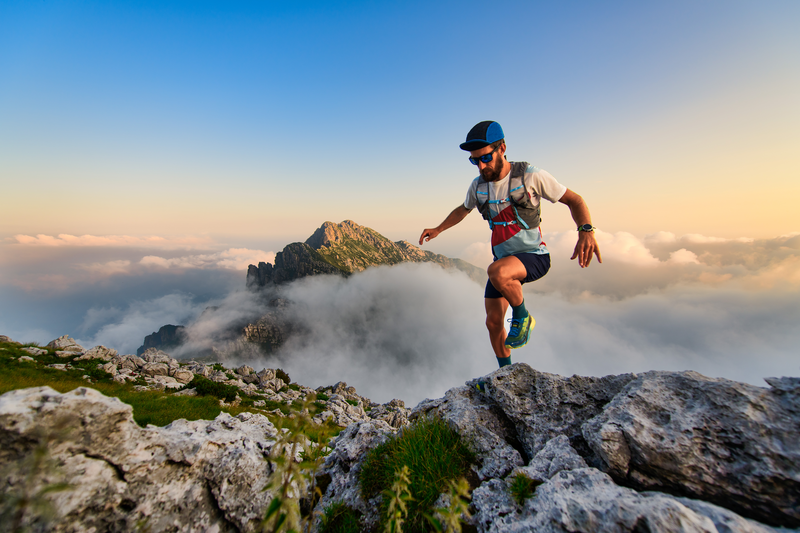
94% of researchers rate our articles as excellent or good
Learn more about the work of our research integrity team to safeguard the quality of each article we publish.
Find out more
ORIGINAL RESEARCH article
Front. Sustain. Food Syst. , 20 October 2023
Sec. Agroecology and Ecosystem Services
Volume 7 - 2023 | https://doi.org/10.3389/fsufs.2023.1254830
This article is part of the Research Topic Agrobiodiversity, Community Participation and Landscapes in Agroecology View all 14 articles
In the future, the frequency, duration, and intensity of extreme weather may increase, thus posing a threat to soil biodiversity in farmlands, particularly in agricultural production bases. However, little is known about the effect of cold wave events on the soil fauna community compared with other extreme weathers. Laboratory experiments fail to capture the complicated field environment of cold wave events. We investigated soil fauna communities in facility farmland (strawberry) and open farmland (green cabbage) during a cold wave event in Ningbo City, southeastern China. The results showed that the taxonomic richness of the total soil fauna community in facility farmland was significantly lower than that in open farmland, but the difference in abundance was not significant. The taxonomic richness and body size of soil mites and collembolan communities in facility farmland were significantly lower than those in the open farmland. Obvious differences in abundance, mean body length, mean body width, and ratio of body width to body length of Scheloribatidae, Galumnidae, Onychiuridae, Entomobryidae, and Enchytraeidae were detected between facility farmland and open farmland. The results of this study showed that the taxonomic richness, abundance, and body size of the soil fauna community in open farmland were significantly higher than those in facility farmland during a cold wave event. We suggested that the open farmland could be a “refuge” and “hot spot” of soil fauna community during the cold wave events.
Current and future global change is one of the most serious challenges that ecosystems and biodiversities face. Global warming is causing drastic increase in the frequency, intensity, and duration of various abiotic stresses, such as extremely high temperatures, drought, and cold waves (Organization WWM, 2020). These stresses negatively affect agricultural ecosystems and soil biodiversity (Rivero et al., 2021).
Most studies performing controlled experiments have focused on the effects of high temperature and drought on tolerance (Xie et al., 2023), reproduction (Organization WWM, 2020), biomass (Thakur et al., 2023), and vertical distribution (Dooremalen et al., 2012) of soil fauna populations and communities. However, few studies have focused on the effects of cold waves on soil fauna diversity, particularly through field experiments.
Owing to global warming, the increased frequency of soil freeze-thaw cycles featuring low temperatures have reduced the reproductive success of the soil nematode population (Scottnema lindsayae) in Taylor Valley, Antarctica (Knox et al., 2016). In fact, some soil invertebrates exhibit strong cold hardiness in the field. In the continental areas of Northeast Asia, some insects overwinter in a supercooled state and survive at temperatures ranging from −12 to −35°C, and earthworms can withstand temperatures ranging from −5 to −45°C in a frozen state (Berman and Leirikh, 2018). However, some soil invertebrates, such as termites (Reticulitermes flavipes), are not cold-tolerant. Termites likely rely on burrowing into the deep soil layers to avoid extremely low temperatures (Clarke et al., 2013).
Body size is a central functional trait in the community ecology of soil fauna (Andriuzzi and Wall, 2018). The temperature–size rule states that the adult body size of most ectotherms decreases with warming (Atkinson, 1994; Pequeno et al., 2018). Climatic changes may contribute to extreme body size diversity in terrestrial invertebrates (Karagkouni et al., 2016). Climate warming directly and indirectly results in body size changes in soil fauna (Frelich et al., 2012). For example, a warmer climate leads to higher litter quality, which indirectly promotes the replacement of small-bodied detritivores with large-bodied exotic earthworms (Frelich et al., 2012). Fluctuation around a mean temperature of 12°C affected nematode body size to a greater extent than when the average temperature was constant (Cedergreen et al., 2016). Although a few studies have focused on the relationship between temperature and the body size of soil fauna, the effects of cold waves featuring low temperatures on the body size of soil fauna communities is poorly understood.
China has experienced an unprecedented frequency of cold events (Chang and Xiao, 2023). Cold air influences high and low latitudinal areas (Abdillah et al., 2021). An extreme high–temperature event in the summer of 2022 had a negative effect on soil fauna diversity in agricultural ecosystems in Ningbo City, southeast China (Gao et al., 2023). Subsequently, a cold wave event affected the same area in January 2023. Cold wave events are often accompanied by extremely low temperatures and rapid temperature drops (Chang and Xiao, 2023), resulting in more frequent exposure to extremely low temperatures and sudden temperature fluctuations for the soil fauna community.
China is the largest producer of greenhouse vegetables (facility farmlands) worldwide (Yuan et al., 2022). Sunlight, temperature, and humidity inside the facility farmland are controlled for the production of high-quality vegetables, fruits, and crops throughout the year; thus, the microclimate inside the facility is significantly different from that of an open farmland (Liu et al., 2021). Therefore, we hypothesized that (1) the richness and abundance of the soil fauna community in the facility farmland was higher than that in the open farmland during the cold wave event, as it was protected from cold air and strong temperature fluctuations, and (2) the body size of the soil fauna community in the facility farmland was smaller than that in the open farmland during cold wave events based on the temperature–size rule.
To identify the composition and body size of the soil fauna community during the cold wave event of January 2023, we investigated the soil fauna community in facility farmland and open farmland in Ningbo, southeastern China. We expected that the facility farmland would be a refuge and shelter for the soil fauna community during the cold wave event. Therefore, we assumed that a “hot spot” of the soil fauna community would be observed in the facility farmland.
The study area is located in the Ningbo city (28°51′-30°33′ N, 120°55′-122°16′ E) in Zhejiang Province, southeastern China. Ningbo is an important port city on the southeast coast of China and an economic center in the southern wing of the Yangtze River Delta. At the end of 2022, the permanent resident population of Ningbo was 9.618 million, and the proportion of the urban population to the total population (i.e., urbanization rate) was 78.9%. Ningbo has a dominant subtropical monsoon climate. The mean annual temperature is 16.4°C. The highest temperature in July is 28.0°C, and the lowest in January is 5.4°C. The mean annual precipitation is approximately 1,480 mm, and rainfall in May–September accounts for 60% of the annual precipitation. The plain area of Ningbo accounts for 40.3% of its land area (http://www.ningbo.gov.cn/col/col1229099787/index.html).
