- 1Food Science Program, Division of Food, Nutrition and Exercise Sciences, University of Missouri, Columbia, MO, United States
- 2Department of Chemical and Biomedical Engineering, University of Missouri, Columbia, MO, United States
Introduction: Disposal of the acid whey waste stream is one of the major challenges faced by the Greek yogurt industry. However, based on its physicochemical characteristics and composition analysis, it has huge potential in the health industry. Likewise, millets are highly nutritive and health benefitting ancient grains. Combining acid whey and millet matrix can help in solving the problem of both food losses and food security. Hence, the objective of the study is to analyze the enhancement or degradation of the nutrition profile when acid whey is combined with millet matrix using spray-drying technology.
Method: The methodology of the study includes composition analysis and functional characterization based on antioxidant properties, microbial analysis, and sensory evaluation.
Results and discussion: The results showed that there was enhancement of functional properties of the millet-acid whey formulation matrix specifically in terms of polyphenolic compounds, lactose content, and mineral content. Overall, the study aims to provide an alternative method for the utilization and consumption of acid whey that is currently being mishandled and considered as waste by the Greek yogurt manufacturing companies.
Highlights
– Acid whey can be upcycled for the development of nutritious functional food.
– Food matrix containing acid whey and millets are rich in polyphenolic compounds.
– The days of storage did not impact the yeast and mold count of the product.
– Acid whey and millet-acid whey matrix is perceived differently in terms of flavor sensory analysis.
1. Introduction
According to the Food and Agriculture Organization (FAO), a sustainable food system is defined as a food system that aims to achieve food security and nutrition fulfilling economic, social, and environmental bases. It should be inclusive of the marginalized population, should not cause harm to the environment and, at the same time, should be able to deliver healthy and nutritious food to the community (Nguyen, 2018). Upcycling of side streams of various food products can help in achieving a more sustainable food system (Askew, 2021).
The Greek yogurt industry faces extensive challenges in the disposal of acid whey which is a by-product in the straining step of production. Acid whey contains 6%–10% lactic acid by dry weight in comparison to sweet whey which only contains about 2% or less lactic acid by dry weight. Increased lactic acid concentration hinders protein aggregation by hydrophobic interactions and induces protein denaturation. Even though the acid whey currently being dumped into the surroundings, acid whey as an ingredient, has the potential for providing nutritional benefits. It has a rich protein profile with α-Lactalbumin, β-Lactoglobulin, bovine serum albumin (BSA), and lactoferrin. It has a considerable amount of lactose and a rich fatty acid profile (Erickson, 2017). Acid whey is also known to be a good medium for the growth of probiotic bacteria with a prolonged survival time (Skryplonek et al., 2019). Various studies have been conducted to find alternative method for acid whey utilization. For example, in a study conducted by Sady et al. (2013) it was found that orange and whey beverages could be a nutritious product in the developing market for functional foods. Acid whey can be used for in propionic acid fermentation which involves lactic acid as the carbon source. In a recent study conducted by Nani and Krishnaswamy (2023) acid whey was considered to be a viable replacement for titanium dioxide. Along the same lines, millets are one of the main underutilized crops whose proper utilization can help in strengthening food security. It has been demonstrated through various studies that millets are a good source of bioactive compounds. They can be consumed as a good source of protein, fiber, vitamins, and minerals. Acid whey and millets can be combined to form a shelf stable ingredient that can be used for production of various nutritious food products specifically complementary food powder (Malik et al., 2021).
The spray-drying method can be used for the encapsulation of bioactive compounds to allow for an improved retention and delivery times. These bioactive compounds include vitamins, phytochemicals, polyphenolic compounds, probiotics, and antibacterial agents. Microencapsulation using spray-drying includes mixing of a wall material with the suspension that contains the bioactive compounds prior to drying. The wall material usually consists of various kinds of carbohydrates, proteins and lipid compounds. It also includes cellulose material, such as carboxymethyl cellulose, ethyl cellulose butylate-pthalate, and gums such as sodium alginate, carrageenan, and gum Arabic (Kandasamy and Naveen, 2022). Millets contains a high amount of carbohydrates (63%–70%), including starch, cellulose, pentosans and free sugars (Wankhede et al., 1979). The protein content in millets is also of superior quality with vital amino acids present, such as lysine, valine, and threonine (Hassan et al., 2021). The alkalinity of millet grains helps in neutralization of acid whey that helps in enabling production of a free-flowing powder (Malik et al., 2021). The carbohydrate and protein content in millets can potentially enable the encapsulation process in the matrix. Based on the size the millets are divided into two categorizes; major and minor millets wherein the major varieties of millets include pearl, foxtail, finger, and proso. The minor varieties include barnyard, kodo, little and foxtail millet (Belton and Taylor, 2002; Amadoubr and Le, 2013; Shahidi and Chandrasekara, 2013).
Hence, the objective of the study was to conduct a nutritional characterization of a complementary food made from acid whey and millets (major variety; proso & minor variety; kodo) to evaluate the efficacy of a microencapsulation process for polyphenolic compounds. The study also aimed at conducting microbial shelf-life analysis and sensory evaluation of the developed food product to determine its commercial viability.
2. Materials and methods
2.1. Product development
2.1.1. Preparation of acid whey from Greek yogurt processing
Acid whey was separated from Greek yogurt prepared in the lab. The yogurt preparation was done by inoculating 10 g of yogurt starter culture into 1 L of pasteurized milk (Grade A, Vitamin D whole milk, Central Dairy, Columbia, MO, United States). The milk was first heated at 82.2°C and cooled to 43.3°C. After the milk cooled down, the starter culture containing some of the common starter cultures was added to it followed by incubation (45°C for 8 h). The starter culture consisted of Lactobacillus delbrueckii subsp. bulgaricus, Streptococcus salivarius subsp. thermophilus, and supplemental probiotic cultures, Lactobacillus bifidus, Lactobacillus acidophilus and Lactobacillus casei. After the yogurt was set, it was centrifuged (Beckman Coulter™ Model J6-MI, Brea, California, United States) at 3018.6 × g for 20 min at 4°C. Commercially centrifugal separators are used as they give considerable amount of control for ensuring safety of the product and increasing the yield. The whey was separated from the yogurt and filtered using a Whatman qualitative paper (Grade 4, pore size 25 μm). For prolonged storage, the acid whey was kept in a freezer at −18°C for 2 weeks and consequently recultured.
2.1.2. Millet processing
Millet grains in the form of powders were used for the experiment. Both kodo and proso millets were ground in a mixer grinder (Butterfly Rapid Mixer Grinder™, Butterfly Gandhimati Appliances®, Chennai, India) followed by sieving, using a 150 μm sieve in a sieve shaker (IKA-VIBRAX-VXR, Janke & Kunkel, Markham, ON, Canada).
2.1.3. Sample matrix preparation and spray-drying
The sample matrix was made by mixing millet powder in the acid whey [50 weight of powder (g)/Volume of acid whey (mL) %]. After mixing, the slurry was then passed through a vacuum filter using a Whatman qualitative paper (Grade 4, pore size 25 μm). The obtained filtrate was then further spray-dried at 140°C using a spray dryer (BUCHI, Mini Spray Dryer B-290, New Castle, DE, United States). Parameters, such as the flowmeter of air, feed flow rate and aspirator were adjusted for maximum yield. The spray-dried mixture of acid whey and kodo millets was termed KAW sample, while acid whey and proso millets was termed PAW sample.
2.2. Proximate analysis
The nutritional characterization was done by analyzing the composition of the spray dried powder (KAW50 & PAW50), and comparing them with the pure millet sample (kodo and proso). The proximate analysis was conducted for crude protein [Kjeldahl method, AOAC Official method 984.13 (A–D), 2006], crude fat [AOAC Official Method 920.39 (A)], crude fiber (AOAC Official Method 978.10, 2006) and ash content (AOAC Official Method 942.05). Further, the products were also analyzed for sugar profile and amino acid profile [AOAC Official method 982.30 E (A–C), 2006]. The method consisted of sample preparation by acid hydrolysis, oxidation, acid and alkaline hydrolysis. Eventually the total recovery is based on nitrogen content for each of the amino acid. The analysis was conducted by the Agricultural Experiment Station Chemical Laboratories, University of Missouri, Columbia. Mineral analysis was also conducted using Inductively Coupled Plasma-Optical Emission Spectroscopy (ICP-OES) at the Soil and Plant Testing Laboratory, University of Missouri.
2.3. Antioxidant properties
2.3.1. Thiobarbituric acid reactive substance analysis
Analysis of thiobarbituric acid reactive substance (TBARs) helps in the detection of lipid peroxidation. The assay measures malondialdehyde (MDA), which is one of the end products of lipid peroxidation. A pink colored chromogen is formed when MDA reacts with thiobarbituric acid (TBA) in the presence of heat. The MDA can be quantified using a regression equation using a calibration curve of MDA. For sample preparation, 0.5 g sample was mixed with 5 mL of 80% ethanol, the mixture vortexed for 2 min and kept at 25°C for 24 h in fume hood so that the maximum extraction could take place. Centrifugation of the sample was done at 3018.6 × g (5,000 rpm) for 15 min and the supernatant was collected. Further, 1 mL of the supernatant of each sample was mixed with 1 mL of 4 mM TBA followed by heating at 95°C for 60 min. The absorbance for the color was measured at wavelength 532 nm. The calibration curve was prepared by mixing 1 mL of different concentrations of (0.1 to 0.8 mM) MDA with 1 mL of TBA, respectively, followed by heating at 95°C for 60 min. For statistical analysis, one way ANOVA was conducted using the JMP software (Cary, NC, United States), and for the post-hoc test, Tukey’s honest significant difference test was used.
2.3.2. DPPH radical scavenging
1,1-diphenyl-2-picrylhydrazyl (DPPH) radical scavenging test is a colorimetric assay that can be used for estimating the radical scavenging ability of an extract. DPPH changes its color from violet to yellow when it reacts with an antioxidant compound. A higher intensity of yellow color indicates a higher concentration of antioxidants. The sample preparation method used was similar to the method used for TBARs analysis (section 3.3.1), The supernatant (0.2 mL) was then mixed with 1 mL of 0.5 mmol/L solution of DPPH and 2 mL of ethanol. The incubation of the solution was done for 30 min at 25°C in a dark place. After 30 min, the absorbance was measured at 517 nm using a spectrophotometer (Nanodrop oneC, Thermofisher Scientific, Waltham, Massachusetts, United States), and compared with blank. For statistical analysis, one-way ANOVA was conducted using the JMP software (Cary, NC, United States) and for the post-hoc test, Tukey’s honest significant difference test was used.
2.3.3. Total polyphenol content
The phenolic compounds were analyzed using the Folin–Ciocalteu method (Singleton and Rossi, 1965). The method aims to quantify phenolic compounds by measuring the absorption of a blue-colored solution that gets its color from the formation of the phosphomolybdic-photungstic-phenol complex. The complex is formed when the active phenolic extracts react with the Folin–Ciocalteu reagent in an alkaline medium. Further, the total phenolic content can be calculated by using the regression equation obtained from the calibration curve wherein it is expressed as gallic acid equivalent (GAE) milligram per gram of the extract. The sample preparation method was similar to the method used for TBARs and DPPH analysis (section 3.3.1). The sample was then centrifuged at 3018.6 × g (5,000 rpm) for 15 min. Two milliliters of the extract were then taken and mixed with 0.5 mL Folin–Ciocalteu reagent and 2 mL of 20% sodium bicarbonate solution. The absorption readings were taken after 30 min in a spectrophotometer (Nanodrop oneC, Thermofisher Scientific, Waltham, Massachusetts, United States) at wavelength 765 nm. For statistical analysis, one-way ANOVA was conducted using the JMP software (Cary, NC, United States), and for the post-hoc test, Tukey’s honest significant difference test was used.
2.3.4. In vitro digestibility of total polyphenolic compounds
The in-vitro digestibility analysis for polyphenolic compounds was conducted following the protocol published by Minekus et al. (2014). The initial sample was prepared by mixing 1 g powder with 5 mL of DI water. The solution was further mixed with 3.5 mL of electrolyte solution. A mortar and pestle were used for grinding the food until a paste like consistency was achieved. To the paste, simulated salivary fluid and salivary α-amylase of activity 75 U/mL was added, which was followed by the addition of 25 μL of 0.075 mM calcium chloride. The whole solution was maintained at pH 7 and kept in the incubator at 37°C for 2 min. After 2 min, to the 10 mL of oral bolus was mixed with 7.5 mL of simulated gastric electrolyte solution, followed by the addition of Porcine pepsin of activity 2000 U/mL and 5 μL of 0.075 M of calcium chloride. The solution was maintained at pH 3 by the addition of 1 M hydrochloric acid and incubated for 2 h at 37°C. For the intestinal phase, 20 mL gastric chyme was mixed with 11 mL of intestinal electrolyte solution, followed by the addition of porcine pancreatin of activity 100 U/mL and lipase of activity 2000 U/mL, 2.5 mL bile salts and 1.31 mL of DI water. The intestinal solution was maintained at pH 7 by the addition of 1 M sodium hydroxide, and incubated for 2 h at 37°C. From each phase after incubation, 1 mL of solution was drawn for total polyphenol extraction. The obtained sample was then analyzed using the Folin–Ciocalteu method as before. Table 1 summarizes the simulated conditions for the in vitro digestion in terms of volume used, electrolyte and enzyme composition.
