- 1Laboratorio de Alimentos Funcionales y Bioprocesos, Departamento de Industrias Alimentarias y Biotecnología, Universidad Nacional de Frontera, Sullana, Peru
- 2Instituto de Investigación de Biotecnología Alimentaria y Biodiversidad, Universidad Nacional de Frontera, Sullana, Peru
- 3Laboratorio de Biotecnología Microbiana, Universidad Nacional de Frontera, Sullana, Peru
- 4Centro de Investigación de la Biodiversidad y Recursos Genéticos de Ancash, Facultad de Ciencias, Universidad Nacional Santiago Antúnez de Mayolo, Huaraz, Peru
Swiss-type artisanal cheese is highly appreciated sensorially, its flavor is often associated with the lactic acid bacteria involved in its production, which in many cases are indigenous. Three artisanal Swiss-type cheeses of greatest preference in the market of Huaraz (Ancash) were selected. The main LAB were isolated and identified and their safety properties and technological potential for their application in new cheeses were verified in vitro and evaluated by consumers. Eleven strains were confirmed as LAB by Gram-positive and catalase-negative biochemical tests; according to 16S rDNA, seven strains belonged to Lacticaseibacillus paracasei (KQ3, EQ1, CQ1, YQ1, LQ2, GQ2 and TQ1), three strains to Lentilactobacillus parabuchneri (BQ2, OQ2 and RQ3), and one to Lactiplantibacillus sp. (QQ3). In safety assays, LAB did not exhibit gelatinase or hemolytic activities. In addition, L. paracasei KQ3, GQ2 and L. parabuchneri BQ2 effectively inhibited pathogens such as S. aureus, E. coli and L. monocytogenes. Antibiotic susceptibility was variable among strains. L. paracasei CQ1, EQ1, KQ3, TQ1 and Lactiplantibacillus sp. QQ3 showed high milk acidification capacity (0.16-1.44%) and reduced pH from 6.6 to 3.5 after 72 h of incubation. L. paracasei CQ1, Lactiplantibacillus sp. QQ3 and L. paracasei KQ3 showed the highest casein degradation zones (20.8-11.5 mm). All strains showed lipolytic activity, with Lactiplantibacillus sp. QQ3, L. paracasei CQ1 and L. parabuchneri BQ2 standing out with halos of 30.8-36.3 mm. Lactiplantibacillus sp. QQ3 and L. paracasei TQ1 showed ability to produce diacetyl. The best strains were tested in cheese production where L. paracasei CQ1 showed the best sensory qualities. Finally, the native BAL strains showed a high potential for the production of natural, safe and sensorially acceptable dairy products.
1 Introduction
Lactic acid bacteria (LAB) involved in cheese making can determine its physicochemical and sensory qualities (Afshari et al., 2020). Based on their properties and their role in the fermentation process, LAB are classified into two groups: (i) starter strains, which accelerate fermentation due to their ability to produce high levels of lactic acid (De Melo Pereira et al., 2020; Zarzecka et al., 2020), and (ii) adjunct or non-starter lactic cultures, which are involved in improving the flavor and aroma during the cheese ripening process (Baptista et al., 2018; Levante et al., 2020a).
There are about 2,000 varieties of cheese in the world (Berno and Fusté-Forné, 2020). In addition, each cheese can contain up to 100,000 million bacteria per gram (Kilcawley, 2016). These microorganisms use the carbohydrates, proteins and lipids of the milk, producing various volatile compounds and contributing to the good taste of the final products (Kilcawley, 2016; Blaya et al., 2018). Among the diversity of cheese, the Swiss type is a hard or semi-hard cheese whose interior structure has holes called “eyes”(Hu, 2012; Fröhlich-Wyder et al., 2017) and includes some subtypes like Emmental, Comte, Maasdam, Beauford, Grevé, Gruyère français, Appenzeller, Tilsit, and others (Fröhlich-Wyder et al., 2013, 2017; Hayaloglu, 2016; Wolf et al., 2016). Although there is no international definition for the Swiss type cheese, it is identified by lactic fermentation in its first stage of elaboration, followed by propionic fermentation (Hu, 2012; Cheng, 2013). Its preparation includes the addition of Streptococcus thermophilus as a starter strain that reduces the pH and induces the growth of inoculated Lactobacillus helveticus (Baptista et al., 2018). The proteinases and peptidases produced by these lactobacilli play an important role in the breakdown of casein during cheese fermentation and ripening, contributing to the synergistic growth of the strains of S. thermophilus and L. helveticus (Hayaloglu, 2016) and allowing the development of the flavor (Cao et al., 2021). In addition, propionic fermentation is responsible for the holes inside the cheese through CO2 production and its mild flavor (Hu, 2012; Fox and Guinee, 2013).
Taxonomic identification of the cheese microbiota generally uses physiological, phenotypic and biochemical methods, but species levels are sometimes not reached (Hassanzadazar et al., 2017; Sharma et al., 2020). Molecular tools, such as 16 s rDNA sequencing, are applied to taxonomically identify the cheese-associated bacterial species (Hejazi et al., 2019; Hajigholizadeh et al., 2020). The unknown diversity of LAB in artisanal cheese and other fermented foods is attractive to the food industry because native bacterial species could be used to improve the nutritional, technological, and safety characteristics of different foods, also increasing their shelf life (Chen et al., 2008; Ashaolu, 2019). In this sense, the technological-food characterization and molecular taxonomy tools would be good alternatives to value these native bacteria strains from artisanal dairy products to improve traditional food technologies and develop new products.
The main food technological characteristics of LAB useful in dairy foods are (i) acidification activity to reduce the pH of milk in 6–24 h of incubation (Cogan et al., 1997; Beresford et al., 2001; Gonzalez-Gonzalez et al., 2019), (ii) proteolytic activity (Ardö et al., 2017; Meng et al., 2018) that hydrolyze casein into peptides and essential amino acids, improving flavor, texture, the production of volatile compounds and achieving better aroma (Biscola et al., 2016; Meng et al., 2018), (iii) lipolytic activity (El Soda et al., 1995; Silva et al., 2020) that biotransform free fatty acids to produce molecules, such as ethyl hexanoate and ethyl butanoate (De Oliveira et al., 2021) and producing diacetyl that confers it a strong butter aroma (Wang S. et al., 2019; Kim et al., 2021). Hence, resistance to antibiotics is another property sought for microorganisms that do not have horizontal gene transfer against intestinal pathogenic or opportunistic bacteria (Miranda et al., 2021) and can be used as starters in functional foods (Shi et al., 2018).
In Peru, the main production of Swiss-type artisanal cheese is located in the regions of Cajamarca, La Libertad and Ancash, these products have great sensory acceptance by consumers (Barreto et al., 2010; Gálvez Antinori, 2020). However, the lactic microorganisms associated with this characteristic are scarcely studied, and even less their technological-food characterization. Therefore, lactic acid bacteria were isolated and characterized from Swiss-type cheese sold in Huaraz markets (Ancash, Peru). Finding LAB strains with technological-food benefits due to the reduction of acidification time, aroma improvement through their proteolytic and lipolytic activities, and resistance to some antibiotics that can support the recovery of the gastrointestinal microbiota.
2 Materials and methods
2.1 Sample
Three pieces of Swiss type cheese (Q1, Q2 and Q3) were purchased from a well-known cheese factory in Huaraz (Ancash, Peru). The samples were stored in aseptic conditions and transported to the laboratory for LAB isolation within 24 h collection.
2.2 Isolation and selection of lactic acid bacteria
All samples (Q1, Q2 and Q3) were washed with sterile water. Under sterile conditions, the outer layer (approx. 10 mm) was removed, cut, and three parts were randomly chosen to be adjusted to 25 g. Each sample was placed in a sterile Erlemmeyer flask with 225 mL of NaCl (0.85% w/v), and homogenized for 20 min at 700 rpm with a magnetic stirrer. Bacterial isolation was carried out by serial dilution, then 100 uL of 104 dilution of each sample was inoculated on MRS (Man, Rogosa and Sharpe) agar plates supplemented with nystatin (50 μg/mL) to prevent fungal and yeast growth and incubated at 37°C for 48 h under anaerobic conditions. Three replicates were prepared for each sample. Different colonies (color, size, border, etc.) were selected and consecutive streaks were made until a pure culture was obtained. Catalase activity and Gram staining were performed using standard protocols. Strains of catalase negative and Gram (+) bacteria were selected, main characteristics of LAB; to later be cryopreserved according to Ulloa-Muñoz et al. (2020).
2.3 Molecular identification
The taxonomic identification of the selected strains was carried out according to Ulloa-Muñoz et al. (2020). For this, the DNA was extracted from fresh bacterial culture pellets (MRS broth) with the GeneJET Genomic DNA Kit (Thermo Scientific, United States) following the manufacturer’s guide. The 16S rDNA fragment was amplified by PCR using the 27F/1492R primer set. The quality of the amplicons was checked by agarose electrophoresis (1%) and sequenced at Macrogen Inc. (Seoul, Korea) with the 518F/800R primer set. The forward and reverse sequences were edited, assembled and compared with the standard sequences of the Genbank,1 which were downloaded to carry out the phylogenetic analysis that was executed in Mega V.7 using the Maximum Likelihood and Kimura-2-parameter methods with 1,000 bootstraps.
2.4 Inoculum preparation
The selected bacterial strains were grown anaerobically in MRS broth (5 mL) at 37°C for 24 h. The cultures were centrifuged at 2000 g x 5 min, the supernatant was discarded, the pellet was washed and resuspended in sterile NaCl (0.85%) and the optical density (λ = 600 nm) was adjusted to optical density at 600 nm (OD600): 0.8 equivalent to approximately 1 × 108 UFC/mL, according to Vera-Peña and Rodriguez-Rodriguez (2020) in a spectrophotometer UV–Vis (Thermo Scientific Genesys 150, United States).
2.5 Biosafety testing of LAB
2.5.1 Gelatinase activity
The essay was executed according to Sarasan et al. (2021) using MRS agar supplemented with gelatin (1%). Three sterile filter paper disks (5 mm) soaked with 5 μL of fresh bacterial inoculum (OD600 = 0.8) were placed. For the negative control, 5 μL of 0.8% saline solution was used. All samples were incubated at 37°C for 5 days. The gelatinase activity was revealed using a mercury (II) chloride solution (15 g of HgCl2, 20 mL of HClcc and 100 mL of distilled water) added around the disks. Transparent halos reveal the production of gelatinases (Zafiro et al., 2007).
2.5.2 Hemolytic activity
The experiment was carried out according to the methodology proposed by Srinivash et al. (2023) using sterile TSA agar supplemented with 5% human blood under aseptic conditions. 3 sterile filter paper disks (5 mm) soaked with 5 μL of fresh bacterial inoculum (OD600 = 0.8) were placed. For the negative control, 5 μL of 0.8% saline solution. All samples were incubated at 37°C for 2 days. Green or light halos indicate hemolytic activity.
2.5.3 Antibacterial activity
One hundred μL of fresh LAB (OD600 = 0.8) was inoculated by incorporation into 20 mL of MRS agar, poured into a Petri dish and incubated at 37°C for 7 days under anaerobic conditions to induce the production of antimicrobial metabolites according to Tamariz-Angeles et al. (2023) with a few modification. Staphylococcus aureus ATCC25923, Listeria monocytogenes ATCC7644, Escherichia coli ATCC10536 and Salmonella enterica sv Typhimurium ATCC14028 were used for the antibacterial test. The strains were cultured in TSB broth (5 mL) at 37°C for 24 h and adjusted to an optical density of OD620 = 0.08 (Tamariz-Angeles et al., 2018), then 100 μL of the broth was spread by extension on plates with Müller-Hinton Agar. Immediately, two BAL agar disks (5 mm) were cut and transfected onto the plates containing the pathogens. The plates were incubated at 37°C for 24 h. For the negative control, MRS agar disks (5 mm) without LAB were used. Clear halos indicated antibacterial activity and were measured in millimeters (mm).
2.5.4 Antibiotic sensitivity
The diffusion method was performed according to Singla et al. (2018) with some modifications. Standard disks of ampicillin (10 μg), erythromycin (15 μg), gentamicin (10 μg), penicillin (10 UI), rifampicin (30 μg), tetracycline (30 μg) and chloramphenicol (30 μg) were used. MRS agar plates were inoculated with 100 μL of fresh bacterial inoculum (DO600 = 0.8) and superficially spread. After 10 min, three disks per antibiotic were placed on the inoculated plates. They were incubated at 37°C for 24 h in anaerobic conditions. The diameters of the inhibition halos were measured with a digital vernier, and the results were expressed according to the Clinical and Laboratory Standards Institute (CLSI).
2.6 Technological characterization of LAB
2.6.1 Acidifying activity
The acidifying potential was determined according to Tsigkrimani et al. (2022) with some modifications. 100 mL of UHT (ultra-high temperature pasteurized) skimmed milk were inoculated with fresh cultures at a concentration of 1 × 108 UFC/mL, and incubated anaerobically at 37°C for 72 h. Three repetitions were performed for the strains and non-inoculated samples (negative control). The pH was measured with a potentiometer, the pH variation was obtained with formula (1), and the acidity was determined by volumetric titration with formula (2) according to Tyl and Sadler (2017). Both parameters were obtained at 0, 3, 6, 12, 24, 48 and 72 h of incubation under anaerobic conditions.
Where:
Vc: Volume of NaOH used in the titration.
N: Normality of the standard solution NaOH.
Ew: Lactic acid equivalent weight (90.08 g).
Sv: Sample volume.
1,000: Conversion factor (mg/g).
2.6.2 Proteolytic activity
The analysis was performed according to Petkova et al. (2021) with some modifications. The diffusion technique was used in wells (3 mm in diameter) in milk Agar (0.5% casein peptone, 0.25% yeast extract, 0.1% dextrose, 2.5% skimmed milk powder and 1.5% agar-agar) adjusted to pH 6.5 with HCl (3 N). Then, 50 μL of fresh bacterial inoculum was inoculated (OD600 = 0.8) in each well, and the plates were incubated at 37°C for 48 h under anaerobic conditions. Sterile MRS broth was used as a negative control and three replicates were prepared. The clear halo around a hole indicated positive proteolytic activity.
2.6.3 Lipolytic activity
The lipolytic activity was performed according to Dogan and Taskin (2021) and Carrim et al. (2006) with some modifications. The medium for the lipolytic screening contained: peptone (1.0%), CaCl2 (0.01%) and agar-agar (1.5%). The pH was adjusted for 6.5 and supplemented with sterile Tween 20 (1.0%) under sterile conditions. Four sterile filter paper disks (5 mm) soaked with 5 μL of fresh bacterial inoculum were placed (OD600 = 0.8) in the lipolytic medium and incubated anaerobically at 37°C for 24 h. The opaque area around the disks was considered a positive result.
