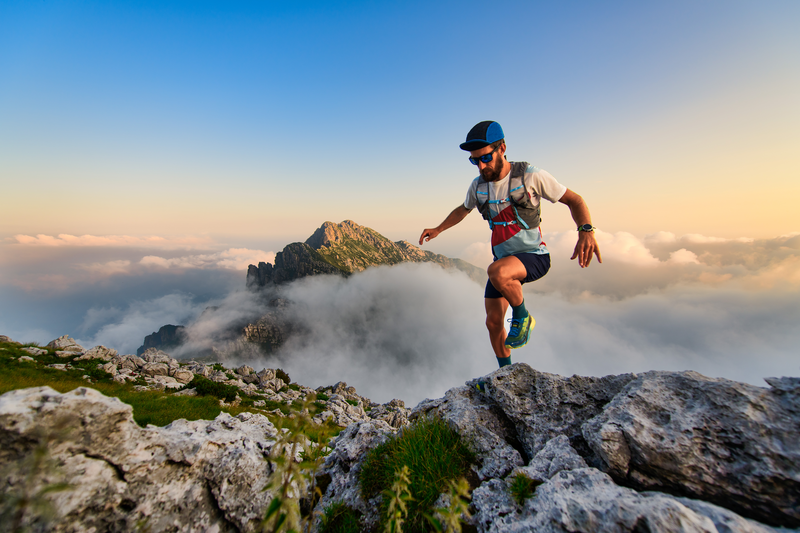
94% of researchers rate our articles as excellent or good
Learn more about the work of our research integrity team to safeguard the quality of each article we publish.
Find out more
ORIGINAL RESEARCH article
Front. Sustain. Food Syst. , 24 July 2023
Sec. Crop Biology and Sustainability
Volume 7 - 2023 | https://doi.org/10.3389/fsufs.2023.1198649
This article is part of the Research Topic Interrelationships Between Food Security, Crop Field Management and Technology View all 9 articles
To cope with the change of precipitation pattern, we explored the effects of conservation tillage and precipitation variability on soil moisture status and silage maize productivity, seeking solutions to improve the yield and quality of maize in the karst areas of China. In this study, precipitation variability and conservation tillage were jointly analyzed by precipitation levels (normal precipitation, 30% lower and 30% greater than normal precipitation), tillage methods (conventional tillage and no-tillage) and cover crop mulching (mulching and no mulching). It was found that no tillage (NT) increased the maize yield by 12.85% compared with the conventional tillage (CT) treatments. Above-normal precipitation treatments did not significantly increase biomass and soil water content across different fertility stages, but below-normal precipitation treatments reduced maize yield, crude protein (CP) content and soil water content at all soil levels. CT treatment significantly increased soil water content in the tillage layer (0–40 cm), but NT treatment significantly increased dry matter yield, plant height and CP content at harvest by 10.99, 3.41, 5.62%. Cover crop mulching significantly increased maize yield by 8.26% and soil moisture content at the seedling stage in 0–40 cm soil by 2.76 and 2.52%. The three-way interaction effects involving precipitation levels, tillage methods and cover crop mulching were significant for maize yield, CP content and soil water content (p < 0.05). In addition, this study found that soil water content was positively correlated with crude protein content of maize (p < 0.05). In conclusion, this study concluded that a combination of NT and cover crop mulching should be used to mitigate maize production risks caused by precipitation variability in the karst areas.
Maize (Zea mays L.), as the crop with the highest share of global grain production, its yield is critical to global food security (Zhang, K. P., et al., 2022a). Maize is one of the crops that are sensitive to soil moisture and fertility conditions (Jia et al., 2018). Maize yield is greatly affected by growing season length and rainfall amount (Calviño et al., 2003). Meanwhile, precipitation variation can cause significant impacts on forage quality (Li et al., 2020), as well as reduction of acidic and neutral detergent fibers to improve forage nutritive value (Ahmad et al., 2022). Generally speaking, water stress hastens senescence and reduces nutrient uptake by roots, thus, reduce nutritive value and crude protein content. In addition, it also affects the water use efficiency (WUE) for biomass production (Ren et al., 2008; Li et al., 2022). However, information related to forage nutritive value under moderate reduced/increased irrigation levels in areas with ample precipitation seems scanty. Particularly, in the subtropics, extreme precipitation events are becoming more frequent and will intensify in the future due to global warming (Norris et al., 2020). Changes in precipitation quantity and distribution will pose new challenges to maize production in these regions.
The plight of agricultural production in karst areas has been an important constraint on regional economic development, especially in southwest China (Bai et al., 2022). The fragile ecological environment has led to a severe shortage of arable land, forcing farmers to plant maize on marginal and sloping land (Gao et al., 2022), where severe soil erosion and nutrient leaching usually occur (Herout et al., 2018). At the same time, the unique geomorphology of karst areas also affects soil hydrological properties, causing fast infiltration rate, poor water storage capacity, and low resistance to surface runoff (Jiang et al., 2014). Therefore, despite the average regional rainfall exceeds 1,000 mm/yr., maize growth is still largely constrained by soil moisture conditions.