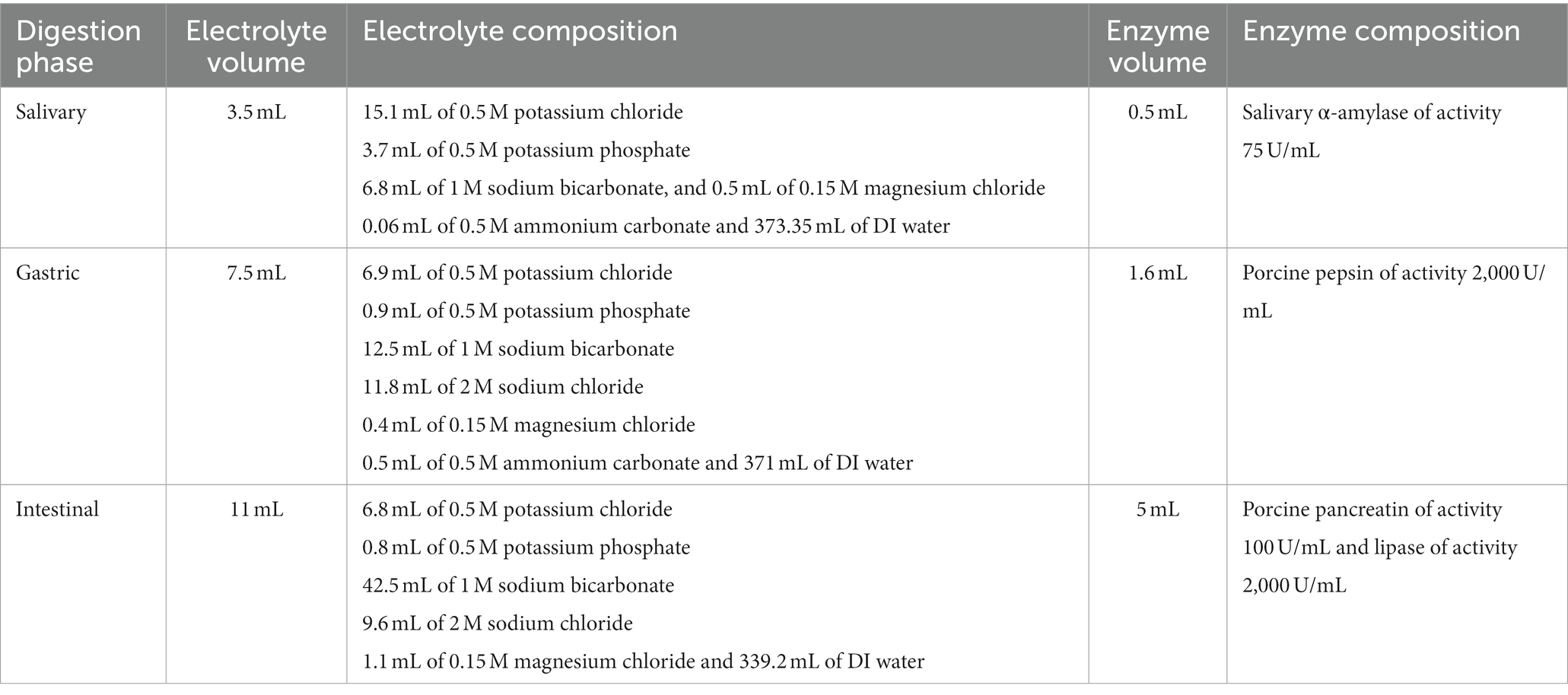
Table 1. Simulated conditions for in-vitro digestion analysis for salivary, gastric and intestinal phases Minekus et al. (2014).
2.4. Antinutritional factors: tannin content
Vanillin hydrochloride reagent was used for quantification of tannins (Broadhurst and Jones, 1978; Bharudin et al., 2013). For preparation of vanillin reagent, 8% hydrochloric acid in methanol and 4% vanillin in ethanol solution were mixed together in equal volumes. Sample preparation was done by mixing 0.5 g of the spray-dried sample with 80% methanol for 6–8 h. After the extraction time, the solution was centrifuged. The supernatant was then combined with the reagent in 1 to 5 ratio. After mixing with reagent, the absorbance was noted using a spectrophotometer (Nanodrop oneC, Thermofisher Scientific, Waltham, Massachusetts, United States) at wavelength 500 nm. The calibration curve was made by mixing catechin with the reagent. For statistical analysis, one-way ANOVA was conducted using the JMP software (Cary, NC, United States), and for the post-hoc test, Tukey’s honest significant difference test was used.
2.5. Microbial analysis for shelf life
For the end-of-shelf life, microbiology analysis was conducted for the samples to analyze the growth of bacteria, yeast, and mold. Buffered peptone water (Millipore Sigma, Burlington, MA) was used as the dilution buffer for diluting samples before plating. Plate count analysis was conducted using Standard plate count agar (Oxoid, ON K2G 1E8, Canada) and for yeast and molds, potato dextrose agar (Millipore Sigma, Burlington, MA) was used. The plates were incubated at 30°C–35°C for 72 h. For statistical analysis, one-way ANOVA was conducted using the JMP software (Cary, NC, United States), and for the post-hoc test, Tukey’s honest significant difference test was used. The samples used for analysis included the spray-dried powders (KAW & PAW) and vitamin-encapsulated spray-dried powders. The vitamin-encapsulated powders were prepared by mixing all the three components (millet powder, folic acid, and acid whey) using a homogenizer (IKA Eurostar 40, Wilmington, NC, United States) at 1,000 rpm for 30 min. Two separate slurries were formulated wherein one contained kodo millets (KAWF) and the other contained proso millets (PAWF). The slurries were then passed through a vacuum filtration process and the filtered liquid was collected and spray-dried.
2.6. Sensory analysis
The Quantitative Descriptive Analysis (QDA®) technique was used for sensory evaluation. The technique consisted of first training the panelist and then conducting the test (IRB Review # 380735). The training consisted of two sessions of 1 h each. The first session included all the participants who were selected to serve on the panel participating in a discussion lead by the panel leader. The discussion consisted of creation of vocabulary (lexicons) of the perceived attributes of a food product. The second training session consisted of tasting various food products that helped establish a relative judgement of the food. The judgement was formed on the attributes that were decided in the previous training sessions. The testing session included giving samples of different formulations to the panelist wherein they provided their feedback on the sample based on the relative judgement that was established in the previous training session.
Sample tested by panelist:
1. KAW: 30 g KAW sample was mixed with 300 mL water. Further, the sample was heated to 90°C for duration of 10 min followed by addition of sugar and 3 mL banana flavor.
2. PAW: 30 g PAW sample was mixed with 300 mL water. Further, the sample was heated to 90°C for duration of 10 min followed by addition of sugar and 3 mL banana flavor
3. AW: the acid whey solution separated from yogurt was heated at 90°C for duration of 10 min followed by addition of sugar and banana flavor.
These samples were given random three-digit numbers. A 10 × 10 Latin square design following the serving pattern was generated for the 10 panelists, as shown in Table 2. The test session consisted of tasting 9 samples wherein the panelists were given graham crackers and water to clean their pallet in between samples. For statistical analysis, one-way ANOVA was conducted using the JMP software (Cary, NC, United States), and for the post-hoc test, Tukey’s honest significant difference test was used.
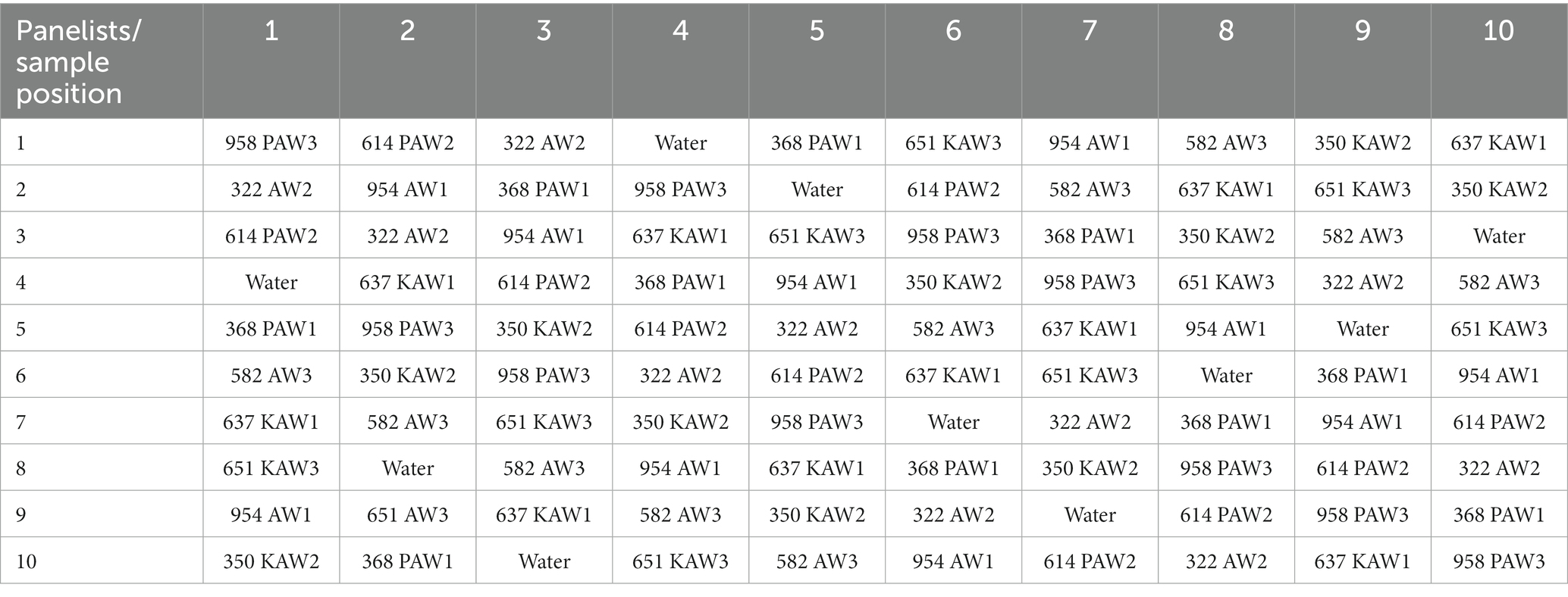
Table 2. Serving pattern generated for the 10 panelists for 9 samples using a 10 × 10 Latin square design.
During testing, 3 mL of each sample would be given in small food grade plastic cups. The panelist was asked to take a sip of the sample (1–2 mL) and give their feedback. The panelist provided the feedback independently at individual booth without reference.
3. Results and discussion
3.1. Proximate analysis
For crude protein analysis, the pure proso millet powder and proso acid whey spray-dried powder were observed to have a significantly higher protein content (p < 0.05), as shown in Figure 1. Proso millet is known to be an effective source of plant protein (9.5%–17%). For kodo millet, the protein content is found to be around 8.3% (Bunkar, 2021). The mixed matrix of acid whey and millet powder was observed to have a decreased amount of crude protein content (Figure 1). This can be attributed to the low pH of acid whey that denaturizes the weak hydrogen bonds in the protein structure (Wang et al., 2021).
