- Department of Biotechnology and Food Science, Norwegian University of Science and Technology, Trondheim, Norway
This study’s main aim was to utilize green extraction protocols to recover collagen from by-products originating from European plaice. Moreover, the objective was to evaluate pre-treatments, the composition of the up-cycled product as well as to identify the most promising by-product fraction. Microwave (MAE) and ultrasound-assisted extractions (UAE) were performed on untreated, pre-treated (salt-washed or enzymatic hydrolysis) fractions of backbones, skins, and heads. Both MAE and UAE were performed for 15 and 35 min. After pre-treatment and extraction, the quality and yield of products were evaluated. Protein and collagen concentration, as well as amino acid profiles, were measured. Skins deliver significantly higher yields of collagen and protein than heads and backbones (p < 0.05). Enzymatic hydrolysis resulted in the highest collagen yields for skins (77%), while salt-washing gave the highest results for backbones (43%) and heads (41%) regardless of extraction method and time. Total and free amino acid profiles differed between the three fractions, with backbones and heads showing overall more similarity in composition compared to skins. The study showed that MAE and UAE technologies are suitable for generating collagen from marine by-products. Additional research is recommended to optimize pre-treatment and extraction for skin, as most promising collagen supplier.
1. Introduction
Global fish production reached a substantial 176 million tonnes in 2020, of which 157 million tonnes were used for direct human consumption and an increasing share of fish getting further processed and not sold as whole fish (FAO, 2022). This leads to a high amount of by-products, which can reach up to 70% of the total mass of the fish. By-products refer to the portions of the catch that are not considered the main saleable product, including skins, scales, heads, viscera, backbones, and fins (Ozogul et al., 2021). Europe has increased its by-product utilization significantly in the past year, however, the main share is used for low-value applications like fishmeal, fish oil, biogas or fertilizers (FAO, 2020). However, over the past few decades, extensive research has revealed that these by-product fractions contain valuable components that can be further up-cycled into products for human consumption (Al Khawli et al., 2019; Ozogul et al., 2021). Especially collagen has versatile fields of applications, such as a functional food ingredient or stabilizer in food, as biomaterial to regenerate skin and bones as well as applications in cosmetics as skincare products, proven to exhibit bioactive properties (Avila Rodríguez et al., 2018; Lu et al., 2023). Moreover, collagen, in its application as a novel functional food ingredient, contains valuable essential amino acids which positively impact the nutritional quality of the product and moreover exhibit bioactive properties suitable for application as natural antioxidants (Pal and Suresh, 2016).
The demand for a more sustainable global food production system prompted the European Union (EU) Commission to launch the Circular Economy Action Plan in 2020, building upon strategies outlined in the European Green Deal of 2015. This plan aims to establish a framework that promotes the norm of sustainable products and contributes to achieving the 2030 Sustainable Development Goals (Commission and Communication, 2020). Especially novel technologies, such as green extraction procedures for obtaining bioactive components from marine by-products have attracted considerable interest in recent years, due to their reduced impact on the environment and decreased economic waste (Ozogul et al., 2021) Pal and Suresh (2016) list ultrasonic (ultrasound), microwave, super critical fluids and high pressure as emerging green extraction methods for marine collagen, but also states that these extractions require heating which potentially affect the structure of marine collagen. Previously, ultrasound-assisted extraction has been used to extract collagen from marine by-products (Ali et al., 2018; Shaik et al., 2021; Lu et al., 2023). Moreover, chitin and chitosan from shrimp shells have formerly been generated by microwave-assisted extraction (El Knidri et al., 2019; Santos et al., 2019). Ozogul et al. (2021) state that the extraction of marine collagen is most efficiently performed with organic acids or with enzymatic hydrolysis. However, to our knowledge, the research and knowledge on the utilization of ultrasound or microwave-assisted extraction on generating collagen from fish by-products has not been followed-up to date.
Thus, the present study aimed to investigate the utilization of green extraction technologies for valorizing three by-product fractions derived from European plaice (Pleuronectes platessa). Specifically, the focus was on extracting collagen from backbones, skins and heads using microwave or ultrasound-assisted extraction. Next to collagen, the total protein content and amino acid profiles were investigated. Additionally, the study examined the effects of enzymatic hydrolysis or salt-washing prior to extraction as suitable pre-treatments. By exploring these objectives, the study aimed to provide insights into optimal green extraction procedures and conditions for obtaining collagen from different by-product fractions of European plaice.
2. Materials and methods
2.1. Raw material and experimental design
Skins, heads and backbones from European plaice (Pleuronectes platessa), previously collected during the study of Kendler et al. (2023b), were separated, homogenized and kept frozen at −80°C until further analyses. Samples underwent pre-treatment by salt-washing or enzymatic hydrolysis or were used untreated for further extractions by microwave or ultrasound technologies, each for 15 and 35 min (MAE15, MAE35, UAE15, and UAE35). After extraction, samples got lyophilized for 24 h at −50°C and 13.3 Pa and frozen prior to further analyses.