The improvement of soil physicochemical properties and soil quality through conservation tillage has been widely demonstrated (Dikgwatlhe et al., 2014; Turmel et al., 2015; Clay et al., 2019). These effects include increased soil water storage (Lampurlanés et al., 2016), reduction in evaporation and infiltration, and increased topsoil water content (Liu et al., 2013). Cover crop mulching has indicated capacity to reduce water evaporation, enhance soil C and N, reduce soil erosion (Lal et al., 2004), improve soil enzyme activity and increase soil microbial populations (Chen et al., 2014; Zhang et al., 2016). However, there is spatial and temporal heterogeneity in many studies, making it difficult to draw consistent conclusions about the effects of conservation tillage. For example, many studies have shown that conservation tillage has no or even negative effects on maize yield (Peng et al., 2020; Zhang, K. P., et al., 2022a). In addition, many studies conducted in the karst areas focused only on the impact of tillage practices (Jia et al., 2019; Bai et al., 2022), ignoring the possible interactive effects with other factors, such as precipitation quantity and distribution (Zhang, Q., et al., 2022b), fertilization, crop type, and other agronomic practices (Wang et al., 2018).
Therefore, this paper investigates the changes in maize yield, quality and soil water content under the combined impacts of different precipitation intensities, tillage practices, and mulching measures. The objectives were to discover, (i) whether maize biomass differs among growth stages under the combined effect of precipitation variation and tillage/mulching practices, and (ii) how can grain yield, quality, and soil water content respond to these changes (iii) What is the inter-relationship between maize yield, quality and soil moisture in each soil layer? The answers to these questions will provide reasonable and feasible solutions for maize production in the karst areas of China.
The experimental was carry out at the Cultivation Experiment Station of Guizhou University in Dafang County (27°41′N, 105°89′E; Altitude 1,723 m), Bijie City, Guizhou Province. The site has a humid subtropical monsoon climate and represents a quintessential Guizhou Karst topography and climatic condition (Figure 1A). The precipitation and temperature in the study area are shown in Figure 1B. According to the International Society of Soil Sciences standards, the main soil type is Ali-Perudic Argosols (APA). The main crops in the area are silage maize (Zea mays L.) and ryegrass (Lolium multiflorum Lam.). The test site was managed as long-term ryegrass hay field. The main cultivation methods are Plough tillage at a depth of 20 cm and no tillage. The soil properties and nutrient conditions, measured at a soil depth of 0 to 100 cm before this experiment, started in 2021 are shown in Supplementary Table S1.
Figure 1. (A) Study location in southeast China. (B) The precipitation and mean temperature of maize growing season in the study area in 2021.
The experiment was carried out in a completely randomized group design with three factors: Precipitation (P), tillage (T) and cover crop mulching (M). Precipitation was set at three levels: normal precipitation (P, natural precipitation), precipitation reduction (P–, using precipitation reduction devices to reduce precipitation by 30%) and precipitation augmentation (P+, adding the retained precipitation from P– using rain capturing devices). Two levels of tillage were established as conventional tillage (CT, rototilling at depth at about 20 cm) and no-tillage (NT). Two levels of cover crop mulching were implemented as mulching (M, ryegrass cut to 5–10 cm and evenly mulched on the soil surface at 10 Mg ha−1) and no cover crop mulch (NM). The final treatments include: normal precipitation with conventional tillage and cover crop mulching (CTMP), normal precipitation with conventional tillage without cover crop mulching (CTP), normal precipitation without tillage cover or crop mulching (NTMP), normal precipitation without tillage cover or crop mulch (NTP), reduced precipitation with conventional tillage and cover crop mulch (CTMP–), reduced precipitation with conventional tillage but no cover crop mulching (CTP–), reduced precipitation without tillage but with cover crop mulching (NTMP–), reduced precipitation without tillage or cover crop mulch (NTP–), increased precipitation with conventional tillage and cover crop mulching (CTMP+), increased precipitation with conventional tillage but without cover crop mulching (CTP+), increased precipitation without tillage but with cover crop mulching (NTMP+), and increased precipitation without tillage or cover crop mulching (NTP+). All treatments were replicated three times with a total of 36 plots (Supplementary Figure S1).
“Qianqing 446” silage maize was sown on April 24, 2021. The plot area was 30.24 m2 (7.2 m × 4.2 m), and four rows were planted in each plot in a wide (80 cm)—narrow (40 cm) row pattern. The plant spacing was 30 cm and the sowing density was 54,000 plants ha−1. 10,000 kg hm−2 of organic fertilizer (fermented sheep manure: 0.8% N, 0.5% P2O5, 0.5% K2O) was applied as a base application before sowing, and 5,000 kg hm−2 of organic fertilizer was applied as a follow-up application at the nodulation stage. Harvesting was conducted on September 8, 2021 (late lactation to early waxing).