During the summer of 2022 (June–August), Ningbo reported an extremely high temperature event (Ningbo Climate Center, 2022; Gao et al., 2023). Subsequently, Ningbo was affected by a strong cold wave in January 2023 (winter). The temperature in Ningbo dropped from 17.5°C (January 13) to 1.7°C (January 15) and −5.3°C (January 23) (Ningbo Climate Center, 2023) (Figure 1). During the cold wave period, some crops, vegetables, and fruits in open farmlands exhibited cold-related damage (Ningbo Climate Center, 2023). However, plants grown in facility farmlands were not affected by the strong cold wave event because of the relatively warm and stable temperatures inside the facility (field survey data).
The study was conducted at the Feihong Farm (29.99 N, 121.56 E) located in Zhenhai District, Ningbo. Feihong Farm was established in 2006 and covers an area of 46.66 hm2. There were 33.33 hm2 facility farmland structures in steel sheds. In 2007, the vegetable output of Feihong Farm was more than 1500 tons, making it an important “vegetable basket” for Ningbo. In recent years, the main planting mode in facility farmlands has been tomato/strawberry rotation. Simultaneously, farmers also grow various vegetables in small areas of open farmlands adjacent to facility farmlands. The main planting mode in open farmland was a green cabbage/lettuce/carrot/onion rotation, which depends on the preferences of workers and farmers. These vegetables were mainly used as food resources for workers in Feihong Farm and were not sold. When this experiment was conducted, strawberry and green cabbage were planted in the facility and open farmlands, respectively (Figure 2). The soil types of both farmlands were red soil (Argi-Udic Ferrosols under the Chinese Soil Taxonomy and Adults under the USDA soil classification system) (Gong et al., 2007). The facility farmland was irrigated using drip irrigation tubes. There was no irrigation system in the open farmland, and soil water mainly originated from rainfall and casual manual watering from canals.
Three plots (covering areas of 3×3 m2 and set 50 m apart) were set up as three replicates in both the facility and open farmlands. The facility farmland covered an area of 4,320 m2 with a width of 60 m and length of 72 m. For open farmland, at least one canal with a width of 2 m and a height of 1.5 m separated each plot. Three cylindrical soil cores (7-cm diameter, 15-cm depth) were randomly collected from each of the six plots. The samples were collected on January 21, 2023 (winter). In total, 18 samples (three replicates in each plot × three plots for each type of farmland × two types of farmlands) were collected.
A Berlese–Tullgren funnel was used to extract the soil fauna (Straalen and Rijninks, 1982). The soil samples were placed on a sieve (2 mm-meshed diameter) above a funnel and exposed to ambient temperature for 10 days. Soil fauna were collected in plastic bottles with 95% alcohol and preserved in a refrigerator at −20°C.
All extracted soil fauna were picked out for further study. The soil fauna were calculated and identified under a stereo-microscope (Olympus Lympus SZX16 and Nikon Eclipse 80i) according to past studies (Bellinger et al., 1996–2012; Yin et al., 1998; Krantz and Walter, 2009). Collembola, oribatids, and Enchytraeidae were identified at the family level, whereas other soil fauna were identified at the order level and labeled as different taxa. Body length (μm) and body width (μm) were measured using a stereo-microscope (Olympus SZX16) and Image View software (Moretti et al., 2017). The body width to body length (body ratio) was calculated. Soil water content was measured gravimetrically by drying the soil samples at 105°C for 48 h. Soil water content was described as a percentage of dry weight (Lu, 2000).
Taxonomic richness (taxonomic number) and abundance (individual number) were used to describe the diversity of the soil fauna community. Mean body length (mm), mean body width (mm), and body ratio were used to describe the body size features of the soil fauna community.
Community dominance degree was calculated to reveal the dominance of soil fauna taxon in abundance:> = 10% of the total abundance of individuals represented the dominant taxon (+++); 1–10% the common taxon (++); and < 1% was rare taxon (+) (Wei et al., 2022).
Differences in richness, abundance, mean body length, mean body width, body ratio, and soil water content between the facility and open farmlands were examined using a nonparametric Mann-Whitney U test. A linear mixed effect model was used to evaluate the effects of soil water content on richness, abundance, mean body length, mean body width, and body ratio of soil fauna and abundance of each taxon using the function “lmer” in the “lme4” package (Bates et al., 2015). The fixed effect was soil water content and the random effect was the two types of farmlands. Principal component analysis (PCA) was performed to evaluate the associations among richness, abundance, mean body length, mean body width, and body ratio of soil fauna using the functions “prcomp” and “fviz_pca_biplot” in the “tidyverse” (Wickham et al., 2019) and “factoextra” (Kassambara and Mundt, 2020) packages. All calculations were performed in the R 4.2.2 (R Core Team, 2022).
In total, eight taxa were recorded in the facility farmland, whereas 19 taxa were collected from the open farmland. Isotomidae and Mesostigmata sp.1 were the dominant abundant taxa in the facility farmland. Mesostigmata sp.1 was the dominant taxon in the open farmland (Table 1). The density of the total soil fauna community was 3,637.83 ± 2,268.58 individuals/m2 in the facility farmland, and 4,850.44 ± 2,083.56 individuals/m2 in the open farmland (Table 1).
Table 1. Composition and abundance of soil fauna communities in facility and open farmlands during the cold wave event.
The taxonomic richness of the total soil fauna community in the facility farmland was significantly lower than that in the open farmland (p < 0.01) (Figure 3A). Soil fauna abundance did not differ significantly between the facility and open farmlands (Figure 3B).
Figure 3. Taxonomic richness (A) and abundances (B) of total soil fauna communities in facility and open farmlands during the cold wave event. NS, not significant; **p < 0.01.
The taxonomic richness of the soil mites (p < 0.01; Figure 4A) and collembolan (p < 0.01; Figure 4C) communities in the facility farmland was significantly lower than that in the open farmland. However, no significant difference was detected in the abundance of soil mites and collembolan communities between the two farmlands (Figures 4B, D).
Figure 4. Taxonomic richness and abundances of soil mite (A, B) and collembolan (C, D) communities in facility and open farmlands during the cold-wave event. NS, not significant; **p < 0.01.
The abundances of Scheloribatidae (p < 0.01; Figure 5A), Galumnidae (p < 0.05; Figure 5B), Onychiuridae (p < 0.01; Figure 5C), Entomobryidae (p < 0.05; Figure 5D), and Enchytraeidae (p < 0.05; Figure 5E) in the facility farmland were significantly lower than those in the open farmland.
Figure 5. Taxonomic abundances of Scheloribatidae (A), Galumnidae (B), Onychiuridae (C), Entomobryidae (D), and Enchytraeidae (E) in facility and open farmlands during the cold wave event. *p < 0.05; **p < 0.01.
Mean body length, mean body width, and body ratio of the total soil fauna community in the facility and open farmlands was 918.83 ± 989.72 mm and 1,107.07 ± 296.91 mm, 213.34 ± 145.26 mm and 296.77 ± 74.61 mm, 0.23 ± 0.09 and 0.27 ± 0.1, respectively. There was no significant difference in mean body length, mean body width, or body ratio of the total soil fauna communities between the facility and open farmlands (Figures 6A–C).
Figure 6. Mean body lengths (A), mean body widths (B), and body ratios (C) of total soil fauna communities in facility and open farmlands during the cold wave event. NS, not significant.
