- 1Guizhou Institute of Dry Grain, Guizhou Academy of Agricultural Sciences, Guiyang, China
- 2Guizhou Jinnong Technology Co., Ltd, Guiyang, China
- 3Urban Governance and Design Thrust, Society Hub, Hong Kong University of Science and Technology (Guangzhou), Guangzhou, China
- 4Innovation, Policy, and Entrepreneurship Thrust, Society Hub, Hong Kong University of Science and Technology (Guangzhou), Guangzhou, China
- 5Institute of Plant Protection, Guizhou Academy of Agricultural Sciences, Guiyang, China
Introduction: To improve maize yield in China, multiple herbicides have been simultaneously applied to control more weeds. However, this combined application raises concerns about potential residues and their subsequent risks to human health. Therefore, evaluating the residues and dietary risk of new herbicide formulations is critical for the sustainability of maize production.
Methods: Using UHPLC-MS/MS, we developed quick methods for the determination of residues of mesotrione, nicosulfuron, atrazine and its four metabolites with acceptable accuracy and precision. The limits of quantification (LOQs) were 0.01 mg/kg for mesotrione and atrazine-desethyl-desopropyl, and 0.005 mg/kg for nicosulfuron, atrazine, 6-deisopropyl atrazine, 2 hydroxyatrazine, and deethylatrazine. Field trials were conducted at 12 different locations in China. And the risk quotient (RQ) model was used to evaluate the chronic risk of residues of these herbicides.
Results: The residues of straw samples were in the ranges of <0.01 mg/kg for mesotrione, <0.005–0.010 mg/kg for nicosulfuron, and <0.037–0.254 mg/kg for accumulated atrazine. In green straw, the observed ranges were <0.01 mg/kg for mesotrione, <0.005–0.007 mg/kg for nicosulfuron, and <0.037–0.211 mg/kg for accumulated atrazine. Meanwhile, for both green and fully-grown maize, residues were <0.01 mg/kg for mesotrione, <0.005 mg/kg for nicosulfuron, and <0.037 mg/kg for accumulated atrazine. And all RQ values of green and fully-grown maize were below 100%.
Conclusion: Based on the RQ model, the dietary risk of exposure to three herbicides through maize was acceptable by consumers. This study helps guide the rational use of mesotrione, nicosulfuron and atrazine to ensure the safe production of maize and our human health.
1. Introduction
Maize (Zea mays L.) is one of the most important cereal crops globally and holds particular significance in China. In 2022, China’s maize sown area was 43.07 million hectares, producing 277.20 million tons of maize annually (National Bureau of Statistics of China, 2022). As a staple food for a large proportion of the population, maize is an integral part of food security. Besides its role in human nutrition, maize is also a fundamental raw material for various industries like starch production and biofuel (Ranum et al., 2014). Equally significant is maize straw, the agricultural waste from maize cultivation, which have diverse uses including fertilization, animal feed, fuel, base stock for edible fungi, and raw materials for biodegradable products (National Development and Reform Commission of the People’s Republic of China and Ministry of Agriculture of the People’s Republic of China, 2016; National Development and Reform Commission of the People’s Republic of China, 2021; Liu et al., 2022).
The yield of maize can be severely affected by the presence of weeds, with estimates of potential grain yield losses ranging from 37 to 44% in maize due to such competition (Oerke, 2006). As an effective approach, herbicides have been widely utilized to control the effects of weeds and improve yields in China for over 40 years (Zhao et al., 2017). Presently, a variety of herbicides, including mesotrione, nicosulfuron, and atrazine (Figure 1), have been individually utilized in maize fields. Mesotrione is a selective herbicide used primarily for pre- and post-emergence control of broadleaf weeds and some grasses in maize fields. It acts as a highly effective inhibitor of 4-hydroxyphenylpyaunate dioxygenase (HPPD), quickly absorbed by weeds and distributed throughout the plant, while maize exhibits tolerance to it due to selective metabolism and slower uptake, making it a suitable selective herbicide in maize cultivation (Mitchell et al., 2001). Nicosulfuron can control annual weeds, e.g., giant foxtail, increasing grain yield of maize through enhanced post-emergence weed control (Dobbels and Kapusta, 1993; Kapusta et al., 1994). As for atrazine, it has a longer history of use and shows efficacy against a broad spectrum of weeds, mainly annual broadleaf and grass species, which can be degraded to the metabolites including 6-deisopropyl atrazine (DIA), Deethylatrazine (DEA), 2-hydroxyatrazine (HA) and Atrazine-desethyl-desisopropyl (DACT) (Figure 1; Skrzypczak et al., 2011).
Despite their effectiveness in weed control, the presence of herbicide residues and their metabolites in maize raises considerable concern about potential health hazards to consumers, like the association between exposure to specific herbicides and the development of human diseases (Morrison et al., 1992). In particular, mesotrione has been found to potentially stimulate the growth of breast cancer cells (Jabłońska-Trypuć et al., 2020). Nicosulfuron is known for its environmental persistence. Studies indicated that it may induce hypoglycemia in humans, increase the risk of cardiovascular disease, and exert toxic effects on human serum albumin, which can lead to peptide chain alterations and protein denaturation (Olayinka et al., 2022; Zhong et al., 2023). As for atrazine, a positive association was found between its exposure and preterm birth (Rinsky et al., 2012). And dermal and ingestion exposures to atrazine-contaminated soil may pose cancer risks to humans when the residues exceed the US EPA limit (US EPA, 2018; Dehghani et al., 2022). In addition, studies have shown that atrazine and their metabolites may have endocrine disrupting properties and may have clastogenic potential at high concentrations (MacLennan et al., 2003; Jowa and Howd, 2011). In general, health risks associated with herbicides depend on the extent of exposure, including direct contact and bioaccumulation through the food chain. Therefore, understanding and evaluating herbicide residues in maize is critical since it provides the basis for assessing the potential health risks associated with herbicide exposure and facilitates informed decision-making regarding safe agricultural practices.
Some studies have investigated residue levels after the application of mentioned herbicides in maize (Sun et al., 2013; Tandon and Singh, 2015; Ahmadi et al., 2017). However, they have predominantly focused on the analysis of individual herbicides, neglecting the intricate complexities that arise from the simultaneous application of multiple herbicides. As a result, the residue patterns from mixed herbicide applications may differ significantly from those observed with single herbicide applications. Besides, only a few studies have addressed the potential risks posed by the metabolites of these herbicides, which raise additional concerns for human health (Alferness and Wiebe, 2002; Du et al., 2017). In addition, the influence of different climatic conditions, including precipitation, temperature, and soil characteristics, on the variation of residue levels in different regions within China must be considered (Tong et al., 2018). Although several geographically specific studies have been conducted (Wu et al., 2012; Du et al., 2017; Tong et al., 2018; Liu et al., 2021), a comprehensive assessment, considering diverse diets of a larger population in the country, is still needed to evaluate the safety level of rational herbicide applications. Thus, there is an urgent need to evaluate the determination of residues and associated dietary risks associated with the new formulation of mesotrione, nicosulfuron, and atrazine in different geographical locations. Such an evaluation will provide invaluable insights that will enable growers to make informed decisions about the appropriate use and production of maize products while maintaining acceptable risk.
