- 1Indian Council of Agricultural Research (ICAR)-Indian Grassland and Fodder Research Institute, Jhansi, India
- 2Rani Lakshmibai Central Agricultural University, Jhansi, India
- 3University of Horticultural Sciences, Bagalkot, India
- 4School of Public Health, University of California, Berkeley, Berkeley, CA, United States
- 5Department of Plant Production, College of Food and Agriculture Sciences, King Saud University, Riyadh, Saudi Arabia
Soil function encompasses numerous functions and services the soil provides and can be measured using physical, chemical, and biological characteristics. In any event, research on fertility and biological activity is increasingly being stressed as indices of ecosystem services. Rangeland degradation is one of the world's most widespread land use changes, with serious consequences for soil processes and ecosystem services. The influence of uncontrolled grass cover on soil functioning in semiarid environments is little understood. In the current study, the following seven varieties of grass coverings were studied in a semiarid area of central India: Cenchrus ciliaris (CC), Megathyrsus maximus (MM), Chrysopogon fulvus (CF), Heteropogon contortus (HC), Sehima nervosum (SN), Vetiveria zizanioides (VZ), and Tri-Specific Hybrid (TSH). The carbon accumulation proficiency of TSH, MM, and HC was ~2.23, 2.17, and 2.07 times greater than fallow. The nutrient cycling capacity of grasses was ~1.1 to 1.6 times greater than that of fallow, despite the grasses' depleted macronutrients in the soil. The biological activity under MM and HC was ~1.23 and 1.67 times higher than fallow. Overall soil functionality under TSH and HC was 83 and 25% greater than MM, respectively. These soil functions augment ecosystem services like climate regulation, biomass production, nutrient recycling, water, and airflow. However, more attention should be paid to the management of inputs for greater ecosystem services from this grass cover in semiarid, degraded land.
Highlights
- The nutrient cycling capacity of grasses was ~1.1 to 1.6 times greater than that of fallow.
- The carbon accumulation proficiency of grasses improved by ~2 times over fallow.
- Soil functionality under TSH was 83% greater than that under Megathyrus.
- Grass-augmented services such as climate regulation, biomass production, and nutrient recycling.
1. Introduction
Soils, also designated as “reserves of natural capital”, serve many ecosystem services. To verify the role of soil in the sustainability of ecosystems, researchers have developed the idea of soil function (Pulleman et al., 2012; Baradwal et al., 2022). The capacity of soil to support microbial activity, the delivery of nutrient elements, structural stability, and support for biomass production are the prime qualities of soil functioning. As most ecosystem services could be linked to soils, restoring degraded land's potential to support soil functions and ecosystems should be a conservation goal (Perring et al., 2015).
Low rainfall, inefficient crop water and nutrient usage, and poor soil fertility are key challenges in semiarid regions (Wani et al., 2007), leading to below-average yields, little agricultural revenue, little organic carbon, and insufficient nutritional inputs in the region (Nosetto et al., 2006; Wani et al., 2007). Growing range grasses with a high capacity for sequestering carbon might therefore be a solution for semiarid areas (Li et al., 2017; Notenbaert et al., 2021). The capacity of grasses to bind soil particles, reduce erosion, establish themselves quickly, and create humus makes them more remarkable (Ola et al., 2015). The influence of different grasses on certain soil parameters was described by earlier workers (Wilekson et al., 2010). As a result, grasses can be paired with mechanical techniques to provide soil protection (Prajapati et al., 1973). Water cycling, carbon sequestration, nitrogen cycling, gas exchange with the environment, climate change mitigation, and aesthetic enjoyment are all critical activities of rangeland grasses in dry and semiarid environments (Franzluebbers, 2012; Fuglie et al., 2021). In semiarid regions, the primary stable food source for the majority of ruminants worldwide is grasses; they contain higher carbohydrate content but lower levels of protein, minerals, vitamins, and lipids than legumes (Tambara et al., 2017; Waliszewska et al., 2021). Despite this, grasses are the most frequently used fodder.
The primary ecosystem services of soil are carbon sequestration, food or biomass production, provision of microbial habitat, nutrient recycling, etc. (Tellen and Yerima, 2018). However, the actual magnitude of soil functions provided by range grasses has never been quantified. Moreover, there is little data on the impact of range grasses on soil functional indicators in semiarid regions. The goal of this study was to assess the impact of range grasses on soil functionality and establish a strong linkage with ecosystem services in semiarid ecosystems in central India. Three research issues are addressed in this study: (1) Do the soil fertility levels and nutrient supply capacity of the soil change differently for various grasses? (2) Under which grasses are microbial communities and soil biota most active? (3) How does the range of grasses impact the soil functionality in various soil layers? In response to these inquiries, we postulated that range grasses would boost carbon sequestration, food or biomass production, provision of microbial habitat, and nutrient recycling at various soil depths (i.e., 0–15 and 16–30 cm). Our findings are anticipated to help us boost ecosystem services, optimize land cover plans, and better understand how soil operates under various range grasses.
2. Materials and methods
2.1. Experimental site description
The study was carried out in India's Jhansi District (Figure 1). The study site is located in the Bundelkhand area of India. The soil type at the experimental site was sandy loam. They are shallow, well-drained, and vary in color from yellowish red to dark brown. A field experiment was carried out between 2015 and 2022 at the ICAR-Indian Grassland and Fodder Research Institute's Technology Demonstration Unit (25° 31′ 36.33′′ N lat, 78° 32′ 54.18′′ E long, and altitude 177 m above mean sea level). The research location is located in a semiarid environment with high temperatures, irregular rainfall, a high aridity index, and inadequate soil moisture. The study area's long-term average annual rainfall (1939–2015) is 908 mm, with most of it falling between June and August (Rai et al., 2018). The climate here is characterized by dry air, an excessively hot summer, and a chilly winter (late November to the middle of March). From July to September, the southwest monsoon occurs. The average annual rainfall (2015–2023) in the district is 840 mm. Approximately 90% of it occurs during the southwest monsoon, and the rest occurs throughout the year. Rainfall patterns in the Bundelkhand area are variable, resulting in cyclic droughts. The average daily maximum and lowest temperatures in January were 21.34 and 6.84°C, respectively (the coldest month). The average daily maximum temperature in May from 2015 to 2020 was 41.48°C (the warmest month). Peak temperatures in May and June can reach 48°C. The month of June had the highest mean daily evaporation (12.80 mm day−1).
2.2. Factors and level of land degradation
Wind erosion and a lack of fertile soil are the reasons that make the land susceptible to land degradation. All characteristics were compared to a fallow land to remove any room for doubt (located on a comparable slope, topography, soil texture, and parent material). In terms of soil nutrients and biological characteristics, we considered that fallow land had experienced minimal changes. We also assumed that climate had a comparable impact on the soils of alternate land use systems (ALUS) and fallow land, respectively. The soil texture at the study site comprised sandy loam and was a member of the Typic Haplustepts hypothermic family. They range from pale yellowish-red to dark brown. The soil lacked mineral N (183 kg ha−1), low SOC (0.35%), low plant-available P (9 kg ha−1), and medium K contents (250 kg ha−1). These soils have a modest ability to retain nutrients and water. The soil's ability to store water at saturation level was 32.5% (v/v). Throughout May and July, the average wind speed is >8 km/h, which results in soil erosion of between 35 and 50 Mg ha−1 yr−1.
