- 1Harvard University Extension School, Cambridge, MA, United States
- 2Lund University Centre for Sustainability Studies (LUCSUS), Lund, Sweden
Introduction: Regenerative agriculture commonly aims to increase soil carbon sequestration, with potential benefits for human and ecosystem health, climate mitigation, and biodiversity. However, the effectiveness of various regenerative practices at increasing carbon sequestration is unclear.
Methods: This study identified and quantified the yearly soil carbon sequestration rate of regenerative practices in arable cropland and vineyard ecosystems through a literature review. We examined N=345 soil carbon sequestration measures across seven regenerative practices – agroforestry, cover cropping, legume cover cropping, animal integration, non-chemical fertilizer, non-chemical pest management, and no tillage.
Results: Our findings indicate that all seven practices effectively increased the carbon sequestration rate. There were no statistically significant differences among the practices. Combining these practices may further enhance soil carbon sequestration.
Discussion: We propose a sequence of regenerative practices that farmers can adopt, balancing ease of implementation and carbon sequestration effectiveness. To address under-studied practices, we recommend further research, including long-term monitoring studies and randomized controlled trials in perennial systems. Our recommendations aim to enhance the implementation and effectiveness of regenerative practices while mitigating the current challenge of limited sample sizes for quantifying carbon sequestration.
1 Introduction
Healthy, living soil is the basis for all life on Earth (European Commission, 2020), through its capacity to sustain and enhance plant and animal life and health, and to regenerate water and air quality health (Zehetner et al., 2015). Among its multiple critical ecosystem services and functions, soil is the second-largest active carbon pool after the oceans, and plays a crucial role in the global carbon cycle (FAO and ITPS, 2018) and climate regulation (Swiss Confederation, 2020). Moreover, healthy soil is vital for human nutrition and for the human gut microbiome, for which it is a major inoculant and provides microorganisms to the gut that are essential to the “microbiome-gut-brain axis” and thus to human health (Blum et al., 2019; Brevik et al., 2020, p. 10). Soil health is the main goal of one the European Commission’s five Missions, aiming to achieve a 75% increase in healthy soils by 2030 (European Commission, 2020). Moreover, soil is a key element of food security and climate adaptation in the face of a new and more extreme climate as our planet warms (United Nations Environment Programme, 2021).
Globally, agriculture is the leading driver of soil degradation (Olsson et al., 2019). Current industrialized farming practices deplete the beneficial microbes in soil essential for ecosystem and human microbiome diversity and functioning (Blum et al., 2019). Such practices include mechanization, soil tillage, soilless cultivation (such as hydroponics), soil erosion, nutrient depletion in soil, monocultures, separation of animals from crops, excessive use of agrochemicals such as mineral fertilizers and pesticides, and use of antibiotics and hormones (Blum et al., 2019). The habitat loss driven by such practices also makes agriculture the strongest driver of biodiversity loss (Scholes et al., 2018). In order to regulate the Earth system that the Anthropocene threatens to destabilize, an urgent mindset shift is needed for agricultural ecosystems to be perceived as possibly the Earth’s largest biome, with the greatest impact on the planet’s nitrogen, phosphorus, water and carbon cycles (Rockström et al., 2020).
Soil organic carbon (SOC, hereafter abbreviated C) is the main terrestrial pool in the carbon cycle (Chen et al., 2022) and is an indicator of soil health (FAO and ITPS, 2020). Soil organic carbon includes carbon fractions of varying stability, from labile carbon in decaying plant material with very short soil lifetimes, to recalcitrant carbon with very long lifetimes. Agronomic studies measuring or modeling soil carbon may refer to short-term carbon “storage” and reserve the term “sequestration” for carbon that is physically or chemically protected and thus stored in soil long-term. However, recent research on the potential of agriculture to contribute to climate mitigation tends to use “carbon sequestration” as the latest IPCC report does, to mean “the process of storing carbon in a carbon pool” (van Diemen et al., 2023) without specifically referring to the timeframe of carbon storage achieved. IPCC uses the term “sequestration potential” to refer to “the quantity of greenhouse gasses that can be removed from the atmosphere by anthropogenic enhancement of sinks and stored in a pool” (van Diemen et al., 2023), and includes both C that is chemically or physically protected and decomposes slowly, as well as C that supports microbial activity, decomposes faster, and can create carbon emissions via soil respiration. Here, following this broad IPCC definition, we use “carbon sequestration” to mean the process of increased carbon storage in soils due to adopting a specific management practice, expressed as a rate: tons of total organic carbon stored in the soil carbon pool per hectare per year.
Increasing available organic C in soil through enhanced carbon sequestration can increase soil microbial mass (Fierer, 2017) and the network complexity of soil communities, including keystone microbe and mycorrhizae species that play an important role in soil life, plant life, and carbon cycling (Xue et al., 2020). However, soil organic C stocks globally in agricultural soils are declining (Wiesmeier et al., 2019). Soil management practices can cause carbon to be absorbed by soil (act as a carbon sink) or emitted from the soil to the atmosphere (act as a carbon source) (ADEME, 2013). Therefore, identifying and quantifying practices that can sequester soil organic C is vital to counteract climate change and soil degradation.
While sustainability aims to maintain the status quo, regenerative practices and regenerative societal change aims to speed the behavior of systems toward positive socio-ecological outcomes (Casarejos, 2020). Regenerative agriculture has the potential to go beyond sustainability, providing systemic, positive benefits (Figure 1). Of course, carbon and climate are just one piece of the equation for a holistic planetary health approach. The biodiversity focus of regenerative agriculture means that it tends to be complex, knowledge-intensive, context-specific, and positive for healthy soil life (FAO, 2019).
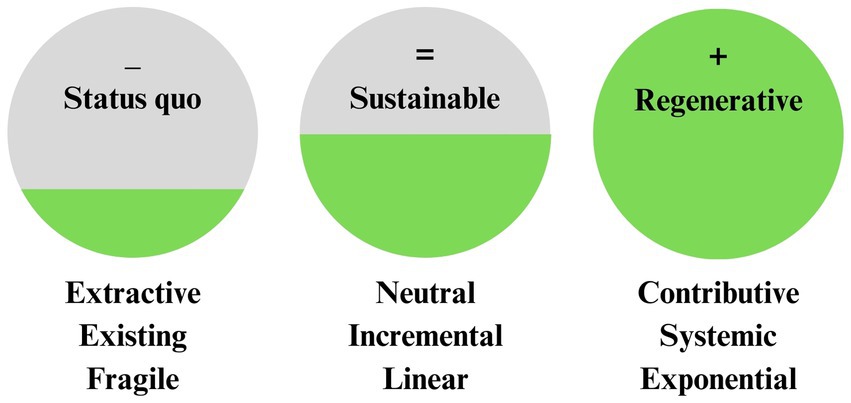
Figure 1. Going beyond the depleting existing model of agriculture and beyond sustainability, the regenerative approach aims to increase overall system well-being. Figure adapted with permission from Fitzgerald (2021).
There is no formal or widely accepted definition of “regenerative agriculture,” with some sources emphasizing specific farming practices such as no-till or cover crops, others focusing on achieving outcomes such as increased soil carbon or biodiversity, and others referring to using regenerative practices to achieve specific outcomes (Newton et al., 2020). For example, the NGO Regeneration International describes regenerative agriculture as a set of both practices and outcomes, namely “farming and grazing practices that, among other benefits, reverse climate change by rebuilding soil organic matter and restoring degraded soil biodiversity” (Regeneration International, 2017, p. 1). In a review of 229 peer-reviewed articles and 25 practitioner websites, Newton et al. (2020) found that the most common description of regenerative agriculture was outcome-based, namely the outcome of improving soil health including soil organic matter, mentioned by 86% of practitioners and 40% of scientific papers. Sixty-four percent of practitioners mentioned increased soil carbon sequestration specifically (Newton et al., 2020). Moreover, widespread adoption and policy encouragement of regenerative practices is occurring (Al-Kaisi and Lal, 2020). However, Newton et al. (2020) note a lack of evidence to support the sometimes extraordinary claims of the potential outcomes of following regenerative practices, such as the potential to approach zero-carbon farming (Giller et al., 2021).
Given the need for evidence on the outcomes of specific regenerative practices, our study begins the long journey ahead in quantifying how much carbon different regenerative practices sequester in agricultural soils. We particularly focused on viticulture (winegrowing) due to its traditional focus on terroir (soil and the whole ecosystem surrounding vines) and its higher environmental, economic and cultural strength to innovate compared with other cropping systems. The fact that soil and its characteristics are recognized as essential to wine quality was also a factor (Giffard et al., 2022). Additionally, viticulture is a perennial crop, and in regenerative agriculture perennials have been found to have beneficial effects on soil, water, biodiversity and climate (Scott et al., 2022). For instance, the soil organic C sequestration potential for viticulture using regenerative practices could be up to four times higher than for other types of cropland (Zomer et al., 2017; Payen et al., 2021). Specifically, we synthesized the existing literature to identify and quantify the carbon sequestration potential of the following nine soil practices: agroforestry, the use of cover crops (non-legume), the use of legume cover crops, animal integration, low traffic of farm equipment, non-chemical fertilizer, non-chemical pest management, no tillage, and redesigning the system at the landscape level. By identifying these nine practices and quantifying the amount of carbon that they can sequester in woody perennial cropland (including viticulture) vs. arable land, we develop a novel taxonomy of soil regenerative practices applicable to viticulture, and contribute to practical and urgently needed research for regenerating soil structure and biodiversity in agriculture (FAO et al., 2020).
