- 1College of Food Science and Engineering, Central South University of Forestry and Technology, Changsha, China
- 2Hunan Horticultural Research Institute, Changsha, China
- 3Hunan Provincial Engineering Technology Research Center of Seasonings Green Manufacturing, Changsha, China
- 4Hunan Institute of Chemical Engineering, Changsha, China
The bacterial pathogen Pseudomonas syringae pv. actinidiae (Psa) adheres to the surface of kiwifruit and can spread over long distances during fruit transport and sales. It is crucial to investigate efficient approaches to eliminate Psa from the surface of kiwifruit, ensuring the prevention of Psa dissemination and maintaining the overall quality of kiwifruit. Vanillin is used for the preservation of agricultural products due to its antiseptic and bactericidal effect. In this paper, Psa was used to infect Cuiyu kiwifruits, and inhibition of exogenous vanillin on Psa and effects on the quality of the treated kiwifruits were investigated. According to the results of the inhibition of Psa through exposure to vanillin, the effectiveness of inhibition on the surface of kiwifruit was as follows: 61.29, 92.33, 96.79, and 100% for the concentrations of vanillin at 5.0 mg·mL−1, 6.0 mg·mL−1, 7.0 mg·mL−1, 8.0 mg·mL−1, 9.0 mg·mL−1, and 10.0 mg·mL−1, respectively. According to the quality results, kiwifruits treated with 8.0 mg·mL−1 vanillin exhibited a significant reduction in weight loss and rotting rate, with a decrease of 23.09 and 28.39% respectively, as compared to the control group. Additionally, thesetreated samples maintained a high level of total soluble solids, exhibited delayed degradation of total sugar content during a later period, inhibited an increase in respiratory intensity, and demonstrated increased firmness. Furthermore, vanillin treatment significantly increased the active values of superoxide, catalase, and peroxidase dismutase by 16.37, 28.75, and 23.92% respectively, compared to the control group. However, the levels of vitamin C and titratable acidity were not significantly affected by vanillin treatment. In conclusion, exogenous vanillin treatment exhibited potent inhibition capabilities against Psa, and effectively maintained the quality of kiwifruits during their shelf life. This study could provide reference basis for controlling the propagation of Psa and developing a new and natural kiwifruit preservative.
1. Introduction
Kiwifruit bacterial canker is a highly destructive and invasive disease, primarily affecting the stems and leaves of kiwifruit plants, and is caused by the Pseudomonas syringae pv. actinidae (Psa) (Li et al., 2020). Psa is highly virulent and can rapidly reproduce and spread within infected plants, leading to a decline in tree vigor and death within a short period of 1–2 years (Donati et al., 2020). Previous studies have shown that Psa can be detected in tissues such as pollen and fruit samples of kiwifruit (Barrett-Manako et al., 2021). Stefani and Giovanardi demonstrated that Psa could be detected in kiwifruit fruits at the 3rd, 9th, and 15th weeks by PCR after pollination with Psa-contaminated pollen (Stefani and Giovanardi, 2011). Gallelli et al. tested 32 kiwifruit fruit samples for Psa using real-time PCR technology, and 22 samples were positive (Gallelli et al., 2011). Zhu et al. used nested PCR technology to detect Psa on the surface of Hayward kiwifruit during storage in Zhouzhi County, Shaanxi Province, and the results showed that Psa was found in eight of 40 kiwifruit samples (Zhu et al., 2017). In 2020, China imported a total of about 116,800 t of kiwifruit and exported a total of 12,600 t. As Psa will adhere to the fruit surface and spread with the transportation and sales of kiwifruit, international trade may become the main way for Psa to spread over a long distance in the world (Vanneste et al., 2014). Consequently, studying effective methods to eradicate Psa from the surface of kiwifruit is crucial in preventing the pathogen’s spread and reducing the incidence of kiwifruit canker, while also ensuring the overall quality of the fruit (Cao et al., 2019).
As a natural substance in plants, plant polyphenols are a type of secondary metabolic compounds containing phenolic groups, which are divided into five compounds (Meng et al., 2023), namely phenolic acid, stilbene, flavonoid, coumarin and tannin. Plant polyphenols and their active components have become natural bactericides to control fruit postharvest diseases (Gao et al., 2018; Li et al., 2019; Jia et al., 2021; Cheng et al., 2022). In addition, plant polyphenols are also natural antioxidants that have an important effect on the color, flavor and oxidative stress of fruits (Dróżdż and Pyrzynska, 2018). Vanillin, derived from the seeds of the Vanilla fragrans orchid plant, is a polyphenolic compound widely recognized as one of the most extensively employed food flavoring agents globally (Chen et al., 2014). In addition to its role as a flavor enhancer and fixative, vanillin also has antibacterial, antioxidant, and stabilizing effects (Zabka and Pavela, 2013; Lv et al., 2015; Wei et al., 2021). According to Pei et al. (2021), vanillin was observed to effectively inhibit the growth of fungi in milk, resulting in prolonged spore germination time, reduced growth rate, and decreased mycelia growth. A concentration of 100 mg/mL of vanillin was found to reduce the colony growth rate of Actinomyces elegans by 88.45%, increase the lag phase by 8.60 times, and inhibit hypha growth by 85.93%, leading to damaged hypha morphology. Safari et al. (2020) adopted the combination of vanillin and chitosan for tomato storage and fresh-keeping and concluded that the treatment with 15 mM vanillin, in combination with a 1.5% chitosan coating, can significantly reduce the disease incidence rate and severity during tomato storage, by 74.16 and 79% respectively, and that the coating treatment of “vanillin+ chitosan” can also reduce the rot rate, fruit firmness and respiratory rate of tomatoes, and extend the shelf-life of fruits for 25 d. Li et al. (2020) adopted the compound coating treatment of 2 mg/mL vanillin and 1% chitosan, which could prolong the shelf-life of frozen turbot for 6–7 days.
Currently, there is limited research on the use of vanillin to kill Psa on the surface of kiwifruit and regulate post-harvest physiology. In this study, various concentrations of vanillin solutions were employed to perform indoor antibacterial experiments on Psa, which revealed a substantial inhibitory effect of vanillin on the pathogen. Furthermore, the impact of vanillin solution treatment on the shelf-life quality of kiwifruit was analyzed to develop efficient, safe, and environmentally friendly plant-based preservatives, and to provide scientific and effective references for controlling the spread of Psa on kiwifruit and for the application of storage and preservation technology.
2. Materials and methods
2.1. Materials and reagents
The “Cuiyu” kiwifruit (Actinidia chinensis cv. Cuiyu) was harvested from the kiwifruit experimental base of Hunan Academy of Agricultural Sciences. The Psa strain JF8 was kindly provided by Professor Liu Pu of Anhui Agricultural University. Vanillin was purchased from Shanghai Macklin Biochemical Technology Co., Ltd. with a purity of 98%. All other reagents utilized in this study were domestically produced and of analytical grade quality.
2.2. Methods
2.2.1. Laboratory bacteriostatic test of vanillin against Psa
The method described by Shi et al. (2005) was used with slight modifications to determine the in vitro antibacterial activity of vanillin against Psa using a paper disk diffusion assay. The size of the paper disk was 5 mm. Vanillin solutions were prepared at concentrations of 1.0, 5.0, 10.0, 15.0, and 20.0 mg·mL−1, and the paper disks were soaked in the respective solutions. Psa was cultured in liquid luria-bertani (LB) medium, and its bacterial density was adjusted to 1 × 108 CFU·mL−1. Then, 100 μL of the bacterial suspension was spread evenly onto luria-bertani agar plates. Sterilized distilled water was used as a negative control (CK) instead of vanillin. The soaked paper disks were positioned onto the surface of the LB agar plates that had been previously inoculated with Psa and subsequently incubated at 25°C for 24 h. The diameter of the inhibition zone was measured with a caliper using the cross-intersection method.
2.2.2. Determination of the minimum inhibitory concentration of vanillin against Psa
According to the study by Pei et al. (2022), a gradient dilution method was employed to determine the minimum inhibitory concentration of vanillin, with some modifications. Initially, an appropriate amount of liquid luria-bertani medium was taken from the logarithmic growth phase of Psa culture, and the bacterial concentration was adjusted to 1 × 108 CFU·mL−1. Subsequently, vanillin solutions of different concentrations, ranging from 0, 0.5, 1.0, 1.5, 2.0, 2.5, 3.0, 3.5, 4.0, 4.5, 5.0, 5.5, and 6.0 mg·mL−1, were added. After thorough mixing, the culture was incubated for 24 h at 25°C with agitation at 200 rpm. Following the guidelines of the Clinical and Laboratory Standards Institute (CLSI), the minimum inhibitory concentration was determined by visually examining the clarity of the liquid without agitation (CLSI, 2013). Subsequently, 100 μL of the culture medium was inoculated onto the agar plate and incubated at 25°C for 48 h to observe colony formation. The minimum bactericidal concentration (MBC) was determined based on the concentration at which no growth of colonies was observed.