2.6.4 Diacetyl production
This parameter was carried out according to Margalho et al. (2020) with some modifications. A single colony was selected from a fresh culture plate, transferred to MRS broth (5 mL) and incubated anaerobically at 37°C for 24 h. Then, the culture was centrifuged at 4500 g x 15 min. Its precipitate was recovered and 0.01 g was resuspended in 10 mL of peptone solution (7.6%). This suspension (5 mL) was transferred to tubes with UHT skimmed milk (5 mL) and incubated anaerobically at 37°C for 24 h. One milliliter of culture was mixed with 250 μL of alpha-naphthol in 96° ethanol (1% w/v) and 250 μL of KOH (16% w/v), and incubated at 37°C for 10 min. An uninoculated sample was added as a control. Diacetyl production was considered positive by the presence of a red colored ring in the mixture. Its intensity was qualitatively quantified.
2.7 Application of selected LAB in cheese
2.7.1 Starter culture preparation
Lacticaseibacillus paracasei (CQ1 and KQ3) and Lactiplantibacillus sp. (QQ3) strains were selected due to the good potential they showed in previous tests. The selected strains were reactivated following the procedure previously described in section “2.4” with two washes to remove any remains of the broth. The strains were inoculated in 250 mL of UHT milk and incubated at 37°C for 24 h.
2.7.2 Production of traditional cheese from the Ancash region
The preparation of traditional cheese was carried out as shown in Figure 1. A volume of 20 L of raw milk with 6.6 pH and a density of 1.026 was acquired. The density was readjusted to a density of 1.030 [range recommended by NTP 202.001:2016 (NTP, 2016)] with powdered milk, pasteurized at 65°C for 30 min, CaCl2 (0.025%) was added during pasteurization. It was then cooled to 38°C and divided into 5 L samples. The samples were inoculated with L. paracasei CQ1, L. paracasei KQ3 and Lactiplantibacillus sp. QQ3. A control without inoculum was produced. The samples were homogenized with a sterile steel paddle for 15 min. Rennet (enzyme) was added Rhizomucor miehei, CHR HANSEN, Denmark to each sample according to the manufacturer’s instructions, always maintaining the temperature around 38°C, letting it rest for 45 min until the formation of curd which was later cut using a sterile stainless-steel knife, ensuring the size of 1 cm cubes. Later, it was drained using sterile meshes, the mass obtained was salted with 0.5% NaCl. This mass was placed in 500 g PVC molds to be molded and pressed for 4 h. Finally, the cheese was removed from the mold and stored at 8°C for 8 days.
2.7.3 Sensory analysis
Sensory analysis was carried out according to Mauricio-Sandoval et al. (2023). Thirty-five panelists participated, regular cheese consumers, whose ages ranged from 18 to 74 years old. Each participant tasted four cheese samples of 10 g each, randomly coded. The sensorial attributes evaluated were flavor, odor, texture and general appearance, with scores from 1 to 5, where: (1) I dislike it a lot, (2) It displeases me, (3) It does not please me or it displeases me, (4) It does not please me it pleases me (5) It pleases me very much.
2.7.4 Physicochemical characteristics of traditional cheeses
Analyzes of moisture, ash, fat, protein, carbohydrates and pH of the cheeses were performed according to the procedures described in Barrios Renteria et al. (2022), according to the following methods:
Determination of moisture by difference in weight according to IDF-IDF 151:2005.
Determination of ash content according to A.O.A.C. 945.46:2002 method.
Determination of fat content according to IDF-IDF method 116A:1996.
Determination of protein according to the method of NTP 202.119:2014.
Determination of carbohydrates by the difference method of Collazos et al. (1993).
2.8 Statistical analysis
To determine the statistical significance of the values, the mean ± standard deviation (SD), ANOVA and Tukey’s test were used (α =0.05), using the Statistical Package for the Social Sciences (SPSS) v.23.
3 Results and discussion
3.1 Isolation and phenotypic characterization of Swiss-type cheese bacteria
Fourteen strains were isolated from three pieces of artisanal cheese (Q1, Q2 and Q3) obtained in a traditional market in Huaraz (Peru). All isolates were Gram positive, while only 11 were catalase negative (Table 1). These strains had a bacillary shape consistent with Falsen et al. (1999) and Pundir et al. (2013), who described lactic acid bacteria as facultative anaerobic, Gram-positive, without endospore, catalase-negative and producing lactic acid as the main product.
3.2 Molecular taxonomic identification
Before the 20th century, the taxonomy of lactic acid bacteria was based on phenotypic characteristics (Klein et al., 1998), but currently the analysis of 16S rDNA has recorded a greater diversity of this bacterial group (Tringe and Hugenholtz, 2008). At present, the genus Lactobacillus has been reclassified by Zheng et al. (2020) in 23 new genera, such as Lacticaseibacillus, Lentilactobacillus and Lactiplantibacillus, using a polyphasic method (phenotypic and genotypic).
64% of the bacterial strains isolated from the Swiss-type cheese analyzed belonged to Lacticaseibacillus paracasei (GQ2, EQ1, TQ1, YQ1, LQ2, CQ1, and KQ3), 27% to Lentilactobacillus parabuchneri (BQ2, OQ2, and RQ3) and 9% a Lactiplantibacillus sp. (QQ3) (Figure 2).
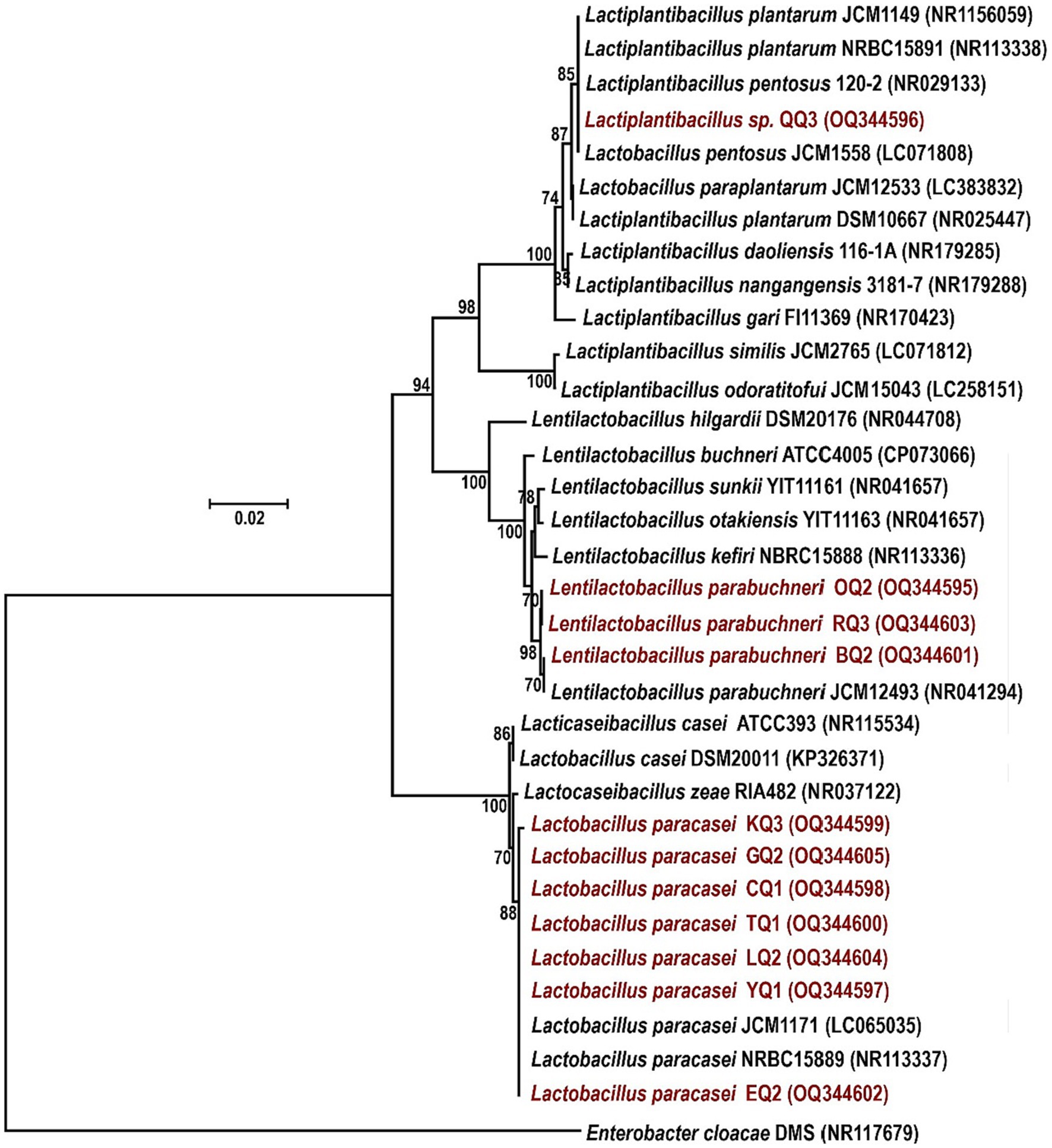
Figure 2. Phylogenetic analysis of lactic acid bacteria (LAB) from Swiss-type cheese from Ancash (Peru) using complete 16S rDNA. All phylogenetic trees were performed using the Likelihood and Kimura-2-parameter methods with 1,000 bootstraps. The letters in red are the isolated strains, in black the GenBank type strains, and in parentheses the GenBank access number.
Our findings were similar to those obtained by Meral Aktaş and Erdoğan (2022) who identified by 16S rDNA Lacticaseibacillus paracasei spp. tolerans L41 isolated from Turkish Beyaz cheese (white), classified as ripened cheese. Luiz et al. (2017) found Lacticaseibacillus, Lentilactobacillus and Lactiplantibacillus in Brazilian artisanal cheese, in addition to other bacterial strains belonging to the genera Lactobacillus, Enterococcus, Lactococcus and Pediococcus. However, Mohammed and Çon (2021) in Turkish white cheese isolated bacteria of the genera Enterococcus, Lactiplantibacillus and Loigolactobacillus, but no Lacticaseibacillus and Lentilactobacillus were found. The isolation of the diversity of bacteria is related to the medium used (Domingos-Lopes et al., 2017). Therefore, some factors that determine this diversity could be the variety of cheese, the manufacturing process, coagulation, the types of whey drainage (Afshari et al., 2020), milk pasteurization, addition of lactic ferments (Zoumpopoulou et al., 2020), place of provenance of the cheese sample, species and breed of mammal from which the milk comes (Tulini et al., 2016; Islam et al., 2021). In this investigation, the high percentage of species associated with the genus Lactobacillus may be due to the selective property of the MRS medium.
Lacticaseibacillus strains belong to the homofermentative group, produce lactic acid from glucose and grow between 10 and 45°C. Some species are used as starter cultures or probiotics (Zheng et al., 2020). Other species have no effect on initial acidification, but play an important role in ripening (Levante et al., 2020b). Barzideh et al. (2022) found an abundance of 15–90% of the species in cheeses aged 13–32 months. In addition, this genus had been identified and used in different types of cheeses such as Parmigiano Reggiano (Levante et al., 2020a), Pecorino di Farindola (Aprea et al., 2021), Turkish Beyaz (Meral Aktaş and Erdoğan, 2022), traditional Iranian (Afshari et al., 2022), Cheddar (Leeuwendaal et al., 2022), DOP Greek Galotyri (Samelis and Kakouri, 2022). Similarly, the genus Lactiplantibacillus belongs to the group of homofermentatives. They can metabolize different phenolic acids by various metabolic pathways, also characterized as starter cultures or probiotics and have been isolated from fermented products and vertebrate intestinal tracts (Zheng et al., 2020). This genus has been identified and used in Greek Feta cheese (Papadopoulou et al., 2018), Cheddar (Wang J. et al., 2019), Turkish white cheese (Mohammed and Çon, 2021), Brazilian artisanal cheese (Luiz et al., 2017). Unlike the genera Lacticaseibacillus and Lactiplantibacillus, bacteria of the genus Lentilactobacillus are heterofermentative and slow growing at temperatures between 15 and 45°C (Zheng et al., 2020), this genus has been cited as a microbiota of Greek Galotyri DOP cheese (Samelis and Kakouri, 2022), DOP Azeitão cheese (Cardinali et al., 2021), “Serrano” cheese (Margalho et al., 2021). Lentilactobacillus parabuchneri FAM 21835, a non-histamine strain, isolated from Swiss Tilsit type cheese, influences ripening and is responsible for the formation of the cheese eye, flavor, texture, and the production of ornithine, glutamic acid, γ-aminobutyric acid and histidine (Fröhlich-Wyder et al., 2015), and could present an inhibitory effect against mycelial fungi (Liang et al., 2022).
The identification of bacterial strains of the genera Lacticaseibacillus, Lactiplantibacillus and Lentilactobacillus of Swiss-type cheeses from Huaraz allowed to know their microbial diversity and the role of these strains in this type of cheese, in addition, according to the technological characteristics, each bacterial strain could be proposed as a candidate to improve or produce new products. Mainly, in the elaboration of the Swiss type cheese, it is used: pasteurized cow’s milk, lactic culture (R-707 = Lactococcus lactis subsp. lactis biovar diacetylactis), rennet, calcium chloride, preservative and salt (Bekele et al., 2019; Guevara Cruzado and Reyes Flores, 2019), but during its ripening or semi-ripening, a natural secondary microflora grows that is responsible for its sensory acceptability (Blaya et al., 2018). In addition, species of Lentilactobacillus were detected in raw milk (Carafa et al., 2019). The Ancash Swiss-type artisanal cheese is mainly made with pasteurized milk without the addition of microorganisms, but three kinds of LAB were found. According to Pundir et al. (2013), its presence in this type of products may be due to contamination after milk pasteurization because there are microorganisms in the air, the equipment or the ingredients involved in its preparation; also some LAB may be resistant to pasteurization or transfer during product storage (Stefanovic et al., 2018). Furthermore, Lactiplantibacillus species are used as starter cultures in various fermented foods to develop organoleptic and probiotic characteristics (Metrouh et al., 2022).
3.3 Biosafety testing of LAB
3.3.1 Gelatinase and hemolytic activity
The selected LAB did not show gelatinase activity, likewise, it was negative for the α-hemolysis test and β-hemolysis. Cizeikiene and Jagelaviciute (2021) reported that the genders Lactobacillus and Bifidobacterium did not present gelatinase activity. Srinivash et al. (2023) isolated four LAB (L. delbruecki, L. hircilactis, L. jhonsonii and L. lechmannii) which also did not show hemolytic activity, as reported for L. plantarum UBLP40 (Ahire et al., 2021). Both assays with negative results are requirements for the selection of safe probiotic strains (Ahire et al., 2021; Srinivash et al., 2023).
