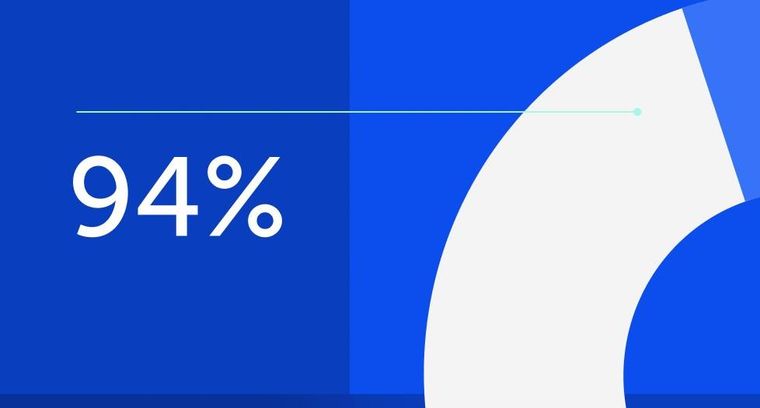
94% of researchers rate our articles as excellent or good
Learn more about the work of our research integrity team to safeguard the quality of each article we publish.
Find out more
ORIGINAL RESEARCH article
Front. Sustain. Food Syst., 20 July 2023
Sec. Sustainable Food Processing
Volume 7 - 2023 | https://doi.org/10.3389/fsufs.2023.1223027
This article is part of the Research TopicRecent Knowledge on the Applications of Molecular Hydrogen in Plant Physiology, Crop Production, and Food ProcessingView all 5 articles
The impact of hydrogen infusion into solvents on the extraction of phytochemicals was evaluated. Pure (water, ethanol, methanol) and hydrogen-rich solvents at temperatures (25°C and 35°C) were used for extracting phenolics, flavonoids, anthocyanins, and antioxidants from lemon peel. Incorporating hydrogen into all solvents increased the extraction of all phytochemicals. The hydrogen incorporation into solvents led to the highest increase in phenolics and flavonoid contents shown for hydrogen-rich methanol (HRM) followed by hydrogen-rich ethanol (HRE) at both 25°C and 35°C. The highest antioxidant extraction was shown for HRM. The hydrogen incorporation showed the highest phytochemical extraction in methanol and at 35°C. The increase in temperature increased the extraction efficiency, but less efficiently than the hydrogen incorporation. The HPLC phenolic profile confirmed the positive effect of both temperature and hydrogen incorporation on the extraction of flavonoids and non-flavonoids. The improvement of the phytochemical extraction by the proposed method can bring many economic and ecological advantages.
A huge agricultural and food waste is produced annually worldwide forming adverse effects on the environment, economy, and society. The total global food losses and waste have been estimated to be about 1.3 billion tons annually with a value of 1,000 billion dollars. Although the best choice is the prevention and reduction of the quantity of these agricultural and food wastes, their valorization is considered the best strategy when other measures are not possible (Capanoglu et al., 2022).
Agro-food wastes are phytochemical-rich sources with a significant added value that can be used in different industries such as cosmetics, pharmacy, and food. Intensive research has been carried out around the world to evaluate the phytochemical-rich food and agricultural wastes such as fruit seeds and peels (Wolfe et al., 2003; Yan et al., 2006; Ghasemi et al., 2009). On the other hand, the global demand for phytochemicals is exponentially increasing due to the awareness of their beneficial health properties and consumer anxiety about the potentially harmful effects of synthetic additives used during food production. The use of plant extracts in their raw form or their pure phytochemicals may remove the concerns of consumers (Rao and Rathod, 2019).
Food and agricultural wastes are considered a significant source of many nutrients and phytochemicals such as carbohydrates, proteins, lipids, vitamins, phenolics, flavonoids, anthocyanins, and carotenoids. Fruit peels contain significant levels of phytochemicals compared with the edible part such as phenolics, flavonoids, carotenoids, and anthocyanins (Shrikhande, 2000; Someya et al., 2002; George et al., 2004; Gorinstein et al., 2004). The valuable phytochemicals are generally obtained from these wastes by extraction methods. However, increasing the efficiency and choosing the appropriate extraction method and conditions with economic feasibility form one of the main challenges for industries and researchers. For example, the high volume of solvent used in conventional extraction methods generates a huge amount of toxic and expensive chemical waste. Therefore, the reduction of solvent consumption, the increase in extraction efficiency, and the use of greener solvents are considered the best measures for a more effective, cleaner, and greener extraction process (Capanoglu et al., 2022). Although many methods have been proposed for extracting phytochemicals, the reduction of energy and solvent consumption was the main challenge from an economic and environmental point of view (Capanoglu et al., 2022). Several advanced methods have been proposed to overcome the disadvantages of conventional methods, however, the costly equipment needs, the destruction of thermolabile phytochemicals, the difficulties in the process optimization at the industrial scale, and the high energy consumption are the main limitations of these novel methods (Xu et al., 2017; Martillanes et al., 2018). Thus, the search for methods that are eco-friendly, cost-effective, have low energy consumption, and minimal equipment and pretreatment needs are the main goals of researchers and processors.