2.2.3. Inhibition effect of vanillin on Psa on kiwifruit surface
Based on the experimental results outlined in Section 2.2.1, a starting concentration of 5.0 mg·mL−1 was selected to prepare a gradient of vanillin solutions at concentrations of 5.0, 6.0, 7.0, 8.0, 9.0, and 10.0 mg·mL−1. Fresh and undamaged kiwifruits of uniform size were randomly selected, with 10 kiwifruits per sample. The kiwifruits were treated with UV sterilization in a super-clean bench for 30 min to kill surface bacteria on the fruit. Psa (concentration of 1 × 108 CFU·mL−1) suspension was evenly sprayed onto the surface of the fruit using a micro-sprayer to ensure uniform bacterial adhesion. Sterile ddH2O was used for the control (CK1) and placed in a sterilized storage container. After 2 h, the vanillin solutions with concentrations of 5.0, 6.0, 7.0, 8.0, 9.0, and 10.0 mg·mL−1 were evenly sprayed onto the surface of the kiwifruit treated with bacterial spray using a micro-sprayer. Sterile ddH2O was used as a positive control (CK2). CK1 was treated with sterile ddH2O instead. Each treatment was set up with three replicates.
2.2.4. Analysis on the inhibition effect of vanillin solution on Psa of kiwifruit surface
With slight modifications to the method in Section 2.2.3, three fruits were randomly selected from each treatment, and a needle with a diameter of 1.6 mm was used to randomly sample the skin of the fruit to create small puncture wounds. The samples were immersed in 30 mL of liquid medium and subsequently incubated at a constant temperature of 25°C with shaking for 6 h. After dilution by 300 times, 100 μL of the solution was evenly spread onto an LB agar plate, and the number of single bacterial colonies was counted. This process was repeated three times. The inhibition rate was calculated using Formula 1.
2.2.5. Effect of vanillin on fruit quality of kiwifruit
Randomly selected kiwifruit fruits of similar size and shape, without any mechanical damage, were used as samples, with a sample size of 150 kiwifruits per group. The kiwifruit was placed in a 50 L container, completely immersed in a solution of 8.0 mg·mL−1 of vanillin for 20 min. Following the treatment, the fruit was removed and left to air dry before being stored at room temperature with a humidity level of 90%. The negative control group was treated with sterile distilled water instead of vanillin solution and processed in the same manner. Sampling was conducted once every 3 days, with 6 randomly selected fruits measured for each physiological parameter of interest. Each treatment was replicated 3 times.
2.2.6. Determination of physiological indicators
2.2.6.1. Determination of weight loss and rotting rate
Following the method of Pan et al. (2019), the fruit weight and the number of rotten fruits were counted every 3 days. The weight loss and the rotting rate were calculated according to Formulas 2, 3, respectively.
2.2.6.2. Determination of total soluble solids and titratable acidity
The ATAGO PAL-1 hand-held digital refractometer was used to measure the total soluble solids (TSS) of kiwifruit. Fruit pulp was squeezed to extract juice into a beaker, mixed thoroughly and measured, expressed as a percentage. Each sample was measured thrice, and the mean value was recorded. The acid–base titration method was utilized to determine the titratable acidity content (Gao, 2006), expressed as a percentage.
2.2.6.3. Determination of vitamin C and total sugar content
Vitamin C was determined by 2, 6-dichlorindophenol titration (Gao, 2006). Total sugar content was determined by Anthrone colorimetry (Gao, 2006).
2.2.6.4. Respiratory rate measurement
The FS-3080A fruit and vegetable respirometer was used to measure the respiratory rate, with a measurement temperature of 20°C and a gas flow rate of 1.0 L/min. Six fruits were measured at a time (approximately 0.4 kg), and the measurement time was 20 min, with three repetitions. Results were expressed in units of mg·kg−1·h−1 of CO2.
2.2.6.5. Determination of fruit firmness
The kiwifruit was peeled at the equator, and four points were randomly and evenly selected at the equator of the fruit for texture analysis using a texture analyzer (SMSTA XT Plus; StableMicro Systems Ltd., United Kingdom). A p/2 model probe, which had a diameter of 2 mm, was utilized for the test. The test parameters included a pre-test speed of 2 mm/s, a test speed of 2 mm/s, a post-test speed of 5 mm/s, a puncture depth of 8 mm, and a trigger force of 5 g, with the unit measured in N.
2.2.6.6. Determination of antioxidant enzyme activity
Superoxide dismutase (SOD), catalase (CAT), and peroxidase (POD) activities were evaluated using the SOD activity assay kit (BC0175), CAT activity assay kit (BC4785), and POD activity assay kit (BC009) respectively, provided by Beijing Solarbio Science and Technology Co., Ltd. Each assay was performed in triplicate, and the mean value was calculated.
2.3. Data processing
The data were statistically analyzed and plotted using Excel 2010 software. The collected data were analyzed using IBM SPSS Statistics 19.0 software and expressed as the mean ± standard deviation. The significance of differences (p < 0.05) was determined using a t-test.
3. Results and analysis
3.1. Laboratory bactericidal effect of vanillin on Psa
As shown in Figure 1, when the concentration of vanillin solution was 1.0 mg·mL−1, 5.0 mg·mL−1, 10.0 mg·mL−1, 15.0 mg·mL−1 and 20.0 mg·mL−1, the size of the inhibition zone was 0.00 ± 0.00 mm, 9.66 ± 0.46 mm, 13.53 ± 0.61 mm, 20.13 ± 1.41 mm, and 21.83 ± 1.64 mm, respectively, indicating that the inhibitory effect of vanillin on Psa was observed when the concentration of the vanillin solution was higher than 5.0 mg·mL−1.
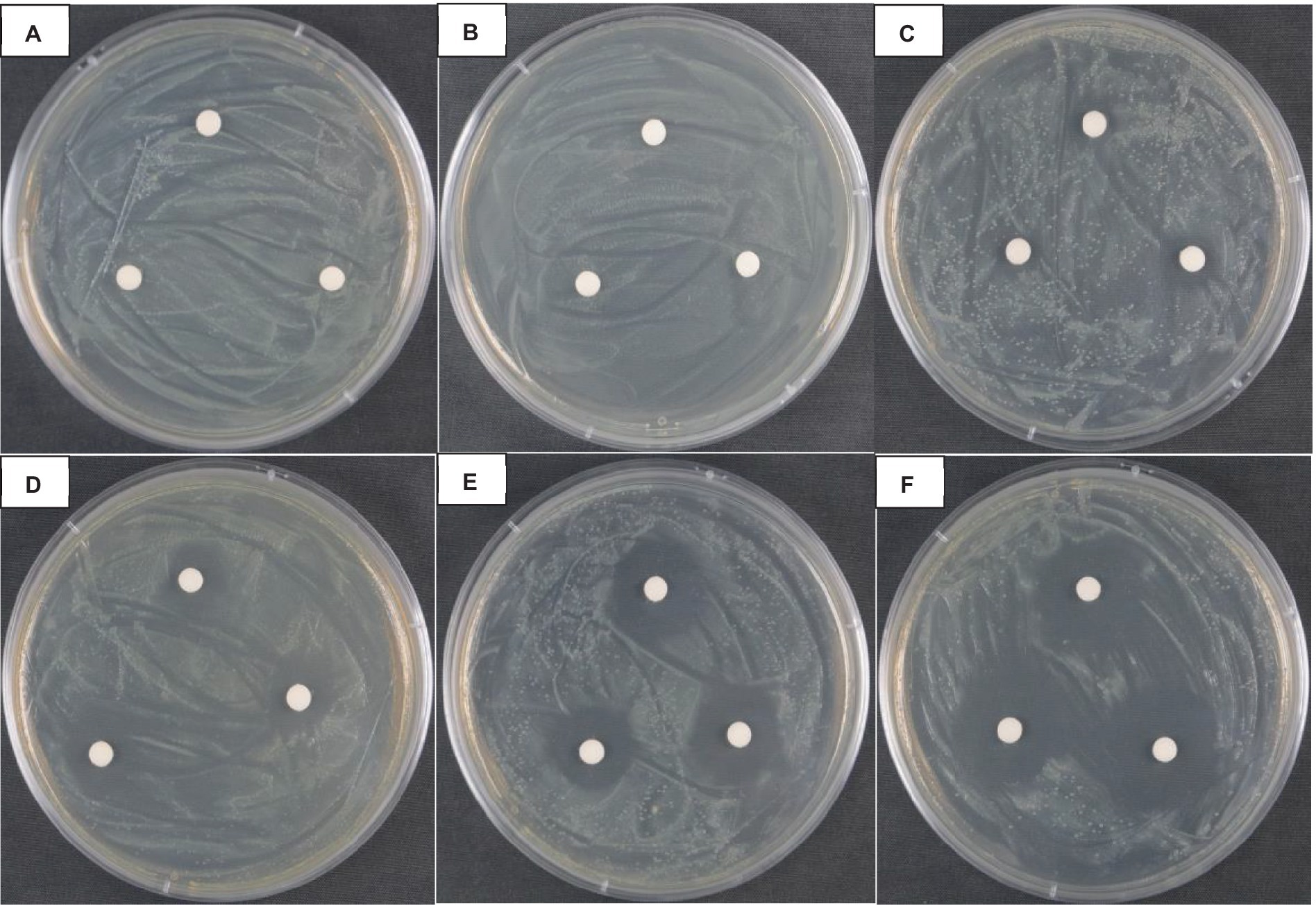
Figure 1. Effect of vanillin bactericidal on Psa. (A) ddH2O negative control; (B–F) The concentration of vanillin solution was respectively: 1.0 mg·mL−1, 5.0 mg·mL−1, 10.0 mg·mL−1, 15.0 mg·mL−1, and 20.0 mg·mL−1.