2.2. Pre-treatments
2.2.1. Salt pre-treatment
The salt pre-treatment followed the method of Kołodziejska et al. (2008) with minor modifications. Minced raw material was thawed at 4°C. A 0.45 M NaCl-solution (6:1 v/w) was added and shaken with the material for 10 min at 4°C. The solution was filtered, and the salt-wash was discarded. Steps one and two were repeated two more times. The material was then washed in cold tap water. Next, the material was shaken in a 10% ethanol solution (6:1 v/w) for 30 min at 4°C, filtered again, and subsequently washed in cold tap water. The salt pre-treated material was stored at 4°C until extraction with microwave or ultrasound.
2.2.2. Enzymatic hydrolysis
Enzymatic hydrolysis was performed by following an in-house protocol, as described by Hjellnes et al. (2021, p. 26), using the peptidase Alcalase 2.4 L (provided by Novozymes AS, Denmark) as the catalyst. The hydrolysis was carried out in a laboratory-scale bioreactor (Model No. 2101000; Syrris Atlas) equipped with a stirring unit, thermostat, and pH meter.
Pre-heated water (50°C) and raw material (1:1% ww) were added and stirred in the reactor. Once the mixture reached 50°C, a portion was taken out as a 0-sample (prior to enzyme addition) and deactivated in a 90°C water bath for 5 min with stirring. Alcalase 2.4 L was then added to the remaining sample (0.1% of weight), and the stirring was initiated. Samples were taken out at 60 min and deactivated as described earlier. The pH was maintained at 7.0 throughout the hydrolysis, with NaOH added if necessary. After 60 min, the hydrolysis was stopped.
All samples were centrifuged and frozen overnight at −40°C. The following day, the frozen samples were divided into three fractions: fat, protein hydrolysate, and sediment. The weight of each fraction was recorded for yield calculation, and the sediment and protein hydrolysate fractions were further analyzed. To determine which fraction contained more collagen for subsequent extractions, both fractions were lyophilized and assessed for collagen content.
2.3. Green extraction technologies
Both untreated and pre-treated raw material was subjected to microwave-assisted extraction (MAE) and ultrasound-assisted extraction (UAE).
2.3.1. Microwave-assisted extraction
Microwave-assisted extraction (MAE) was conducted with a MARS 6 microwave digestion system (CEM Corporation, NC), equipped with 30 digestion vessels. About 30–40 g (ww) raw material was homogenized (Polytron PT3100; Kinematica) at 10000 rpm for 1 min and subsequently mixed with deionized water (6:1 v/w). For hydrolysates, 2–3 g lyophilized samples were used and deionized water was added (6:1 v/w according to wet weight of hydrolysates). The water-sample mixture was shaken vigorously to mix water and hydrolysate. Finally, the material was added to the digestion vessels and following parameters were chosen for each extraction round: ramp time (5 min), hold time (15 or 35 min), temperature 40°C, power (350 W), stirring (medium speed).
2.3.2. Ultrasound-assisted extraction
For the Ultrasound-assisted extraction (UAE), an ultrasonic cleaning bath, of type USC-T (VWR) was used. The material was prepared, and same sample amount used as described in section 2.31. Basic 50 mL centrifuge tubes were used for extraction. The ultrasound water bath was pre-heated to 40°C and the centrifuge tubes were placed in the water bath when the temperature reached 35°C to obtain a ramp time of 5 min, like MAE.
After water, MAE or UAE extraction, the samples were centrifuged (34,000 g, 10 min, 20°C) and the two obtained phases, being the sediment and soluble protein phase were separated and weighted. The soluble protein phase was then lyophilized for further analyses.
2.4. Chemical analyses
2.4.1. Total protein and amino acid profile
The determination of total crude protein (%) was carried out using the Kjeldahl method (AOAC, 1990), using a Kjeldahl apparatus equipped with a digestion and auto titration unit (K-449 and K-375, Büchi Labortechnik, Schwitzerland). The procedure was carried out as previously described in Kendler et al. (2023a,b). A conversion factor of 6.25 x nitrogen (%) was applied to determine the protein content of samples (NMKL, 2003).
The determination of the total amino acid (TAA) distribution (%) followed the method of Blackburn (1978) utilizing acid hydrolysis. The procedure was previously described in detail by, Kendler et al. (2023a,b).
The method from Osnes and Mohr (1985) was followed to determine the free amino acid (FAA) distribution (%). The sample preparation was previously described in Kendler et al. (2023a,b).
The HPLC analysis for TAA and FAA distributions (%) was conducted by a ultra-high-performance liquid chromatography system (HPLC, UltiMate 300, Thermo Fisher Scientific, United States). The same HPLC system setup as previously described in detail by Kendler et al. (2023a,b) was used.
2.5. Collagen content
Collagen is a protein composed of repetitive triple helixes with proline and hydroxyprline as composites Zanaboni et al. (2000). Which is why the determination of hydroxyproline in section 2.5.1 gives information of the total collagen content.
2.5.1. Hydroxyproline
The determination of hydroxyproline content is based on the method of Leach (1960). An L-hydroxyproline stock solution was used (0.05 g hydroxyproline in 400 mL distilled water, 20 mL concentrated HCl, adding distilled water until volume reached 500 mL). From the stock solution, four standards of 2.5, 5.0, 10.0 and 15.0 μg hydroxyproline/mL were prepared.
