- 1Department of Aquatic Animal Health and Welfare, Norwegian Veterinary Institute, Oslo, Norway
- 2Aquaculture and Fisheries Group, Wageningen University and Research, Wageningen, Netherlands
- 3Aquatic Ecology and Water Quality Management Group, Wageningen University and Research, Wageningen, Netherlands
- 4Fisheries Commission, Ministry of Fisheries and Aquaculture Development, Accra, Ghana
Unusual fish mortalities in aquaculture threaten global food security and carry significant socio-economic burdens. In 2018, Nile tilapia (Oreochromis niloticus) suffered unusual patterns of mortalities, attributed to disease-causing agents in Lake Volta cage aquaculture. In recent times, disease investigations have shifted from single to consideration of multiple factors to understand the puzzling range of causal risk factors. This study therefore aimed at expanding on tilapia mortality risk factors, while documenting fish health and Lake Volta management practices for sustainable aquaculture. We interviewed relevant aquaculture stakeholders operating on Lake Volta and conducted thematic analysis on their responses to map out mortality risk factors and management practices. The identified risk factors were conceptualized in established models of causation web and Social-Ecological System to explain the practical significance of the findings. The results showed that the risk factors of tilapia mortalities are a combination of pathogens and non-infectious factors mediated by weak law enforcement. The results further suggested mortality reinforcing mechanisms through the horizontal transmission of pathogens, namely, Streptococcus agalactiae and Infectious Spleen and Kidney Necrosis Virus. Moreover, the recognition of weak enforcement as a possible factor reinforcing human activities is a non-infectious route that can be deleterious to fish health. Health management practices comprised phytotherapy, vaccination, heat shock treatment, biological controls, and best husbandry practices. Lake management involves creating a waterfront buffer of 85.34 m, surveillance, and executing the framework guiding aquaculture development on the Lake. The findings are suggestive of complementary quantitative studies that augment the qualitative evidence herein. Such follow up studies can disclose precise mortality risk factors to inform policy directives and effective remedial strategies that can secure fish and lake health.
1. Introduction
Lake Volta is a man-made system primarily purposed for generating hydroelectric power in Ghana. Over the years, the Lake evolved to support other commercial activities among which capture fisheries and aquaculture are dominant. The Lake has become the hub of cage aquaculture in Ghana, producing Nile tilapia (Oreochromis niloticus), African catfish (Clarias gariepinus), and African arowana (Heterotis niloticus) (Béné, 2007; Rurangwa et al., 2015). Nile tilapia accounts for 80% of Ghana’s total fish production, while African catfish and African arowana together represent 20% (Fisheries Commission, 2021). In 2016, Ghana produced 47,174 MT of fish from 8,415 floating cages, majority of which were concentrated on Lake Volta (Ragasa et al., 2018). Currently, 1,200 of these cages were reportedly abandoned on the Lake, due to fish mortalities driven by poor water quality and disease outbreaks (Mantey et al., 2020). In mid-October 2018, disease-induced mortalities in cage-farmed tilapia significantly hampered the species’ contributions to total fish production. Two pathogens, namely Streptococcus agalactiae and Infectious Spleen and Kidney Necrosis Virus (ISKNV), have been introduced through imports of live biological materials and incriminated in these mortalities (Verner-Jeffreys et al., 2018; Ramírez-Paredes et al., 2021). These pathogens are currently prevalent and are occurring in coinfections to cause diseases, a finding consistent with gross pathologies in cage-farmed tilapia examined on the Lake (Ramírez-Paredes et al., 2021). While S. agalactiae and ISKNV are recognized as economically important fish pathogens in Lake Volta, it remains uncertain whether other risks factors from the Lake Environment and fish farms contribute to an increased likelihood of fish diseases and mortalities.
During the early 20th century, diseases occurring in animals and humans were often attributed to single agents (e.g., pathogens) (Barreto, 2005). However, the focus of disease investigation has shifted from singular agents to considering the involvement of multiple agents. The shift started in 1960 with the development of the web of causation model, which allowed rethinking the potential causalities or risks factors in contracting diseases (MacMahon et al., 1960). Moving forward, the web of causation has been applied in mapping the potential diseases risk factors and examining the interrelationship among risk factors to design effective interventions (MacMahon and Pugh, 1970; Joffe et al., 2012). The causation web provided the first evidence of lung cancer’s multiple risks, ranging from tobacco smoking to environmental, behavioral, and hereditary factors. The environmental factors include exposure to chemicals such as arsenic or asbestos and unintentional inhalation of smoke from burning tobacco products (Pope et al., 1995). The behavioral factors are characterized by people’s attitude of excessively smoking tobacco, while hereditary factors entail the transmission of disease variants to offspring (Fichtenberg and Glantz, 2002; Benusiglio et al., 2021). By adopting the web of causation, this study wants to broaden the understanding of disease and mortality risk factors of cage-farmed tilapia to move beyond the focus solely on pathogens. The primary aim of this study therefore is to leverage on the causation web model to elucidate the practical implications of risk factors while substantiating the presence of multiple contributing factors to Nile tilapia diseases and mortalities.
Streptococcus agalactiae and ISKNV pose threats to Nile tilapia throughout the production chain in Lake Volta. For instance, tilapia hatcheries sourcing water from Lake Volta experience a loss of >50% fry due to ISKNV infections (Ramírez-Paredes et al., 2021). Likewise, S. agalactiae infections cause 40–70% tilapia mortalities during the grow-out phases in submerged cages (Verner-Jeffreys et al., 2018). There has been no study conducted in Lake Volta to explain the underlying mechanisms driving consistent tilapia mortalities apart from the wide attribution to pathogenic risks. Reinforcing mechanism is a conceptual model within the broader social ecological systems (SES) that drives growth or decline processes (Downing et al., 2014). Growth processes could involve increased chemical use in agro farms at a Lake front thereby producing increase nutrient loading through surface runoffs. Increased nutrient loading causes algal blooms in the aquatic ecosystem. High algae blooms damages fish gills and reduce oxygen uptake with a resultant fish population decline, an example of a decline process.
This study conceptualize the SES model to study reinforcing mechanisms for tilapia diseases and mortalities in Lake Volta. Disease and mortality risk factors must be managed for a sustainable aquaculture industry. There is however, limited information on management practices initiated by fish farmers and regulators to counteract risk factors associated with diseases and mortalities in the Lake. Hence, the secondary aim of this study is to ascertain reinforcing mechanisms of Nile tilapia diseases and mortalities and document management practices initiated by stakeholders to attenuate the risk factors. Qualitative interviews to fish farmers and regulatory institutions managing aquaculture and lake resources were used to respond to the research aims. Studies on SES entail qualitative and quantitative modeling whereby the latter is dependent on the type of data, their availability or the possibility to collect the data (Haraldsson et al., 2020). The qualitative approach bridges these barriers by allowing the incorporation of variables that are difficult to measure and to sacrifice precision for generality and realism (Levins, 1966).
2. Materials and methods
2.1. Conceptualization
Risk factors are broadly categorized into environmental, behavioral, physiological, genetic, and demographic components (Virolainen et al., 2022). Building upon this background information on risk factors, we examined the drivers of cage-farmed tilapia mortalities by looking at risk factors influencing, shaping, or bringing about mortalities (Hammersley, 2012).
Risk factors are intricately related hence studying their relationship is essential in understanding their contributions to a particular outcome (Vial et al., 2020). The concept developed by MacMahon et al. (1960) on lung cancer has been adapted to study the relationships among risk factors and their contributions to cage-farmed tilapia mortalities in Lake Volta. The risk factors identified in this study served as foundational elements in building the causation web. Some controversies have been raised on using qualitative research to infer on causal relationships. Therefore, Maxwell (2019) recommended literature to complement qualitative data in giving causal explanations. This study complemented the qualitative data with relevant literature to support how risk factors are related or influence each other in establishing mortalities, as exemplified by Odhiambo et al. (2020).
Risk factors carry wider ecological implications and disrupt the overall balance and functioning of ecosystems (Myers et al., 2013). A single risk factor can trigger a cascade of changes in an ecosystem. The cascade effects of risk factors are modeled in a SES to demonstrate their far-reaching effects across social and ecological domains (Ann et al., 2006). The cascade effects of risk factors represented in a SES model can produce positive and negative feedbacks based on the relationships among the risk factors (Nassl and Löffler, 2015). Positive feedback relationships among risk factors represent reinforcing mechanisms that carry the potential to destabilize the overall balance and functioning of ecosystems (Levins, 1974; Downing et al., 2014). A negative feedback loop is a stabilizing mechanism capable of limiting the disturbances of risk factors to preserve the balance and functioning of ecosystems (Levins, 1974; Downing et al., 2014). In light of this, we will adopt the concept of positive and negative feedback within the larger SES model to establish how cage-farmed tilapia mortalities and diseases have been uninterrupted in Lake Volta.
2.2. Study area
Lake Volta has a mean depth of 19 m, covers 3.6% of Ghana’s surface area, and lies between longitude 1° 30° W and 0° 20° E and latitude 6° 15´ N and 9° 10´ N. Most cage farms on the Lake are found in the Asuogyaman District of the Eastern Region, with clusters of developing farms in the South Dayi District - Volta Region (Kassam, 2014). This subsequently informed the selection of Eastern and Volta region, and their respective districts for the study (Figure 1).