3.2. Assessment of the minimum inhibitory concentration and minimum bactericidal concentration values of vanillin against Psa
According to Table 1, it can be observed that different degrees of turbidity were observed in the medium when vanillin was administered at concentrations ranging from 0.0 mg·mL−1 to 3.5 mg·mL−1 against Psa. However, when the concentration was increased to 4.0 mg·mL−1 to 6.0 mg·mL−1, the medium became clear with no bacterial growth. Therefore, the minimum inhibitory concentration (MIC) of vanillin against Psa was determined to be 4.0 mg·mL−1. Results from plate coating experiments indicated that when the concentration of vanillin was 5.0 mg·mL−1 to 6.0 mg·mL−1, no bacterial colonies were observed when 100 μL of the culture medium was coated on the plate. In contrast, when the concentration was within the range of 0.0 mg·mL−1 to 4.5 mg·mL−1, bacterial colonies were present on the plate. Thus, the minimum bactericidal concentration (MBC) of vanillin against Psa was determined to be 5.0 mg·mL−1.
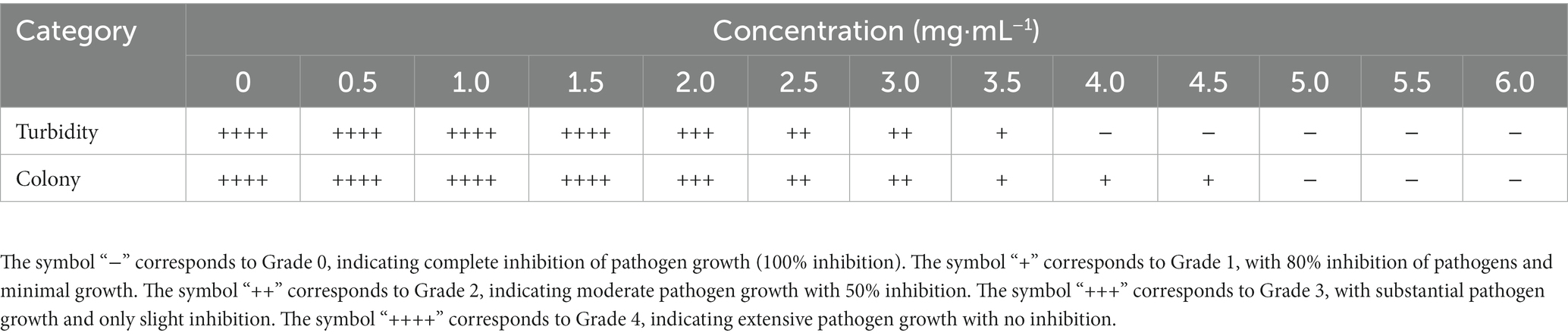
Table 1. Determination of the minimum inhibitory concentration (MIC) and minimum bactericidal concentration (MBC) of vanillin against Psa.
3.3. Effect of exogenous vanillin on the bactericidal effect of Psa
According to Figure 2, when the concentration of vanillin solution was 5.0, 6.0, 7.0, 8.0, 9.0, and 10.0 mg·mL−1, the colony forming units on the coated plate were 972.00 ± 79.49, 192.67 ± 8.18, 74.33 ± 6.80, 0.00 ± 0.00, 0.00 ± 0.00, and 0.00 ± 0.00, respectively. Figure 2A shows that the colony forming units in CK1 were 0, indicating no interference from other bacteria during the experiment; the positive control CK2 without vanillin treatment had a colony forming unit of 2,313 ± 158.12. According to Figure 3B, when the concentration of vanillin solution was 5.0, 6.0, 7.0, 8.0, 9.0, and 10.0 mg·mL−1, the bactericidal rates of Psa on the surface of kiwifruit were 61.29 ± 3.17%, 92.33 ± 0.33%, 96.79 ± 0.29%, 100.00 ± 0.00%, 100.00 ± 0.00%, and 100.00 ± 0.00%, respectively.
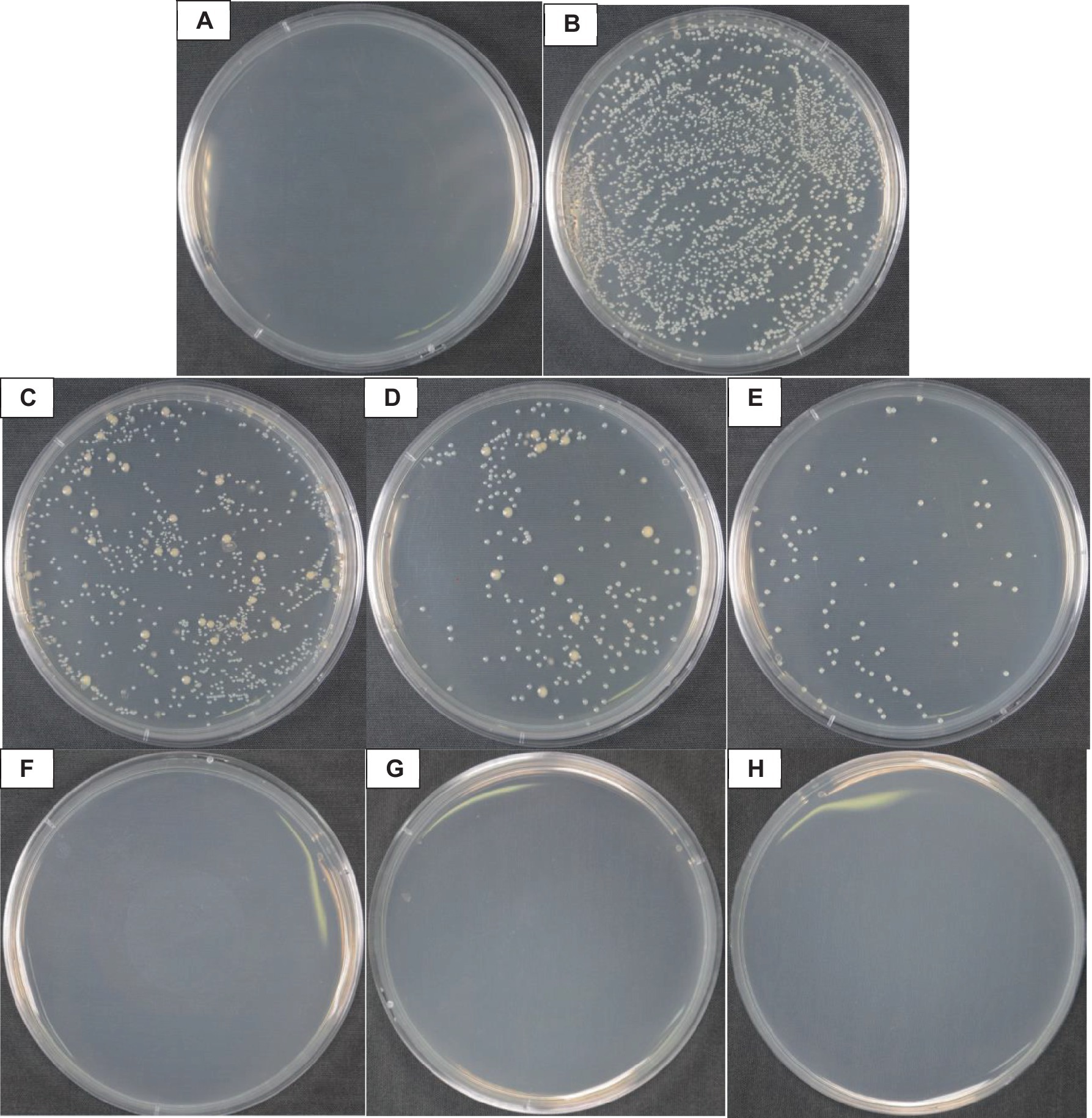
Figure 2. Effect of vanillin bactericidal on Psa of kiwifruit surface. (A) CK1; (B) CK2; (C–H) The concentration of vanillin solution was respectively: 5.0 mg·mL−1, 6.0 mg·mL−1, 7.0 mg·mL−1, 8.0 mg·mL−1, 9.0 mg·mL−1, and 10.0 mg·mL−1.
