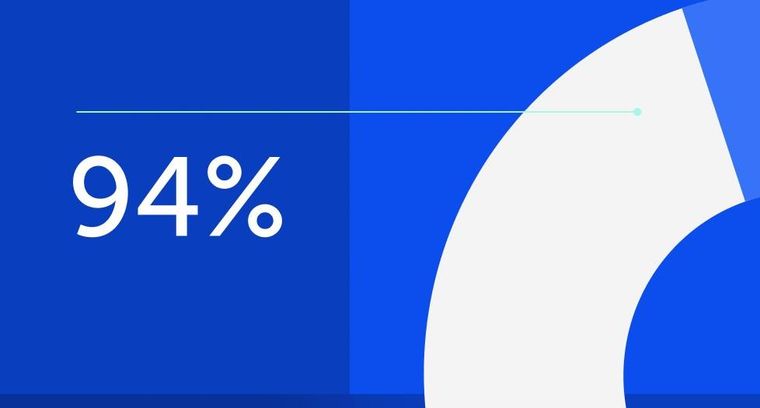
94% of researchers rate our articles as excellent or good
Learn more about the work of our research integrity team to safeguard the quality of each article we publish.
Find out more
ORIGINAL RESEARCH article
Front. Sustain. Food Syst., 20 July 2023
Sec. Crop Biology and Sustainability
Volume 7 - 2023 | https://doi.org/10.3389/fsufs.2023.1204293
This article is part of the Research TopicSpeed Breeding Systems For FoodView all 12 articles
Introduction: Soybean breeding in southwestern China has vastly improved soybean yields with the increasing demand for nutrients such as phosphorus (P) and nitrogen (N). This study aimed to assess the impact of soybean breeding on P and N utilization efficiencies.
Methods: Field experiments with split-plot experimental designs were conducted at two locations [Dafang (DF) and Shiqian (SQ)] in the 2019 growing season to determine the agronomic efficiency of P fertilizer (AEp), P and N utilization efficiencies, and P and N accumulation and partitioning in different soybean organs under 0 (P0) and 35 (P35) kg ha−1 P supply.
Results: The results showed that soybean breeding targeting high seed yield also improved AEp (p < 0.05) and P (p < 0.05) and N utilization efficiencies (p < 0.05), with the improvement in AEp associated with the high yield response to P supply. P and N accumulation significantly increased in pods (p < 0.05) and leaves (p < 0.05) but not in stems or roots with year of release, while P and N concentrations did not change in any organ with year of release. In addition, only pod dry weight significantly increased (p < 0.01) with year of release, and P and N partitioning increased to pods (p < 0.05) but decreased to stems (p < 0.05) with year of release. Correlation and PCA analyses revealed P and N utilization efficiencies positively correlated with P and N partitioning to pods but negatively correlated with P and N partitioning to stems. While P supply increased P and N accumulation, it reduced P utilization efficiency.
Discussion: We conclude that (1) soybean breeding improved AEp and P and N utilization efficiencies; (2) the increased P and N partitioning to pods but decreased partitioning to stems contributed to the high P and N utilization efficiencies in new soybean cultivars, reducing the demand for N and P; (3) P supply increased nutrient accumulation but reduced P utilization efficiency. These results highlight the significance of appropriate resource allocation among organs and efficient P management for enhancing nutrient utilization and reducing fertilizer requirements.
Soybean (Glycine max (L.) Merrill) is an important crop cultivated for its rich protein content (Wu et al., 2015). However, in China, soybean production faces a shortfall in domestic soybean production, relying heavily on imports (>80%). Southwestern China, a key soybean production area, has undertaken numerous efforts, including soybean breeding, to improve soybean yields (Yang et al., 2022; Zhang et al., 2022). Breeding programs aimed at increasing seed yield have significantly improved yields (Todeschini et al., 2019; de Felipe et al., 2020), primarily through increased biomass in China (Jin et al., 2010; Yang et al., 2022), United States (Cafaro La Menza et al., 2017) and South America (Todeschini et al., 2019). Increases in harvest index have contributed to soybean yield gains worldwide (He et al., 2016; Todeschini et al., 2019; Tamagno et al., 2020; Feng et al., 2022). Changes in yield components, such as seed number and seed size, have also been associated with increased soybean seed yields in China (Wang et al., 2016; Qin et al., 2017; Zhang et al., 2022), United States (Kumudini et al., 2001), and South America (de Felipe et al., 2016). Genetic gains in soybean seed yield range from 0.4 to 2.2% y−1 worldwide and about 2.0% y−1 in southwestern China (Yang et al., 2022). Improved lodging resistance has also helped boost yields during soybean breeding (Jin et al., 2010; Kumudini et al., 2001; Rogers et al., 2015; Milioli et al., 2022). However, the impact of soybean breeding on P and N utilization efficiencies in southwestern China remains unclear.