2.3. Experimental details
Seven perennial tropical range grasses, namely Cenchrusciliaris (CC), Megathyrsus maximus (MM), Chrysopogon fulvus (CF), Heteropogon contortus (HC), Sehima nervosum (SN), Vetiveria zizanioides (VZ), and Tri-Specific Hybrid (TSH: Pennisetum glaucum x Pennisetum purpureum x Pennisetum squamulatum) were evaluated in this study. The grass seedlings were transplanted to the field on 15th July 2015. At the establishment stage, 2–3 irrigations were permitted to ensure uniformity and were afterward maintained in rainfed circumstances. Every year on 15th November, the grasses were trimmed 5 cm from the root and left to grow during the wet season (June–July). The biomass production of grasses was estimated using a uniform plot size of 10 × 15 m.
2.4. Collection of soil and plant samples
Areas with comparable physiography, geology, and climatic conditions were chosen to create a homogenous sampling arrangement. In each of the grasses, three locations were selected. During winter, after cutting grass in October 2021, five soil samples (from the four corners and the center of the plots) were collected from two layers of 0–15 and 16–30 cm at each location. Soil samples were also collected from fallow land. Soil samples were immediately taken to the laboratory and divided into two halves: one half was air-dried and utilized for physical and chemical analyses, while the other half was maintained at 4°C for microbiological property evaluation. The plants were harvested, kept in perforated brown paper bags, suitably labeled, and dried to attain constant weight in a thermostatically controlled oven at 70 ± 2°C (Ghosh et al., 2019). The dry weight was recorded and expressed in terms of dry matter accumulation by plants (g m−2). Root morphology was studied using a root analyzer coupled with Win-Rhizo.
2.5. Analysis of soil physical, chemical, and biological parameters
Soil particle size distribution (soil texture), bulk density, pH, and electrical conductivity were measured (Jackson, 1973). Soil organic carbon (SOC) and nitrogen were determined using chromic acid oxidation and permanganate oxidation. Available phosphorus (P) was determined by NaHCO3 extraction (Olsen, 1954), followed by spectrophotometric estimation. Available potassium (K) was extracted using the acetate salt of ammonium (Hanway and Heidel, 1952). The availability of micronutrients (iron, manganese, zinc, and copper) was determined using diethylene triaminepenta acetic acid. Microbial biomass C was measured with 45% extraction efficiency (Jenkinson and Powlson, 1976). Important nutrient-cycling enzymes, such as the activities of β-D-glucosidase, urease, and alkali phosphatase, were assessed (Dick, 2020).
2.6. Computation of indices of soil functionality for understanding ecosystem services
The pertinent ecosystem services and their indicator soil parameters have been listed in Table 1. Soil functionality was thought to be a function of nutrient supply capacity, carbon accumulation proficiency, biomass output, and microbial activity. It was calculated to determine the total potential of range grasses to boost soil functions.
A biological activity index was computed by using a formula (Ghosh et al., 2021).
where Pi is the ratio of the activity of the ith enzyme in experimental soils to that in fallow land. BAI is typically calculated as the average of all enzyme activity responses in cultivated land over fallow ground.
Nutrient supply capacity (NSC), a measure of nutrient dynamics, was calculated (Baradwal et al., 2022).
Ni is the proportion of the availability of ith nutrient in ALUS to that in fallow.
Carbon accumulation proficiency (CAP), a measure of ecosystem carbon sequestration, was calculated (Baradwal et al., 2022).
Cr is SOC accumulation in restored land, and Cf is SOC accumulation in fallow land. The biomass yield of grasses was normalized using a Z-score.
x is the biomass yield of grass, μ is the mean yield of grasses, and € is the standard deviation of grass yield.
Soil functionality (SF) was estimated as (Baradwal et al., 2022).
2.7. Statistical analysis
The collected data were processed for variance analysis (ANOVA) as applicable to one-way ANOVA to examine for differences among the range grasses specified by Gomez and Gomez (1984). Tukey's honest significant difference test (P < 0.05) was employed as a mean separation test. Node analysis was performed to understand the contribution of each grass to specific ecosystem services. The figures were created using MS Office Excel 2020–2021.
3. Results
3.1. Biomass productivity and root growth of grasses
The TSH produced the highest fodder yield. It had ~138, 87, and 64% greater yields than SN, VZ, and HC. The yield of CC and CF was similar. However, the yield of MM was ~101, 58, and 39% greater than that of SN, VZ, and HC, respectively. The biomass productivity of SN was the lowest (Table 2). Important qualities such as root length density (RLD) and root surface density (RSD) were highest for Vetiveria zizanoides grass (64.71 cm cm−3 and 8.59 cm2 cm−3, respectively), followed by Hetropogon contortus and Chrysopogon fulvus grass. However, the root weight density (RWD) was higher under TSH grass (117.01 mg cm−3), followed by Megathyrsus maximus grass (110.07 mg cm−3), and the lowest was observed in Cenchrus ciliaris (79.53 mg cm−3), whereas Megathyrsus maximus had the lowest RLD (39.73 cm cm−3), and RSD (6.07 cm2 cm−3). Similarly, the highest number of root tips was recorded for Megathyrsus maximus grass (9,115.25), followed by Vetiveria zizanoides grass (6,944.50). The average root diameter of different grasses varied from 0.30 to 0.46 mm. The maximum root diameter was recorded for Sehima nervosum grass (0.46 mm), followed by TSH grass (0.38 mm) and Vetiveria zizanoides grass (0.38 mm) (Table 2). In the case of root volume, the highest root volume (157.49 cm3) and root dry weight (23.07 g plant−1) were observed for Megathyrsus maximus grass, while the lowest values were with Heteropogon contortus and Cenchrus ciliaris grass.
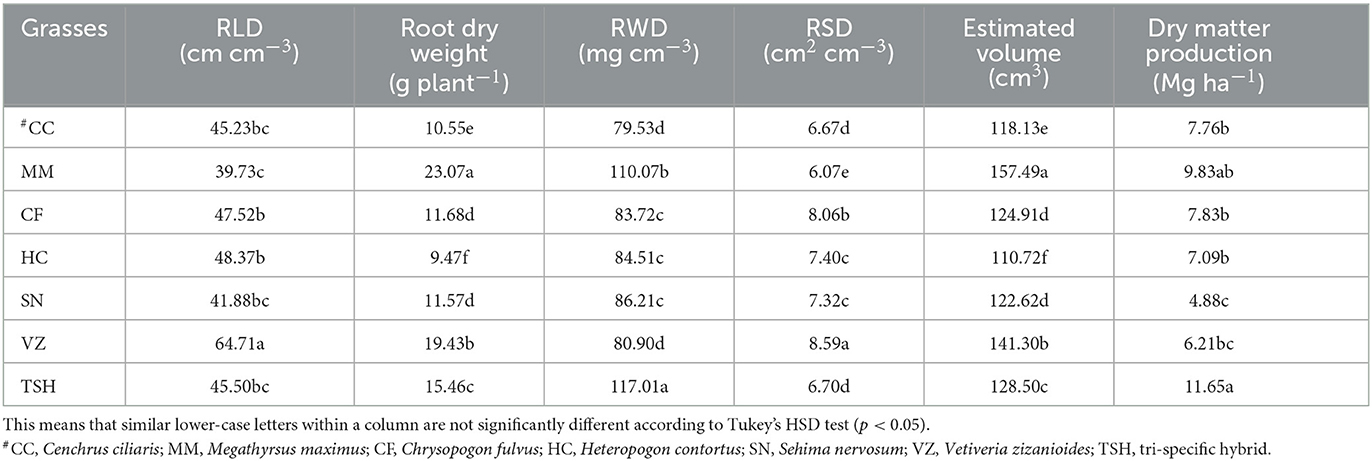
Table 2. Root morphological traits [root length density (RLD), root weight density (RWD), and root surface density (RSD)] and biomass productivity of different tropical perennial range grasses in semiarid India.