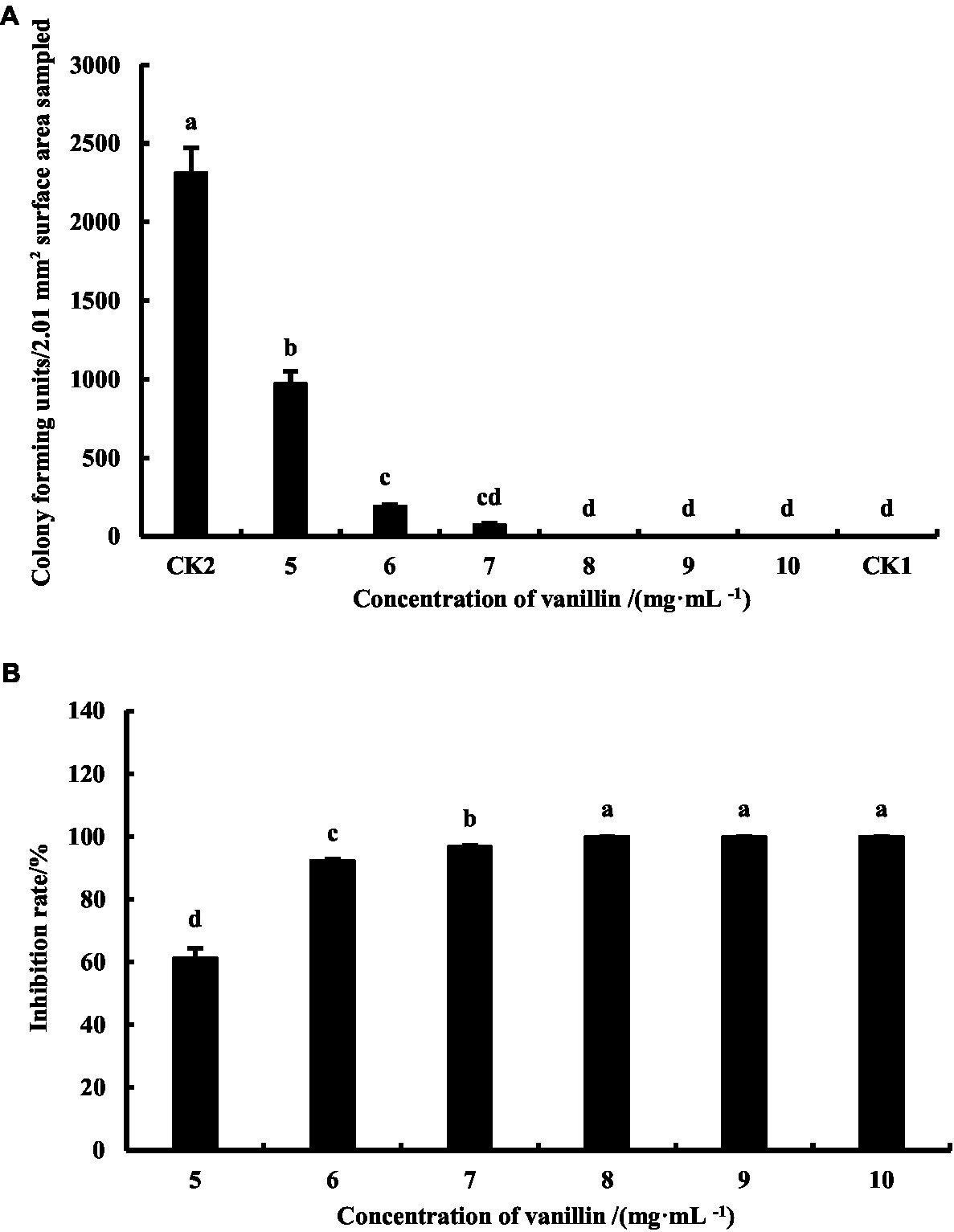
Figure 3. Colony forming units (A) and inhibition rate (B) with different concentrations of vanillin treating. Different lowercase letters indicate significant difference (p < 0.05).
3.4. Effects of exogenous vanillin on weight loss and rotting rate of kiwifruits
Figure 4A illustrates that both the treated and control groups experienced a considerable increase in fruit weight loss during storage. This outcome is attributed to the fruit’s respiration and transpiration processes, which result in the consumption of internal moisture. Over the storage duration, the treatment group displayed a relatively gradual and lower weight loss compared to the control group. A noticeable variation in weight loss between the treated and control groups was observed after day 6, with a significant difference (p < 0.05). The weight loss percentages of the treatment and control groups on day 18 were 1.23 and 1.47%, respectively. The above results reveal that soaking kiwifruit in a vanillin solution can effectively inhibit moisture loss in the fruit, thereby inhibiting the increase in kiwifruit weight loss.
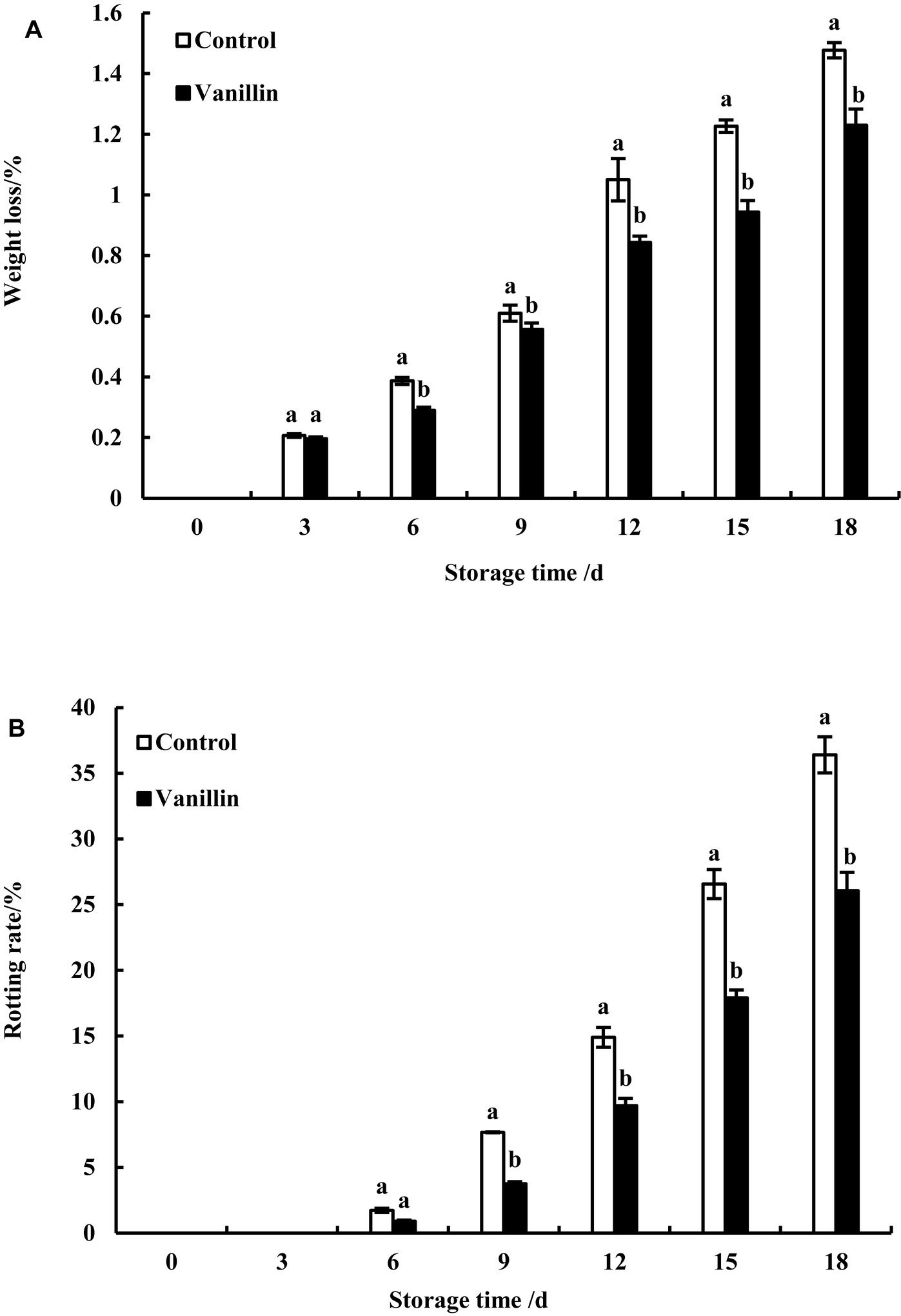
Figure 4. Effect of exogenous vanillin on the weight loss (A) and rotting rate (B) of kiwifruit. Significant differences (p < 0.05) between values within the same column are indicated by different lowercase letters.