Nutrient uptake, especially nitrogen (N) and phosphorus (P) is vital for crop growth and productivity (Jat and Bijay-Singh, 2014; Salvagiotti et al., 2021; Meng et al., 2022). Both P and N are involved in leaf photosynthesis, essential for crop growth, and high accumulation of these nutrients is important for achieving high seed yields (Tamagno et al., 2017) and efficient nutrient utilization (Meng et al., 2022). High nutrient accumulation is needed to accumulate high biomass (Cafaro La Menza et al., 2017), associated with high seed number and/or seed size and, thus, seed yield (He et al., 2019; Meng et al., 2022). Thus high-yielding soybean cultivars have high N (Salvagiotti et al., 2008; Gaspar et al., 2017) and P accumulation (He et al., 2017b, 2019), requiring increased P and N uptake through root inputs and/or modifications to root structure characteristics, such as increased adventitious root density and shallow root angle (He et al., 2017b; Lynch, 2019). Recent studies have shown that N accumulation increased with seed yield improvement during soybean breeding in Argentina (de Felipe et al., 2020) and United States (Donahue et al., 2020). However, the relative contributions of increased biomass and nutrient concentration to nutrient accumulation during soybean breeding are poorly understood. Enhancing nutrient utilization efficiencies (seed yield/total nutrient accumulation) under low fertilizer inputs can enhance yields and potentially reduce N and P demands, promoting sustainable agriculture and increasing food security (An et al., 2018; Wu et al., 2019). Nutrient utilization efficiencies have been associated with harvest index, a key trait determining grain yield (Meng et al., 2022). Genetic variations in nutrient utilization efficiencies have been reported for various crops, including wheat (Ortiz-Monasterio et al., 1997; Sadras and Lawson, 2013), maize (Ciampitti and Vyn, 2012), barley (Muurinen et al., 2006; Bingham et al., 2012), and cotton (Rochester and Constable, 2015). However, the effects of breeding on nutrient use efficiencies vary among different species. For example, P and N utilization efficiencies significantly increased in cotton cultivars released in Australia from 1973 to 2006 (Rochester and Constable, 2015), while N utilization efficiency did not change with year of release in barley cultivars (Muurinen et al., 2006). These inconsistent results suggest that changes in nutrient utilization efficiency during cultivar improvement may be species-specific.
Understanding changes in nutrient partitioning among plant organs could help reduce N and P demands by directing limited nutrients to essential organs, such as reproductive organs. For example, increased seed biomass accumulation changes the N partitioning between other plant organs (Sinclair, 1998). Nutrient partitioning is associated with biomass partitioning (Donald and Hamblin, 1976; Tamagno et al., 2017). While soybean breeding has increased plant biomass (Yang et al., 2022), it remains unclear if dry weights and nutrient accumulation have improved in all organs, how P and N partitioning among organs has changed, and how P and N utilization efficiencies have been affected.
This study investigated changes in the agronomic efficiency of P fertilizer (AEp), P and N accumulation and utilization efficiencies, and their partitioning to different organs in a historic set of 12 soybean cultivars bred for high seed yield. The study was condcuted under two P rates [0 (P0) and 35 (P35) kg ha−1 P] at two field sites [Dafang (DF) and Shiqian (SQ)] during the 2019 growing season. The hypotheses tested were: (1) soybean breeding has increased P and N utilization efficiencies with seed yield; (2) enhanced P and N utilization efficiencies are associated with high P and N partitioning to pods.
This study evaluated a historic set of 12 soybean cultivars (released from 1995 to 2016, Supplementary Table S1) grown by local farmers (past and present) at two field sites [Shiqian (SQ) and Dafang (DF)] in Guizhou Province, China, in 2019. The cultivars were collected from three provinces in southwest China (Sichuan, Yunnan, and Guizhou), where soybean breeding focused on increasing seed yield. All cultivars can grow in Guizhou province, with maturity times ranging from 115 to 121 days after sowing (DAS) for SQ and 124 to 130 DAS for DF. The soil pH, total P, and plant available soil P were 6.8, 0.77 g kg−1 and 33 mg kg−1 for SQ, and 6.7, 0.92 g kg−1 and 33 mg kg−1 for DF, respectively. The mean temperature and precipitation were 849 mm and 20.2°C for SQ and 573 mm and 23.6°C for DF, respectively (Supplementary Figure S1). The split-plot design had two P levels [zero P (P0) and 35 kg ha−1 (P35) applied as calcium superphosphate] as the main plots, with cultivars as the sub-plots. Each cultivar in each main plot had three replicates, for a total of 72 plots at each site. Each plot was 12.8 m2 (3.2 m wide × 4 m long), with rows spaced 0.4 m apart. The straight line between the two main plots was 2 m. Two days before sowing, N and K fertilizers were applied to all plots as urea (75 kg N ha−1) and K2SO4 (40 kg K ha−1) according to our previous study (Zhang et al., 2022), with the same N and K rates used at both experimental sites. The fertilizers were broadcast and mixed into the soil using a rotary cultivator. The seeds were sown (April 2019) at about 5 cm depth with a 40 cm row spacing. After germination, each plot was thinned to 18 seedlings per m2. No irrigation was applied. Weeds were removed by hand, with pesticides used as needed. The upper 20 cm of soil was collected to analyze the basic nutrient status before applying the fertilizer. Weather data were collected from weather stations near the field sites (straight-line distance ranged from 0.5–19.2 km).
Plant samples were harvested at the R6 stage when the pods contained full-sized green beans on one of the four uppermost nodes with a completely unrolled leaf (Fehr et al., 1971). For each plot, about 0.5 m2 of soybean plants were cut just above the soil surface, placed in paper bags, transported to the laboratory, divided into pods, leaves, and stems, and oven-dried at 60°C for 72 h. After drying, the samples were weighed, stored, and later used to determine P and N concentrations. After shoot removal, a standard spade was used to excavate roots to 20 cm depth (depth of most roots), which were washed carefully to remove root-attached soil, oven-dried at 80°C for 48 h, and weighed. The root samples were stored for later determination of P and N concentrations.