However, the polarity of herbicides and metabolites are quite different (Martín-Pozo et al., 2023). More importantly, the components in the straw and maize are diverse (Wang D. et al., 2022; Mattoo et al., 2023). These can produce various matrix effects influencing analytical efficiency. To quickly determine the residues in four matrices with high precision and accuracy, in this study, methods (including two extraction procedures and three elution programs) were developed for the determination of mesotrione, nicosulfuron, atrazine and its four metabolites. And the field trials were conducted in 12 locations in China, followed by the residue determination of seven chemicals in green maize, fully-grown maize, green straw and straw. Based on the dietary composition of different age groups in urban and rural areas, we finally conducted a comprehensive dietary risk assessment of mesotrione, nicosulfuron and atrazine. This study can not only provide evidence for the safe application of these three herbicides, but also promote the sustainable production of maize in China.
2. Materials and methods
2.1. Chemicals and reagents
Mesotrione (C14H13NO7S, CAS No. 104206–82-8, 98.7% purity) was purchased from the National Quality Inspection and Testing Center for Pesticide Products (Nanjing, China). Nicosulfuron (C15H18N6O6S, CAS No. 111991–09-4, 99.1% purity) was purchased from Alta Scientific Co., Ltd. (Tianjing, China). 6-deisopropyl atrazine (DIA, C5H8ClN5, CAS No. 1007-28-9, 98.9% purity) was bought from CATO Research Chemicals Inc. (Guangzhou, China). Atrazine (C8H14ClN5, CAS No. 1912-24-9, 98.0% purity), Deethylatrazine (DEA, C6H10ClN5, CAS No. 6190-65-4, 98.0% purity), 2-hydroxyatrazine (HA, C8H15N5O, CAS No. 2163-68-0, 98.0% purity) and Atrazine-desethyl-desisopropyl (DACT, C3H4ClN5, CAS No. 3397-62-4, 97.2% purity) were purchased from A Chemtek Inc. (Worcester, USA). A 30% concentrated oil-based suspension (4% mesotrione, 6% nicosulfuron, 20% atrazine) was obtained from Shanghai Shennong Pesticide Co., Ltd. (Shanghai, China).
HPLC-grade acetonitrile (ACN), formic acid, aqueous ammonia and methanol and MS-grade ammonium acetate were bought from Beijing Mairuida Technology Co., Ltd. (Beijing, China). Two milliliter syringes and 0.22 μm nylon syringe filters were purchased from Jiangsu Zhiyu Medical Equipment Co., Ltd. (Jiangsu, China) and Tianjin Jinteng Experimental Equipment Co., Ltd. (Tianjin, China), respectively.
2.2. Field trials
All field experiments were carried out between April 2021 and October 2021 at the following 12 sites in China: Shaoxing city, Zhejiang province; Changsha city, Hunan province; Nanning city, Guangxi province; Daxing district, Beijing; Shenyang city, Liaoning province; Lanzhou city, Gansu province; Zhengzhou city, Henan province; Hohhot, Inner Mongolia; Changchun city, Jilin province; Qingdao city, Shandong province; Guiyang city, Guizhou province and Danzhou city, Hainan province. All treatments were conducted based on the Guidelines on Pesticide Residue Trials in China (NY/T 788–2018). Each site was divided into two 100 m2 areas, which were separated from each other by a buffer zone and protective signs.
After mixing 18 ml of the concentrated oil-based suspension with 6 L of water, the 100 m2 experimental area was treated by stem-leaf spraying when the crop was at the three-leaf stage. Green maize (at least 2 kg) and fully-grown maize (at least 1 kg) were collected from at least 12 plants randomly. Green straw and straw samples were harvested from not less than 12 plants randomly. Each plant should be cut into three equidistant segments (including leaves). The composite sample consisting of four top, four middle and four bottom segments with a total weight of at least 2 kg should then be assembled. All samples were transported to the laboratory stored at the −18°C chamber.
2.3. Extraction of samples
Green maize was cut into 1–2 cm pieces, and fully-grown maize was threshed. Green straw and straw were cut into small pieces smaller than 1 cm, completely mixed in stainless steel pots, labeled, and stored at −18°C.
A weight of 2.5 ± 0.1 g of each homogenized tissue was placed into the 50 mL centrifuge tube. Two extraction procedures were used. For the determination of mesotrione, acetonitrile/water (7:3, v/v, 25 ml) was added to the tube and vortexed for 15 min. The mixture was centrifuged for 5 min at 4000 rpm in a model CL5 centrifuge. The supernatant was then injected through a 0.22 μm nylon syringe filter and transferred to a glass vial for subsequent analysis. For the determination of nicosulfuron, atrazine and its four metabolites, acetonitrile/water/formic acid (7:2.9:0.1, v/v/v, 25 ml) was injected. After that, the mixture was vortexed for 15 min and centrifuged for 5 min at 4000 rpm in the same centrifuge. The supernatant was filtered through a 0.22 μm nylon syringe filter and then transferred to a glass vial for subsequent UHPLC–MS/MS analysis.
2.4. UHPLC–MS/MS analysis
All samples were analyzed by an UHPLC–MS/MS system with an electrospray ionization (ESI) source (1290–6,470, Agilent, CA, USA). The analytical column (ZORBAX Eclipse C18; 3.0 × 50 mm, 1.8 μm; Agilent) maintaining 40°C was applied for the separation. For the analysis of mesotrione, the injection volume was 2 μl. The mobile phases consisted of 5 mmol ammonium acetate (solvent A) and methane (solvent B). And the elution program was set as follows: 0–0.5 min, 90% A; 0.5–1 min, 90–5% A; 1–3 min, 5% A; 3–3.1 min, 5–90% A; 3.1–4.5 min, 90% A. As for the analysis of DACT, the injection volume was 1 μl. The mobile phases consisted of methane (solvent B) and 0.02% aqueous ammonia (v/v, solvent C). The elution program was set as follows: 0–1.5 min, 15–60% B; 1.5–2 min, 60–95% B; 2–2.1 min, 95–15% B; 2.1–3.5 min, 15% B. For the analysis of other five analytes, the volume of injection was 1 μl. 0.1% formic acid (v:v, solvent D) and acetonitrile (solvent E) were consisted of the mobile phases. The elution program was as follows: 0–3 min, 5–60% E; 3–4 min, 60–95% E; 4–4.1 min, 95–5% E; 4.1–5.5 min, 5% E.
Every substance was determined with the corresponding commercial standards by multiple reaction monitoring (MRM) mode using an ESI mass spectrometer. The common MS operating conditions were as follows: gas temperature 300°C; gas flow 7.0 L/min and sheath gas flow 11.0 L/min. Other detection parameters and fragmentation transitions for qualification and quantification were listed in Supplementary Table S1.