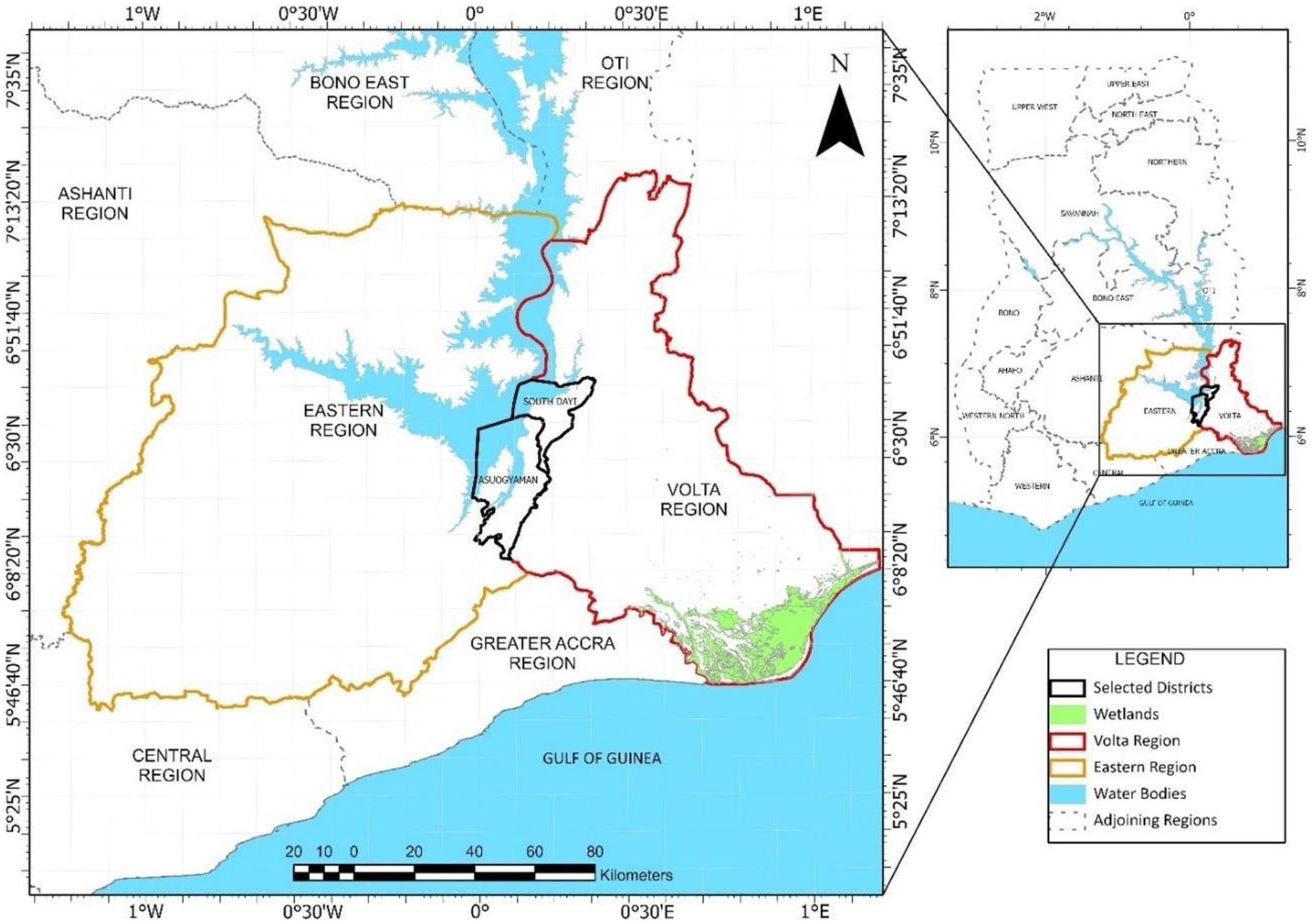
Figure 1. Map of Ghana showing Eastern region (Asuogyaman district) and Volta region (South Dayi district).
2.3. Description of participants
This study employed a participatory research approach conducted in two stages: (1) online interviews from March–July 2021 and (2) a stakeholder workshop in May 2022. The interviews and stakeholder workshop targeted fish farmers, regulators, and researchers, as they represent the key stakeholders with a vested interest in Lake Volta aquaculture. The participants in the online interviews were selected purposively from the two study districts. The selection was based on long-term experiences and knowledge in Lake Volta cage aquaculture and the related fish health challenges. The interviews were conducted online due to Covid-19 traveling restrictions. In total, we encountered a sample size of 26 participants (Figure 2) determined through data saturation (Coenen et al., 2012). Based on the methodology of Coenen et al. (2012), we reached data saturation after three consecutive interviews when no new themes on risk factors and management practices were realized. Of the 26 interview participants, 15 are cage farmers, eight regulators, and three researchers. The stakeholder workshops (n = 40) validated the perspectives of the 26 interviewees. Of the 40 participants, 6 were cage farmers, 32 regulators, and 2 researchers (Figure 2).
The participating fish farms were small (<50 tonnes), medium (50–100 tonnes), and large-scale (>100 tonnes) tilapia producers. The regulator institutions were Fisheries Commission (FC), Water Resource Commission (WRC), Volta River Authority (VRA), Environmental Protection Agency (EPA), and District Assembly (DA). These institutions were selected because of their mandate to regulate the Lake environment, cage aquaculture activities, and other economic activities along the lake. The researchers were represented by the Aquaculture Research and Development Center (ARDEC), including other aquaculture education and research institutions with an interest in cage aquaculture on the Lake.
2.4. Online interviews
The participants completed an ethical clearance form prior to the online interviews in Microsoft Teams. Data was collected through open questions categorized in four themes: (a) farm information (b) risk factors of tilapia diseases and mortalities, (c) fish health management practices and (d) Lake Volta management practices. Open-ended questions enabled the provision of broad information and allowed the use of follow-up questions to clarify responses from interviewees. To ensure confidentiality, personal information regarding farms and participants are withheld, while focusing on risk factors of fish mortalities/diseases and management practices. The digital recordings from the interviews were transcribed and complemented with handwritten notes for further processing.
2.5. Analyzing interview data
We conducted thematic analyzes through three stages to analyze interview data (Krippendorff, 2004; Creswell, 2014; Sarku et al., 2020). The thematic analysis was done in Atlas.ti to identify themes relevant to the study objectives. Firstly, the transcripts were analyzed by identifying responses to specific questions, realigning sentence structures, and clarifying sentence structures (Wolfinger, 2002; Dey, 2003). Secondly, we applied inductive coding to identify initial codes (Krippendorff, 2004; Creswell, 2014; Sarku et al., 2020). The initial codes are common responses in the narrations relating to (a) risk factors of tilapia diseases and mortalities, (b) fish health management practices, and (c) Lake Volta management practices. For example, we encountered initial codes such as ISKNV (virus), S. agalactiae (bacteria), agro-chemicals (herbicides and pesticides), overfeeding, and overstocking during the inductive coding of risk factors. Subsequently, the initial codes were grouped into various themes such as pathogens, water pollution, dietary factors, and husbandry factors. Thirdly, the themes identified were visualized graphically using the number of responses on each initial codes as a percentage of the total responses to each question.
2.6. Synthesizing interview data to construct causation web and SES model
We adapted the methodology of Scavarda et al. (2006) to construct the causation web from the interview data. Through an iterative consensus-driven process, we reflected on the interview narrations and coded data on diseases and mortality risk factors to identify factors that can be mapped to create the causation web. The mapping was done by examining how two or more disease/mortality risk factors can influence each other. For example, the informants revealed the continuous release of agro-chemical contaminants, including herbicides and pesticides from nearby farms into the Lake. This subsequently led to a reduction in water quality, which was consistent with past instances of fish mortality events, as described by the participants. This narration was evaluated by the study team using literature, leading to a consensus that chemical pollutants can degrade water quality to stress the fish and render them vulnerable to mortalities (Chinnadurai et al., 2022). These iterative consensus-driven processes aided in building an initial causation web for subsequent evaluation in an expert group workshop.
Similarly, the approach outlined by Hossain et al. (2020) was adopted to construct the SES model as an attempt, to ascertain the social and ecological implications of the diseases and mortality risk factors. This was done in four stages: (a) Identifying key disease and mortality risk factors in terms of social and ecological implications (b) Creating linkages among key risk factors in terms of their probability to influence diseases and mortalities (c) Determine the outcomes of the relationships among risk factors considering both positive and negative feedbacks, and (d) Analyze positive and negative feedback loops using the methodologies of Downing et al. (2014) and Cooper and Dearing (2019). By following the four stages, the study team first synthesized the responses to the open questions, which were telling to deduce social and ecological implications of the disease and mortality risk factors. For example, the informants went beyond merely mentioning risk factors like pathogens. They provided narratives detailing the pathway through which the pathogens were introduced, including the socio-economic and ecological consequences.
Literature information contributed to broadening the socio-economic and ecological components by incorporating spillover effects induced by the risk factors. For example, the informants revealed the practice of utilizing unprocessed tilapia cadavers as feed for catfish. Subsequently, literature information was consulted to validate this scenario in the form of cross-infections between tilapia and catfish (Wise et al., 2021). Secondly, through an iterative consensus-driven process, the study team created linkages among risk factors, considering their potential to influence diseases and mortalities. The linkages were also supported with literature information under the framework of social and ecological implications of the risk factors. Next, we determined the outcomes of the relationships among the disease and mortality risk factors by considering how increasing one risk factor could trigger an increase in the second or successive risk factors. If an increase in one risk factor causes an increase in the succeeding risk factor, the sign is positive (+). On the other hand, if an increase in one risk factor causes a decrease in the succeeding risk factor, the sign is negative (−).