Molecular hydrogen (H2) is the simplest and smallest molecule in nature with many beneficial biological effects shown in the plant, animal, and human studies (Buchholz et al., 2008; Cachon and Alwazeer, 2019; Alwazeer et al., 2021). Although it has low solubility in water, significant beneficial impacts of H2 on the protection of antioxidants, phenolics, flavonoids, and pigments of different food products have been reported (Alwazeer, 2018, 2019, 2020; Alwazeer and Örs, 2019; Alwazeer et al., 2020; Alwazeer and Özkan, 2022). These beneficial effects of H2 might be related to its reducing and antioxidant properties, small size, and high diffusion rate in the atmosphere and tissues (Alwazeer, 2020; Alwazeer et al., 2021). In recently published studies, our team showed that the incorporation of hydrogen gas into both methanol or hexane improved the extraction of antioxidants and phenolics from vegetable oils (Ceylan et al., 2023), by-products (Alwazeer et al., 2023b,c) and agri-food wastes (Alwazeer and Elnasanelkasi, 2023; Alwazeer et al., 2023a).
This study aimed to evaluate the effects of hydrogen incorporation into different solvents and the temperature on the efficiency of the phytochemical extraction from the lemon peel.
Lemon fruit (Citrus lemon L.) was obtained from a local market. Whole lemon peels were dry-lyophilized (Christ, Alpha 1–2 LD, Germany) then ground into powder by a plastic mortar and stored at −80°C until analysis.
All standards and chemicals (ABTS, DPPH, Trolox, quercetin, ascorbic acid, 3,4,5 trihydroxy benzoic acid, Folin–Ciocalteu reagent, potassium persulfate, ethanol, methanol, and others) were purchased from Sigma (MO, United States) and Merck (Darmstadt, Germany).
One gram of peel-dried powder was added to 20 mL of solvent [pure water, ethanol, methanol, hydrogen-rich water (HRW), hydrogen-rich ethanol (HRE), hydrogen-rich methanol (HRM)] followed by 24 h-mixing phase in a shaking incubator at 25°C and 35°C and 120 rpm. Hydrogen-rich solvents were prepared by bubbling pure hydrogen gas (99.9%, Elite Gaz Teknolojileri, Ankara, Turkey) in solvent for 3 min at 1 L/min. The mixtures were then filtered through filter paper and the solvent was evaporated by a rotary evaporator (35°C) for methanolic and ethanolic solvents and by vacuum drying (35°C) for aqueous ones. The extracts were then kept at −80°C until analysis. All extracts were dissolved (1 mg/mL) in pure water (Millipore, Milli-Q, United States) for phenolics, flavonoids, and antioxidant activity analysis, and in methanol (80%) for HPLC analysis.
1 mL of peel extract was added to a mixture of 2.3 mL pure water and 1 mL of Folin-Ciocalteau reagent followed by a vortex step for 30 s. The mixture was then incubated at 25°C for 5 min in the dark. Afterward, 2 mL of 7% Na 2CO 3 was added, and the mixture was then incubated for 30 min at 25°C in the dark. The absorbance was then measured using a spectrophotometer (AQUA-MATE UV–VIS, China) at 765 nm. Gallic acid was used as a standard and total phenolics content was calculated as mg Gallic Acid Equivalent (mg GAE) per g dry extract.
300 μL of peel extract was added to a mixture of 150 μL of aluminum chloride solution (50 g/L) and 2,550 μL of methanol. The mixture was then kept in the dark at 25°C for 30 min. The absorbance was then read at 425 nm. Quercetin was used as a standard, and flavonoid content was calculated as mg Quercetin Equivalent (mg QE) per g dry extract.
The content of anthocyanins was determined using the pH differential method using hydrochloric acid and potassium chloride solution (pH 1) and sodium acetate solution (pH 4.5). 2 mL of peel extract was diluted separately with solutions of pH 1 and pH 4.5 to a total of 25 mL. The mixtures were then incubated at room temperature for 30 min. The absorbance was then read at 510 nm and 700 nm using a spectrophotometer (AQUA-MATE UV–VIS, China). The concentration of anthocyanins was determined according to the following equation:
where:
Peel extract (0.5 mL) was added to 2.5 mL of DPPH solution (6 × 10−5 M). The DPPH mixture was then incubated at room temperature for 90 min and the absorbance was measured at 515 nm. DPPH scavenging activity was expressed as mg Ascorbic Acid Equivalent (mg AAE) per g dry extract and calculated according to the following formula:
An ABTS solution (7 mM) containing 2.45 mM potassium persulfate was prepared and kept in the dark at room temperature for 12–16 h. A sodium acetate (20 mM) solution was prepared, and the pH value was adjusted to 4.5 with 0.1 N HCl. The prepared ABTS solution was diluted with 20 mM sodium acetate to obtain an absorbance of 0.700 ± 0.01 nm at 734 nm. 100 μL of peel extract was mixed with 1900 μL of diluted ABTS solution, and at the end of 5 min, the absorbance was measured at 734 nm. ABTS scavenging activity was expressed as μmol Trolox Equivalent (μmol TE) per g dry extract and calculated according to the following formula:
The phenolic compounds profile was determined by the HPLC method according to Alwazeer and co-workers (Alwazeer and Elnasanelkasi, 2023). Methanolic lemon peel extract solution (1 g/mL) was filtrated through a 0.22 μm membrane filter (Millex HV13, Millipore) and then placed in 1.5 mL HPLC vials (Agilent, screw tab 5,182-0176). A reverse phase C18 column was used.