As for quality control, two concentrations were used for each batch. The concentrations of mesotrione and DACT were set as 0.01 mg/kg and 0.05 mg/kg. 0.005 mg/kg and 0.1 mg/kg were chosen as the set concentrations. The added concentrations of atrazine, DIA, DEA and HA were 0.005 mg/kg and 0.05 mg/kg. Two parallels were provided for each concentration as quality control samples. And quantification was performed by the external standard single-point comparison method. Results of their recoveries were presented in Supplementary Table S2.
2.5. Method validation
According to SANTE/11312/2021 and NY/T 788–2018, the method was validated from the determination of sensitivity, linearity, accuracy and precision (Ministry of Agriculture and Rural Affairs of the People’s Republic of China, 2018; European Commission, 2021). For the evaluation of linearity, the standard of each herbicide were analyzed at five concentrations with three replicates (0.0005 mg/L, 0.001 mg/L, 0.005 mg/L, 0.01 mg/L, and 0.02 mg/L for mesotrione; 0.0005 mg/L, 0.001 mg/L, 0.005 mg/L, 0.02 mg/L, and 0.05 mg/L for DACT; 0.00025 mg/L, 0.0005 mg/L, 0.005 mg/L, 0.01 mg/L, and 0.05 mg/L for nicosulfuron, atrazine, DIA, DEA and HA). The slope, coefficient of determination (R2), intercept of every fitted curve was determined. And the matrix effect (ME) was determined by the equation (1), where k1 and k2 represents the slopes in matrix and in solvent, respectively.
To calculate the average recovery, three concentrations of seven analytes (0.01 mg/kg, 0.05 mg/kg and 0.1 mg/kg for mesotrione; 0.005 mg/kg, 0.1 mg/kg and 0.2 mg/kg for nicosulfuron; 0.01 mg/kg, 0.05 mg/kg and 0.2 mg/kg for DACT; 0.005 mg/kg, 0.05 mg/kg and 0.2 mg/kg for atrazine, DIA, DEA and HA) were spiked to different blank samples with five replicates per level. The lowest validated concentration with an acceptable recovery of ≥70% and a relative standard deviation (RSD) of less than 20% was defined as the limit of quantitation (LOQ) (Wang et al., 2023).
2.6. Dietary risk assessment
To keep the safe use of mesotrione, nicosulfuron and atrazine, the risk quotient (RQ) model was applied for the evaluation (Wang D. et al., 2022; Wang W. et al., 2022). It can determine the chronic risk of these three herbicides evaluated as RQ that can be calculated using formula (2) and (3):
NEDI (mg/kg bw) is the national estimated daily intake, ADI (mg/kg bw) represents the acceptable daily intake (0.5 mg/kg for mesotione, 2 mg/kg for nicosulfuron and 0.02 mg/kg for atrazine) obtained from official information of The Joint FAO/WHO Meeting on Pesticide Residues (2007, 2014a,b), STMRi (mg/kg) refers to the supervised median residue in the field trials, and Fi is the dietary intake (g/d). The risk is correlated with the value of the RQ. RQ ≤100% means that the chronic risk to humans is acceptable, whereas RQ >100% means that the risk is unacceptable.
Considering that atrazine can be transformed to four metabolites, the residue of accumulated atrazine (C) for the evaluation of dietary risk was expressed as follows.
In the equation (4), CAtrazine, CDIA, CDEA, CHA, and CDACT indicate the residue level of atrazine, DIA, DEA, HA and DACT, respectively. And values of 1.242, 1.149, 1.093 and 1.482 represents the corresponding ratio of the molecule weight of DIA, DEA, HA and DACT to it of atrazine.
3. Results and discussion
3.1. Method validation
During the development of the methods, the detector response of each standard solution of seven substances was compared with that of four matrices added with different concentrations of the analytes, which also determined the matrix effects. According to the results, the values of respective peak area were obtained from the measurement of the analyte concentration in the different standard working solutions. At last, a linear regression equation was established. As shown in the Supplementary Table S3, over the range of five concentrations, the linearity between each concentration and the corresponding peak area was acceptable since all correlation coefficients (R2) were all higher than 0.99. And the representative chromatograms were presented in Figure 2.
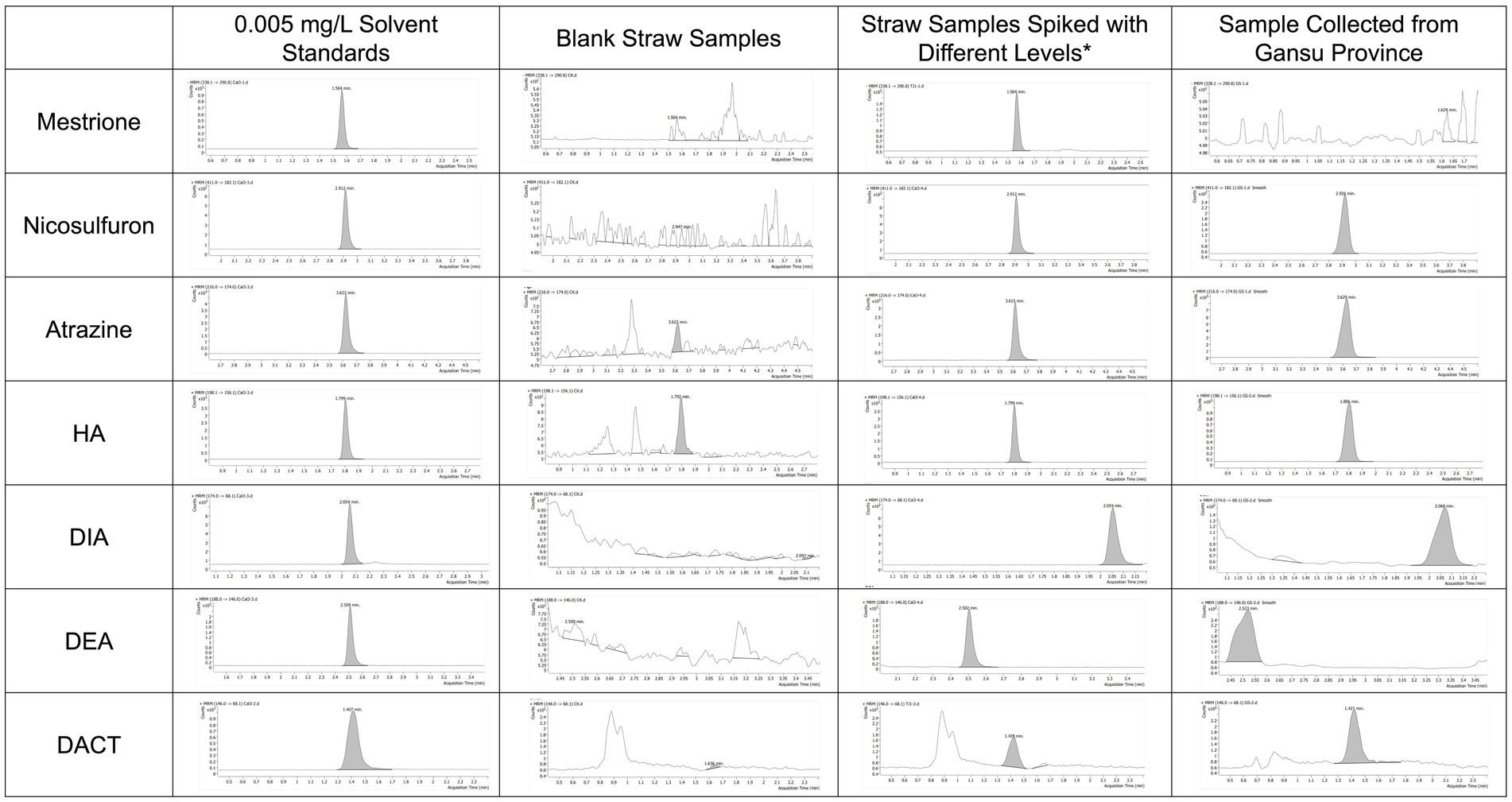
Figure 2. The representative UHPLC–MS/MS chromatograms. * Represents straw samples spiked with 0.01 mg/kg for mesotrione and DACT as well as 0.005 mg/kg for nicosulfuron, atrazine, HA, DIA and DEA. HA, 2-hydroxyatrazine; DIA, 6-deisopropyl atrazine; DEA, Deethylatrazine; DACT, Atrazine-desethyl-desisopropyl.