P and N concentrations were measured according to He et al. (2019). All samples were ground to a fine powder using an Ultra Centrifugal Mill (ZM200, Retsch, GmbH, Düsseldorf, Germany). Samples (~0.2 g) were digested with H2SO4-H2O2 to determine total N concentration using the Kjeldahl method (SKD-800, Shanghai Peiou Analytical Instruments Co. Ltd., Shanghai, China) and total P concentration using the molybdenum–stibium anti-spectrophotometry method (UV-1800 Spectrophotometer, Shanghai Meipuda Instrument Co. Ltd., Shanghai, China). P (N) accumulation in pods (stems, leaves, roots) was obtained by multiplying pod (stem, leaf, root) P (N) concentration by pod (stem, leaf, root) DW. Total P (N) accumulation was obtained by summing P (N) accumulation in different plant parts. P (N) partitioning to pod (stem, leaf, root) = P (N) accumulation in pod (stem, leaf, root)/total P (N) accumulation (Feng et al., 2021). The P and N utilization efficiencies calculated as (Meng et al., 2022):
P utilization efficiency = seed yield/total P accumulation.
N utilization efficiency = seed yield/total N accumulation.
Two center rows (0.8 m × 4 m = 3.2 m2) in each plot were harvested at physiological maturity (He et al., 2017a) before placing the pods into bags, transporting them to the laboratory, and oven-drying at 60°C for 72 h. Dried pods were threshed by hand to remove the seeds, which were weighed to calculate seed yield (seed weight/harvest area). The agronomic efficiency of P fertilizer (AEp) and yield response to P were calculated as:
AEp = (seed yield at P35 − seed yield at P0)/P fertilizer application rate.
Yield response to P = (seed yield at P35 − seed yield at P0)/seed yield at P0.
A three-way analysis of variance (ANOVA) analyzed the effects of genotype, P level, location, and their interactions on P and N utilization efficiencies, pod, leaf, stem, and root dry weights, P and N concentrations, and P and N accumulation, and P and N partitioning to pod, leaves, stems, and roots using the GenStat 19.0 statistical package (VSN International Ltd., Rothamsted, England). Changes in the measured parameters with year of release were fitted with a linear model for each site. The linear or sigmoid model was used to evaluate the relationships between N utilization efficiency and N accumulation and partitioning to pods, leaves, stems, and roots and between P utilization efficiency and P accumulation and partitioning to pods, leaves, stems, and roots. All data determined in the field experiment were combined to perform principle component analysis (PCA) with Origin (Pro 2023, Origin Lab, Northampton, MA, United States).
Soybean genotype and experimental site significantly affected P and N utilization efficiencies, while P level only affected P utilization efficiency (Supplementary Table S2). Genetic variation in P and N utilization among the 12 soybean cultivars occurred (p < 0.001). The P utilization efficiencies at Shiqian (SQ) ranged from 92–150 g g−1 (average 121 g g−1) under 35 kg P ha−1 (P35) supply and 115–152 g g−1 (average 137 g g−1) under 0 kg P ha−1 (P0) supply, and at Dafang (DF) ranged from 73–117 g g−1 (average 100 g g−1) under P35 and 90–138 g g−1 (average 116 g g−1) under P0 (Figure 1). P supply significantly decreased P utilization efficiency at SQ (19%; p < 0.001) and DF (16%; p < 0.001; Supplementary Table S2; Figures 1A,B). The yield response to P application ranged from 5.5–32.7 at SQ and 7.8–39.5 at DF (Figure 1).
Figure 1. Changes in soybean (A) P and (B) N utilization efficiencies with year of release under 0 (P0) and 35 (P35) kg ha−1 P supply and changes in (C) yield response to P and (D) agronomy efficiency of P with year of release at Shiqian (SQ) and Dafang (DF). *p < 0.05, **p < 0.01, and ***p < 0.001.
Soybean genotype, P level, experimental site, and their interactions significantly affected P and N concentrations in pods, leaves, stems, and roots, genotype and P level significantly affected leaf and pod dry weights, and genotype and experimental site significantly affected root and stem dry weights. For genotypes, root dry weights ranged from 0.95 to 2.78 g plant−1 (p < 0.001), and stem dry weights ranged from 5.77 to 9.45 g plant−1 (p < 0.001). Average root dry weights were 1.8 g plant−1 at SQ and 1.6 g plant−1 at DF (p < 0.001), and average stem dry weights were 6.7 g plant−1 at SQ and 7.2 g plant−1 at DF (p < 0.05; Supplementary Table S2). P supply significantly increased pod (18%, p < 0.001) and leaf (16%, p < 0.001) dry weights but did not affect stem or root dry weights (Supplementary Table S2; Figure 2). Only soybean genotype and P level affected pod and leaf P accumulation (Supplementary Table S2). P supply increased P accumulation in pods (25%, p < 0.001), leaves (31%, p < 0.001), and stems (69%, p < 0.001; Supplementary Table S2; Figures 3A,C,E).
Figure 2. Changes in soybean (A) pod, (B) leaf, (C) stem, and (D) root dry weights and (E), (I) pod, (F), (J) leaf, (G), (K) stem, and (H), (L) root P and N concentrations with year of release under 0 (P0) and 35 (P35) kg ha−1 P supply at Shiqian (SQ) and Dafang (DF). **p < 0.01 and ***p < 0.001.
Figure 3. Changes in soybean (A), (B) pod, (C), (D) leaf, (E), (F) stem, and (G), (H) root P and N accumulation with year of release under 0 (P0) and 35 (P35) kg ha−1 P supply at Shiqian (SQ) and Dafang (DF). *p < 0.05, **p < 0.01, and ***p < 0.001.