Lastly, we conducted qualitative loop analyses to identify feedback loops in the SES model. A loop is a pathway through a number of system risk factors (or elements). When initiating with any given element, the pathway will eventually circle back to the initial element, thereby creating a loop in the process. The individual signs (+/−) between connected elements in a loop cancel each other to arrive at an overall positive or negative sign (e.g., overall + = − + − +). An overall positive sign in a closed loop is a positive feedback loop with the potential of reinforcing disturbances that can change the equilibrium state of a system. An overall negative sign is a negative feedback loop which can self-regulate disturbances to keep systems in their equilibrium state (Levins, 1974; Downing et al., 2014). By adopting the concepts of negative and positive feedback loops, we determined the reinforcing mechanisms of fish diseases/mortalities and identified stabilizing mechanisms for adoption in management practices.
2.7. Validating causation web and SES model
In the stakeholder workshop, the study team presented the research aims and the risk factors gathered from the online interviews to create the draft causation web and SES model. Thereafter, expert discussions were initiated to examine the strengths and interrelationships among the risk factors in both the causation web and SES model. The expert discussions aided the study team to refine the linkages between risk factors, which were created as a draft from the interview narratives. This helped in finalizing the causation web and SES model. Additionally, the final SES model produced new feedback loops that were assessed in a consensus-driven manner during the workshop (Figure 2).
Figure 2 encapsulates the step-by-step procedures followed in creating and validating the causation web and SES model.
3. Results
3.1. Risk factors of tilapia diseases and mortalities
The results of the study indicate that water pollution in the lake was the highest risk factor of tilapia diseases/mortalities at 34.6% followed by pathogens (S. agalactiae and ISKNV) at 30.8% (Figure 3). Water pollution is caused by human-mediated pollutants from mining sand and minerals, aquaculture waste, wood processing, agrochemicals, and domestic waste (Figure 4). Weak enforcement drives fish mortalities through uncontrolled human activities as the regulations and laws governing aquaculture and lake users are poorly implemented (Figure 4). The responses during the interviews highlighted enforcement as an important confounding variable. Although complex, enforcement plays a major role in influencing the pollution of the Lake. Moreover, weak enforcement also facilitated the importation of infected live fish and eggs that were banned in Ghana (Figure 4). The fish farmers do not have good physical structures to contain the infected live fish and eggs, resulting in the transfer of exotic pathogens, and inbreeding among imported tilapia and the local tilapia strain for aquaculture in Ghana. Furthermore, the occurrence of escapees in aquaculture facilitates the movement of fish from the cages into the wild environment, leading to admixed fish populations in the lake and potential gene pollution of wild tilapia population (Figure 4). Gene pollution implies a loss of genetic integrity arising from unregulated breeding activities among exotic and local tilapia strains. Following this, pathogen load and gene pollution, along with stress from water pollution can cause impaired fish immunity to induce fish mortalities. Furthermore, husbandry and dietary factors influencing fish diseases/mortalities were recorded at 15.4 and 7.7%, respectively, (Figure 3). In the causal web, husbandry and dietary factors were collectively termed husbandry practices. These practices encompass the utilization of dead fish and rancid feed as food sources, overfeeding, overstocking, and the manner in which fish are handled (Figure 4). The respondents perceived these husbandry practices as factors contributing to fish stress, which in turn could compromise fish immunity, leading to diseases and mortalities (Figure 4). A fraction of the stakeholders (11.5%) who gave “no response” upon follow up questions, were oblivious to risk factors of tilapia diseases and mortalities (Figure 3).
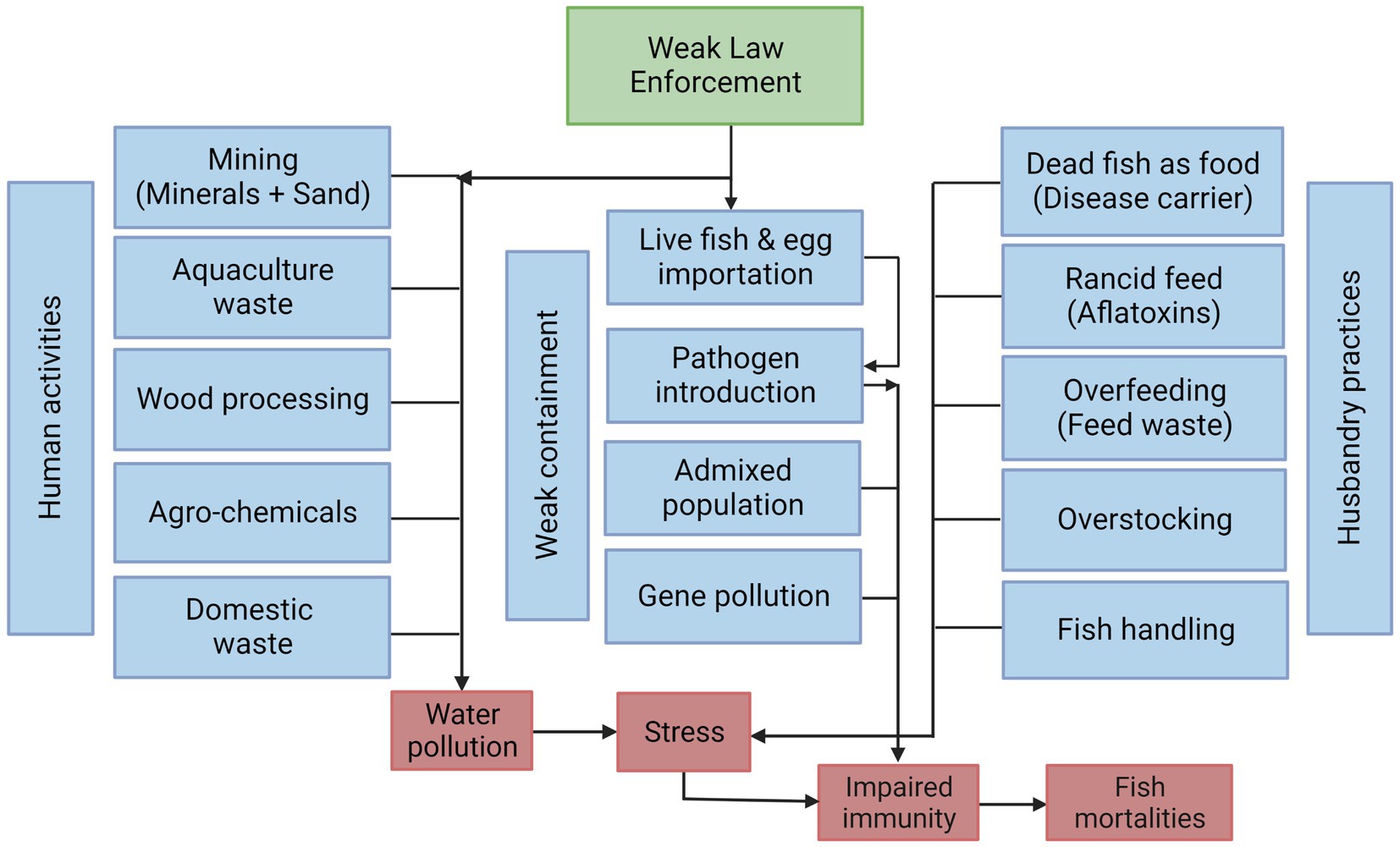
Figure 4. Causation web of Nile tilapia diseases and mortalities in Lake Volta cage aquaculture. The causal web was created with BioRender.com.
3.2. Social and ecological system
The SES model encompassed Lake Volta cage aquaculture including a snapshot of land-based activities. The land-based activities were included in the model, because of their influence on overall fish health status in Ghana’s aquaculture. The risk factors constituting the model were assigned IDs (Table 1). The social components of the SES model include disease and mortality risk factors such as enforcement, human activities, and husbandry practices. Conversely, the ecological components include biological fitness, fish stress, genetic diversity, and cross-infection.