3.2. Soil bulk density, porosity, moisture content, and penetration resistance
The plots under VZ, HC, TSH, CF, SN, CC, and MM had ~ 134, 116, 116, 108, 62, 53, and 15.78% higher soil moisture than fallow land in the 0–15 cm soil surface. However, the VZ, HC, TSH, CF, SN, CC, and MM had ~ 111, 107, 91, 58, 45, 39, and 13%, respectively, superior soil moisture as compared to fallow land at the 16–30 cm soil layer, while the moisture of the MM plot was comparable to the moisture of fallow land in both soil layers (Table 3).
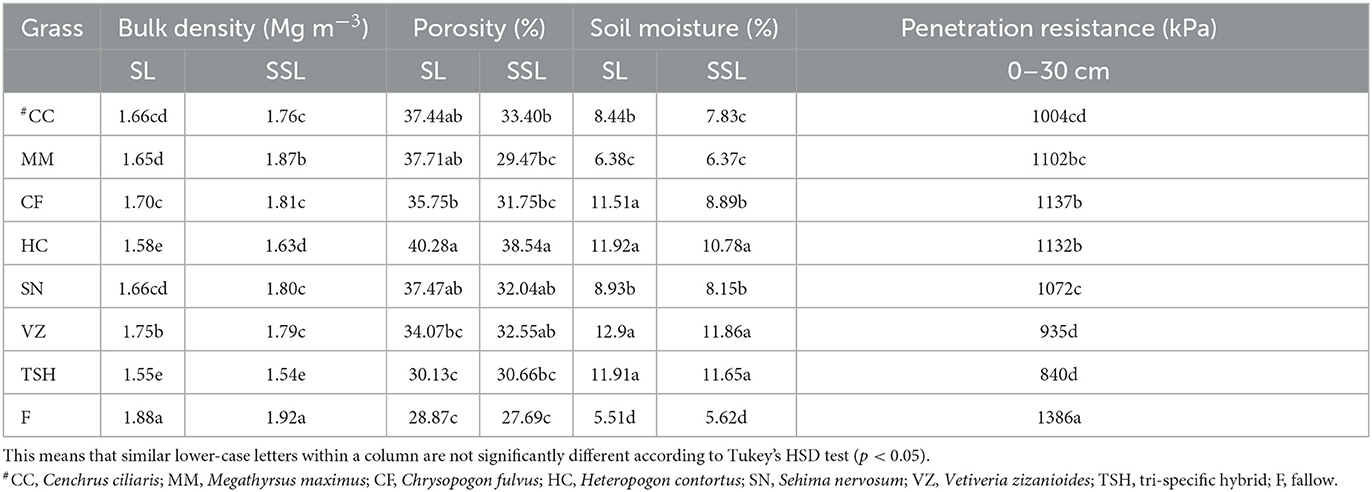
Table 3. Bulk density, porosity, soil moisture content, and penetration resistance of soil under different tropical perennial range grasses at the surface (SL; 0–15 cm) and subsurface (SSL; 16–30 cm) soil layers in semiarid India.
At the surface and subsurface soil, porosity increased by nearly 4–40% under grass cover. At the 0–15 cm layer, porosity under MM and HC was ~27 and 37% higher than TSH, whereas, at the 16–30 cm layer, HC and TSH had ~40 and 11% greater porosity than fallow. At the 0–15 cm soil surface, the soil under HC had a 15% lower bulk density than the fallow land (Table 3). However, at the 16–30 cm soil layer, HC, MM, SN, CC, and CF had ~16, 13, 12, 12, and 10% lower soil bulk density than fallow land (Figure 2). The plots under TSH, VZ, SN, CC, HC, MM, and CF had ~39, 33, 23, 13, 11, 6, and 4% higher soil penetration resistance than fallow land (Table 3).
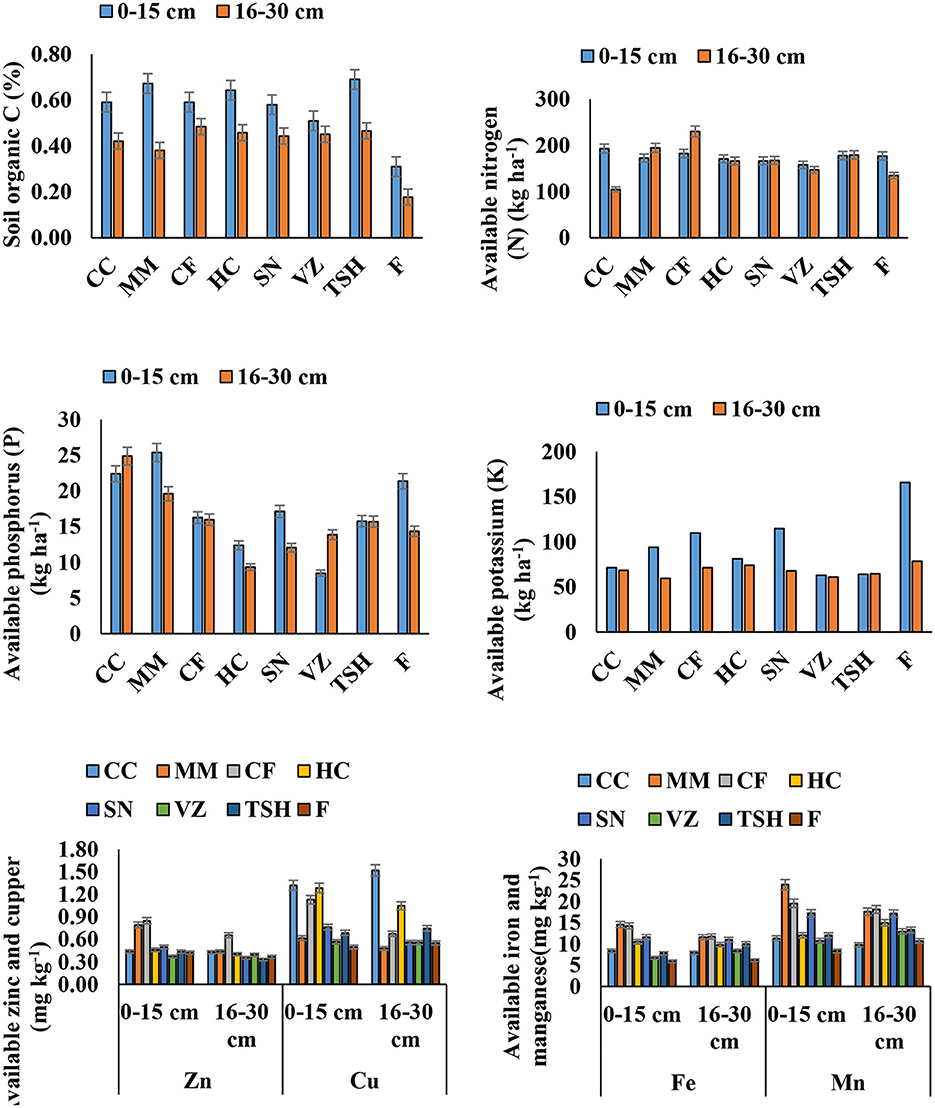
Figure 2. Nutrient availability in soil under different tropical perennial range grasses in semiarid India. The error bars indicate the LSD value according to Tukey's HSD test (p < 0.05). CC, Cenchrus ciliaris; MM, Megathyrsus maximus; CF, Chrysopogon fulvus; HC, Heteropogon contortus; SN, Sehima nervosum; VZ, Vetiveria zizanioides; TSH, tri-specific hybrid; F, fallow.