Except the mites community (Figure 7A), mean body lengths of the collembolan community (p < 0.01; Figure 7B), Scheloribatidae (p < 0.01; Figure 7C), Galumnidae (p < 0.05; Figure 7D), Onychiuridae (p < 0.01; Figure 7E), Entomobryidae (p < 0.05; Figure 7F), and Enchytraeidae (p < 0.05; Figure 7G) were significantly difference between facility and open farmlands.
Figure 7. Mean body lengths of mite community (A), collembolan community (B), Scheloribatidae (C), Galumnidae (D), Onychiuridae (E), Entomobryidae (F), and Enchytraeidae (G) in facility and open farmlands during the cold wave event. NS, not significant; *p < 0.05; **p < 0.01.
Mean body width of the soil mite community (p < 0.05; Figure 8A), collembolan community (p < 0.05; Figure 8B), Scheloribatidae (p < 0.01; Figure 8C), Galumnidae (p < 0.05; Figure 8D), Onychiuridae (p < 0.01; Figure 8E), Entomobryidae (p < 0.05; Figure 8F), and Enchytraeidae (p < 0.05; Figure 8G) were significantly different between the facility and open farmlands.
Figure 8. Mean body widths of soil mite community (A), collembolan community (B), Scheloribatidae (C), Galumnidae (D), Onychiuridae (E), Entomobryidae (F), and Enchytraeidae (G) in facility and open farmlands during the cold wave event. *p < 0.05; **p < 0.01.
The body ratio of soil mite community (p < 0.01; Figure 9A), collembolan community (p < 0.05; Figure 9B), Scheloribatidae (p < 0.01; Figure 9C), Galumnidae (p < 0.05; Figure 9D), Onychiuridae (p < 0.01; Figure 9E), Entomobryidae (p < 0.05; Figure 9F), and Enchytraeidae (p < 0.05; Figure 9G) was significantly different between the two farmlands.
Figure 9. Body ratios of soil mite community (A), collembolan community (B), Scheloribatidae (C), Galumnidae (D), Onychiuridae (E), Entomobryidae (F), and Enchytraeidae (G) in facility and open farmlands during cold wave event. *p < 0.05; **p < 0.01.
Mean soil water content was 28.49% and 27.90% in the facility farmland and open farmland, respectively, exhibiting no significant variation. The mean soil water content had no significant effect on taxonomic richness, abundance, mean body length, mean body width, and body ratio of the total soil fauna. Mean soil water content significantly affected abundance (F = 9.94, p < 0.01), mean body length (F = 8.68, p < 0.01), mean body width (F = 9.65, p < 0.01), and body ratio (F = 10.22, p < 0.01) of Mesostigmata sp.3, and body ratio of Staphylinidae (F = 4.95, p < 0.05) (Table 2).
Table 2. Effects of mean soil water content on richness, abundance, mean body length, mean body width, and body ratio of soil fauna community, and on abundance of each taxon.
The first two components, accounted for 72.7% of the cumulative variation. Significant correlations were identified between mean body length and mean body width, richness, and body ratio (Figure 10).
Figure 10. Biplot of principle component analysis (PCA). The x-axis and y-axis represent principal component 1 (Dim1) and principal component 2 (Dim2), respectively. The percentages on the x- and y-axes represent the variance explained by each principal component (% variances explained). The abun, rich, length, width, and ratio represent abundance, richness, mean body length, mean body width, and body ratio, respectively.
Contrary to the first hypothesis of this study, the richness and abundance of the soil fauna community in the facility farmland was lower than that in the open farmland after the cold wave event.
The taxonomic richness of the total soil fauna community was more than two-fold higher in the open farmland than in the facility farmland, which was a significant difference. The abundance of the total soil fauna community in the open farmland was one-third higher than that in the facility farmland, although the difference was not statistically significant. Previous studies in temperate regions have demonstrated that the richness, abundance, and biodiversity indices of soil fauna communities in facility farmlands were lower than those in open farmlands (Dong et al., 2008; Wang, 2008; Chen et al., 2019). This study exhibited a similar finding for richness in the subtropical region during the cold wave event but not for abundance. After continuous investigation of more than a year in the study areas, researchers reported that the daily maximum and daily minimum temperatures in facility farmlands that planted strawberry were higher by 5–10°C and 3–6°C, respectively, than that in open farmlands (Fu et al., 2011). In fact, the temperature was warmer by approximately 11°C in the facility farmland (20°C at 10 a.m., field measurement using a thermometer) than that in open farmland (8.5°C on average, https://m.tianqibag.com/ningbo2yuetianqi/). Furthermore, soil water content had no significant effect on the richness or abundance of the total soil fauna community in either farmland types during the experimental period (Figure 10). Therefore, we were unable to determine the impact of the cold wave event and sudden temperature drops on the differences in richness and abundance of the total soil fauna community between the facility and open farmlands. We speculate that overfertilization, over irrigation, pesticide application, and continuous monocropping in facility farmland might have contributed to this observed phenomenon, as these factors cause considerable soil degradation and soil-borne disease spread (Wan et al., 2023), and they may have contributed to the decreased soil fauna diversity (Jiang et al., 2019).
Soil mites and collembolan communities were dominant in both the facility and open farmlands during the cold wave event, which is consistent with the results of other studies (Dong et al., 2008; Wang, 2008). For example, the abundance of both soil mites and Collembola accounted for 56.9% (Dong et al., 2008) and 91.9% (Wang, 2008), respectively, of the total abundance of soil fauna communities in facility farmlands in temperate regions. Additionally, soil collembolan communities were dominant in the facility farmland (54.80%) in the present study, whereas the soil mite community was dominant in the open farmland (55.40%). A study in temperate regions also reported that the abundance of Collembola rather than soil mites prevailed in facility farmlands during different years of cultivation (Wang, 2008). Winter air temperature regulates soil mite and Collembola populations at the local scale in Arctic ecosystems (Coulson et al., 2023). A higher abundance was observed for Oribatid in the environment at −2°C compared with that at +2°C in a sub-arctic soil (Sjursen et al., 2005). In a study on black soil farmlands, the richness and abundance of soil mites were significantly higher than those of Collembola, indicating that soil mites are more tolerant to cold winter temperatures in temperate regions (Zhang et al., 2020). Additionally, Scheloribatidae, Galumnidae, Onychiuridae, and Entomobryidae were significantly abundant in the open farmland in the present study but absent in the facility farmland. Similarly, a different study observed the abundance of Onychiuridae and Entomobryidae in open corn farmlands adjacent to facility farmlands (Chen et al., 2019). Therefore, we speculated that the low temperature constrained the abundance of the Collembolan community rather than that of soil mites in the open field, indicating that soil mites were better able to withstand the low temperature in open farmland during cold wave events.
Enchytraeidae are important biological indicators that are sensitive to chemical stress in farmlands (Didden and RoKmbke, 2001). Therefore, the obvious preference of Enchytradae worms for open farmland rather than facility farmland can be attributed to the chemical stress from intensive management such as fertilization and pesticide application in the facility farmland. Precipitation, temperature, and pH influence species composition rather than mean abundance in Enchytraeid communities (Didden, 1993). Enchytraeids prefer habitats with sufficient moisture, organic matter, and oxygen (Niva et al., 2015). However, the facility farmland with relatively warmer air temperatures and moist soil failed to maintain a relative abundance of worms, indicating that the cold temperature during the cold wave event was not a significant regulator of Enchytraeidae worms.