Matrix effect (ME) can be triggered by the interaction between the co-ionization of the ESI source and the components in different matrices influencing the quantitative accuracy of these methods. The absolute values of ME of three metabolites of atrazine (DIA, DEA, and HA) in green maize, fully-grown maize, green straw and straw were all below than 20%, which may represent the effects could be moderate. For atrazine, only the ME value in green maize was higher than the parameter (20%) showing matrix suppression, which was similar in previous studies (Cunha and Fernandes, 2011; Daniel and Lucio, 2019). In addition, strong MEs of mesotrione and DACT in four matrices could not be ignored. For mesotrione, higher ME values were also determined in maize grain (64%) and maize straw (41%) by Pang et al. (2016). Du et al. (2017) also found that the MEs of the herbicide in rice, rice hull and rice plant were significant. As for DACT, considerable MEs could be also found in some products like Astragali radix, Glehniae radix, Cuscutae semen, and mussels from Adriatic Sea (Liu et al., 2018; Interino et al., 2023). However, it was interesting that a slight matrix inhibition could be observed in other sea products like crayfish, carp and Chinese mitten crab collected from Hubei and Hunan Provinces in China (Peng et al., 2023).
To guarantee the accuracy of these methods, matrix-matched standard curves were chosen for quantitative analysis. 0.01 mg/kg was regarded as the values of the limit of quantitation (LOQ) for mesotrione and DACT in these four matrices. And 0.005 mg/kg was defined as the LOQ values of nicosulfuron, atrazine, DIA, DEA, and HA.
When it comes to the accuracy and precision of these methods, based on the results (Supplementary Table S4), all recoveries and RSD values were in compliance with the demands of the Guidelines on Pesticide Residue Trials in China (NY/T 788–2018). Specifically, when the spiked levels of seven herbicides in four matrices were in the range (0.001 mg/kg < spiked levels ≤0.01 mg/kg), the values were in the ranges of 78–101% for recoveries and 1.8–15.4% for relative standard deviation (RSD). As for the range (0.01 mg/kg < spiked levels ≤0.1 mg/kg), the recoveries and RSD values of seven substances in matrices were ranged from 79 to 101% and 0.3 to 6.1%, respectively. For the spiked level of 0.2 mg/kg, the range of recoveries was from 79 to 98% and it of RSD values was from 0.6 to 12.5%.
In conclusion, the methods can be applied for the residue analysis of mesotrione, nicosulfuron, atrazine, and its four metabolites in the samples of maize crops collected from different fields.
3.2. Terminal residues of seven substances in four matrices of maize
After the application of three herbicides, the residues of them and relative metabolites were determined (Supplementary Table S5). For green straw and straw samples, the residues of mesotrione were all lower than 0.01 mg/kg. In straw samples, the range of nicosulfuron residue was from <0.005–0.010 mg/kg. The residues of atrazine and its four metabolites were in the ranges of <0.005–0.140 mg/kg for atrazine, <0.005–0.009 mg/kg for DIA, <0.005–0.005 mg/kg for DEA, <0.005–0.029 mg/kg for HA and < 0.005–0.027 mg/kg for DACT. Thus, the accumulative atrazine residue was in the range of <0.037–0.254 mg/kg. As for green straw samples, the range of nicosulfuron residues was in the range of <0.005–0.007 mg/kg. The HA residues were all less than 0.005 mg/kg. In addition, the residues of atrazine, DIA, DEA and DACT were from <0.005–0.090 mg/kg, <0.005–0.006 mg/kg, <0.005–0.063 mg/kg and < 0.005–0.024 mg/kg, respectively. And the accumulative atrazine levels were from <0.037–0.211 mg/kg. All residues in straw samples were all less than maximum residue levels (MRLs) set by USA (0.01 mg/kg for mestrione, 0.1 mg/kg for nicosulfuron and 0.5 mg/kg for atrazine).
However, compared with the residues among these locations (Figure 3), the levels in Gansu province were generally higher than those of other places in China. It may be influenced by the higher annual mean temperature and less annual mean precipitation, which may be correlated to relatively small vegetation and forest areas as well as the desertification problem (He et al., 2022; Zhao and He, 2022). Therefore, nature resources and climate conditions in the ecological environment could play an important role in the quality and safety of agricultural products, which are also quite crucial for the sustainable agriculture (Delcour et al., 2015; Seleiman and Hafez, 2021).