2 Methods
This section outlines the methods used in this study to identify and select key soil regenerative practices relevant to viticulture. The selection criteria included practices that are regenerative, have positive impacts on the environment and human health, and where possible, are beneficial for crop yield, farm income, and resource efficiency. We identified the selected practices through a literature review and mapped practices from three foundational sources. Table 1 shows the nine identified regenerative practices along with their definitions and sources.
2.1 Selecting soil regenerative practices to include
We first identified the nine key soil regenerative practices (Table 1) by conducting a literature review and mapping practices from three foundational sources: two pioneer European initiatives beginning to identify emerging soil-improving cropping systems and soil carbon sequestering practices that combat biodiversity decline, desertification, erosion and more, namely (1) the European SoilCare project (Oenema et al., 2017) and (2) the 4 per 100 initiative (Pellerin et al., 2020), as well as (3) a study defining key elements of regenerative farming (LaCanne and Lundgren, 2018). The criteria for selecting practices to quantify included:
• Practices relevant to viticulture (for example since grapevines are a permanent crop, crop rotations as a soil regeneration practice were discarded)
• Regenerative, closed-loop system practices (for example irrigation as a practice is generally an input external to the system as opposed to rainfed agriculture, so irrigation was discarded as not regenerative)
• Practices that have a positive impact on the environment and human health (for example increasing biodiversity and soil life, and avoiding health-impairing chemicals)
• Practices that where possible and indicated by Oenema et al. (2017) are beneficial for crop yield, farm income, and resource efficiency
We then further revised our practice categories and nomenclature according to practices found in the literature review.
2.2 Identifying literature and quantifying the practices
To quantify the observed impact on annual soil carbon sequestration rates of these nine regenerative practices, we performed an observational synthesis similar to Georgiou et al. (2022) from studies that included soil C sequestration data for each practice. To locate studies, we ran two Google Scholar queries. In the first query, we used the following search terms: (“vineyard” OR “viticulture” OR “wine” OR “grape*” OR “permanent crop”) AND (“management practice” OR “cropping system”) AND (“carbon sequestration” OR “soil organic carbon”) AND (“Kg C”). For the second query, we used the following terms: (“vitis vinifera”) AND (“practice” OR “manage*”) AND (“soil organic carbon”) AND (“Switzerland”). The overall goal was to find soil C sequestration figures relevant to viticulture, with the second query having a secondary focus on Switzerland to find studies for a separate but related qualitative examination of barriers and enablers to adopting regenerative practices among 20 French-speaking Swiss winegrowers (Villat, 2021). As with all literature searches, our search terms constrained the articles we found; see the ‘Future research’ section for our recommendations to build on the present work in the future.
The studies were then sorted for relevance and scanned to determine if they actually included a comparable C sequestration measure. Of the relevant studies from which a C sequestration figure could be extracted, 70% came from the first query and 30% came from the second query. Where relevant and possible, the data from meta studies was expanded and studies that were referenced were added. Additional sources recommended by entities such as the Changins School of Viticulture and Oenology, the Swiss Research Institute of Organic Agriculture (FiBL), and the University of Geneva’s Institute for Environmental Sciences were also included.
Observational, experimental, as well as modeling studies were sampled, coming primarily from North and South America, Europe, and China. There were also a few samples from Southeast Asia, Africa, Australia, and New Zealand. Studies included measured soil organic C at depths varying from 10 to 100 cm (averaging 21 cm across all studies gathered). The average study length was 15 years.
To be included, studies had to report the change in annual soil carbon sequestration upon adopting a regenerative practice. Studies were eliminated if they did not report a soil organic C sequestration rate directly (or a baseline measurement which enabled calculating a sequestration rate over time), or if the causal effect of the practice could not be dissociated from other practices. For a baseline, we looked for studies that indicated initial soil organic C before a regenerative practice was applied on control plots, or that measured the difference in soil organic C before and after the application of a regenerative practice. We did not include studies that simply indicated an absolute soil organic C value after applying the practice, because the effect of the regenerative practice could not be calculated. In some cases, a proxy practice was used, such as manure integration (a byproduct of animal integration), since there were few studies on animal integration directly.
For each relevant study, we noted the document identifier (DOI), author, year, title of the study, depth of the soil sample, length of the study in years, and which of the nine soil regenerative practices the study referred to. We also classified land use type as either “arable cropland” or “woody perennials” (Gattinger et al., 2019). Arable cropland included figures from arable, vegetable and mixed land for annual cropping systems as well as from grasslands. The term ‘mixed land’ is used to refer to studies or meta studies on the combined effect of a certain practice on soil organic C across a number of different sites, plots or studies with different types of annual crops. Woody perennial cropland included perennial agroecosystems, such as vineyards and coffee agroecosystems, which recent research indicates can be assessed for soil threats in the same way (Diti et al., 2020). Woody perennials are analyzed separately because they have a longer lifespan than annual arable crops and have a greater average gain in soil C over time (Ehrlén and Lehtilä, 2002; Ledo et al., 2020).
The carbon sequestration rate for each study was noted according to practice and standardized to tons of carbon per hectare per year (t C/ha/yr). The rate value came from averages calculated in independent studies. We compared the carbon sequestration rate for each practice within each of the two land use types using an ANOVA, followed by t-tests to compare means within practices. We also identified meta-analyses of average global carbon sequestration rates using soil-organic-C-enhancing practices for both arable and woody perennial agriculture, which we used as a point of comparison (Zomer et al., 2017; Payen et al., 2021).
3 Literature search results
Our literature search yielded a total of 658 studies, of which 345 reported relevant soil organic C sequestration data for seven of the nine regenerative practices, and two combinations of two practices, primarily for arable land use (Table 2). The two combinations were where a cover crop and no-tillage were applied together, and where double cover crops—one legume and one non-legume—were applied in succession during the annual cycle. Although both low traffic and redesigning the system at the landscape level were identified in previous literature as important practices for soil regeneration and C sequestration, no studies were found measuring C sequestration for them, so these practices could not be quantified. The largest sample sizes were for non-chemical fertilizer, due to inclusion of a data-rich meta-study on organic farming, which featured 72 independent studies, many with multiple data points (Gattinger et al., 2019). Overall sample sizes were low, with only three additional practices (agroforestry, cover crop, and no-till) featuring more than 10 samples in arable land, and smaller sample sizes in woody perennial land.
3.1 Arable land use
On arable land, agroforestry and the use of double cover crops (one legume and one non-legume) had mean sequestration rates of 1.22 t C/ha/yr. (N = 14) and 1.20 t C/ha/yr. (N = 2), respectively (Figure 2). Combining a (non-legume) cover crop and no-tillage had a mean of 1.01 t C/ha/yr. (though also with a low number of samples, N = 6). Non-chemical pest management had a mean of 0.89 (N = 4), animal integration 0.67 (N = 8), and cover cropping 0.58 t C/ha/yr. (N = 15). Non-chemical fertilizer, no-till, and legume cover-cropping had similar sequestration means, averaging 0.48 t C/ha/yr. together (N = 187, N = 25, and N = 3, respectively). The widest range in values across all practices was for non-chemical fertilizers (N = 187), from emitting 3.8 to sequestering 5.9 t C/ha/yr., and was the only practice with negative outliers (Figure 2), indicating a source of C emissions in some cases.