Genetic variations in nutrient partitioning to different plant parts occurred (p < 0.001), which were significantly affected by soybean genotype, P level, experimental site, and their interactions (Supplementary Table S2). Pods had the highest P and N partitioning (average 47% for P, 51% for N), while roots had the lowest (3.5% for P, 1.3% for N; Figure 4). P supply decreased P and N partitioning to pods (9.4% for P, p < 0.001; 6.9% for N, p < 0.001) but increased P partitioning to stems (18.5% for P, p < 0.001; 5.9% for N, p < 0.05) (Supplementary Table S1; Figures 4A,E). SQ had significantly higher N partitioning to pods than DF (p < 0.001), and the reverse was true for N partitioning to stems (p < 0.001; Figures 4B,F).
Figure 4. Changes in soybean (A), (B) pod, (C), (D) leaf, (E), (F) stem, and (G), (H) root P and N partitioning with year of release under 0 (P0) and 35 (P35) kg ha−1 P supply at Shiqian (SQ) and Dafang (DF). *p < 0.05, **p < 0.01, and ***p < 0.001.
P utilization efficiency positively correlated with pod P accumulation (r = 0.44, p = 0.009) but negatively correlated with leaf (r = −0.52, p < 0.001) and stem (r = −0.62, p < 0.001) P accumulation (Figure 5). N utilization efficiency positively correlated with pod N accumulation (r = 0.55, p < 0.001) but negatively correlated with stem P accumulation (r = −0.58, p < 0.001; Figure 6). P and N utilization efficiencies positively correlated with pod P (r = 0.59, p < 0.001) and N (r = 0.51, p < 0.001) partitioning but negatively correlated with stem P (r = −0.50, p < 0.001) and N (r = −0.50, p < 0.001) partitioning, respectively (Figures 5, 6). The principal component analysis showed a clear separation into two groups related to P rate (Figure 7). PC1 and PC2 represent 52.7% of the variation, with P and N utilization efficiencies, pod and root P and N partitioning, pod N concentration, and root dry weight tending to increase under P0. In contrast, P and N accumulation, leaf and pod dry weights, and leaf and pod P and N accumulation tend to increase under P35.
Figure 5. Relationship between P utilization efficiency and (A) pod P accumulation, (B) leaf P accumulation, (C) stem P accumulation, (D) root P accumulation, (E) pod P partitioning, (F) leaf P partitioning, (G) stem P partitioning, and (H) root P partitioning under 0 (P0) and 35 (P35) kg ha−1 P supply at Shiqian (SQ) and Dafang (DF).
Figure 6. Relationship between N utilization efficiency and (A) pod N accumulation, (B) leaf N accumulation, (C) stem N accumulation, (D) root N accumulation, (E) pod N partitioning, (F) leaf N partitioning, (G) stem N partitioning, and (H) root N partitioning under 0 (P0) and 35 (P35) kg ha−1 P supply at Shiqian (SQ) and Dafang (DF).
Figure 7. The principle component analysis (PCA) of the measured parameters in a historic set of 12 soybean cultivars under 0 (P0) and 35 (P35) kg P ha−1 supply in two experiment sites. PUE, P utilization efficiency; NUE, N utilization efficiency; PDW, pod dry weight; LDW, leaf dry weight; SDW, stem dry weight; RDW, root dry weight; PPC, pod P concentration; LPC, leaf P concentration; SPC, stem P concentration; RPC, root P concentration; PNC, pod N concentration; LNC, leaf N concentration; SNC, stem N concentration; RNC, root N concentration; PPA, pod P accumulation; LPA, leaf P accumulation; SPA, stem P accumulation; RPA, root P accumulation; PA, P accumulation; PNA, pod N accumulation; LNA, leaf N accumulation; SNA, stem N accumulation; RNA, root N accumulation; NA, P accumulation; Pod PP, pod P partition; Leaf PP, leaf P partition; Stem PP, stem P partition; Root PP, root P partition; Pod NP, pod N partition; Leaf NP, leaf N partition; Stem NP, stem N partition; Root NP, root N partition.
P and N utilization efficiencies significantly (p < 0.05) increased from 1995 to 2016 under both P levels at both sites (Supplementary Table S2; Figures 1A,B). The yield response to P fertilizer significantly increased with year of release (p = 0.04) at SQ (Figure 1C). The agronomic efficiency of P fertilizer (AEp) also significantly increased (p = 0.04 for DF and p = 0.016 for SQ) during soybean breeding from 1995–2016 (Figure 1D), ranging from 2.4–23.4 at SQ and 3.3–21.3 at DF. Pod dry weight significantly increased (p < 0.05) with year of release at SQ and DF under P35 and P0, while leaf, stem, and root dry weights did not change (Figure 2). P and N concentrations in pods, leaves, stems, and roots did not change with year of release (Figure 2). Pod P (p < 0.01) and N (p < 0.05) accumulation significantly increased with year of release (Figure 3). Leaf P accumulation significantly increased (p < 0.05) with year of release; leaf N accumulation had a weak positive correlation (p = 0.07) with year of release under P35 (Figures 3C,D). Stem and root P and N accumulation did not change with year of release (Figures 3E–H). P and N partitioning significantly increased to pods (p < 0.05) but decreased to stems (p < 0.05) with year of release (Figure 4). P and N partitioning to leaves and roots did not change with year of release (Figure 4).