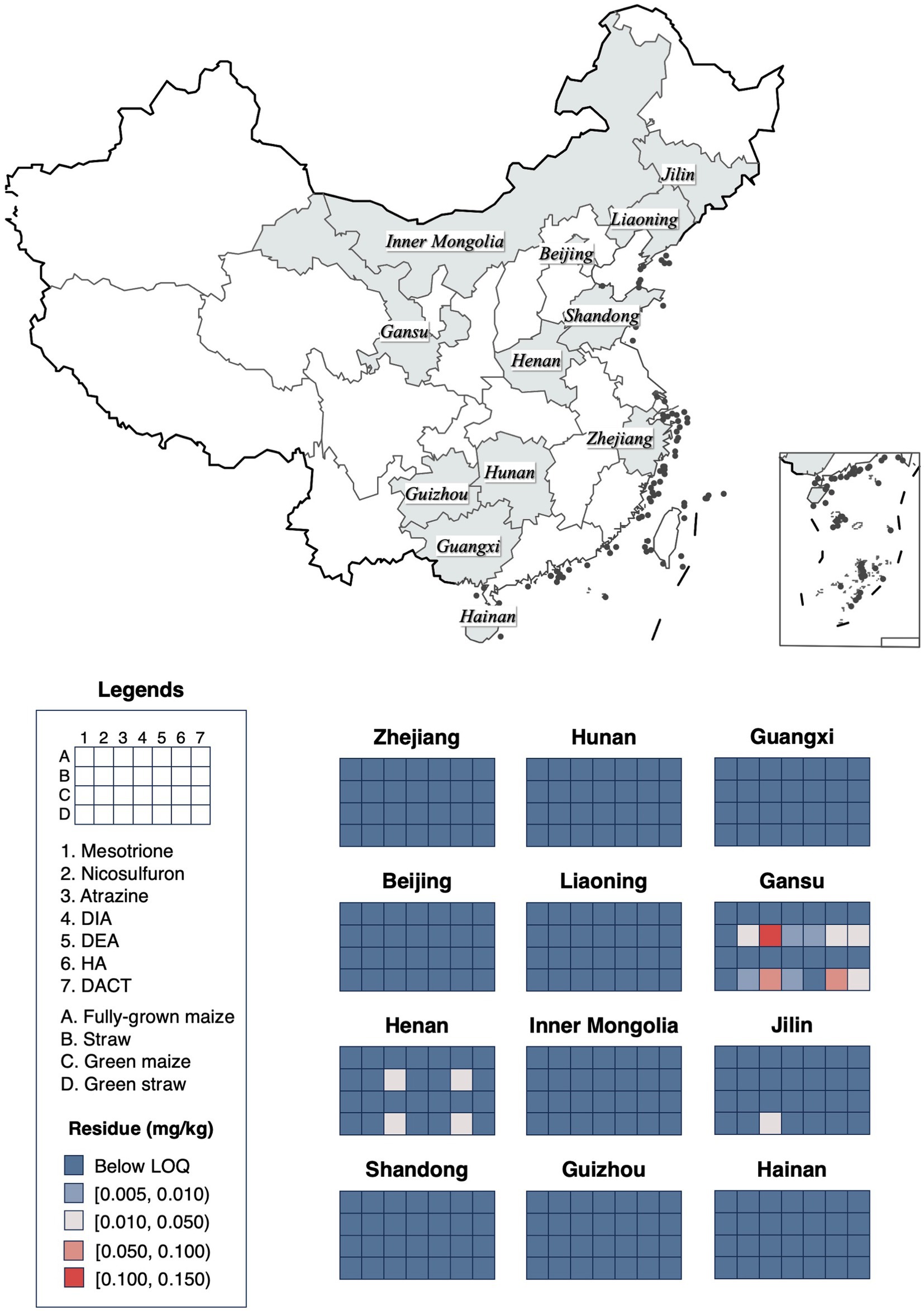
Figure 3. The residues of mesotrione, nicosulfuron, atrazine and its four metabolites in four matrices of different locations. HA, 2-hydroxyatrazine; DIA, 6-deisopropyl atrazine; DEA, deethylatrazine; DACT, atrazine-desethyl-desisopropyl.
Because of the wide utilization of straws, the potential risk of them to human health must be highlighted. Based on the official reports for the comprehensive utilization of straw, it knew that the using amount of straw was nearly 720 million tons, whose comprehensive utilization rate reached 80.1% (National Development and Reform Commission of the People’s Republic of China and Ministry of Agriculture of the People’s Republic of China, 2016; People’s Government of Yunnan Province, 2022). Specifically, 43.2% maize straws can be applied in straw cover to amend soils. Nevertheless, the residues of straw can influence soil microorganism constructure as well as the soil properties. Then it could make undesirable effects on the agriculture products (Inamuddin Ahamed and Lichtfouse, 2020; Siedt et al., 2021). And 18.8% straws can be used for the animal feeds. However, after the digestion by animals, the residues could be enriched in their bodies. Through the food chain, higher residual level could be consumed by human (Inamuddin Ahamed and Lichtfouse, 2020; Fu et al., 2022). Also, 2.7% maize straws can be used to produce eco-friendly tableware such as straw chopsticks. And these materials can be used by consumers. Therefore, the residues of straws can indirectly pose hazards to human health through these mentioned pathways, which deserve more attention in future work.
For the samples of fully-grown maize and green maize, the residues of mesotrione and DACT were lower than 0.01 mg/kg. And the residues of nicosulfuron, atrazine, DIA, DEA and HA were all lower than the corresponding LOQ value (0.005 mg/kg). As for the sum of atrazine residues, the values of two kinds of maize were all lower than 0.037 mg/kg. In summary, the residues of mesotrione, nicosulfuron and atrazine of maize were all below MRLs set by different regions (0.01 mg/kg in Japan, CAC, China, EU and US for mesotrione; 0.01 mg/kg in CAC and EU as well as 0.1 mg/kg in Japan, China and US for nicosulfuron; 0.05 mg/kg in CAC, China and EU as well as 0.2 mg/kg in Japan and US for atrazine). It also means that the rational use of these mixed herbicides will not influence the export of maize in these regions.
3.3. Dietary exposure risk of Mesotrione, Nicosulfuron and atrazine In maize
Chronic dietary risk assessment is a crucial approach to evaluate the safety of agricultural product after the application of chemicals in fields. For a more comprehensive risk assessment, gender, age and region should be considered (Lan et al., 2022). Based on the dietary structure and population characteristics of Chinese residents (Jin, 2008), the body weights were ranged from 13.2–69.3 kg for urban residents and 12.1–64.7 kg for rural residents. Besides, the consumptions of maize in these two regions were also different. The fully-grown maize consumptions were in ranges of 7.0–21.5 g/d for urban regions and 10.3–33.0 g/d for rural regions. For the consumption of green maize, the ranges were from 73.1–173.6 g/d for urban people and 82.4–225.5 g/d for rural people. All calculation process of different groups in two regions was presented from Supplementary Tables S6–S8.
Due to the higher STMR and lower ADI of atrazine on two categories of maize, the risk of this chemical was higher than that of mesotrione or nicosulfuron (Supplementary Table S9). When it comes to the risk of fully-grown maize in two regions, the RQ values were from 0.00027 to 0.00183% for mesotrione, 0.00003 to 0.00023% for nicosulfuron and 0.02467 to 0.16922% for atrazine. But the RQs of green maize were generally higher than fully-grown maize because of its higher consumption, whose values were in the ranges of 0.00402–0.01424% for mesotrione, 0.00050–0.00178% for nicosulfuron and 0.37226–1.31697% for atrazine. As for the risk between two different regions, rural residents have higher dietary risk than urban residents, which could be attributed to the low weight and high consumption of rural people as well as the different dietary culture in two regions (Cui et al., 2021; Zhang et al., 2023). For the risk of different ages, the highest exposure risk of three herbicides was found at rural male (age 2–3 years old) on fully-grown maize. Similarly, the largest risk on green maize was found at the same group but aged from 4 to 6 years old, which could be attributed to the lighter body weight of children.
To further ensure the safe use of these herbicides, the total chronic dietary risk needs to be discussed. According to New Zealand Ministry for Primary Industries (2023), MRLs of the three herbicides set by different regions were summarized in Supplementary Table S10. Since mesotrione and nicosulfuron were only registered on maize in China, the risk of atrazine was further discussed. Considering the MRLs of atrazine on ginger (0.1 mg/kg), grape (0.05 mg/kg), sugarcane (0.05 mg/kg) and tea (0.1 mg/kg), the total chronic risk was from 0.76 to 3.76% for urban male, 0.87 to 4.03% for urban female, 0.82 to 4.11% for rural male and 0.92 to 4.39% for rural female (Figure 4). And the order of the total risk from high to low was as follows: Rural Female>Rural Male>Urban Female>Urban Male. It may be caused by the differences of the intake of fruits, sugar and other foods (including spring onion, ginger, pear, apple, tea etc.). With the increasing of ages, although different groups have similar dietary patterns in both urban and rural regions, the total dietary risk gradually decreased, which may be explained by the differences of the body weight.