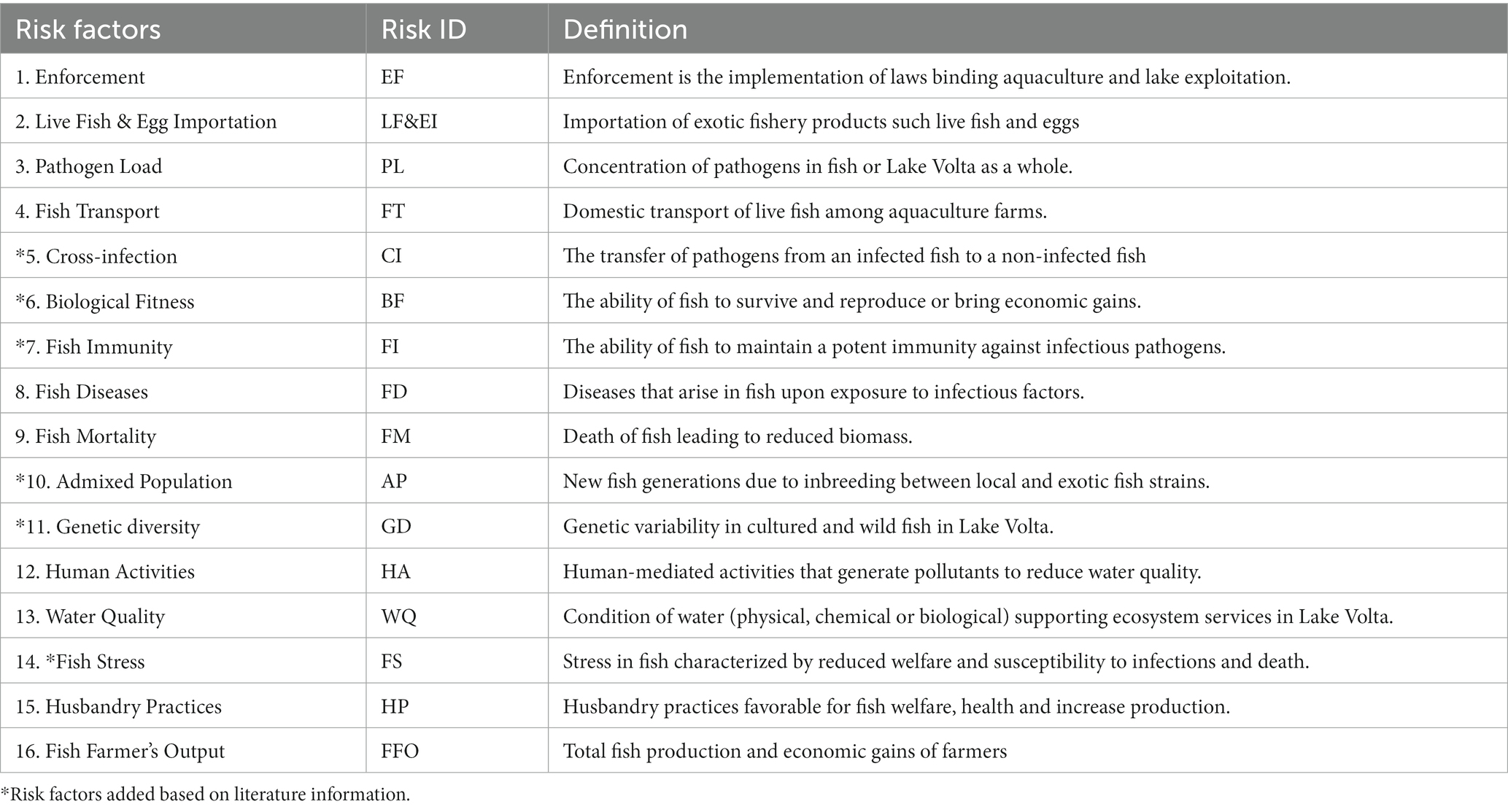
Table 1. The risk factors elicited from interviews served as inputs for the Social-Ecological System (SES) model.
The SES model is driven externally by enforcement (EF), which leads to multiple pathways of tilapia diseases and mortalities in Lake Volta. The multiple pathways consist of elements leading to decreased water quality in the lake, reduced fish immunity, diseases and mortalities. The interaction between two elements were assessed independently by considering their effect(s) on the succeeding element if the preceding element increases. For instance, EF is governance structure regulating aquaculture activities and lake development. Hence, an increase in EF decreased live fish and importation (LF&EI) and decreased human activities (HA) around the lake (Figure 5). In practice, high enforcement will lead to no LF&EI nor unacceptable human practices (identified by blue arrows). However, high LF&EI increased pathogen load (PL) in the lake and fish, shown with a positive sign (identified by green arrow) as a growth process. From the interview narratives, LF&EI was driven by the perceived underperforming local tilapia seeds compared to the purportedly superior performing foreign seeds for improved fish production. The SES model accounted for fish production as Fish Farmer’s Output (FFO). It became evident from the narratives that FFO is dependent on farmers’ husbandry practices (HP). Additionally, farmers rely on the authorities to enforce regulations binding sustainable Lake exploitation, including regulations on ban of LF&EI to have high FFO. The enforcement of these regulations is however low, thereby reducing FFO through imported diseases and deteriorated water quality. In consequence, farmers have lost trust in the regulatory authorities and are antagonistic to regulations being enforced. This informed the linkages created to unite FFO with HP, LF&EI, and EF. We emphasize that some elements in the model were added using literature information. For example, the inclusion of biological fitness (BF) and fish stress (FS) were informed by literature. Increased pathogen load in the aquatic environment decreases fish biological fitness (e.g., immune potential) by lowering survival and reproduction rates (Walker and Winton, 2010; Iregui et al., 2014). Through literature, we also accounted for genetic impacts of interbreeding between imported and local strains denoted as Genetic Diversity (GD) in the model (Anane-Taabeah et al., 2019).
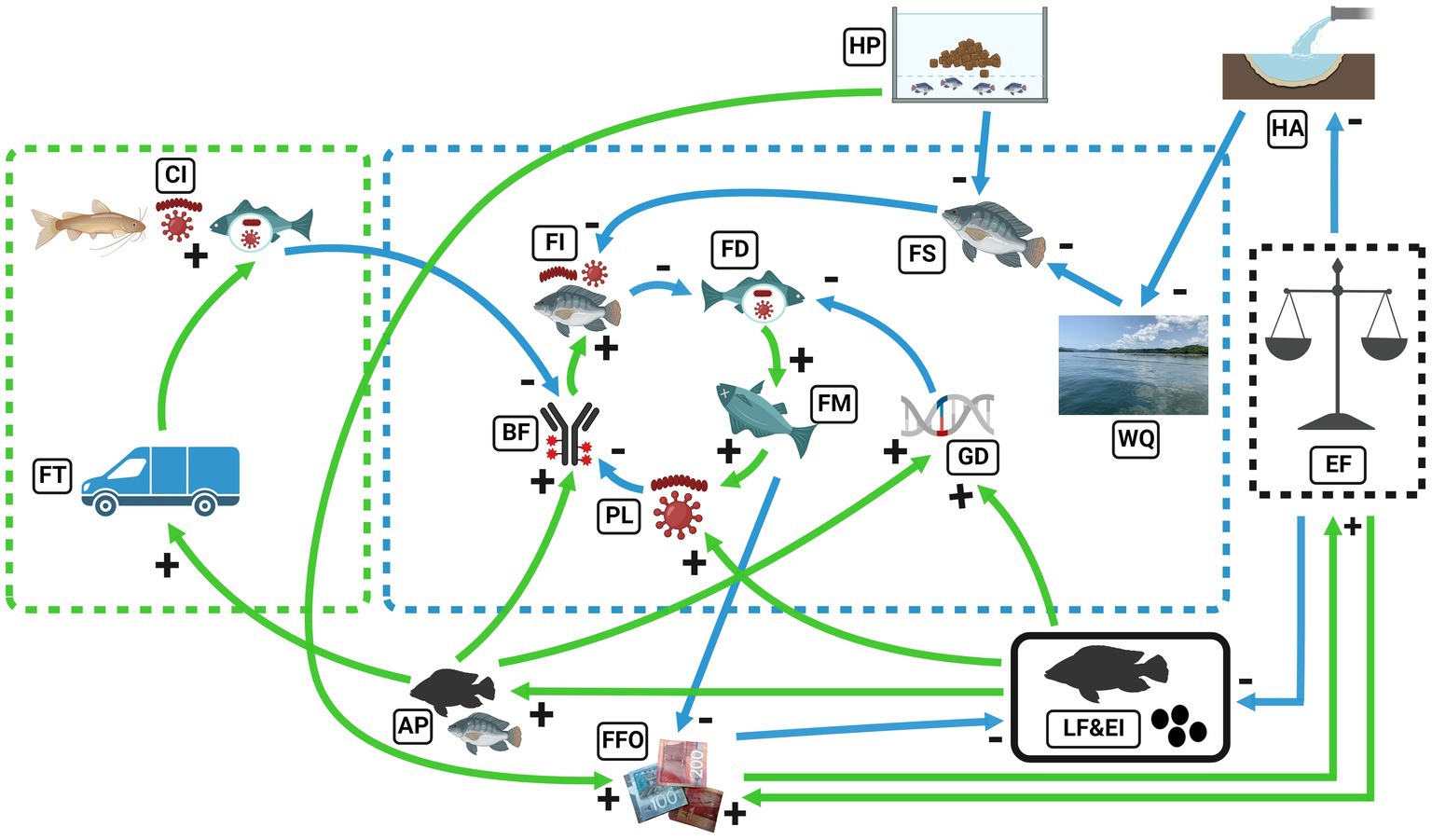
Figure 5. Qualitative SES model of Lake Volta aquaculture and land-based activities. Blue dotted lines encompass aquaculture-ecological interactions in Lake Volta; green dotted lines represent land-based activities; blue continuous arrows represent reduction processes (− signs); and green continuous arrows represent growth processes (+ signs). EF, Enforcement; HA, Human Activities; WQ, Water Quality; HP, Husbandry Practices; FS, Fish Stress; FI, Fish Immunity; FD, Fish Diseases; FM, Fish Mortality; PL, Pathogen Load; FFO, Fish Farmer’s Output; LF&EI, Live Fish and Egg Importation; AP, Admixed Population; GD, Genetic Diversity; FT, Fish Transport, CI, Cross-Infection and BF, Biological Fitness. The SES image was created with BioRender.com. Additional image citation: (WQ- Duodu et al., 2022).
3.3. Loop analyzes of the qualitative SES model
The SES model encompassed sixteen (16) feedback loops representing Lake Volta aquaculture and land-based activities (Figure 5). The self-reinforcing loops and self-regulating loops are, respectively, eight in number (Table 2). Redundant feedback loops were excluded because they yielded the same feedback signs (+/−) without additional information. For instance, LF&EI and HA are key findings, but we did not include separate loops for these variables. This is because LF&EI and HA are embedded in the major feedback processes driven by enforcement. We included Pathogen Load (PL) because it has a direct influence on fish diseases and mortalities. Moreover, Fish Farmer’s Output (FFO) was included because it has an influence on enforcement of aquaculture laws. In this context, fish farmers will not obey regulations perceived to be limiting their outputs.