3.4 Antibacterial activity
Overuse of antibiotics makes pathogenic bacteria more resistant, causing the search for new LAB strains that can inhibit these pathogens (Miao et al., 2016) and be used in the production of new foods, especially cheese (Abosereh et al., 2016) and extend their shelf life (Silva et al., 2023).
The selected LAB showed inhibitory action on pathogenic bacteria (Figure 3). The strains that presented the best results were L. paracasei (KQ3) which inhibited S. aureus (3 mm) and E. coli (4.5 mm), L. parabuchneri (BQ2) inhibited L. monocytogenes (8 mm) (Supplementary Figure S1) and S. enterica sv Typhimurium (5.5 mm) and L. paracasei (GQ2) inhibited E. coli (4.5 mm). The inhibitory action of species of the Lactobacillus genus was reported by Tambekar and Bhutada (2010) and Shehata et al. (2022).
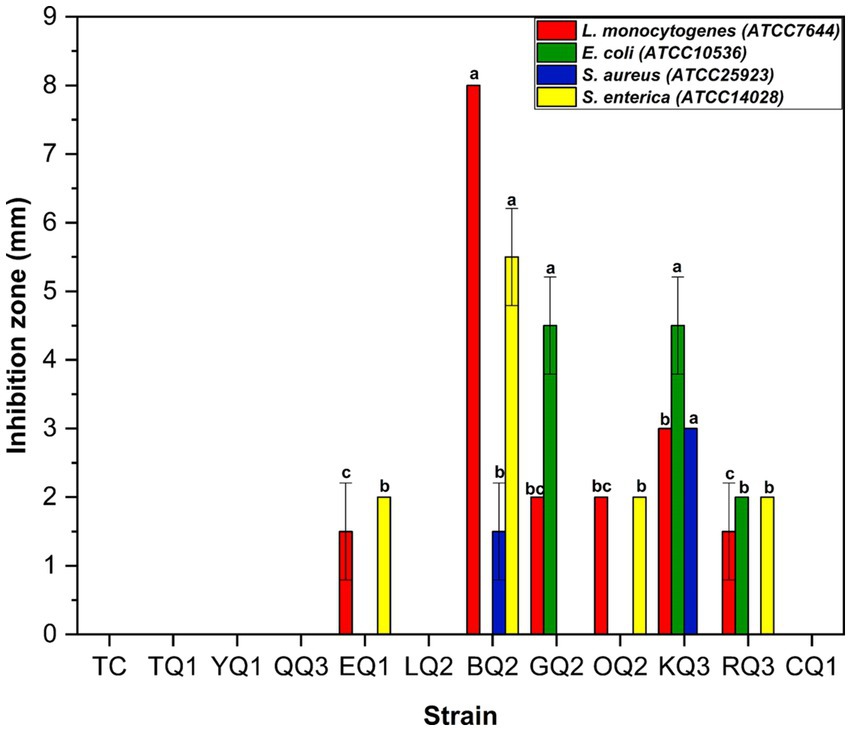
Figure 3. Inhibitory action of LAB isolated from Swiss-type artisanal cheese on pathogenic bacteria. Mean of the two replicates ± SD. The letters indicate different statistical groups according to the Tukey’s test (p < 0.05). Strains without bars or letters did not present inhibitory activity.
The antibacterial activity of LAB is possibly related to the production of organic acids, mainly lactic acid (Zhang et al., 2020), and others such as acetic acid, citric and tartaric acids (Zalán et al., 2010) or bacteriocins (Zhang et al., 2020) which inhibit pathogenic microbes and generates minimal resistance (Miao et al., 2016). It can be related to the production of ethanol, diacetyl or substances similar to antibiotics (Bintsis, 2017). The metabolites synthesized by the LAB can prevent the colonization of pathogens in the body by competing for adhesive and nutrient receptors (Barzegar et al., 2021), using preventive and therapeutic purposes to control enteric infections (Tambekar and Bhutada, 2010).
3.5 Antibiotic sensitivity
Genes of resistance to transmissible or transferable antibiotics of the Lactobacillus genera are of increasing concern (Lucumi-Banguero et al., 2021) for human and animal health (Reuben et al., 2020), because some probiotic strains could transfer antibiotic resistance genes to pathogenic bacteria causing problems for their consumers (Mangia et al., 2019; Wang K. et al., 2019).
The antibiotic susceptibility results performed by the diffusion method are found in Table 2. It was observed that all LAB strains (100%) were sensitive to rifampicin (30 μg), erythromycin (15 μg), penicillin (10 IU) and gentamicin (10 μg); 64% (NQ1, EQ1, LQ2, BQ2, GQ2, KQ3 and CQ1) were sensitive to tetracycline (30 μg), 18% (QQ3 and LQ2) to ampicillin (10 μg) and 9% (QQ3) to chloramphenicol (30 μg) and vancomycin (30 μg) (Supplementary Figure S2). Similar to this study, L. paracasei FS103 isolated from sheep cheese showed sensitivity to penicillin, erythromycin, and tetracycline, among others (Mangia et al., 2019). Meral Aktaş and Erdoğan (2022) and Zoumpopoulou et al. (2017) found that the strains L. paracasei subsp. tolerans L41, ACA-DC 177, and ACA-DC 196 isolated from Turkish Beyaz (white) and Greek Kasseri cheese that showed sensitivity to chloramphenicol but resistance to gentamicin, these results were similar to other strains reported by Metrouh et al. (2022). L. paracasei subsp. tolerans isolated from traditional Ethiopian fermented food presented sensitivity to tetracycline, ampicillin and erythromycin (Mulaw et al., 2019).
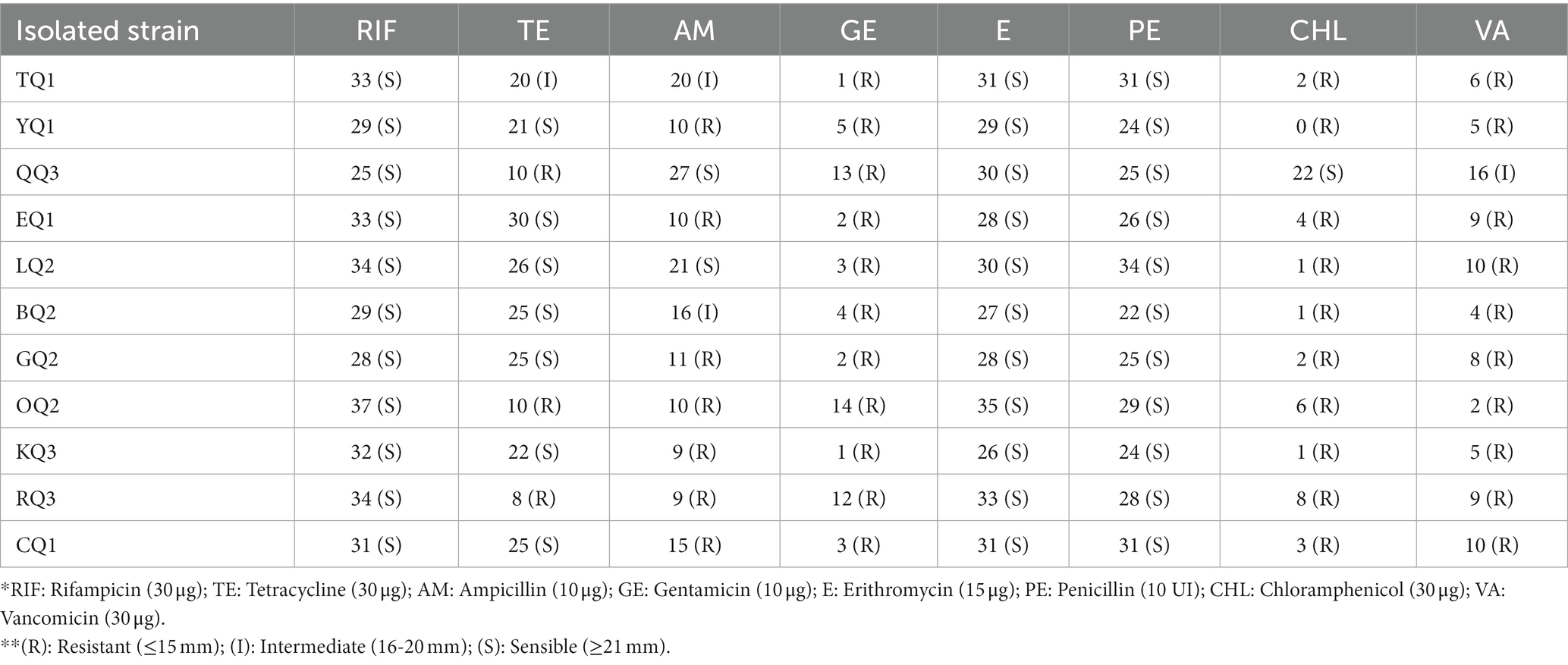
Table 2. Antibiotic susceptibility test by the diffusion method of LAB isolated from Swiss-type artisanal cheese.
Regarding antibiotic resistance of Lentilactobacillus sp., Nawaz et al. (2011) isolated 12 L. parabuchneri of fermented vegetables, and seven strains were resistant to gentamicin and two strains to chloramphenicol. This demonstrated that not all LAB of the same species have resistance to the same antibiotics and vary by antibiotic concentration (Barzegar et al., 2021; Randazzo et al., 2021) or by intrinsic genetics of Lactobacillus strains that are resistant to aminoglycosides or glycopeptides such as gentamicin and vancomycin (Akpınar Kankaya and Tuncer, 2020; Jomehzadeh et al., 2020). Therefore, it is suggested that LAB resistance to antibiotics may be convenient to be studied whether this characteristic is associated with mobile genetic elements, such as plasmids or transposons, to determine the possibility of horizontal transfer to pathogenic bacteria (Kang et al., 2020; Parlindungan et al., 2021) or by conjugation of LAB isolated from raw milk, artisanal and non-artisanal cheese resistant to penicillin, erythromycin, tetracycline and rifampicin (Russo et al., 2018; Margalho et al., 2020; Výrostková et al., 2020). There is a particular case of resistance to chloramphenicol associated with the chloramphenicol acetyltransferase (cat) gene located in Lactobacillus plasmids that is not common in bacteria of this genus (Leeuwendaal et al., 2021; Sharma et al., 2021). Furthermore, the cat gene is not transferred between food and non-food bacteria (Abriouel et al., 2015).
Some authors consider that the antibiotic resistance of LAB could be an opportunity for the food industry because these strains can help to reposit the intestinal microbiota during the administration of antibiotics to control diarrheal problems (Wang K. et al., 2019; Huang et al., 2021; Islam et al., 2021), but it is a desirable that the gene involved cannot be transmitted to pathogenic bacteria (Kamarinou et al., 2022). In addition, antibiotic resistance in LAB could be due to the indiscriminate use of antibiotics in animals, mainly in this context, this trait in LAB isolated from dairy products marketed in rural areas, where antimicrobial agents are rarely used, could be associated with intrinsic resistance to non-transferable genes (Kakelar et al., 2019), and strains belonging to the Lactobacillus group could have natural antibiotic resistance genes associated with their chromosome (Kamarinou et al., 2022). Therefore, the resistance to antibiotics shown by the bacteria isolated in this research could be the focus of future studies.
3.6 Acidifying activity of LAB
The lactic acid produced by LAB is necessary for the preparation of cheese and fermented foods, it increases acidity and lowers pH, improving flavor and rheological properties and eliminates pathogenic or spoilage bacteria (Campagnollo et al., 2018; Barzegar et al., 2021). It could also enhance biological activity through the production of bacteriocins and exopolysaccharides (Belleggia et al., 2022).
Figure 4 shows the pH of the inoculated milk at different times, where the initial pH of the milk was 6.6. The pH reduction was weak after 6 h in all LAB strains tested, however, L. paracasei KQ3 strain reduced the milk pH to 5.2 in 12 h, and this strain and L. paracasei CQ1 showed higher pH reduction near 4.0 (Supplementary Table S1). Moreover, the △pH in all the samples inoculated with LAB isolated from Swiss type cheese was 0.43–2.47 and 1.90–3.10 at 24 and 72 h, respectively, in which L. paracasei (KQ3, CQ1, TQ1, and EQ1) and Lactiplantibacillus sp. (QQ3) can be considered as potential acidifying strains. A similar result was found in L. paracasei subsp. tolerans HUM15, isolated from camel milk, which could decrease the pH to 4.42 after 72 h of incubation (Hawaz et al., 2016). On the other hand, Pisano et al. (2022) reported that L. plantarum 4/16898 from sheep’s milk cheese presented 2.22 △pH at 24 h, value less than Lactiplantibacillus sp. QQ3.
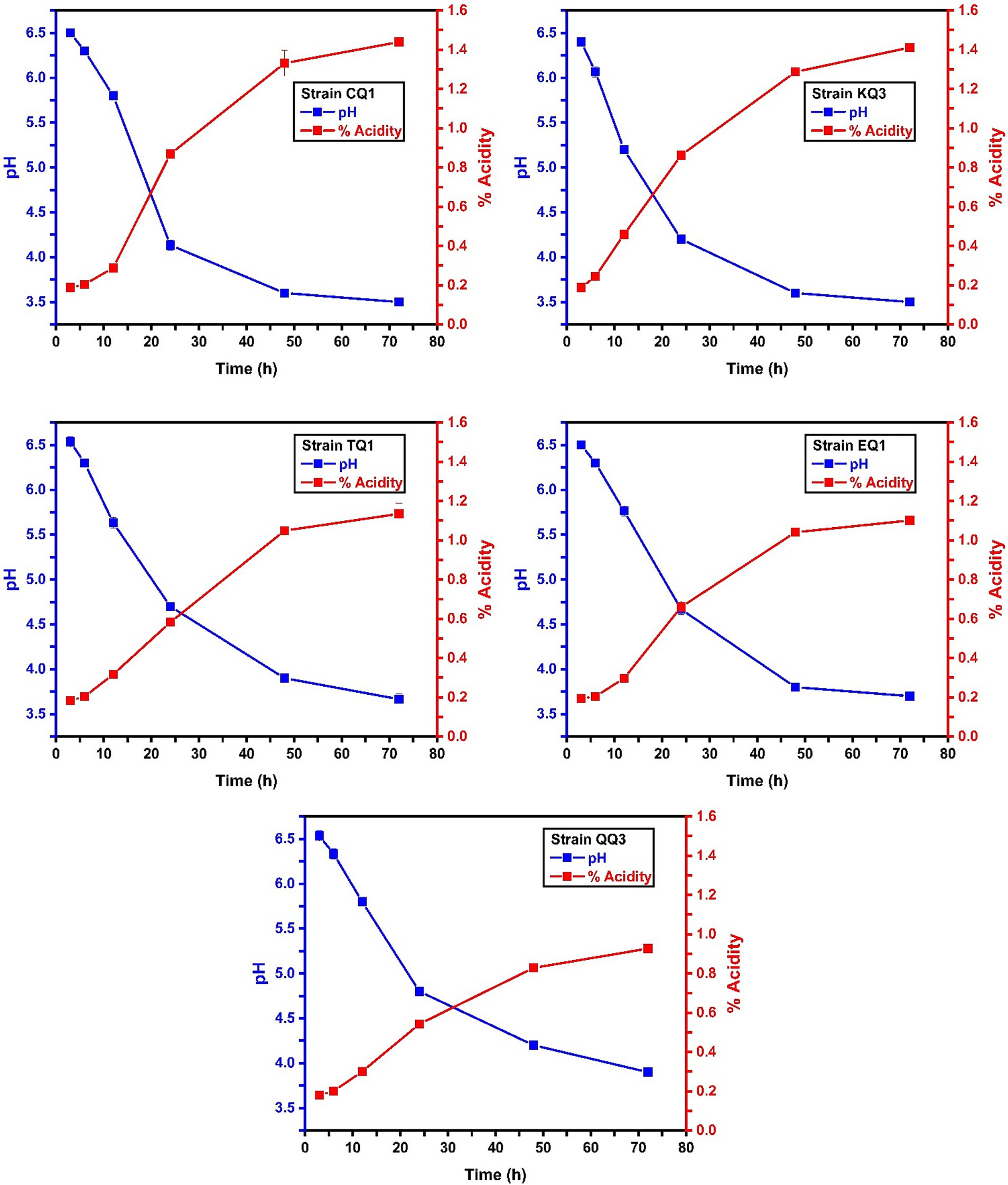
Figure 4. pH and Acidity versus time. The figure shows the behavior of the pH and acidity of the strains (CQ1, EQ1, GQ2, KQ3, QQ3 and TQ1) inoculated in milk, versus their incubation time (3, 6, 12, 24, 48 and 72 h).