In summary, all RQ values were less than 100% representing the acceptable risk of mesotrione, nicosulfuron and atrazine for Chinese. After the application of mixed herbicides, however, the residues can be transferred to different sites of the ecological system like soil and underground water, which can amplify the exposure risk of human via the food chain (Li et al., 2021). Thus, more attention needs to be paid on the effects of these chemicals on the whole agricultural ecosystem to keep its sustainable development. In addition, rural female from 2 to 6 years old had the highest dietary amount per unit body weight. Therefore, the dietary constructures of relevant groups are recommended to be changed so that the risk of exposure to the chemicals should be reduced.
4. Conclusion
In this study, we developed reliable and rapid analytical methods for the accurate determination of mesotrione, nicosulfuron, atrazine and their four metabolites in maize. Results from field experiments showed that residues of these three herbicides in fully-grown and green maize were below 0.01 mg/kg for mesotrione, 0.005 mg/kg for nicosulfuron, and 0.037 mg/kg for accumulative atrazine. The residues of mesotrione were less than 0.01 mg/kg in straws and green straws. As for other two herbicides, the nicosulfuron and accumulative atrazine residues were ranged from <0.005–0.010 mg/kg and <0.037–0.254 mg/kg in straw samples, respectively. In green straw samples, the residues were in the ranges of <0.005–0.007 mg/kg for nicosulfuron and <0.037–0.211 mg/kg for accumulative atrazine. However, given the wide application of straw in different fields, the residues of green straw and straw samples should receive more attention since they can pose potential hazards to human health through various pathways of the food chain.
Through the RQ model, the dietary risk was evaluated considering different consumption, rural–urban and age differences. All calculated values were less than 100%, indicating that the dietary risks to consumers are acceptable. This study confirms the safety of maize produced under the mixed application of these three herbicides and contributes to a comprehensive evaluation of herbicide use in maize production, highlighting the potential of sustainable maize production in China.
Data availability statement
The original contributions presented in the study are included in the article/Supplementary material, further inquiries can be directed to the corresponding author.
Author contributions
HC: Formal analysis, Investigation, Resources, Writing – original draft. JL: Investigation, Visualization, Writing – original draft. YW: Investigation, Validation, Writing – review & editing. YZ: Validation, Writing – original draft. ZD: Methodology, Validation, Writing – review & editing. TD: Writing – review & editing, Conceptualization, Methodology, Resources.
Funding
The author(s) declare financial support was received for the research, authorship, and/or publication of this article. This work was funded by the Special Project of the Central Government Guiding Local Science and Technology of Guizhou Province (Qian[2022]4008) and the Science and Technology Support Plan of Guizhou Province (Qiankehe Support[2022]029–1).
Acknowledgments
We appreciate the help of Ming Li, Hualong Yu, and Shaowen Wang (all from Guizhou Institute of Plant Protection) in conducting field experiments and indoor sample detection. We appreciate the help of Hang He (from Nanjing University of Aeronautics and Astronautics) in preparing the graphical abstract. And we thank Weitao Wang, affiliated with Hong Kong University of Science and Technology (Guangzhou), for help with language and formatting.
Conflict of interest
HC and ZD were employed by the company Guizhou Jinnong Technology Co., Ltd.
The remaining authors declare that the research was conducted in the absence of any commercial or financial relationships that could be construed as a potential conflict of interest.
Publisher’s note
All claims expressed in this article are solely those of the authors and do not necessarily represent those of their affiliated organizations, or those of the publisher, the editors and the reviewers. Any product that may be evaluated in this article, or claim that may be made by its manufacturer, is not guaranteed or endorsed by the publisher.
Supplementary material
The Supplementary material for this article can be found online at: https://www.frontiersin.org/articles/10.3389/fsufs.2023.1263879/full#supplementary-material
References
Ahmadi, A. R., Shahbazi, S., and Diyanat, M. (2017). Analysis of nicosulfuron residues in maize field soil by high-performance liquid chromatography. Qual. Assur. Saf. Crops Foods. 9, 229–235. doi: 10.3920/QAS2015.0741
Alferness, P., and Wiebe, L. (2002). Determination of mesotrione residues and metabolites in crops, soil, and water by liquid chromatography with fluorescence detection. J. Agric. Food Chem. 50, 3926–3934. doi: 10.1021/jf011696y
Cui, K., Wu, X., Wei, D., Zhang, Y., Cao, J.-L., Xu, J., et al. (2021). Health risks to dietary neonicotinoids are low for Chinese residents based on an analysis of 13 daily-consumed foods. Environ. Int. 149, –106385. doi: 10.1016/j.envint.2021.106385
Cunha, S. C., and Fernandes, J. O. (2011). Multipesticide residue analysis in maize combining acetonitrile-based extraction with dispersive liquid-liquid microextraction followed by gas chromatography-mass spectrometry. J. Chromatogr. A 1218, 7748–7757. doi: 10.1016/j.chroma.2011.08.066
Daniel, D., and Lucio, L. (2019). Determination of multiclass pesticides residues in corn by QuEChERS and capillary electrophoresis tandem mass spectrometry. Food Anal. Methods 12, 1684–1692. doi: 10.1007/s12161-019-01501-y
Dehghani, M., Gharehchahi, E., Jafari, S., Moeini, Z., Derakhshan, Z., Ferrante, M., et al. (2022). Health risk assessment of exposure to atrazine in the soil of shiraz farmlands, Iran. Environ. Res. 204:112090. doi: 10.1016/j.envres.2021.112155
Delcour, I., Spanoghe, P., and Uyttendaele, M. (2015). Literature review: impact of climate change on pesticide use. Food Res. Int. 68, 7–15. doi: 10.1016/j.foodres.2014.09.030
Dobbels, A. F., and Kapusta, G. (1993). Postemergence weed control in corn (Zea Mays) with Nicosulfuron combinations. Weed Technol. 7, 844–850. doi: 10.1017/S0890037X00037866
Du, P., Wu, X., Xu, J., Dong, F., Liu, X., Wei, D., et al. (2017). Determination and dissipation of mesotrione and its metabolites in Rice using UPLC and triple-quadrupole tandem mass spectrometry. Food Chem. 229, 260–267. doi: 10.1016/j.foodchem.2017.02.079
European Commission. (2021). Guidance document on analytical quality control and method validation for pesticide residues analysis in food and feed Sante. Available at: https://food.ec.europa.eu/system/files/2022-02/pesticides_mrl_guidelines_wrkdoc_2021-11312.pdf.