Isotomidae thrived on facility farmland with 13 times more abundance in the facility farmland than in the open farmland. A previous study reported that Isotomidae showed dominant abundance in facility farmlands in temperate regions (Chen et al., 2019). However, in this study, only one family of Collembola existed on the facility farmland. Some Collembola have been proposed as potential regulators that suppress pathogens and diseases in facility farmlands by promoting the activities of soil microbes and feeding on pathogens (Zhang et al., 2023). For example, Folsomia hidakana (Isotomidae) suppresses damping-off disease in cabbage by feeding on Rhizoctonia solani (Shiraishi et al., 2003) and Proisotoma minuta (Isotomidae) suppresses pathogens and diseases in cotton (Lartey et al., 1994) in facility farmlands. Common diseases that have been reported in strawberry facility farmlands in Ningbo include powdery mildew, Verticillium wilt, downy mildew, anthracnose, gray mold, sharp eyespot, and spot blotch (Lian et al., 2018). Pathogens of these diseases may serve as food resources for Isotomidae in the facility farmland. However, direct evidence for Isotomidae feeding on pathogens is limited to the study area, and further studies are needed to validate this claim.
In this study, Staphylinidae were detected in the facility farmland and not in the open farmland during the cold wave event. This could be attributed to their sensitivity to the temperature in farmlands (Porhajašová et al., 2018). Staphylinidae (Quedius pellax) is a winter-active taxon with a preferred distribution among habitats with relatively high winter temperatures and humid environments (Topp and Smetana, 1998). This suggests that the low temperature and sudden temperature drop (particularly at night) (Lima et al., 2015) in the open farmland constrained the activity of Staphylinidae. Another possible reason is the relatively sufficient food resources available for Staphylinidae at the facility farmland. Staphylinidae is an important pest predator that suppresses the soil-dwelling life stages of western flower thrips (Li et al., 2019) and controls fungus gnats (Jandricic et al., 2006) and maggot (Read, 1962) in facility farmlands. Natural predators that control pests in open farmland are usually absent in the facility farmland, resulting in a more rapid and severe development of pests in the facility farmland than in the open farmland (Thao et al., 2022). Therefore, Diptera larva, mites (Perumalsamy et al., 2009), Collembola (Jaloszynsk, 2012), and other possible pests in the facility farmland might provide more food resources for Staphylinidae.
Soil parameters (Minor et al., 2016) and cover crops (Madzaric et al., 2017) affected the composition and diversity of soil fauna community in facility farmland and in cold field environment. Soil water content did not significantly affect taxonomic richness and abundance of total soil fauna community, and abundance of each taxon, except for Mesostigmata sp.3 in this study. Numerous studies have found that soil fauna, such as Collembola (Dombos, 2001), oribatid mites (Jakšová et al., 2020), and spider mites (Gill et al., 2023) respond to changes in soil water content. However, certain studies reported that soil water content did not affect soil fauna communities (Sinka et al., 2007; Gergocs and Hufnagel, 2009). The low soil moisture difference between the facility and open farmlands might not result from their own textural characteristics but from irrigation management. Soil water content in the facility farmland in the present study primarily originated from irrigation; however, that in the open farmland primarily originated from rainfall and casual manual irrigation. In fact, irrigation also affected the richness and abundance of Collembola (Cutz-Pool et al., 2007) and oribatid mites (Iglesias et al., 2019) except for rainfall in farmlands. Therefore, sources of soil water, that is irrigation and rainfall, might affect soil fauna in both farmlands. Additionally, crop rotation affected the richness and abundance of Collembola (Twardowski et al., 2016), oribatid mites (Bosch-Serra et al., 2023), and other soil fauna (O'Rourke et al., 2008). A tomato/strawberry rotation with intensive management was performed in the facility farmland, whereas a green cabbage/lettuce/carrot/onion rotation system with less intensive management was conducted in the open farmland. The soil management, fertilization, and pest and disease control were less intensive and casual in the open farmland, and depended on the preferences of the farmers and workers. Therefore, soil parameters and different management strategies, including the irrigation and rotation system, should be studied further.
The body sizes, including the mean body length, mean body width, and body ratio, of soil mites and collembolan communities in the facility farmland were significantly smaller than those in the open farmland. The body sizes of Scheloribatidae, Galumnidae, Onychiuridae, Entomobryidae, and Enchytraeidae in the facility farmland were also significantly smaller than those in the open farmland. Soil water content significantly affected mean body length, mean body width, and body ratio of Mesostigmata sp.3 and body ratio of Staphylinidae, but not for any other soil fauna.
Temperature is an important factor that affects the body size of soil fauna (Cedergreen et al., 2016). Climate warming is often more detrimental to fauna with relatively large body sizes than to those with relatively small body sizes (Thakur et al., 2023). The total biomass of soil Collembola declined in warm soil primarily because the species density decreased as the body size increased (Thakur et al., 2023). The adult body size of spider mites (Tetranychus ludeni Zacher) (Ristyadi et al., 2021) and Enchytraeidae decreases with increasing temperature (Didden and RoKmbke, 2001; Holmstrup et al., 2022). Therefore, we inferred that the reduction in the body sizes of soil mites and collembolan communities possibly resulted from the relatively warmer and more stable temperature in the facility farmland compared with those in the open farmland.
Additionally, the distribution of soil fauna depends on aggregate pores (Quénéhervé and Chotte, 1996). For example, soil pore space influences the nematode body size (Briar et al., 2011). Intensive management of farmland results in increased soil compaction and bulk density and decreased total porosity and capillary porosity (Wang et al., 2010). Therefore, the small body sizes of soil mites and Collembola might allow for easy movement and increased activity within small soil pore spaces in facility farmlands.
However, no significant difference in the body size of the total soil fauna community was detected between the facility and open farmlands. Given that the body size of soil fauna varies remarkably (Andriuzzi and Wall, 2018), we were unable to account for the considerable heterogeneity in the body sizes of different taxa and identify any significant differences between the body sizes in the facility and open farmlands in the present study.
Bottom-up and top-down effects have been reported with respect to body size determination in soil fauna communities and soil parameters (Andriuzzi et al., 2020), resource availability (Andriuzzi and Wall, 2018), habitat type (Palmer, 1994), and disturbance (Tyler, 2008) have been proposed as significant underlying reasons. Compared to the open farmland, soil water content in vertical distribution might be more even due to thorough irrigation and plowing in the facility farmland. Considering the characteristics of the strong vertical stratification of soil fauna (Dooremalen et al., 2012), soil fauna with small body sizes might remain active in the top soil layer without being affected by drought. Although we did not detect significant effects of soil water content (top soil) on the body sizes of collembolan and soil mites communities and taxa abundances, soil water content in vertical layers should be studied further. Additionally, soil temperature has been identified as an important factor affecting body size of soil fauna (Xu et al., 2012; Lindo, 2015; Thakur et al., 2023). Moreover, other soil parameters, such as soil pH, soil texture, soil compaction, total carbon, soil organic matter, and aridity exhibited significant effects on the body size of soil fauna (Costa-Milanez et al., 2017; Schmidt F. A. et al., 2017; Andriuzzi et al., 2020; Wang et al., 2023). Agricultural fertilization (Liu et al., 2015; Niu et al., 2022), management intensification (Yin et al., 2020), herbivores and grazing (Andriuzzi and Wall, 2018), crop rotation (Postma-Blaauw et al., 2010), crop diversity (Postma-Blaauw et al., 2010), and plant presence (Gao et al., 2022) were found to affect the body sizes of soil fauna. Unfortunately, this study did not consider other factors except for soil water content; more environmental and human factors should be considered in future research studies. To reveal the objective of this study and avoid the influences of planting time, crop variety, and agricultural management among other factors, one farmland (Feihong Farm) was selected in the present study. This limitation would be resolved through selecting more farms in future studies. Additionally, comparing the facility farmland with adjacent soil with non-agricultural activity in the future would be beneficial to constructing “baseline data” of soil quality and biodiversity. This analysis would reveal the general effect of air and soil temperature changes in the study area.