According to the classification of Albayrak and Duran (2021), none of the strains in this research could be considered good acidifiers because they defined that good acidifying bacteria reduce the pH of milk from 6.6 to 5.3 or increase the acidity ≥0.25 g of lactic acid per 100 mL after 6 h of incubation. Nevertheless, Mohammed and Çon (2021) classified bacteria as strong acidifiers when ΔpH 24 h ≥ 1.61 or ΔpH 72 h ≥ 1.63 and weak acidifiers with ΔpH 24 h ≤ 1.11. L. paracasei TQ1, EQ1, CQ1 and KQ3, and Lactiplantibacillus sp. QQ3 exhibited △pH 24 h between 1.8–2.47. These bacterial strains are strong acidifiers in contrast to L. parabuchneri BQ2, OQ2, RQ3, and L. paracasei YQ1, LQ2, GQ2 which showed a weak acidification capacity following the classification of Mohammed and Çon (2021).
The pH is inversely proportional to acidity (Fauziah et al., 2020). Figure 4 shows that the initial acidity percentage of the milk was 0.16 and that L. paracasei TQ1, EQ1, CQ, KQ3 and Lactiplantibacillus sp. QQ3 increased this acidity to 0.38–0.71 (24 h) and 0.77–1.28 (72 h), considered high acidification values. Meral Aktaş and Erdoğan (2022) isolated L. paracasei subsp. tolerans L41 of Turkish Beyaz (white) cheese, which barely increased the acidity of the milk close to 0.20 with pH > 6.0 after 24 h. Furthermore, a strain of L. parabuchneri involved in the production of PDO Italian Bitto cheese showed a slow acidification process (Morandi et al., 2011) similar to L. parabuchneri BQ2, OQ2, RQ3 isolated in this investigation (Supplementary Table S1).
Although it is true that bacteria of the genus Lactobacillus metabolize lactose slowly (Dagdemir and Ozdemir, 2008), it was observed that after 24 h of incubation, some strains increased the acidity of the milk equal to or more than the acidifying bacteria (Ribeiro et al., 2021). According to González-González et al. (2022), LAB should reduce the pH < 4.7 after 24 h of incubation, but Monteagudo-Mera et al. (2011) mention that the artisanal production of cheese only requires bacterial strains with the capacity to reduce the pH of milk to 5.10 after 24 h of incubation. In agreement, L. paracasei TQ1, EQ1, CQ1, KQ3 and Lactiplantibacillus sp. QQ3 isolated in this research, show an interesting potential to be used as acidifying bacteria in dairy products.
3.7 Proteolytic activity
The proteolytic activity of LAB improves the sensory characteristics of fermented foods and plays an important role in the growth of other fermented bacteria (Islam et al., 2021). During the proteolytic event, high weight and complex proteins (casein micelles) are degraded to low molecular weight proteins and/or amino acids (Bezerra et al., 2016; Ardö et al., 2017), aromatic compounds such as aldehydes, alcohols, ketones, esters, etc. are also produced and released (De Souza and Silva Dias, 2017; Meng et al., 2018). In this investigation, all isolates showed proteolytic activity (Figure 5) in which L. paracasei CQ1, Lactiplantibacillus sp. QQ3, and L. paracasei KQ3 exhibited better degradation halos of 20.8, 16.0 and 11.5 mm, respectively (Table 3; Supplementary Figure S3).
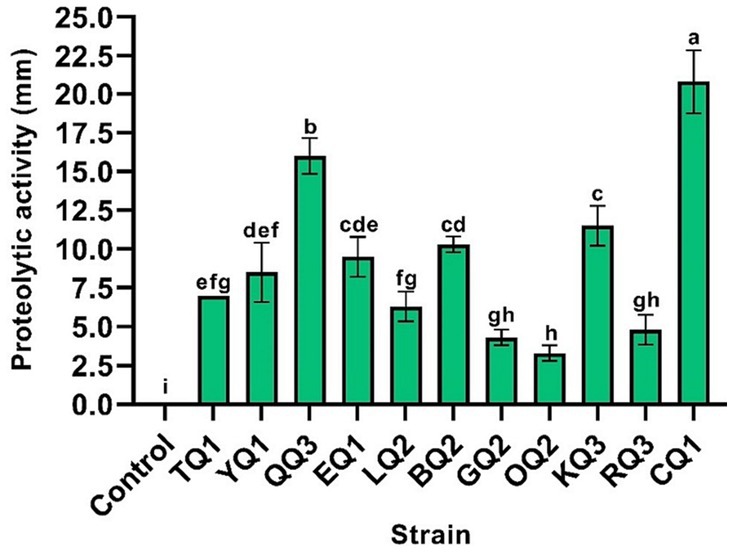
Figure 5. Proteolytic activity of LAB isolated from Ancash Swiss cheese. It is expressed as halos of milk protein degradation after incubation on agar plates. Mean of four replicates ± SD. The letters indicate different statistical groups according to the Tukey’s Test (p < 0.05).
Likewise, L. paracasei subsp. tolerans HUM15 isolated from camel milk showed proteolytic activity with a casein degradation halo of 15 mm (Hawaz et al., 2016), on the other hand, L. paracasei subsp. tolerans L41 isolated from Turkish Beyaz (white) cheese did not show proteolytic activity (Meral Aktaş and Erdoğan, 2022). Strains of Lentilactobacillus, L. kefiri and L. buchneri, isolated from cheese, showed a high proteolytic activity (Margalho et al., 2021; Akbulut et al., 2022). As for the species Lactiplantibacillus SJ14 isolated from Argelia cheese showed a 13 mm proteolytic halo on milk agar plates (Metrouh et al., 2022).
In general, the proteolytic activity of LABs is considered a desirable feature in the manufacture of fermented food (Panebianco et al., 2021), but it also contributes to the health sector, because they are used to prevent allergies in children (0–3 years) with poor digestion of the proteins of some dairy products (Meng et al., 2018; Islam et al., 2021). Thus, the LAB isolated in this research could be used in the manufacture of dairy products with better digestibility due to their proteolytic activity.
3.8 Lipolytic activity
Lipolytic enzymes (lipases) isolated from microorganisms are widely required in the food industry (Chandra et al., 2020) due to their stability, low production cost and easy access compared to plant or animal lipases (Tanasupawat et al., 2015). This enzyme is mainly used in dairy, meat, pickle, and bakery products to improve flavor (Salgado et al., 2022). In this investigation, all isolated LAB showed lipolytic activity, while Lactiplantibacillus sp. QQ3, Lacticaseibacillus paracasei CQ1, and LQ2, and Lentilactobacillus parabuchneri BQ2 showed higher degradative halos (30.8–36.3 mm) (Table 3; Supplementary Figure S3), in contrast to the other strains showed degradation halos below 27 mm (Figure 6).
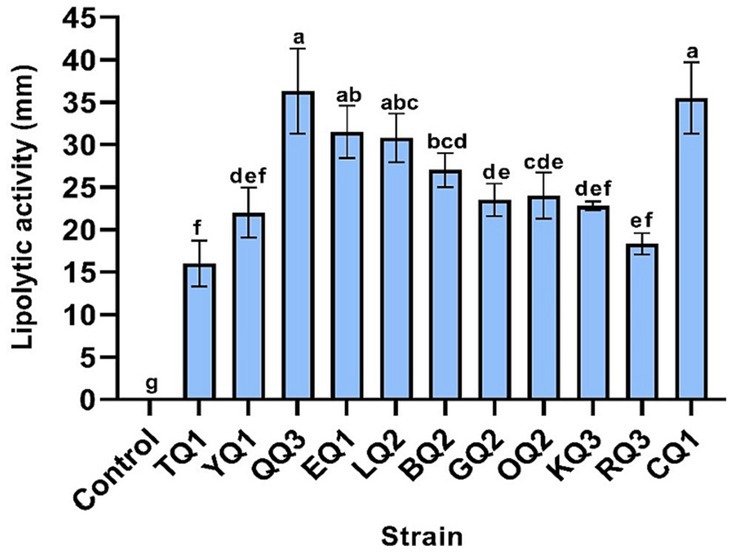
Figure 6. Lipolytic activity of LAB isolated from Ancash Swiss cheese. It is expressed as the diameter of the degradation halos after incubation on agar plates supplemented with Tween-20. Mean of four replicates ± SD. The letters indicate different statistical groups according to the Tukey test (p < 0.05).
Metrouh et al. (2022) reported a strain of Lactiplantibacillus plantarum SJ14 isolated from Argelia cheese and found lipolytic activity in culture media containing olive oil (3%) and Tween 80 (5%) with degradation halos of 14.5 mm and 10 mm, respectively. These values were lower than the halo of degradation of Lactiplantibacillus sp. QQ3 (36.3 mm) isolated from Ancash Swiss type cheese. In addition, some studies reported that strains of Lacticaseibacillus casei and L. paracasei isolates from Iranian raw cow’s milk cheese, artisan cheese and goat cheese did not show lipolytic activity (Câmara et al., 2019; Barzegar et al., 2021). The absence of lipolysis could be due to the culture medium used (Tributyrin agar). Carrazco-Palafox et al. (2018) mentioned that the commercial medium sometimes lacks calcium or magnesium ions that allow to intensify and stabilize giving greater visibility to the halo of degradation. However, Araújo-Rodrigues et al. (2021) found that L. paracasei isolated from PDO Serpa cheese showed a high lipolytic activity assayed on tributyrin agar, so this activity may be related to the genetic component of this species.
Lentilactobacillus strains isolated in the present study have lipolytic activity while Lentilactobacillus otakiensis and L. buchneri strains isolated from pig feces and artisanal Pico cheese did not show such activity (Câmara et al., 2019; Marchwińska and Gwiazdowska, 2022). These results may be related to the weak production of lipase in some Lactobacillus bacteria (Ramakrishnan et al., 2019) including other genera later reclassified by Zheng et al. (2020). Then, all LAB isolated in the present investigation could be used effectively in the food industries.
3.9 Diacetyl production
Diacetyl is a volatile aromatic compound (Kim et al., 2021) highly required in dairy food and it is found mainly in cheese, yogurt, butter, buttermilk, etc (Chen et al., 2017; Domingos-Lopes et al., 2017). Lactobacillus species are considered main producers of diacetyl, but currently, strains belonging to the genera Streptococcus, Leuconostoc, Streptococcus, Pediococcus and Oenococcus also show this capacity (Clark and Winter, 2015; Wang S. et al., 2019). Its synthesis is carried out by citrate lyase and α-acetolactate synthase that degrade milk citrate to α-acetolactate (an unstable molecule), which is decarboxylated as diacetyl at low pH (Hugenholtz et al., 2000; De Souza and Silva Dias, 2017).
The diacetyl produced by LAB improves the sensory acceptability of fermented products such as ripened cheese (Khattab et al., 2019), providing end products with buttery, creamy, sweet and milky flavors (Tian et al., 2020). In this study, it was found that Lactiplantibacillus sp. QQ3 showed better diacetyl production, and the fermented milk reacted immediately to the specific test, followed by L. paracasei TQ1, which showed a slow positive reaction after 10 min (Table 3). Metrouh et al. (2022) found out that L. plantarum SJ14, isolated from Algerian cheese, moderately produced diacetyl. Eight strains of L. paracasei out of 16 strains isolated from goat milk produced diacetyl (Meng et al., 2018), five strains of L. paracasei (PN 7, PN 59, PN 76, PN 105 and PN 145) of six strains isolated from PDO Provola dei Nebrodi Aquiartisanal cheese also showed the ability to produce this compound (Randazzo et al., 2021). Regardless of whether LAB are taxonomically classified by similar characteristics, strains of the same species may have different behavior or metabolic capacity to produce various types of volatile aromatic compounds or other metabolites (Agostini et al., 2018), these characteristics depend on the intrinsic phylogenetic properties of each strain (Carvalho et al., 2014; Ouattara et al., 2017).
Another application of diacetyl in food is its antimicrobial activity against pathogens (Meng et al., 2018; Wang S. et al., 2019) because it crosses the bacterial membrane and prevents the assimilation of arginine that blocks the enzymes involved in microbial development and growth (de Souza and Silva Dias, 2017). Thus, L. paracasei TQ1 and Lactiplantibacillus sp. QQ3 isolated from Ancash cheese could be used in fermented products to improve aroma, and perhaps extend shelf life if the diacetyl produced inhibits the growth of pathogenic or spoilage bacteria.
3.10 Sensory analysis
The sensory analysis was carried out on the first and eighth days of ripening (Figure 7). On the first day of ripening, both the cheeses inoculated with the LAB strains and the control without inoculum did not show statistically significant differences (p < 0.05; Supplementary Table S2). However, after 8 days of ripening, the cheese inoculated with LAB presented better attributes of flavor, aroma, texture and general appearance compared to the first day and the uninoculated control. This demonstrates the fermentative capacity in the food matrix of the LAB (Figure 7) improved the quality of the cheese. Awad et al. (2007) reported that the low acceptability of cheese is due to short fermentation periods and the lack of bacterial consortia.