Fu, H., Tan, P., Wang, R., Li, S., Liu, H., Yang, Y., et al. (2022). Advances in organophosphorus pesticides pollution: current status and challenges in Ecotoxicological, sustainable agriculture, and degradation strategies. J. Hazard. Mater. 424:127494. doi: 10.1016/j.jhazmat.2021.127494
He, Q., Chun, K. P., Dieppois, B., Chen, L., Fan, P. Y., Toker, E., et al. (2022). Investigating and predicting spatiotemporal variations in vegetation cover in transitional climate zone: a case study of Gansu (China). Theor. Appl. Climatol. 150, 283–307. doi: 10.1007/s00704-022-04140-2
Inamuddin Ahamed, M. I., and Lichtfouse, E. (2020). Sustainable agriculture reviews, Vol. 48. New York, USA: Springer Nature, 311–336.
Interino, N., Comito, R., Simoni, P., Franzellitti, S., Palladino, G., Rampelli, S., et al. (2023). Extraction method for the multiresidue analysis of legacy and emerging pollutants in marine mussels from the Adriatic Sea. Food Chem. 425:136453. doi: 10.1016/j.foodchem.2023.136453
Jabłońska-Trypuć, A., Wydro, U., Wołejko, E., Rodziewicz, J., and Butarewicz, A. (2020). Possible protective effects of TA on the cancerous effect of mesotrione. Nutrients 12:1343. doi: 10.3390/nu12051343
Jin, S. (2008). The tenth report of nutrition and health status for China residents: nutrition and health status of annual 2002. Beijing, China: People’s Medical Publishing House.
Jowa, L., and Howd, R. (2011). Should atrazine and related Chlorotriazines be considered carcinogenic for human health risk assessment? J. Environ. Sci. Health C 29, 91–144. doi: 10.1080/10590501.2011.577681
Kapusta, G., Krausz, R. F., Khan, M., and Matthews, J. L. (1994). Effect of nicosulfuron rate, adjuvant, and weed size on annual weed control in corn (Zea Mays). Weed Technol. 8, 696–702. doi: 10.1017/S0890037X00028542
Lan, T., Yang, G., Li, J., Chi, D., and Zhang, K. (2022). Residue, dissipation and dietary intake risk assessment of Tolfenpyrad in four leafy green vegetables under greenhouse conditions. Food Chem. 13:100241. doi: 10.1016/j.fochx.2022.100241
Li, C., Zhu, H., Li, C., Qian, H., Yao, W., and Guo, Y. (2021). The present situation of pesticide residues in China and their removal and transformation during food processing. Food Chem. 354:129552. doi: 10.1016/j.foodchem.2021.129552
Liu, C., Dou, X., Zhang, L., Li, Q., Qin, J., Duan, Y.-P., et al. (2018). Determination of triazine herbicides and their metabolites in multiple medicinal parts of traditional Chinese medicines using streamlined pretreatment and UFLC-ESI-MS/MS. Chemosphere 190, 103–113. doi: 10.1016/j.chemosphere.2017.09.085
Liu, W., Liu, Y., Liu, G., Xie, R., Ming, B., Yang, Y., et al. (2022). Estimation of maize straw production and appropriate straw return rate in China. Agric. Ecosyst. Environ. 328:107865. doi: 10.1016/j.agee.2022.107865
Liu, J., Zhou, J. H., Guo, Q. N., Ma, L. Y., and Yang, H. (2021). Physiochemical assessment of environmental behaviors of herbicide atrazine in soils associated with its degradation and bioavailability to weeds. Chemosphere 262:127830. doi: 10.1016/j.chemosphere.2020.127830
MacLennan, P., Delzell, E., Sathiakumar, N., and Myers, S. (2003). Mortality among Triazine herbicide manufacturing workers. J. Toxicol. Environ. Health A 66, 501–517. doi: 10.1080/15287390306356
Martín-Pozo, L., Arena, K., Cacciola, F., Dugo, P., and Mondello, L. (2023). Development and validation of a multi-class analysis of pesticides in corn products by comprehensive two-dimensional liquid chromatography-tandem mass spectrometry. J. Chromatogr. A 1701:464064. doi: 10.1016/j.chroma.2023.464064
Mattoo, A. K., Cavigelli, M. A., Mišić, D. M., Gašić, U., Maksimović, V. M., Kramer, M., et al. (2023). Maize metabolomics in relation to cropping system and growing year. Front. Sustain. Food Syst. 7:1130089. doi: 10.3389/fsufs.2023.1130089
New Zealand Ministry for Primary Industries. (2023). Pesticide maximum residue level legislation around the world. Available at: [https://www.mpi.govt.nz/agriculture/plant-products-requirements-and-pesticide-levels/pesticide-maximum-resi-due-levels-mrls-for-plant-based-food-for-nz-and-other-countries/pesticide-maximum-residue-level-legislation-around-the-world/].
Ministry of Agriculture and Rural Affairs of the People’s Republic of China. (2018). NY/T 788–2018 guideline for the testing of pesticide residues in crops. Beijing, China: China Agricultural Press.
Mitchell, G., Bartlett, D. W., Fraser, T. E. M., Hawkes, T. R., Holt, D. C., Townson, J. K., et al. (2001). Mesotrione: a new selective herbicide for use in maize. Pest Manage Sci. 57, 120–128. doi: 10.1002/1526-4998(200102)57:2<120::AID-PS254>3.0.CO;2-E
Morrison, H. I., Wilkins, K., Semenciw, R., Mao, Y., and Wigle, D. (1992). Herbicides and Cancer. J. Natl. Cancer Inst. 84, 1866–1874. doi: 10.1093/jnci/84.24.1866
National Bureau of Statistics of China. (2022). Bulletin on the National Grain Output in 2022. Available at: http://www.stats.gov.cn/english/PressRelease/202212/t20221215_1891098.html (Accessed June 7, 2023).
National Development and Reform Commission of the People’s Republic of China. (2021). On the “14th five-year plan” guidance on the comprehensive utilization of bulk solid waste. Available at: https://www.gov.cn/zhengce/zhengceku/2021-06/03/content_5615185.html (Accessed June 1, 2023).
National Development and Reform Commission of the People’s Republic of China and Ministry of Agriculture of the People’s Republic of China. (2016). On the “13th five-year plan” guidance on the comprehensive utilization of crop straw (in Chinese). Available at: https://www.ndrc.gov.cn/xxgk/zcfb/tz/201612/t20161207_962800.html (Accessed June 7, 2023).
Oerke, E.-C. (2006). Crop losses to pests. J. Agric. Sci. 144, 31–43. doi: 10.1017/S0021859605005708
Olayinka, E. T., Kehinde, S. A., and Olajide, A. T. (2022). Protective effect of Hesperetin on Nicosulfuron-induced testicular oxidative stress in male Wistar rats. J. Appl. Sci. Environ. Manag. 26, 1451–1456. doi: 10.4314/jasem.v26i8.19
Pang, N., Wang, T., and Hu, J. (2016). Method validation and dissipation kinetics of four herbicides in maize and soil using QuEChERS sample preparation and liquid chromatography tandem mass spectrometry. Food Chem. 190, 793–800. doi: 10.1016/j.foodchem.2015.05.081
Peng, J., Gan, J., Liu, T., Ju, X., Chen, J., and He, L. (2023). Development and application of a rapid screening and quantification method for multi-class herbicide residues in fishery products using UPLC-Q-Tof-MS/MS: evidence for Prometryn residues in shellfish. Food Control 148:109672. doi: 10.1016/j.foodcont.2023.109672
People’s Government of Yunnan Province. (2022). Yunnan Province "14th five-year plan" comprehensive utilization of crop straw implementation plan. Available at: https://nync.yn.gov.cn/uploadfile/s38/2022/1011/20221011044730733.pdf (Accessed June 5, 2023).