The newer soybean cultivars exhibited higher seed yields and greater yield responses to P application than older cultivars. The higher seed yields of newer cultivars are the result of soybean breeding efforts worldwide (Jin et al., 2010; Todeschini et al., 2019; de Felipe et al., 2020; Yang et al., 2020, 2022). The greater yield response to P application in the newer cultivars can be attributed to the significant yield improvements compared to older cultivars under P supply, leading to improved agronomic efficiency of P fertilizer (AEp). In maize, increased yield with N fertilizer supply was associated with increased grain numbers (Liu et al., 2022), indicating the important role of yield components in the yield response to fertilizer supply, such as seed number and seed size (Kumudini et al., 2001; de Felipe et al., 2016; Wang et al., 2016; Qin et al., 2017; Zhang et al., 2022). Understanding the underlying mechanisms responsible for soybean’s high response to P supply, particularly related to yield components, would be valuable for future research.
Soybean breeding simultaneously increased yield and P and N accumulation, consistent with similar studies in Argentina (de Felipe et al., 2020) and United States (Donahue et al., 2020). High N accumulation positively correlated with leaf and pod biomass (Figure 7), indicating that increased soil N uptake sustains leaf and seed development (He et al., 2019; Jin et al., 2022). Similarly, high P accumulation may be associated with root traits associated with P acquisition (He et al., 2017b, 2021; Lynch, 2019). While soybean breeding did not change root dry weights, which did not correlate with P or N accumulation (Figure 7), other root traits, such as shallow root growth angle, could improve P uptake (Lynch, 2019) and contribute to P and N accumulation.
In this study, biomass accumulation increased during soybean breeding, consistent with other studies (Jin et al., 2010; Todeschini et al., 2019; de Felipe et al., 2020; Yang et al., 2022), which could play a role in increasing nutrient accumulation. Soybean breeding did not change P and N concentrations despite the increase in biomass, suggesting that the increase in biomass accumulation primarily drives P and N accumulation, which is influenced by factors such as the duration after flowering (Yang et al., 2022) and/or high photosynthesis rate (Todeschini et al., 2019). However, it is important to consider the role of soil nutrient status in nutrient accumulation. For example, DF with high soil-available P had higher P and N concentrations than SQ with low soil-available P, which were associated with the high leaf and stem P and N accumulation driven by the high stem and leaf biomass. Thus, soil nutrient status can increase nutrient accumulation by increasing biomass accumulation.
P and N accumulation increased with P supply. In addition, P and N accumulation positively correlated with leaf and pod dry weights (Figure 7) without diluting P and N concentrations, indicating that biomass accumulation with P supply primarily drove pod and leaf P accumulation. Pods had significantly higher genetic gains in P and N accumulation (average 26.9 mg m−2 y−1 for P, 239 mg m−2 y−1 for N) than leaves (11.9 mg m−2 y−1 for P, 91 mg m−2 y−1 for N), demonstrating that pods contributed more to P and N accumulation than leaves. The high genetic gains of pod P and N accumulation were also associated with the high demand for P and N during seed development; furthermore, the partitioning of P and N from leaves to seeds also contributed to pod P and N accumulation (Gaspar et al., 2017). Thus, enhanced pod P and N accumulation was associated with high seed numbers, a key driver for seed yield improvement during soybean breeding (Jin et al., 2010; He et al., 2019; Yang et al., 2022).
Soybean breeding improved P and N utilization efficiencies, supporting our first hypothesis. Seed yield had a higher genetic gain (average 2.0% y−1) than P (1.2% y−1) or N accumulation (1.0% y−1), contributing to improved P and N utilization efficiencies. Similar improvements in P and N utilization efficiencies have been observed in other crops, such as wheat (Ortiz-Monasterio et al., 1997; Sadras and Lawson, 2013), cotton (Rochester and Constable, 2015), and rice (Meng et al., 2022). However, it is worth noting that P and N utilization efficiencies can vary among cultivars and crops (Dhugga and Waines, 1989; Ortiz-Monasterio et al., 1997; Ciampitti and Vyn, 2012), as observed in the soybean cultivars used in this study. Soybean had higher P and N utilization efficiencies (74–152 g g−1 for P, 15–22 g g−1 for N) than cotton (65–80 g g−1 for P, 12–15 g g−1 for N; Rochester and Constable, 2015), but lower P and N utilization efficiencies than rice (159–180 g g−1 for P, 45–54 g g−1 for N; Meng et al., 2022), indicating room for improvement in soybean. The P supply reduced the P utilization efficiency (Figure 7) at both sites, possibly because the rate of seed yield improvement (16.8%) with P supply was lower than the rate of P accumulation (33.2%). Despite the impact of soybean breeding on P utilization efficiency, the PUE was significantly affected by the interaction of genotype and P rate (p < 0.01). Moreover, SQ with low plant-available soil P had a significantly higher P utilization efficiency than DF with high soil-available P, highlighting the importance of soil P status and P management practices in regulating P utilization efficiency. One possible explanation for the difference between the two sites is the lower average P accumulation at SQ (1.8 g m−2) than DF (2.2 g m−2) but similar seed yield.