3.4. Management practices for fish and lake health
Table 3 is a summary of key responses from stakeholders during online interviews on the management practices for fish and Lake Health.
4. Discussion
Our findings consolidate and confirm the results of other studies regarding the risk factors of tilapia diseases and mortalities (Verner-Jeffreys et al., 2018; Ramírez-Paredes et al., 2021). The findings also hint at reinforcing mechanisms that warrant consideration in the design of management practices aimed at addressing fish health challenges in Lake Volta.
4.1. Risk factors of tilapia mortalities
This study categorizes the risk factors contributing to tilapia diseases and mortalities in Lake Volta aquaculture into two main groups. These are pathogens and non-infectious factors, attributable to inadequate law enforcement measures. The Aquaculture Regulations of Ghana (Aquaculture and Fisheries Regulations, 2020) is legally binding and must be enforced for responsible aquaculture operations. The regulations are poorly enforced resulting in the violation of the ban on importing live fish, exotic fish species, and eggs/gametes, except with permit from the Fisheries Commission. The opinion-based evidence in the study suggest that failed enforcement of the regulations allowed S. agalactiae and ISKNV to be introduced into Ghana’s aquaculture industry through imports of fishery products (Figure 4). These pathogens have been confirmed in Lake Volta for causing recurrent diseases and mortalities in cage farmed tilapia (Verner-Jeffreys et al., 2018; Ramírez-Paredes et al., 2021). Other infectious risk factors were revealed through the husbandry practices of using dead fish and rancid feed as feed sources in catfish and tilapia production, respectively (Figure 4). Catfish mortalities were recorded from using unprocessed tilapia cadaver as feed source. Reportedly, the cadavers employed as catfish feed were believed to be contaminated with S. agalactiae and ISKNV during tilapia production process. This narrative lacked substantial evidence as it was founded solely on participants’ opinions. However, fish cadaver carrying infectious factors is a source of pathogenic stress that can impair fish immunity and cause fish mortalities (Novoslavskij et al., 2016). Additionally, feeding tilapia with rancid feed increases the likelihood of introducing mycotoxins that can induce tilapia mortality. In fish, high concentrations of mycotoxins can lead to a myriad of effects, namely reduced weight, compromised immune system, and impaired liver function, ultimately leading to mortalities (Anater et al., 2016; Marijani et al., 2019).
Non-infectious risk factors that can influence the occurrence of tilapia diseases and mortalities have been identified as water pollution in the lake, and husbandry practices of improper handling, overfeeding, and overstocking (Figure 4). Interestingly, findings from a quantitative study suggested that the Lake has a fairly good chemical health status (Tay, 2021). However, the results from a qualitative study corroborate the findings in this study, as water quality was purported to have aggravated disease incidences in farmed fish on the Lake (Abarike et al., 2022). The results in this study thus suggest the need to formulate testable hypothesis on specific water quality contaminants, such as herbicides and pesticides, as disclosed by the participants. Information on quantifiable water quality contaminants in the Lake and their effects on fish health can guide the formulation of specific management policies and remedial measures. Nevertheless, Tay (2021) recommended the continuous monitoring of the Lake water quality by competent authorities to preserve it for human and animal use. Stress due to the husbandry practices of overstocking and improper handling can manifest into diseases and mortalities. This observation is consistent with the findings of Wanja et al. (2020), where the occurrence of diseases and tilapia mortalities were consistent with overstocking and improper handling. Overstocking and improper handling can lead to competition for space and injuries that can serve as entry points for opportunistic pathogens to establish diseases and mortalities. The husbandry practice of overfeeding does not directly result in fish mortalities. This notion is supported by Kamler et al. (2006), who reported no confirmed fish mortalities in a feeding trial with different feed concentrates in Trench fish. However, overfeeding can contribute to the accumulation of uneaten feed, excess nutrients and organic matter to deteriorate water quality. This in turn can cause stress, diseases, and mortalities in fish species (Riche et al., 2004; Kamler et al., 2006; Gao et al., 2015). The qualitative evidence gathered in this study suggests that the risk factors of tilapia diseases and mortalities are a combination of both pathogens and non-infectious factors.
4.2. Reinforcing mechanisms behind tilapia diseases and mortalities
Reinforcing mechanisms represent positive feedbacks that amplify negative changes in a system rather than reverse them. Such changes happen within stable systems when they encounter disturbances induced naturally or by human interventions. Natural disturbances are not limited to windstorms, landslides, droughts, and flooding. Human disturbances include among others, deforestation, pollution, and overpopulation (White and Pickett, 1985; Spagnuolo et al., 2009; Dornelas, 2010). These disturbances could be likened to the risk factors of tilapia diseases and mortalities in Lake Volta. In fact, mortality is an essential effect of disturbances in an ecosystem (Sousa, 1984). Within the framework of the SES model, we examined the feedback loops to identify positive feedback mechanisms that might be reinforcing the risk factors associated with tilapia diseases and mortalities.
Aquatic ecosystems like Lake Volta host a myriad of naturally occurring pathogens where aquatic life have adapted and coexisted with them over the years. The introduction of new pathogens can disturb the balance that have been established between fish and the in-situ microbial community. The introduction of new pathogens parallels the introduction of invasive species, both of which holds the potential of disturbing the stability of species communities and affect the balanced food web (Downing et al., 2014). The introduction of S. agalactiae and ISKNV serves as an evidence of invasive pathogens within Lake Volta, resulting in the establishment of a short and strong feedback loop (Loop 7; Table 2). Short and strong feedbacks are known for causing rapid ecosystem changes (Soto-Ortiz, 2015). The short feedback loop stemming from pathogen load may have reduced the biological fitness of farmed fish and compromised their immune potential, thereby making the fish susceptible to diseases and mortalities. Fish mortalities caused by pathogens in cage aquaculture pose the risk of shedding pathogens into the water body. According to Zhu et al. (2017), this process is a horizontal disease transmission pathway with the potential of re-infecting new fish. This study hints at the occurrence of horizontal pathogen transfers as a reinforcing mechanism, which can manifest through shedding of pathogens from dead tilapia into the water column. Ultimately, this could lead to re-infection of non-infected tilapia that are farmed on the Lake. Ayiku et al. (2022) confirmed the horizontal transfer of S. agalactiae and ISKNV among farmed tilapia in Lake Volta. This underscores how the Lake may be functioning as a pathogen reservoir, thereby reinforcing tilapia diseases and mortalities. Therefore, timely removal of fish cadaver in aquaculture becomes imperative.
The identified confounding variable of weak enforcement has the potential of reinforcing anthropogenic activities through non-infectious pathways that impact fish mortalities. The non-implementation of the laws and regulations binding sustainable lake could potentially contribute to anthropogenic wastes in the lake as a reinforcement of low water quality. Low water quality induced by built-up of anthropogenic wastes can persist once there is non-enforcement of laws binding lake users. Loop 3 exemplifies the role of weak enforcement in a positive feedback loop as it reinforces human activities to reduce water quality, cause fish stress, reduce immunity, establish diseases/mortalities, and reduces fish production (Table 2). As fish production reduces among farmers, a loss of trust in regulators occurs. This exacerbates the challenges of enforcement to ultimately reinforce the introduction of anthropogenic wastes into the Lake. The perceived loss of trust in regulators have been reported in Ghana’s aquaculture industry by Agyei (2022). Regulators are encouraged to partner with aquaculture stakeholders in their regulatory processes for efficient enforcement (Lebel et al., 2019). This can be applied in a comprehensive manner to all stakeholders engaged in the exploitation of lake resources to dampen reinforcing processes conflicting fish and lake health.
All the feedback loops involving Genetic Diversity (GD) and Admixed Population (AP) resulted in stabilizing feedbacks. Stabilizing feedback processes serve to limit disturbances to preserve equilibrium states (Downing et al., 2014). This suggests that GD and AP may be self-regulating the negative impacts of LF&EI to preserve genetic integrity and maintain fish health. From Table 2, GD and AP can stimulate fish biological fitness to reduce diseases and mortalities caused by LF&EI (Loop 9: GD➔FD; Loop 10: AP➔BF). In practice, intentional breeding programs as reported by Eszterbauer et al. (2015), should be implemented to deliberately select favorable alleles that can enhance the fish’s ability to withstand disease impacts. This way, genetic variabilities in tilapia through breeding programs can reduce disease-induced mortalities while increasing the growth rate of fish. Nevertheless, if fish disease-induced mortalities persist in the Lake, mortalities can become a self-regulating mechanism to depopulate the number of cage farmers. New fish farmers who are privy to the mortality risks of lake production will be compelled to pursue inland production in ponds and other closed systems. Fish farmer’s output which is dependent on good enforcement and husbandry practices takes a complicated pathway to produce both reinforcing and stabilizing feedbacks (loop 5 and 12, Table 2). This suggest that fish farmer’s output is intrinsically linked to other confounding variables to either reinforce fish mortalities or dampen risk factors of tilapia diseases and mortalities.