Each plot was equipped with a 3.0 m × 2.0 m micro-plot (located in the central position of the plot containing 4 rows and 10 columns of maize), surrounded by a three-sided ring of isolation baffles around the micro-plot, with no isolation baffles on the eastern side of each plot. The isolation baffle was a transparent PVC sheet 0.1 cm thick and 20 cm wide, with the bottom 10 cm of the baffle inserted into the soil and the top 10 cm above the soil surface to reduce the influence of the outside world on the micro-plot. The precipitation reduction treated plots were constructed with a 3.0 m × 2.0 m precipitation reduction device above the micro-plot. The rain reduction device was divided into two parts, the bottom half was a four-cornered lifting bracket device, and the stainless steel four-cornered bracket was fixed to the ground with steel nails so that it was in the shape of a right-angle ladder (the ground was at a right angle, and the remaining two angles were 60° and 120°), and the bracket could be manually adjusted at 1.0–3.0 m to facilitate the normal conduct of the experiment in the later stages of maize growth. The upper part of the rainwater retention device mainly comprises of a “U” shaped acrylic pipe, a “U” shaped PVC precipitation collection tank and a rainwater collection barrel. The “U” shaped acrylic pipe was 3 m long and 10 cm in diameter, covering an area 1.8 m2 to intercept 30% of the incoming precipitation. The “U” shaped PVC catch basin was installed at 30° in the direction of the acrylic pipe at an angle of 120°, 5 cm away from the acrylic pipe. The end of the “U” shaped PVC catch basin was connected to a pipe leading to a 100-L collection barrel to collect rainwater. The collected rainwater was evenly sprinkled into the micro-plot of the P+ treatment cells through a spraying can immediately after each precipitation to achieve a 30% increase in total precipitation. The transparent “U” shaped pipe could affect solar radiation, which may not have a significant impact on crop growth.
Three representative plants from each micro-plot were sampled for aboveground biomass calculation during several maize growth stage, including seedling stage (May 26), Jointing stage (July 4), Big trumpet stage (July 26), and Tasseling stage (August 5). Another three representative plants of the plot were selected from the micro-plot at harvest time (September 8) to measure maize plant height (height of maize vertically to the ground in its natural state), leaf length (length from the tip of the leaf to the bottom of the leaf) and leaf width (length of the leaf at its widest position). Dry matter yield was calculated at 105°C for 30 min and then baked at 65°C until constant weight (Lai et al., 2022). The dried samples were pulverized with a plant powder sampler and sealed through a 2 mm sieve for storage pending measurement. The samples were used for the determination of total nitrogen (TN), neutral detergent fiber (NDF) and acid detergent fiber (ADF) content by Kjeldahl nitrogen determination, salicylic acid colorimetric method, Indotrione colorimetric method and Van’s cellulose content determination method, respectively (Zhang, K. P., et al., 2022a).
The leaf area index (LAI) was calculated as follows:
Where, S1 is the leaf area of single maize plant (cm2), L is leaf length (cm), W is leaf width, Leaf area coefficient of 0.75 (The leaf area coefficient of unexpanded leaves was 0.5), n is number of maize plants per unit area. A is unit land area (cm2).
The crude protein (CP) was calculated as follows:
where, TN is the total nitrogen of maize plant (g kg−1), Conversion factor of 6.25.
The relative feed value (RFV) was calculated as follows:
Where, DMI is the dry matter intake (% Body weight), NDF is neutral detergent fiber (%), DDM is digestible dry matter content (%), ADF is acid detergent fiber (%).
A 0.8-m deep measuring tube was installed in the micro-plot and the volumetric water content of the soil was measured every 7 day using a profiling soil moisture sensor (TRIME-PICO IPH 2, IMKO, Germany) in the 0–20, 20–40 cm and 40–60 cm soil layers, averaged three times for each layer (120° rotation each time). Conversion from volumetric water content to mass water content.
The Soil water content (SWC) was calculated as follows:
where, VWC is the volumetric water content (cm3 cm−3), is bulk density (g cm−3).
The water use (WU, mm) was calculated as follows:
where, P is the precipitation (mm) and ΔS is the change in stored soil water of the soil profile (mm). Based on the consideration of groundwater table, terrain, etc., we omitted some components of the traditional field water balance formula, including irrigation, upward flow into the root zone, surface runoff, and downward drainage out of the root zone.
The water use efficiency (kg m−3) was calculated as follows:
where, Y is the maize dry matter yield (kg ha−1).
All variance analyses were performed using SPSS statistics software 26 (IBM, United States). T-test was used to compare the differences among the two tillage systems and two cover crop mulch patterns at p < 0.05 level. One-way ANOVA was used to compare the differences among the three precipitation scenarios and the biomass in the same growth period for different treatments at p < 0.05 level. Three-way ANOVA was used to compare the systems, precipitation scenarios, cover crop mulching patterns, and their interaction at p < 0.05 level. The R “corrplot” package was used to calculate the Pearson’s correlation matrix between indicators at, and the “PerformanceAnalytics” package was used for data visualization.