Results were subjected to one-way ANOVA with GraphPad Prism 9 Software and the degree of significance was denoted as **p < 0.01 and ***p < 0.001 and Tukey’s post hoc tests followed by Duncan’s multiple comparison test using the IBM SPSS Statistics 26 package program. Statistically significant differences were considered at the level of p < 0.05. All assays were performed in three replicates (n = 3).
Table 1 shows the total phenolic contents of lemon peel extracts obtained by pure solvents (water, ethanol, methanol) and hydrogen-rich solvents (HRW, HRE, HRM) at 25°C and 35°C.
Table 1. Total phenolics, flavonoids, and monomeric anthocyanins contents of lemon peel extract according to temperature and hydrogen incorporation.
At 25°C, the highest levels of total phenolics content of lemon peel were obtained for methanol in the pure (non-H2) solvents group and hydrogen-rich methanol (HRM) for the hydrogen-rich solvents group with 18.31 and 27.31 mg GAE/g extract, respectively (Table 1). Whereas the lowest ones were observed for ethanol in the pure non-H2 solvents and HRW for the hydrogen-rich solvents with 16.29 and 23.58 mg GAE/g extract. The same trend was observed for 35°C.
The incorporation of hydrogen into solvents led to a significant increase in phenolic matter extraction by 35.05%, 49.15%, and 53.52% at 25°C, and by 35.85%, 48.16%, and 57.63% at 35°C for HRW, HRE, and HRM, respectively (p < 0.0001) (Supplementary Table S1 and Figures 1A,B). Results show that incorporating hydrogen into solvent led to the highest increase in phenolic extraction shown for HRM followed by HRE at both 25°C and 35°C. These results reveal that hydrogen incorporation into solvents was more potent for phenolic extraction, especially in the case of methanol and at the higher temperature, 35°C.
Figure 1. Total phenolics content of lemon peel extract according to hydrogen incorporation (A,B) and temperature (C,D) (nsp > 0.05, *p < 0.05, **p < 0.01, ***p < 0.001, ****p < 0.0001).
The rise in the extraction temperature from 25 to 35°C led to a significant increase in phenolic extraction by 3.66, 6.93, and 3.05% for non-hydrogen incorporated water, ethanol, and methanol, respectively (p < 0.05, p < 0.01, and p < 0.0001); whereas, it increased by 4.28%, 5.45%, and 5.45% for HRW, HRE, and HRM, respectively (p < 0.05 and p < 0.01) (Supplementary Table S1 and Figures 1C,D).
Table 1 shows the total flavonoid contents of lemon peel extracts obtained by pure solvents (water, methanol, ethanol) and hydrogen-rich solvents (HRW, HRE, HRM) at 25°C and 35°C.
At 25°C, while there was no difference in flavonoid content between non-hydrogen-infused solvents, HRM showed the highest value of flavonoid extraction from the lemon peel in the hydrogen-rich solvents group (Table 1). The same trend was observed for 35°C.
The incorporation of hydrogen into solvents led to a significant increase in flavonoid extraction by 20.59%, 30.33%, and 41.5% at 25°C, whereas it increased by 10.6%, 18.78%, and 26.83% at 35°C for HRW, HRE, and HRM, respectively (p < 0.0001) (Supplementary Table S1 and Figures 2A,B). Incorporating hydrogen into solvents led to the highest increase in flavonoid extraction shown for HRM followed by HRE at both 25°C and 35°C. These results reveal that hydrogen incorporation into solvents was the most potent method for extracting flavonoids, especially in the case of methanol and at 35°C.
Figure 2. Total flavonoids of lemon peel extract according to hydrogen incorporation (A,B) and temperature (C,D) (nsp > 0.05, *p < 0.05, **p < 0.01, ***p < 0.001, ****p < 0.0001).
The rise in the extraction temperature from 25°C to 35°C led to a significant increase in flavonoid extraction by 16.47%, 15.75%, and 16.86% for non-hydrogen-infused water, ethanol, and methanol (p < 0.001 and p < 0.0001); and by 6.83%, 5.49%, and 4.74% for HRW, HRE, and HRM, respectively (p < 0.01) (Supplementary Table S1 and Figures 2C,D).
Table 1 shows the total monomeric anthocyanins of lemon peel extracts obtained by pure solvents (water, ethanol, methanol) and hydrogen-rich solvents (HRW, HRE, HRM) at 25°C and 35°C.
At 25°C, there was no significant difference in the total anthocyanin content among non-hydrogen-infused solvents and hydrogen-infused solvents (Table 1). The same trend was observed for 35°C.
The total amount of anthocyanins in lemon extracts obtained with hydrogen-rich solvents (HRW, HRE, HRM) was generally higher than that of the extracts obtained with non-hydrogen-infused (pure) solvents.
The infusion of hydrogen into solvents led to a significant increase in total anthocyanins content by 85.22%, 301.99%, and 250.66% at 25°C, and by 147.4%, 233.05%, and 251.19% at 35°C for HRW, HRE, and HRM, respectively (p < 0.05, p < 0.01, and p < 0.001) (Supplementary Table S1 and Figures 3A,B). The incorporation of hydrogen into solvent led to the highest extraction increase of total anthocyanins content shown for HRE at 25°C and HRM at 35°C.