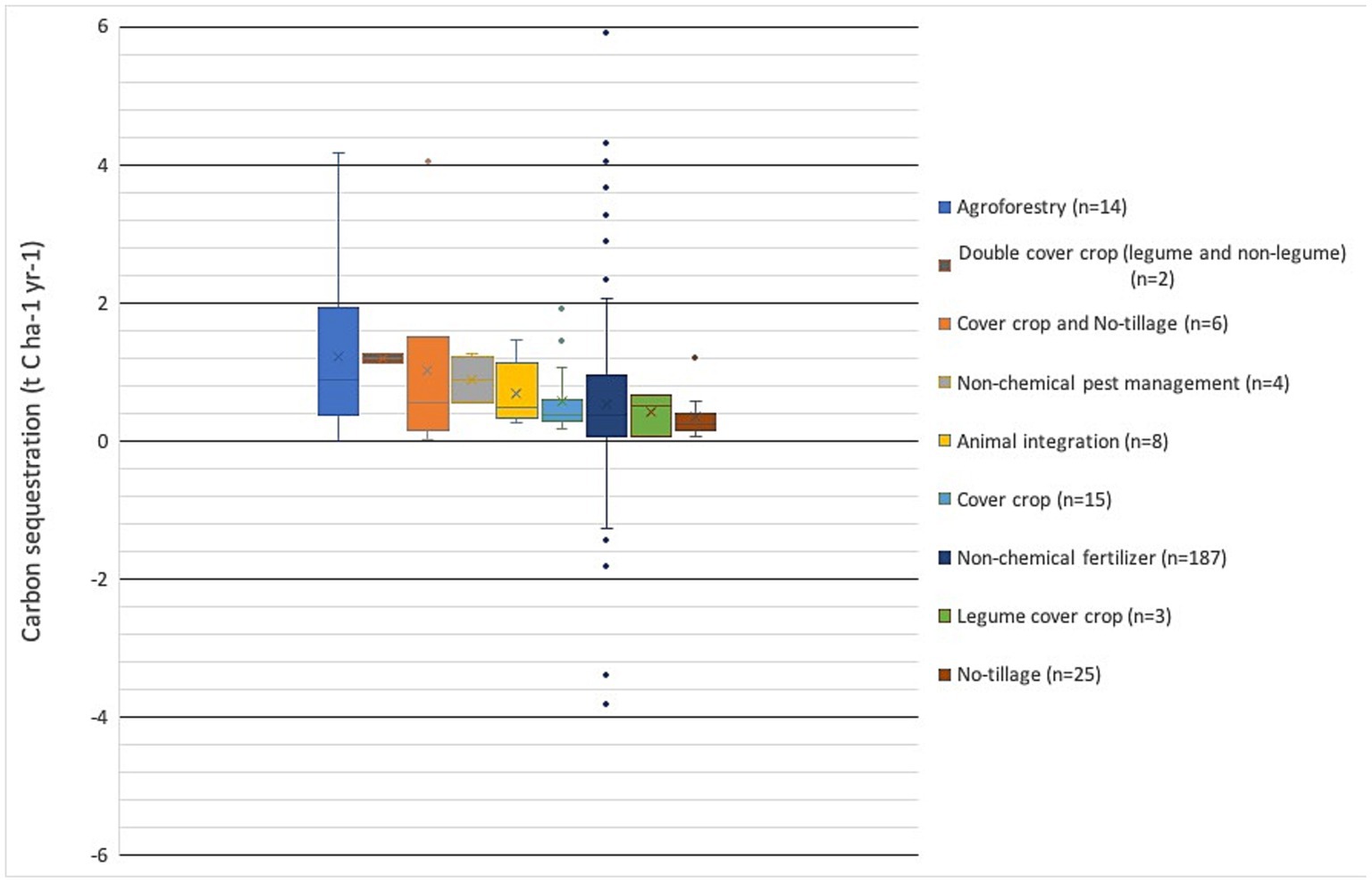
Figure 2. Below-ground C sequestration rates for regenerative practices on arable land. Boxplots represent the 25th–75th percentile of data, the “x” represents the mean, and horizontal lines represent the median. Each dot represents averages calculated in one independent study, with the total number of studies for each practice shown in the legend. Positive values represent a below-ground carbon sink, while negative values represent C emissions.
3.2 Woody perennial land use
For woody perennial land use, animal integration had a mean sequestration rate of 2.05 t C/ha/yr. (N = 4), and non-chemical pest management 1.86 t C/ha/yr. (N = 1) (Figure 3). Combining a cover crop and no-tillage (N = 2) had a mean sequestration rate of 1.43 t C/ha/yr., while the use of a cover crop alone (N = 6) had a mean of 1.31 t C/ha/yr. The use of a legume cover crop (N = 2) and no-tillage alone (N = 5) had a mean sequestration rate of 0.75 and 0.73 t C/ha/yr., respectively, while agroforestry (N = 5) had a mean of 0.67 t C/ha/yr. Non-chemical fertilizer had a mean sequestration rate of 0.04 t C/ha/yr. (N = 56), and included the lowest estimate (emitting 6.5 t C/ha/yr).
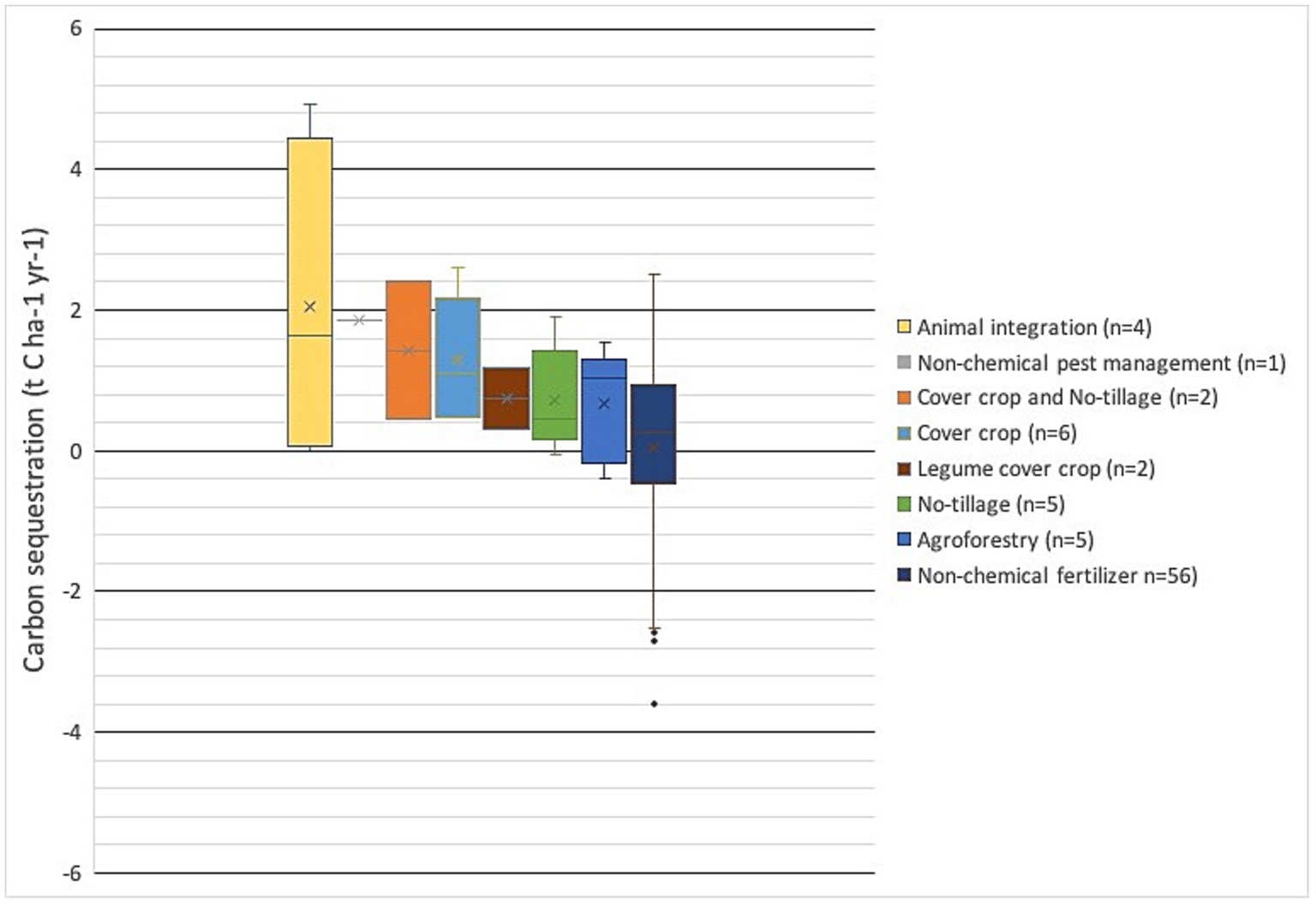
Figure 3. Below-ground C sequestration rates for regenerative practices on woody perennial land. Boxplots represent the 25th–75th percentile of data, the “x” represents the mean, and horizontal lines represent the median. Each dot represents averages calculated in one independent study, with the total number of studies for each practice shown in the legend. Positive values represent a below-ground carbon sink, while negative values represent C emissions.
3.3 Comparing sequestration in arable and woody perennial land use
We found generally higher sequestration rates for regenerative practices in woody perennials (average across practices: 1.10) compared with arable land use (average across practices: 0.76) (Figure 4). Paired t-tests between arable and woody perennial land use revealed that although only the averages for non-chemical fertilizer were significantly different, animal integration yielded more than twice the mean carbon sequestration in woody perennial as in arable cropland (Figure 4). Non-chemical pest management sequestered 71% and the use of a cover crop sequestered 77% more in woody perennial than arable cropland.