At the seedling stage, the biomass of CTP– and NTMP+ treatments were significantly greater than that of NTP–, NTP+, NTMP, NTMP– (Figure 2; p < 0.05). NTP+ treatment was significantly lower than that of CTP, CTMP, CTMP–, CTMP+, NTP. At the jointing stage, the biomass of CTMP treatment was significantly greater than that of CTP+, NTP, NTP–, NTP+, NTMP–. NTP+ treatment was significantly lower than that of CTP, CTP–, CTMP–, CTMP+, NTMP, NTMP+.
Figure 2. The biomass of maize in different growth stage in the study area in 2021. Notes: Conventional tillage (CT), no-tillage (NT), cover crop mulching (M), increased precipitation (P+), decreased precipitation (P−) and normal precipitation (P).
At the big trumpet stage, the NTMP treatment was significantly greater than all other treatments (Figure 2; p < 0.05). the CTMP treatment was significantly greater than CTMP–, CTMP+, NTP–, NTP+, NTMP–, and NTMP+. The NTP– treatment was significantly lower than the other treatments except NTMP–. At the tasseling stage, the CTMP and NTMP+ treatments were significantly greater than all other treatments except NTP+. the CTP+ treatment was significantly lower than CTP, NTP, NTP+, and NTMP. the CTMP+ treatment was significantly lower than all other treatments. At milk stage, the NTMP+ treatment was significantly greater than the other treatments except for the CTMP and NTMP treatments. The CTMP– treatment was significantly lower than the NTP– treatment.
The results showed that T had a highly significant effect on maize yield (p < 0.001; Table 1), P and M had a significant interaction (p < 0.01). M × P and T × M × P interactions were also significant (p < 0.05). P, M, M × P and T × M × P interactions had a significant effect on maize dry matter yield (p < 0.05), and T had a greater significant effect on yield (p < 0.01). In terms of plant height, T had a significant effect (p < 0.01). P, T × M, P × M, and T × M × P interactions were also significant (p < 0.05). For LAI, P × M had a significant effect (p < 0.001). P and T × M had a significant effect (p < 0.01), and T × M × P interactions were also significant (p < 0.05).
Table 1. Mean yield, dry matter yield, plant height and leaf area index (LAI) under different treatment in growth season for conventional tillage (CT), no-tillage (NT), cover crop mulching (M), no cover crop mulching (NM), increased precipitation (P+), decreased precipitation (P–) and normal precipitation (P).
T and P had a highly significant effect on CP (p < 0.001; Table 2). M, T × M × P interactions were significant (p < 0.05). There was no significant effect of each factor on NDF, ADF and RFV.
Table 2. Mean crude protein (CP), neutral detergent fiber (NDF), acid detergent fiber (ADF) and relative feed value (RFV) under different treatment in growth season for conventional tillage (CT), no-tillage (NT), cover crop mulching (M), no cover crop mulching (NM), increased precipitation (P+), decreased precipitation (P–) and normal precipitation (P).
Tillage had a highly significant effect on the SWC within the 0–20 cm soil layer at seedling, seed setting and total growth period stages (p < 0.001; Table 3). A more significant effect was also observed at the jointing and big trumpet stages (p < 0.01), and a significant effect was observed at the tasseling stage (p < 0.05). Cover crop mulching made a significant increase of the SWC within the 0–20 cm soil layer by 2.75% at the seedling stage (p < 0.05). Precipitation had a highly significant effect on SWC at 0–20 cm soil layer at seedling, jointing, seed setting and across the total growth period stages (p < 0.001), a more significant effect at the big trumpet stage (p < 0.01), and a significant effect at the tasseling stage (p < 0.05). The T × M × P interaction had a highly significant effect of SWC at 0–20 cm soil layer across the total growth period (p < 0.001), at the seedling stage (p < 0.01), and at all other growth stages (p < 0.05).
Table 3. Mean soil mass water content under different treatment in growth season for conventional tillage (CT), no-tillage (NT), cover crop mulching (M), no cover crop mulching (NM), increased precipitation (P+), decreased precipitation (P–) and normal precipitation (P) in 0–20 cm soil layer.
Tillage had a highly significant effect (p < 0.001; Table 4) of SWC at 20–40 cm soil layer at seedling, nodulation, trumpeting, fruiting and total fertility stages, and a highly significant effect (p < 0.01) at the stage-drawing stage. Compared to no cover crop mulching, cover crop mulch made a more significant increase of the SWC at 20–40 cm soil layer by 2.75% at seedling stage (p < 0.01), and a highly significant increase by 5.57% at total growth period stage (p < 0.001). Precipitation had a highly significant effect (p < 0.001) of SWC at the 20–40 cm soil layer during the seedling, jointing, seed setting and total fertility stages, with a more significant effect (p < 0.01) at the large trumpet stage, and a significant effect (p < 0.05) at the male drawing stage. The T × M × P interaction had a highly significant effect of SWC at 20–40 cm soil layer at the total reproductive stage (p < 0.001), a significant effect at the seedling (p < 0.01), and all other growth stages (p < 0.05).