Figure 3. Total monomeric anthocyanins of lemon peel extract according to hydrogen incorporation (A,B) and temperature (C,D) (nsp > 0.05, *p < 0.05, **p < 0.01, ***p < 0.001, ****p < 0.0001).
The rise of extraction temperature from 25°C to 35°C led to a significant increase in anthocyanin extraction by 42.04, 136.00, and 66.66% for non-hydrogen-infused water, ethanol, and methanol, respectively (nsp > 0.05 and p < 0.05); and by 175.99%, 95.52%, and 66.92% for HRW, HRE, and HRM, respectively (p < 0.01) (Supplementary Table S1 and Figures 3C,D).
Table 2 shows the DPPH radical scavenging activity of lemon peel extracts obtained by pure solvents (water, ethanol, methanol) and hydrogen-rich solvents (HRW, HRE, HRM) at 25°C and 35°C.
Table 2. DPPH and ABTS scavenging activity of lemon peel extract according to temperature and hydrogen incorporation.
At 25°C, there was no difference in levels of DPPH scavenging activity of lemon peel between different solvents in the pure solvents group. However, HRM showed the highest value in the hydrogen-rich solvents group with 12.62 mg AAE/g extract, whereas the lowest one was observed for HRW in the hydrogen-rich solvents group with 9.85 mg AAE/g dry extract (Table 2). The same trend was observed for 35°C.
The incorporation of hydrogen into solvents led to a significant increase in DPPH radical scavenging activity by 25%, 53.7%, and 62.4% at 25°C, and increased by 108.9%, 131.5%, and 161.0% at 35°C for HRW, HRE, and HRM, respectively (p < 0.0001) (Supplementary Table S2 and Figures 4A,B). The incorporation of hydrogen into solvents led to the highest increase in DPPH scavenging activity shown for HRM followed by HRE at both 25°C and 35°C. These results reveal that the hydrogen incorporation into solvents was more potent for extracting antioxidants from the lemon peel (expressed by DPPH scavenging activity), especially in the case of methanol and at 35°C.
Figure 4. DPPH scavenging activity of lemon peel extract according to hydrogen incorporation (A,B) and temperature (C,D) (nsp > 0.05, *p < 0.05, **p < 0.01, ***p < 0.001, ****p < 0.0001).
The rise in extraction temperature from 25°C to 35°C led to a significant increase in DPPH radical scavenging activity by 7.1%, 9.4%, and 6.8% for non-hydrogen-infused water, ethanol, and methanol, respectively (p < 0.001 and p < 0.0001); and by 79%, 64.8%, and 71.6% for HRW, HRE, and HRM, respectively (p < 0.0001) (Supplementary Table S2 and Figures 4C,D).
Table 2 shows the ABTS radical scavenging activity of lemon peel extracts obtained by pure solvents (water, ethanol, methanol) and hydrogen-rich solvents (HRW, HRE, HRM) at 25°C and 35°C.
At 25°C, the highest levels of ABTS scavenging activity were obtained for water in the pure (non-hydrogen-infused) solvents and HRM in the hydrogen-rich solvents with 10.37 and 12.63 mg TE/g extract, respectively (Supplementary Table S2). The lowest ones were observed for methanol in the non-hydrogen-infused solvents and HRW for the hydrogen-rich solvents with 8.76 and 11.87 mg TE/g extract. The same trend was observed for 35°C.
The incorporation of hydrogen into solvents led to a significant increase in the extraction of antioxidants (expressed by ABTS radical scavenging activity) by 14.46%, 34.02%, and 44.06% at 25°C and increased by 24.06%, 43.67%, and 58.42% at 35°C for HRW, HRE, and HRM, respectively (p < 0.0001) (Supplementary Table S2 and Figures 5A,B). The incorporation of hydrogen into solvents led to the highest increase in antioxidant extraction (expressed by ABTS scavenging activity) shown for HRM followed by HRE at both 25°C and 35°C.
Figure 5. ABTS scavenging activity of lemon peel extract according to hydrogen incorporation (A,B) and temperature (C,D) (nsp > 0.05, *p < 0.05, **p < 0.01, ***p < 0.001, ****p < 0.0001).
The rise in extraction temperature from 25°C to 35°C led to a significant increase in the extraction of antioxidants (expressed by ABTS radical scavenging activity) by 5.78%, 6.4%, and 11.75% for non-hydrogen-infused water, ethanol, and methanol, respectively (p < 0.01 and p < 0.0001); and by 14.65%, 11.48%, and 22.90% for HRW, HRE, and HRM, respectively (p < 0.0001) (Supplementary Table S2 and Figures 5C,D). These results reveal that the hydrogen incorporation into solvents was most potent for the extraction of antioxidants, especially in the case of methanol and at 35°C.
Phenolic compounds found in lemon extracts are shown in Table 3. The phenolic compound amounts of pure water extracts at 25°C can be ranked as follows: catechin > epicatechin > chlorogenic acid > gallic acid > rutin > trans-ferulic acid > rosmarinic acid > p-coumaric acid (Table 3). Caffeic acid was not detected in pure water and HRW extracts. This may be due to its hydrophobic property and its low solubility in water (Letsididi et al., 2018). Moreover, the amount of quercetin in the extracts could not be determined because its level was below the detection limit of the instrument.