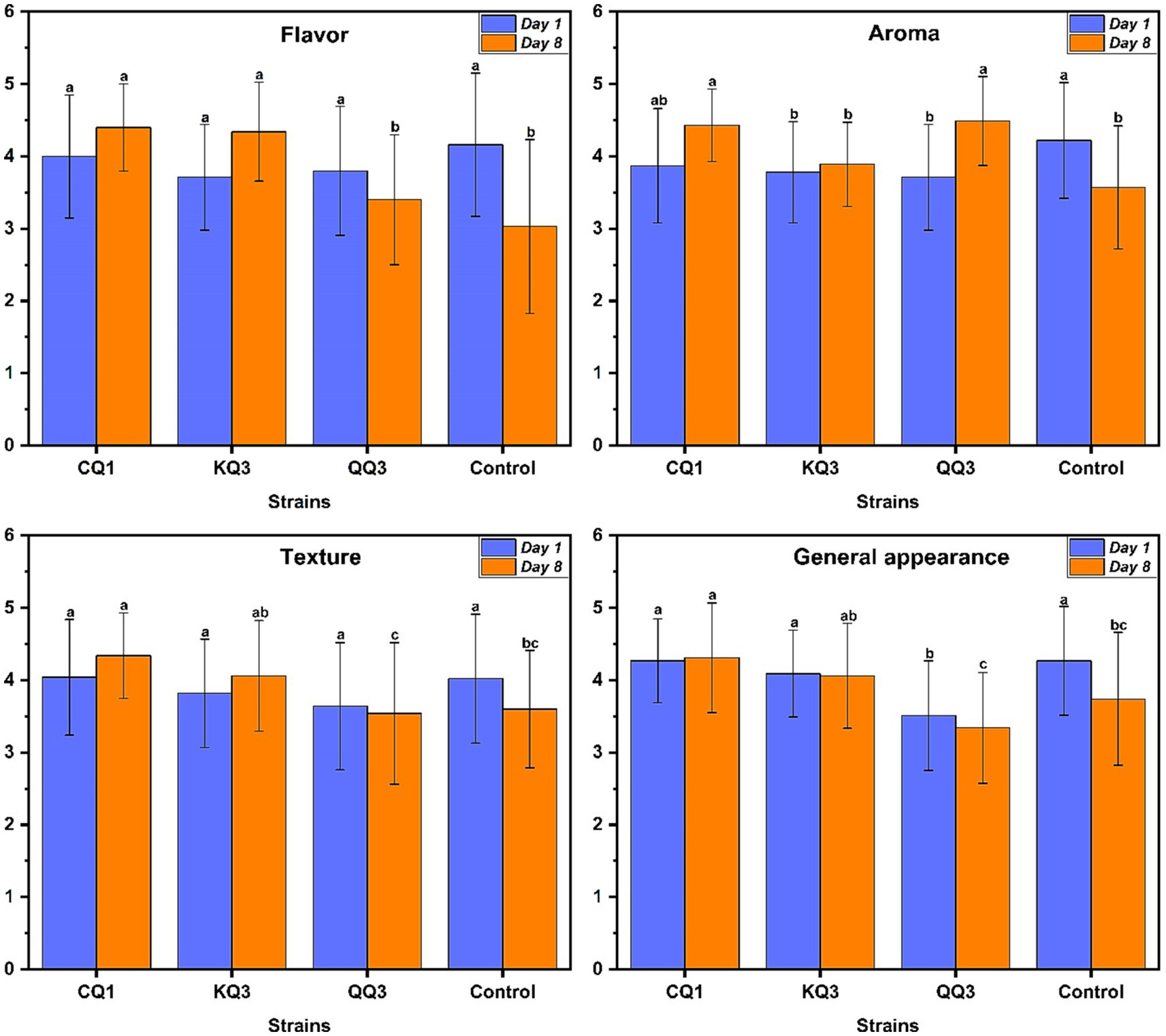
Figure 7. Effect of strains on the sensory properties of cheese. The figure shows the effect of the three selected strains (CQ1: L. paracasei, KQ3: L. paracasei and QQ3: Lactiplantibacillus sp.) in the sensory properties of the cheese (flavor, smell, texture and appearance in general) on the first and eighth days of fermentation. The letters indicate different statistical groups according to Tukey’s test (p < 0.05).
Cheese made with the CQ1 strain received the highest scores for flavor (4.4 ± 0.60), texture (4.34 ± 0.59) and general appearance (4.31 ± 0.76). For aroma, the strains CQ1 (4.43 ± 0.50) and QQ3 (4.49 ± 0.61) obtained the highest score (Supplementary Table S3). Therefore, the acceptance of cheese inoculated with strains CQ1 and KQ3 may be related to the level of proteolysis and lipolysis exerted by these strains (Figures 5, 6). Coelho et al. (2022) and Araújo-Rodrigues et al. (2023) mention that amino acids and fatty acids released during proteolysis and lipolysis play an active role as substrates in several secondary reactions that drive the flavor and texture of cheese. The good acceptance of the aroma of cheese inoculated with the QQ3 strain could be related to the production of diacetyl (Supplementary Table S2), which is one of the main compounds responsible for the flavor and aroma in dairy products such as cheese and yogurt (Hernandez-Valdes et al., 2020). Likewise, Jo et al. (2018) demonstrated the contribution of diacetyl to the buttery flavor in young Gouda cheese.
In general, the strains used in cheese production seem to be promising in improving the sensory properties of cheese (Supplementary Figure S4). However, sensory acceptability could be further improved by increasing the maturation time and using these LAB in consortia in which each bacteria could contribute specific characteristics to the final product. Awad et al. (2007) mentioned that cheese made with bacterial consortia made up of L. paracasei subsp. paracasei added along with cultures such as L. lactis subsp. lactis, Lactococcus lactis subsp. cremoris, Streptococcus thermophilus and Lactobacillus helveticus are sensory more acceptable.
3.11 Physicochemical characteristics of traditional cheeses
Table 4 shows the proximal composition of the cheese made with L. paracasei CQ1 (sensorially better rated cheese) and the control (without inoculum) at 8 days of ripening, these results are within the expected parameters. The moisture content of cheese made with L. paracasei CQ1 was recorded at 34%, lower than the control (44.29%). This suggests that the reduction of pH in cheese with L. paracasei CQ1 is due to the production of lactic acid by this strain, which in turn facilitates gel syneresis and whey expulsion, as mentioned in previous research (Doolan et al., 2014). As a consequence of this syneresis, ash, fat, protein and carbohydrate values increased relative to the total sample mass. Values close to these results have been reported at 7 days of ripening in Graviera cheese, when strains of Lactococcus lactis, Leuconostoc mesenteroides, Streptococcus thermophilus and Lactobacillus helveticus were added (Ioannidou et al., 2022). In addition, these authors noted that the decrease in moisture during the ripening process affects the proximal composition of both raw and pasteurized cheeses.
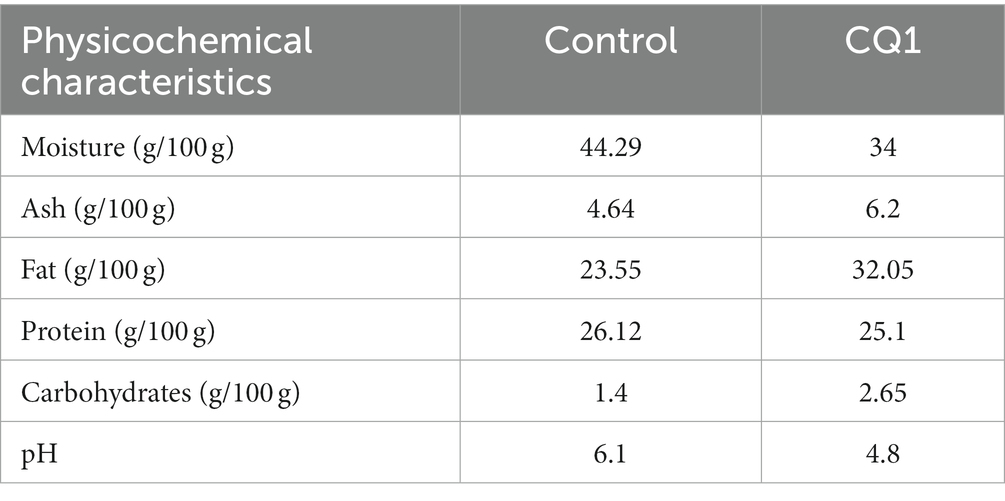
Table 4. Physicochemical characteristics of cheese without inoculum and cheese made with L. paracasei CQ1.
It should be noted that the results of the physicochemical characterization of the cheeses made using isolated strains are preliminary because no manufacturing replicates were carried out.
4 Conclusion
The native LAB strains selected from artisanal Swiss-type cheese from Ancash (Peru) were identified as L. paracasei (TQ1, YQ1, EQ1, LQ2, GQ2, KQ3, CQ1), L. parabuchneri (BQ2, OQ2, RQ3) and Lactiplantibacillus sp. (QQ3). Safety tests demonstrated that the strains did not present health risks because they did not show gelatinase or hemolytic activity. In addition, the antimicrobial activity of L. paracasei KQ3, GQ2 and L. parabuchneri BQ2 against S. aureus, E. coli and L. monocytogenes was highlighted. Variable susceptibility to antibiotics was also observed. Desirable technological characteristics were evidenced, such as acidifying, proteolytic, lipolytic activity and diacetyl production. As for cheese production with L. paracasei CQ1, L. paracasei KQ3 and Lactiplantibacillus sp. QQ3, an improvement was obtained in terms of flavor, aroma, texture and general appearance after 8 days of ripening. All this demonstrates that the strains isolated from Swiss-type artisanal cheese are safe at the in vitro level, have a good technological potential and improve the sensory qualities of the cheeses.
The results open an interesting scenario for the development of new research in cheese formulation using combinations of isolated bacterial strains together with commercial starter cultures, in order to increase sensory acceptability. In addition, it is convenient to study the effect of the ripening period of cheeses inoculated with LAB reported in the present study, on the profiles of volatile compounds and sensory acceptability.
Data availability statement
The datasets presented in this study can be found in online repositories. The names of the repository/repositories and accession number(s) can be found in the article/Supplementary material.
Author contributions
JV-M: conceptualization, formal analysis, investigation, methodology, writing–original draft, and writing–review and editing. NA-T: formal analysis, investigation, methodology, writing–original draft, and writing–review and editing. EV-P: supervision, investigation, validation and methodology, and writing–review and editing. LR-F: writing–review and editing, research, and visualization. MR-C: writing–review and editing, data curation, and formal analysis. CT-A: methodology, writing–review and editing, and molecular analysis. PO-G: writing–review and editing and molecular analysis. LE-E: methodology, investigation, supervision, writing–original draft, and writing–review and editing.
Funding
This research had financial support from the Universidad Nacional de Frontera, Peru, according to Organizing Commission Resolution No. 103-2022-UNF/CO, Agreement No. 007-2022-UNF; and Dean’s Resolution No 105-2023-UNF-VPAC/FIIAB.
Conflict of interest
The authors declare that the research was conducted in the absence of any commercial or financial relationships that could be construed as a potential conflict of interest.
Publisher’s note
All claims expressed in this article are solely those of the authors and do not necessarily represent those of their affiliated organizations, or those of the publisher, the editors and the reviewers. Any product that may be evaluated in this article, or claim that may be made by its manufacturer, is not guaranteed or endorsed by the publisher.
Supplementary material
The Supplementary material for this article can be found online at: https://www.frontiersin.org/articles/10.3389/fsufs.2023.1212229/full#supplementary-material
Footnotes
References
Abosereh, N. A., Abd, S., Ghani, E., Gomaa, R. S., and Fouad, M. T. (2016). Molecular identification of potential probiotic lactic acid bacteria strains isolated from Egyptian traditional fermented dairy products. Biotechnology 15, 35–43. doi: 10.3923/biotech.2016.35.43
Abriouel, H., Casado Muñoz, M. C., Lavilla Lerma, L., Pérez Montoro, B., Bockelmann, W., Pichner, R., et al. (2015). New insights in antibiotic resistance of Lactobacillus species from fermented foods. Food Res. Int. 78, 465–481. doi: 10.1016/j.foodres.2015.09.016
Afshari, A., Hashemi, M., Tavassoli, M., Eraghi, V., Mohammad, S., and Noori, A. (2022). Probiotic bacteria from 10 different traditional Iranian cheeses: isolation, characterization, and investigation of probiotic potential. Food Sci. Nutr. 10, 2009–2020. doi: 10.1002/fsn3.2817
Afshari, R., Pillidge, C. J., Dias, D. A., Osborn, A. M., and Gill, H. (2020). Cheesomics: the future pathway to understanding cheese flavour and quality. Crit. Rev. Food Sci. Nutr. 60, 33–47. doi: 10.1080/10408398.2018.1512471
Agostini, C., Eckert, C., Vincenzi, A., Machado, B. L., Jordon, B. C., Kipper, J. P., et al. (2018). Characterization of technological and probiotic properties of indigenous Lactobacillus spp. from South Brazil. 3 Biotech 8:451. doi: 10.1007/s13205-018-1469-7
Ahire, J. J., Jakkamsetty, C., Kashikar, M. S., Lakshmi, S. G., and Madempudi, R. S. (2021). In vitro evaluation of probiotic properties of Lactobacillus plantarum UBLP40 isolated from traditional indigenous fermented food. Probiotics Antimicrob. Proteins 13, 1413–1424. doi: 10.1007/s12602-021-09775-7
Akbulut, S., Baltaci, M., Adiguzel, G., and Adiguzel, A. (2022). Identification and biotechnological characterization of lactic acid cacteria isolated from white cheese samples. J. Pure Appl. Microbiol. 16, 2912–2922. doi: 10.22207/JPAM.16.4.66
Akpınar Kankaya, D., and Tuncer, Y. (2020). Antibiotic resistance in vancomycin-resistant lactic acid bacteria (VRLAB) isolated from foods of animal origin. J. Food Process. Preserv. 44:e14468. doi: 10.1111/jfpp.14468
Albayrak, Ç. B., and Duran, M. (2021). Isolation and characterization of aroma producing lactic acid bacteria from artisanal white cheese for multifunctional properties. LWT 150:112053. doi: 10.1016/j.lwt.2021.112053
Aprea, G., Alessiani, A., Rossi, F., Sacchini, L., Boni, A., Angelantonio, D. D., et al. (2021). Characterization of lactic acid bacteria in pecorino di Farindola cheese and manufacturing with a Lacticaseibacillus paracasei autochthonous culture. Appl. Sci. 11:7897. doi: 10.3390/app11177897
Araújo-Rodrigues, H., dos Santos, M. T. P. G., Ruiz-Moyano, S., Tavaria, F. K., Martins, A. P. L., Alvarenga, N., et al. (2021). Technological and protective performance of LAB isolated from Serpa PDO cheese: towards selection and development of an autochthonous starter culture. LWT 150:112079. doi: 10.1016/j.lwt.2021.112079
Araújo-Rodrigues, H., Martins, A. P. L., Tavaria, F. K., Dias, J., Santos, M. T., Alvarenga, N., et al. (2023). Impact of LAB from Serpa PDO cheese in cheese models: towards the development of an autochthonous starter culture. Foods 12, 1–24. doi: 10.3390/foods12040701
Ardö, Y., McSweeney, P. L. H., Magboul, A. A. A., Upadhyay, V. K., and Fox, P. F. (2017). “Biochemistry of cheese ripening: proteolysis” in Cheese: Chemistry, physics and microbiology. ed. Y. Ardö (Amsterdam, Netherlands: Elsevier Ltd.), 445–482.