Table 4. Mean soil mass water content under different treatment in growth season for conventional tillage (CT), no-tillage (NT), cover crop mulching (M), no cover crop mulching (NM), increased precipitation (P+), decreased precipitation (P–) and normal precipitation (P) in 20–40 cm soil layer.
Tillage treatments did not significantly affect (p < 0.001; Table 5) the SWC of the 40–60 cm soil layer at any reproductive stage. Cover crop mulch made a significant increase of the SWC at 40–60 cm soil layer by 1.16% at the total growth period stage. Precipitation had a more significant effect (p < 0.01) at the tasseling stage, and all other growth stages (p < 0.001). The T × M × P interaction had a significant effect of SWC at 40–60 cm soil layer at the tasseling stage (p < 0.05), a significant effect at the seedling stage (p < 0.01), and at all other growth stages (p < 0.001).
Table 5. Mean soil mass water content under different treatment in growth season for conventional tillage (CT), no-tillage (NT), cover crop mulching (M), no cover crop mulching (NM), increased precipitation (P+), decreased precipitation (P–) and normal precipitation (P) in 40–60 cm soil layer.
Water use was mainly influenced by the highly significant effect of precipitation (p < 0.001; Figure 3). Precipitation had a highly significant effect on WUE (p < 0.001). Tillage and cover crop mulching had a significant effect on WUE (p < 0.01). NTMP– had significantly greater WUE than other treatments (except for CTP– and NTP–). CTP–, CTMP–, CTMP, NTP–, NTMP had significantly greater WUE than other treatments (except for NTMP–). CTP+ had significantly greater WUE than CTP and NTP.
Figure 3. Correlation matrix between leaf area index (LAI), Plant height, Yield, Dry weight, relative feed value (RFV), crude protein (CP), neutral detergent fiber (NDF), acid detergent fiber (ADF) and 0–20 cm soil mass water content (SWC_Layer1), 20–40 cm soil mass water content (SWC_Layer2), 40–60 cm soil mass water content (SWC_Layer3). Confidence interval of 95%.
Yield had a highly significant positive correlation with dry matter yield, plant height, LAI and RFV (p < 0.001; Figure 4); as well as a highly significant negative correlation with CP, SWC of the three soil layers (p < 0.001) ADF (p < 0.05). Dry matter yield had a highly significant positive correlation with plant height, LAI, RFV (p < 0.001); CP, NDF, ADF, SWC at 0–20 cm soil layer and 40–60 cm soil layer (p < 0.001); SWC at 20–40 cm soil layer (p < 0.01). Plant height was highly significantly and positively correlated with LAI (p < 0.001) and RFV (p < 0.05), with a highly significant negative correlation with CP, SWC of the three soil layers (p < 0.001), and with ADF. LAI had a highly significant negative correlation with CP, SWC across three soil layers (p < 0.001; Figure 4), and a significant positive correlation with NDF (p < 0.05). CP had a highly significant positive correlation with ADF, SWC (p < 0.001), and a more significant negative correlation with RFV (p < 0.01). NDF had a highly significant positive correlation with ADF (p < 0.001), a highly significant negative correlation with RFV (p < 0.001), and a more significant negative correlation with SWC at the 0–20 cm soil layer (p < 0.01). ADF had a highly significant negative correlation with RFV (p < 0.001) and a significant negative correlation with SWC at 0–20 cm layer (p < 0.05).
Figure 4. The water use (WU) and water use efficiency (WUE) in different treatment in the study area in 2021. Conventional tillage (CT), no-tillage (NT), cover crop mulching (M), increased precipitation (P+), decreased precipitation (P–) and normal precipitation (P).
Soil moisture is one of the main factors limiting maize yield (Wang et al., 2022). Low precipitation in spring and high soil water evaporation in summer are the main reasons for low water use efficiency and yield reduction in maize production (Liu et al., 2013). In this study, we found that soil moisture content in the 0–40 cm soil layer was significantly higher in all CT treatments than in NT, which is consistent with the findings of Kitonyo et al. (2018). This may be due to the greater compaction and compromised preferential flows, which reduce water infiltration (Kitonyo et al., 2018), while the loose soil under conventional tillage increases the opportunity for water infiltration into the soil (Guto et al., 2012). However, the effect of CT did not extend to the 40–60 cm soil layer, and apparently, its effect on soil moisture content may only occur in the tillage layer due to the existence of plowpan layer. The effect of tillage on soil moisture content is controversial, and although some studies suggest that no-till has a positive effect on soil water content (Liu et al., 2013; Wang et al., 2022), others have shown that tillage practices do not make significant differences in SWC (Zhang et al., 2018). The effect of NT on soil moisture content is influenced by other factors, such as crop type, climatic conditions, and fertilizer application (Wang et al., 2018). In addition, it is undeniable that the shorter experiment duration (2 years of implementation of conservation tillage practices in this study) might cause limited differences between treatments; as some soil properties (e.g., physical structure of the soil, hydrological properties) requires much longer implementation time to be detected (Wang et al., 2018).