The absorbance of the standard and sample solutions were measured against a blank using a GENESYS 10S UV–VIS Spectrophotometer (Thermo Fisher Scientific Inc., Unite States) at a wavelength of 555 nm.
A standard curve based on the absorbance of the standards was used to calculate the concentration of hydroxyproline in samples. The collagen content was then determined by using the equations 1–3 with the species specific collagen conversion factor of 9.6 for flounder (Sikorski et al., 1984).
Where:
OD, Optical density (absorbance from spectrophotometer); DF, Dilution factor; a, A-value from standard curve (y = ax + b) (y = absorbance, x = hyp-content); b, B-value from standard curve; V, mL sample after acid hydrolysis; m, Mass of lyophilized sample for hydrolysis; CF, Conversion factor Statistics.
2.6. Statistics
All analyses were performed in triplicates, if not other stated (n = 3). The data from the analyses was tested by a General Linear Model (GLM) using the software IBM SPSS (release 28, IBM Corporation, United States). Where applicable, analysis of variance (ANOVA) was carried out, and when significance detected in the GLM or ANOVA, a Tukey HSD post hoc test was carried out to investigate the differences between groups. The α-level was set to 0.05.
3. Results and discussion
3.1. Pre-assessment of raw material quality and fractions
Analyses of the three raw material fractions prior to pre-treatment and extraction showed significant differences in their initial proximate composition. The skins protein content of 17.37 ± 1.4 g/100 g ww (wet weight) is significantly higher (p < 0.001) compared to backbones (11.88 ± 0.4 g/100 g ww) and heads (12.57 ± 0.8 g/100 g ww). Additionally, the skins ash content (1.31 ± 0.03 g/100 g ww) is significantly lower (p < 0.001) than the content of the backbones (5.44 ± 1.06 g/100 g ww) and heads (5.41 ± 1.5 g/100 g ww), which can be explained by the higher content of inorganic matter in backbones and heads. No significant differences in lipid and moisture content were observed. Moreover, the initial collagen content of skins (32.54 ± 0.4 g/100 g dw; dry weight) is significantly higher (p < 0.001) compared to backbones (18.71 ± 1.1 dw) and heads (12.73 ± 0.2 g/100 g dw) which is of special relevance for the extraction of collagen.
Analyses on the collagen content of sediment and protein hydrolysates generated through enzymatic hydrolysis showed that skin protein hydrolysates contain collagen of 52.07 ± 13.0% dw followed by heads protein hydrolysates with 34.63 ± 12.1% dw. Moreover, the collagen content in the sediment fractions of skins (28.3 ± 3.0% dw) and heads (13.2 ± 4.8%) were significantly lower (p = 0.043) than for their respective protein fraction. The lowest collagen content was found in the sediment fraction of backbones (12.03 ± 1.7% dw). Following these results, merely the protein hydrolysate fractions were considered for further MAE and UAE, to guarantee the highest possible yield.
3.2. Total protein and collagen content after extraction
Analyzing the main effects of raw material, pre-treatments and extraction methods and times, it was found that regardless of pre-treatment, extraction and time, skins have the significantly (p < 0.001) highest collagen (%) and highest protein (%) concentrations of all three fractions. Moreover, pre-treatment could significantly increase collagen content (p < 0.001). In addition, the GLM demonstrated that both MAE and UAE, including both times, lead to a significant (p < 0.001) increase in the collagen (%) and protein content (%) compared to untreated raw material and without extraction.
Figure 1 shows the collagen and protein concentrations depending on pre-treatment (a, c) as well as after extractions with microwave or ultrasound for two different times (b, d). It is visible (Figure 1A) that skin yields the significantly highest collagen, regardless of pre-treatment (p < 0.05). Moreover, salt-washing is most effective in generating collagen for heads and backbones, whereas enzymatic hydrolysis yielded the most collagen for skins regardless of extraction method and time (p < 0.05). When looking at the different pre-treatments (Figure 1C) and their effect on the yield of protein of each raw material, it is visible that skins show significantly highest protein concentration for each pre-treatment. Thus, skins being the fraction with highest collagen and total protein yield regardless of pre-treatment. For both collagen and protein yield of skins, enzymatic hydrolysis was the most effective method. In addition, Figure 1B highlights that skins, regardless of extraction method and time, produce the highest collagen content (p < 0.05). Among the different extraction methods applied to backbones, MAE 35 has a significantly (p < 0.05) lower collagen content (27.96 ± 5.6%) compared to MAE 15, UAE 15, and UAE 35. No significant differences were observed among MAE 15, UAE 15, and UAE 35 for backbone as raw material. A different trend was observed for skins, where MAE 35 led to significantly higher collagen (75.58 ± 4.2%) compared to the lowest concentrations observed for UAE 15. Generally, the same trend for a suitable pre-treatment or extraction method and time was observed for backbones and heads, with enzymatic hydrolysis and UAE 35 yielding the highest protein concentrations for both fractions.