In this study, P and N partitioning significantly increased to pods but decreased to stems during soybean breeding, with this trend consistent across the two P rates tested. Decreased nutrient partitioning to low-demand organs such as stems and increased partitioning to seeds can enhance yield (Weiner, 2019). This trade-off between P and N partitioning in pods and stems during soybean breeding was important for improving nutrient utilization efficiencies (Ciampitti and Vyn, 2012, 2013). The increase in P and N partitioning to seeds is likely associated with the increase in seed number, the key driver of yield improvement during crop breeding (Jin et al., 2010; Qin et al., 2017; Meng et al., 2022; Zhang et al., 2022). More seeds require increased P and N to support seed development and quality, including protein formation. Thus, increasing seed yield through higher seed numbers could increase P and N partitioning to seeds and subsequently improve P and N utilization efficiencies. In addition, nutrient partitioning may be related to dry matter partitioning, as indicated by the increased harvest index (pod harvest index) and decreased dry matter partitioning to stems during soybean breeding (Yang et al., 2022). On the other hand, the trade-off between P and N partitioning to pods and stems could reduce P and N demand, as supported by the lower genetic gains for P (average 1.2% y−1) and N accumulation (1.0% y−1) than seed yield (2.0% y−1). Thus P and N partitioning can help improve P and N utilization efficiencies, supporting our second hypothesis.
This study evaluates whether soybean breeding increased P and N utilization efficiencies in southwestern China. We confirmed that selecting for high seed yield during soybean breeding improved P and N utilization efficiencies, attributed to increased P and N partitioning to pods while reducing their partitioning to stems. The increased P and N accumulation in pods, driven by pod biomass, played a significant role in the overall P and N accumulation during soybean breeding. We conclude that soybean breeding increased P and N utilization efficiencies by regulating P and N accumulation and partitioning.
The raw data supporting the conclusions of this article will be made available by the authors, without undue reservation.
JH and YJ conceived the experiments. XL and RD performed the experiments. XL, YJ, and RD analyzed the data. XL, KS, and JH wrote this manuscript. All authors contributed to the article and approved the submitted version.
This research was supported by the National Natural Science Foundation of China (32060427), Guizhou Science and Technology Support Program Project (Qiankehezhicheng (2021) yiban217).
The authors declare that the research was conducted in the absence of any commercial or financial relationships that could be construed as a potential conflict of interest.
All claims expressed in this article are solely those of the authors and do not necessarily represent those of their affiliated organizations, or those of the publisher, the editors and the reviewers. Any product that may be evaluated in this article, or claim that may be made by its manufacturer, is not guaranteed or endorsed by the publisher.
The Supplementary material for this article can be found online at: https://www.frontiersin.org/articles/10.3389/fsufs.2023.1204293/full#supplementary-material
An, N., Wei, W., Qiao, L., Zhang, F., Christie, P., Jiang, R., et al. (2018). Agronomic and environmental causes of yield and nitrogen use efficiency gaps in Chinese rice farming systems. Eur. J. Agron. 93, 40–49. doi: 10.1016/j.eja.2017.11.001
Bingham, I. J., Karley, A. J., White, P. J., Thomas, W. T. B., and Russell, J. R. (2012). Analysis of improvements in nitrogen use efficiency associated with 75 years of spring barley breeding. Eur. J. Agron. 42, 49–58. doi: 10.1016/j.eja.2011.10.003
Cafaro La Menza, N., Monzon, J. P., Specht, J. E., and Grassini, P. (2017). Is soybean yield limited by nitrogen supply? Field crop. Res. 213, 204–212. doi: 10.1016/j.fcr.2017.08.009
Ciampitti, I. A., and Vyn, T. J. (2012). Physiological perspectives of changes over time in maize yield dependency on nitrogen uptake and associated nitrogen efficiencies: a review. Field Crop Res. 133, 48–67. doi: 10.1016/j.fcr.2012.03.008
Ciampitti, I. A., and Vyn, T. J. (2013). Grain nitrogen source changes over time in maize: a review. Crop Sci. 53, 366–377. doi: 10.2135/cropsci2012.07.0439
de Felipe, M., Borras, L., Truong, S. K., McCormick, R. F., and Rotundo, J. L. (2020). Physiological processes associated with soybean genetic progress in Argentina. Agrosyst. Geosci. Environ. 3:e20041. doi: 10.1002/agg2.20041
de Felipe, M., Gerde, J. A., and Rotundo, J. L. (2016). Soybean genetic gain in maturity groups III to V in Argentina from 1980 to 2015. Crop Sci. 56, 3066–3077. doi: 10.2135/cropsci2016.04.0214
Dhugga, K. S., and Waines, J. G. (1989). Analysis of nitrogen accumulation and use in bread and durum-wheat. Crop Sci. 29, 1232–1239. doi: 10.2135/cropsci1989.0011183X002900050029x
Donahue, J. M., Bai, H., Almtarfi, H., Zakeri, H., and Fritschi, F. B. (2020). The quantity of nitrogen derived from symbiotic N fixation but not the relative contribution of N fixation to total N uptake increased with breeding for greater soybean yields. Field Crop Res. 259:107945. doi: 10.1016/j.fcr.2020.107945