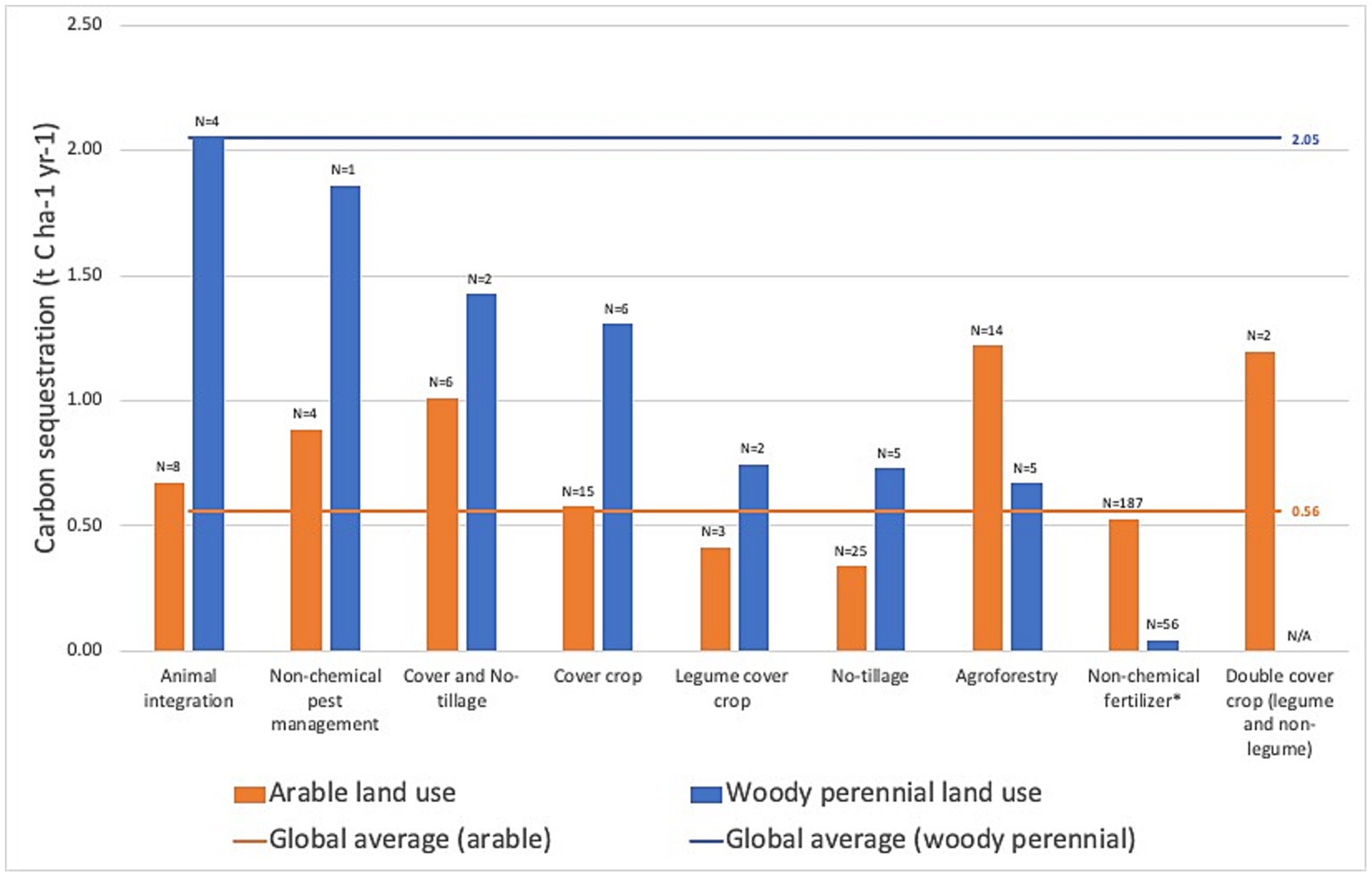
Figure 4. Average C sequestration per land use of various soil regeneration practices. Positive values represent a below-ground carbon sink. “N” equals the number of samples from studies where a measurable carbon sequestration figure was found. The only statistically significant difference between practices on different land uses was for non-chemical fertilizer (denoted with *). The global average for arable land uses comes from Zomer et al. (2017) and for woody perennials from Payen et al. (2021).
The average sequestration rate from our dataset was lower for woody perennials than the 2.05 t C/ha/yr. reported as the global average by Payen et al. (2021) (Figure 4), whose study was based on a literature search of worldwide soil organic C sequestration in experimental studies using the following vineyard carbon sequestration practices: cover crops, no-tillage, amendments, biochar application, hedging and agroforestry (Payen et al., 2021). However, our data was higher for arable croplands than the global average of 0.56 t C/ha/yr. reported by Zomer et al. (2017) (Figure 4) whose study was based on soil organic C sequestration data found for cropland in global geospatial datasets, and using current status and a 20-year modeling scenario to show the potential increase from adopting practices including organic manures, cover cropping, mulching, conservation tillage, agroforestry, and rotational grazing (Zomer et al., 2017).
Our results indicate that combining practices that foster biodiversity rather than applying them individually may increase carbon sequestration. For example, for both arable and woody perennial land, combining the two practices of cover crop and no-tillage yielded a higher mean belowground sequestration value, at 1.01 t C/ha/yr. and 1.43 t C/ha/yr., than a cover crop (leguminous or not) or no tillage alone (Figures 2, 3). For arable land, double cover crops (one legume and one non-legume) yielded a higher mean sequestration rate (1.20 t C/ha/yr) than either (non-legume) cover cropping (0.58) or legume cover crops (0.41) individually (Figure 2).
For the seven regenerative practices we were able to quantify, we found that on average, all practices sequestered carbon. The only practice to show a statistically significant difference between land uses was non-chemical fertilizer, where sequestration was higher on arable land (Figure 4).
4 Discussion
In this study, we investigated the soil carbon sequestration potential of seven regenerative farming practices—agroforestry, cover cropping, legume cover cropping, animal integration, non-chemical fertilizer, non-chemical pest management, and no tillage—for both arable and woody perennial land. Although generally small sample sizes hindered detecting any statistically significant differences in soil organic carbon sequestration between the various regenerative practices, our results indicate that all seven practices contribute to below-ground carbon sequestration on average.
We found that some practices exhibited higher mean sequestration rates for particular land uses (agroforestry for arable and animal integration for woody perennial land). Interestingly, we found that combining certain practices yielded higher mean soil carbon sequestration rates than when applying them separately. For instance, the combination of cover crops and no-tillage resulted in a higher mean soil carbon sequestration rate than when these practices were applied individually. Additionally, double cover cropping (combining one legume and one non-legume) resulted in a higher mean soil carbon sequestration rate in arable land compared to either cover crop alone (Figure 2). Overall, our findings suggest that the combined application of multiple regenerative farming practices may lead to greater carbon sequestration outcomes.
Notably, our study suggests that all practices, except for non-chemical fertilizer and legume cover crop, have the potential to sequester more carbon in woody perennial than arable cropland soils. This result is expected, as arable systems tend to experience both lower belowground carbon inputs and higher and more frequent soil disturbances compared to orchards and vineyards, leading to increased soil respiration and carbon emissions. This finding is particularly relevant for viticulture, which has been identified as a potential carbon sink, and indicates the importance of adopting regenerative practices to enhance carbon uptake.
Overall, our results provide valuable insights into the potential benefits of regenerative farming practices for carbon sequestration. While we did not observe statistically significant differences in the carbon sequestration potential of various practices, our study highlights the potential of using a combination of regenerative practices to sequester soil carbon.
4.1 Comparison with previous literature
The high C sequestration for animal integration for woody perennials (Figure 3), the figures for which came mostly from studies of vineyards, aligns with findings of applying animal manure sequestering 20% more soil organic C than applying crop residues (Bolinder et al., 2020). Previous studies have shown additional benefits from integrating animals beyond carbon sequestration, including pest and fire prevention and reduced fertilizer and pesticide requirements from cattle grazing (Barbosa et al., 2019), as well as reduced nitrate leaching into groundwater, pests, and erosion, and increased water retention and nitrogen fixation from including perennial forages and incorporating animal manure (Asbjornsen et al., 2014). However, the overall impacts of integrated crops and animals largely depend on the interaction between grazing management and intensity (Lazcano et al., 2022).
For arable croplands, our finding that agroforestry sequesters a high amount of soil organic C fits with previous literature. This previous literature shows that combining woody perennials with other crops can increase soil carbon storage via net primary production, and through the return of tree litter to the earth, root and rhizosphere decomposition, and non-tillage of areas around woody plants (Chenu et al., 2014). This is also the case for traditional polyculture agroecosystems, such as olive-grapevine groves in Italy, where soil C was higher than in either crop grown intensively alone as a monoculture (Brunori et al., 2020). Increasing plant diversity can increase microbial biomass and therefore soil organic C (Chen et al., 2019). Allowing biodiversity to regenerate can also help strengthen vineyard pest resilience by regulating the ecosystem and make it difficult for pests to establish themselves (Guo et al., 2019).
Our study indicated that combining practices may increase C sequestration. For example, a combination of both a cover crop and no-tillage can be beneficial for mycorrhizal fungi development, thereby contributing to carbon sequestration. Symbiotic neighboring plants such as cover crops, interrow grass cover and certain weeds can allow arbuscular mycorrhizae to develop (Trouvelot et al., 2015). These mycorrhizae contribute not only to C reserve mobilization but also to plant development and prevention of soil erosion and water loss, which can otherwise be a limiting factor in biomass accumulation (including soil C) (Trouvelot et al., 2015). Meanwhile, no tillage has been found to allow mycorrhiza to form with less disturbance, even when rotating crops (Moitinho et al., 2020).
Our results for arable land indicate that a double cover crop (combining one legume and one non-legume) sequesters more soil C than either individually, and further studies may be able to show that the result also applies to viticulture, for the result is in line with recent studies that have shown that mixing grass and legume cover crops in vineyards worldwide with different soil types increases not only nitrogen but also soil organic carbon stocks (Ball et al., 2020).
Combining regenerative practices can help farmers combat climate change and biodiversity loss and help them move to what Soloviev and Landua (2016) call the second, multifunctional stage of regenerative farming. In this stage, an optimal mix of multifunctional practices, crop species, and carbon-sequestering strategies is adopted for the farm’s ecology, focused on improving the vitality of entire living ecosystems in soil and beyond (Soloviev and Landua, 2016). Indeed, regenerative practices may work best in concert (Figure 4) (Trouvelot et al., 2015; Teague, 2018), and combining soil regenerative practices that foster biodiversity rather than applying them individually may sequester more carbon. The long-term Jena Experiment shows that carbon storage strongly increases with increasing plant species richness (Weisser et al., 2017). Applying animal integration, non-chemical pest management, diverse cover crops, agroforestry, non-chemical fertilizer, and redesigning the system at the landscape level can all contribute to this species richness. Research is also emerging on the greater resilience to climate change of perennial plant communities with high species diversity, due to the greater probability of including species with traits adapted to climate change (Asbjornsen et al., 2014).