Ashaolu, T. J. (2019). A review on selection of fermentative microorganisms for functional foods and beverages: the production and future perspectives. Int. J. Food Sci. Technol. 54, 2511–2519. doi: 10.1111/ijfs.14181
Awad, S., Ahmed, N., and El Soda, M. (2007). Evaluation of isolated starter lactic acid bacteria in Ras cheese ripening and flavour development. Food Chem. 104, 1192–1199. doi: 10.1016/j.foodchem.2007.01.043
Baptista, D. P., Galli, B. D., Cavalheiro, F. G., Negrão, F., Eberlin, M. N., and Gigante, M. L. (2018). Lactobacillus helveticus LH-B02 favours the release of bioactive peptide during Prato cheese ripening. Int. Dairy J. 87, 75–83. doi: 10.1016/j.idairyj.2018.08.001
Barreto, J. I., Henostroza, T. J., and Castro, R. R. (2010). Calidad sensorial de quesos en la ciudad de Huaraz. Aporte Santiaguino 3, 41–46. doi: 10.32911/as.2010.v3.n1.419
Barrios Renteria, J. C., Espinoza-Espinoza, L. A., Valdiviezo-Marcelo, J., and Moreno-Quispe, L. A. (2022). Sensorially accepted Mangifera indica and Myrciaria dubia yogurts with high ascorbic acid content. Front. Sustain. Food Syst. 6, 1–12. doi: 10.3389/fsufs.2022.999400
Barzegar, H., Alizadeh Behbahani, B., and Falah, F. (2021). Safety, probiotic properties, antimicrobial activity, and technological performance of Lactobacillus strains isolated from Iranian raw milk cheeses. Food Sci. Nutr. 9, 4094–4107. doi: 10.1002/fsn3.2365
Barzideh, Z., Siddiqi, M., Mohamed, H. M., and LaPointe, G. (2022). Dynamics of starter and non-starter lactic acid bacteria populations in long-ripened Cheddar cheese using propidium monoazide (PMA) treatment. Microorganisms 10:1669. doi: 10.3390/microorganismos10081669
Bekele, B., Hansen, E. B., Eshetu, M., Ipsen, R., and Hailu, Y. (2019). Effect of starter cultures on properties of soft white cheese made from camel (Camelus dromedarius) milk. J. Dairy Sci. 102, 1108–1115. doi: 10.3168/jds.2018-15084
Belleggia, L., Ferrocino, I., Rita Corvaglia, M., Cesaro, C., Milanović, V., Cardinali, F., et al. (2022). Profiling of autochthonous microbiota and characterization of the dominant lactic acid bacteria occurring in fermented fish sausages. Food Res. Int. 154:110990. doi: 10.1016/j.foodres.2022.110990
Beresford, T. P., Fitzsimons, N. A., Brennan, N. L., and Cogan, T. M. (2001). Recent advances in cheese microbiology. Int. Dairy J. 11, 259–274. doi: 10.1016/S0958-6946(01)00056-5
Berno, T., and Fusté-Forné, F. (2020). Imaginaries of cheese: revisiting narratives of local produce in the contemporary world. Ann. Leis. Res. 23, 608–626. doi: 10.1080/11745398.2019.1603113
Bezerra, T. K. A., De Araujo, A. R. R., Do Nascimento, E. S., De Matos Paz, J. E., Gadelha, C. A., Gadelha, T. S., et al. (2016). Proteolysis in goat “coalho” cheese supplemented with probiotic lactic acid bacteria. Food Chem. 196, 359–366. doi: 10.1016/j.foodchem.2015.09.066
Bintsis, T. (2017). Foodborne pathogens. AIMS Microbiol. 3, 529–563. doi: 10.3934/microbiol.2017.3.529
Biscola, V., Tulini, F. L., Choiset, Y., Rabesona, H., Ivanova, I., Chobert, J. M., et al. (2016). Proteolytic activity of Enterococcus faecalis VB63F for reduction of allergenicity of bovine milk proteins. J. Dairy Sci. 99, 5144–5154. doi: 10.3168/jds.2016-11036
Blaya, J., Barzideh, Z., and LaPointe, G. (2018). Symposium review: interaction of starter cultures and nonstarter lactic acid bacteria in the cheese environment. J. Dairy Sci. 101, 3611–3629. doi: 10.3168/jds.2017-13345
Câmara, S. P., Dapkevicius, A., Riquelme, C., Elias, R. B., Silva, C. C. G., Malcata, F. X., et al. (2019). Potential of lactic acid bacteria from Pico cheese for starter culture development. Food Sci. Technol. Int. 25, 303–317. doi: 10.1177/1082013218823129
Campagnollo, F. B., Margalho, L. P., Kamimura, B. A., Feliciano, M. D., Freire, L., Lopes, L. S., et al. (2018). Selection of indigenous lactic acid bacteria presenting anti-listerial activity, and their role in reducing the maturation period and assuring the safety of traditional Brazilian cheeses. Food Microbiol. 73, 288–297. doi: 10.1016/j.fm.2018.02.006
Cao, W., Aubert, J., Maillard, M. B., Boissel, F., Leduc, A., Thomas, J. L., et al. (2021). Fine-tuning of process parameters modulates specific metabolic bacterial activities and aroma compound production in semi-hard cheese. J. Agric. Food Chem. 69, 8511–8529. doi: 10.1021/acs.jafc.1c01634
Carafa, I., Stocco, G., Franceschi, P., Summer, A., Michael, K., Bittante, G., et al. (2019). Evaluation of autochthonous lactic acid bacteria as starter and non-starter cultures for the production of Traditional Mountain cheese. Food Res. Int. 115, 209–218. doi: 10.1016/j.foodres.2018.08.069
Cardinali, F., Ferrocino, I., Milanovi, V., Belleggia, L., Corvaglia, M. R., Garofalo, C., et al. (2021). Microbial communities and volatile profile of Queijo de Azeitão PDO cheese, a traditional mediterranean thistle-curdled cheese from Portugal. Food Res. Int. 147:110537. doi: 10.1016/j.foodres.2021.110537
Carrazco-Palafox, J., Rivera-Chavira, B. E., Ramírez-Baca, N., Manzanares-Papayanopoulos, L. I., and Nevárez-Moorillón, G. V. (2018). Improved method for qualitative screening of lipolytic bacterial strains. MethodsX 5, 68–74. doi: 10.1016/j.mex.2018.01.004
Carrim, A. J. I., Barbosa, E. C., and Vieira, J. D. G. (2006). Enzymatic activity of endophytic bacterial isolates of Jacaranda decurrens Cham. (Carobinha-do-campo). Braz. Arch. Biol. Technol. 49, 353–359. doi: 10.1590/s1516-89132006000400001
Carvalho, B., Ávila, C., Pinto, J., Neri, J., and Schwan, R. (2014). Microbiological and chemical profile of sugar cane silage fermentation inoculated with wild strains of lactic acid bacteria. Anim. Feed Sci. Technol. 195, 1–13. doi: 10.1016/j.anifeedsci.2014.04.003
Chandra, P., Enespa, S., Singh, R., and Arora, P. K. (2020). Microbial lipases and their industrial applications: A comprehensive review. Microb. Cell Factories 19, 1–42. doi: 10.1186/s12934-020-01428-8
Chen, C. N., Wu, G. M., Feng, W. S., and Jean, H. W. (2008). Direct patterning of silicon nitride thin film by projection photoablation for fabricating thin-film transistor liquid crystal displays. Jpn. J. Appl. Phys. 47, 2152–2154. doi: 10.1143/JJAP.47.2152
Chen, C., Zhao, S., Hao, G., Yu, H., Tian, H., and Zhao, G. (2017). Role of lactic acid bacteria on the yogurt flavour: A review. Int. J. Food Prop. 20, S316–S330. doi: 10.1080/10942912.2017.1295988
Cheng, N. (2013). Application of Fourier-transform infrared (FTIR) for quality control of Swiss cheese [Master thesis, Ohio State University]. Available at: http://rave.ohiolink.edu/etdc/view?acc_num=osu1366111160.
Cizeikiene, D., and Jagelaviciute, J. (2021). Investigation of antibacterial activity and probiotic properties of strains belonging to Lactobacillus and Bifidobacterium genera for their potential application in functional food and feed products. Probiotics Antimicrob. Proteins 13, 1387–1403. doi: 10.1007/s12602-021-09777-5
Clark, S., and Winter, C. K. (2015). Diacetyl in foods: A review of safety and sensory characteristics. Compr. Rev. Food Sci. Food Saf. 14, 634–643. doi: 10.1111/1541-4337.12150
Coelho, M. C., Malcata, F. X., and Silva, C. C. G. (2022). Lactic acid bacteria in raw-milk cheeses: from starter cultures to probiotic functions. Foods 11, 1–32. doi: 10.3390/foods11152276
Cogan, T. M., Barbosa, M., Beuvier, E., Bianchi-Salvadori, B., Cocconcelli, P. S., Fernandes, I., et al. (1997). Characterization of the lactic acid bacteria in artisanal dairy products. J. Dairy Res. 64, 409–421. doi: 10.1017/S0022029997002185
Collazos, C., White, P. L., White, H. S., Viñas, E., and Alvestur, E. (1993). La composición de alimentos de mayor consumo en el Perú (No. Q04 C6–R). Peru: Lima Ministerio de Salud; Instituto Nacional de Nutrición.
Dagdemir, E., and Ozdemir, S. (2008). Technological characterization of the natural lactic acid bacteria of artisanal Turkish White pickled cheese. Int. J. Dairy Technol. 61, 133–140. doi: 10.1111/j.1471-0307.2008.00394.x
De Melo Pereira, V. G., De Carvalho Neto, D. P., Junqueira, A. C. D. O., Karp, S. G., Letti, L. A. J., Magalhães Júnior, A. I., et al. (2020). A review of selection criteria for starter culture development in the food fermentation industry. Food Rev. Int. 36, 135–167. doi: 10.1080/87559129.2019.1630636
De Oliveira, A. P. D., De Oliveira Almeida, T. J., Santos, T. M. B., and Dias, F. S. (2021). Symbiotic goat milk ice cream with umbu fortified with autochthonous goat cheese lactic acid bacteria. LWT 141:110888. doi: 10.1016/j.lwt.2021.110888
De Souza, J. V., and Silva Dias, F. (2017). Protective, technological, and functional properties of select autochthonous lactic acid bacteria from goat dairy products. Curr. Opin. Food Sci. 13, 1–9. doi: 10.1016/j.cofs.2017.01.003
Dogan, G., and Taskin, B. (2021). Hydrolytic enzymes producing bacterial endophytes of some poaceae plants. Pol. J. Microbiol. 70, 297–304. doi: 10.33073/PJM-2021-026
Domingos-Lopes, M. F. P., Stanton, C., Ross, P. R., Dapkevicius, M. L. E., and Silva, C. C. G. (2017). Genetic diversity, safety and technological characterization of lactic acid bacteria isolated from artisanal Pico cheese. Food Microbiol. 63, 178–190. doi: 10.1016/j.fm.2016.11.014
Doolan, I. A., Nongonierma, A. B., Kilcawley, K. N., and Wilkinson, M. G. (2014). Partitioning of starter bacteria and added exogenous enzyme activities between curd and whey during Cheddar cheese manufacture. Int. Dairy J. 34, 159–166. doi: 10.1016/j.idairyj.2013.07.005
El Soda, M., Law, J., Tsakalidou, E., and Kalantzopoulos, G. (1995). “Lipolytic activity of cheese related microorganisms and its impact on cheese flavour” in Developments in food science. ed. M. El Soda (Amsterdam, Netherlands: Elsevier), 1823–1847.
Falsen, E., Pascual, C., Sjoden, B., Ohlén, M., and Collins, M. D. (1999). Phenotypic and phylogenetic characterization of a novel Lactobacillus species from human sources: description of Lactobacillus iners sp. nov. Int. J. Syst. Evol. 49, 217–221. doi: 10.1099/00207713-49-1-217
Fauziah, R., Malaka, R., and Yuliati, F. N. (2020). Titratable acidity and pH changes of pasteurized milk by addition of Roselle flower extract in the refrigerator storage. IOP Conf. Ser.: Earth Environ. 492:012057. doi: 10.1088/1755-1315/492/1/012057
Fox, P. F., and Guinee, T. P. (2013). “Cheese science and technology” in Milk and dairy products in human nutrition: Production, composition and health. eds. Y. W. Park and G. F. Haenlein (New York: John Wiley & Sons, Ltd), 357–389.
Fröhlich-Wyder, M. T., Bisig, W., Guggisberg, D., Irmler, S., Jakob, E., and Wechsler, D. (2015). Influence of low pH on the metabolic activity of Lactobacillus buchneri and Lactobacillus parabuchneri strains in Tilsit-type model cheese. Dairy Sci. Technol. 95, 569–585. doi: 10.1007/s13594-015-0238-1
Fröhlich-Wyder, M. T., Bisig, W., Guggisberg, D., Jakob, E., Turgay, M., and Wechsler, D. (2017). “Cheeses with propionic acid fermentation” in Cheese: Chemistry, physics and microbiology. 4th ed (Amsterdam, Netherlands: Elsevier Ltd.), 889–910.
Fröhlich-Wyder, M. T., Guggisberg, D., Badertscher, R., Wechsler, D., Wittwer, A., and Irmler, S. (2013). The effect of Lactobacillus buchneri and Lactobacillus parabuchneri on the eye formation of semi-hard cheese. Int. Dairy J. 33, 120–128. doi: 10.1016/j.idairyj.2013.03.004
Gálvez Antinori, K. E. (2020). Estrategias innovadoras en las plantas queseras en Cajamarca, La Libertad y Ancash [Tesis de Maestría, Universidad Nacional Agraria La Molina]. Available at: https://hdl.handle.net/20.500.12996/4368.