3.3. Soil organic C and nutrient availability
Range grasses significantly improved the SOC status at both soil layers over fallow land. The soil under TSH had ~35, 19, and 17% greater SOC than VZ, SN, and CF at the soil surface. However, the soil under HC had ~26, 10, and 8% greater SOC than VZ, SN, and CF, respectively (Figure 2). At the 16–30 cm soil layer, soils under all grasses had similar SOC, except MM. The range grasses either depleted or improved the soil mineral N in the surface layer. However, N under VZ and SN was depleted by ~11 and 6%, respectively, compared to fallow land. However, the N status under TSH, HC, and MM was maintained at the soil surface. Interestingly, at the subsurface, TSH and SN improved mineral N status by ~33 and 24% over fallow. All other range grasses except CC and VZ significantly improved the mineral N status at the soil subsurface (Figure 2). Range grasses significantly reduced the available P at the soil surface. The CF, HC, SN, VZ, and TSH depleted P by ~24, 42, 20, 60, and 26% over fallow. At the 16–30 cm soil layer, CC, MM, CF, and TSH improved soil P status by 73, 37, 11, and 9% over fallow. Soil P availability declined by ~35 and 16% under HC and SN over fallow. All the range grasses depleted soil K remarkably over the fallow period. The VZ and TSH contributed to the highest depletion of K at the surface and subsurface layers. The concentrations of micronutrients (Fe, Mn, Zn, and Cu) were mostly improved by range grasses at both soil layers, although a few trend breakers were present (Figure 2).
3.4. Soil microbial activity
Soils under TSH, HC, VZ, MM, and CF had ~130, 119, 90, 70, and 50% higher urease enzyme activity than fallow land in a 0–15 cm soil layer. However, CC and SN had ~30 and 29% lower urease enzyme activity than fallow land in a 0–15 cm soil layer (Figure 3). At the 16–30 cm soil layer, TSH, HC, and SN had ~93, 66, and 31% higher urease enzyme activity than fallow land. However, CF, MM, CC, and VZ had ~57, 50, 50, and 49% lower urease enzyme activity than fallow land. Soils under VZ had ~15% lower alkaline phosphatase enzyme activity than fallow land in a 0–15 cm soil layer. However, MM had ~22% lower alkaline phosphatase activity than fallow land. The soils under HC, CF, and MM had ~192, 58, and 45%, respectively, more DHA enzyme activity than fallow land in 0–15 cm soil layers. However, SN, VZ, CC, and TSH had ~80, 70, 44, and 42%, respectively, less DHA enzyme activity than fallow land in a 0–15 cm soil layer. At a 16–30 cm soil layer, CF had ~45% higher DHA enzyme activity than fallow land (Figure 3). However, CC, TSH, MM, SN, VZ, and HC had ~93, 91, 90, 60, 55, and 15%, respectively, lower DHA enzyme activity than fallow land. In the 16–30 cm soil layer, TSH, SN, and CF had ~68, 57, and 34% higher β- glucosidase enzyme activity than fallow land. However, MM, VZ, CC, and HC had ~74, 58, 34, and 11% lower β-glucosidase enzyme activity than fallow land, respectively. The soils under CF, HC, SN, MM, TSH, and CC had ~173, 135, 63, 58, 22, and 5% higher β-glucosidase enzyme activity than fallow land in 0–15 cm soil layer. However, VZ had ~34% minimum β-glucosidase enzyme activity than fallow land in a 0–15 cm soil layer. At the 16–30 cm soil layer, TSH, SN, and CF had ~68, 57, and 34% higher β- glucosidase enzyme activity than fallow land. However, MM, VZ, CC, and HC had ~74, 58, 34, and 11% lower β-glucosidase enzyme activity than fallow land (Figure 3).
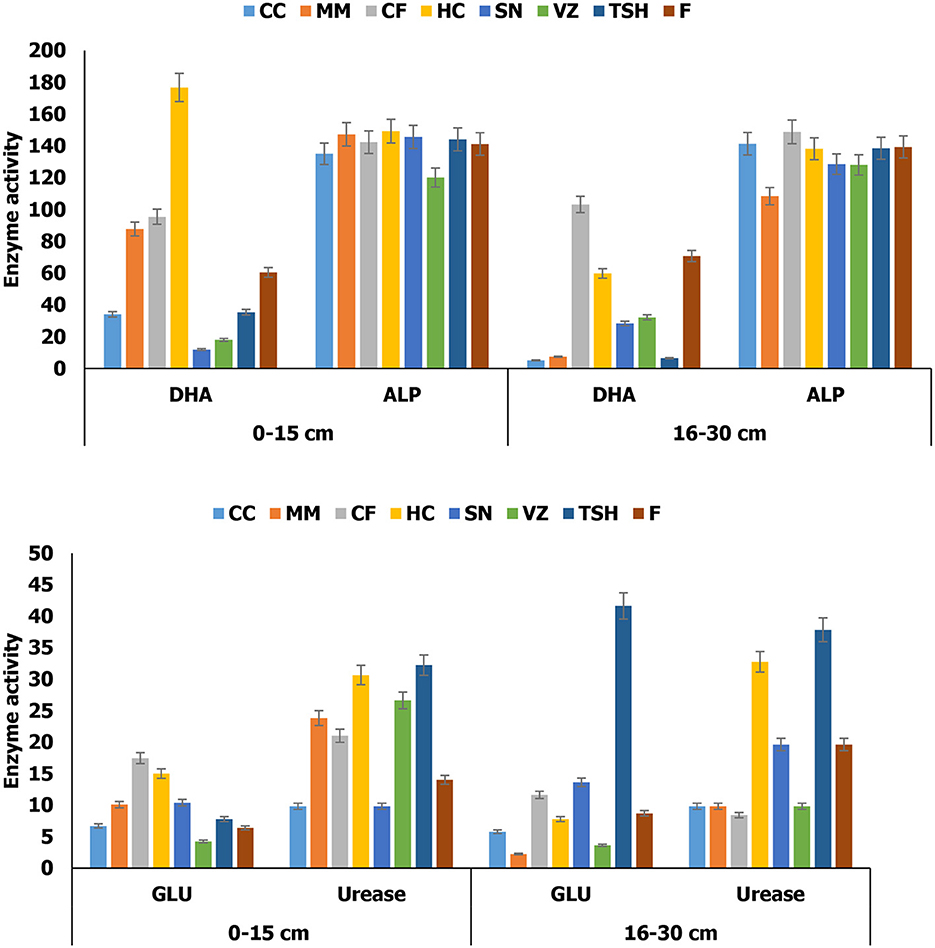
Figure 3. The activity of dehydrogenase (DHA; μg TPF g soil−1 24 h−1) alkaline phosphatase (ALP; μg PNP g soil−1 2 h−1), glucosidase (GLU; μg PNP g soil−1 h−1), and urease (μg NHg soil−1 2 h−1) in soil under different tropical perennial range grasses in semiarid India. The error bars indicate the LSD value according to Tukey's HSD test (p < 0.05). CC, Cenchrus ciliaris; MM, Megathyrsus maximus; CF, Chrysopogon fulvus; HC, Heteropogon contortus; SN, Sehima nervosum; VZ, Vetiveria zizanioides; TSH, tri-specific hybrid; F, fallow.