4.2 Practices contribute differently to C sequestration in woody perennials
Woody perennial (including vineyard) soil generally exhibited higher increases in carbon sequestration with regenerative practices than arable agricultural soil, although the only statistically significant difference for a practice between land uses was for non-chemical fertilizer, which sequestered more carbon in arable cropland (Figure 4). Considering our sample size was an order of magnitude larger for this practice thanks to data from a meta study on organic farming (Gattinger et al., 2019), significant differences were easier to detect. However, it should be noted that Gattinger et al. (2019) considered organic fertilizer in the form of slurry or stacked manure to be non-chemical fertilizer, whereas for our analysis, we considered only compost or waste products from crop residues as non-chemical fertilizer, and considered manure application as the practice of animal integration. Indeed, regenerative agriculture as a closed-loop system would tend to use only manure produced on the farm, but organic agriculture [the focus of the Gattinger et al. (2019) study] allows the import of external sources of manure and slurry, which may have contributed to the net C emissions we observed for the negative outliers for organic farms using imported non-chemical fertilizer in Figures 2, 3. Regardless of the source, the amount of fertilizer incorporated must be carefully monitored to avoid problems related to overuse including nutrient loss, soil acidification or alkalinization, reduction of microbial communities, and water contamination (Han et al., 2016).
The combination of no-tillage and the use of a cover crop appeared to have a slightly greater effect in woody perennials (Figure 4). While arable crops often have their roots disturbed or removed and have to start from scratch each year, woody perennials like grapevines have the time to develop symbiotic arbuscular mycorrhizae. These mycorrhizae enhance the vine’s ability to obtain water and nutrients from the soil, and to collect carbon to store below ground (Trouvelot et al., 2015). No tillage in combination with cover crops that allow mycorrhizae to colonize roots may therefore contribute to higher carbon sequestration, especially in perennial crops like grapes that stay rooted in the ground longer.
4.3 Soil-regenerative practices as part of a greater system
Regenerative practices not only potentially work in concert to sequester more soil organic C, but are part of a whole system that includes biodiversity, human health and well-being, long-term economic health and more. Drawing together our findings, we visualized a framework for how the different soil regenerative practices and their extractive opposites could affect outcomes such as biodiversity, soil carbon sequestration, long-term agricultural productivity, and human health and wellbeing (Figure 5). The different regenerative practices we quantified here act as levers to increase the rate of soil organic C sequestration (inflow). Meanwhile, their opposites, for which we do not have C emission data, are hypothesized to act as levers to increase the rate of carbon emissions into the atmosphere (outflow). For example, as the reinforcing green arrows from animal integration show, well-managed animal integration contributes positively to outcomes such as biodiversity and increasing the carbon sink rate (which in turn augments the soil carbon stock). In contrast, high nitrogen fertilizer input tends to decrease biodiversity, as shown by the detracting red arrow. Note the reinforcing loop with two positive flows between the outcomes of long-term vineyard productivity and human health and well-being, showing that the two are mutually dependent on one another, notably when the human in question is the grower.
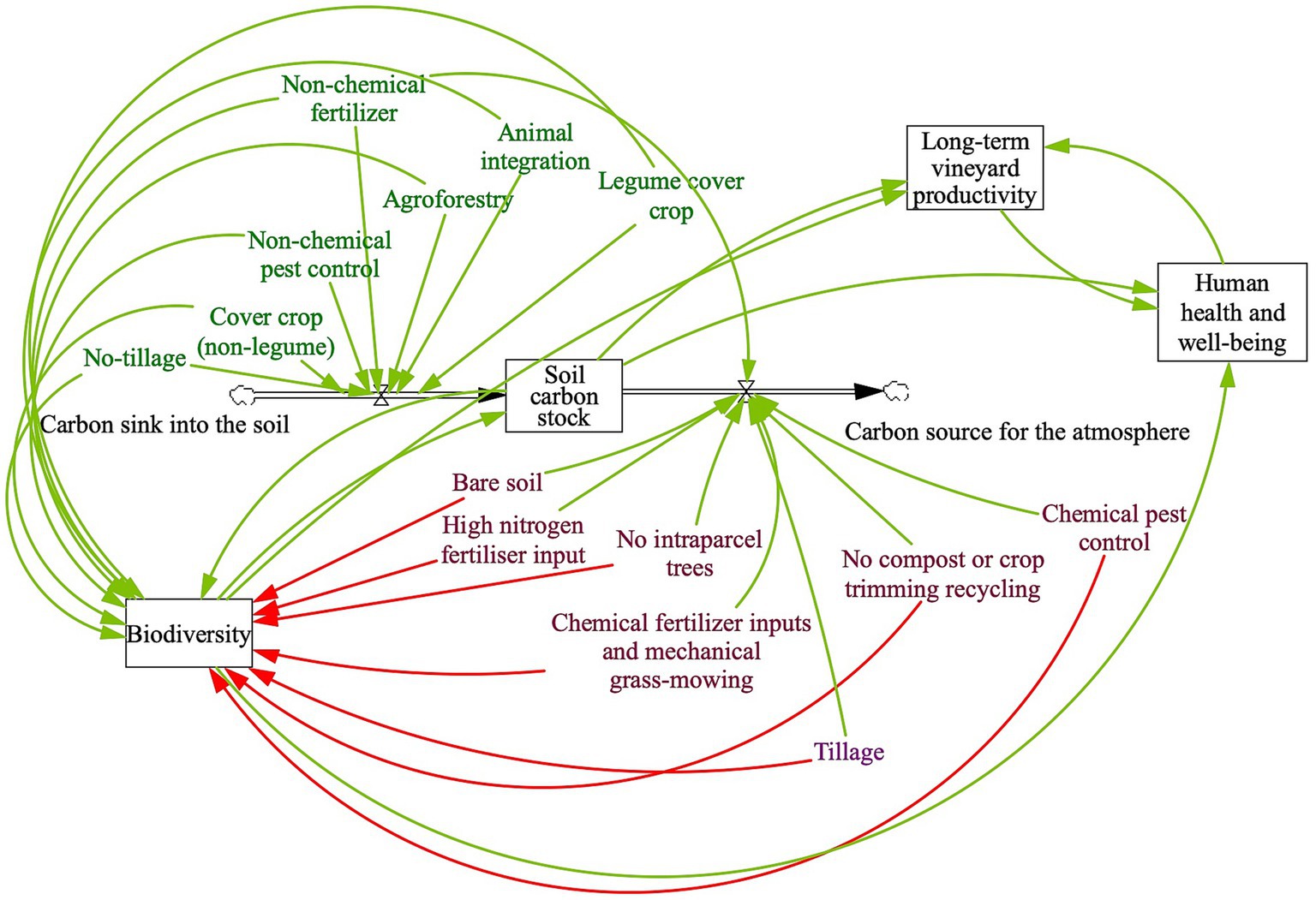
Figure 5. Outcomes of Regenerative vs. Non-Regenerative Farming. Visualized with practices (text in color), outcomes (boxes), and flows (arrows). Green text denotes the seven regenerative practices we found C sequestration data for in this study, and the purple text their opposites. Green arrows denote a reinforcing flow effect, while red arrows denote a dampening effect. Data from the soil carbon sequestration impact of the seven practices, and inferred based on the literature review. Source: authors’ own drawing using Vensim (Smart et al., 2019).
In sum, carbon sequestration and soil management practices are pieces of a bigger regenerative ecosystem. Even though reversing biodiversity loss is central to regenerative agriculture, it is often disregarded in favor of other soil health indicators (such as carbon sequestration) when discussing recommended practices (Giller et al., 2021). However, adopting soil regenerative practices has the potential to help regenerate degraded biodiversity, increase soil carbon sequestration for climate mitigation, ensure long-term farm or vineyard productivity, and secure human health and wellbeing. On arable croplands, agroforestry may sequester slightly more carbon, and in woody perennials, animal integration may sequester slightly more than other practices. Overall, we found evidence that any of the seven soil regenerative practices we studied can increase soil C sequestration; combining practices may further strengthen carbon sequestration and other elements of a healthy ecosystem. Because woody perennials, such as grapevines, sequester more soil organic C than arable land uses, they may be a particularly favorable crop to lead the way for regenerative agriculture.
4.4 Farming recommendations
To make best use of limited resources, winegrowers and other farmers might consider a sequenced adoption approach for regenerative practices that balances the effectiveness of carbon sequestration with the perceived ease of adoption (Villat, 2021). Visualizing the average soil C sequestration of each practice (from the average values combining arable and woody perennials from Figure 4) and the perceived ease of adoption of the practice based on interviews with 20 winegrowers in Switzerland (Villat, 2021), we recommend growers start with potential quick wins, where soil C sequestration potential is highest and the practices are perceived as easiest to adopt (upper right quadrant, Figure 6). This quadrant includes the combined use of a cover crop and no-tillage, non-chemical pest management, the use of a cover crop, and the use of a double cover crop (combining a legume and a non-legume). The top left quadrant, including agroforestry and animal integration, represents practices that need promotion, or need to be made easier, for they are perceived as more difficult to adopt but represent high soil C sequestration potential. Incentives or strategies for enabling these practices are therefore important. The C sequestration potential of redesigning the system at the landscape level and reduced traffic is unknown and farmers perceive them as difficult to adopt; further research is needed to quantify their outcomes before these processes are prioritized. Finally, practices that are perceived as easy to adopt but whose carbon sequestration potential is relatively lower need less time spent on incentives or promotion (lower right quadrant).