Table 3. Effect of hydrogen incorporation into solvent and temperature increase on the phenolic compounds profile of lemon peel.
In non-hydrogen-infused solvents at 25°C, the content of chlorogenic acid and epicatechin compounds was in the following order: methanol >water >ethanol; while that of catechin and gallic acids was in the following order: water>methanol>ethanol. In the hydrogen-rich water group at 25°C, the amount of chlorogenic acid, catechin, and epicatechin compounds increased by 76.55%, 9.52%, and 137.15%, and at 35°C, it increased by 18.50%, 13.02%, and 49.29%, respectively.
HPLC analysis shows that the increase in temperature from 25°C to 35°C increased the extraction of gallic acid (hydroxybenzoic acid) by 150%, 230%, and 401% for pure water, ethanol, and methanol, and by 49%, 141%, and 42% for H2-rich solvents, respectively (Supplementary Table S3). Similarly, the hydroxycinnamic acids increased up to 187% for ferulic acid in pure ethanol and 67% for caffeic acid in pure methanol. Regarding flavonoids, epicatechin (flavan-3-ols) increased up to 121% and 36% for pure water and HRW, respectively; while rutin (flavonoid glycoside) increased by 93% and 117% for pure ethanol and HRW, respectively (Supplementary Table S3). It can be noted that when the temperature of extraction increased from 25°C to 35°C, the majority of the phenolic compounds had increased extraction.
HPLC analysis shows also an increase in both flavonoid and non-flavonoid amounts when H2-solvents had been used in the extraction process. This increase was shown notably for all solvents at 25°C and for HRW at 35°C (Supplementary Table S4).
The results of this study show an increase in all phytochemicals when hydrogen had been incorporated into various solvents. It could be expected that the increase in TPC found in HRW samples was due to the preservation of phenolic substances from oxidative reactions and maybe by the liberation of conjugated and cell wall-bound phenolic substances. On the other hand, the standard reduction potential of 2H+/H2 involved in the biological electron transfer system equals −420 mV (at pH 7) (Roehm, 2001) showing that dissolved hydrogen possesses a possible thermodynamic reducing property to help maintain the redox homeostasis inside the cell and preserve phenolics and antioxidants from oxidative reactions (Hu et al., 2021). It has been reported that the incorporation of SO2 (reducing agent) into the water, which forms a solution with redox properties similar to that of HRW, improved the extraction of anthocyanins from red wine grapes (Bakker et al., 1998) and phenolics from milled black currants (Cacace and Mazza, 2003). However, the reactivity of SO2 in aqueous solutions is significantly greater than H2. In contrast to H2, when dissolved in protic solvents, SO2 acts as a Lewis acid to form the ephemeral sulphurous acid (H2SO3), which then immediately results in its conjugate bases of bisulfate (HSO3−) and sulfite ions (SO32−). These anions are excellent reducing agents that can remove chlorine, oxygen, and other oxidants from solution, which molecular hydrogen cannot do. However, the use of SO2 in food products is limited due to its harmful effects on health.
On the other hand, polyphenol oxidase (PPO) and phenolic compounds are localized separately inside the cell in a latent state. During pretreatment phases such as grinding, PPO and phenolic substances are liberated and come in contact, and the oxidation of phenolics is initiated producing o-quinones that can oxidize other phenolic compounds (Renard et al., 2017). The o-quinones are submitted to disproportionation to form semiquinone radicals that react with O2 to generate superoxide anions (●O2−) and the regeneration of o-quinones (Boeckx et al., 2015). The formed superoxide anions will be dismutated either enzymatically via superoxide dismutase or non-enzymatically to form hydrogen peroxide (H2O2). Hydrogen peroxide can react with divalent metal ions such as iron (II) via a Fenton reaction to generate extremely non-selective oxidant hydroxyl radicals (●OH) (Boeckx et al., 2015). Since the infusion of hydrogen gas would have removed any dissolved oxygen gas, then it is possible that fewer oxygen radicals (e.g., ●O2-, ●OH) were produced because there was not enough O2 to react with semiquinone radicals. Similarly, since O2 itself can induce oxidation as a diradical, then having less O2 available would likewise result in less oxidation of phytochemicals. Further studies with other inert gases (e.g., He, N2, etc.) may help elucidate the chemical mechanism for these observed effects.
The phenolics, which have a high molecular weight and are attached to the plant cell wall, might pass into free form with the effect of high temperature leading to an increase in the total phenolics content (Shofian et al., 2011). In the literature, it has been reported that the total phenolic content of lemon peels extracted with methanol:water (80:20, V/V) ranged between 3.17 and 4.63 mg GAE/g wet product (Xi et al., 2017), the flavonoid content extracted with ethanol was 7.12 μg QE/g wet product (Leja et al., 2013), and the total anthocyanin content extracted with water was 3.98–22.87 μg/g dry product (Guzel and Akpinar, 2017). It has been found that the DPPH● scavenging capacity of lemon peel extract obtained with methanol: water (80:20/ v: v) was 7.45 μmol TE/g wet weight (Xi et al., 2017), the ABTS+ scavenging capacity of extract obtained by methanol:water (80:20, V/V) was 8.65 μmol TE /g wet weight (Xi et al., 2017), and the phenolic profile of aqueous extracts was in the following order: rutin> trans-ferulic acid> P-coumaric acid (Gomez-Mejia et al., 2019).