Ranum, P., Peña-Rosas, J. P., and Garcia-Casal, M. N. (2014). Global maize production, utilization, and consumption. Ann. N. Y. Acad. Sci. 1312, 105–112. doi: 10.1111/nyas.12396
Rinsky, J. L., Hopenhayn, C., Golla, V., Browning, S., and Bush, H. M. (2012). Atrazine exposure in public drinking water and preterm birth. Public Health Rep. 127, 72–80. doi: 10.1177/003335491212700108
Seleiman, M. F., and Hafez, E. M. (2021). “Optimizing inputs Management for Sustainable Agricultural Development” in Mitigating environmental stresses for agricultural sustainability in Egypt. eds. H. Awaad, M. Abu-hashim, and A. Negm (Cham: Springer Water. Springer) doi: 10.1007/978-3-030-64323-2_18
Siedt, M., Schäffer, A., Smith, K. E. C., Nabel, M., Roß-Nickoll, M., and van Dongen, J. T. (2021). Comparing straw, compost, and biochar regarding their suitability as agricultural soil amendments to affect soil structure, nutrient leaching, microbial communities, and the fate of pesticides. Sci. Total Environ. 751:141607. doi: 10.1016/j.scitotenv.2020.141607
Skrzypczak, G., Sobiech, Ł., and Waniorek, W. (2011). Evaluation of the efficacy of mesotrione plus nicosulfuron with additives as tank mixtures used for weed control in maize (Zea Mays L.). J. Plant Protect. Res. 51, 300–305. doi: 10.2478/v10045-011-0049-1
Sun, Y., Xu, Y., Sun, Y., Qin, X., and Wang, Q. (2013). Dissipation and dynamics of Mesotrione in maize and soil under field ecosystem. Bull. Environ. Contam. Toxicol. 90, 242–247. doi: 10.1007/s00128-012-0907-1
Tandon, S., and Singh, A. (2015). Field dissipation kinetics of atrazine in soil and post-harvest residues in winter maize crop under subtropical conditions. Chem. Ecol. 31, 273–284. doi: 10.1080/02757540.2014.950567
The Joint FAO/WHO Meeting on Pesticide Residues. (2007). JMPR Report Atrazine, No.7. Available at: https://www.fao.org/fileadmin/templates/agphome/documents/Pests_Pesticides/JMPR/Report07/Atrazine.pdf (Accessed June 5, 2023).
The Joint FAO/WHO Meeting on Pesticide Residues. (2014a). JMPR report Mesotrione, no.277. Available at: https://www.fao.org/fileadmin/templates/agphome/documents/Pests_Pesticides/JMPR/Report2014/5.19_MESOTRIONE__277_.pdf (Accessed June 5, 2023)
The Joint FAO/WHO Meeting on Pesticide Residues. (2014b). JMPR Specification Nicosulfuron. Available at: https://www.fao.org/fileadmin/templates/agphome/documents/Pests_Pesticides/Specs/Nicosulfuron2014.pdf (Accessed June 5, 2023).
Tong, Z., Duan, J., Wu, Y., Liu, Q., He, Q., Shi, Y., et al. (2018). A survey of multiple pesticide residues in pollen and beebread collected in China. Sci. Total Environ. 640-641, 1578–1586. doi: 10.1016/j.scitotenv.2018.04.424
US EPA. (2018) Atrazine: Triazine cumulative human health risk assessment. United States Environmental Protection, Jul. 10, 2018. Available at: https://www.regulations.gov/document/EPA-HQ-OPP-2013-0266-1159 (Accessed June 7, 2023).
Wang, W., Chen, H., Gao, D., Long, J., Long, H., and Zhang, Y. (2022). Dissipation and dietary risk assessment of thiacloprid and tolfenpyrad in tea in China. Agronomy 12:3166. doi: 10.3390/agronomy12123166
Wang, W., Huang, W., Mao, J., Zhang, X., Wang, H., Kaium, A., et al. (2023). Dissipation and dietary risk assessment of cyflumetofen, bifenazate and their metabolites in citrus in China. Cogent Food Agric. 9:2157091. doi: 10.1080/23311932.2022.2157091
Wang, D., Sun, C., Cui, M., Shen, X., Zhang, Y., Xiao, J., et al. (2022). An integrated analysis of transcriptome and metabolome provides insights into the responses of maize (Zea mays L.) roots to different straw and fertilizer conditions. Environ. Exp. Bot. 194:104732. doi: 10.1016/j.envexpbot.2021.104732
Wu, X., Zhong, H., Ma, C., and Zhang, J. (2012). Residues and dissipation dynamics of nicosulfuron in corn and soil. J. Henan Agric. Sci. 41, 87–91. doi: 10.15933/j.cnki.1004-3268.2012.01.015
Zhang, B., Pan, X., Yang, Y., Dong, F., Xu, J., Wu, X., et al. (2023). Dissipation dynamics and comparative dietary exposure assessment of mefentrifluconazole in Rice. Ecotoxicol. Environ. Saf. 250:114482. doi: 10.1016/j.ecoenv.2022.114482
Zhao, P., and He, Z. (2022). Temperature change characteristics in Gansu Province of China. Atmos. 13:728. doi: 10.3390/atmos13050728
Zhao, N., Zuo, L., Li, W., Guo, W., Liu, W., and Wang, J. (2017). Greenhouse and field evaluation of Isoxaflutole for weed control in maize in China. Sci. Rep. 7:12690. doi: 10.1038/s41598-017-12696-7
Keywords: maize, mesotrione, nicosulfuron, atrazine, residue, dietary risk assessment
Citation: Chen H, Li J, Wang Y, Zhou Y, Duan Z and Duan T (2023) Residue determination and dietary risk assessment of mesotrione, nicosulfuron, atrazine and its four metabolites in maize in China. Front. Sustain. Food Syst. 7:1263879. doi: 10.3389/fsufs.2023.1263879
Edited by:
Md Meftaul Islam, The University of Newcastle, AustraliaReviewed by:
Jinfang Nie, Guilin University of Technology, ChinaMohammad Dalower Hossain Prodhan, Bangladesh Agricultural Research Institute, Bangladesh
Md Al Amin, The University of Newcastle, Australia
Copyright © 2023 Chen, Li, Wang, Zhou, Duan and Duan. This is an open-access article distributed under the terms of the Creative Commons Attribution License (CC BY). The use, distribution or reproduction in other forums is permitted, provided the original author(s) and the copyright owner(s) are credited and that the original publication in this journal is cited, in accordance with accepted academic practice. No use, distribution or reproduction is permitted which does not comply with these terms.
*Correspondence: Tingting Duan, gzdtt@126.com