Although both the treated and control groups had rotten fruits after the 6th day of storage, as depicted in Figure 4B, there was no significant difference observed between them. The rotting rate of both groups increased rapidly with the extension of the storage period. However, the rotting rate of the treatment group was lower than that of the control group, with significant differences observed (p < 0.05). The highest rotting rate was observed on the 18th day, with a rotting rate of 26.06% in the treatment group and 36.40% in the control group. The main cause of rotten kiwifruit during storage is the susceptibility to external microbial invasion. The lower rotting rate in the treatment group may be related to its antibacterial effect. These results indicate that the use of vanillin solution to soak kiwifruit can effectively inhibit the increase of rotting rate.
3.5. Effects of exogenous vanillin on the nutritional quality of kiwifruit
The taste, flavor, and nutritional quality of kiwifruit are predominantly determined by the levels of TSS, TA, Vc, and TSC. The increase in the TSS of kiwifruit during storage might be attributed to the breakdown of carbohydrates into simple sugars and glucose. As presented in Table 2, the TSS content of kiwifruit increased progressively with the extension of storage time, in accordance with the traits of post-ripening fruits. The growth rate of TSS in fruits was fast during the period of 0–9 days, and slow after 9 days. The TSS content of kiwifruit treated with vanillin was considerably higher throughout the entire storage duration compared to the control group, with significant differences observed (p < 0.05). The findings suggest that treating kiwifruit with vanillin can lead to a substantial improvement in the TSS content and nutritional quality of the fruit.
TA is one of the important quality traits of fruit and a significant factor affecting fruit flavor quality. The decrease in the TA mass fraction of kiwifruit was generally observed with the increase in storage time, as depicted in Table 2. On the 18th day of storage, a notable difference (p < 0.05) was observed in the TA content between the control and treatment groups, where the former declined to 1.11%, and the latter to 1.16%. In the remaining time periods, no significant difference (p > 0.05) was observed between the two groups. The above results indicate that the effect of vanillin treatment on the TA content of kiwifruit is not significant, indicating a good retention effect.
The abundance of Vc content in kiwifruit is remarkable and considered to be one of the most critical quality characteristics of the fruit, with its concentration being several times or even tens of times higher than that in fruits like citrus or apple. The Vc content in kiwifruit followed an increasing-decreasing-increasing pattern during storage, as depicted in Table 2. However, the overall magnitude of change was relatively small. The Vc content in kiwifruit treated with vanillin solution did not significantly vary from the control group, except for the 3rd and 15th day of storage (p > 0.05). This suggests that vanillin treatment has a beneficial effect in maintaining and preserving the Vc content of kiwifruit.
According to Table 2, the TSC content of kiwifruit increased during storage, indicating that the sugar content of kiwifruit continuously accumulated and the fruit maturity increased. The TSC content in kiwifruit treated with vanillin was consistently lower than the control group during the entire storage period. No significant difference was observed between the two groups during the initial 0–9 days period (p > 0.05); however, from day 12 to 18, a significant difference was detected (p < 0.05), suggesting that vanillin treatment can effectively delay the production of TSC during the late storage period and consequently delay the ripening and senescence of kiwifruit.
3.6. Effects of exogenous vanillin on respiration and firmness of kiwifruit
As depicted in Figure 5A, vanillin treatment effectively inhibited the respiration rate of kiwifruit, resulting in significantly lower respiration compared to the control group. Exhibited a gradual increase, peaking at the 6th day, with the control group showing 1.32 times higher respiration than the treatment group. Subsequently, the respiration gradually decreased and then increased, with a second peak appearing on the 12th day for the treatment group and on the 15th day for the control group. During the entire storage period, the respiration rate of the treatment group was consistently lower than that of the control group. After the 6th day, the respiration rate of the treatment group exhibited a significant reduction compared to the control group (p < 0.05). Respiration can accelerate fruit softening and ripening, and the above results indicate that vanillin can delay fruit senescence.
As illustrated in Figure 5B, while the firmness of both the control group and kiwifruit treated with vanillin decreased throughout the storage period, the latter group exhibited significantly higher firmness compared to the former (p < 0.05). Firmness showed a significant difference between the treated and control groups during the storage period from day 3 to day 18 (p < 0.05). After 18 days of storage, the firmness of kiwifruit in the vanillin-treated group was 2.67 times higher than that of the control group, with values of 0.8 N and 0.3 N, respectively. These findings suggest that the application of vanillin can effectively preserve the firmness of kiwifruit and prevent fruit softening.
3.7. Investigating the impact of exogenous vanillin on the activities of antioxidant enzymes in kiwifruit
The main function of superoxide dismutase (SOD) is to facilitate the dismutation of superoxide anions (O2−) into H2O2 and O2, playing an important role in the body’s antioxidant and anti-aging functions (Cai et al., 2018). According to Figure 6A, the application of external vanillin led to an increase in SOD activity, reaching its highest peak on the 9th day at 840.72 U/g, and then decreased, which was 16.37% higher than the control group with 722.46 U/g. Throughout the 0–18 day storage period, the vanillin-treated group exhibited significantly higher SOD activity compared to the control group (p < 0.05), indicating that vanillin treatment can effectively increase the activity of SOD, maintain O2− metabolism balance, reduce O2− damage to kiwifruit, and thus extend its storage performance.
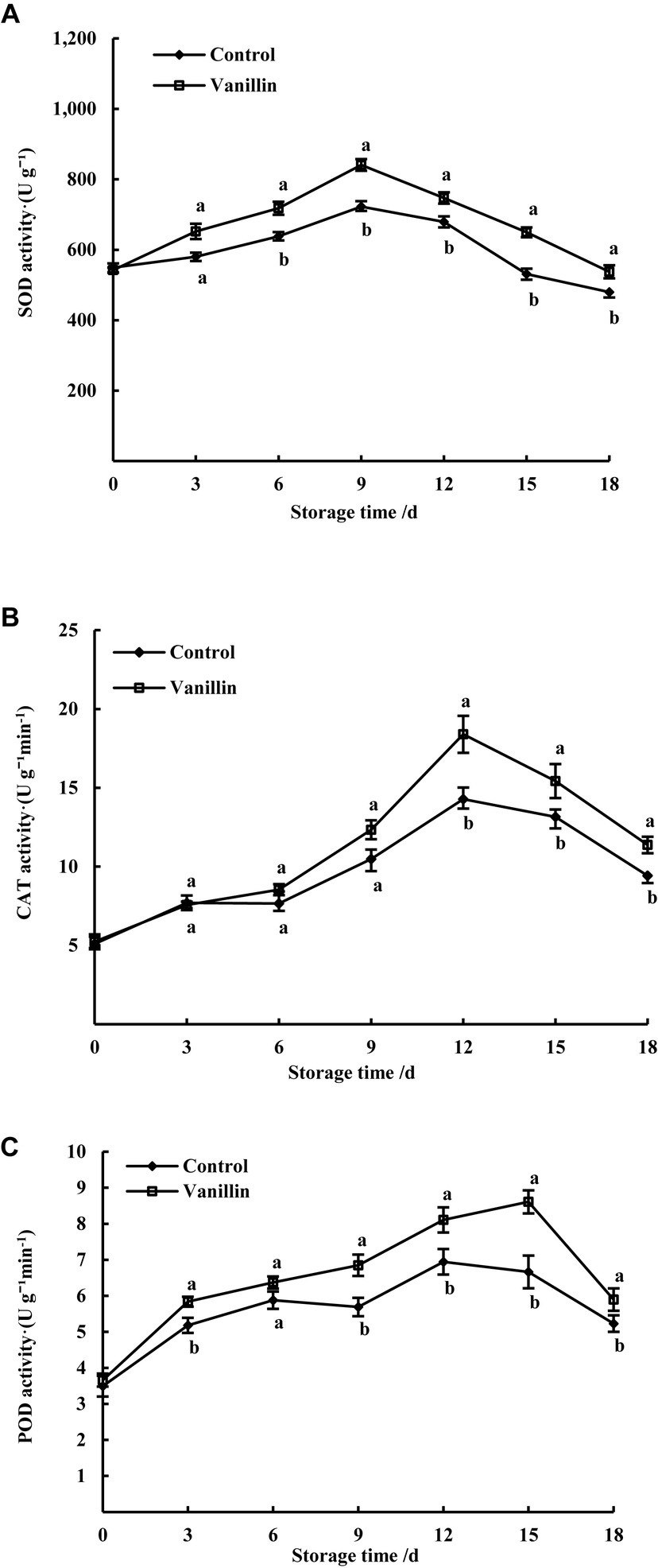
Figure 6. Effects of exogenous vanillin on antioxidant enzyme activity of kiwifruit. (A) SOD activity; (B) CAT activity; (C) POD activity.