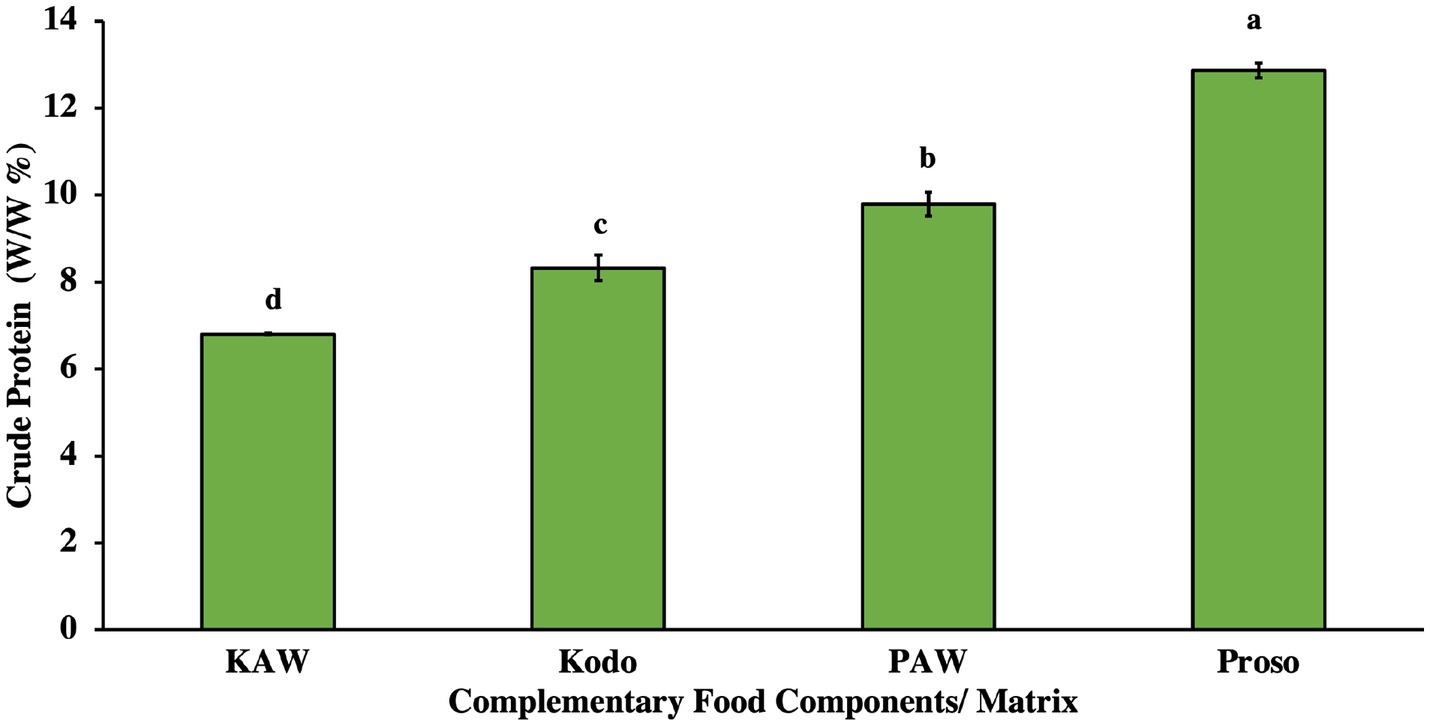
Figure 1. Crude protein analysis for pure millets (kodo & proso millets) and spray-dried powder containing acid whey and millets (KAW & PAW). Different lowercase letters on the bar graph denote significant differences between the protein content (p < 0.05) obtained using Tukey’s test.
Figure 2 summarizes the amino acid profile (essential and non-essential) of pure millet and spray dried powders. The proso millet protein is gluten-free and is rich in amino acids, such as leucine, phenylalanine, valine, isoleucine, and threonine. On the other hand, kodo millet is rich in essential amino acids such as leucine, phenylalanine, and valine. It is found to be deficient in tryptophan. For non-essential amino acids, a high amount of glutamic acid was observed. Glutamic acid functions as a neurotransmitter that is an intermediate compound for various important biochemical reactions. It is also a natural antioxidant in the body (Ubuka, 2021).
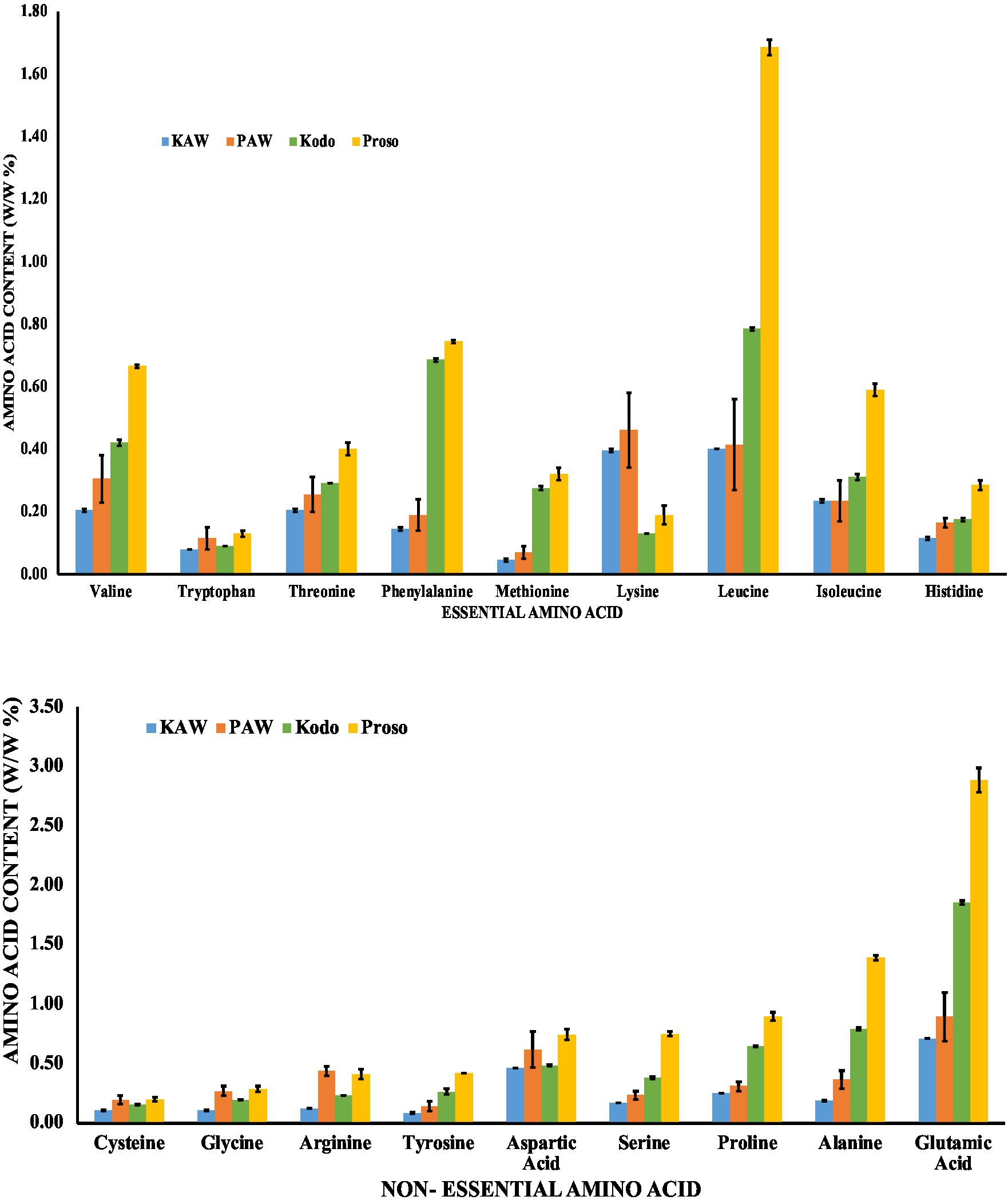
Figure 2. Amino acid analysis for pure millet powder (kodo & proso variety) and spray-dried powder containing acid whey and millets (KAW & PAW).
In terms of spray-dried powder, significantly lower amount of amino acid content was observed. Amino acids are susceptible to heat damage that further leads to oxidization and racemization (Hendriks, 2018). The results were consistent with the study conducted by Chen et al. (2021), that analyzed the effect of spray-drying and freeze-drying of Liluva. It was observed that there was a significant reduction of the amino acids, lysine and methionine, for the spray-dried powder.
In terms of crude fat, there was no significant difference observed (p > 0.05) between the pure millet powders and spray-dried powders, as shown in Figure 3. Crude fat includes a crude mixture of a fat-soluble material, ether extract or free lipid content. The lipid material include mono, di and triglycerides, free fatty acid, chlorophyll, fat soluble vitamins etc. Millets have been observed to contain a lower fat content, specifically kodo millets (1%). The fat in the millets consists of 78%–82% of unsaturated fatty acids and 17.9%–21.6% of saturated fatty acids (Chauhan et al., 2018).
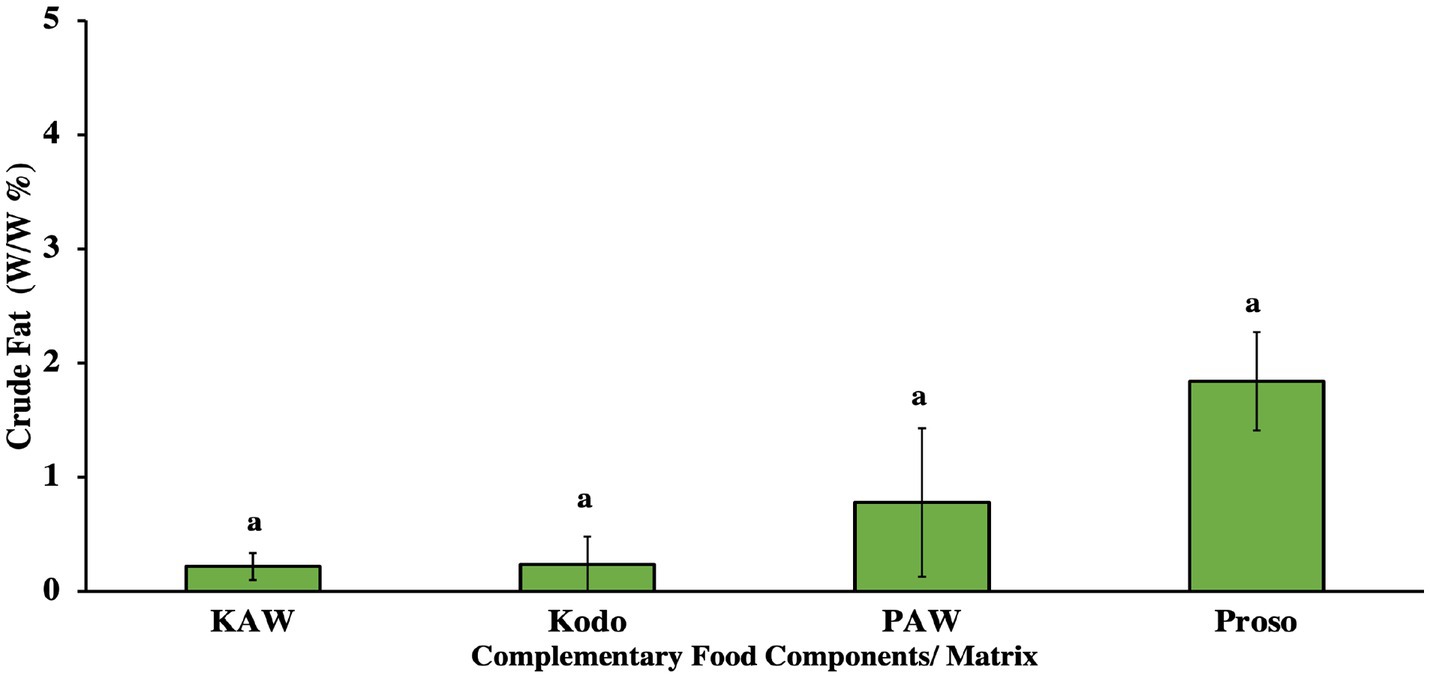
Figure 3. Crude fat analysis for pure millet powder (kodo & proso variety) and spray-dried powder containing acid whey and millets (KAW & PAW). Same lowercase letters on the bar graph denote that there was no significant difference obtained in the crude fat amount (p > 0.05) obtained using Tukey’s test.
Crude fiber consists of residues, such as cellulose and lignin, that remain after the food components are treated with acidic and alkali solutions. They are the major skeletal carbohydrates that are present in plant cells. Millets are known to have a higher quantity of fiber, when compared to rice. They have an abundance of lignocellulosic biomass that can further be used to isolate cellulose, hemicellulose and lignin. Among all millet varieties, pearl, foxtail and barnyard millet have a high amount of crude fiber percentage (Verma et al., 2015; Dresch et al., 2022). The crude fiber analysis conducted showed in Figure 4 there was significantly higher amount in the proso variety (p < 0.05) and there was no significant decrease in the spray-dried powder (p > 0.05).
