J. Food Prot. 73, 812–818. doi: 10.4315/0362-028X-73.5.812
Lagouri, V., Blekas, G., Tsimidou, M., Kokkini, S., and Boskou, D. (1993). Composition and antioxidant activity of essential oils from oregano plants grown wild in Greece. Zeitschrift für Lebensmittel-Untersuchung und-Forschung 197, 20–23. doi: 10.1007/BF01202694
Li, W., Coffin, D. R., Jin, T. Z., Latona, N., Liu, C.-K., Liu, B., et al. (2012). Biodegradable composites from polyester and sugar beet pulp with antimicrobial coating for food packaging. J. Appl. Polym. Sci. 116, 1–12. doi: 10.1002/app.36885
Liu, M., Feng, J., Shen, Y., and Zhu, B. (2023). Microplastics effects on soil biota are dependent on their properties: a meta-analysis. Soil Biol. Biochem. 178:108940. doi: 10.1016/j.soilbio.2023.108940
Liu, L., Jin, T. Z., Coffin, D. R., and Hicks, K. B. (2009). Preparation of antimicrobial membranes: Coextrusion of poly (lactic acid) and Nisaplin in the presence of plasticizers. J. Agric. Food Chem. 57, 8392–8398. doi: 10.1021/jf902213w
Liu, W., and Wang, J. Y. (2005). Modifications of protein by polyunsaturated fatty acid ester peroxidation products. Biochimica et Biophysica Acta-Proteins and Proteomics 1752, 93–98. doi: 10.1016/j.bbapap.2005.07.011
Manalo, M. R., and Gabriel, A. A. (2020). Influence of Antemortem and slaughtering practices on the pH of pork and chicken meats. Philipp. J. Sci. 149, 1–19.
Mancini, R. A., and Hunt, M. C. (2005). Current research in meat color. Meat Sci. 71, 100–121. doi: 10.1016/j.meatsci.2005.03.003
Marengo, V. A., Vercelheze, A. E. S., and Mali, S. (2013). Compósitos biodegradáveis de amido de mandioca e resíduos da agroindústria. Química Nova. 36, 680–685. doi: 10.1590/S0100-40422013000500012
Margeta, A., Šabalja, Đ., and Đorđević, M. (2021). The presence and danger of microplastics in the oceans. Pomorstvo 35, 224–230. doi: 10.31217/p.35.2.4
Mello, L. R. P. F., and Mali, S. (2014). Use of malt bagasse to produce biodegradable baked foams made from cassava starch. Ind. Crop. Prod. 55, 187–193. doi: 10.1016/j.indcrop.2014.02.015
Mexis, S. F., Chouliara, E., and Kontominas, M. G. (2012). Shelf life extension of ground chicken meat using an oxygen absorber and a citrus extract. LWT-Food Sci.Technol. 49, 21–27. doi: 10.1016/j.lwt.2012.04.012
Mir, S. A., Dar, B. N., Wani, A. A., and Shah, M. A. (2018). Trends in Food Science & Technology E ff ect of plant extracts on the techno-functional properties of biodegradable packaging fi lms. Trends Food Sci. Technol. 80, 141–154. doi: 10.1016/j.tifs.2018.08.004
Mlalila, N., Hilonga, A., Swai, H., Devlieghere, F., and Ragaert, P. (2018). Antimicrobial packaging based on starch, poly (3-hydroxybutyrate) and poly (lactic-co-glycolide) materials and application challenges. Trends Food Sci. Technol. 74, 1–11. doi: 10.1016/j.tifs.2018.01.015
Moreno, I., Lerayer, A. L. S., Baldini, V. L. S., and Leitão, M. F. F. (2000). Characterization of bacteriocins produced by Lactococcus lactis strains. Braz. J. Microbiol. 31, 183–191. doi: 10.1590/S1517-83822000000300007
Muñoz-Pabon, K. S., González-Callejas, C. A., and Villada-Castillo, H. S. (2022). Biocompuesto bicapa incorporado con nisina: caracterización y eficacia contra Escherichia coli. Información tecnológica 33, 235–244. doi: 10.4067/s0718-07642022000100235
Muñoz Pabon, S. K., Ayala, A., Alfredo, A., Solanilla, D., José, F., and Villada, H. S. (2021). Characterization and antimicrobial efficacy of active biocomposite containing polylactic acid, oregano essential oil and nisin for pork storage. Food Sci. Technol. 2061, 1–15. doi: 10.1590/fst.67420
Navia, D. P., Ayala, A. A., and Villada, H. S. (2015). Biocompuestos de harina de yuca obtenidos por termo-compresión. efecto de las condiciones de proceso. Informacion Tecnol. 26, 55–62. doi: 10.4067/S0718-07642015000500008
Requena, R., Vargas, M., and Chiralt, A. (2019). Study of the potential synergistic antibacterial activity of essential oil components using the thiazolyl blue tetrazolium bromide (MTT) assay. Lwt 101, 183–190. doi: 10.1016/j.lwt.2018.10.093
Salmieri, S., Islam, F., Khan, R. A., Hossain, F. M., Ibrahim, H. M. M., Miao, C., et al. (2014). Antimicrobial nanocomposite films made of poly (lactic acid)-cellulose nanocrystals (PLA-CNC) in food applications: part A-effect of nisin release on the inactivation of Listeria monocytogenes in ham. Cellulose 21, 1837–1850. doi: 10.1007/s10570-014-0230-6