Additionally, soil fauna have been used as biological control agents and indicators for facility farmland management (Campos-Herrera and Gutiérrez, 2009; Schmidt J. M. et al., 2017). The study results suggested that facility farmland failed in the provision of refuge or shelter for soil fauna community in cold wave events. By providing habitats, refuge, and food resources for pests and their natural enemies, open-field crops around facility farmlands could affect pest dynamics in facility farmlands (Doehler et al., 2023). Therefore, pest management methods in facility farmland should consider using the surrounding environment (Doehler et al., 2023). Installing hedgerows around facility farmlands to maintain natural enemies and encourage biological pest control is a useful practical management strategy (López-Felices et al., 2022). However, local farmers are reluctant to implement these measures despite the obvious economic and environmental advantages (López-Felices et al., 2022). According to the study results, open field farmlands could be a hotspot, refuge, shelter, and recruitment pool for soil fauna communities for facility farmlands. The study results also indicate the usefulness of more open farmlands with small areas adjacent to facility farmlands. We believe having open farmlands near the facility farmlands would not cause conflict in the demands of agricultural products; Moreover, their areas and shapes vary and they require non-intensive management. The soil biodiversity of facility farmlands can be recovered and rescued through such suitable management strategies. The study findings indicate that the management of open farmlands adjacent to facility farmlands is a relatively effective and economical restoration method and conservation strategy for agricultural management.
This study aimed to reveal the diversity and body size of the soil fauna community in facility and open farmlands during a cold wave event in Ningbo in the winter of 2023. The richness, abundance, and body size of the soil fauna community in the facility farmland were significantly lower than those in the open farmland, indicating that the facility farmland failed to be a refuge or shelter for soil fauna communities during the cold wave event. This study also suggests that the open farmland adjacent to the facility farmland is a “hot spot” for the soil fauna community during cold wave events in subtropical regions. However, more factors including soil parameters, food resources, and agricultural managements, which were important factors affecting soil fauna community, should be further studied. The results of this study emphasize that the maintenance mechanisms of soil fauna communities are affected by cold wave events and provide useful information for soil biodiversity restoration in intensively managed facility farmlands.
The original contributions presented in the study are included in the article/supplementary material, further inquiries can be directed to the corresponding author.
The manuscript presents research on animals that do not require ethical approval for their study.
MG: Conceptualization, Data curation, Methodology, Writing—original draft, Writing—review and editing. YJ: Investigation, Methodology, Writing—original draft, Data curation. JS: Investigation, Methodology, Writing—original draft, Data curation. TL: Writing—original draft, Methodology, Investigation. YZ: Methodology, Investigation, Writing—original draft. JLa: Methodology, Writing—review and editing, Formal analysis. JLi: Conceptualization, Methodology, Supervision, Writing—original draft, Writing—review and editing.
This research was supported by grants from the National Natural Science Foundation of China (42271051), the Zhejiang Public Welfare Technology Application Research Project (LGN22D010006), the Ningbo Natural Science Foundation Project (2021J129 and 2023J006), and the Science and Technology Program of Jilin Province (No. CXGC2021ZY120).
The authors would like to thank Zhijing Xie and Xue Pan for identifying the Collembola and Oribatid, respectively, and the management of Feihong Farm for their support and cooperation during the field experiments.
The authors declare that the research was conducted in the absence of any commercial or financial relationships that could be construed as a potential conflict of interest.
All claims expressed in this article are solely those of the authors and do not necessarily represent those of their affiliated organizations, or those of the publisher, the editors and the reviewers. Any product that may be evaluated in this article, or claim that may be made by its manufacturer, is not guaranteed or endorsed by the publisher.
Abdillah, M. R., Kanno, Y., Iwasaki, T., and Matsumoto, J. (2021). Cold surge pathways in east Asia and their tropical impacts. J. Climate. 34, 157–170. doi: 10.1175/JCLI-D-20-0552.1
Andriuzzi, W. S., Franco, A. L. C., Ankrom, K. E., Cui, S., d. Tomasel, C. M., Guan, P., et al. (2020). Body size structure of soil fauna along geographic and temporal gradients of precipitation in grasslands. Soil Biol. Biochem. 140, 107638. doi: 10.1016/j.soilbio.2019.107638
Andriuzzi, W. S., and Wall, D. H. (2018). Grazing and resource availability control soil nematode body size and abundance–mass relationship in semi-arid grassland. J. Anim. Ecol. 87, 1407–1417. doi: 10.1111/1365-2656.12858
Atkinson, D. (1994). Temperature and Organism Size–A Biological Law for Ectotherms? London: Academic Press.
Bates, D., Mächler, M., Bolker, B., and Walker, S. (2015). Fitting linear mixed-effects models using lme4. J. Stat. Soft. 67, 1–48. doi: 10.18637/jss.v067.i01
Bellinger, P. F., Christiansen, K. A., and Janssens, F. (1996–2012). Checklist of the Collembola of the World. Available online at: http://www.collembola.org (accessed April 28, 2023).
Berman, D. I., and Leirikh, A. N. (2018). Cold hardiness of mass soil invertebrate animals of Northeastern Asia: 1. Cold hardiness and the mechanisms of its maintenance. Biol. Bull. 45, 669–679. doi: 10.1134/S1062359018070038
Bosch-Serra, À. D., Molina, M. G., González-Llinàs, E., Boixadera-Bosch, R. R., Martínez, B., Orobitg, J., et al. (2023). Oribatid mites in different Mediterranean crop rotations fertilized with animal droppings. Exp. Appl. Acarol. 90, 185–202. doi: 10.1007/s10493-023-00812-4
Briar, S. S., Fonte, S. J., Park, I., Six, J., Scow, K., and Ferris, H. (2011). The distribution of nematodes and soil microbial communities across soil aggregate fractions and farm management systems. Soil Biol. Biochem. 43, 905–914. doi: 10.1016/j.soilbio.2010.12.017
Campos-Herrera, R., and Gutiérrez, C. (2009). Screening Spanish isolates of steinernematid nematodes for use as biological control agents through laboratory and greenhouse microcosm studies. J. Invertebr. Pathol. 100, 100–105. doi: 10.1016/j.jip.2008.11.009