3.5. Soil functions and their indices
In the surface soil (0–15 cm), carbon accumulation proficiency (CAP) was found to be highest under the SN grass system, which was statistically at par with the MM and HC grass systems. The lowest CAP was found in the VZ-based system. CAP in the SN, MM, and HC systems was ~122, 117, and 107% higher than the fallow (Table 4). In sub-surface soil (16–30 cm), CAP was found to be highest under the CF-based system, which was statistically at par with the HC, VZ, and SN-based systems. The lowest CAP was found in the MM-based system. CAP in the CF, TSH, HC, VZ, and SN-based systems was ~91, 87, 107, 65, and 122%, significantly higher than fallow (Table 4). In the surface soil (0–15 cm), nutrient supply capacity (NSC) was found to be highest in the VZ-based system. The lowest NSC was found in the CF-based system, which was significantly NSC under VZ based system was ~39% significantly higher than the CF-based system. TSH and HC-based systems also showed ~24 and 19% higher NSC than CF-based systems, respectively (Table 4). NSC was found highest on the CC-based system, followed by the VZ-based system in sub-surface soil (16–30 cm). The lowest NSC was found in the CF-based system. CC and VZ-based systems show ~43 and 27% higher NSC than the CF-based system, respectively (Table 4). The biological activity index (BAI) in surface soil (0–15 cm) was found to be highest in the HC-based system, followed by the CF-based system, which was statistically at par with the MM-based system. The VZ-based system found the lowest BAI, which was statistically at par with the CC and SN-based systems. The HC-based system showed ~120% higher BAI than the VZ-based system (Table 4).
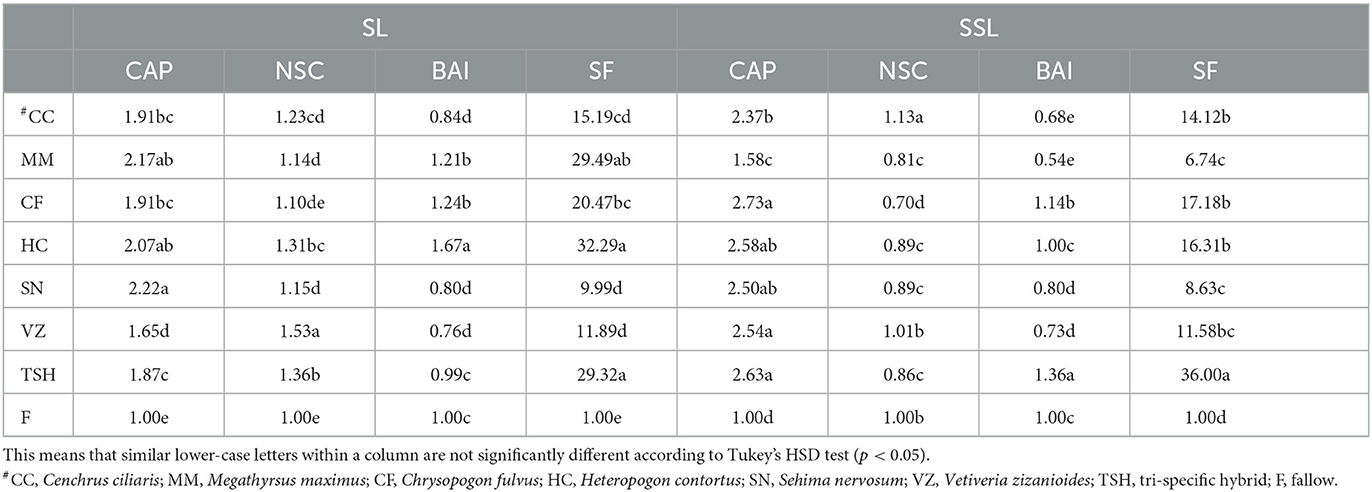
Table 4. Carbon accumulation proficiency (CAP), nutrient supply capacity (NSC), biological activity index (BAI), and soil functionality (SF) of soil under different tropical perennial range grasses at the surface (SL; 0–15 cm) and subsurface (SSL; 16–30 cm) soil layers in semiarid India.
In sub-surface soil (16–30 cm), BAI was found highest in the TSH-based system, followed by the CF-based system. CC-based systems showed the lowest BAI, which was statistically at par with the MM-based systems. The TSH-based system showed ~100% higher BAI than the CC-based system. In the surface soil (0–15 cm), soil functionality (SF) was found to be highest in the HC-based system. SF was found in the order: HC > TSH > MM > CF > CC > VZ > SN > F. HC and TSH-based systems showed ~ 223 and 193% higher SF over SN-based systems, respectively. SF was found highest on the TSH-based system, followed by the CF-based system in sub-surface soil (16–30 cm). The lowest SF was found in the MM-based system. SF was found in the order TSH > CF > HC > CC > VZ > SN > MM > F (Table 4).
4. Discussion
4.1. Tropical grasses could deplete macronutrient status
The chemical and physical properties of the soil can be significantly impacted by grass cover. The amount and type of crop debris and organic materials that reach the soil also affect the possible outcomes (Bakhshandeh et al., 2019; Paramesh et al., 2022). The emphasis in this situation is on studying and evaluating the condition of C and soil nutrients (as indicators of soil function) in addition to the numerous physical and chemical features of the soil (Kooch et al., 2019; Fan and Han, 2020). In actuality, changes in the spatial distribution of environmental factors, such as land use and management, microbial activity, and soil organic matter, affect soil fertility conditions (Basso et al., 2000). Our findings demonstrated that grass cover dramatically changed the nutritional condition of the soil. Since grasses absorb nitrogen, grass cover did not increase the N content, even though the C input from straw and grassroots considerably enhanced SOC (Chamberlain Lucas et al., 2022). The results of this study are consistent with those of de Oliveira et al. (2015), who discovered that land use clearly affects the quality and quantity of litter input, litter decomposition rates, and organic matter stabilization processes in soils. As a result, land use and the corresponding plant cover significantly alter the organic matter content. However, there are a number of other potential causes for the fall in soil P and K content under grass cover, including soil erosion, nitrogen absorption by these grasses, nutrient leaching during the wet season, etc. Increased micronutrient status under grass cover may be caused by weak absorption and the creation of strong bonds with organic materials.