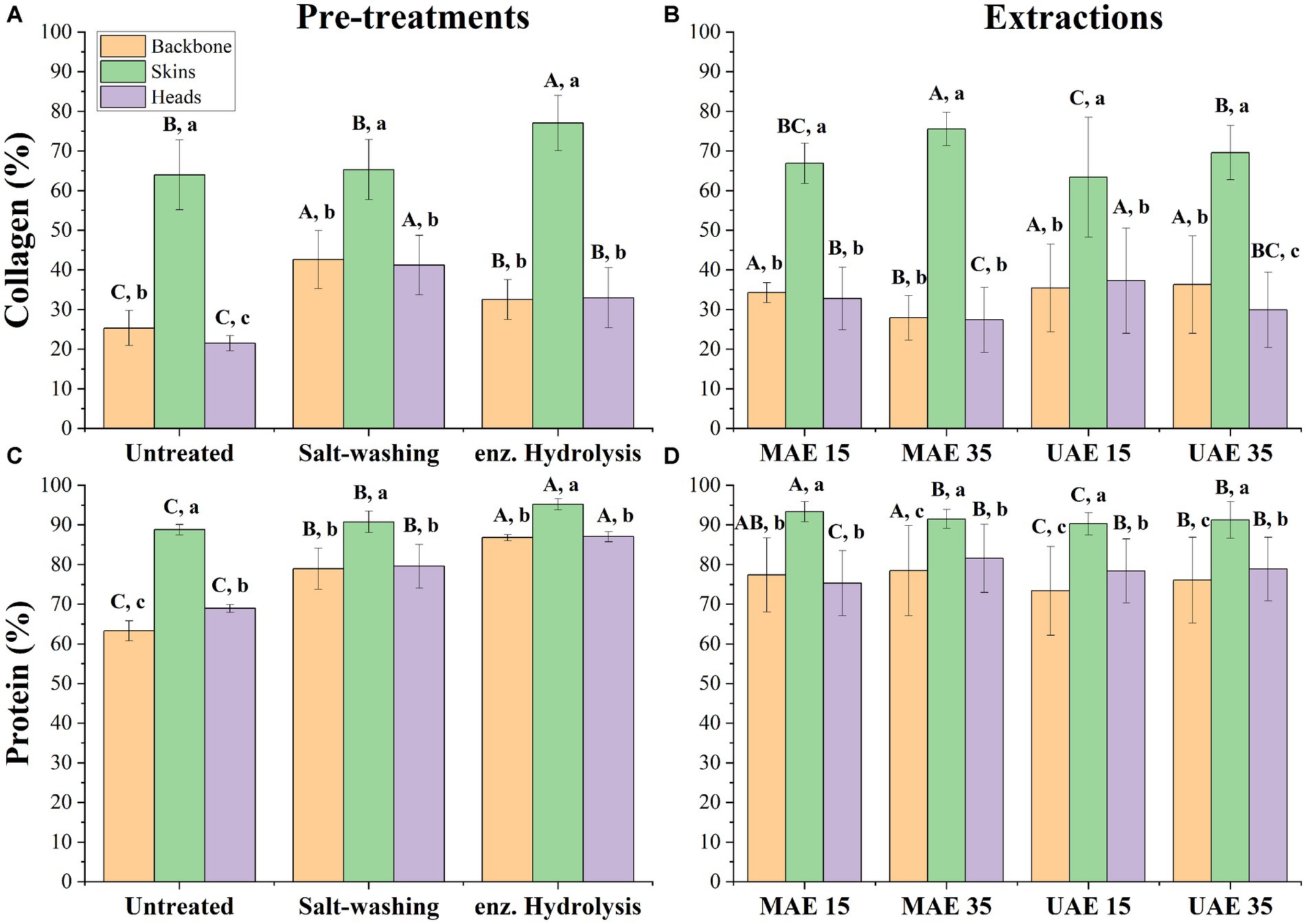
Figure 1. Overview of collagen and protein content (%) for backbones, skins and heads depending on pre-treatment in (A,C) and depending on extraction method in (B,D); Error bars show SD. ANOVA was applied on raw materials for pre-treatments and extractions; where a significant difference between raw materials was detected (α < 0.05), a Tukey HSD post hoc test was applied. Values with different small letters (A,B) are significantly different (p < 0.05) between raw material for each pre-treatment/ extraction; values with different big letters (A,B) indicate significant differences (p < 0.05) of pre-treatment/extraction on raw material.