Another possibility may have to do with the possible formation of hydrogen bubbles that occurred during the mixing process. The saturated levels of hydrogen in solution make it easy for gas bubble formation due available gaseous solutes and abundant nucleation sites. The bubbles may undergo cavitation and bubble degradation, which is known to facilitate the release of plant metabolites from intracellular structures (Blicharski and Oniszczuk, 2017). This assumption will be investigated in a future research.
The higher solubility of H2 in methanol compared to water and ethanol (Young, 1981), may explain the advantage of the use of methanol over water and ethanol for the extraction of antioxidants. On the other hand and contrary to water, the solubility of hydrogen in n-alcohols such as methanol and ethanol increases with the increase in temperature (D‘Angelo and Francesconi, 2001). This phenomenon may explain the reasons behind the high extraction efficiency of antioxidants, phenolics, flavonoids, and anthocyanins at 35°C compared with 25°C.
Agro-food wastes are phytochemical-rich sources with a significant added value that can be used in different industries such as cosmetics, pharmacy, and foods. The valorization of agro-food wastes is still an important challenge, especially in light of the increasing crisis of low production of agricultural products due to global warming and climate change. The extraction of phytochemicals from these wastes can bring many economic benefits to industries. In the present study, An improvement in the extraction efficiency of phytochemicals from the lemon peel when hydrogen was incorporated into conventional solvents such as water and n-alcohol was obtained, with the highest increase shown for hydrogen-rich methanol. Additionally, a significant increase in the extraction of the phytochemical could be obtained when the extraction temperature was increased. The highest extraction levels of phenolics, flavonoids, anthocyanins, and antioxidants were found for the HRM at 35°C. The phenolic profile of lemon peel extract was enhanced by both hydrogen incorporation and temperature increase. It should be noted that the incorporation of hydrogen into solvents was more effective in improving the extraction efficiency of phytochemicals than increasing the temperature. The incorporation of hydrogen into water led to significant improvement in phytochemical extraction, which can be considered a green extraction process. This method of hydrogen incorporation into solvents may be an interesting alternative to hazardous and expensive organic solvents and has various economic and ecological advantages. Future research should investigate the effect of other inert gases to determine if the increased extraction is unique to the physical chemistry properties of hydrogen, or simply to the removal of O2 and inclusion of gaseous solutes and bubbles that may have been produced during the mixing.
The raw data supporting the conclusions of this article will be made available by the authors, without undue reservation.
DA: conceptualization, methodology, validation, visualization, and supervision. ME: formal analysis. ME, TE, AÇ, and TL: investigation. DA and ME: writing—original draft preparation. DA, ME, and TL: writing—review and editing. DA: project administration. All authors contributed to the article and approved the submitted version.
TE and AÇ are 100/2000 Ph.D. Scholars of the Council of Higher Education (CoHE) in the Bioengineering and Sciences program in Turkiye.
The authors declare that the research was conducted in the absence of any commercial or financial relationships that could be construed as a potential conflict of interest.
All claims expressed in this article are solely those of the authors and do not necessarily represent those of their affiliated organizations, or those of the publisher, the editors and the reviewers. Any product that may be evaluated in this article, or claim that may be made by its manufacturer, is not guaranteed or endorsed by the publisher.
The Supplementary material for this article can be found online at: https://www.frontiersin.org/articles/10.3389/fsufs.2023.1223027/full#supplementary-material
Alwazeer, D. (2018). Reducing atmosphere drying as a new technique for the preservation of the color of dried foods. J. Inst. Sci. Technol. 8, 125–131. doi: 10.21597/jist.418232
Alwazeer, D. (2019). Reducing atmosphere packaging technique for extending the shelf-life of food products. J. Inst. Sci. Technol. 9, 2117–2123. doi: 10.21597/jist.539744
Alwazeer, D. (2020). Importance of consideration of oxidoreduction potential as a critical quality parameter in food industries. Food Res. Int. 132:109108. doi: 10.1016/j.foodres.2020.109108