In the 0–20 and 20–40 cm soil layers of the maize seedling stage, the soil moisture content under cover crop mulch was found to be significantly higher than that of the no-mulch treatment. There was also a significant effect for the two soil layers at 20–60 cm depth for the total growth period. This is consistent with the results reported by Zhang et al. (2008) in the Loess Plateau. Meanwhile, in the normal precipitation treatments, the WUE of treatments containing cover crop mulch were all significantly higher than those without mulch, which is consistent with the results of Peng et al. (2020). This suggests that cover crop mulching can reduce soil moisture evaporation to some extent (Chen et al., 2020), enhance precipitation harvesting (Shao et al., 2016), and increase soil moisture infiltration, thus improving soil moisture content and moisture use efficiency (Yang et al., 2016). At the same time, cover crop mulch can form a physical barrier to block solar radiation, which can regulate soil temperature and facilitate plant root growth and moisture nutrient uptake (Wang et al., 2022). In addition, it helps to increase the source of soil organic carbon and indirectly improves the water retention of the soil (Rawls et al., 2003).
Precipitation is a factor that directly affect soil water content and water consumption of maize. In this study, it was found that the precipitation reduction treatment resulted in a significant decrease in SWC in all soil layers at 0–60 cm depth in all fertility periods of maize compared to normal precipitation. This indicates that the precipitation reduction affects the soil moisture content throughout the maize reproductive period. It is worth noting that although there was a positive effect of the precipitation increase treatment on SWC in each soil layer at 0–60 cm depth compared to the precipitation decrease treatment, the effect was not significant for the two soil layers at 0–40 cm depth at the seedling and fruiting stages. This may be due to the seasonal distribution of precipitation in the study area, which is limited during the seedling and jointing stages, and thus the effect of precipitation augmentation is not significant. Compared to normal precipitation, precipitation augmentation treatments did not significantly increase soil moisture content. This may be related to the particular geomorphology of karst areas, where precipitation water typically move as subsurface seepage on the soil surface rather than infiltration water (Gao et al., 2022). Unlike soil water content, rainfall increase did not significantly increase maize water use efficiency. This is consistent with the conclusion of Lai et al. (2022) indicating that the increase in precipitation is detrimental to water use efficiency. This study also found that no-till treatments significantly increased WUE at reduced rainfall levels under mulching conditions. This may be due to the greater evaporation from conventional tillage (Peng et al., 2020), compared to no-till mulching which greatly reduces evaporation loss under extreme dry weather.
Biomass accumulation is the basis for yield production (Peng et al., 2020). Three factors, precipitation variability, tillage and mulch, contribute to the differences in silage maize biomass at different growth stages. The low temperature effect of no-till affects maize emergence and early growth (Lal et al., 2004). Thus, compared with CT, NT accumulated less biomass in the early stage of maize reproduction (seedling to big trumpet stage) and gradually increased biomass accumulation in the late stage of reproduction (tasseling and lactation). In this study, we found that the NTSP+ treatment maintained high biomass at the seedling stage despite mulching and increased precipitation, suggesting that straw mulching and ample precipitation might mitigate or even negate the negative effects of NT on maize productivity (Peng et al., 2020). However, no significant differences were found between NTSP and NTSP+ at the tasseling and milk stages, which is consistent with the results of Orfanou et al. (2019), suggesting that increasing moisture input during maize reproductive stage is less effective for increasing overall biomass production.
The results of studies on the effect of tillage on crop yield are inconsistent. Vita et al. (2007) showed that wheat yields were higher with no-till treatments than with conventional tillage. A meta-analysis by Wang et al. (2018) in northwestern and northern China showed that NT had no effect on maize yield, and even led to yield reduction without crop rotation. In this study, combining cover crop and precipitation variation based on previous tillage studies, we found that T, SM, and P all had an effect on yield, and the interaction effect of the three was significant. This is in agreement with Zhang, Q., et al. (2022b) that efficiency gains in maize yield from no-till can also occur under a combination of mulch and appropriate precipitation conditions. Apparently, the effects of tillage on dry matter yield, plant height, and LAI provide additional contributions to explain yield differences, as evidenced by their significant correlation (Yerli et al., 2023). Interestingly, CP was significantly and negatively correlated with yield, plant height, and LAI. This may be caused by the negative correlation between crop senescence level and CP concentration.