3.3. Amino acid profile
Table 1 visualizes the TAA distribution of the three by-product fractions after extraction with MAE15, MAE35, UAE15, and UAE35. Pre-treatment by salt-washing or enzymatic hydrolysis both led to an increase in the TAA content of backbones (p = 0.009) and heads (p < 0.001) compared to no pre-treatment, while pre-treatments showed no difference in the composition for skins (p = 0.418). No between-subjects effects of extraction+time and pre-treatment for any of the three raw materials (p > 0.05) was observed. Moreover, the extraction method and time did not significantly influence the total amino acid contents (p > 0.05). Moreover, backbones and heads show significantly higher (p < 0.05) values for, e.g., histidine, isoleucine, leucine and valine, all essential amino acids, after extraction with MAE15 compared to skins. This pattern can also be observed for MAE35, UAE15, and UAE35. Nevertheless, when looking closer at the sum of all the TAA, a significant difference (p < 0.05) between the three by-products was only observed for UAE35, with skin having a significantly higher TAA content. This leads to the assumption that skins have a significantly different amino acid profile than the two other fractions, but all in all same total content. Skins showed significantly (p < 0.05) higher values in serine for UAE15 and UAE35. Moreover, the alanine concentrations in skins are higher in all four extraction methods compared to backbones and heads. In addition, Table 1 points out that some amino acids are more influenced by extraction method and time than other amino acids, which show no significant different concentrations. Moreover, arginine/glycine were identified as the highest concentrations in all three fractions, which was also observed (for glycine) by Ali et al. (2018); Tamilmozhi et al. (2013). This is reasonable because the strict repeating of Gly-X-Y creates the α-triple-helix of collagen (Zanaboni et al., 2000).
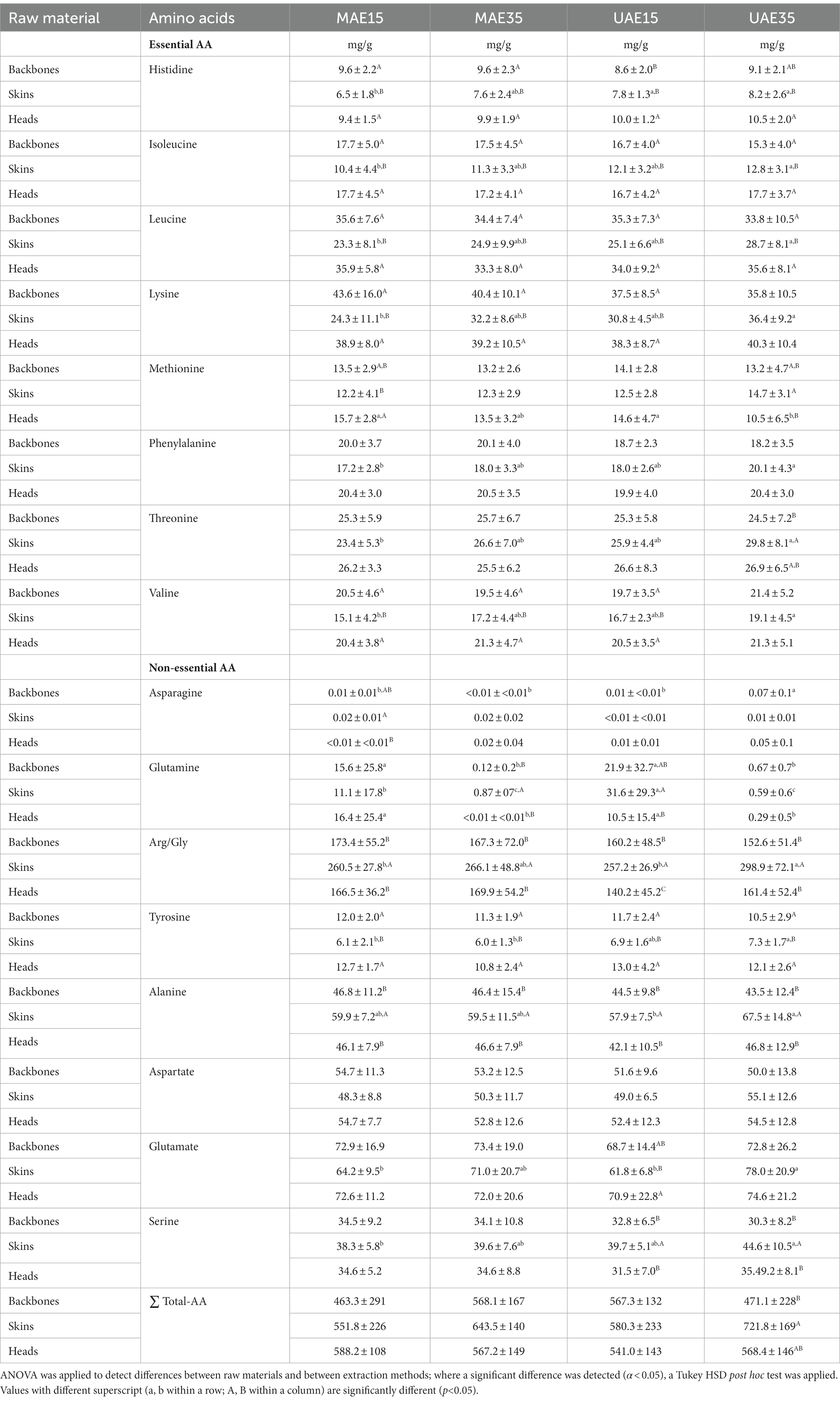
Table 1. Total amino acid composition (mg/g) of backbones, skins and heads depending on extraction methods, regardless of pre-treatment; values presented as mean ± SD.