Alwazeer, D., and Elnasanelkasi, M. M. A. (2023). Hydrogen-rich water as a green solvent for the extraction of phytochemicals from agri-food wastes. Sustain. Chem. Pharm. 33:101035. doi: 10.1016/j.scp.2023.101035
Alwazeer, D., Elnasanelkasim, M. A., Çiçek, S., Engin, T., Çiğdem, A., and Karaoğul, E. (2023a). Comparative study of phytochemical extraction using hydrogen-rich water and supercritical fluid extraction methods. Process Biochem. 128, 218–226. doi: 10.1016/j.procbio.2023.01.022
Alwazeer, D., Elnasanelkasim, M. A., Çiğdem, A., Engin, T., Kanmaz, H., Hayaloglu, A. A., et al. (2023b). Hydrogen incorporation into solvents can improve the extraction of phenolics, flavonoids, anthocyanins, and antioxidants: a case-study using red beetroot. Ind. Crop. Prod. 202:117005. doi: 10.1016/j.indcrop.2023.117005
Alwazeer, D., Elnasanelkasim, M. A., Engin, T., and Çiğdem, A. (2023c). Use of hydrogen-rich water as a green solvent for the extraction of phytochemicals: case of olive leaves. J. Appl. Res. Med. Aromat. Plants, 100472, 35, doi: 10.1016/j.jarmap.2023.100472
Alwazeer, D., Liu, F. F.-C., Wu, X. Y., and LeBaron, W. T. (2021). Combating oxidative stress and inflammation in COVID-19 by molecular hydrogen therapy: mechanisms and perspectives. Oxid. Med. Cell. Longev. doi: 10.1155/2021/5513868, 2021: 5513868
Alwazeer, D., and Örs, B. (2019). Reducing atmosphere drying as a novel drying technique for preserving the sensorial and nutritional notes of foods. J. Food Sci. Technol. 56, 3790–3800. doi: 10.1007/s13197-019-03850-2
Alwazeer, D., Örs, B., and Tan, K. (2020). Reducing atmosphere packaging as a novel alternative technique for extending shelf life of fresh cheese. J. Food Sci. Technol. 57, 3013–3023. doi: 10.1007/s13197-020-04334-4
Alwazeer, D., and Özkan, N. (2022). Incorporation of hydrogen into the packaging atmosphere protects the nutritional, textural and sensorial freshness notes of strawberries and extends shelf life. J. Food Sci. Technol. 59, 3951–3964. doi: 10.1007/s13197-022-05427-y
Bakker, J., Bridle, P., Bellworthy, S. J., Garcia‐Viguera, C., Reader, H. P., and Watkins, S. J. (1998). Effect of sulphur dioxide and must extraction on colour, phenolic composition and sensory quality of red table wine. J. Sci. Food Agri. 78, 297–307. doi: 10.1016/j.procbio.2023.01.022
Blicharski, T., and Oniszczuk, A. (2017). Extraction methods for the isolation of isoflavonoids from plant material. Open Chem. 15, 34–45. doi: 10.1515/chem-2017-0005
Boeckx, T., Winters, A. L., Webb, K. J., and Kingston-Smith, A. H. (2015). Polyphenol oxidase in leaves: is there any significance to the chloroplastic localization? J. Exp. Bot. 66, 3571–3579. doi: 10.1093/jxb/erv141
Buchholz, B. M., Kaczorowski, D. J., Sugimoto, R., Yang, R., Wang, Y., Billiar, T. R., et al. (2008). Hydrogen inhalation ameliorates oxidative stress in transplantation induced intestinal graft injury. Am. J. Transplant. 8, 2015–2024. doi: 10.1111/j.1600-6143.2008.02359.x
Cacace, J. E., and Mazza, G. (2003). Mass transfer process during extraction of phenolic compounds from milled berries. J. Food Eng. 59, 379–389. doi: 10.1016/S0260-8774(02)00497-1
Cachon, R., and Alwazeer, D. (2019). “Quality performance assessment of gas injection during juice processing and conventional preservation technologies” in Value-added ingredients and enrichments of beverages. eds. A. M. Grumezescu and A. M. Holban (Cambridge, MA: Academic Press), 465–485.
Capanoglu, E., Nemli, E., and Tomas-Barberan, F. (2022). Novel approaches in the valorization of agricultural wastes and their applications. J. Agric. Food Chem. 70, 6787–6804. doi: 10.1021/acs.jafc.1c07104
Ceylan, M. M., Silgan, M., Elnasanelkasim, M. A., and Alwazeer, D. (2023). Impact of washing crude olive pomace oil with hydrogen-rich water and incorporating hydrogen into extraction solvents on quality attributes and phytochemical content of oil. J. Food Meas. Charact. 17, 2029–2040. doi: 10.1007/s11694-022-01801-8
D‘Angelo, J. V. H., and Francesconi, A. Z. (2001). Gas−liquid solubility of hydrogen in n-alcohols (1 ≤ n ≤ 4) at pressures from 3.6 MPa to 10 MPa and temperatures from 298.15 K to 525.15 K. J. Chem. Eng. Data 46, 671–674. doi: 10.1021/je000189u
George, B., Kaur, C., Khurdiya, D. S., and Kapoor, H. C. (2004). Antioxidants in tomato (Lycopersium esculentum) as a function of genotype. Food Chem. 84, 45–51. doi: 10.1016/S0308-8146(03)00165-1
Ghasemi, K., Ghasemi, Y., and Ebrahimzadeh, M. A. (2009). Antioxidant activity, phenol and flavonoid contents of 13 citrus species peels and tissues. Pak. J. Pharm. Sci. 22, 277–281.