Through the above analysis, this study concluded that the effect contributed by cover crop and precipitation variation cannot be ignored for maize production. For example, Wang et al. (2020) found that maize yield increased and then decreased with increasing growing season and precipitation, and high maize yield occurred when the precipitation was 300–500 mm. Too much or too little precipitation causes moisture stress in the crop, resulting in lower yield (Ren et al., 2008; Jia et al., 2018). In this study, there was no benefit in yield, plant height or leaf area with the increased precipitation. This is consistent with the finding that yield and related physiological parameters are negatively correlated with soil moisture content especially when soil moisture level exceeds certain threshold (Rusinamhodzi et al., 2011). This could also be due to the special geological characteristics of karst areas, where high precipitation and soil infiltration capacity are bound to carry more nutrients, which may be detrimental to crop growth (Gao et al., 2022), which contradicts with rainfed agriculture in arid and semi-arid regions (Shao et al., 2016). Similarly, this nutrient loss is not conducive to CP accumulation, and thus rain-fed treatments significantly reduced CP content (Li et al., 2020). Nevertheless, a significant positive correlation between soil moisture and CP was found in this study. This is well explained by the accumulation process of CP, which is mainly dependent on the N uptake by roots, and adequate soil moisture content is very favorable for the growth of early plant root systems and adequate soil water solutions for supplying inorganic N (Bi et al., 2020). Chen et al. (2020) suggested that mulching reduces soil temperature and delays plant development. In contrast, this study found that mulching had significant positive effects on yield, dry matter content, and CP. This is consistent with the findings of Kerbouai et al. (2022) in wheat. On one hand, this may be due to the fact that the cover crop in this study was ryegrass, which was high in protein content and decompose quickly after termination. On the other hand, the highly fibrous roots and dense canopy of ryegrass greatly reduce the risk of nutrient loss through infiltration (Baets et al., 2011), and provide fast-decomposing organic matter with low C:N ratio upon termination and a better temperature and moisture environment for the maize root systems (Shao et al., 2016). For conservation tillage practices, we need to obtain longer-term data to further explore their effects on silage maize production under different precipitation variations. In addition, further exploration of soil nitrogen will help us better understand the relationship between forage quality and water input.
This study investigated the effects of precipitation variability and conservation tillage on soil moisture, maize yield and quality in karst areas. The results showed that increasing soil precipitation during the reproductive period did not significantly increase biomass and soil moisture content, but decreasing precipitation had significant effects on maize yield, CP content and soil moisture content at all soil levels. No-tillage had a significant effect on maize yield, dry matter yield, plant height and CP content. CT treatment significantly increased soil water content in the tillage layer (0–40 cm), while cover crop mulching only had an effect on maize yield and soil water content at the seedling stage. Under precipitation variation, the combination of no-till and cover crop mulch had a significant effect on enhancing maize yield, CP content and soil water content. Therefore, this study concluded that the combination of no-till and cover crop mulching is recommended to cope with the precipitation variability and soil conditions associated with the geomorphological characteristics of karst areas.
The original contributions presented in the study are included in the article/Supplementary material, further inquiries can be directed to the corresponding author.
ZL, LLN, and WFQ: conceptualization and data curation. LLN, WFQ, and QSM: formal analysis. ZL and CC: funding acquisition, project administration, and resources. LLN, WFQ, QSM, JP, LHT, and GX: investigation. ZL and YLY: methodology. ZL: supervision. LLN: validation and visualization. WFQ: writing—original draft. ZL, YLY, and CC: writing—review and editing. All authors contributed to the article and approved the submitted version.
This research was financed by the National Natural Science Foundation of China (32160337). Guizhou Provincial Key Technology R&D Program (Qian Ke He Zhi Cheng [2022] Yi Ban 106; Qian Ke He Zhi Cheng [2023] Yi Ban 473), the Special Natural Science Foundation of Guizhou University (Gui Da Zhuan Ji He Zi [2020]08) and the Guizhou Provincial Science and Technology Projects (QKHPTRC-CXTD [2022]011).
The authors declare that the research was conducted in the absence of any commercial or financial relationships that could be construed as a potential conflict of interest.
All claims expressed in this article are solely those of the authors and do not necessarily represent those of their affiliated organizations, or those of the publisher, the editors and the reviewers. Any product that may be evaluated in this article, or claim that may be made by its manufacturer, is not guaranteed or endorsed by the publisher.