Table 2 shows the FAA profile of untreated by-products after extraction with MAE15, MAE35, UAE15, and UAE35. Overall, similar FAA profiles between backbones and heads were found, with significantly higher (p < 0.05) values of leucine and glutamine, regardless of extraction method. Moreover, the values of lysine are significantly (p < 0.05) lower in skins when compared to the two other fractions. The total FAA content differs significantly between the three by-products as well as between the four extractions. For backbones, no significant differences between the four extractions were found, whereas UAE15 was identified to deliver most FAA in skins, and UAE15 as well as UAE35 for heads. Similar to TAA, glycine (combined Arg/Gly) was also identified as the main FAA in all three fractions. Higher free glycine can be linked to a disruption of the α-triple-helix and potentially increase the interfacial properties, as previously described in the study of Feng et al. (2021) on microwave extraction of fish skin gelatine.
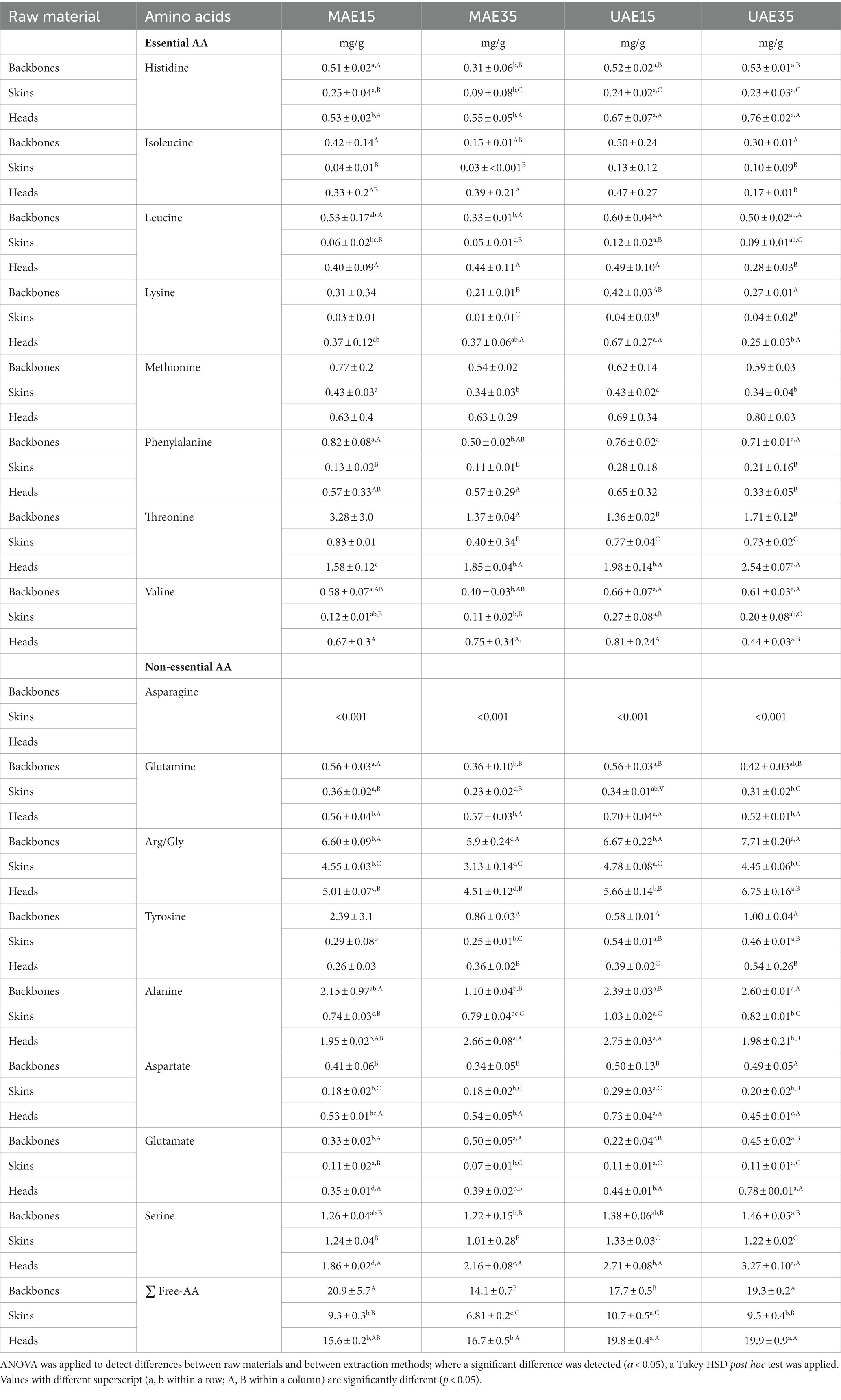
Table 2. Free amino acid composition (mg/g) of backbones, skins and heads depending on extraction methods, excluding effect of pre-treatment; values presented as mean ± SD.
The study highlights the potential value of the three studied fractions for collagen extraction. The results support the findings of Mohamad Razali et al. (2023), stating that green extraction methods such as UAE and MAE increase the extraction efficiency of collagen. Moreover, Mohamad Razali et al. (2023) review the current progress of green extraction methods for collagen and state that MAE is a versatile technology, enabling the transfer of technology from small (lab) to big (industry) scale (Destandau et al., 2013). Furthermore, Mohamad Razali et al. (2023) highlights that UAE and MAE have been successfully applied in multiple studies to increase the extraction efficiency of collagen, hereby stressing the significance of these green extraction methods. The study carried out by Shaik et al. (2021) on UAE of stingray skin collagen found UAE to increase the collagen yield, which agrees with the present study, underlining skin as a high collagen and high protein fraction with great potential for further utilization.