4.3. Management practices for fish and lake health
Small-scale farmers practice phytotherapy by grinding neem tree (Azadirachta indica) leaves with salt solution and mixing it with the feed. Fish farmers have trust in this practice because from experience it offers treatment and protection against S. agalactiae and ISKNV infections. Neem tree leaves can enhance immune responses in tilapia due to bioactive ingredients serving as antioxidant, anti-inflammatory, immunomodulatory, and apoptotic agents (Kapinga et al., 2018; Mondal et al., 2020; Sarkar et al., 2021). Heat shock treatment is also administered when tilapia (<5 g) is ISKNV positive. The optimum temperature of the fish is nonlethally manipulated by the farmers around ±10°C. This practice enhances tilapia immunity by producing heat shock proteins (Dini et al., 2006; Chen et al., 2014). Additionally, farmers use ducks as biological controls that feed on debris on the production net to enhance water circulation and improve dissolved oxygen. Using animals as biological controls demand precautionary measures because of their disease vector abilities. Moreover, farmers improve water quality by reducing feeding levels during disease outbreaks. This practice can reduce organic wastes and nitrogenous compounds to improve water quality, prevents fish stress, and maintains good immunity (Amirkolaie, 2011). Finally, farmers minimize fish stress through best husbandry practices such as reduce fish handling, and optimal stocking density combined with biosecurity measures. To reduce transport-related stress and minimize mortalities, farmers transport fish in smaller batches.
The Ghana Fisheries Commission launched vaccination exercises to control the infectious pathogens in tilapia. According to farmers, the vaccination exercise was partially successful. Fish must be vaccinated with a potent vaccine ahead of exposure to give sufficient time to develop immunity (Ayalew and Fufa, 2018). As reported by farmers, fish vaccination was carried out during an outbreak. This is an incorrect practice that will create skepticism to vaccination and give vaccination bad reputations. Depending on the vaccine type, boosters are usually recommended for better protection (Tobar et al., 2015; Thu Lan et al., 2021). VRA governs lake resources by reinforcing the lake buffer of 280 ft. (85.3440 meters) to control developments along the lakefront. As an example, special bamboo trees are planted to create a waterfront buffer against encroachment. Furthermore, VRA organizes joint surveillance programs in collaboration with FC, EPA, DA, and WRC to monitor fish farmers and other lake users. Monitoring fish farms is guided by VRA’s framework for aquaculture development. We therefore recommend registering and licensing lake users, law enforcement, and awareness on sustainable lake exploitation. These regulatory measures should be accompanied with biosecurity measures and surveillance programs to effectively anticipate the risk factors of tilapia diseases and mortalities.
The method of data saturation was used to determine the sample size (n = 26) of online interviewees (Hennink and Kaiser, 2022). Saturation emerged as the ‘gold standard’ in qualitative inquiry at which point investigators do not realize any new data or themes after a consecutive number of interviews and focus group discussions (Guest et al., 2006; Fusch and Ness, 2015; Nelson, 2017). Statistically, 26 is considered not representative in comparison to the population of relevant stakeholders associated with Lake Volta aquaculture. The findings must therefore be interpreted with caution as it only offers a glimpse into risk factors and management practices through the opinions of relevant stakeholders involved in the study. People’s opinion are rooted in their experiences, culture, feelings, and beliefs, often lacking scientific validation (Douglas and Wildavsky, 1983; Grimble and Wellard, 1997; Commodari and La Rosa, 2020). Nonetheless, the study has discerned mortality and disease risks factors that merit testable hypothesis through quantitative research for conclusive remarks.
Standardized quantitative methods that could be applied are ecological risk assessment, quantitative microbial risk assessment, hazard identification, exposure assessment, and probabilistic risk assessment (Yeak et al., 2022; Domínguez et al., 2023; Hunt et al., 2023; Ma et al., 2023; Ntakiyisumba et al., 2023). Despite these limitations, this study pioneers the conceptualization of causation web and social-ecological system models to explain the practical implications of risk factors associated with fish health. A further limitation was the 15 fish farmers out of 26 participants. This is a clear misrepresentation since it does not accurately reflect the overall representation of the different stakeholder groups in the study. The dominant farmer group may introduce some biases even though the study is grounded in expert consensus and literature validation. In retrospect, the predominant representation of farmers disclosed their pleas, which can be useful for government to know policies that are likely to support farmers. While we recognize the limitations of opinion-based approaches in risk determination, complementing personal perspectives with quantitative analyzes can offer broader outlooks to build robust remedial measures (Hodgson et al., 2019).
5. Conclusion
Based on the opinion-based evidence, the risk factors of tilapia diseases and mortalities in Lake Volta cage aquaculture are a combination of pathogens and non-infectious factors. The pathogenic factors are S. agalactiae and ISKNV, which aligns with quantitative evidence in literature. Conversely, the qualitative evidence regarding non-infectious factors are improper handling, overfeeding, overstocking, water pollutants (e.g., herbicides, and pesticides). These non-infectious factors require testable hypotheses in future studies to establish conclusive insights into their roles in exacerbating tilapia diseases and mortalities. This must include a quantitative study to investigate the assertions of horizontal transfer of S. agalactiae and ISKNV from tilapia cadaver to catfish, along with the study of mycotoxins and their permissible limits in tilapia. While the study hints at some management practices, complementary quantitative studies on the risk factors can be pivotal in shaping policy directives and strategies aimed at effectively managing fish and lake health.
Data availability statement
The original contributions presented in the study are included in the article/supplementary material, further inquiries can be directed to the corresponding authors.
Author contributions
JZ: conceptualization, methodology, data collection, data analysis, visualization, writing–original draft, and review and editing. ST, EB, PZ, and IL: conceptualization, methodology, and writing–review and editing. PA and JA: methodology, data collection, and writing–review and editing. KC: conceptualization, methodology, resources, writing–review and editing, and supervision. All authors contributed to the article and approved the submitted version.
Funding
This study contributed to the Ghana Fish for Development (FfD) project funded by Norwegian Agency for Development Cooperation (NORAD). The workshop and article processing charge were funded by NORAD (Grant No. GHA 17/0005).
Acknowledgments
The first author appreciates the Norwegian Veterinary Institute for the opportunity as an intern on the NORAD-funded Fish for Development Program to conduct this work as part of a Master’s program in Wageningen University. The authors are indebted to the stakeholders for participating in the interviews and workshop; Geert Wiegertjes (Wageningen University) for technical feedback on the conceptual models; Jacob Adzikah (Chamber of Aquaculture Ghana) and Derrick Kwesi Asare (Ghana Fisheries Commission) for organizing fish farmers to participate; Rebecca Sarku (ClimAgri-Food Consult) for guidance in analyzes; and Michael Yeboah and Grace Darling Atopah (Wageningen University) for peer discussions on the conceptual models.
Conflict of interest
The authors declare that the research was conducted in the absence of any commercial or financial relationships that could be construed as a potential conflict of interest.
Publisher’s note
All claims expressed in this article are solely those of the authors and do not necessarily represent those of their affiliated organizations, or those of the publisher, the editors and the reviewers. Any product that may be evaluated in this article, or claim that may be made by its manufacturer, is not guaranteed or endorsed by the publisher.
References
Abarike, E., Atuna, R. A., Dandi, S. O., Agyekum, S., Appenteng, P., Alhassan, E. H., et al. (2022). Preliminary survey on the health and management practices among small-scale cage Tilapia farmers on Lake Volta.
Agyei, B. P. (2022). Sustainability of pond and cage fish farming Systems in the Ashanti, bono, eastern and Volta regions in Ghana (unpublished master’s thesis). Norway: Norwegian University of Life Sciences.
Amirkolaie, A. K. (2011). Reduction in the environmental impact of waste discharged by fish farms through feed and feeding. Rev. Aquac. 3, 19–26. doi: 10.1111/j.1753-5131.2010.01040.x
Anane-Taabeah, G., Frimpong, E. A., and Hallerman, E. (2019). Aquaculture-mediated invasion of the genetically improved farmed Tilapia (GIFT) into the lower Volta Basin of Ghana. Diversity 11:188. doi: 10.3390/d11100188
Anater, A., Manyes, L., Meca, G., Ferrer, E., Luciano, F. B., Pimpão, C. T., et al. (2016). Mycotoxins and their consequences in aquaculture: a review. Aquaculture 451, 1–10. doi: 10.1016/j.aquaculture.2015.08.022
Ann, P. K., Paul, R., Michel, E., Helen, A., Thomas, E., and Brian, H. W. (2006). Resilience and regime shifts: assessing cascading effects. Ecol. Soc. 11:20. doi: 10.5751/ES-01678-110120
Aquaculture and Fisheries Regulations. (2020). L. I. 1968, Fish seed production certificate and fish transfer permit. Available at: https://faolex.fao.org/docs/pdf/gha151991.pdf. (Accessed June 25, 2023).
Ayalew, A., and Fufa, A. (2018). Maintenance of fish health in aquaculture: review of epidemiological approaches for prevention and control of infectious disease of fish. Vet. Med. Int. 2018, 1–10. doi: 10.1155/2018/5432497
Ayiku, A., Adelani, A., Appenteng, P., Nkansah, M., Ngoi, J. M., Collins, M., et al. (2022). Molecular epidemiology of infectious spleen and kidney necrosis virus (ISKNV). bioRxiv 2022:516701. doi: 10.1101/2022.11.15.516701
Barreto, M. (2005). Epidemiologists and causation in an intricate world. Emerg. Themes Epidemiol. 2, 2–3. doi: 10.1186/1742-7622-2-3
Béné, C. (2007). Diagnostic study of the Volta Basin fisheries: part 1 overview of the fisheries resources. WorldFish p. 31.