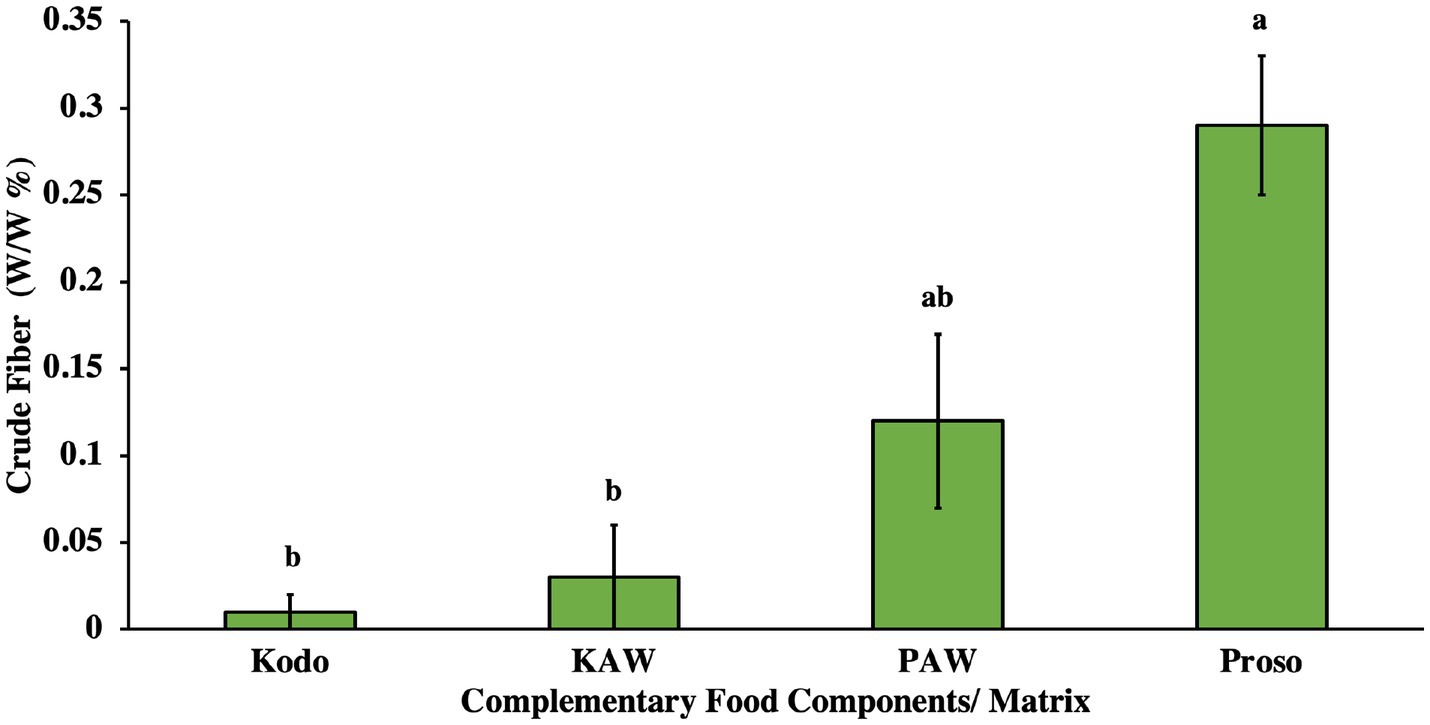
Figure 4. Crude fiber analysis for pure millet powder (kodo & proso variety) and spray-dried powder containing acid whey and millets (KAW & PAW). Different lowercase letters on the bar graph denote significant differences in the crude fiber content (p < 0.05) obtained using Tukey’s test.
In terms of sugar profile, for spray-dried powders, lactose was found to be in a higher content. A summary of sugar profile observed in the different products is shown in Table 3. The statistical analysis concluded that the sugar content of the spray-dried powder and the pure millet powder is significantly different (p < 0.05) (Table 3).
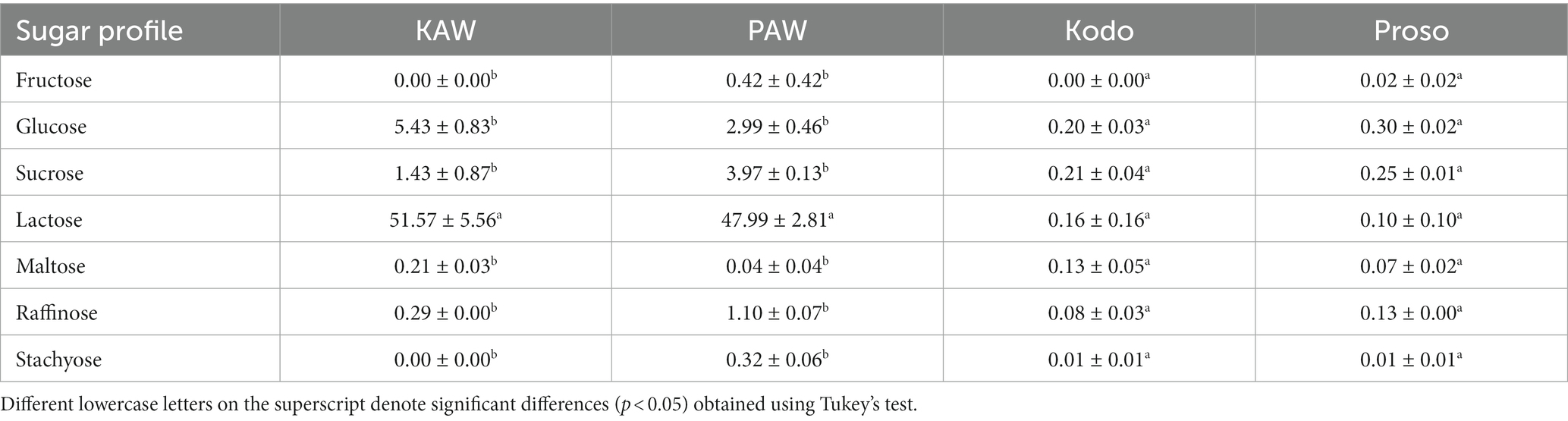
Table 3. Summary of sugar profile present in pure millet powder (kodo & proso variety) and spray-dried powder containing acid whey and millets (KAW & PAW).
The ash content in the spray-dried powder was observed to higher in the spray-dried samples, as shown in Figure 5 (p < 0.05). The ash content constitutes the inorganic materials, such as compound and essential minerals. This result can be attributed to the fact that acid whey consists of higher levels of acid that contribute to its acidic and salty taste (Lievore et al., 2015).
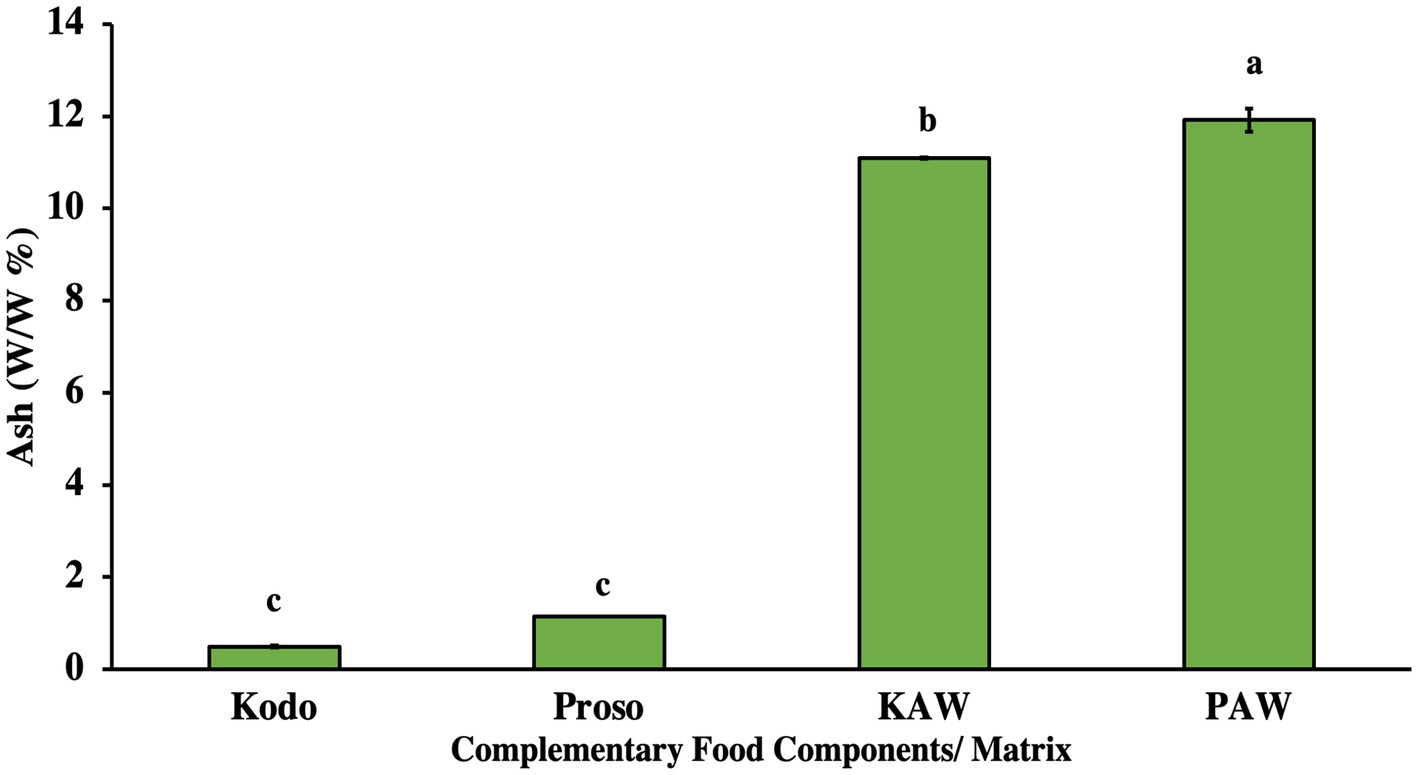
Figure 5. Ash content analysis (w/w %) for pure millet powder (kodo & proso variety) and spray-dried powder containing acid whey and millets (KAW & PAW). Different lowercase letters on the bar graph denote significant differences in the ash content (p < 0.05) obtained using Tukey’s test.
The major minerals present in the spray-dried powder include nitrogen (1.03%–1.76%), phosphorous (1.21%–1.33%), potassium (2.4%–2.94%), calcium (1.7%–1.86%), magnesium (0.31%–0.42%), zinc (42.8–96.8 ppm), iron (7.9–11.6 ppm), manganese (12.6–22.8 ppm), copper (11.9–19 ppm) and sodium (0.47–0.5 ppm).
3.2. Antioxidant properties
Oxidative stress is often linked with lifetime consequences. For children, damage from oxidative stress comes from two reasons; one from inadequate concentrations of antioxidants and the other is from an impaired ability to synthesize antioxidants. The oxidative stress-induced diseases include bronchopulmonary dysplacia, periventricular leukomalacia, etc. It also plays an important role in causing anxiety disorders in children (Guney et al., 2014; Hanson et al., 2016). Hence, a complementary food should have an adequate amount of antioxidants that can be consumed by children. In food, lipid oxidation causes major degradative processes that further lead to a loss of quality of the food. It also leads to the production of advanced lipid oxidation products that are cytotoxic and genotoxic (Kanner, 2007).
Dietary antioxidants can help in inhibition of lipid oxidation. They are either organic or inorganic compounds. Phenolic compounds constitute natural antioxidants that are either in the conjugated or complex form. The hydroxyl group on these compounds helps with the antioxidant property. The example of polyphenolic compounds include phenolic acids, lignans, tocopherols, carotenoids, flavonoids, flavones, isoflavones, and anthocyanidins (Stahl, 2000).
Millets contain different amount of bound and insoluble phenolic extracts that have antioxidant and metal-chelating properties. Chandrasekara and Shahidi (2010) investigated the quantity of soluble and insoluble-bound phenolic extracts obtained from different varieties of millets. It was observed that kodo millet had one of the highest amount of polyphenols (Chandrasekara and Shahidi, 2010). Proso millet primarily contains phenolic compounds, such as N′-caffeoyl-N″-feruloylspermidine, N′,N″-dicaffeoylspermidine and N-(p-coumaroyl) serotonin, chlorogenic acid in free fraction. In the bound fraction, it consists of ferulic and p-coumaric acid (Yuan et al., 2022).
Figure 6 summarizes the total polyphenol content in the samples wherein the spray-dried samples were observed to have a significantly higher polyphenol (p < 0.05) content as compared to the pure millet powders. Millets contain phenolic acids and flavonoids in their seed coats. The phenolic acid observed in millets include hydroxybenzoic and hydroxycinnamic acid. The content of the total phenol content varies with the millet variety and also depends on the kind of processing method. Methods, such as fermentation, have been found to increase the polyphenol content as it liberates the biologically active ingredient through the action of microorganisms.