Catalase (CAT) is an antioxidant enzyme that plays a crucial role in protecting plant cells from oxidative stress by catalyzing the decomposition of hydrogen peroxide (H2O2) into O2 and H2O, thereby reducing oxidative damage caused by H2O2 (Liu et al., 2016). As illustrated in Figure 6B, the kiwifruit’s CAT content exhibited an initial increase followed by a decrease throughout the entire storage period. Additionally, the vanillin-treated group had a higher CAT content than the control group at various time intervals. Both the treatment and control groups reached their highest values on day 12, with values of 18.36 U/g and 14.26 U/g, respectively. The CAT content in the vanillin-treated group was found to be 28.75% higher than the control group. The CAT content in the kiwifruit treated with vanillin did not show a significant difference compared to the control group on days 3, 6, and 9 of storage (p > 0.05). After day 12, the CAT content in kiwifruit in both groups decreased, with a significant difference in CAT content between the two groups (p < 0.05), indicating that vanillin treatment can increase the CAT content in kiwifruit, effectively clearing the accumulated H2O2 in the fruit, and prolonging the storage time and quality of the fruit.
Peroxidase (POD) can catalyze various oxidation reactions involving hydrogen peroxide to maintain the balance of oxidation–reduction reactions (Luo et al., 2015). There was an initial increase followed by a decrease in POD activity in both the vanillin-treated and control groups during storage, as depicted in Figure 6C. The vanillin-treated group exhibited significantly higher POD activity than the control group, except for day 6 (p < 0.05). Day 15 was when the vanillin-treated group had the highest activity of 8.60 U/g, surpassing the control group’s peak activity of 6.94 U/g observed on day 12. Vanillin treatment led to a 23.92% increase in POD activity in the fruit, which is significantly higher than the POD activity observed in the control group, demonstrating the positive effect of vanillin on the fruit’s antioxidant capacity.
4. Discussion
Although various copper compounds and antibiotics are widely used in China and abroad to prevent and control Psa, long-term and excessive use can lead to the development of drug resistance in the pathogen and also affect the safety and quality of fruit. Thus, the development of safe, efficient, and plant-based Psa bactericides has become an urgent priority, considering the challenges posed by conventional bactericides (Lin et al., 2020). Plant polyphenols, because of their diverse types, non-polluting nature, and absence of pesticide residue, are an effective alternative to copper-based and antibiotic pesticides (Pei et al., 2022). Previous studies have shown that plant polyphenols can enhance the antioxidant capacity of horticultural products, thus achieving the goal of controlling postharvest fruit diseases (Valverde et al., 2015; Chen et al., 2019). Kiwifruit is a climacteric fruit, and various factors such as postharvest diseases accelerate fruit ripening and senescence, leading to rapid decline in fruit quality during storage and shelf life. Therefore, there is an urgent need for safe and efficient preservatives to control postharvest diseases in kiwifruit. However, there are currently almost no reports on the antimicrobial effects of vanillin on Psa in postharvest kiwifruit, nor any reports on the impact of vanillin on fruit quality during the storage period of postharvest kiwifruit. In this study, it was shown that treatment with an 8.0 mg·mL−1 solution of vanillin on the surface of kiwifruit for 12 h resulted in a 100% killing rate of Psa on the surface of the fruit. Furthermore, vanillin can also regulate the postharvest physiology and quality of kiwifruit, enhancing fruit storage performance and preservation effects.
Not only does vanillin contain an aldehyde group, but it also possesses a phenol structure with eight carbon atoms, indicating its strong antibacterial activity (Lv et al., 2015). The main targets of vanillin’s antibacterial activity are primarily located at three sites: acting on cell membranes to disrupt their integrity, acting on enzymes to deactivate essential enzymes, and acting on DNA to render them inactive or structurally damaged (Fitzgerald et al., 2004). Vanillin exhibits broad-spectrum antibacterial effects and has been applied in the preservation and storage of fruits such as papaya, pineapple, cantaloupe, and apple (Rupasinghe et al., 2006; Sangsuwan et al., 2008; Wang et al., 2012). Studies by Moon et al. (2006) have demonstrated that the antibacterial activity of vanillin in apple juice is influenced by its concentration and pH value, with higher vanillin concentrations and lower pH values favoring increased antibacterial activity. Additionally, the antibacterial effects of vanillin vary among different bacterial species, with better efficacy against Escherichia coli compared to other strains. In vitro antibacterial assays have shown that the antibacterial efficacy of vanillin against Psa is concentration-dependent, with higher concentrations of vanillin enhancing its antibacterial effects (Figures 1, 3). This study indicates that when the concentration of vanillin is 8.0 mg·mL−1, it achieves a 100% bactericidal effect against Psa on the surface of kiwifruit, suggesting a potential correlation between the targets of vanillin’s antibacterial action and its effectiveness.
The storage properties and preservation effects of fruits are mainly evaluated through indicators of post-harvest physiology and quality changes (Xue et al., 2015). It is widely believed that important quality attributes affecting consumer acceptance of kiwifruit include fruit weight, hardness, color, aroma, and sweetness, etc. This study demonstrates that compared to the control, kiwifruit treated with vanillin showed a significant reduction in weight loss rate (Figure 4A) and decay rate (Figure 4B), inhibited the increase in respiration rate (Figure 5A), and improved fruit hardness (Figure 5B), thus delaying the ripening and senescence of kiwifruit after harvest and enhancing its storage tolerance. We believe that the vanillin-treated kiwifruit forms a thin layer of vanillin coating on the fruit surface, which enhances the structural integrity of the fruit surface, restricts the spread of pathogens, and helps maintain cell wall integrity against fungal attacks, thereby slowing down pathogenic infections and maintaining a lower decay rate. Respiration rate is an important parameter for evaluating postharvest metabolism and fruit quality. We speculate that the thin layer formed after vanillin treatment inhibits the respiration rate of fruit by blocking the stomata on the fruit peel and reducing water vapor and gas exchange, thus maintaining fruit hardness, restraining the increase in weight loss rate, and consequently delaying the postharvest senescence of kiwifruit. Previous studies using exogenous methyl jasmonate (MeJA) (Pan et al., 2019), citral (Wei et al., 2021), 2,4-epibrassinolide (Wang et al., 2020), and other treatments on kiwifruit have shown similar results, with reduced weight loss rate, decay rate, and controlled increase in respiration intensity, thereby extending fruit storage.
The process of plant maturation is considered as an oxidative phenomenon accompanied by reactive oxygen species (ROS) load (Xia et al., 2016). High levels of ROS can lead to oxidative stress-mediated damage in plant tissues. This study suggests that treatment with vanillin in kiwifruit resulted in lower O2− generation rates, indicating that vanillin treatment can protect kiwifruit from ROS-mediated oxidative damage. The elimination of ROS is related to the activity of antioxidant enzymes such as SOD, CAT, and POD in fruits and vegetables (Goffi et al., 2020). SOD plays a key role in the conversion of O2− to H2O2, while CAT clears H2O2, making CAT indispensable for detoxifying ROS (Zhu et al., 2014). POD is a key enzyme in the synthesis and oxidation of phenolic compounds (Foyer et al., 1994). Therefore, higher antioxidant enzyme activity and their synergistic effects may be potential mechanisms for reducing lipid peroxidation and delaying aging in fruit. Exogenous treatment with vanillin helps induce increased SOD, POD, and CAT activity in kiwifruit, thereby reducing oxidative damage and enhancing fruit storage performance.