González-González, F., Delgado, S., Ruiz, L., Margolles, A., and Ruas-Madiedo, P. (2022). Functional bacterial cultures for dairy applications: towards improving safety, quality, nutritional and health benefit aspects. J. Appl. Microbiol. 133, 212–229. doi: 10.1111/jam.15510
Gonzalez-Gonzalez, C. R., Machado, J., Correia, S., McCartney, A. L., Stephen Elmore, J., and Jauregi, P. (2019). Highly proteolytic bacteria from semi-ripened Chiapas cheese elicit angiotensin-I converting enzyme inhibition and antioxidant activity. LWT 111, 449–456. doi: 10.1016/j.lwt.2019.05.039
Guevara Cruzado, A. Y., and Reyes Flores, K. E. (2019). Propuesta de implementación del sistema de análisis de peligros y puntos críticos de control (HACCP) en la industria alimentaria “HUACARIZ S.A.C”, para mejorar la calidad sanitaria del queso semimaduro tipo suizo Tesis de Título, Universidad Privada del. Available at: https://hdl.handle.net/11537/21081.
Hajigholizadeh, M., Mardani, K., Moradi, M., and Jamshidi, A. (2020). Molecular detection, phylogenetic analysis, and antibacterial performance of lactic acid bacteria isolated from traditional cheeses, North-West Iran. Food Sci. Nutr. 8, 6007–6013. doi: 10.1002/fsn3.1887
Hassanzadazar, H., Mardani, K., Yousefi, M., and Ehsani, A. (2017). Identification and molecular characterisation of lactobacilli isolated from traditional Koopeh cheese. Int. J. Dairy Technol. 70, 556–561. doi: 10.1111/1471-0307.12396
Hawaz, E., Guesh, T., Kebede, A., and Menkir, S. (2016). Characterization of lactic acid bacteria from camel milk and their technological properties to use as a starter culture. East Afr. J. Sci. 10, 49–60.
Hejazi, M. A., Ghafouri-Fard, S., Eslami, S., Afshar, D., Barzegari, A., and Khorshidian, N. (2019). Polyphasic characterization of Enterococcus strains isolated from traditional Moghan cheese in Iran. J. Food Saf. 39, 1–9. doi: 10.1111/jfs.12631
Hernandez-Valdes, J. A., Solopova, A., and Kuipers, O. P. (2020). Development of Lactococcus lactis biosensors for detection of diacetyl. Front. Microbiol. 11, 1–15. doi: 10.3389/fmicb.2020.01032
Hu, F. (2012). Application of high performance liquid chromatography (HPLC) and fourier transform infrared (FTIR) spectroscopy to Swiss-type cheese split defects [master thesis, Ohio State University]. Available at: http://rave.ohiolink.edu/etdc/view?acc_num=osu1343392495.
Huang, H., Peng, F., Li, J., Liu, Z., Xie, M., and Xiong, T. (2021). Isolation and characteristics of lactic acid bacteria with antibacterial activity against Helicobacter pylori. Food Biosci. 44:101446. doi: 10.1016/j.fbio.2021.101446
Hugenholtz, J., Kleerebezem, M., Starrenburg, M., Delcour, J., De Vos, W., and Hols, P. (2000). Lactococcus lactis as a cell factory for high-level diacetyl production. Appl. Environ. Microbiol. 66, 4112–4114. doi: 10.1128/AEM.66.9.4112-4114.2000
Ioannidou, M. D., Maggira, M., and Samouris, G. (2022). Physicochemical characteristics, fatty acids profile and lipid oxidation during ripening of graviera cheese produced with raw and pasteurized milk. Foods 11:2138. doi: 10.3390/foods11142138
Islam, M. Z., Uddin, M. E., Rahman, M. T., Islam, M. A., and Harun-ur-Rashid, M. (2021). Isolation and characterization of dominant lactic acid bacteria from raw goat milk: assessment of probiotic potential and technological properties. Small Rumin. Res. 205:106532. doi: 10.1016/j.smallrumres.2021.106532
Jo, Y., Benoist, D. M., Ameerally, A., and Drake, M. A. (2018). Sensory and chemical properties of gouda cheese. J. Dairy Sci. 101, 1967–1989. doi: 10.3168/jds.2017-13637
Jomehzadeh, N., Javaherizadeh, H., Amin, M., Saki, M., Al-Ouqaili, M. T. S., Hamidi, H., et al. (2020). Isolation and identification of potential probiotic Lactobacillus species from feces of infants in Southwest Iran. Int. J. Infect. Dis. 96, 524–530. doi: 10.1016/j.ijid.2020.05.034
Kakelar, H. M., Barzegari, A., Hanifian, S., Barar, J., and Omidi, Y. (2019). Isolation and molecular identification of Lactobacillus with probiotic potential from abomasums driven rennet. Food Chem. 272, 709–714. doi: 10.1016/j.foodchem.2018.08.081
Kamarinou, C. S., Papadopoulou, O. S., Doulgeraki, A. I., Tassou, C. C., Galanis, A., Chorianopoulos, N. G., et al. (2022). Mapping the key technological and functional characteristics of indigenous lactic acid bacteria isolated from Greek traditional dairy products. Microorganisms 10:246. doi: 10.3390/microorganisms10020246
Kang, W., Pan, L., Peng, C., Dong, L., Cao, S., Zhang, C., et al. (2020). Isolation and characterization of lactic acid bacteria from human milk. J. Dairy Sci. 103, 9980–9991. doi: 10.3168/jds.2020-18704
Khattab, A. R., Guirguis, H. A., Tawfik, S. M., and Farag, M. A. (2019). Cheese ripening: A review on modern technologies towards flavor enhancement, process acceleration and improved quality assessment. Trends Food Sci. Technol. 88, 343–360. doi: 10.1016/j.tifs.2019.03.009
Kilcawley, K. N. (2016). “Cheese flavour” in Fundamentals of cheese science. ed. K. N. Kilcawley. 2nd ed (Boston, MA: Springer), 443–473.
Kim, Y., Yoon, S., Shin, H., Jo, M., Lee, S., and Kim, S. H. (2021). Isolation of Lactococcus lactis ssp. cremoris LRCC5306 and optimization of diacetyl production conditions for manufacturing sour cream. Food Sci. Anim. Resour. 41, 373–385. doi: 10.5851/KOSFA.2021.E3
Klein, G., Pack, A., Bonaparte, C., and Reuter, G. (1998). Taxonomy and physiology of probiotic lactic acid bacteria. Int. J. Food Microbiol. 41, 103–125. doi: 10.1016/S0168-1605(98)00049-X
Leeuwendaal, N. K., Hayes, J. J., Stanton, C., O’Toole, P. W., and Beresford, T. P. (2022). Protection of candidate probiotic lactobacilli by Cheddar cheese matrix during simulated gastrointestinal digestion. J. Funct. Foods 92:105042. doi: 10.1016/j.jff.2022.105042
Leeuwendaal, N., Stanton, C., O’Toole, P. W., and Beresford, T. P. (2021). The potential of non-starter lactic acid bacteria from Cheddar cheese to colonise the gut. J. Funct. Foods 83:104425. doi: 10.1016/j.jff.2021.104425
Levante, A., Bancalari, E., Tambassi, M., Lazzi, C., Neviani, E., and Gatti, M. (2020a). Phenotypic diversity of Lactobacillus casei group isolates as a selection criterion for use as secondary adjunct starters. Microorganisms 8:128. doi: 10.3390/microorganisms8010128
Levante, A., Bertani, G., Bottari, B., Bernini, V., Lazzi, C., Gatti, M., et al. (2020b). How new molecular approaches have contributed to shedding light on microbial dynamics in Parmigiano Reggiano cheese. Curr. Opin. Food Sci. 38, 131–140. doi: 10.1016/j.cofs.2020.11.005
Liang, N., Zhao, Z., Curtis, J. M., and Gänzle, M. G. (2022). Antifungal cultures and metabolites of lactic acid bacteria for use in dairy fermentations. Int. J. Food Microbiol. 383:109938. doi: 10.1016/j.ijfoodmicro.2022.109938
Lucumi-Banguero, R. S., Ramírez-Toro, C., and Bolívar, G. A. (2021). Potential use of lactic acid bacteria with pathogen inhibitory capacity as a biopreservative agent for chorizo. PRO 9, 1–16. doi: 10.3390/pr9091582
Luiz, L. M. P., Castro, R. D., Sandes, S. H. C., Silva, J. G., Oliveira, L. G., Sales, G. A., et al. (2017). Isolation and identification of lactic acid bacteria from Brazilian Minas artisanal cheese. CYTA J. Food 15, 1–4. doi: 10.1080/19476337.2016.1219392
Mangia, N. P., Saliba, L., and Deiana, P. (2019). Functional and safety characterization of autochthonous Lactobacillus paracasei FS103 isolated from sheep cheese and its survival in sheep and cow fermented milks during cold storage. Ann. Microbiol. 69, 161–170. doi: 10.1007/s13213-018-1416-1
Marchwińska, K., and Gwiazdowska, D. (2022). Isolation and probiotic potential of lactic acid bacteria from swine feces for feed additive composition. Arch. Microbiol. 204, 61–21. doi: 10.1007/s00203-021-02700-0
Margalho, L. P., Feliciano, M. D. E., Silva, C. E., Abreu, J. S., Piran, M. V. F., and Sant’Ana, A. S. (2020). Brazilian artisanal cheeses are rich and diverse sources of nonstarter lactic acid bacteria regarding technological, biopreservative, and safety properties–insights through multivariate analysis. J. Dairy Sci. 103, 7908–7926. doi: 10.3168/jds.2020-18194
Margalho, L. P., Kamimura, B. A., Brexó, R. P., Alvarenga, V. O., Cebeci, A. S., Janssen, P. W. M., et al. (2021). High throughput screening of technological and biopreservation traits of a large set of wild lactic acid bacteria from Brazilian artisanal cheeses. Food Microbiol. 100:103872. doi: 10.1016/j.fm.2021.103872
Mauricio-Sandoval, E. A., Espinoza-Espinoza, L. A., Ruiz-Flores, L. A., Valdiviezo-Marcelo, J., Moreno-Quispe, L. A., and Cornelio-Santiago, H. P. (2023). Influence of the pulp of Mangifera indica and Myrciaria dubia on the bioactive and sensory properties of ice cream. Front. Sustain. Food Syst. 7:1126448. doi: 10.3389/fsufs.2023.1126448
Meng, Z., Zhang, L., Xin, L., Lin, K., Yi, H. X., and Han, X. (2018). Technological characterization of Lactobacillus in semihard artisanal goat cheeses from different Mediterranean areas for potential use as nonstarter lactic acid bacteria. J. Dairy Sci. 101, 2887–2896. doi: 10.3168/jds.2017-14003
Meral Aktaş, H., and Erdoğan, A. (2022). Characterization of technological properties of lactic acid bacteria isolated from Turkish Beyaz (white) cheese. J. Food Process. Preserv. 46:e16837. doi: 10.1111/jfpp.16837
Metrouh, R., Fares, R., Mechai, A., Debabza, M., and Menasria, T. (2022). Technological properties and probiotic potential of Lactiplantibacillus plantarum SJ14 isolated from Algerian traditional cheese “Jben.”. J. Food Process. Preserv. 46, 1–13. doi: 10.1111/jfpp.16482
Miao, J., Liu, G., Ke, C., Fan, W., Li, C., Chen, Y., et al. (2016). Inhibitory effects of a novel antimicrobial peptide from kefir against Escherichia coli. Food Control 65, 63–72. doi: 10.1016/j.foodcont.2016.01.023
Miranda, C., Contente, D., Igrejas, G., Câmara, S. P., Dapkevicius, M. D. L. E., and Poeta, P. (2021). Role of exposure to lactic acid bacteria from foods of animal origin in human health. Foods 10:2092. doi: 10.3390/foods10092092
Mohammed, S., and Çon, A. H. (2021). Isolation and characterization of potential probiotic lactic acid bacteria from traditional cheese. LWT 152:112319. doi: 10.1016/j.lwt.2021.112319
Monteagudo-Mera, A., Caro, I., Rodriguez-Aparicio, L. B., Rua, J., Ferrero, M. A., and Garcia-Armesto, M. R. (2011). Characterization of certain bacterial strains for potential use as starter or probiotic cultures in dairy products. J. Food Prot. 74, 1379–1386. doi: 10.4315/0362-028X.JFP-10-392
Morandi, S., Brasca, M., and Lodi, R. (2011). Technological, phenotypic and genotypic characterisation of wild lactic acid bacteria involved in the production of Bitto PDO Italian cheese. Dairy Sci. Technol. 91, 341–359. doi: 10.1007/s13594-011-0016-7
Mulaw, G., Tessema, T. S., Muleta, D., and Tesfaye, A. (2019). In vitro evaluation of probiotic properties of lactic acid bacteria isolated from some traditionally fermented Ethiopian food products. Int. J. Microbiol. 2019, 7179514–7179521. doi: 10.1155/2019/7179514
Nawaz, M., Wang, J., Zhou, A., Ma, C., Wu, X., Moore, J. E., et al. (2011). Characterization and transfer of antibiotic resistance in lactic acid bacteria from fermented food products. Curr. Microbiol. 62, 1081–1089. doi: 10.1007/s00284-010-9856-2
NTP. (2016). Norma Técnica peruana - NTP 202.001:2016 - Milk and milk products. Raw milk. Requirements, pp. 1–9.