Cedergreen, N., Nørhave, N. J., Svendsen, C., and Spurgeon, D. J. (2016). Variable temperature stress in the nematode Caenorhabditis elegans (Maupas) and its implications for sensitivity to an additional chemical stressor. PLoS ONE 11, e0140277. doi: 10.1371/journal.pone.0140277
Chang, S., and Xiao, A. (2023). Characteristics of extreme cold wave events over Southwest China and their possible relationship with the North Atlantic SST. Clim. Dynam. 60, 1619–1634. doi: 10.1007/s00382-022-06383-1
Chen, Q., Li, Q., Tang, W., Wang, Z., Tao, J., Xu, Y., et al. (2019). Effects of different cultivation facilities on the diversity of soil meso- and micro-fauna. Chin. J. Eco-Agr. 27, 1147–1156. doi: 10.13930/j.cnki.cjea.180618
Clarke, M. W., Thompson, G. J., and Sinclair, B. J. (2013). Cold tolerance of the eastern subterranean termite, Reticulitermes flavipes (Isoptera: Rhinotermitidae), in Ontario. Environ. Entomol. 42, 805–810. doi: 10.1603/EN12348
Costa-Milanez, C. B., Majer, J. D., Castro, P. T. A., and Ribeiro, S. P. (2017). Influence of soil granulometry on average body size in soil ant assemblages: implications for bioindication. Perspect. Ecol. Conser. 15, 102–108. doi: 10.1016/j.pecon.2017.03.007
Coulson, S. J., Convey, P., Schuuring, S., and Lang, S. I. (2023). Interactions between winter temperatures and duration of exposure may structure Arctic microarthropod communities. J. Therm. Biol. 114, 103499. doi: 10.1016/j.jtherbio.2023.103499
Cutz-Pool, L. Q., Palacios-Vargas, J. G., Castaño-Meneses, G., and García-Calderón, N. E. (2007). Edaphic Collembola from two agroecosystems with contrasting irrigation type in Hidalgo State, Mexico. Appl. Soil Ecol. 36, 46–52. doi: 10.1016/j.apsoil.2006.11.009
Didden, W., and RoKmbke, J. (2001). Enchytraeids as indicator organisms for chemical stress in terrestrial ecosystems. Ecotox. Environ. Safe. 50, 25–43. doi: 10.1006/eesa.2001.2075
Doehler, M., Chauvin, D., Ralec, A. L., Vanespen, É., and Outreman, Y. (2023). Effect of the landscape on insect pests and associated natural enemies in greenhouses crops: the strawberry study case. Insects 14, 302. doi: 10.3390/insects14030302
Dombos, M. (2001). Collembola of loess grassland: effects of grazing and landscape on community composition. Soil Biolo. Biochem. 33, 2037–2045. doi: 10.1016/S0038-0717(01)00125-0
Dong, B., Zhang, R., Jing, S., Xie, Y., and Yao, Y. (2008). Animal community structure in greenhouse soils with different planting years in Shouguang City. Chin. J. Appl. Ecol. 19, 1769–1774. doi: 10.13287/j.1001-9332.2008.0325
Dooremalen, C., Berg, M. P., and Ellers, J. (2012). Acclimation responses to temperature vary with vertical stratification: implications for vulnerability of soildwelling species to extreme temperature events. Global Change Biol. 19, 975–984. doi: 10.1111/gcb.12081
Frelich, L. E., Peterson, R. O., Dovciak, M., Reich, P. B., Vucetich, J. A., and Eisenhauer, N. (2012). Trophic cascades, invasive species and body-size hierarchies interactively modulate climate change responses of ecotonal temperate –boreal forest. Philos. T. R. Soc. B 367, 2955–2961. doi: 10.1098/rstb.2012.0235
Fu, G., Zhang, B., Yang, Z., Fei, Y., Huang, H., and Sun, J. (2011). Research on the microclimate characteristics and inside temperature prediction model for plastic greenhouse. Chin. Agr. Sci. Bull. 27, 242–248.
Gao, H., Li, C., Jiao, Y., Zhang, Y., and Feng, Q. (2022). Shrubs alter alpha and beta diversity of soil fauna in a semiarid grassland. Rangeland J. 44, 213–220. doi: 10.1071/RJ22054
Gao, M., Peng, C., Hu, Y., Liu, W., Ye, Y., Zheng, Y., et al. (2023). Composition and vertical distribution of agricultural soil Macrofauna community after an extreme high temperature event in the summer of 2022. Ecol. Indic. 2023, 110439. doi: 10.1016/j.ecolind.2023.110439
Gergocs, V., and Hufnagel, L. (2009). Application of oribatid mites as indicators (review). Appl. Ecol. Env. Res. 7, 79–98. doi: 10.15666/aeer/0701_079098
Gill, G. S., Bui, H., Clark, R. M., and Ramirez, R. A. (2023). Spider mite resistant maize lines, B75 and B96, maintain resistance under water-stress. J. Pest Sci. 96, 1117–1132. doi: 10.1007/s10340-022-01584-3
Gong, Z. T., Zhang, G. L., and Chen, Z. C. (2007). Pedogenesis and Soil Taxonomy. Beijing: Science Press.
Holmstrup, M., Sørensen, J. G., Dai, W., Krogh, P. H., Schmelz, R. M., and Slotsbo, S. (2022). Analysis of heat and cold tolerance of a freeze-tolerant soil invertebrate distributed from temperate to Arctic regions: evidence of selection for extreme cold tolerance. J. Comp. Physiol. B 192, 435–445. doi: 10.1007/s00360-022-01433-w
Iglesias, R., Palacios-Vargas, J. G., and Castaño-Meneses, G. (2019). Comparison of oribatid mites from agricultural soils with contrasting irrigation types in Hidalgo State, Mexico: a case study. Rev. Mex. Biodivers. 90, e902780. doi: 10.22201/ib.20078706e.2019.90.2780
Jakšová, P., Luptáčik, P., Miklisov,á, D., Horváthov,á, F., and Hlavatá, H. (2020). Oribatida (Acari) communities in arable soils formed under waterlogged conditions: the influence of a soil moisture gradient. Biologia. 75, 243–257. doi: 10.2478/s11756-019-00291-2
Jaloszynsk, P. (2012). Adults of European ant-like stone beetles (Coleoptera: Staphylinidae: Scydmaeninae) Scydmaenus tarsatus Müller and Kunze and Scydmaenus hellwigii (Herbst) prey on soft-bodied arthropods. Entomol. Sci. 15, 35–41. doi: 10.1111/j.1479-8298.2011.00479.x
Jandricic, S., Scott-Dupree, C. D., Broadbent, A. B., Harris, C. R., and Murphy, G. (2006). Compatibility of Atheta coriaria with other biological control agents and reduced-risk insecticides used in greenhouse floriculture integrated pest management programs for fungus gnats. Can. Entomol. 138, 712–722. doi: 10.4039/n05-106
Jiang, L., Geng, Y., Wang, L., Peng, Y., Liu, H., Xu, Y., et al. (2019). Acute risks of pesticide residues in greenhouse tomato and cucumber field soil to earthworms. J. Agro-Environ. Sci. 38, 2278–2286. doi: 10.11654/jaes.2019-0275
Karagkouni, M., Sfenthourakis, S., Feldman, A., and Meiri, S. (2016). Biogeography of body size in terrestrial isopods (Crustacea: Oniscidea). J. Zool. Syst. Evol. Res. 54, 182–188. doi: 10.1111/jzs.12125
Kassambara, A., and Mundt, F. (2020). Factoextra: Extract and Visualize the Results of Multivariate Data Analyses. R package version 1.0.7. Available online at: https://cran.csiro.au/web/packages/factoextra/index.html (accessed May 16, 2023).
Knox, M. A., Wall, D. H., Virginia, R. A., Vandegehuchte, M. L., Gil, I. S., and Adams, B. J. (2016). Impact of diurnal freeze–thaw cycles on the soil nematode Scottnema lindsayae in Taylor Valley, Antarctica. Polar Biol. 39, 583–592. doi: 10.1007/s00300-015-1809-6
Krantz, G. W., and Walter, D. W. (2009). A manual of Acarology. Lubbock, TX: Texas Tech University Press.