Donald, C. M., and Hamblin, J. (1976). The biological yield and harvest index of cereals as agronomic and plant breeding criteria. Adv. Agron. 28, 361–405. doi: 10.1016/S0065-2113(08)60559-3
Fehr, W., Caviness, C., Burmood, D., and Pennington, J. (1971). Stage of development descriptions for soybeans, Glycine max (L.) Merrill. Crop Sci. 11, 929–931. doi: 10.2135/cropsci1971.0011183X001100060051x
Feng, Y. Y., He, J., Jin, Y., and Li, F. M. (2021). High phosphorus acquisition and allocation strategy is associated with soybean seed yield under water- and P-limited conditions. Agronomy 11:574. doi: 10.3390/agronomy11030574
Feng, Y. Y., Richards, A. R., Jin, Y., Siddique, K. H. M., Li, F. M., and He, J. (2022). Yield and water-use related traits in landrace and new soybean cultivars in arid and semi-arid areas of China. Field Crop Res. 283:108559. doi: 10.1016/j.fcr.2022.108559
Gaspar, A. P., Laboski, C., Naeve, S. L., and Conley, S. P. (2017). Dry matter and nitrogen uptake, partitioning, and removal across a wide range of soybean seed yield levels. Crop Sci. 57, 2170–2182. doi: 10.2135/cropsci2016.05.0322
He, J., Du, Y. L., Wang, T., Turner, N. C., Xi, Y., and Li, F. M. (2016). Old and new cultivars of soya bean (Glycine max L.) subjected to soil drying differ in abscisic acid accumulation, water relations characteristics and yield. J. Agron. Crop Sci. 202, 372–383. doi: 10.1111/jac.12143
He, J., Du, Y. L., Wang, T., Turner, N. C., Yang, R. P., Jin, Y., et al. (2017a). Conserved water use improves the yield performance of soybean (Glycine max (L.) Merr.) under drought. Agric. Water Manag. 179, 236–245. doi: 10.1016/j.agwat.2016.07.008
He, J., Jin, Y., Du, Y. L., Wang, T., Turner, N. C., Yang, R. P., et al. (2017b). Genotypic variation in yield, yield components, root morphology and architecture, in soybean in relation to water and phosphorus supply. Front. Plant Sci. 8:1499. doi: 10.3389/fpls.2017.01499
He, J., Jin, Y., Siddique, K. H. M., and Li, F. M. (2021). Trade-off between root efficiency and root size is associated with yield performance of soybean under different water and phosphorus levels. Agriculture 11:481. doi: 10.3390/agriculture11060481
He, J., Jin, Y., Turner, N. C., Chen, Z., Liu, H. Y., Wang, X. L., et al. (2019). Phosphorus application increases root growth, improves daily water use during the reproductive stage, and increases grain yield in soybean subjected to water shortage. Environ. Exp. Bot. 166:103816. doi: 10.1016/j.envexpbot.2019.103816
Jat, M. L., and Bijay-Singh, G. B. (2014). Nutrient management and use efficiency in wheat systems of South Asia. Adv. Agron. 125, 171–259. doi: 10.1016/B978-0-12-800137-0.00005-4
Jin, Y., He, J., Zhu, Y., and Siddique, K. H. M. (2022). Nodule formation and nitrogen use efficiency are important for soybean to adapt to water and P deficit conditions. Agriculture 12:1326. doi: 10.3390/agriculture12091326
Jin, J., Liu, X., Wang, G., Mi, L., Shen, Z., Chen, X., et al. (2010). Agronomic and physiological contributions to the yield improvement of soybean cultivars released from 1950 to 2006 in Northeast China. Field Crop Res. 115, 116–123. doi: 10.1016/j.fcr.2009.10.016
Kumudini, S., Hume, D. J., and Chu, G. (2001). Genetic improvement in short season soybeans: I. dry matter accumulation partitioning, and leaf area duration. Crop Sci. 41, 391–398. doi: 10.2135/cropsci2001.412391x
Liu, Z., Hao, Z. H., Sha, Y., Huang, Y. W., Guo, W. Q., Ke, L. H., et al. (2022). High responsiveness of maize grain yield to nitrogen supply is explained by high ear growth rate and efficient ear nitrogen allocation. Field Crop Res. 286:108610. doi: 10.1016/j.fcr.2022.108610
Lynch, J. P. (2019). Root phenotypes for improved nutrient capture: an underexploited opportunity for global agriculture. New Phytol. 223, 548–564. doi: 10.1111/nph.15738
Meng, T. Y., Zhang, X. B., Ge, J. L., Chen, X., Zhu, G. L., Chen, Y. L., et al. (2022). Improvements in grain yield and nutrient utilization efficiency of japonica inbred rice released since the 1980s in eastern China. Field Crop Res. 277:108427. doi: 10.1016/j.fcr.2021.108427
Milioli, A. S., Meira, D., Panho, M. C., Madella, L. A., Woyann, L. G., Todeschini, M. H., et al. (2022). Genetic improvement of soybeans in Brazil: south and Midwest regions. Crop Sci. 62, 2276–2293. doi: 10.1002/csc2.20820
Muurinen, S., Slafer, G. A., and Peltonen-Sainio, P. (2006). Breeding effects on nitrogen use efficiency of spring cereals under northern conditions. Crop Sci. 46, 561–568. doi: 10.2135/cropsci2005-05-0046
Ortiz-Monasterio, J. I., Sayre, K. D., Rajaram, S. S., and McMahon, M. (1997). Genetic progress in wheat yield and nitrogen use efficiency under four nitrogen rates. Crop Sci. 37, 898–904. doi: 10.2135/cropsci1997.0011183X003700030033x
Qin, X., Feng, F., Li, D., Herbert, S. J., Liao, Y., and Siddique, K. H. M. (2017). Changes in yield and agronomic traits of soybean cultivars released in China in the last 60 years. Crop Pasture Sci. 68, 973–984. doi: 10.1071/CP17002
Rochester, I. J., and Constable, G. A. (2015). Improvements in nutrient uptake and nutrient use-efficiency in cotton cultivars released between 1973 and 2006. Field Crop Res. 173, 14–21. doi: 10.1016/j.fcr.2015.01.001
Rogers, J., Chen, P. Y., Shi, A. N., Zhang, B., Scaboo, A., Smith, S. F., et al. (2015). Agronomic performance and genetic progress of selected historical soybean varieties in the southern USA plant breeding, vol. 134, 85–93.