Some studies have also reported an inseparable relationship between the content of phenolic compounds and the antioxidant capacity of postharvest fruits. Jin et al. (2012) found that yangmei (Myrica rubra) treated with linalool and cinnamaldehyde exhibited higher antioxidant capacity, primarily associated with higher phenolic compound content. High levels of antioxidants can delay physiological deterioration, thus slowing down the senescence of fruits. Tai et al. (2011) evaluated the antioxidant properties of vanillin using various methods and found that vanillin exhibited stronger antioxidant activity compared to vitamin C (Vc) and water-soluble Trolox (vitamin E analog). This indicates that vanillin also possesses a higher level of DPPH radical scavenging ability, thereby reducing damage caused by ROS. Therefore, the research results suggest that vanillin treatment effectively induces antioxidant enzyme activity, helping to balance the levels of ROS in kiwifruit. The interaction among these antioxidant enzymes triggers higher antioxidant activity, thereby maintaining the quality of postharvest kiwifruit and extending its storage time.
In summary, treatment of “Cuiyu” kiwifruit with vanillin at a concentration of 8.0 mg·mL−1 not only eliminates Psa on the fruit surface but also maintains the nutritional quality and commercial value of the fruit. Vanillin, as a secondary metabolite in plants, is a natural phenolic compound with safe and non-toxic biological characteristics. Taking advantage of this, we can explore its potential as a novel fruit preservative. The next step will focus on studying the antibacterial mechanism of vanillin against Psa, optimizing the optimal treatment concentration and duration of vanillin, investigating the synergistic antibacterial effects of vanillin in combination with other substances, and assessing the impact of vanillin on the quality of kiwifruit during cold storage.
Data availability statement
The original contributions presented in the study are included in the article/supplementary material, further inquiries can be directed to the corresponding authors.
Author contributions
WLL, WL, and CY contributed to the motivation, the interpretation of the methods, the data analysis and results, and provided the draft versions and revised versions, references. TH, FL, and KH provided the data and results. BG, FX, and SY helped with the revised versions and references. FB, FT, and JC provided the related concepts and minor recommendations, and extracted the conclusion and discussion. All authors contributed to the article and approved the submitted version.
Funding
This project was supported by Natural Science Foundation of Hunan Province, No. 2020JJ4050; Fruit plant protection position of Hunan Fruit Industry Technology System in 2022 and 2023; National citrus industry technology system, kiwifruit Changsha comprehensive experimental station.
Conflict of interest
The authors declare that the research was conducted in the absence of any commercial or financial relationships that could be construed as a potential conflict of interest.
Publisher’s note
All claims expressed in this article are solely those of the authors and do not necessarily represent those of their affiliated organizations, or those of the publisher, the editors and the reviewers. Any product that may be evaluated in this article, or claim that may be made by its manufacturer, is not guaranteed or endorsed by the publisher.
References
Barrett-Manako, K., Andersen, M. T., Martínez-Sánchez, M., Jenkins, H., Hunter, S., Reese-George, J., et al. (2021). Real-time PCR and droplet digital PCR are accurate and reliable methods to quantify Pseudomonas syringae pv. actinidiae biovar 3 in kiwifruit infected plantlets. Plant Dis. 105, 1748–1757. doi: 10.1094/PDIS-08-20-1703-RE
Cai, J., Zhao, X., Zhou, J., Xiao, T., and Zhang, M. (2018). Effects of 1-MCP and micro-porous films on quality of sweet potato leaves. Food Ferment. Ind. 44, 199–206. doi: 10.13995/j.cnki.11-1802/ts.016025
Cao, F., Gao, G. T., Wang, D., Lei, Y. S., and Zhao, W. Q. (2019). Sterilization of chlorine dioxide on Pseudomonas syringae pv. actinidiae of kiwifruit surface sterilization and its effect on the shelf life of fruits. J. Nucl. Agric. Sci. 33, 88–95. doi: 10.11869/j.issn.100-8551.2019.01.0088
Chen, C., Cai, N., Chen, J., and Wan, C. (2019). Clove essential oil as an alternative approach to control postharvest blue mold caused by Penicillium italicum in citrus fruit. Biomol. Ther. 9:197. doi: 10.3390/biom9050197
Chen, P., Li, S., Yan, X., Wang, N., Yu, L., and Liang, N. (2014). Progress on mechanism of antimicrobial action from vanillin. Prog. Microbiol. Immunol. 42, 50–53. doi: 10.13309/j.cnki.pmi.2014.02.012
Cheng, X., Yang, Y., Zhu, X., Yuan, P., Gong, B., Ding, S., et al. (2022). Inhibitory mechanisms of cinnamic acid on the growth of Geotrichum citri-aurantii. Food Control 131:108459. doi: 10.1016/j.foodcont.2021.108459
CLSI (2013). Performance standards for antimicrobial susceptibility testing; twenty-third informational supplement. CLSI Doc. M100-S23 33, 142–159.
Donati, I., Cellini, A., Sangiorgio, D., Vanneste, J. L., Scortichini, M., Balestra, G. M., et al. (2020). Pseudomonas syringae pv actinidiae: ecology, infection dynamics and disease epidemiology. Microb. Ecol. 80, 81–102. doi: 10.1007/s00248-019-01459-8
Dróżdż, P., and Pyrzynska, K. (2018). Assessment of polyphenol content and antioxidant activity of oak bark extracts. Eur. J. Wood Wood Prod. 76, 793–795. doi: 10.1007/s00107-017-1280-x
Fitzgerald, D. J., Stratford, M., Gasson, M. J., Ueckert, J., Bos, A., and Narbad, A. (2004). Mode of antimicrobial action of vanillin against Escherichia coli, lactobacillus plantarum and Listeria innocua. J. Appl. Microbiol. 97, 104–113. doi: 10.1111/j.1365-2672.2004.02275.x
Foyer, C. H., Descourvieres, P., and Kunert, K. J. (1994). Protection against oxygen radicals: an important defence mechanism studied in transgenic plants. Plant Cell Environ. 17, 507–523. doi: 10.1111/j.1365-3040.1994.tb00146.x
Gallelli, A., Talocci, S., L’aurora, A., and Loreti, S. (2011). Detection of Pseudomonas syringae pv. actinidiae, causal agent of bacterial canker of kiwifruit, from symptomless fruits and twigs, and from pollen. Phytopathol. Mediterr. 50, 462–472. doi: 10.14601/Phytopathol_Mediterr-10039
Gao, Y., Ma, S., Dai, M., Wang, M., and Feng, X. (2018). Progress in research on the biosynthesis pathway and metabolic regulation of phenolic acids. Food Sci. 39, 286–293. doi: 10.7506/spkx1002-6630-201809043
Goffi, V., Magri, A., Botondi, R., and Petriccione, M. (2020). Response of antioxidant system to postharvest ozone treatment in “Soreli” kiwifruit. J. Food Qual. 100, 961–968. doi: 10.1002/jsfa.10055
Jia, R., Cai, D., Ge, S., Ren, S., and Liu, J. (2021). Antibacterial activity and mechanism of polyphenol extracts from adzuki bean seed coat against two pathogens. Food Sci. 42, 64–71. doi: 10.7506/spkx1002-6630-20201202-035
Jin, P., Wu, X., Xu, F., Wang, X., Wang, J., and Zheng, Y. (2012). Enhancing antioxidant capacity and reducing decay of Chinese bayberries by essential oils. J. Agric. Food Chem. 60, 3769–3775. doi: 10.1021/jf300151n
Li, X., Long, Y., Yin, X., Wu, X., Zhao, Z., Fan, R., et al. (2019). Mechanism of action of methyl jasmonate against kiwifruit soft rot and its effect on fruit quality. Food Sci. 40, 108248–108459. doi: 10.7506/spkx1002-6630-20180625-467
Li, T., Sun, X., Chen, H., He, B., Mei, Y., Wang, D., et al. (2020). Effect of the combination of vanillin and chitosan coating on the microbial diversity and shelf-life of refrigerated turbot filets. Front. Microbiol. 11, 462–472. doi: 10.3389/fmicb.2020.00462