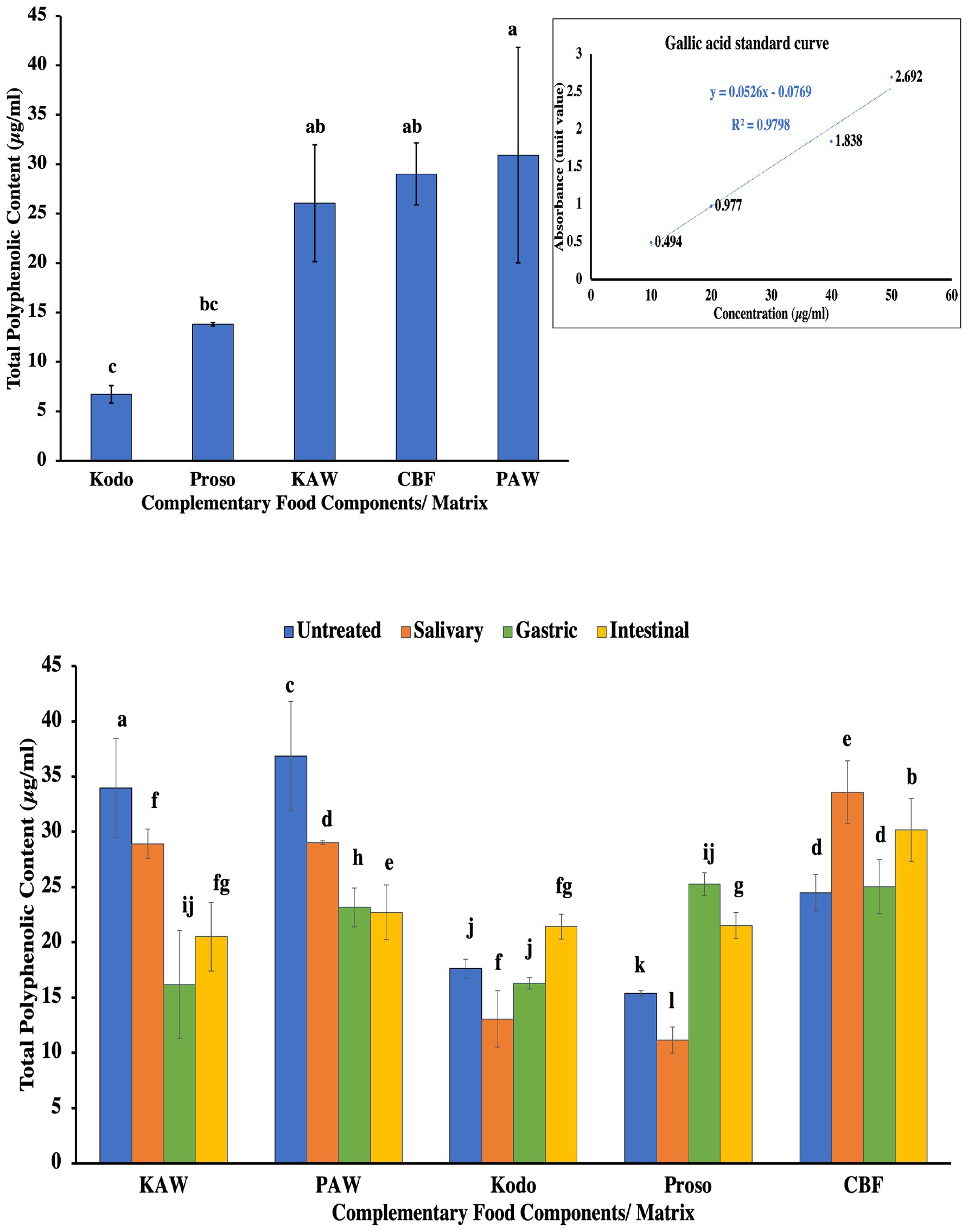
Figure 6. Total polyphenol content for pure millet powder (kodo & proso variety) and spray-dried powder containing acid whey and millets (KAW & PAW) and its in-vitro digestion in different simulated conditions (salivary, gastric and intestinal) compared with the commercial baby food (CBF). Different lowercase letters on the bar graph denote significant differences (p < 0.05) obtained using Tukey’s test.
The bioaccessibility of polyphenolic compounds changes as it goes through different phases of digestion. This is attributed to the interaction of these compounds with other components, such as suppressors, cofactors, and digestive enzymes. The other factors that impact the digestion include the plant matrix, and the complexity of the phytochemical and physiological conditions. Studies have reported that 42%–48% of polyphenols are digested in the intestinal phase. The release of phenolic compounds occur when millet grains are subjected to the different stages of simulated digestion. These released phenolic compounds exhibit different amounts of antioxidant activities wherein the variety of millet is also an important deciding factor (Wojtunik-Kulesza et al., 2020).
In the conducted experiment, it was observed that all the samples had different amounts of polyphenolic compounds with spray-dried proso-acid whey (PAW) containing the maximum amount. However, as the digestion occurred, a decrease in the content was observed. Likewise in spray-dried kodo-acid whey (PAW), a decrease was observed. However, in the pure millet powders, the digestion led to an increased polyphenolic content. This can be attributed to the presence of antinutritional factors that limit the bioactivity of the polyphenolic compounds. In commercial complementary foods, a high activity was observed in the salivary and intestinal phase.
Thiobarbituric acid reactive substances (TBARS) is a marker for oxidation stress induced by lipid peroxidation. In the tested samples, it was observed that the spray-dried proso acid whey sample (PAW) had significantly higher (p < 0.05) TBARS value followed by commercial baby food, spray-dried kodo-acid whey sample and pure millet powders, as shown in Figure 7. In terms of the 2,2-diphenyl-1-picrylhydrazyl (DPPH) assay, the commercial baby food was observed to have the highest amount of antioxidants, followed by the spray-dried powders, proso millet powder and kodo millet powder, as shown in Figure 7 (Shen et al., 2018).
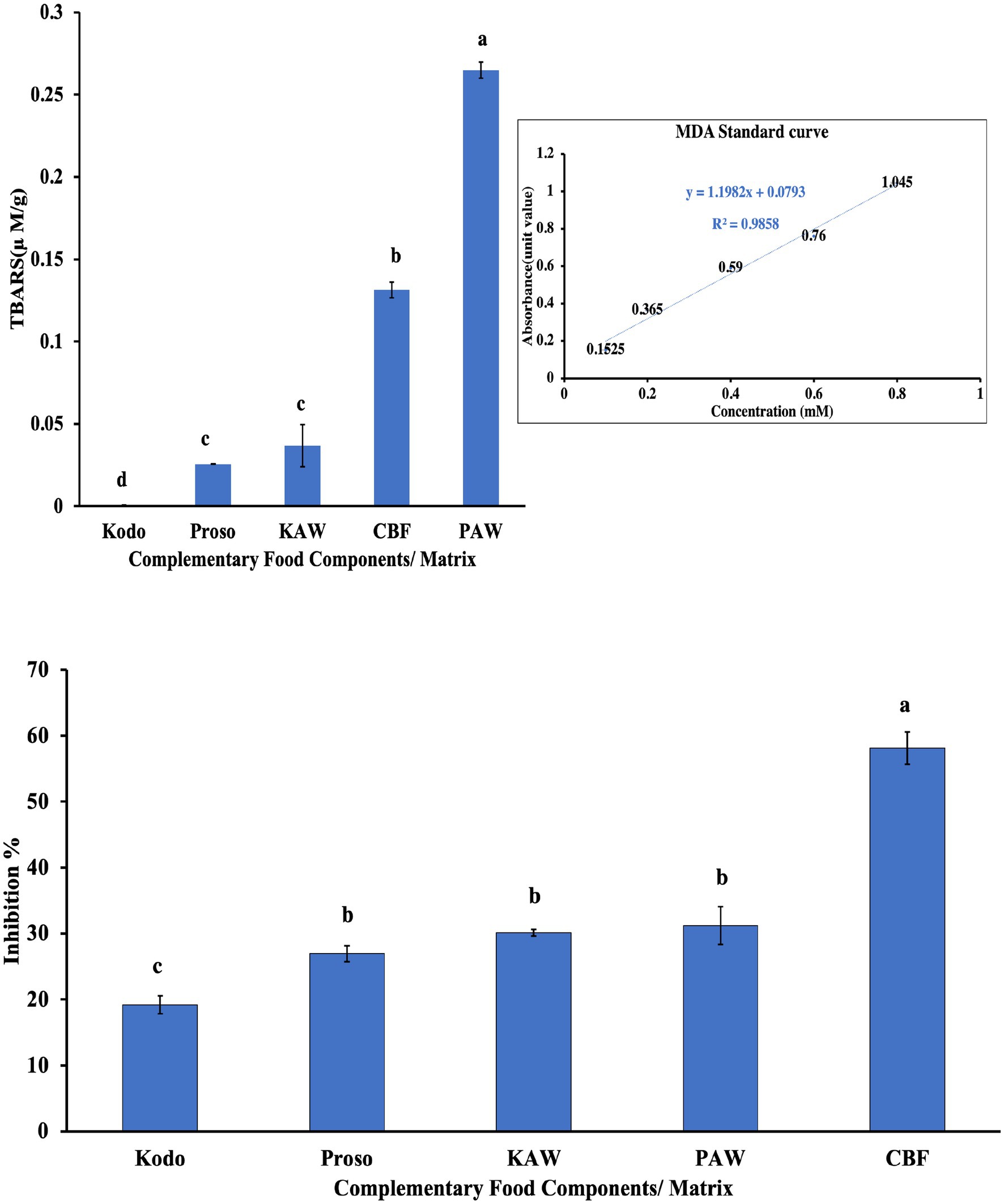
Figure 7. TBARS & DPPH analysis for pure millet powder (kodo & proso variety) and spray-dried powder containing acid whey and millets (KAW & PAW) compared with the commercial baby food (CBF). Different lowercase letters on the bar graph denote significant differences (p < 0.05) obtained using Tukey’s test.
Tannins are a type of water-soluble phenolic compound categorized under hydrolysable and condensed tannins. They tend to form strong hydrogen bonds with nutrients which inhibits their interaction with digestive enzymes and hence, are known as antinutritional factors. However, tannins have beneficial health properties as well, for example, they are antibacterial, antioxidative and have free radical scavenging properties (Yacout, 2016). They are commonly found in grains such as sorghum, specifically in the pigmented testa layer, finger millet, and barley. The tannin content varies with the genetic background of the grain, temperature, and humidity (Amarowicz, 2007). In the conducted experiment, spray-dried proso powder (PAW) was observed to have a significantly higher content of tannins in terms of catechin equivalent as compared to the other samples as shown in Figure 8. Catechin is generally categorized as tannin as 85% components are similar of both the compounds (Nakagawa, 2022).
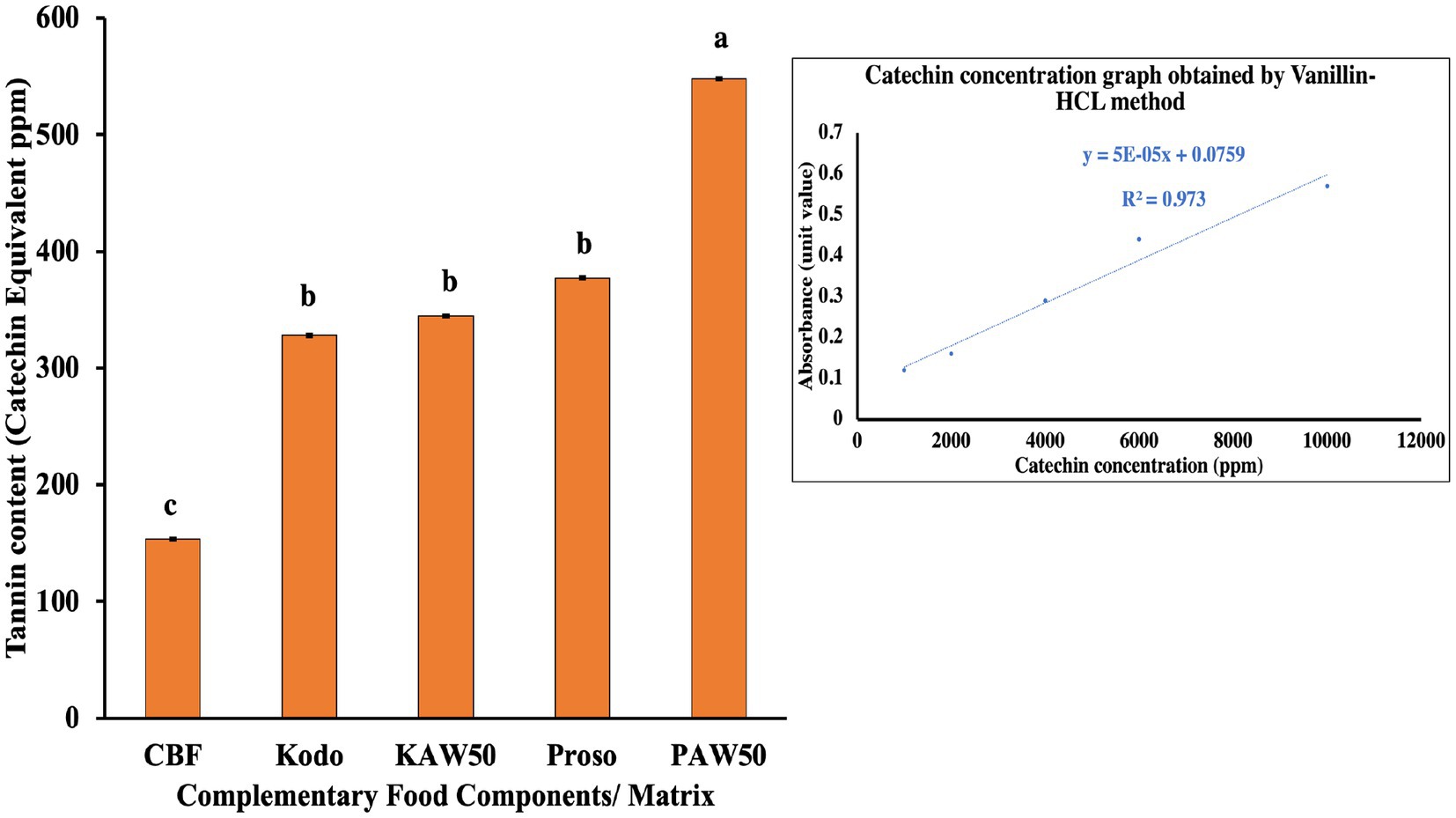
Figure 8. Tannin analysis for pure millet powder (kodo & proso variety) and spray dried powder containing acid whey and millets (KAW & PAW) in terms of catechin equivalent compared with the commercial baby food (CBF). Different lowercase letters on the bar graph denote significant differences (p < 0.05) obtained using Tukey’s test.