Benusiglio, P. R., Fallet, V., Sanchis-Borja, M., Coulet, F., and Cadranel, J. (2021). Lung cancer is also a hereditary disease. Eur. Respir. Rev. 30:210045. doi: 10.1183/16000617.0045-2021
Chen, M., Wang, R., Liping, L., Liang, W., Wang, Q., Huang, T., et al. (2014). Immunological enhancement action of endotoxin-free tilapia heat shock protein 70 against Streptococcus iniae. Cell. Immunol. 290, 1–9. doi: 10.1016/j.cellimm.2013.12.008
Chinnadurai, K., Prema, P., Veeramanikandan, V., Kumar, K. R., Nguyen, V. H., Marraiki, N., et al. (2022). Toxicity evaluation and oxidative stress response of fumaronitrile, a persistent organic pollutant (pop) of industrial waste water on tilapia fish (Oreochromis mossambicus). Environ. Res. 204:112030. doi: 10.1016/j.envres.2021.112030
Coenen, M., Stamm, T. A., Stucki, G., and Cieza, A. (2012). Individual interviews and focus groups in patients with rheumatoid arthritis: a comparison of two qualitative methods. Qual. Life Res. 21, 359–370. doi: 10.1007/s11136-011-9943-2
Commodari, E., and La Rosa, V. L. (2020). Adolescents in quarantine during COVID-19 pandemic in Italy: perceived health risk, beliefs, psychological experiences and expectations for the future. Front. Psychol. 11:2480. doi: 10.3389/fpsyg.2020.559951
Cooper, G. S., and Dearing, J. A. (2019). Modelling future safe and just operating spaces in regional social-ecological systems. Sci. Total Environ. 651, 2105–2117. doi: 10.1016/j.scitotenv.2018.10.118
Creswell, J. W. (2014). Research design: Qualitative, quantitative, and mixed methods approaches. 4th Edn. Thousand Oaks, CA: SAGE Publications.
Dey, I. (2003). Qualitative data analysis: A user friendly guide for social scientists. Abingdon: Routledge.
Dini, L., Lanubile, R., Tarantino, P., Mandich, A., and Cataldi, E. (2006). Expression of stress proteins 70 in tilapia (Oreochromis mossambicus) during confinement and crowding stress. Ital. J. Zool. 73, 117–124. doi: 10.1080/11250000600679652
Domínguez, R. C., Guadian, J. E. R., Guerrero, J. L., and Mares, J. I. P. (2023). Hazard identification for risk assessment using the pra technique in the automotive industry. Saf. Sci. 160:106041. doi: 10.1016/j.ssci.2022.106041
Dornelas, M. (2010). Disturbance and change in biodiversity. Philos. Trans. R. Soc. Lond. Ser. B Biol. Sci. 365, 3719–3727. doi: 10.1098/rstb.2010.0295
Douglas, M., and Wildavsky, A. (1983). Risk and culture - an essay on the selection of technological and environmental dangers, Berkeley, CA: University of California Press.
Downing, A. S. E., Van Nes, J., Balirwa, J., Beuving, P., Bwathondi, L. J., Chapman, I. J. M., et al. (2014). Coupled human and natural system dynamics as key to the sustainability of Lake Victoria’s ecosystem services. Ecol. Soc. 19:190431. doi: 10.5751/ES-06965-190431
Duodu, S., Jansen, M. D., Nkansa, M., Ayiku, A. N. A., Adelani, A. A., Erkinharju, T., et al. (2022). Fish for Development baseline study to map the disease occurrence in tilapia farms on Lake Volta. Report, 36/2022.
Eszterbauer, E., Forró, B., Tolnai, Z., Guti, C., Zsigmond, G., Hoitsy, G., et al. (2015). Parental genetic diversity of brown trout (Salmo trutta m. fario) brood stock affects offspring susceptibility to whirling disease. Parasit. Vectors 8:141. doi: 10.1186/s13071-015-0744-2
Fichtenberg, C. M., and Glantz, S. A. (2002). Effect of smoke-free workplaces on smoking behaviour: systematic review. Br. Med. J. 325:188. doi: 10.1136/bmj.325.7357.188
Fusch, P. I., and Ness, L. R. (2015). Are we there yet? Data saturation in qualitative research. Qual. Rep. 20, 1408–1416. doi: 10.46743/2160-3715/2015.2281
Gao, Y., Wang, Z., Hur, J. W., and Lee, J. Y. (2015). Body composition and compensatory growth in Nile tilapia Oreochromis niloticus under different feeding intervals. Chin. J. Oceanol Limnol. 33, 945–956. doi: 10.1007/s00343-015-4246-z
Grimble, R., and Wellard, K. (1997). Stakeholder methodologies in natural resource management: a review of principles, contexts, experiences and opportunities. Agric. Syst. 55, 173–193. doi: 10.1016/S0308-521X(97)00006-1
Guest, G., Bunce, A., and Johnson, L. (2006). How many interviews are enough? An experiment with data saturation and variability. Field Methods 18, 59–82. doi: 10.1177/1525822X05279903
Hammersley, M. (2012). “Qualitative causal analysis: grounded theorizing and the qualitative survey” in Challenging the qualitative-quantitative divide: Explorations in case-focused causal analysis. eds. B. Cooper, J. Glaesser, R. Gomm, and M. Hammersley (London, England: Continuum), 72–95.
Haraldsson, M., Raoux, A., Riera, F., Hay, J., Dambacher, J., and Niquil, N. (2020). How to model social-ecological systems? – a case study on the effects of a future offshore wind farm on the local society and ecosystem, and whether social compensation matters. Mar. Policy 119:104031. doi: 10.1016/j.marpol.2020.104031
Hennink, M., and Kaiser, B. N. (2022). Sample sizes for saturation in qualitative research: a systematic review of empirical tests. Soc. Sci. Med. 292:114523. doi: 10.1016/j.socscimed.2021.114523
Hodgson, E. E., Essington, T. E., Samhouri, J. F., Allison, E. H., Bennett, N. J., Bostrom, A., et al. (2019). Integrated risk assessment for the blue economy. Front. Mar. Sci. :6:609. doi: 10.3389/fmars.2019.00609
Hossain, M. S., Ramirez, J., Szabo, S., Eigenbrod, F., Johnson, F. A., Speranza, C. I., et al. (2020). Participatory modelling for conceptualizing social-ecological system dynamics in the Bangladesh delta. Reg. Environ. Chang. 20, 1–14. doi: 10.1007/S10113-020-01599-5/TABLES/3
Hunt, K., Doré, B., Keaveney, S., Rupnik, A., and Butler, F. (2023). A quantitative exposure assessment model for norovirus in oysters harvested from a classified production area. Microb. Risk Anal. 23:100247. doi: 10.1016/j.mran.2023.100247
Iregui, C., Barato, P., Rey, A., Vasquez, G., and Verjan, N. (2014). “Epidemiology of Streptococcus agalactiae and Streptococcosis in tilapia (Oreochromis sp.)” in Epidemiology 1: theory, research and practice. ed. M. Jansen (Hong Kong: iConcept Press), 251–268.
Joffe, M., Gambhir, M., Chadeau-Hyam, M., and Vineis, P. (2012). Causal diagrams in systems epidemiology. Emerg. Themes Epidemiol. 9, 1–18. doi: 10.1186/1742-7622-9-1
Kamler, E., Myszkowski, L., Kamiński, R., Korwin-Kossakowski, M., and Wolnicki, J. (2006). Does overfeeding affect tench Tinca tinca (L.) juveniles? Aquac. Int. 14, 99–111. doi: 10.1007/s10499-005-9018-x
Kapinga, I. B., Limbu, S. M., Madalla, N. A., Kimaro, W. H., and Tamatamah, R. A. (2018). Aspilia mossambicensis and Azadirachta indica medicinal leaf powders modulate physiological parameters of Nile tilapia (Oreochromis niloticus). Int. J. Vet. Sci. Med. 6, 31–38. doi: 10.1016/j.ijvsm.2018.03.003
Kassam, L. (2014). Aquaculture and food security, poverty alleviation and nutrition in Ghana: case study prepared for the aquaculture for food security, Poverty Alleviation and Nutrition project. Penang, Malaysia: WorldFish
Krippendorff, K. (2004). Content analysis: An introduction to its methodology. Thousand Oaks, CA: SAGE Publications, p. 93.
Lebel, L., Lebel, P., and Chuah, C. J. (2019). Water use by inland aquaculture in Thailand: stakeholder perceptions, scientific evidence, and public policy. Environ. Manag. 63, 554–563. doi: 10.1007/s00267-019-01143-0
Levins, R. (1974). The qualitative analysis of partially specified systems. Ann. N. Y. Acad. Sci. 231, 123–138. doi: 10.1111/j.1749-6632.1974.tb20562.x
Ma, X., Xu, J., Pan, J., Yang, J., Wu, P., and Meng, X. (2023). Detection of marine oil spills from radar satellite images for the coastal ecological risk assessment. J. Environ. Manag. 325:116637. doi: 10.1016/j.jenvman.2022.116637
MacMahon, B., and Pugh, T. F. (1970). Epidemiology principles and methods. Boston: Little Brown and Co.
MacMahon, B., Pugh, T. F., and Ipsen, J. (1960). Epidemiological methods. Boston, MA: Little, Brown and Co.