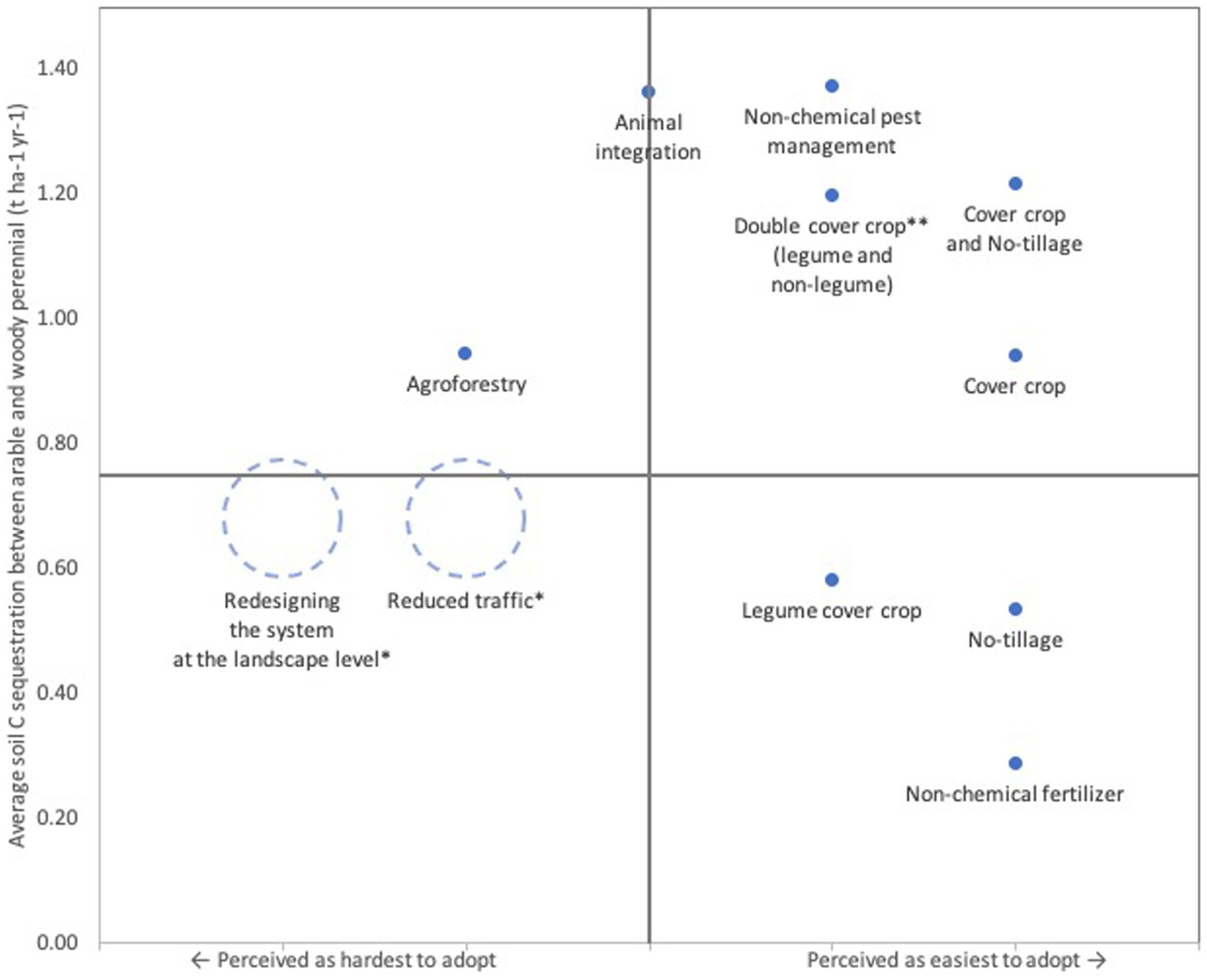
Figure 6. Regenerative Practices, Their Ease of Adoption and C Sequestration. The ease of adoption is based on an analysis of 20 interviews with winegrowers in Switzerland (Villat, 2021). *No C sequestration figures were available for redesigning the system at the landscape level and reduced traffic, so to include them, we positioned them randomly at the halfway C sequestration point. **The C sequestration figure for the use of a double cover crop is only for arable land use, and unknown for woody perennials.
Based on current research and evidence, we advise farmers and policymakers to prioritize the adoption of effective and feasible agricultural practices. These may include a combination of using cover crops and no-till methods, implementing non-chemical pest management strategies, and utilizing double (legume and non-legume) cover crops. Additionally, attention should be directed toward the support and development of animal integration methods, which have been proven to be effective in terms of carbon sequestration but may be met with resistance from farmers. It is crucial to recognize the potential benefits of these practices and provide adequate resources to facilitate their implementation.
4.5 Future research
Although robust measurements are needed to back up the claimed benefits of regenerative agriculture, available data are often not sufficient to do so. Data demonstrating the beneficial outcomes for many soil health practices remains a challenge (Mattila et al., 2022). It is notable that the practices with the largest sample sizes had the lowest estimated C sequestration rates (Figures 2, 4), so it is important to be cautious about the quantitative potential for regenerative practices to sequester carbon until more data are available.
In this study, we were able to provide a robust estimate of the impact of non-chemical fertilizer on soil carbon sequestration (N = 243), and reasonably robust estimates for three practices in arable crops: no-till (N = 25), cover crop (N = 15), and agroforestry (N = 14) (Table 2). Three of the remaining single practices (legume cover crop, animal integration, and non-chemical pest management) and two combined practices [combining a legume and non-legume cover crop (double cover crop) and combining cover crop and no-tillage] were only quantified in a handful of cases, particularly for woody perennial systems (Table 2). Two of the nine regenerative practices we identified from the literature (low traffic and redesigning the system at the landscape level) did not have any quantification of their effect on soil carbon outcomes available in the literature. More studies are needed to assess carbon sequestration potential for these practices, to achieve greater statistical power and higher confidence in sequestration rates. There is a particular need for more studies in woody perennial systems such as vineyards, and particularly from long-term experimental and monitoring studies and randomized controlled trials, which despite difficulties in detecting small changes in soil carbon pools in agricultural soils, provide a necessary and valuable complement to modeling estimates. Now that we have identified relevant regenerative practices, we recommend future synthesis studies search the existing literature for observed sequestration rates of specific regenerative practices by name, building off of the practices listed in Table 2 (e.g., searching for “animal integration,” “low traffic,” etc., and related synonyms).
Ideally, we would have analyzed soil C rates according to soil depth measured, length of study, bulk density, and soil type. However, 28% of studies did not report soil depth at which soil C was measured, and 22% did not report study length, despite reliable soil C figures only accruing over several years. Many studies did not report bulk density, making it difficult to estimate the extent of the effect of bulk density on reported soil C accrual rates. Soil type was also not always reported, either. Often these were studies measuring a number of other factors aside from soil C. Moreover, in the studies synthesized we found a range of 14 different units used to estimate soil C, from t C ha−1 y−1 to SOC % increase per year, to grams per kilogram increases, all which had to be converted to the t C ha−1 y−1 standard for comparability. For better comparability, we recommend that future studies measuring soil C use the international measurement unit “t C ha−1 y−1” and report as much information as possible, but at least measurement depth, bulk density and soil type.
5 Conclusion
Current conventional soil management practices must evolve for climate change to be addressed and for nature, including people, to thrive. Adopting soil regenerative practices has many potential benefits, including soil organic C sequestration, that contribute to the European Union’s goal of increasing healthy soils 75% by 2030. All seven regenerative farming practices investigated in the study contributed to below-ground carbon sequestration on average, and have the potential to support climate goals and additional outcomes such as biodiversity, long-term soil productivity, and human health and wellbeing. Regenerative farming practices may be more effective at carbon sequestration when applied in a holistic manner, with the combined application of multiple practices leading to more significant carbon sequestration outcomes. Finally, most of the practices showed the potential to sequester more soil organic C in woody perennial than arable cropland, indicating that woody crops such as grapevines could be a particularly favorable crop to lead the way for regenerative agriculture.
The agricultural sector, and notably viticulture because of its innovation and carbon sequestration potential, have an essential role to play in transforming the food system that has been responsible for the degradation of soil in past generations. By nurturing or transitioning to regenerative farming practices, much of the Earth’s topsoil can be regenerated rather than degraded (Scharmer, 2020), thereby improving both soil and human health. In its ideal form, regenerative agriculture thrives together with a regenerative culture, including ritual, foods, ceremonies, songs, stories, music and all the things that embed agriculture in a supportive and meaningful community, without which farming is socially isolated, and hard work (Soloviev and Landua, 2016). Adopting regenerative practices and carefully documenting their outcomes for soil, biodiversity, and other goals can help turn agriculture from one of the largest contributors of anthropogenic greenhouse gas emissions to a system of delivering ecosystem and health benefits for nature including people.