4. Conclusion
The findings of the present study contribute to the development of sustainable extraction processes of marine collagen from plaice by-products. Furthermore, the results feature how pre-treatment and extraction techniques affect the final protein and collagen concentration of the three studied by-products, with skins being the most promising collagen supplier. However, to fully understand and interpret the impact of the results, the knowledge of collagen extraction from skin, should be expanded. In conclusion, the current study highlights the potential of the applied green extraction procedures suitable for application in food, ingredient and cosmetic industries and suggests further research on the characteristics of the collagen structure as the next step to understand the functionality and applicability of the gained product.
Data availability statement
The raw data supporting the conclusions of this article will be made available by the authors, without undue reservation.
Author contributions
SKe: Conceptualization, Methodology, Investigation, Formal analysis, Writing – original draft. SKo: Conceptualization, Investigation, Formal analysis. KM: Validation, Methodology, Formal analysis. AJ: Conceptualization, Supervision, Validation, Writing – review & editing, Funding acquisition. JL: Conceptualization, Supervision, Validation, Writing – review & editing, Project administration, Resources, Funding acquisition.
Funding
The author(s) declare financial support was received for the research, authorship, and/or publication of this article. This study was supported by the OPTiMAT (optimal utilization of marine resources) project, funded by the Norwegian University of Science and Technology (NTNU).
Acknowledgments
We want to thank the technical staff of the food science division at NTNU for practical help in the analytical and food processing laboratory and express a special thank you to Siri Stavrum for her expertise with the HPLC.
Conflict of interest
The authors declare that the research was conducted in the absence of any commercial or financial relationships that could be construed as a potential conflict of interest.
Publisher’s note
All claims expressed in this article are solely those of the authors and do not necessarily represent those of their affiliated organizations, or those of the publisher, the editors and the reviewers. Any product that may be evaluated in this article, or claim that may be made by its manufacturer, is not guaranteed or endorsed by the publisher.
References
Al Khawli, F., Pateiro, M., Domínguez, R., Lorenzo, J. M., Gullón, P., Kousoulaki, K., et al. (2019). Innovative green Technologies of intensification for valorization of seafood and their by-products. Mar. Drugs 17:689. doi: 10.3390/md17120689
Ali, A. M. M., Kishimura, H., and Benjakul, S. (2018). Extraction efficiency and characteristics of acid and pepsin soluble collagens from the skin of golden carp (Probarbus Jullieni) as affected by ultrasonication. Process Biochem. 66, 237–244. doi: 10.1016/j.procbio.2018.01.003
AOAC. (1990). Official Methods of Analysis. 5th Edn, DC,Washington: Association of Official Analytical Chemist.
Avila Rodríguez, M. I., Rodríguez Barroso, L. G., and Sánchez, M. L. (2018). Collagen: a review on its sources and potential cosmetic applications. J. Cosmet. Dermatol. 17, 20–26. doi: 10.1111/jocd.12450
Blackburn, S. (1978). Amino acid determination: methods and techniques. 2nd Edn., rev. and expanded. New York: Marcel Dekker.
Commission and Communication (2020). Circular economy action plan: for a cleaner and more competitive Europe Brussels: Publications Office of the European Union,
Destandau, E., Michel, T., and Elfakir, C. (2013). Microwave-assisted extraction (Vol. 4). The Royal Society of Chemistry London, UK.
El Knidri, H., Dahmani, J., Addaou, A., Laajeb, A., and Lahsini, A. (2019). Rapid and efficient extraction of chitin and chitosan for scale-up production: effect of process parameters on deacetylation degree and molecular weight. Int. J. Biol. Macromol. 139, 1092–1102. doi: 10.1016/j.ijbiomac.2019.08.079
FAO (2022). The State of World Fisheries and Aquaculture 2022. Towards Blue Transformation. Rome, FAO. doi: 10.4060/cc0461en
Feng, X., Dai, H., Ma, L., Fu, Y., Yu, Y., Zhu, H., et al. (2021). Effect of microwave extraction temperature on the chemical structure and oil-water interface properties of fish skin gelatin. Innovative Food Sci. Emerg. Technol. 74:102835. doi: 10.1016/j.ifset.2021.102835
Hjellnes, V., Norges teknisk-naturvitenskapelige universitet Institutt for bioteknologi og, m., & Norges teknisk-naturvitenskapelige, u. (2021). "It's not uncomplicated to say you simply cannot do it like this anymore": a study of the Norwegian whitefish industry and the potential for improved utilization of rest raw materials (Publication Number p. 26) Norwegian University of Science and Technology, Faculty of Natural Sciences, Department of Biotechnology and Food Science. Trondheim.