Lartey, R. T., Curl, E. A., and Peterson, C. M. (1994). Interactions of mycophagous collembola and biological control fungi in the suppression of Rhizoctonia solani. Soil Biol. Biochem. 26, 81–88. doi: 10.1016/0038-0717(94)90198-8
Li, Y., Cloyd, R. A., Bello, N. M., and Dara, S. (2019). Effect of integrating the entomopathogenic fungus (Hypocreales: Cordycipitaceae) and the rove beetle (Coleoptera: Staphylinidae) in suppressing western flower thrips (Thysanoptera: Thripidae) populations under greenhouse conditions. J. Econ. Entomol. 112, 2085–2093. doi: 10.1093/jee/toz132
Lian, Y., Wu, Y., Tang, W., Shen, Q., Hu, Y., Wang, J., et al. (2018). Investigation and suggestions on the application of pesticide and chemical fertilizer in strawberry cultivation in Ningbo City. Zhejiang Agr. Sci. 59, 1881–1885. doi: 10.16178/j.issn.0528-9017.20181047
Lima, D. C. B., Costa, A. A. V., and Silva, F. S. (2015). Abundance and night hourly dispersal of the vesicating beetles of the Genus Paederus (Coleoptera: Staphylinidae) attracted to fluorescent, incandescent, and black light sources in the Brazilian Savanna. J. Med. Entomol. 52, 50–55. doi: 10.1093/jme/tju006
Lindo, Z. (2015). Warming favours small-bodied organisms through enhanced reproduction and compositional shifts in belowground systems. Soil Biolo. Biochem. 91, 271–278. doi: 10.1016/j.soilbio.2015.09.003
Liu, H., Yin, C., Gao, Z., and Hou, L. (2021). Evaluation of cucumber yield, economic benefit and water productivity under different soil matric potentials in solar greenhouses in North China. Agr. Water Manage. 243:106442. doi: 10.1016/j.agwat.2020.106442
Liu, T., Guo, R., Ran, W., Whalen, J. K., and Li, H. (2015). Body size is a sensitive trait-based indicator of soil nematode community response to fertilization in rice and wheat agroecosystems. Soil Biolo. Biochem. 88, 275–281. doi: 10.1016/j.soilbio.2015.05.027
López-Felices, B., Aznar-Sánchez, J. A., Velasco-Muñoz, J. F., and Mesa-Vázquez, E. (2022). Installation of hedgerows around greenhouses to encourage biological pest control: farmers' perspectives from Southeast Spain. J. Environ. Manage. 323, 116210. doi: 10.1016/j.jenvman.2022.116210
Lu, R. K. (2000). Analytical Method of Soil and Agricultural Chemistry. Beijing: Agricultural Science and Technology Press.
Madzaric, S., Ceglie, F. G., Depalo, L., Bitar, L. A., Mimiola, G., Tittarelli, F., et al. (2017). Cover crops as a part of organic greenhouse rotation: implication on soil arthropods dynamics. Acta Horticulturae 1170, 1063–1069. doi: 10.17660/ActaHortic.2017.1170.137
Minor, M. A., Babenko, A. B., Ermilov, S. G., Khaustov, A. A., and Makarova, O. L. (2016). Effects of cushion plants on high-altitude soil microarthropod communities: cushions increase abundance and diversity of mites (Acari), but not springtails (Collembola). Arct. Antarct Alp. Res. 48, 485–500. doi: 10.1657/AAAR0015-064
Moretti, M., Dias, A. T. C., Bello, F., Altermatt, F., Chown, S. L., Azcarate, F. M., et al. (2017). Handbook of protocols for standardized measurement of terrestrial invertebrate functional traits. Funct. Ecol. 31, 558–567. doi: 10.1111/1365-2435.12776
Ningbo Climate Center (2022). Ningbo Annual Climate Bulletin 2022. Available online at: https://www.qx121.com/weather/rainImg/910.pdf (accessed July 3, 2023).
Ningbo Climate Center (2023). Ningbo Climate Bulletin, January 2023. Available online at: https://www.qx121.com/weather/rainImg/975.pdf (accessed July 1, 2023).
Niu, X., Cheng, Y. F., Feng, X. P., Sun, F., and Gu, Y. F. (2022). Effects of fertilizer and weed species richness on soil nematode community in a microcosm field experiment. Soil Ecol. Lett. 5, 151–168. doi: 10.1007/s42832-021-0123-1
Niva, C. C., Cezar, R. M., Fonseca, P. M., Zagatto, M. R. G., Oliveira, E. M., Bush, E. F., et al. (2015). Enchytraeid abundance in Araucaria Mixed Forest determined by cold and hot wet extraction. Braz. J. Biol. 75, S169–S175. doi: 10.1590/1519-6984.08414
Organization WWM (2020). Global climate Report - August 2020 | Atate of the Climate. Available online at: https://www.ncdc.noaa.gov/sotc/global/202008 (accessed June 30, 2023).
O'Rourke, M. E., Liebman, M., and Rice, M. E. (2008). Ground beetle (Coleoptera: Carabidae) assemblages in conventional and diversified crop rotation systems. Environ. Entomol. 37, 121–130. doi: 10.1603/0046-225X(2008)37[121:GBCCAI]2.0.CO;2
Palmer, M. (1994). Ecological factors associated with body size in populations of Macrothorax morbillosus (F.) (Carabidae, Coleoptera). Acta Oecol. 15, 689–699.
Pequeno, P. A. C. L., Franklin, E., Norton, R. A., and Morais, J. W. (2018). A tropical arthropod unravels local and global environmental dependence of seasonal temperature–size response. Biol. Letters 14, 20180125. doi: 10.1098/rsbl.2018.0125
Perumalsamy, K., Selvasundaram, R., Roobakkumar, A., Rahman, V. J., Babu, A., and Muraleedharan, N. N. (2009). Life table and predation of Oligota pygmaea (Coleoptera: Staphylinidae) a major predator of the red spider mite, Oligonychus coffeae (Acarina: Tetranychidae) infesting tea. Biol. Control 51, 96–101. doi: 10.1016/j.biocontrol.2009.07.002
Porhajašová, J. I., Babošová, M., Noskovič, J., and Ondrišík, P. (2018). Long-term developments and biodiversity in carabid and staphylinid (Coleoptera: Carabidae and staphylinidae) fauna during the application of organic fertilizers under agroecosystem conditions. Pol. J. Environ. Stud. 27, 2229–2235. doi: 10.15244/pjoes/77072
Postma-Blaauw, M. B., Goede, R. G. M., Bloem, J., Faber, J. H., and Brussaard, L. (2010). Soil biota community structure and abundance under agricultural intensification and extensification. Ecology. 91, 460–473. doi: 10.1890/09-0666.1
Quénéhervé, P., and Chotte, J. L. (1996). Distribution of nematodes in vertisol aggregates under a permanent pasture in Martinique. Appl. Soil Ecol. 4, 193–200. doi: 10.1016/S0929-1393(96)00118-7
R Core Team (2022). R: A Language and Environment for Statistical Computing. Vienna: R Foundation for Statistical Computing. Available online at: https://www.R-project.org/ (accessed May 7, 2023).