3.3. Microbial analysis
Food and Drug Administration Code of regulation (CFR) title 21 covers the requirements pertaining to infant formula in terms of quality control and current good manufacturing practices. According to the regulation, the manufacturing system of infant formula should be established in a way wherein there should not be any kind of adulteration because of microbial growth, specifically in terms of Cronobacter spp. and Salmonella spp. (FDA, 2019).
Spray-drying is a commonly used method for production of shelf stable powders, but the method does not necessarily cause any microbial inactivation. Even if the inlet and outlet temperatures for processing reaches 200°C and 100°C, respectively, the time of exposure of the matrix to the temperature is quite less. This short period generally injures the microorganisms but most of the times, is not lethal enough to cause cell death. The powder sources from milk-based ingredients are susceptible to the growth of spore-forming bacteria. Also, to mitigate safety risk, it is important to analyze the vitamin premix quality that is used in the formulation. Generally, the vitamin premixes do not go through high heat processing, hence, a small amount of premix impacts a large quantity of product. In the conducted experiment, it was observed that the microbial growth for all the samples ranged between 4 to 5 log CFU/mL in terms of standard plate count analysis as well as yeast and mold analysis, as observed in Figures 9, 10.
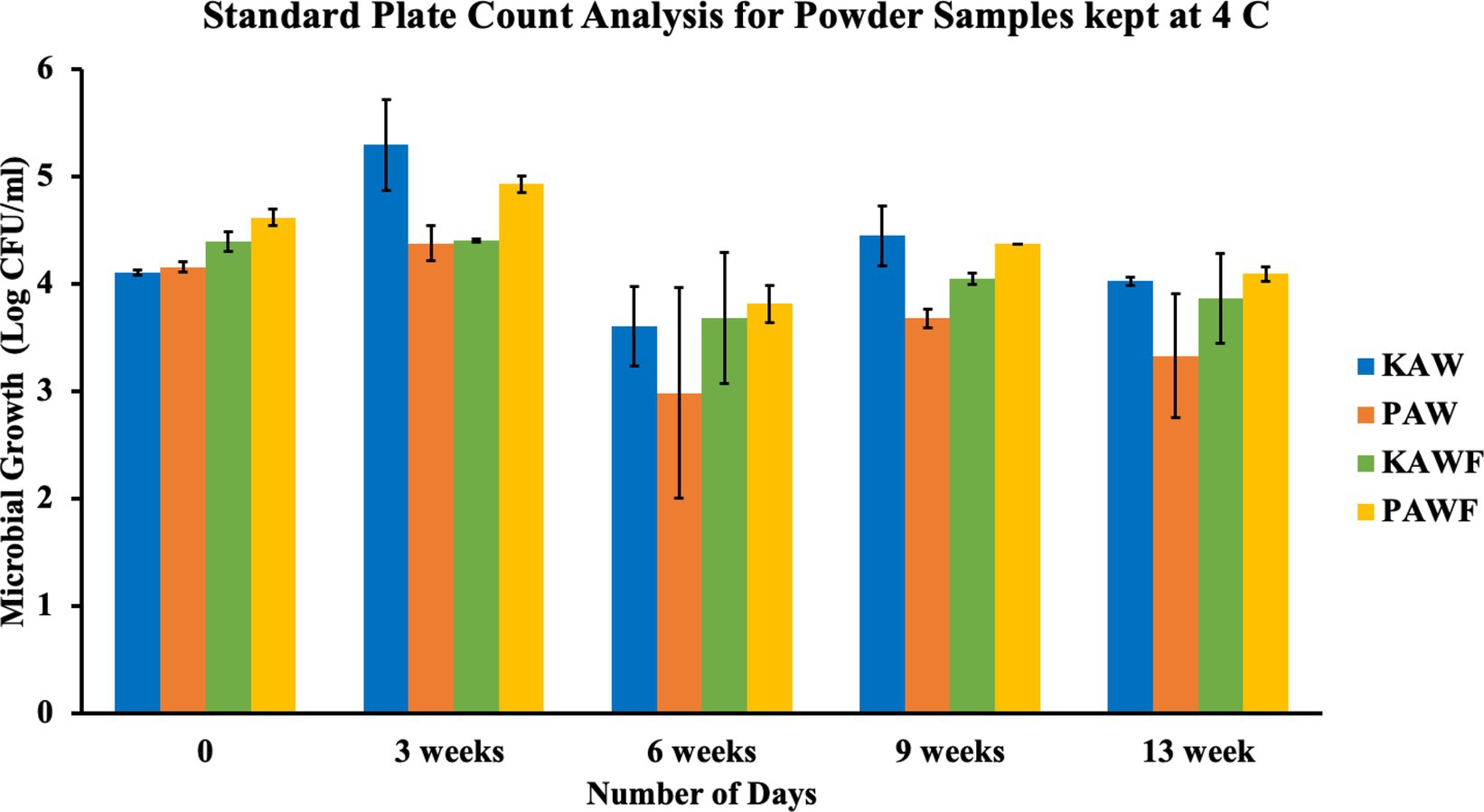
Figure 9. Standard plate count analysis for spray dried acid whey-millet powders (KAW & PAW) and vitamin encapsulated spray dried acid whey-millet powder samples (KAWF & PAWF).
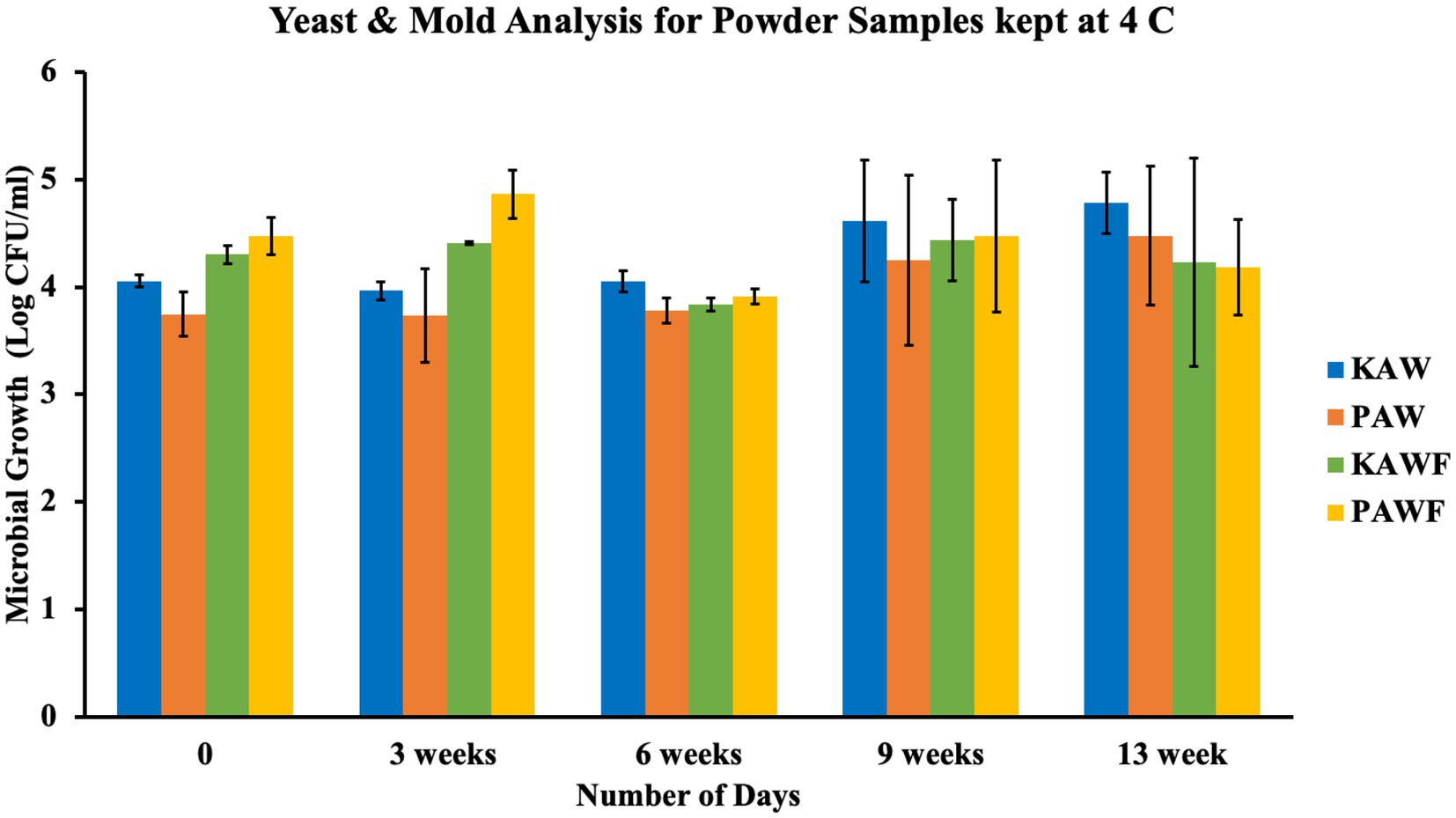
Figure 10. Yeast and mold analysis for spray dried acid whey-millet powders (KAW & PAW) and vitamin encapsulated spray-dried acid whey-millet powder samples (KAWF & PAWF).
According to the two factor ANOVA analysis, it was observed that a significant difference (p < 0.05) was observed between different days of analysis in the case of standard plate count analysis, but there was no significant difference (p > 0.05) observed among different samples. The aerobic plate count method generally indicates the level of bacterial population in a product. The test does not differentiate between different types of bacteria but is often used for evaluation of the surrounding sanitary conditions, as an indicator of safety and organoleptic acceptability. If the product has a high amount of aerobic plate count it can be assumed to be a source of potential hazard. However, the growth observed for the samples can also be attributed to the fact that fermented food naturally tends to have a high aerobic plate count (Alvarenga et al., 2018). In case of yeast and mold analysis as well, there was no significant difference (p > 0.05) in either number of days of analysis or among different samples. Yeast and mold analysis also indicate the deterioration and decomposition of food over time. Growth of yeast and molds attributes to a huge economic loss to both producer and consumer. If consumed, it can also be hazardous with the production of toxic metabolites in the body.
3.4. Sensory analysis
Descriptive sensory analysis helps in comparing the product prototypes in terms of perception of different sensory attributes. Quantitative descriptive analysis gives high precision qualitative data that help in tracking the shelf life of a food product and investigate the variables that change during the course of time (Armstrong, 1999). Various studies have concluded that whey, as an ingredient, affects the odor and flavor of a food product. In a study conducted by Beucler et al. (2006), sensory analysis of a beverage made from whey permeate was conducted. It was observed that the beverage showed brothy and sour flavors. Whey was also observed to have a predominant salty flavor (Beucler et al., 2006). Martini and Walsh (2012) conducted an analysis on the sensory characterization of sonicated whey. The study concluded that pH impacts various sensory attributes, such as astringency, maltiness, and sourness.
Likewise for millet-based products, the common attributes that are tested are rancidity, dryness in the product, sweetness, and bitterness. In a study conducted by Kebakile et al. (2008), the sensory characteristics of sorghum porridge was studied, and it was observed that the milling process is a major factor that determines the color and flavor of the product. Syeunda et al. (2019) investigated the sensory attributes of complementary porridge made from cowpea and finger millet. The major attribute that was observed were malty flavor, aroma and aftertaste.
Panel discussion for the lexicon building yielded the attributes discussed in Table 4. The focus was how whey and millets can collectively affect the appearance, flavor and texture of a product. For appearance, focus was on how a beverage looks when it’s reconstituted from a powder. Hence, transparency, solubility, sedimentation, and homogenization were key attributes. For flavor, the characteristic flavors of yogurt and cereals were included, such as milkiness, sourness, sweetness, and maltiness. For texture, viscosity and chalkiness were important attributes.