To assess the state of ecosystems, one factor that may be taken into account is soil nutrients (Kooch et al., 2019). The C-P and C-N ratios in the current investigation revealed statistically superior variations among the grasses, and it appears that their absolute values were better able to capture the deterioration in soil quality. Significant data indicates that soil enzymatic activity can be utilized to indicate soil fertility and soil microbial activities. Soil enzyme activities are particularly responsive to grass cover (Wang et al., 2012; Parsapour et al., 2018; Ram et al., 2022). Wider C-N and C-P ratios suggest that the decreased BAI values under CC, SN, and VZ may result from severe food restriction. Several soil function indicators in the current study showed considerable improvements, whereas others showed significant declines. To sustain the soil nutrient strategy on semiarid, degraded land, greater focus should be placed on managing grasses. Although grass covers typically receive favorable responses, range grasses appear to require a fertilizing approach to enhance soil conditions.
4.2. Grasses strengthen the linkage between soil functions and ecosystem services
4.2.1. Climate regulation through carbon preservation
The CAP parameter investigates how grass cover may affect carbon flows, retention, and sequestration. The CAP provides a signal for C cycles, flows, and the systems' relative operational supremacy (Ghosh et al., 2021). The capacity of the grass cover to store and transport atmospheric carbon and produce biomass, among other things, enhanced CAP (Sainepo et al., 2018). The technology of grass cover had a more protected SOC than fallow land because of the comparatively high CAP ratings. In semiarid India, this research discovered that TSH and MM are viable choices for conserving or recovering SOC contents (Figure 4).
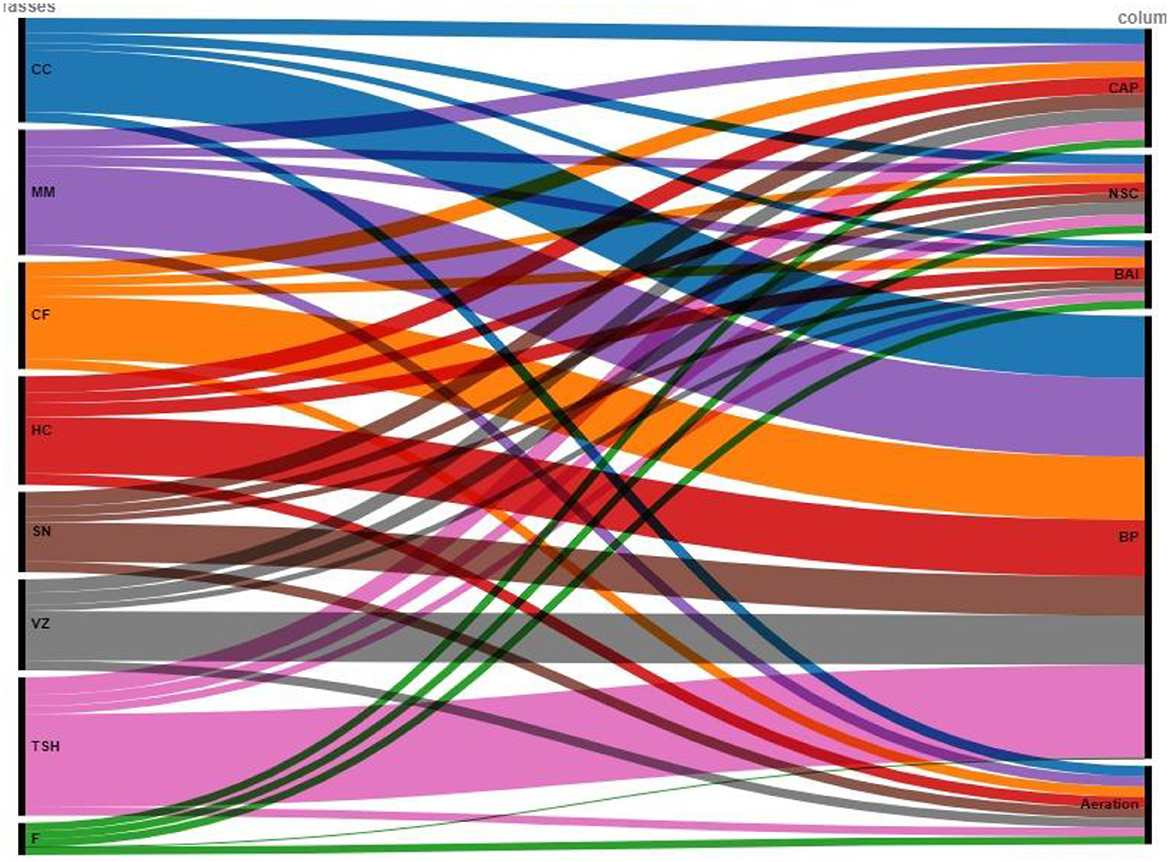
Figure 4. Node analysis plot depicting the contribution of different grasses toward soil functional indicators and their linkage to ecosystem services. The width of the lines indicates the strength of the linkage. CAP, Carbon accumulation proficiency; NSC, nutrient supply capacity; BAI, biological activity index; SF, soil functionality; BP, biomass productivity. CC, Cenchrus ciliaris; MM, Megathyrsus maximus; CF, Chrysopogon fulvus; HC, Heteropogon contortus; SN, Sehima nervosum; VZ, Vetiveria zizanioides; TSH, tri-specific hybrid; F, fallow.
4.2.2. Microbial activity and nutrient supply capacity
The ongoing supply of nutrients provided by live roots is beneficial to microbial cells. In contrast to fallow, CC, VZ, and SN, this may have increased BAI in MM and HC and maintained BAI under TSH (Guyonnet et al., 2018). The capacity to thrive in poor soil conditions, thick, deep networks of fine roots, and faster biomass development are just a few of the traits that assist in boosting biological activity under MM, HC, and TSH (Hu et al., 2021). By possibly increasing biomass productivity, these grasses help maintain soil biological activity, biodiversity, and ecosystem processes (Ghosh et al., 2021). The NSC parameter also evaluates the dynamics of nutrients in agroecosystems. Crop yields are increased due to the mineralization and decomposition of litter under grass cover (Figure 4). Nutrient deposition from leaves and rain may supplement litter decomposition, which is the main source of nutrients.
Moreover, the observed increases in NSC levels may result from a decrease in the distribution of nutrients out of the systems due to limited leaching in tree rows brought on by the impact of sheltering (Alva et al., 1999). Furthermore, the observed increase in SOC beneath the grass cover may result in a greater CEC and a consequent rise in the aptitude to retain nutrients (Lehmann, 2007; Bambrick et al., 2010). The nutrient outflow has been seen to have an impact on cations, particularly K+, in soil profiles (Jobbágy and Jackson, 2004). Litter deposit and breakdown are crucial to preserving NSC, particularly in less naturally fertile soils used for raising grasses. These processes are regulated by fauna, soil temperature, and management options (de Freitas et al., 2018; Ghosh et al., 2020).
4.2.3. Biomass productivity and soil airflow
The higher SOC content and root development may be related to the higher soil porosity under all grass cover compared to fallow. As a result, during the crucial low flow phase, soil water status and the ongoing river water flow to the soil profile are maintained. In the end, the deteriorated soil produced a sizable quantity of biomass due to the NSC and CAP (Figure 4). The production of plant biomass (fodder) serves as the foundation for ecosystem services connected to animal production. The productivity of grasses was lower than the stated yield, even though fodder production fluctuates with soil nutrients and moisture.