Data availability statement
The original contributions presented in the study are included in the article/Supplementary material. Further inquiries can be directed to the corresponding author.
Author contributions
JV: conceptualization, methodology, analysis, and writing – original draft. KN: conceptualization, methodology, review, and supervision. All authors contributed to the article and approved the submitted version.
Acknowledgments
We would like to thank Mark Leighton from Harvard University for providing inspiration, crucial guidance and hope for this study and for all things regenerative. We would also like to thank Martin Schlaepfer from the University of Geneva, and Niki Rust from the UK Department for Environment, Food and Rural Affairs, for offering feedback at the early conceptualization stage of this study, and David Montgomery for inspiring research on soil. We would also like to thank all the researchers whose prior studies on soil organic carbon enabled us to compile this study, and all those who continue to give living soil the front seat it deserves. Open access for this article was generously funded by Lund University.
Conflict of interest
The authors declare that the research was conducted in the absence of any commercial or financial relationships that could be construed as a potential conflict of interest.
Publisher’s note
All claims expressed in this article are solely those of the authors and do not necessarily represent those of their affiliated organizations, or those of the publisher, the editors and the reviewers. Any product that may be evaluated in this article, or claim that may be made by its manufacturer, is not guaranteed or endorsed by the publisher.
Supplementary material
The Supplementary material for this article can be found online at: https://www.frontiersin.org/articles/10.3389/fsufs.2023.1234108/full#supplementary-material
Supplementary Data | This data sheet shows the list of N=345 soil carbon sequestration samples found in the literature search and used as C sequestration measures in the study. The samples span seven regenerative practices – agroforestry, cover cropping, legume cover cropping, animal integration, non-chemical fertilizer, non-chemical pest management, and no tillage. Sequestration figures (t C ha/yr) in the data sheet are shown by study, practice, and land use.
References
ADEME . (2013). Contribution de l’ADEME à l’élaboration de visions énergétiques 2030–2050. Paris: The French Agency for Ecological Transition. Available at: https://www.ademe.fr/sites/default/files/assets/documents/85536_vision_2030-2050_document_technique.pdf (Accessed July 10, 2020).
Al-Kaisi, M. M., and Lal, R. (2020). Aligning science and policy of regenerative agriculture. Soil Science Society of America Journal 84, 1808–1820. doi: 10.1002/saj2.20162
Asbjornsen, H., Hernandez-Santana, V., Liebman, M., Bayala, J., Chen, J., Helmers, M., et al. (2014). Targeting perennial vegetation in agricultural landscapes for enhancing ecosystem services. Renew. Agric. Food Syst. 29, 101–125. doi: 10.1017/S1742170512000385
Ball, K. R., Baldock, J. A., Penfold, C., Power, S. A., Woodin, S. J., Smith, P., et al. (2020). Soil organic carbon and nitrogen pools are increased by mixed grass and legume cover crops in vineyard agroecosystems: detecting short-term management effects using infrared spectroscopy. Geoderma 379:114619. doi: 10.1016/j.geoderma.2020.114619
Barbosa, O., Colson, D., Godoy, K., Jones, A., Jones, G., Harris, M., et al. (2019). A natural capital approach to landscape planning: a pilot project in Colchagua Valley, Chile 32 (JNCC Report 634; p. 32). JNCC.
Beach, H. M., Laing, K. W., Walle, M. V. D., and Martin, R. C. (2018). The current state and future directions of organic no-till farming with cover crops in Canada, with case study support. Sustainability 10:373. doi: 10.3390/su10020373
Berge, H. F. M. ten, Schoeder, J. J., Oleson, J. E., and Giraldez Cervera, J. V. (2017). Research for AGRI Committee - Preserving agricultural soils in the EU. European Parliament, Policy Department for Structural and Cohesion Policies, Brussels.
Blum, Z.-B., Zechmeister-Boltenstern, S., and Keiblinger, K. M. (2019). Does soil contribute to the human gut microbiome? Microorganisms 7:287. doi: 10.3390/microorganisms7090287
Bolinder, M. A., Crotty, F., Elsen, A., Frac, M., Kismányoky, T., Lipiec, J., et al. (2020). The effect of crop residues, cover crops, manures and nitrogen fertilization on soil organic carbon changes in agroecosystems: a synthesis of reviews. Mitigat. Adapt. Strat. Glob. Change 25, 929–952. doi: 10.1007/s11027-020-09916-3
Brevik, E. C., Slaughter, L., Singh, B. R., Steffan, J. J., Collier, D., Barnhart, P., et al. (2020). Soil and human health: current status and future needs. Air Soil Water Res. 13:117862212093444. doi: 10.1177/1178622120934441
Brunori, E., Maesano, M., Moresi, F. V., Matteucci, G., Biasi, R., and Mugnozza, G. S. (2020). The hidden land conservation benefits of olive-based (Olea europaea L.) landscapes: An agroforestry investigation in the southern Mediterranean (Calabria region, Italy). Land Degrad. Dev. 31, 801–815. doi: 10.1002/ldr.3484
Casarejos, F. (2020). Casting long-term and regenerative perspectives on global sustainability through systems and resilience thinking. Sustainability 12:1230. doi: 10.3390/su12031230
Chen, C., Chen, H. Y. H., Chen, X., and Huang, Z. (2019). Meta-analysis shows positive effects of plant diversity on microbial biomass and respiration. Nat. Commun. 10:1332. doi: 10.1038/s41467-019-09258-y
Chen, W., Meng, H., Song, H., and Zheng, H. (2022). Progress in dust modelling, global dust budgets, and soil organic carbon dynamics. Land 11:176. doi: 10.3390/land11020176
Chenu, C., Klumpp, K., Bispo, A., Angers, D., Colnenne, C., and Metay, A. (2014). Stocker du carbone dans les sols agricoles: évaluation de leviers d’action pour la France. Innovat. Agronom. 37, 23–37.
Diti, I., Legler, S. E., Caffi, T., Rossi, V., Canali, G., Bosso, A., et al. (2020). A new integrated approach for management of soil threats in the vineyard ecosystem. Catena 195:104788. doi: 10.1016/j.catena.2020.104788
Ehrlén, J., and Lehtilä, K. (2002). How perennial are perennial plants? Oikos 98, 308–322. doi: 10.1034/j.1600-0706.2002.980212.x
European Commission (2020). Caring for soil is caring for life: Ensure 75% of soils are healthy by 2030 for healthy food, people, nature and climate: Interim report of the mission board for soil health and food. Luxembourg: Publications Office of the European Union. Available at: https://data.europa.eu/doi/10.2777/918775 (Accessed May 14, 2021).
FAO . (2019). The state of the world’s biodiversity for food and agriculture. eds. J. Bélanger and D. Pilling. Rome: FAO Commission on Genetic Resources for Food and Agriculture Assessments. Available at: http://www.fao.org/3/CA3129EN/CA3129EN.pdf
FAO, ITPS . (2020). Global soil organic carbon map V1.5: technical report. Food and agriculture Organization of the United Nations.
FAO, ITPS, GSBI, CBD, EC . (2020). State of knowledge of soil biodiversity - status, challenges and potentialities, report 2020. FAO: Rome.
Fierer, N. (2017). Embracing the unknown: disentangling the complexities of the soil microbiome. Nat. Rev. Microbiol. 15, 579–590. doi: 10.1038/nrmicro.2017.87
Fitzgerald, D. (2021). What is regenerative technology? [WWW document]. Medium. Available at: https://medium.com/regen-ventures/what-is-regenerative-technology-1adb697299ea (Accessed November 6, 2021).
Gattinger, A., Krause, H.-M., Mayer, J., van der Heijden, M. G. A., Mäder, P., Skinner, C., et al. (2019). The impact of long-term organic farming on soil-derived greenhouse gas emissions. Sci. Rep. 9:1702. doi: 10.1038/s41598-018-38207-w
Georgiou, K., Jackson, R. B., Vindušková, O., Abramoff, R. Z., Ahlström, A., Feng, W., et al. (2022). Global stocks and capacity of mineral-associated soil organic carbon. Nat. Commun. 13:3797. doi: 10.1038/s41467-022-31540-9
Giffard, B., Winter, S., Guidoni, S., Nicolai, A., Castaldini, M., Cluzeau, D., et al. (2022). Vineyard management and its impacts on soil biodiversity, functions, and ecosystem services. Front. Ecol. Evol. 10:850272. doi: 10.3389/fevo.2022.850272
Giller, K. E., Hijbeek, R., Andersson, J. A., and Sumberg, J. (2021). Regenerative Agriculture: An agronomic perspective. Outlook Agric. 50, 13–25. doi: 10.1177/0030727021998063
Gosnell, H., Gill, N., and Voyer, M. (2019). Transformational adaptation on the farm: processes of change and persistence in transitions to ‘climate-smart’ regenerative agriculture. Glob. Environ. Chang. 59:101965. doi: 10.1016/j.gloenvcha.2019.101965
Guo, Q., Fei, S., Potter, K. M., Liebhold, A. M., and Wen, J. (2019). Tree diversity regulates forest pest invasion. PNAS 116, 7382–7386. doi: 10.1073/pnas.1821039116
Han, S. H., An, J. Y., Hwang, J., Kim, S. B., and Park, B. B. (2016). The effects of organic manure and chemical fertilizer on the growth and nutrient concentrations of yellow poplar (Liriodendron tulipifera Lin.) in a nursery system. For. Sci. Technol. 12, 137–143. doi: 10.1080/21580103.2015.1135827
LaCanne, C. E., and Lundgren, J. G. (2018). Regenerative agriculture: merging farming and natural resource conservation profitably. PeerJ 6:e4428. doi: 10.7717/peerj.4428
Larbodière, L., Davies, J., Schmidt, R., Magero, C., Vidal, A., Aroyo Schnell, A., et al. (2020). Notre terrain d’entente: rétablir la santé des terres pour une agriculture durable. International Union for Conservation of Nature: Gland.