Sánchez-safont, E. L., Aldureid, A., and Lagarón, J. M. (2018). AC SC. Composites Part B. 145, 215–225. doi: 10.1016/j.compositesb.2018.03.037
Sarsari, N. A., Pourmousa, S., and Tajdini, A. (2016). Physical and mechanical properties of walnut shell flour-filled thermoplastic starch composites. Bio Resour. 11, 6968–6983. doi: 10.15376/biores.11.3.6968-6983
Seideman, S. C., Cross, H. R., Smith, G. C., and Durland, P. R. (1984). Factors associated with fresh meat color: a review. J. Food Qual. 6, 211–237. doi: 10.1111/j.1745-4557.1984.tb00826.x
Siripatrawan, U., and Noipha, S. (2012). Active film from chitosan incorporating green tea extract for shelf life extension of pork sausages. Food Hydrocoll. 27, 102–108. doi: 10.1016/j.foodhyd.2011.08.011
Smaoui, S., Hsouna, A. B., Lahmar, A., Ennouri, K., Mtibaa-Chakchouk, A., Sellem, I., et al. (2016). Bio-preservative effect of the essential oil of the endemic Mentha piperita used alone and in combination with bac TN635 in stored minced beef meat. Meat Sci. 117, 196–204. doi: 10.1016/j.meatsci.2016.03.006
Soysal, Ç., Bozkurt, H., Dirican, E., Güçlü, M., Bozhüyük, E. D., Uslu, A. E., et al. (2015). Effect of antimicrobial packaging on physicochemical and microbial quality of chicken drumsticks. Food Control 54, 294–299. doi: 10.1016/j.foodcont.2015.02.009
Tarique, J., Sapuan, S. M., and Khalina, A. (2021). Effect of glycerol plasticizer loading on the physical, mechanical, thermal, and barrier properties of arrowroot (Maranta arundinacea) starch biopolymers. Sci. Rep. 11, 13900–13917. doi: 10.1038/s41598-021-93094-y
Trabelsi, I., Ben Slima, S., Ktari, N., Triki, M., Abdehedi, R., Abaza, W., et al. (2019). Incorporation of probiotic strain in raw minced beef meat: study of textural modification, lipid and protein oxidation and color parameters during refrigerated storage. Meat Sci. 154, 29–36. doi: 10.1016/j.meatsci.2019.04.005
Wallace, W. J., Houtchens, R. A., Maxwell, J. C., and Caughey, W. S. (1982). Mechanism of autooxidation for hemoglobins and myoglobins. Promotion of superoxide production by protons and anions. J. Biol. Chem. 257, 4966–4977. doi: 10.1016/S0021-9258(18)34620-9
Wu, M., Ma, Y., Dou, X., Zohaib Aslam, M., Liu, Y., Xia, X., et al. (2023). A review of potential antibacterial activities of nisin against Listeria monocytogenes: the combined use of nisin shows more advantages than single use. Food Res. Int. 164:112363. doi: 10.1016/j.foodres.2022.112363
Yousefi, M., Khorshidian, N., and Hosseini, H. (2020). Potential application of essential oils for mitigation of Listeria monocytogenes in meat and poultry products. Front. Nutr. 7, 6–11. doi: 10.3389/fnut.2020.577287
Keywords: antimicrobial, valorization, stability, Listeria monocytogenes, natural preservatives, oregano essential oil, nisin
Citation: Muñoz-Pabon KS, Castillo HSV, Concha JLH, Ayala Aponte AA and Solanilla Duque JF (2023) Development of a composite based on polylactic acid and lignocellulosic waste: new packaging for meat food storage. Front. Sustain. Food Syst. 7:1265091. doi: 10.3389/fsufs.2023.1265091
Received: 21 July 2023; Accepted: 02 October 2023;
Published: 25 October 2023.
Edited by:
Guadalupe Virginia Nevárez-Moorillón, Autonomous University of Chihuahua, MexicoReviewed by:
Daniela Sanchez Aldana, Universidad Autónoma de Chihuahua, MexicoCopyright © 2023 Muñoz-Pabon, Castillo, Concha, Ayala Aponte and Solanilla Duque. This is an open-access article distributed under the terms of the Creative Commons Attribution License (CC BY). The use, distribution or reproduction in other forums is permitted, provided the original author(s) and the copyright owner(s) are credited and that the original publication in this journal is cited, in accordance with accepted academic practice. No use, distribution or reproduction is permitted which does not comply with these terms.
*Correspondence: Karen Sofia Muñoz-Pabon, a3NwYWJvbkB1bmljYXVjYS5lZHUuY28=
Disclaimer: All claims expressed in this article are solely those of the authors and do not necessarily represent those of their affiliated organizations, or those of the publisher, the editors and the reviewers. Any product that may be evaluated in this article or claim that may be made by its manufacturer is not guaranteed or endorsed by the publisher.
Research integrity at Frontiers
Learn more about the work of our research integrity team to safeguard the quality of each article we publish.