Li, Y., Wang, X., Zeng, Y., and Liu, P. (2020). Metabolic profiling reveals local and systemic responses of kiwifruit to Pseudomonas syringae pv. actinidiae. Plant Direct 4, 1–13. doi: 10.1002/pld3.297
Lin, W., Yin, C., Liu, F., Zhang, Y., Tang, J., and Bu, F. (2020). Cloning and analysis of the copper resistance gene CopB associated with Pseudomonas syringae pv. actinidae. J. Cent. South Univ. For. Technol. 40, 119–126. doi: 10.14067/j.cnki.1673-923x.2020.07.015
Liu, Y., Yang, X., Zhu, S., and Wang, Y. (2016). Postharvest application of MeJA and NO reduced chilling injury in cucumber (Cucumis sativus) through inhibition of H2O2 accumulation. Postharvest Biol. Technol. 119, 77–83. doi: 10.1016/j.postharvbio.2016.04.003
Luo, S., Hu, H., Chen, X., and Li, X. (2015). Effect of modified atmosphere packaging on storage quality and activities of antioxidant enzymes of sword bean. Food Sci. 36, 260–265. doi: 10.7506/spkx1002-6630-201522049
Lv, J., Song, S., Feng, X., Zhu, D., Liang, J., Hou, Y., et al. (2015). Advances in application and research of vanillin in food storage and preservation. Food Sci. 36, 305–309. doi: 10.7506/spkx1002-6630-201517056
Meng, Q., Li, J., Wang, C., and Shan, A. (2023). Biological function of resveratrol and its application in animal production: a review. J. Anim. Sci. Biotechnol. 14:25. doi: 10.1186/s40104-022-00822-z
Moon, K. D., Delaquis, P., Toivonen, P., and Stanich, K. (2006). Effect of vanillin on the fate of listeria monocytogenes and Escherichia coli O157: H7 in a model apple juice medium and in apple juice. Food Microbiol. 23, 169–174. doi: 10.1016/j.fm.2005.02.005
Pan, L., Zhao, X., Chen, M., Fu, Y., and Xiang, M. (2019). Effects of exogenous methyl jasmonate on fruit qualities and antioxidases activities of postharvest kiwifruits. Food Ferment. Ind. 45, 190–196. doi: 10.13995/j.cnki.11-1802/ts.019568
Pei, H. R., Lu, M. X., Long, L., and Long, Z. F. (2022). Primary antibacterial mechanism of ethanol extract of Scutellaria baicalensis against the pathogens of kiwifruit canker. J. South. Agric. 53, 477–485. doi: 10.3969/j.issn.2095-1191.2022.02.021
Pei, X., Tekliye, M., and Dong, M. (2021). Isolation and identification of fungi found in contaminated fermented milk and antifungal activity of vanillin. Food Sci. Human Wellness 10, 214–220. doi: 10.1016/j.fshw.2021.02.011
Rupasinghe, H. P. V., Boulter-Bitzer, J., Ahn, T., and Odumeru, J. A. (2006). Vanillin inhibits pathogenic and spoilage microorganisms in vitro and aerobic microbial growth in fresh-cut apples. Food Res. Int. 39, 575–580. doi: 10.1016/j.foodres.2005.11.005
Safari, Z., Ding, P., Juju Nakasha, J., and Yusoff, S. F. (2020). Combining chitosan and vanillin to retain postharvest quality of tomato fruit during ambient temperature storage. Coatings 10:1222. doi: 10.3390/coatings10121222
Sangsuwan, J., Rattanapanone, N., and Rachtanapun, P. (2008). Effect of chitosan/methyl cellulose films on microbial and quality characteristics of fresh-cut cantaloupe and pineapple. Postharvest Biol. Technol. 49, 403–410. doi: 10.1016/j.postharvbio.2008.02.014
Shi, Y., Chen, Q., Wang, Q., Song, K., and Qiu, L. (2005). Inhibitory effects of cinnamic acid and its derivatives on the diphenolase activity of mushroom tyrosine. Food Chem. 92, 707–712. doi: 10.1016/j.foodchem.2004.08.031
Stefani, E., and Giovanardi, D. (2011). Dissemination of Pseudomonas syringae pv. actinidiae through pollen and its epiphytic life on leaves and fruits. Phytopathol. Mediterr. 50, 489–496. doi: 10.1016/j.phymed.2011.09.068
Tai, A., Sawano, T., Yazama, F., and Ito, H. (2011). Evaluation of antioxidant activity of vanillin by using multiple antioxidant assays. Biochim. Biophys. Acta Gen. Subj. 1810, 170–177. doi: 10.1016/j.bbagen.2010.11.004
Valverde, J. M., Giménez, M. J., Guillén, F., Valero, D., Martínez-Romero, D., and Serrano, M. (2015). Methyl salicylate treatments of sweet cherry trees increase antioxidant systems in fruit at harvest and during storage. Postharvest Biol. Technol. 109, 106–113. doi: 10.1016/j.postharvbio.2015.06.011
Vanneste, J. L., Cornish, D. A., Yu, J., and Stokes, C. A. (2014). First report of Pseudomonas syringae pv. actinidiae the causal agent of bacterial canker of kiwifruit on a Actinidia arguta vines in New Zealand. Plant Dis. 98:418. doi: 10.1094/PDIS-06-13-0667-PDN
Wang, J., Chen, P. S., and Meng, X. C. (2012). Effects of compound coating combined with ultraviolet treatment on quality of cold storage fresh-cut papaya. Trans. CSAE 28, 273–278. doi: 10.3969/j.issn.1002-6819.2012.02.047
Wang, X., Lu, Z., Su, J., Li, Y., Cao, M., and Gao, H. (2020). 24-Epibrassinolide delays senescence in harvested kiwifruit through effects on mitochondrial membrane and antioxidant activity. LWT 118:108833. doi: 10.1016/j.lwt.2019.108833
Wei, L., Chen, C., Wan, C., Chen, M., and Chen, J. (2021). Citral delays postharvest senescence of kiwifruit by enhancing antioxidant capacity under cold storage. J. Food Qual. 2021, 1–9. doi: 10.1155/2021/6684172
Wei, Z., Guo, M., Wang, Y., Duan, Z., Yang, K., and Luan, X. (2021). Recent advances in research on polyphenol compounds in Camellia oleifera seed oil. Food Sci. 42, 311–320. doi: 10.7506/spkx1002-6630-20200301-009
Xia, Y., Chen, T., Qin, G., Li, B., and Tian, S. (2016). Synergistic action of antioxidative systems contributes to the alleviation of senescence in kiwifruit. Postharvest Biol. Technol. 111, 15–24. doi: 10.1016/j.postharvbio.2015.07.026
Xue, M., Gao, G., Zhang, S., Wei, X., and Gen, P. (2015). Effects of chlorine dioxide gas on post-harvest physiology and storage quality of “Huayou” kiwifruit. Food Sci. 36, 257–261. doi: 10.7506/spkx1002-6630-20181130-367
Zabka, M., and Pavela, R. (2013). Antifungal efficacy of some natural phenolic compounds against significant pathogenic and toxinogenic filamentous fungi. Chemosphere 93, 1051–1056. doi: 10.1016/j.chemosphere.2013.05.076
Zhu, D., Cao, F., Gao, G., Wang, D., Xu, Y., Du, Y., et al. (2017). Research of rapid detection on Pseudomonas syringae pv. actinidiae from the surface of kiwifruit during the storage period. J. Nucl. Agric. Sci. 31, 0493–0499. doi: 10.11869/j.issn.100-8551.2017.03.0493
Keywords: vanillin, kiwifruit, Pseudomonas syringae pv. actinidae, fruit quality, kiwifruit surface
Citation: Lin W, Li W, Huang T, Yin C, Liu F, Gong B, He K, Xiao F, Yang S, Bu F, Tang F and Cao J (2023) Inhibitory effect of vanillin on Pseudomonas syringae pv. actinidiae of kiwifruit surface and its effect on the quality of fruits. Front. Sustain. Food Syst. 7:1204442. doi: 10.3389/fsufs.2023.1204442
Edited by:
Ravinder K. Goyal, Agriculture and Agri-Food Canada (AAFC), CanadaReviewed by:
Xiuxiu Sun, Agricultural Research Service (USDA), United StatesLili Liu, Hunan University of Science and Technology, China
Liu Shuang-Qing, Hunan Agricultural University, China
Copyright © 2023 Lin, Li, Huang, Yin, Liu, Gong, He, Xiao, Yang, Bu, Tang and Cao. This is an open-access article distributed under the terms of the Creative Commons Attribution License (CC BY). The use, distribution or reproduction in other forums is permitted, provided the original author(s) and the copyright owner(s) are credited and that the original publication in this journal is cited, in accordance with accepted academic practice. No use, distribution or reproduction is permitted which does not comply with these terms.
*Correspondence: Wen Li, VDIwMjAyNTMyQGNzdWZ0LmVkdS5jbg==; Chunfeng Yin, MzY5NzA2NjY3QHFxLmNvbQ==