Kendler, S., Thornes, F. W., Jakobsen, A. N., and Lerfall, J. (2023a). Nutritional profiling and contaminant levels of five underutilized fish species in Norway. Front. Nutr. 10:8094. doi: 10.3389/fnut.2023.1118094
Kendler, S., Tsoukalas, D., Jakobsen, A. N., Zhang, J., Asimakopoulos, A. G., and Lerfall, J. (2023b). Seasonal variation in chemical composition and contaminants in European plaice (Pleuronectes Platessa) originated from the west-coast of Norway. Food Chem. 401:134155. doi: 10.1016/j.foodchem.2022.134155
Kołodziejska, I., Żelechowska, E., Sadowska, M., Kołodziejski, W., and Niecikowska, C. (2008). Effect of extracting time and temperature on yield of gelatin from different fish offal. Food Chem. 107, 700–706. doi: 10.1016/j.foodchem.2007.08.071
Leach, A. A. (1960). Appendix—notes on a modification of the Neuman & amp; Logan method for the determination of the hydroxyproline. Biochem. J. 74, 70–71. doi: 10.1042/bj0740070
Lu, W.-C., Chiu, C.-S., Chan, Y.-J., Mulio, A. T., and Li, P.-H. (2023). Characterization and biological properties of marine by-product collagen through ultrasound-assisted extraction. Aquaculture Reports 29:101514. doi: 10.1016/j.aqrep.2023.101514
Mohamad Razali, U. H., Ya'akob, H., Sarbon, N. M., Zainan, N. H., Dailin, D. J., and Zaidel, D. N. A. (2023). Improving collagen processing towards a greener approach: current progress. J. Chem. Technol. Biotechnol. 98, 1063–1082. doi: 10.1002/jctb.7332
NMKL (2003). “Nitrogen” in Determination in foods and feeds according to Kjeldahl, Nordic Committee on Food Analysis (NMKL), vol. 6. 4th Edn, Bergen, Norway: NMKL NordVal International.
Osnes, K. K., and Mohr, V. B. (1985). Peptide hydrolases of antarctic krill, Euphausia superba. Comparative biochemistry and physiology. Comp. Biochem. 82, 599–606. doi: 10.1016/0305-0491(85)90496-1
Ozogul, F., Cagalj, M., Šimat, V., Ozogul, Y., Tkaczewska, J., Hassoun, A., et al. (2021). Recent developments in valorisation of bioactive ingredients in discard/seafood processing by-products. Trends Food Sci. Technol. 116, 559–582. doi: 10.1016/j.tifs.2021.08.007
Pal, G. K., and Suresh, P. V. (2016). Sustainable valorisation of seafood by-products: recovery of collagen and development of collagen-based novel functional food ingredients. Innovative Food Sci. Emerg. Technol. 37, 201–215. doi: 10.1016/j.ifset.2016.03.015
Santos, V. P., Maia, P., Alencar, N., Farias, L., Andrade, R. F. S., Souza, D., et al. (2019). Recovery of chitin and chitosan from shrimp waste with microwave technique and versatile application. Arq. Inst. Biol. 86:e0982018. doi: 10.1590/1808-1657000982018
Shaik, M. I., Chong, J. Y., and Sarbon, N. M. (2021). Effect of ultrasound-assisted extraction on the extractability and physicochemical properties of acid and pepsin soluble collagen derived from Sharpnose stingray (Dasyatis zugei) skin. Biocatal. Agric. Biotechnol. 38:102218. doi: 10.1016/j.bcab.2021.102218
Sikorski, Z. E., Scott, D. N., and Buisson, D. H. (1984). The role of collagen in the quality and processing of fish. Crit. Rev. Food Sci. Nutr. 20, 301–343. doi: 10.1080/10408398409527393
Tamilmozhi, S., Veeruraj, A., and Arumugam, M. (2013). Isolation and characterization of acid and pepsin-solubilized collagen from the skin of sailfish (Istiophorus platypterus). Food Res. Int. 54, 1499–1505. doi: 10.1016/j.foodres.2013.10.002
Keywords: green extraction methods, marine collagen, by-product valorization, upcycling, side-streams utilization, innovative collagen extraction
Citation: Kendler S, Kobbenes SMM, Jakobsen AN, Mukhatov K and Lerfall J (2023) The application of microwave and ultrasound technologies for extracting collagen from European plaice by-products. Front. Sustain. Food Syst. 7:1257635. doi: 10.3389/fsufs.2023.1257635
Edited by:
Dandan Ren, Dalian Ocean University, ChinaReviewed by:
Abdo Hassoun, Sustainable AgriFoodtech Innovation & Research (Safir), FranceZhijian Tan, Chinese Academy of Agricultural Sciences, China
Copyright © 2023 Kendler, Kobbenes, Jakobsen, Mukhatov and Lerfall. This is an open-access article distributed under the terms of the Creative Commons Attribution License (CC BY). The use, distribution or reproduction in other forums is permitted, provided the original author(s) and the copyright owner(s) are credited and that the original publication in this journal is cited, in accordance with accepted academic practice. No use, distribution or reproduction is permitted which does not comply with these terms.
*Correspondence: Sophie Kendler, sophie.kendler@ntnu.no