5. Conclusions
Soil functionality indicators, which frequently highlight the traits of soil ecology and fertility, offer important insights into ecosystem services. Functionality indicators in the soil can be considerably impacted by the type of grass. The habitats of semiarid areas, which are frequently situated in vulnerable locations, are particularly significant in this regard. Based on this, the current study examined how seven grasses affected the physical, chemical, and biological characteristics of the soil. According to our research, unmanaged grass coverings decreased soil nutrients, including N, P, and K, even if they increased SOC.
Nonetheless, regardless of the grass type, they could maintain their micronutrient status. Long-term grass growth led to the emergence of soil organisms that are better suited to cycling nutrients. In addition, more plant residuals entered the soil in the long term. Accordingly, grasses boosted soil functions such as green forage productivity, nutrient cycling capacity, carbon accumulation, and water and airflow compared to fallow. These functions of soil augment ecosystem services like climate regulation, biomass production, nutrient recycling, and water and airflow. In the long run, soil functionality under TSH and Heteropogon increased significantly in semiarid regions. In general, more attention should be paid to the management of inputs, such as nutrients, water, and manure, for greater ecosystem services.
Data availability statement
The original contributions presented in the study are included in the article/supplementary material, further inquiries can be directed to the corresponding author.
Author contributions
PP: data analysis and investigation. AS: formal analysis. AG: conceptualization, methodology, writing—original draft preparation, and software. MS: conceptualization, methodology, and supervision. SM and VK: validation. DO and AC: project administration. RC: software, validation, and editing revised manuscript. HE: data analysis, validation, and editing revised manuscript. All authors contributed to the article and approved the submitted version.
Funding
This work was funded by the Research and Innovations “Ministry of Education” in Saudi Arabia (IFKSUOR3-095-3).
Acknowledgments
PP express sincere gratitude to the competent authority of Bundelkhand University and ICAR-IGFRI for facilitating the work. The authors extend their appreciation to the Deputyship for Research and Innovations “Ministry of Education” in Saudi Arabia for funding this research (IFKSUOR3-095-3).
Conflict of interest
The authors declare that the research was conducted in the absence of any commercial or financial relationships that could be construed as a potential conflict of interest.
Publisher's note
All claims expressed in this article are solely those of the authors and do not necessarily represent those of their affiliated organizations, or those of the publisher, the editors and the reviewers. Any product that may be evaluated in this article, or claim that may be made by its manufacturer, is not guaranteed or endorsed by the publisher.
References
Alva, A. K., Prakash, O., Fares, A., and Hornsby, A. G. (1999). Distribution of rainfall and soil moisture content in the soil profile under citrus tree canopy and at the dripline. Irrigat. Sci. 18, 109–115. doi: 10.1007/s002710050051
Bakhshandeh, E., Hossieni, M., Zeraatpisheh, M., and Francaviglia, R. (2019). Land use change effects on soil quality and biological fertility: a case study in northern Iran. Eur. J. Soil Biol. 95, 103119. doi: 10.1016/j.ejsobi.2019.103119
Bambrick, A. D., Whalen, J. K., Bradley, R. L., Cogliastro, A., Gordon, A. M., Olivier, A., et al. (2010). Spatial heterogeneity of soil organic carbon in tree-based intercropping systems in Quebec and Ontario, Canada. Agroforest. Syst. 79, 343–353. doi: 10.1007/s10457-010-9305-z
Baradwal, H., Ghosh, A., Kumar, A., Singh, P. D., Sannagoudar, M. S., Ahamad, S., et al. (2022). Ecological restoration of degraded lands with alternate land use systems improves soil functionality in semiarid tropical India. Land Degrad. Dev. 33, 1076–1087. doi: 10.1002/ldr.4225
Basso, F., Bove, E., Dumontet, S., Ferrara, A., Pisante, M., Quaranta, G., et al. (2000). Evaluating environmental sensitivity at the basin scale through the use of geographic information systems and remotely sensed data: an example covering the Agri basin (Southern Italy). Catena 40, 19–35. doi: 10.1016/S0341-8162(99)00062-4
Chamberlain Lucas, A., Aguayo, T., Zerega, N. J. C., Dybzinski, R., and Egerton-Warburton, L. M. (2022). Rapid improvement in soil health following the conversion of abandoned farm fields to annual or perennial agroecosystems. Front. Sustain. Food Syst. 6, 1010298. doi: 10.3389/fsufs.2022.1010298
de Freitas, W. K., Portz, A., de Carvalho Peres, A. A., Tarré, R. M., and de Melo Campos, M. (2018). Soil nutrient content and plant phytosociology in agroforestry systems of the Rio de Janeiro State highlands, Brazil. Acta Sci. Biol. Sci. 40, 1–8. doi: 10.4025/actascibiolsci.v40i1.35368
de Oliveira, S. P., de Lacerda, N. B., Blum, S. C., Escobar, M. E. O., and de Oliveira, T. S. (2015). Organic carbon and nitrogen stocks in soils of northeastern Brazil converted to irrigated agriculture. Land Degrad. Dev. 26, 9–21. doi: 10.1002/ldr.2264
Fan, L., and Han, W. (2020). Soil respiration after forest conversion to tea gardens: a chronosequence study. Catena 190, 104532. doi: 10.1016/j.catena.2020.104532
Franzluebbers, A. J. (2012). Grass roots of soil carbon sequestration. Carbon Manag. 3, 9–11. doi: 10.4155/cmt.11.73
Fuglie, K., Peters, M., and Burkart, S. (2021). The extent and economic significance of cultivated forage crops in developing countries. Front. Sustain. Food Syst. 5, 712136. doi: 10.3389/fsufs.2021.712136
Ghosh, A., Das, A., Das, D., Ray, P., Bhattacharyya, R., Biswas, D. R., et al. (2020). Contrasting land use systems and soil organic matter quality and temperature sensitivity in North Eastern India. Soil Till. Res. 199, 104573. doi: 10.1016/j.still.2020.104573
Ghosh, A., Kumar, R. V., Manna, M. C., Singh, A. K., Parihar, C. M., Kumar, S., et al. (2021). Eco-restoration of degraded lands through trees and grasses improves soil carbon sequestration and biological activity in tropical climates. Ecol. Eng. 162, 106176. doi: 10.1016/j.ecoleng.2021.106176
Ghosh, A., Kumar, S., Manna, M. C., Singh, A. K., Sharma, P., Sarkar, A., et al. (2019). Long-term in situ moisture conservation in horti-pasture system improves biological health of degraded land. J. Environ. Manag. 248, 109339. doi: 10.1016/j.jenvman.2019.109339
Gomez, K. A., and Gomez, A. A. (1984). Statistical Procedures for Agricultural Research. New York, NY: John Wiley & Sons.
Guyonnet, J. P., Cantarel, A. A., Simon, L., and Haichar, F. E. Z. (2018). Root exudation rate as functional trait involved in plant nutrient-use strategy classification. Ecol. Evol. 8, 8573–8581. doi: 10.1002/ece3.4383
Hanway, J. J., and Heidel, H. (1952). Soil Analysis Methods as Used in Iowa State College Soil Testing Laboratory, Bulletin 57. Ames, IA: Iowa State College of Agriculture. p. 131.
Hu, H., Umbreen, S., Zhang, Y., Bao, M., Huang, C., and Zhou, C. (2021). Significant association between soil dissolved organic matter and soil microbial communities following vegetation restoration in the Loess Plateau. Ecol. Eng. 169, 106305. doi: 10.1016/j.ecoleng.2021.106305
Jenkinson, D. S., and Powlson, D. S. (1976). The effects of biocidal treatments on metabolism in soil—V: A method for measuring soil biomass. Soil Biol. Biochem. 3, 209–213.