Read, D. C. (1962). Notes on the life history of Aleochara bilineata (Gyll.) (Coleoptera: Staphylinidae), and on its potential value as a control agent for the cabbage maggot, Hylemya brassicae (Bouch) (Diptera: Anthomyiidae). Can. Entomol. 94, 417–424. doi: 10.4039/Ent94417-4
Ristyadi, D., He, X., and Wang, Q. (2021). Response to thermal environment in Tetranychus ludeni (Acari: Tetranychidae). Syst. Appl. Acarol. 26, 942–953. doi: 10.11158/saa.26.5.9
Rivero, R., Mittler, R., Blumwald, E., and Zandalinas, S. (2021). Developing climate-resilient crops: improving plant tolerance to stress combination. Plant J. 109, 373–389. doi: 10.1111/tpj.15483
Schmidt, F. A., Schoereder, J. H., and Caetano, M. D. N. (2017). Ant assemblage and morphological traits differ in response to soil compaction. Insects Sociaux 64, 219–225. doi: 10.1007/s00040-016-0532-9
Schmidt, J. M., Pochubay, E. A., Tourtois, J., and Grieshop, M. J. (2017). The inherent complexity of soil and foliar predators for greenhouse biological control. Biol. Control 115, 46–54. doi: 10.1016/j.biocontrol.2017.09.008
Shiraishi, H., Enami, Y., and Okano, S. (2003). Folsomia hhidakana (Collembola) prevents damping-off disease in cabbage and Chinese cabbage by Rhizoctonia solani. Pedobiologia 47, 33–38. doi: 10.1078/0031-4056-00167
Sinka, M., Jones, T. H., and Hartley, S. E. (2007). The indirect effect of above-ground herbivory on collembola populations is not mediated by changes in soil water content. Appl. Soil Ecol. 36, 92–99. doi: 10.1016/j.apsoil.2006.12.004
Sjursen, H., Michelsen, A., and Holmstrup, M. (2005). Effects of freeze-thaw cycles on microarthropods and nutrient availability in a sub-Arctic soil. Appl. Soil Ecol. 28, 79–93. doi: 10.1016/j.apsoil.2004.06.003
Straalen, N. V., and Rijninks, P. (1982). Efficiency of Tullgren apparatus with respect to interpreting seasonal changes in age structure of soil arthropod populations. Pedobiologia 244, 197–209. doi: 10.1016/S0031-4056(23)05882-1
Thakur, M. P., Sigurð*sson, B. D., Sigurð*sson, P., and Holmstrup, M. (2023). Warming shifts the biomass distribution of soil microarthropod communities. Soil Biol. Biochem. 177, 108894. doi: 10.1016/j.soilbio.2022.108894
Thao, L. Q., Cuong, D. D., Anh, N. T., Minh, N., and Tam, N. D. (2022). Pest early detection in greenhouse using machine learning. Revue d'Intelligence Artificielle 36, 209–214. doi: 10.18280/ria.360204
Topp, W., and Smetana, A. (1998). Distributional pattern and development of the winter-active beetle Quedius pellax (Staphylinidae). Global Ecol. Biogeogr. Lett. 7, 189–195. doi: 10.2307/2997374
Twardowski, J. P., Hurej, M., and Gruss, I. (2016). Diversity and abundance of springtails (Hexapoda: Collembola) in soil under 90-year potato monoculture in relation to crop rotation. Arch. Agron. Soil Sci. 62, 1158–1168. doi: 10.1080/03650340.2015.1131270
Tyler, G. (2008). Differences in abundance, species richness, and body size of ground beetles (Coleoptera: Carabidae) between beech (Fagus sylvatica L.) forests on Podzol and Cambisol. Forest Ecol. Manag. 256, 2154–2159. doi: 10.1016/j.foreco.2008.08.006
Wan, L., Zhao, Y., Xia, L., Hu, J., Xue, T., Lv, H., et al. (2023). Assessing the environmental sustainability of different soil disinfestation methods used in solar greenhouse vegetable production systems. Sci. Total Environ. 885, 163962. doi: 10.1016/j.scitotenv.2023.163962
Wang, Q. (2008). Influence of plastic greenhouse on soil animal community. Shandong Agricultural Sciences 4, 80–83. doi: 10.14083/j.issn.1001-4942.2008.04.026
Wang, R., Sun, Q., and Chu, Y. (2010). Study on soil compaction of greenhouse caused by machine repaire in Ningxia. Xinjiang Agr. Sci. 47, 110–113. http://www.cnki.com.cn/Article/CJFDTOTAL-XJNX2010S2027.htm
Wang, S., Zhu, Z., Yang, L., Li, H., and Ge, B. (2023). Body size and weight of pill bugs (Armadillidium vulgare) vary between urban green space habitats. Animals 135, 857. doi: 10.3390/ani13050857
Wei, Q., Zhou, Y., Xiao, N., Huang, J., Xiao, H., and Hu, C. (2022). Effects of three typical grass cultivation patterns on the community structure of soil mites in rocky desertification control area, Guizhou, Chin. Environ. Res. Commun. 4, 054008. doi: 10.1088/2515-7620/ac6656
Wickham, H., Averick, J., Bryan, C. W., McGowan, L. D., François, R., Grolemund, G., et al. (2019). Welcome to the tidyverse. J. Open Source Softw. 4, 1686. doi: 10.21105/joss.01686
Xie, L., Slotsbo, S., and Holmstrup, M. (2023). Tolerance of high temperature and associated effects on reproduction in euedaphic Collembola. J. Therm. Biol. 113, 103439. doi: 10.1016/j.jtherbio.2022.103439
Xu, G. L., Kuster, T. M., Gunthardt-Goerg, M. S. G., Dobbertin, M., and Li, M. H. (2012). Seasonal exposure to drought and air warming affects soil Collembola and mites. PLoS ONE. 7, e43102. doi: 10.1371/journal.pone.0043102
Yin, R., Siebert, J., Eisenhauer, N., and Schadler, M. (2020). Climate change and intensive land use reduce soil animal biomass via dissimilar pathways. Rlife 9:e54749. doi: 10.7554/eLife.54749
Yin, W., Hu, S., Shen, Y., Ning, Y., Sun, X., Wu, J., et al. (1998). Pictorial Keys to Soil Animals of China. Beijing: Science Press.
Yuan, X., Zhang, Y., Fan, L., Wang, W., and Wu, Y. (2022). Temporal dynamics of antibiotic resistance genes in vegetable greenhouse soils following different manure applications. J. Soil Sci. Plant Nut. 22, 5144–5158. doi: 10.1007/s42729-022-00990-x
Zhang, P., Zhang, W., and Hu, S. (2023). Fungivorous nematode Aphelenchus avenae and collembola Hypogastrura perplexa alleviate damping-off disease caused by Pythium ultimum in tomato. Plant Soil 482, 175–189. doi: 10.1007/s11104-022-05680-2
Keywords: soil mite, soil Collembola, body length, body width, facility farmland, open farmland
Citation: Gao M, Jiang Y, Sun J, Lu T, Zheng Y, Lai J and Liu J (2023) Open farmland is a hotspot of soil fauna community around facility farmland during a cold wave event. Front. Sustain. Food Syst. 7:1254830. doi: 10.3389/fsufs.2023.1254830
Received: 07 July 2023; Accepted: 29 September 2023;
Published: 20 October 2023.
Edited by:
Diego Griffon, Central University of Venezuela, VenezuelaReviewed by:
Andrey S. Zaitsev, Senckenberg Museum of Natural History Görlitz, GermanyCopyright © 2023 Gao, Jiang, Sun, Lu, Zheng, Lai and Liu. This is an open-access article distributed under the terms of the Creative Commons Attribution License (CC BY). The use, distribution or reproduction in other forums is permitted, provided the original author(s) and the copyright owner(s) are credited and that the original publication in this journal is cited, in accordance with accepted academic practice. No use, distribution or reproduction is permitted which does not comply with these terms.
*Correspondence: Jinwen Liu, MTU4NDMxNDkwNjZAMTYzLmNvbQ==
Disclaimer: All claims expressed in this article are solely those of the authors and do not necessarily represent those of their affiliated organizations, or those of the publisher, the editors and the reviewers. Any product that may be evaluated in this article or claim that may be made by its manufacturer is not guaranteed or endorsed by the publisher.
Research integrity at Frontiers
Learn more about the work of our research integrity team to safeguard the quality of each article we publish.