Lazcano, C., Gonzalez-Maldonado, N., Yao, E. H., Wong, C. T. F., Merrilees, J. J., Falcone, M., et al. (2022). Sheep grazing as a strategy to manage cover crops in Mediterranean vineyards: short-term effects on soil C, N and greenhouse gas (N2O, CH4, CO2) emissions. Agric. Ecosyst. Environ. 327:107825. doi: 10.1016/j.agee.2021.107825
Ledo, A., Smith, P., Zerihun, A., Whitaker, J., Vicente-Vicente, J. L., Qin, Z., et al. (2020). Changes in soil organic carbon under perennial crops. Glob. Chang. Biol. 26, 4158–4168. doi: 10.1111/gcb.15120
Mattila, T. J., Hagelberg, E., Söderlund, S., and Joona, J. (2022). How farmers approach soil carbon sequestration? Lessons learned from 105 carbon-farming plans. Soil Tillage Res. 215:105204. doi: 10.1016/j.still.2021.105204
Moitinho, M. R., Fernandes, C., Truber, P. V., Marcelo, A. V., Corá, J. E., and da Silva Bicalho, E. (2020). Arbuscular mycorrhizal fungi and soil aggregation in a no-tillage system with crop rotation. J. Plant Nutr. Soil Sci. 183, 482–491. doi: 10.1002/jpln.201900456
Newton, P., Civita, N., Frankel-Goldwater, L., Bartel, K., and Johns, C. (2020). What is regenerative agriculture? A review of scholar and practitioner definitions based on processes and outcomes. Front. Sustain. Food Syst. 4:577723. doi: 10.3389/fsufs.2020.577723
Oenema, O., Heinen, M., Peipei, Y., Rietra, R., and Hessel, R. (2017). A review of soil-improving cropping systems (Scientific report No. 06) Wageningen Environmental Research.
Olsson, L., Barbosa, S., Bhadwal, A., Cowie, K., Delusca, D., Flores-Renteria, K., et al. (2019). “Land degradation” in Climate change and land: An IPCC special report on climate change, desertification, land degradation, sustainable land management, food security, and greenhouse gas fluxes in terrestrial ecosystems
Payen, F. T., Sykes, A., Aitkenhead, M., Alexander, P., Moran, D., and MacLeod, M. (2021). Soil organic carbon sequestration rates in vineyard agroecosystems under different soil management practices: a meta-analysis. J. Clean. Prod. 290:125736. doi: 10.1016/j.jclepro.2020.125736
Pellerin, S., Bamière, L., Launay, C., Martin, R., and Schiavo, M. (2020). Stocker du carbone dans les sols français. Quel potentiel au regard de l’objectif 4 pour 1000 et à quel coût ? Research Report. Paris: INRA, p. 528. Available at: https://hal.archives-ouvertes.fr/hal-03163517
Pisciotta, A., Di Lorenzo, R., Novara, A., Laudicina, V. A., Barone, E., Santoro, A., et al. (2021). Cover crop and pruning residue management to reduce nitrogen mineral fertilization in Mediterranean vineyards. Agronomy 11:164. doi: 10.3390/agronomy11010164
Regeneration International . (2017). What is regenerative agriculture? Available at: https://regenerationinternational.org/wp-content/uploads/2017/02/Regen-Ag-Definition-2.23.17-1.pdf (Accessed April 14, 2021).
Rockström, J., Edenhofer, O., Gaertner, J., and DeClerck, F. (2020). Planet-proofing the global food system. Nat. Food 1, 3–5. doi: 10.1038/s43016-019-0010-4
Scandellari, F., Caruso, G., Liguori, G., Meggio, F., Palese Assunta, M., Zanotelli, D., et al. (2016). A survey of carbon sequestration potential of orchards and vineyards in Italy. Eur. J. Horticult. Sci. 81, 106–114. doi: 10.17660/eJHS.2016/81.2.4
Scharmer, O. (2020). Global climate action I: the power of soil [WWW document]. Medium. Available at: https://medium.com/presencing-institute-blog/global-climate-action-l-the-power-of-soil-a951a3668b65 (Accessed April 19, 2020).
Scholes, R., Huang, Y., Roué, M., Saw, L. G., and Mketeni, F. G. (2018). IPBES (2018): Summary for policymakers of the assessment report on land degradation and restoration of the intergovernmental science-policy platform on biodiversity and ecosystem services.
Scott, E. I., Toensmeier, E., Iutzi, F., Rosenberg, N. A., Lovell, S. T., Jordan, N. R., et al. (2022). Policy pathways for perennial agriculture. Front. Sustain. Food Syst. 6:983398. doi: 10.3389/fsufs.2022.983398
Soloviev, E. R., and Landua, G. (2016). Levels of regenerative agriculture. Ithaca, New York: Terra Genesis International. Available at: https://static1.squarespace.com/static/58510fc42e69cfbfaadb37af/t/588e6ffcc534a52f7732c60b/1485729821343/LevelsofRegenerativeAgricultureFINAL.pdf (Accessed April 5, 2021).
Swiss Confederation . (2020). Swiss Geoportal [WWW Document]. Available at: https://map.geo.admin.ch (October 7, 2020).
Teague, W. R. (2018). Forages and pastures symposium: managing grazing to restore soil health and farm livelihoods. J. Anim. Sci. 96, 1519–1530. doi: 10.1093/jas/skx060
Trouvelot, S., Bonneau, L., Redecker, D., van Tuinen, D., Adrian, M., and Wipf, D. (2015). Arbuscular mycorrhiza symbiosis in viticulture: a review. Agron. Sustain. Dev. 35, 1449–1467. doi: 10.1007/s13593-015-0329-7
United Nations Environment Programme , (2021). Adaptation gap report 2020. Nairobi: United Nations Environment Programme.
van Diemen, R., Matthews, J. B. R., Möller, V., Fuglestvedt, J. S., Masson-Delmotte, C., Mendez, A., et al. (Eds). (2023). IPCC, 2022: Annex I: Glossary, in: IPCC, 2022: Climate change 2022: Mitigation of climate change. Contribution of Working Group III to the Sixth Assessment Report of the Intergovernmental Panel on Climate Change. Cambridge University Press, Cambridge, New York, NY, pp. 1793–1820.
Villat, J. (2021). Down to earth: Identifying and promoting regenerative viticulture practices for soil and human health. Boston, Massachusetts: Harvard University. Available at: https://dash.harvard.edu/handle/1/37370042
Weisser, W. W., Roscher, C., Meyer, S. T., Ebeling, A., Luo, G., Allan, E., et al. (2017). Biodiversity effects on ecosystem functioning in a 15-year grassland experiment: patterns, mechanisms, and open questions. Basic Appl. Ecol. 23, 1–73. doi: 10.1016/j.baae.2017.06.002
Wiesmeier, M., Urbanski, L., Hobley, E., Lang, B., von Lützow, M., Marin-Spiotta, E., et al. (2019). Soil organic carbon storage as a key function of soils - a review of drivers and indicators at various scales. Geoderma 333, 149–162. doi: 10.1016/j.geoderma.2018.07.026
Xue, L., Ren, H., Brodribb, T. J., Wang, J., Yao, X., and Li, S. (2020). Long term effects of management practice intensification on soil microbial community structure and co-occurrence network in a non-timber plantation. For. Ecol. Manag. 459:117805. doi: 10.1016/j.foreco.2019.117805
Zehetner, F., Djukic, I., Hofmann, R., Kühnen, L., Rampazzo-Todorovic, G., Gerzabek, M. H., et al. (2015). Soil organic carbon and microbial communities respond to vineyard management. Soil Use Manag. 31, 528–533. doi: 10.1111/sum.12204
Keywords: regenerative agriculture, soil organic carbon, living soil, regenerative viticulture, farm practices, cover crop, agroforestry
Citation: Villat J and Nicholas KA (2024) Quantifying soil carbon sequestration from regenerative agricultural practices in crops and vineyards. Front. Sustain. Food Syst. 7:1234108. doi: 10.3389/fsufs.2023.1234108
Edited by:
B. Mohan Kumar, Arunachal University of Studies, IndiaReviewed by:
João Coutinho, University of Trás-os-Montes and Alto Douro, PortugalRodolfo Lizcano Toledo, Tolima University, Colombia
Copyright © 2024 Villat and Nicholas. This is an open-access article distributed under the terms of the Creative Commons Attribution License (CC BY). The use, distribution or reproduction in other forums is permitted, provided the original author(s) and the copyright owner(s) are credited and that the original publication in this journal is cited, in accordance with accepted academic practice. No use, distribution or reproduction is permitted which does not comply with these terms.
*Correspondence: Kimberly A. Nicholas, a2ltYmVybHkubmljaG9sYXNAbHVjc3VzLmx1LnNl
†ORCID: Kimberly A. Nicholas https://orcid.org/0000-0002-4756-7851