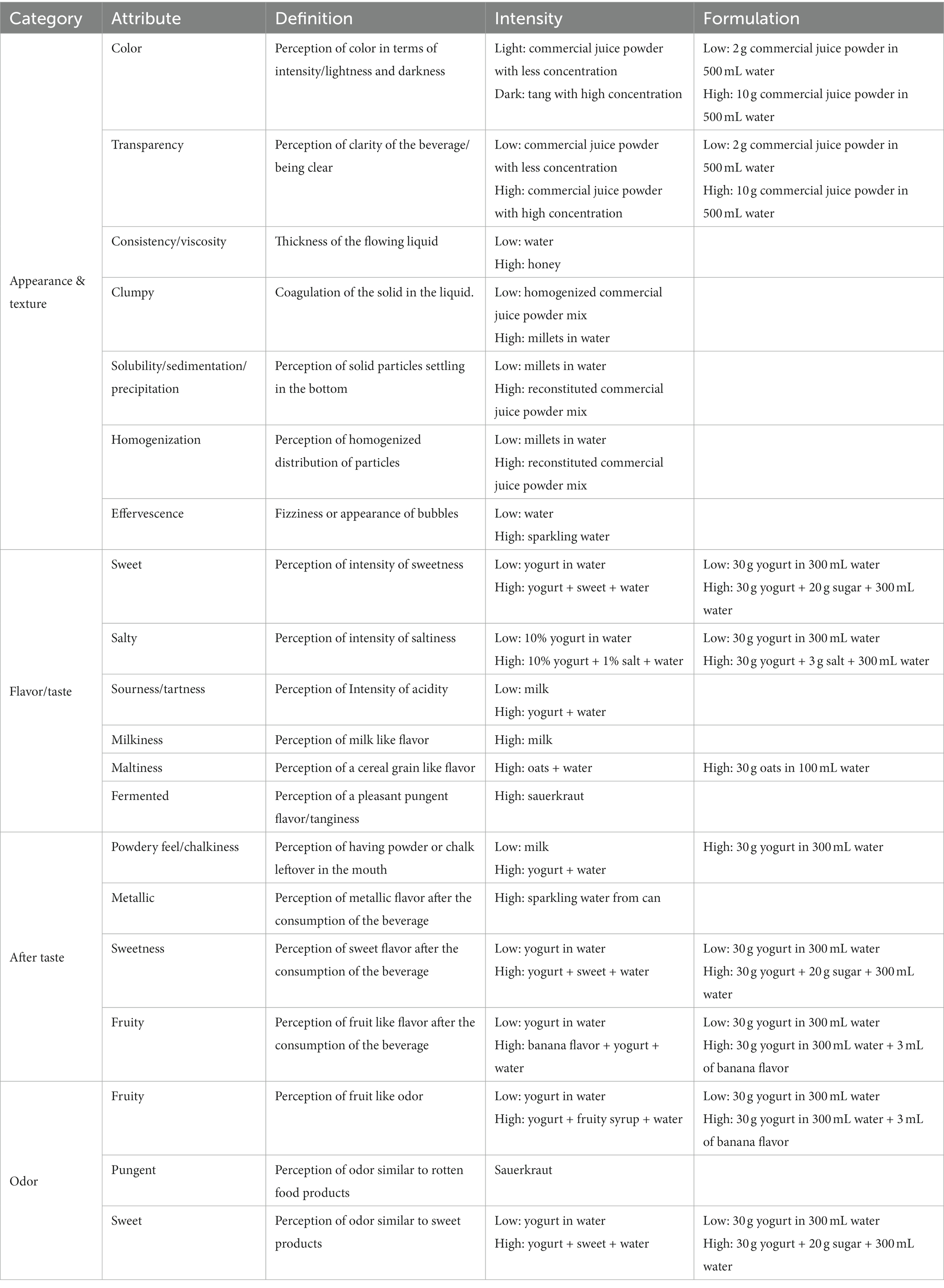
Table 4. Summary of the lexicon built by the 10 panelist and the reference product and formulation used for the training session.
Table 5 and Figure 11 summarize the data obtained from the sensory evaluation describing the differences perceived for the three different samples. In terms of appearance, there was a difference observed in the color and transparency of the material. However, no difference was observed in terms of the sedimentation, clumping and effervescence. All the samples were observed to be highly homogenized. There was no difference (p > 0.05) observed in the odor of the samples. In terms of texture, acid whey beverage and PAW beverage was observed to be different (p < 0.05). In case of flavors, the sweetness and saltiness were perceived in similar manner (p > 0.05) but the intensity of sourness for acid whey sample was observed to be higher. In case of viscosity, the PAW sample was observed to have a significantly higher viscosity and chalkiness (p < 0.05). The aftertaste observed for all the samples did not constitute any significant difference (p > 0.05).
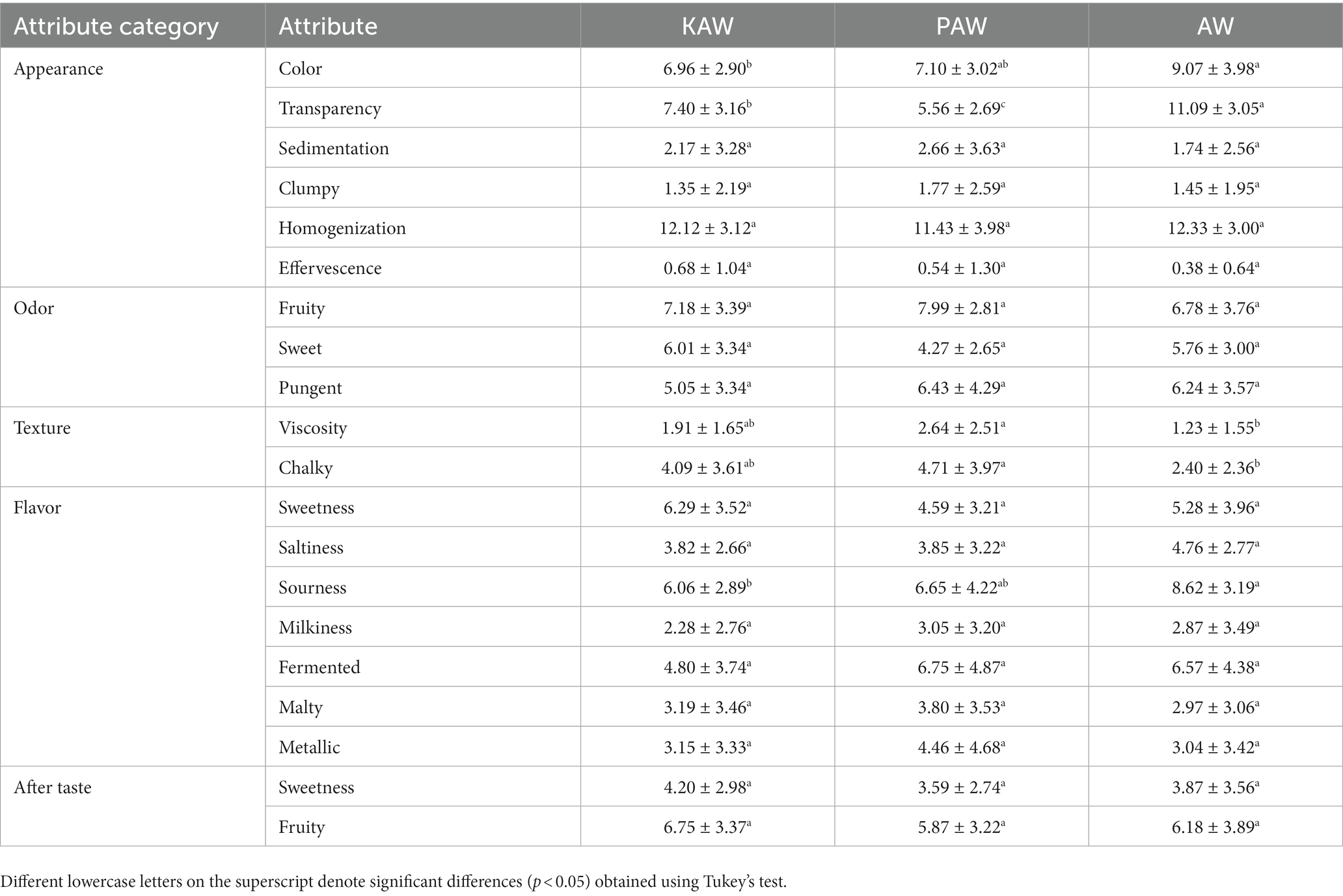
Table 5. Summary of means for all samples and significant descriptors as calculated over replicates and panelists (30 observations for each attribute) and LSD values (p < 0.05) measure over a scale of 15 cm unstructured scale anchored with “low” and “high.”
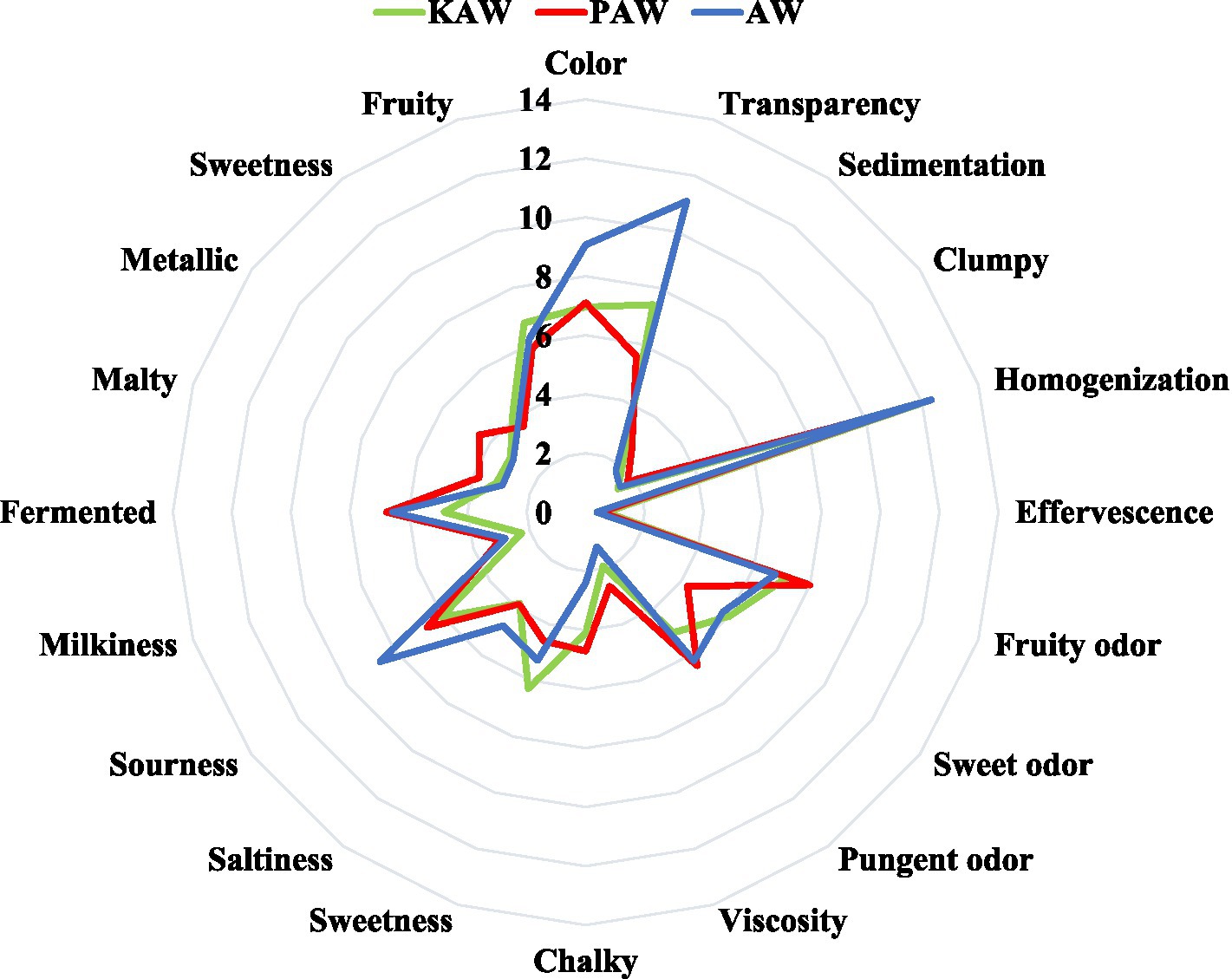
Figure 11. Radar chart differentiating sensory evaluation attributes for beverages made from acid whey (AW), kodo-acid whey (KAW) and proso-acid whey (PAW) matri.