Ouattara, H. D., Ouattara, H. G., Droux, M., Reverchon, S., Nasser, W., and Niamke, S. L. (2017). Lactic acid bacteria involved in cocoa beans fermentation from Ivory Coast: species diversity and citrate lyase production. Int. J. Food Microbiol. 256, 11–19. doi: 10.1016/j.ijfoodmicro.2017.05.008
Panebianco, F., Giarratana, F., Caridi, A., Sidari, R., De Bruno, A., and Giuffrida, A. (2021). Lactic acid bacteria isolated from traditional Italian dairy products: activity against listeria monocytogenes and modelling of microbial competition in soft cheese. LWT 137:110446. doi: 10.1016/j.lwt.2020.110446
Papadopoulou, O. S., Argyri, A. A., Varzakis, E. E., Tassou, C. C., and Chorianopoulos, N. G. (2018). Greek functional feta cheese: enhancing quality and safety using a Lactobacillus plantarum strain with probiotic potential. Food Microbiol. 74, 21–33. doi: 10.1016/j.fm.2018.02.005
Parlindungan, E., Lugli, G. A., Ventura, M., van Sinderen, D., and Mahony, J. (2021). Lactic acid bacteria diversity and characterization of probiotic candidates in fermented meats. Foods 10:1519. doi: 10.3390/foods10071519
Petkova, M., Stefanova, P., Gotcheva, V., and Angelov, A. (2021). Isolation and characterization of lactic acid bacteria and yeasts from typical Bulgarian sourdoughs. Microorganisms 9:1346. doi: 10.3390/microorganisms9071346
Pisano, M. B., Fadda, M. E., Viale, S., Deplano, M., Mereu, F., Blažić, M., et al. (2022). Inhibitory effect of Lactiplantibacillus plantarum and Lactococcus lactis autochtonous strains against Listeria monocytogenes in a laboratory cheese model. Foods 11:715. doi: 10.3390/foods11050715
Pundir, R. K., Rana, S., Kashyap, N., and Kaur, A. (2013). Probiotic potential of lactic acid bacteria isolated from food samples: an in vitro study. J. Appl. Pharm. Sci. 3, 85–93. doi: 10.7324/JAPS.2013.30317
Ramakrishnan, V., Narayan, B., and Halami, P. M. (2019). “Lipase of lactic acid bacteria” in Microbes for sustainable development and bioremediation. eds. R. Chandra and R. C. Sobti (Boca Raton: CRC Press), 313–323.
Randazzo, C. L., Liotta, L., De Angelis, M., Celano, G., Russo, N., Van Hoorde, K., et al. (2021). Adjunct culture of non-starter lactic acid bacteria for the production of provola Dei nebrodi PDO cheese: in vitro screening and pilot-scale cheese-making. Microorganisms 9, 1–18. doi: 10.3390/microorganisms9010179
Reuben, R. C., Roy, P. C., Sarkar, S. L., Rubayet Ul Alam, A. S. M., and Jahid, I. K. (2020). Characterization and evaluation of lactic acid bacteria from indigenous raw milk for potential probiotic properties. J. Dairy Sci. 103, 1223–1237. doi: 10.3168/jds.2019-17092
Ribeiro, S. C., Coelho, M. C., and Silva, C. C. G. (2021). A rapid screening method to evaluate acidifying activity by lactic acid bacteria. J. Microbiol. Methods 185:106227. doi: 10.1016/j.mimet.2021.106227
Russo, N., Caggia, C., Pino, A., Coque, T. M., Arioli, S., and Randazzo, C. L. (2018). Enterococcus spp. in Ragusano PDO and pecorino Siciliano cheese types: A snapshot of their antibiotic resistance distribution. Food Chem. Toxicol. 120, 277–286. doi: 10.1016/j.fct.2018.07.023
Salgado, C. A., dos Santos, C. I. A., and Vanetti, M. C. D. (2022). Microbial lipases: propitious biocatalysts for the food industry. Food Biosci. 45:101509. doi: 10.1016/j.fbio.2021.101509
Samelis, J., and Kakouri, A. (2022). Microbiological characterization of Greek Galotyri cheese PDO products relative to whether they are marketed fresh or ripened. Fermentation 8:492. doi: 10.3390/fermentation8100492
Sarasan, M., Job, N., Puthumana, J., Ravinesh, R., Kachiprath, B., and Philip, R. (2021). Enzyme profiling of macroalgal endophytes: an attempt to uncover the arsenal of novel biocatalysts. J. Mar. Biol. Assoc. India 62, 112–116. doi: 10.6024/jmbai.2020.62.2.2183-14
Sharma, A., Lavania, M., Singh, R., and Lal, B. (2021). Identification and probiotic potential of lactic acid bacteria from camel milk. Saudi J. Biol. Sci. 28, 1622–1632. doi: 10.1016/j.sjbs.2020.11.062
Sharma, A., Lee, S., and Park, Y. S. (2020). Molecular typing tools for identifying and characterizing lactic acid bacteria: a review. Food Sci. Biotechnol. 29, 1301–1318. doi: 10.1007/s10068-020-00802-x
Shehata, M. G., Badr, A. N., and El-aziz, N. M. A. (2022). Optimization, partial purification, and characterization of bioactive peptides of Lactobacillus paracasei isolated from traditional Egyptian cheese. J. Food Qual. Hazards Control 9, 199–214. doi: 10.18502/jfqhc.9.4.11375
Shi, Y., Cui, X., Gu, S., Yan, X., Li, R., Xia, S., et al. (2018). Antioxidative and probiotic activities of lactic acid bacteria isolated from traditional artisanal milk cheese from Northeast China. Probiot. Antimicrob. Proteins 11, 1086–1099. doi: 10.1007/s12602-018-9452-5
Silva, E. O. O., Nespolo, C. R., Sehn, C. P., Pinheiro, F. C., and Stefani, L. M. (2020). Lactic acid bacteria with antimicrobial, proteolytic and lipolytic activities isolated from ovine dairy products. Food Sci. Technol. 40, 293–299. doi: 10.1590/fst.11019
Silva, B. N., Teixeira, J. A., Cadavez, V., and Gonzales-Barron, U. (2023). Mild heat treatment and biopreservatives for artisanal raw milk cheeses: reducing microbial spoilage and extending shelf-life through thermisation, plant extracts and lactic acid bacteria. Foods 12:3206. doi: 10.3390/foods12173206
Singla, V., Mandal, S., Sharma, P., Anand, S., and Tomar, S. K. (2018). Antibiotic susceptibility profile of Pediococcus spp. from diverse sources. 3 Biotech 8, 489–417. doi: 10.1007/s13205-018-1514-6
Srinivash, M., Krishnamoorthi, R., Mahalingam, P. U., Malaikozhundan, B., and Keerthivasan, M. (2023). Probiotic potential of exopolysaccharide producing lactic acid bacteria isolated from homemade fermented food products. J. Agric. Food Res. 11:100517. doi: 10.1016/j.jafr.2023.100517
Stefanovic, E., Kilcawley, K. N., Roces, C., Rea, M. C., Sullivan, M. O., Sheehan, J. J., et al. (2018). Evaluation of the potential of Lactobacillus paracasei adjuncts for flavor compounds development and diversification in short-aged Cheddar cheese. Front. Microbiol. 9:1506. doi: 10.3389/fmicb.2018.01506
Tamariz-Angeles, C., Olivera-Gonzales, P., and Santillán-Torres, M. (2018). Antimicrobial, antioxidant and phytochemical assessment of wild medicinal plants from cordillera Blanca (Ancash, Peru). Bol. Latinoam. Caribe Plant Med. Aromat. 17, 270–285.
Tamariz-Angeles, C., Olivera-Gonzales, P., Santillán-Torres, M., Briceño-Luna, V., Silva-Villafana, A., and Villena, G. K. (2023). Diverse biological activities and secondary metabolites profile of Penicillium brevicompactum HE19ct isolated from the high-Andean medicinal plant Perezia coerulescens. Fungal Biol. 127, 1439–1450. doi: 10.1016/j.funbio.2023.10.002
Tambekar, D. H., and Bhutada, S. A. (2010). Acid and bile tolerance, antibacterial activity, antibiotic resistance and bacteriocins activity of probiotic Lactobacillus species. Recent Res. Sci. Technol. 2, 94–98.
Tanasupawat, S., Phoottosavako, M., and Keeratipibul, S. (2015). Characterization and lipolytic activity of lactic acid bacteria isolated from Thai fermented meat. J. Appl. Pharm. Sci. 5, 006–012. doi: 10.7324/JAPS.2015.50302
Tian, H., Yu, B., Yu, H., and Chen, C. (2020). Evaluation of the synergistic olfactory effects of diacetyl, acetaldehyde, and acetoin in a yogurt matrix using odor threshold, aroma intensity, and electronic nose analyses. J. Dairy Sci. 103, 7957–7967. doi: 10.3168/jds.2019-17495
Tringe, S. G., and Hugenholtz, P. (2008). A renaissance for the pioneering 16S rRNA gene. Curr. Opin. Microbiol. 11, 442–446. doi: 10.1016/j.mib.2008.09.011
Tsigkrimani, M., Panagiotarea, K., Paramithiotis, S., Bosnea, L., Pappa, E., Drosinos, E. H., et al. (2022). Microbial ecology of sheep milk, artisanal feta, and Kefalograviera cheeses. Part II: technological, safety, and probiotic attributes of lactic acid bacteria isolates. Foods 11:459. doi: 10.3390/foods11030459
Tulini, F. L., Hymery, N., Haertlé, T., Le Blay, G., and De Martinis, E. C. P. (2016). Screening for antimicrobial and proteolytic activities of lactic acid bacteria isolated from cow, buffalo and goat milk and cheeses marketed in the southeast region of Brazil. J. Dairy Res. 83, 115–124. doi: 10.1017/S0022029915000606
Tyl, C., and Sadler, G. D. (2017). “pH and titratable acidity” in Food analysis. ed. C. Tyl (Cham: Springer), 389–406.
Ulloa-Muñoz, R., Olivera-Gonzales, P., Castañeda-Barreto, A., Villena, G. K., and Tamariz-Angeles, C. (2020). Diversity of endophytic plant-growth microorganisms from Gentianella weberbaueri and Valeriana pycnantha, highland Peruvian medicinal plants. Microbiol. Res. 233:126413. doi: 10.1016/j.micres.2020.126413
Vera-Peña, M. Y., and Rodriguez-Rodriguez, W. L. (2020). Effect of pH on the growth of three lactic acid bacteria strains isolated from sour cream. Univ. Sci. 25, 341–358. doi: 10.11144/Javeriana.SC25-2.eopo
Výrostková, J., Regecová, I., Kováčová, M., Marcinčák, S., Dudriková, E., and Maľová, J. (2020). Antimicrobial resistance of Lactobacillus johnsonii and Lactobacillus zeae in raw milk. PRO 8:1627. doi: 10.3390/pr8121627
Wang, S., Chen, P., and Dang, H. (2019). “Lactic acid bacteria and γ-aminobutyric acid and diacetyl” in Lactic acid bacteria. ed. S. Wang (Singapore: Springer), 61–91.
Wang, J., Wu, T., Fang, X., and Yang, Z. (2019). Manufacture of low-fat Cheddar cheese by exopolysaccharide-producing Lactobacillus plantarum JLK0142 and its functional properties. J. Dairy Sci. 102, 3825–3838. doi: 10.3168/jds.2018-15154
Wang, K., Zhang, H., Feng, J., Ma, L., De la Fuente-Núñez, C., Wang, S., et al. (2019). Antibiotic resistance of lactic acid bacteria isolated from dairy products in Tianjin, China. J. Agric. Food Res. 1:100006. doi: 10.1016/j.jafr.2019.100006
Wolf, I. V., Peralta, G. H., Candioti, M., and Perotti, M. C. (2016). The role of propionibacteria in the volatile profile of Pategrás cheeses. Dairy Sci. Technol. 96, 551–567. doi: 10.1007/s13594-016-0289-y
Zafiro, L., Arellano, Y., Teresa, M., Cardona, N., Chula Quinto, C., Venancio, R., et al. (2007). Producción de gelatinasa (proteasa) por bacterias aerotransportadas. Available at: https://congresos.cio.mx/17_enc_mujer/cd_congreso/archivos/resumenes/S2/S2-BYQ15.pdf.
Zalán, Z., Hudáček, J., Štětina, J., Chumchalová, J., and Halász, A. (2010). Production of organic acids by Lactobacillus strains in three different media. Eur. Food Res. Technol. 230, 395–404. doi: 10.1007/s00217-009-1179-9
Zarzecka, U., Zadernowska, A., and Chajęcka-Wierzchowska, W. (2020). Starter cultures as a reservoir of antibiotic resistant microorganisms. LWT 127:109424. doi: 10.1016/j.lwt.2020.109424
Zhang, X., Ali, G., Fahad, A., Valan, M., Vijayaraghavan, P., Choon, K., et al. (2020). Probiotic characteristics of Lactobacillus strains isolated from cheese and their antibacterial properties against gastrointestinal tract pathogens. Saudi J. Biol. Sci. 27, 3505–3513. doi: 10.1016/j.sjbs.2020.10.022
Zheng, J., Wittouck, S., Salvetti, E., Franz, C. M. A. P., Harris, H. M. B., Mattarelli, P., et al. (2020). A taxonomic note on the genus Lactobacillus: description of 23 novel genera, emended description of the genus Lactobacillus beijerinck 1901, and union of Lactobacillaceae and Leuconostocaceae. Int. J. Syst. Evol. Microbiol. 70, 2782–2858. doi: 10.1099/ijsem.0.004107
Zoumpopoulou, G., Papadimitriou, K., Alexandraki, V., Mavrogonatou, E., Alexopoulou, K., Anastasiou, R., et al. (2020). The microbiota of Kalathaki and Melichloro Greek artisanal cheeses comprises functional lactic acid bacteria. LWT 130:109570. doi: 10.1016/j.lwt.2020.109570
Zoumpopoulou, G., Tzouvanou, A., Mavrogonatou, E., Alexandraki, V., Georgalaki, M., Anastasiou, R., et al. (2017). Probiotic features of lactic acid bacteria isolated from a diverse pool of traditional Greek dairy products regarding specific strain-host interactions. Probiot. Antimicrob. Proteins 10, 313–322. doi: 10.1007/s12602-017-9311-9
Keywords: protease, lipase, aromatic compounds, antibiotic resistance, molecular identification
Citation: Valdiviezo-Marcelo J, Arana-Torres NM, Vega-Portalatino EJ, Ruiz-Flores LA, Tamariz-Angeles C, Olivera-Gonzales P, Rosales-Cuentas MM and Espinoza-Espinoza LA (2023) Technological potential of native lactic acid bacteria isolated from Swiss-type artisanal cheese (Ancash, Peru) for their application in food. Front. Sustain. Food Syst. 7:1212229. doi: 10.3389/fsufs.2023.1212229
Edited by:
Ana Sanches Silva, National Institute for Agricultural and Veterinary Research (INIAV), PortugalReviewed by:
Guillermo Hugo Peralta, CONICET Instituto de Lactología Industrial (INLAIN), ArgentinaElisa Ale, CONICET Santa Fe, Argentina
Copyright © 2023 Valdiviezo-Marcelo, Arana-Torres, Vega-Portalatino, Ruiz-Flores, Tamariz-Angeles, Olivera-Gonzales, Rosales-Cuentas and Espinoza-Espinoza. This is an open-access article distributed under the terms of the Creative Commons Attribution License (CC BY). The use, distribution or reproduction in other forums is permitted, provided the original author(s) and the copyright owner(s) are credited and that the original publication in this journal is cited, in accordance with accepted academic practice. No use, distribution or reproduction is permitted which does not comply with these terms.
*Correspondence: Luis Alfredo Espinoza-Espinoza, bGVzcGlub3phQHVuZi5lZHUucGU=
†These authors have contributed equally to this work and share first authorship