Mantey, V., Mburu, J., and Chumo, C. (2020). Determinants of adoption and disadoption of cage tilapia farming in southern Ghana. Aquaculture 525:735325. doi: 10.1016/j.aquaculture.2020.7353
Marijani, E., Kigadye, E., and Okoth, S. (2019). Occurrence of Fungi and mycotoxins in fish feeds and their impact on fish health. Int. J. Microbiol. 2019, 1–17. doi: 10.1155/2019/6743065
Mondal, P., Garai, P., Chatterjee, A., and Saha, N. (2020). Toxicological and therapeutic effects of neem (Azadirachta indica) leaf powder in hole-in- the- head (HITH) disease of fish Anabas testudineus. Aquac. Res. 52, 715–723. doi: 10.1111/are.14928
Myers, S. S., Gaffikin, L., Golden, C. D., Ostfeld, R. S., Redford, K. H., Ricketts, T., et al. (2013). Human health impacts of ecosystem alteration. Proc. Natl. Acad. Sci. 110, 18753–18760. doi: 10.1073/pnas.1218656110
Nassl, M., and Löffler, J. (2015). Ecosystem services in coupled social-ecological systems: closing the cycle of service provision and societal feedback. Ambio: a journal of the human. Environment 44, 737–749. doi: 10.1007/s13280-015-0651-y
Nelson, J. (2017). Using conceptual depth criteria: addressing the challenge of reaching saturation in qualitative research. Qual. Res. 17, 554–570. doi: 10.1177/1468794116679873
Novoslavskij, A., Terentjeva, M., Eizenberga, I., Valciņa, O., Bartkevičs, V., and Bērziņš, A. (2016). Major foodborne pathogens in fish and fish products: a review. Ann. Microbiol. 66, 1–15. doi: 10.1007/s13213-015-1102-5
Ntakiyisumba, E., Lee, S., and Won, G. (2023). Identification of risk profiles for salmonella prevalence in pig supply chains in South Korea using meta-analysis and a quantitative microbial risk assessment model. Food Res. Int. 170:112999. doi: 10.1016/j.foodres.2023.112999
Odhiambo, E., Angienda, P. O., Okoth, P., and Onyango, D. (2020). Stocking density induced stress on plasma cortisol and whole blood glucose concentration in nile tilapia fish (Oreochromis niloticus) of Lake victoria, Kenya. Int. J. Zool. 2020, 1–8. doi: 10.1155/2020/9395268
Pope, C. A., Thun, M. J., Namboodiri, M. M., Dockery, D. W., Evans, J. S., Speizer, F. E., et al. (1995). Particulate air pollution as a predictor of mortality in a prospective study of U.S. adults. Am. J. Respir. Crit. Care Med. 151, 669–674. doi: 10.1164/ajrccm/151.3_Pt_1.669
Ragasa, C., Andam, K., Kufoalor, D., and Amewu, S. (2018). A blue revolution in sub-Saharan Africa? Evidence from Ghana's tilapia value chain. Report number: IFPRI GSSP working paper 49.
Ramírez-Paredes, J. G., Paley, R. K., Hunt, W., Feist, S. W., Stone, D. M., Field, T. R., et al. (2021). First detection of infectious spleen and kidney necrosis virus (ISKNV) associated with massive mortalities in farmed tilapia in Africa. Transbound. Emerg. Dis. 68, 1550–1563. doi: 10.1111/tbed.13825
Riche, M., Oetker, M., Haley, D. I., Smith, T., and Garling, D. L. (2004). Effect of feeding frequency on consumption, growth, and efficiency in juvenile tilapia (Oreochromis niloticus). Israel J. Aquacult. 56, 247–255. doi: 10.46989/001c.20386
Rurangwa, E., Agyakwah, S. K., Boon, H., and Bolman, B. C. (2015). Development of aquaculture in Ghana: Analysis of the fish value chain and potential business cases, (Ser. Imares report, c021/15). IMARES Wageningen UR. Available at: http://edepot.wur.nl/337344. (Accessed September 4, 2023).
Sarkar, S., Singh, R. P., and Bhattacharya, G. (2021). Exploring the role of Azadirachta indica (neem) and its active compounds in the regulation of biological pathways: an update on molecular approach. 3 Biotech 11:178. doi: 10.1007/s13205-021-02745-4
Sarku, R., Gbangou, T., Dewulf, A., and Slobbe, E. (2020). Beyond "expert knowledge": Locals and experts in a joint production of weather app and weather information for farming in the Volta Delta, Ghana.
Scavarda, A., Bouzdine-Chameeva, T., Goldstein, S., Hays, J., and Hill, A. (2006). A methodology for constructing collective causal maps. Decis. Sci. 37, 263–283. doi: 10.1111/j.1540-5915.2006.00124.x
Soto-Ortiz, L. (2015). The regulation of ecological communities through feedback loops: a review. Res. Zool. 5, 1–15. doi: 10.5923/j.zoology.20150501.01
Sousa, W. P. (1984). The role of disturbance in natural communities. Annu. Rev. Ecol. Syst. 15, 353–391. doi: 10.1146/annurev.es.15.110184.002033
Spagnuolo, V., Terracciano, S., and Giordano, S. (2009). Trace element content and molecular biodiversity in the epiphytic moss Leptodon smithii: two independent tracers of human disturbance. Chemosphere 74, 1158–1164. doi: 10.1016/j.chemosphere.2008.11.080
Tay, C. K. (2021). Integrating water quality indices and multivariate statistical techniques for water pollution assessment of the Volta lake, Ghana. Sustain. Water Resour. Manag. 7, 1–23. doi: 10.1007/s40899-021-00552-6
Thu Lan, N. G., Salin, K. R., Longyant, S., Senapin, S., and Dong, H. T. (2021). Systemic and mucosal antibody response of freshwater cultured Asian seabass (Lates calcarifer) to monovalent and bivalent vaccines against Streptococcus agalactiae and Streptococcus iniae. Fish Shellfish Immunol. 108, 7–13. doi: 10.1016/j.fsi.2020.11.014
Tobar, I., Arancibia, S., Torres, C., Vera, V., Soto, P., Carrasco, C., et al. (2015). Successive oral immunizations against Piscirickettsia salmonis and infectious salmon anemia virus are required to maintain a long-term protection in farmed salmonids. Front. Immunol. 6:244. doi: 10.3389/fimmu.2015.00244
Verner-Jeffreys, D., Wallis, T., Cano, I., Ryder, D., Haydon, D., Domazoro, J. F., et al. (2018). Streptococcus agalactiae multilocus sequence type 261 is associated with mortalities in the emerging Ghanaian tilapia industry. J. Fish Dis. 41, 175–179. doi: 10.1111/jfd.12681
Vial, A., Van der Put, C., Stams, G. J. J., Assink, M., and Kossakowski, J. (2020). Exploring the interrelatedness of risk factors for child maltreatment: a network approach. Child Abuse Negl. 107:104622. doi: 10.1016/j.chiabu.2020.104622
Virolainen, S. J., VonHandorf, A., Viel, K. C. M. F., Weirauch, M. T., and Kottyan, L. C. (2022). Gene–environment interactions and their impact on human health. Genes Immunity 24, 1–11. doi: 10.1038/s41435-022-00192-6
Walker, P., and Winton, J. R. (2010). Emerging viral diseases of fish and shrimp. Vet. Res. 41:51. doi: 10.1051/vetres/2010022
Wanja, D., Mbuthia, P., Waruiru, R., Mwadime, J., Bebora, L., Nyaga, P., et al. (2020). Fish husbandry practices and water quality in Central Kenya: potential risk factors for fish mortality and infectious diseases. Vet. Med. Int. 2020, 1–10. doi: 10.1155/2020/6839354
White, P. S., and Pickett, S. T. A. (1985). “Natural disturbance and patch dynamics: an introduction” in The ecology of natural disturbance and patch dynamics. ed. S. T. A. Pickett (Orlando, FL: Academic Press)
Wise, A. L., LaFrentz, B. R., Kelly, A. M., Khoo, L. H., Xu, T., Liles, M. R., et al. (2021). A review of bacterial co-infections in farmed catfish: components, diagnostics, and treatment directions. Animals 11:3240. doi: 10.3390/ani11113240
Wolfinger, N. H. (2002). On writing fieldnotes: collection strategies and background expectancies. Qual. Res. 2, 85–93. doi: 10.1177/1468794102002001640
Yeak, K. Y. C., Palmont, P., Rivière, G., Bemrah, N., den Besten, H. M. W., and Zwietering, M. (2022). Microbial and chemical Hazard identification in infant food chains. Glob. Pediatr. 2:100010. doi: 10.1016/j.gpeds.2022.100010
Keywords: Nile tilapia (Oreochromis niloticus), mortalities, Lake Volta, systemic approach, reinforcing mechanisms, pathogens, non-infectious factors, management practices
Citation: Zornu J, Tavornpanich S, Brun E, van Zwieten PAM, van de Leemput I, Appenteng P, Anchirinah J and Cudjoe KS (2023) Understanding tilapia mortalities and fish health management in Lake Volta: a systematic approach. Front. Sustain. Food Syst. 7:1249898. doi: 10.3389/fsufs.2023.1249898
Edited by:
Kwasi Adu Adu Obirikorang, Kwame Nkrumah University of Science and Technology, GhanaReviewed by:
Domitila Kyule, Kenya Marine and Fisheries Research Institute, KenyaEmmanuel Mensah, Council for Scientific and Industrial Research, Ghana
Copyright © 2023 Zornu, Tavornpanich, Brun, van Zwieten, van de Leemput, Appenteng, Anchirinah and Cudjoe. This is an open-access article distributed under the terms of the Creative Commons Attribution License (CC BY). The use, distribution or reproduction in other forums is permitted, provided the original author(s) and the copyright owner(s) are credited and that the original publication in this journal is cited, in accordance with accepted academic practice. No use, distribution or reproduction is permitted which does not comply with these terms.
*Correspondence: Jacob Zornu, amFjb2Iuem9ybnVAdmV0aW5zdC5ubw==; Kofitsyo S. Cudjoe, a29maXRzeW8uY3Vkam9lQHZldGluc3Qubm8=