Sadras, V. O., and Lawson, C. (2013). Nitrogen and water-use efficiency of Australian wheat varieties released between 1958 and 2007. Eur. J. Agron. 46, 34–41. doi: 10.1016/j.eja.2012.11.008
Salvagiotti, F., Cassman, K. G., Specht, J. E., Walters, D. T., Weiss, A., and Dobermann, A. (2008). Nitrogen uptake, fixation and response to fertilizer N in soybeans: a review. Field Crop Res. 108, 1–13. doi: 10.1016/j.fcr.2008.03.001
Salvagiotti, F., Magnano, L., Ortez, O., Enrico, J., Barraco, M., Barbagelata, P., et al. (2021). Estimating nitrogen, phosphorus, potassium, and sulfur uptake and requirement in soybean. Eur. J. Agron. 127:126289. doi: 10.1016/j.eja.2021.126289
Sinclair, T. R. (1998). Historical changes in harvest index and crop nitrogen accumulation. Crop Sci. 38, 638–643. doi: 10.2135/cropsci1998.0011183X003800030002x
Tamagno, S., Balboa, G. R., Assefa, Y., Kovács, P., Casteel, S. N., Salvagiotti, F., et al. (2017). Nutrient partitioning and stoichiometry in soybean: a synthesis-analysis. Field Crop Res. 200, 18–27. doi: 10.1016/j.fcr.2016.09.019
Tamagno, S., Sadras, V. O., Ortez, O. A., and Ciampitti, I. A. (2020). Allometric analysis reveals enhanced reproductive allocation in historical set of soybean varieties. Field Crop Res. 248:107717. doi: 10.1016/j.fcr.2020.107717
Todeschini, M. H., Milioli, A. S., Rosa, A. C., Dallacorte, L. V., Panho, M. C., Marchese, J. A., et al. (2019). Soybean genetic progress in South Brazil: physiological, phenological and agronomic traits. Euphytica 215:214. doi: 10.1007/s10681-019-2439-9
Wang, C., Wu, T., Sun, S., Xu, R., Ren, J., Wu, C., et al. (2016). Seventy-five years of improvement of yield and agronomic traits of soybean cultivars released in the yellow-Huai-Hai River valley. Crop Sci. 56, 2354–2364. doi: 10.2135/cropsci2015.10.0618
Weiner, J. (2019). Looking in the wrong direction for higher-yielding crop genotypes. Trends Plant Sci. 24, 927–933. doi: 10.1016/j.tplants.2019.07.001
Wu, Z., Luo, J., Han, Y., Hua, Y., Guan, C., and Zhang, Z. (2019). Low nitrogen enhances nitrogen use efficiency by triggering NO3-uptake and its long-distance translocation. J. Agr. Food Chem. 67, 6736–6747. doi: 10.1021/acs.jafc.9b02491
Wu, T. T., Sun, S., Wang, C. J., Lu, W. C., Sun, B. H., Song, X. Q., et al. (2015). Characterizing changes from a century of genetic improvement of soybean cultivars in Northeast China. Crop Sci. 55, 2056–2067. doi: 10.2135/cropsci2015.01.0023
Yang, M. H., Jahufer, M. Z. Z., He, J., Dong, R., Hofmann, R., Siddique, K. H. M., et al. (2020). Effect of traditional soybean breeding on water use strategy in arid and semi-arid areas. Eur. J. Agron. 120:126128. doi: 10.1016/j.eja.2020.126128
Yang, J. X., Richards, R. A., Jin, Y., and He, J. (2022). Both biomass accumulation and harvest index drive the yield improvements in soybean at high and low phosphorus in south-West China. Field Crop Res. 277:108426. doi: 10.1016/j.fcr.2021.108426
Keywords: nutrient utilization efficiency, genetic improvement, yield response, nutrient partitioning, nutrient accumulation
Citation: Luo X, Dong R, Siddique KHM, He J and Jin Y (2023) Soybean breeding in southwestern China improved P and N utilization efficiencies by increasing phosphorus and nitrogen partitioning to pods. Front. Sustain. Food Syst. 7:1204293. doi: 10.3389/fsufs.2023.1204293
Received: 13 April 2023; Accepted: 06 July 2023;
Published: 20 July 2023.
Edited by:
Raju Datla, Global Institute for Food Security (GIFS), CanadaReviewed by:
Juan Fernando Hirzel, Agricultural Research Institute (Chile), ChileCopyright © 2023 Luo, Dong, Siddique, He and Jin. This is an open-access article distributed under the terms of the Creative Commons Attribution License (CC BY). The use, distribution or reproduction in other forums is permitted, provided the original author(s) and the copyright owner(s) are credited and that the original publication in this journal is cited, in accordance with accepted academic practice. No use, distribution or reproduction is permitted which does not comply with these terms.
*Correspondence: Jin He, aGVqaW4wODExQDE2My5jb20=; Yi Jin, eWtpbmcxMjI1QDE2My5jb20=
Disclaimer: All claims expressed in this article are solely those of the authors and do not necessarily represent those of their affiliated organizations, or those of the publisher, the editors and the reviewers. Any product that may be evaluated in this article or claim that may be made by its manufacturer is not guaranteed or endorsed by the publisher.
Research integrity at Frontiers
Learn more about the work of our research integrity team to safeguard the quality of each article we publish.