Jobbágy, E. G., and Jackson, R. B. (2004). The uplift of soil nutrients by plants: biogeochemical consequences across scales. Ecology 85, 2380–2389. doi: 10.1890/03-0245
Kooch, Y., Sanji, R., and Tabari, M. (2019). The effect of vegetation change in C and N contents in litter and soil organic fractions of a Northern Iran temperate forest. Catena 178, 32–39. doi: 10.1016/j.catena.2019.03.009
Lehmann, J. (2007). Bio-energy in the black. Front. Ecol. Environ. 5, 381–387. doi: 10.1890/1540-9295(2007)5381:BITB2.0.CO;2
Li, C., Fultz, L. M., Moore-Kucera, J., Acosta-Martínez, V., Horita, J., Strauss, R., et al. (2017). Soil carbon sequestration potential in semiarid grass- lands in the Conservation Reserve Program. Geoderma 294, 80–90. doi: 10.1016/j.geoderma.2017.01.032
Nosetto, M. D., Jobbágy, E. G., and Paruelo, J. M. (2006). Carbon sequestration in semiarid rangelands: comparison of Pinus ponderosa plantations and grazing exclusion in NW Patagonia. J. Arid Environ. 67, 142–156. doi: 10.1016/j.jaridenv.2005.12.008
Notenbaert, A. M., Douxchamps, S., Villegas, D. M., Arango, J., Paul, B. K., Burkart, S., et al. (2021). Tapping into the environmental Co-benefits of improved tropical forages for an agroecological transformation of livestock production systems. Front. Sustain. Food Syst. 5, 742842. doi: 10.3389/fsufs.2021.742842
Ola, A., Dodd, I. C., and Quinton, J. N. (2015). Can we manipulate root system architecture to control soil erosion? Soil 1, 603–612. doi: 10.5194/soil-1-603-2015
Olsen, S. R. (1954). Estimation of available phosphorus in soils by extraction with sodium bicarbonate (No. 939). US Department of Agriculture.
Paramesh, V., Singh, S. K., Mohekar, D. S., Arunachalam, V., Misra, S. D., Jat, S. L., et al. (2022). Impact of sustainable land-use management practices on soil carbon storage and soil quality in Goa State, India. Land Degrad. Dev. 33, 28–40. doi: 10.1002/ldr.4124
Parsapour, M. K., Kooch, Y., Hosseini, S. M., and Alavi, S. J. (2018). Litter and topsoil in Alnus subcordata plantation on former degraded natural forest land: a synthesis of age-sequence. Soil Till. Res. 179, 1–10. doi: 10.1016/j.still.2018.01.008
Perring, M. P., Standish, R. J., and Price, J. N. (2015). Advances in restoration ecology: rising to the challenges of the coming decades. ESA J. 2 6, 1–25. doi: 10.1890/ES15-00121.1
Prajapati, M. C., Phadake, A. B., and Agrawal, M. C. (1973). Studies on suitability of grasses for protection of field earthen structures in Agra region of Yamuna ravines. Ind. For. 99, 193–204.
Pulleman, M., Creamer, R., Hamer, U., Helder, J., Pelosi, C., Peres, G., et al. (2012). Soil biodiversity, biological indicators and soil ecosystem services—an overview of European approaches. Curr. Opin. Environ. Sustain. 4, 529–538. doi: 10.1016/j.cosust.2012.10.009
Rai, S. K., Dixit, A. K., Choudhary, M., and Kumar, S. (2018). Climatic variability and prediction of annual rainfall using stochastic time series model at Jhansi in central India. Mausam 69, 73–80. doi: 10.54302/mausam.v69i1.232
Ram, B., Singh, A. P., Singh, V. K., Durgude, S. A., and Nath, A. (2022). Effect of different land-use systems on microbial population and urease enzyme activity in a mollisol. Biol. For. 14, 455–459.
Sainepo, B. M., Gachene, C. K., and Karuma, A. (2018). Assessment of soil organic carbon fractions and carbon management index under different land use types in Olesharo Catchment, Narok County, Kenya. Carbon Bal. Manag. 13, 1–9. doi: 10.1186/s13021-018-0091-7
Tambara, A. A. C., Sippert, M. R., Jauris, G. C., Flores, J. L. C., Henz, É. L., and Velho, J. P. (2017). Production and chemical composition of grasses and legumes cultivated in pure form, mixed or in consortium. Acta Sci. Anim. Sci. 39, 235–241. doi: 10.4025/actascianimsci.v39i3.34661
Tellen, V. A., and Yerima, B. P. (2018). Effects of land use change on soil physicochemical properties in selected areas in the North West region of Cameroon. Environ. Syst. Res. 7, 1–29. doi: 10.1186/s40068-018-0106-0
Waliszewska, B., Grzelak, M., Gawe,ł, E., Spek-Dzwigała, A., Sieradzka, A., and Czekała, W. (2021). Chemical characteristics of selected grass species from polish meadows and their potential utilization for energy generation purposes. Energies 14, 1669. doi: 10.3390/en14061669
Wang, B., Xue, S., Liu, G. B., Zhang, G. H., Li, G., and Ren, Z. P. (2012). Changes in soil nutrient and enzyme activities under different vegetations in the Loess Plateau area, Northwest China. Catena 92, 186–195. doi: 10.1016/j.catena.2011.12.004
Wani, P. A., Khan, M. S., and Zaidi, A. (2007). Synergistic effects of the inoculation with nitrogen-fixing and phosphate-solubilizing rhizobacteria on the performance of field-grown chickpea. J. Plant Nutr. Soil Sci. 170, 283–287. doi: 10.1002/jpln.200620602
Keywords: grass cover, biomass productivity, nutrient recycling, biological activity, climate regulation
Citation: Patidar P, Sannagoudar MS, Ghosh A, Singh AK, Misra S, Khandibagur V, Ojha D, Casini R, Elansary HO and Chandra A (2023) Tropical range grasses can sustain soil functions despite nutrient depletion in semiarid degraded land. Front. Sustain. Food Syst. 7:1230156. doi: 10.3389/fsufs.2023.1230156
Received: 28 May 2023; Accepted: 08 August 2023;
Published: 13 September 2023.
Edited by:
Carmen Carmona, University of Stirling, United KingdomReviewed by:
Rajendra Kumar Yadav, Agriculture University, Kota, IndiaCheng Meng, University of Oklahoma, United States
Copyright © 2023 Patidar, Sannagoudar, Ghosh, Singh, Misra, Khandibagur, Ojha, Casini, Elansary and Chandra. This is an open-access article distributed under the terms of the Creative Commons Attribution License (CC BY). The use, distribution or reproduction in other forums is permitted, provided the original author(s) and the copyright owner(s) are credited and that the original publication in this journal is cited, in accordance with accepted academic practice. No use, distribution or reproduction is permitted which does not comply with these terms.
*Correspondence: Avijit Ghosh, YXZpaml0Lmdob3NoJiN4MDAwNDA7aWNhci5nb3YuaW4=
†Present address: Manjanagouda S. Sannagoudar, ICAR-Indian Institute of Seed Science, Regional Station, Bengaluru, India