4. Conclusion
The nutritional analysis of the spray-dried acid whey-millet powder showed that proso millet impacted the protein content in the matrix leading to lower protein content attributing to the low pH of acid whey that denaturizes the weak hydrogen bonds in the protein structure. However, the crude fat yielded no significant difference (p > 0.05) whereas the crude fiber analysis showed that there was a significantly higher amount in the pure proso and proso-acid whey matrix as compared to pure kodo and kodo-acid whey. For spray-dried powders, lactose content (50% increase) was found to be a higher content because of acid whey. The ash content in the spray-dried powder was also observed to be higher. The spray-dried samples were observed to have a significantly higher polyphenol content, specifically tannins. For microbial analysis of spray-dried powder conducted for over 13 weeks, it was observed that there were significant differences (p < 0.05) observed between different days of analysis in the case of standard plate count analysis, but there was no significant difference were observed among different samples (p > 0.05). In the case of yeast and mold analysis, there was no significant difference observed in either the number of days of analysis or among different samples (p > 0.05). For the sensory analysis, different attributes yielded results describing and differentiating properties of a beverage sample prepared from acid whey, acid whey-kodo millet, and acid whey-proso millet. Overall, an enhancement in nutritional properties was observed when a pure millet powder was combined with acid whey and made into a product. This information can help in providing the Greek yogurt industry with an alternative utilization of acid whey that is currently being mismanaged by the Greek yogurt industry. The product formulation suggested in the study can be further used in product development of complementary foods, nutritional supplements, or instant beverages health.”
Data availability statement
The original contributions presented in the study are included in the article/supplementary material, further inquiries can be directed to the corresponding author.
Author contributions
SM: methodology, investigation, writing-original draft, visualization, software, and formal analysis. AM: methodology, investigation, resources, writing—review and editing, and supervision. KK: conceptualization, methodology, resources, visualization, software, writing—review and editing, supervision, project administration, and funding acquisition. All authors contributed to the article and approved the submitted version.
Acknowledgments
The authors would like to thank the funding support from Black & Veatch’s NextGen Agriculture-Seed Grant-BV2019.
Conflict of interest
The authors declare that the research was conducted in the absence of any commercial or financial relationships that could be construed as a potential conflict of interest.
Publisher’s note
All claims expressed in this article are solely those of the authors and do not necessarily represent those of their affiliated organizations, or those of the publisher, the editors and the reviewers. Any product that may be evaluated in this article, or claim that may be made by its manufacturer, is not guaranteed or endorsed by the publisher.
References
Alvarenga, V. O., Campagnollo, F. B., Pia, A. K. R., Conceição, D. A., Abud, Y., Sant’Anna, C., et al. (2018). Quantifying the responses of three Bacillus cereus strains in isothermal conditions and during spray drying of different carrier agents. Front. Microbiol. 9:1113. doi: 10.3389/fmicb.2018.01113
Amadoubr, I., and Le, M. (2013). Millets: nutritional composition, some health benefits, and processing - a review. Emirates J. Food Agricul. 25:501. doi: 10.9755/ejfa.v25i7.12045
Amarowicz, R. (2007). Tannins: the new natural antioxidants? Eur. J. Lipid Sci. Technol. 109, 549–551. doi: 10.1002/ejlt.200700145
Armstrong, G. A. (1999). Quantitative descriptive analysis (QDA)—utilising the human instrument. Nutr. Food Sci. 99. doi: 10.1108/nfs.1999.01799faf.001
Askew, K.. (2021). Upcycling solutions for a more efficient food system: ‘If it’s still food grade, there’s still value to extract’ Available at: https://www.foodnavigator.com/Article/2021/05/19/Upcycling-solutions-for-a-more-efficient-food-system-If-it-s-still-food-grade-there-s-still-value-to-extract
Belton, P. S., and Taylor, J. R. N. (2002). Pseudocereals and Less Common Cereals. Berlin, Heidelberg: Springer.
Beucler, J., Drake, M., and Foegeding, E. A. (2006). Design of a beverage from whey permeate. J. Food Sci. 70, S277–S285. doi: 10.1111/j.1365-2621.2005.tb07203.x
Bharudin, M. A., Zakaria, S., and Chia, C. H. (2013). Condensed tannins from acacia mangium bark: characterization by spot tests and FTIR. AIP Conf. Proc. 1571, 153–157. doi: 10.1063/1.4858646
Broadhurst, R. B., and Jones, W. T. (1978). Analysis of condensed tannins using acidified vanillin. J. Sci. Food Agri. 29, 788–794. doi: 10.1002/jsfa.2740290908
Bunkar, D. S. (2021). Nutritional, functional role of kodo millet and its processing: a review. Int. J. Curr. Microbiol. App. Sci. 10, 1972–1985. doi: 10.20546/ijcmas.2021.1001.229
Chandrasekara, A., and Shahidi, F. (2010). Content of insoluble bound phenolics in millets and their contribution to antioxidant capacity. J. Agric. Food Chem. 58, 6706–6714. doi: 10.1021/jf100868b
Chauhan, M., Sonawane, S. K., and Arya, S. S. (2018). Nutritional and nutraceutical properties of millets: a review. Clin. J. Nutr. Diet. 1:10.
Chen, W., Chiu, H. T., Feng, Z., Maes, E., and Serventi, L. (2021). Effect of spray-drying and freeze-drying on the composition, physical properties, and sensory quality of pea processing water (Liluva). Foods 10:1401. doi: 10.3390/foods10061401
Dresch, A. P., Cavali, M., Santos, D. F., Fogolari, O. O., Tironi, S. P., Pinto, V. Z., et al. (2022). Different treatments of pearl millet for cellulose recovery: effects on lignocellulosic composition. In Review. Available at: https://doi.org/10.21203/rs.3.rs-1689771/v1
Erickson, B. E. (2017). Acid whey: is the waste product an untapped goldmine? chemical & engineering news. Available at: https://cen.acs.org/articles/95/i6/Acid-whey-waste-product-untapped.html
FDA. (2019). CFR-code of Federal Regulations Title 21 part 106. Available at: https://www.accessdata.fda.gov/scripts/cdrh/cfdocs/cfcfr/CFRSearch.cfm?CFRPart=106&showFR=1
Guney, E., Fatih Ceylan, M., Tektas, A., Alisik, M., Ergin, M., Goker, Z., et al. (2014). Oxidative stress in children and adolescents with anxiety disorders. J. Affect. Disord. 156, 62–66. doi: 10.1016/j.jad.2013.11.016
Hanson, C., Lyden, E., Furtado, J., Van Ormer, M., and Anderson-Berry, A. (2016). A comparison of nutritional antioxidant content in breast Milk, donor Milk, and infant formulas. Nutrients 8:681. doi: 10.3390/nu8110681
Hassan, Z. M., Sebola, N. A., and Mabelebele, M. (2021). The nutritional use of millet grain for food and feed: a review. Agric. Food Secur. 10:16. doi: 10.1186/s40066-020-00282-6
Hendriks, W. H. (2018). 46 amino acid availability in heat-damaged ingredients. J. Anim. Sci. 96:25. doi: 10.1093/jas/sky073.044
Kandasamy, S., and Naveen, R. (2022). A review on the encapsulation of bioactive components using spray-drying and freeze-drying techniques. J. Food Process Eng. 45, 5937–5952. doi: 10.1111/jfpe.14059
Kanner, J. (2007). Dietary advanced lipid oxidation endproducts are risk factors to human health. Mol. Nutr. Food Res. 51, 1094–1101. doi: 10.1002/mnfr.200600303
Kebakile, M. M., Rooney, L. W., de Kock, H. L., and Taylor, J. R. N. (2008). Effects of sorghum type and milling process on the sensory characteristics of sorghum porridge. Cereal Chem. J. 85, 307–313. doi: 10.1094/CCHEM-85-3-0307
Lievore, P., Simões, D. R. S., Silva, K. M., Drunkler, N. L., Barana, A. C., Nogueira, A., et al. (2015). Chemical characterisation and application of acid whey in fermented milk. J. Food Sci. Technol. 52, 2083–2092. doi: 10.1007/s13197-013-1244-z
Malik, S., Krishnaswamy, K., and Mustapha, A. (2021). Physical properties of complementary food powder obtained from upcycling of Greek yogurt acid whey with kodo and proso millets. J. Food Process Eng. 44:e13878. doi: 10.1111/jfpe.13878
Martini, S., and Walsh, M. K. (2012). Sensory characteristics and functionality of sonicated whey. Food Res. Int. 49, 694–701. doi: 10.1016/j.foodres.2012.09.018
Minekus, M., Alminger, M., Alvito, P., Ballance, S., Bohn, T., Bourlieu, C., et al. (2014). A standardised static in vitro digestion method suitable for food—an international consensus. Food Funct. 5, 1113–1124. doi: 10.1039/C3FO60702J
Nakagawa, M. (2022). Catechin/all about tea. Culture, health benefit, business etc World Green Tea Association Available at: https://www.o-cha.net/english/teacha/scientific/catechin.html.
Nani, M., and Krishnaswamy, K. (2023). A natural whitening alternative from upcycled food waste (acid whey) and underutilized grains (millet). Sci. Rep. 13:6482. doi: 10.1038/s41598-023-32204-4
Sady, M., Jaworska, G., Grega, T., Bernaś, E., and Domagała, J. (2013). Application of acid whey in orange drink production. Food Technol. Biotechnol. 51, 266–277.
Shahidi, F., and Chandrasekara, A. (2013). Millet grain phenolics and their role in disease risk reduction and health promotion: A review. J. Funct. Foods 5, 570–581. doi: 10.1016/j.jff.2013.02.004
Shen, R., Ma, Y., Jiang, L., Dong, J., Zhu, Y., and Ren, G. (2018). Chemical composition, antioxidant, and antiproliferative activities of nine Chinese proso millet varieties. Food Agric. Immunol. 29, 625–637. doi: 10.1080/09540105.2018.1428283
Singleton, V. L., and Rossi, J. A. (1965). Colorimetry of total phenolics with phosphomolybdic-phosphotungstic acid reagents. Am. J. Enol. Viticul. 16, 144–158.
Skryplonek, K., Dmytrów, I., and Mituniewicz-Małek, A. (2019). Probiotic fermented beverages based on acid whey. J. Dairy Sci. 102, 7773–7780. doi: 10.3168/jds.2019-16385
Syeunda, C. O., Anyango, J. O., and Faraj, A. K. (2019). Effect of compositing precooked cowpea with improved malted finger millet on anti-nutrients content and sensory attributes of complementary porridge. Food Nutr. Sci. 10, 1157–1178. doi: 10.4236/fns.2019.109084
Verma, S., Srivastava, S., and Tiwari, N. (2015). Comparative study on nutritional and sensory quality of barnyard and foxtail millet food products with traditional rice products. J. Food Sci. Technol. 52, 5147–5155. doi: 10.1007/s13197-014-1617-y
Wang, H., Li, D., Wan, C., Luo, Y., Yang, Q., Gao, X., et al. (2021). Improving the functionality of Proso millet protein and its potential as a functional food ingredient by applying nitrogen fertiliser. Foods 10:1332. doi: 10.3390/foods10061332
Wankhede, D. B., Shehnaj, A., and Raghavendra Rao, M. R. (1979). Carbohydrate composition of finger millet (Eleusine coracana) and foxtail millet (Setaria italica). Qual. Plant. 28, 293–303. doi: 10.1007/BF01095511
Wojtunik-Kulesza, K., Oniszczuk, A., Oniszczuk, T., Combrzyński, M., Nowakowska, D., and Matwijczuk, A. (2020). Influence of in vitro digestion on composition, bioaccessibility and antioxidant activity of food polyphenols—a non-systematic review. Nutrients 12:1401. doi: 10.3390/nu12051401
Yacout, M. (2016). Anti-nutritional factors & its roles in animal nutrition. J. Dairy Vet. Anim. Res. 4, 237–239. doi: 10.15406/jdvar.2016.04.00107
Keywords: upcycling, kodo millet, proso millet, spray drying, complementary food, nutrition, sensory, microbiology
Citation: Malik S, Mustapha A and Krishnaswamy K (2023) Development of millet-acid whey formulations with nutritional, microbiological, and sensory characterization. Front. Sustain. Food Syst. 7:1233689. doi: 10.3389/fsufs.2023.1233689
Edited by:
Debabandya Mohapatra, Central Institute of Agricultural Engineering (ICAR), IndiaReviewed by:
Saima Tehseen, Government College for Women University, PakistanManoj Kumar Tripathi, Central Institute of Agricultural Engineering (ICAR), India
Copyright © 2023 Malik, Mustapha and Krishnaswamy. This is an open-access article distributed under the terms of the Creative Commons Attribution License (CC BY). The use, distribution or reproduction in other forums is permitted, provided the original author(s) and the copyright owner(s) are credited and that the original publication in this journal is cited, in accordance with accepted academic practice. No use, distribution or reproduction is permitted which does not comply with these terms.
*Correspondence: Kiruba Krishnaswamy, a3Jpc2huYXN3YW15a0B1bXN5c3RlbS5lZHU=