Gomez-Mejia, E., Rosales-Conrado, N., León-González, M. E., and Madrid, Y. (2019). Citrus peels waste as a source of value-added compounds: extraction and quantification of bioactive polyphenols. Food Chem. 295, 289–299. doi: 10.1016/j.foodchem.2019.05.136
Gorinstein, S., Zachwieja, Z., Katrich, E., Pawelzik, E., Haruenkit, R., Trakhtenberg, S., et al. (2004). Comparison of the contents of the main antioxidant compounds and the antioxidant activity of white grapefruit and his new hybrid. LWT – Food Sci. Technol. 37, 337–343. doi: 10.1016/j.lwt.2003.10.005
Guzel, M., and Akpinar, Ö. (2017). Turunçgil Kabuklarının Biyoaktif Bileşenleri ve Antioksidan Aktivitelerinin Belirlenmesi determination of bioactive compounds and antioxidant activities of Citrus peels. Gümüşhane Üniversitesi Fen Bilim. Derg. 7, 153–167. doi: 10.17714/gufbed.2017.07.010
Hu, H., Li, P., and Shen, W. (2021). Preharvest application of hydrogen-rich water not only affects daylily bud yield but also contributes to the alleviation of bud browning. Sci. Hortic. (Amsterdam). 287:110267. doi: 10.1016/j.scienta.2021.110267
Leja, M., Kamińska, I., Kramer, M., Maksylewicz-Kaul, A., Kammerer, D., Carle, R., et al. (2013). The content of phenolic compounds and radical scavenging activity varies with carrot origin and root color. Plant Foods Hum. Nutr. 68, 163–170. doi: 10.1007/s11130-013-0351-3
Letsididi, K. S., Lou, Z., Letsididi, R., Mohammed, K., and Maguy, B. L. (2018). Antimicrobial and antibiofilm effects of trans-cinnamic acid nanoemulsion and its potential application on lettuce. LWT 94, 25–32. doi: 10.1016/j.lwt.2018.04.018
Martillanes, S., Rocha-Pimienta, J., and Delgado-Adámez, J. (2018). “Agrifood by-products as a source of phytochemical compounds” in Descriptive food science. eds. A. V. Díaz and R. M. García-Gimeno (London, UK: IntechOpen)
Rao, P., and Rathod, V. (2019). Valorization of food and agricultural waste: a step towards greener future. Chem. Rec. 19, 1858–1871. doi: 10.1002/tcr.201800094
Renard, C. M. G. C., Watrelot, A. A., and Le Bourvellec, C. (2017). Interactions between polyphenols and polysaccharides: mechanisms and consequences in food processing and digestion. Trends Food Sci. Technol. 60, 43–51. doi: 10.1016/j.tifs.2016.10.022
Roehm, K.-H. (2001). “Electron carriers: proteins and cofactors in oxidative phosphorylation” In eLS, (Ed.). doi: 10.1038/npg.els.0001373
Shofian, N. M., Hamid, A. A., Osman, A., Saari, N., Anwar, F., Pak Dek, M. S., et al. (2011). Effect of freeze-drying on the antioxidant compounds and antioxidant activity of selected tropical fruits. Int. J. Mol. Sci. 12, 4678–4692. doi: 10.3390/ijms12074678
Shrikhande, A. J. (2000). Wine by-products with health benefits. Food Res. Inter. 33, 469–474. doi: 10.1016/S0963-9969(00)00071-5
Someya, S., Yoshiki, Y., and Okubo, K. (2002). Antioxidant compounds from bananas (Musa Cavendish). Food Chem. 79, 351–354. doi: 10.1016/S0308-8146(02)00186-3
Wolfe, K., Wu, X., and Liu, R. H. (2003). Antioxidant activity of apple peels. J. Agric. Food Chem. 51, 609–614. doi: 10.1021/jf020782a
Xi, W., Lu, J., Qun, J., and Jiao, B. (2017). Characterization of phenolic profile and antioxidant capacity of different fruit part from lemon (Citrus limon Burm.) cultivars. J. Food Sci. Technol. 54, 1108–1118. doi: 10.1007/s13197-017-2544-5
Xu, D.-P., Li, Y., Meng, X., Zhou, T., Zhou, Y., Zheng, J., et al. (2017). Natural antioxidants in foods and medicinal plants: extraction, assessment and resources. Int. J. Mol. Sci. 18:96. doi: 10.3390/ijms18010096
Yan, L. Y., Teng, L. T., and Jhi, T. J. (2006). Antioxidant properties of guava fruit: comparison with some local fruits. Sunw. Acad. J. 3, 9–20.
Keywords: phytochemicals, molecular hydrogen, temperature, extraction, solvents, lemon peel
Citation: Alwazeer D, Elnasanelkasim MA, Çiğdem A, Engin T and LeBaron TW (2023) Incorporation of molecular hydrogen into solvents increases the extraction efficiency of phenolics, flavonoids, anthocyanins, and antioxidants: the case of lemon peels. Front. Sustain. Food Syst. 7:1223027. doi: 10.3389/fsufs.2023.1223027
Received: 16 May 2023; Accepted: 07 July 2023;
Published: 20 July 2023.
Edited by:
Cristobal N. Aguılar, Autonomous University of Coahuila, MexicoReviewed by:
Aidé Sáenz-Galindo, Autonomous University of Coahuila, MexicoCopyright © 2023 Alwazeer, Elnasanelkasim, Çiğdem, Engin and LeBaron. This is an open-access article distributed under the terms of the Creative Commons Attribution License (CC BY). The use, distribution or reproduction in other forums is permitted, provided the original author(s) and the copyright owner(s) are credited and that the original publication in this journal is cited, in accordance with accepted academic practice. No use, distribution or reproduction is permitted which does not comply with these terms.
*Correspondence: Duried Alwazeer, YWx3YXplZXJkQGdtYWlsLmNvbQ==
†YÖK 100/2000 PhD Scholarship
Disclaimer: All claims expressed in this article are solely those of the authors and do not necessarily represent those of their affiliated organizations, or those of the publisher, the editors and the reviewers. Any product that may be evaluated in this article or claim that may be made by its manufacturer is not guaranteed or endorsed by the publisher.
Research integrity at Frontiers
Learn more about the work of our research integrity team to safeguard the quality of each article we publish.