The Supplementary material for this article can be found online at: https://www.frontiersin.org/articles/10.3389/fsufs.2023.1198649/full#supplementary-material
Ahmad, I., Yan, Z. G., Kamran, M., Ikram, K., Ghani, M. U., and Hou, F. J. (2022). Nitrogen management and supplemental irrigation affected greenhouse gas emissions, yield and nutritional quality of fodder maize in an arid region. Agric. Water Manag. 269:107650. doi: 10.1016/j.agwat.2022.107650
Baets, S. D., Poesen, J., Meersmans, J., and Serlet, L. (2011). Cover crops and their erosion-reducing effects during concentrated flow erosion. Catena 85, 237–244. doi: 10.1016/j.catena.2011.01.009
Bai, L. Z., Kong, X. Y., Li, H., Zhu, H. B., Wang, C. G., and Ma, S. A. (2022). Effects of conservation tillage on soil properties and maize yield in karst regions, Southwest China. Agriculture (Basel) 12:1449. doi: 10.3390/agriculture12091449
Bi, W. X., Wang, M. K., Weng, B. S., Yan, D. H., Yang, Y. H., and Wang, J. J. (2020). Effects of drought-flood abrupt alternation on the growth of summer maize. Atmosphere 11:21. doi: 10.3390/atmos11010021
Calviño, P. A., Andrade, F. H., and Sadras, V. O. (2003). Maize yield as affected by water availability, soil depth, and crop management. Agron. J. 95, 275–281. doi: 10.2134/agronj2003.2750
Chen, S. Y., Zhang, X. Y., Shao, L. W., Sun, H. Y., Niu, J. F., and Liu, X. W. (2020). Effects of straw and manure management on soil and crop performance in North China plain. Catena 187:104359. doi: 10.1016/j.catena.2019.104359
Chen, L., Zhang, J. B., Zhao, B. Z., Yan, P., Zhou, G. X., and Xin, X. L. (2014). Effects of straw amendment and moisture on microbial communities in Chinese fluvo-aquic soil. J. Soils Sediments 14, 1829–1840. doi: 10.1007/s11368-014-0924-2
Clay, D. E., Alverson, R., Johnson, J. M. F., Karlen, D. L., Clay, S., Wang, M. Q., et al. (2019). Crop residue management challenges: a special issue overview. Agron. J. 111, 1–3. doi: 10.2134/agronj2018.10.0657
Dikgwatlhe, S. B., Chen, Z. D., Lal, R., Zhang, H. L., and Chen, F. (2014). Changes in soil organic carbon and nitrogen as affected by tillage and residue management under wheat-maize cropping system in the North China plain. Soil Tillage Res. 144, 110–118. doi: 10.1016/j.still.2014.07.014
Gao, Z. C., Xu, Q. X., Si, Q., Zhang, S. P., Fu, Z. Y., and Chen, H. S. (2022). Effects of different straw mulch rates on the runoff and sediment yield of young Citrus orchards with lime soil and red soil under simulated rainfall conditions in Southwest China. Water 14:1119. doi: 10.3390/w14071119
Guto, S. N., Pypers, P., Vanlauwe, B., Ridder, N., and Giller, K. E. (2012). Socio-ecological niches for minimum tillage and crop-residue retention in continuous maize cropping Systems in Smallholder Farms of Central Kenya. Agron. J. 104, 188–198. doi: 10.2134/agronj2010.0359
Herout, M., Koukolíček, J., Kincl, D., Pazderů, K., Tomášek, J., Urban, J., et al. (2018). Impacts of technology and the width of rows on water infiltration and soil loss in the early development of maize on sloping lands. Plant Soil Environ. 64, 498–503. doi: 10.17221/544/2018-PSE
Jia, Q. M., Chen, K. Y., Chen, Y. Y., Ali, S., Manzoor, S., Sohail, A., et al. (2018). Mulch covered ridges affect grain yield of maize through regulating root growth and root-bleeding sap under simulated rainfall conditions. Soil Tillage Res. 175, 101–111. doi: 10.1016/j.still.2017.08.017
Jia, L. Z., Zhao, W. W., Zhai, R. J., Liu, Y., Kang, M. M., and Zhang, X. (2019). Regional differences in the soil and water conservation efficiency of conservation tillage in China. Catena 175, 18–26. doi: 10.1016/j.catena.2018.12.012
Jiang, Z. C., Lian, Y. Q., and Qin, X. Q. (2014). Rocky desertification in Southwest China: impacts, causes, and restoration. Earth-Sci. Rev. 132, 1–12. doi: 10.1016/j.earscirev.2014.01.005
Kerbouai, I., M'hamed, H. C., Jenfaoui, H., Riahi, J., Mokrani, K., Jribi, S., et al. (2022). Long-term effect of conservation agriculture on the composition and nutritional value of durum wheat grains grown over 2 years in a Mediterranean environment. J. Sci. Food Agric. 102, 7379–7386. doi: 10.1002/jsfa.12105
Kitonyo, O. M., Sadras, V. O., Zhou, Y., and Denton, M. D. (2018). Nitrogen fertilization modifies maize yield response to tillage and stubble in a sub-humid tropical environment. Field Crop Res. 223, 113–124. doi: 10.1016/j.fcr.2018.03.024
Lai, X. F., Shen, Y. Y., Wang, Z. K., Ma, J. Y., Yang, X. L., and Ma, L. S. (2022). Impact of precipitation variation on summer forage crop productivity and precipitation use efficiency in a semi-arid environment. Eur. J. Agron. 141:126616. doi: 10.1016/j.eja.2022.126616
Lal, R., Griffin, M., Apt, J., Lave, L., and Morgan, M. G. (2004). Managing Soil Carbon. Science 304:393. doi: 10.1126/science.1093079
Lampurlanés, J., Plaza-Bonilla, D., Álvaro-Fuentes, J., and Cantero-Martínez, C. (2016). Long-term analysis of soil water conservation and crop yield under different tillage systems in Mediterranean rainfed conditions. Field Crop Res. 189, 59–67. doi: 10.1016/j.fcr.2016.02.010
Li, Z., Cui, S., Zhang, Q. P., Xu, G., Feng, Q. S., Chen, C., et al. (2022). Optimizing Wheat Yield, Water, and Nitrogen Use Efficiency With Water and Nitrogen Inputs in China: A Synthesis and Life Cycle Assessment. Front. Plant Sci. 13:930484. doi: 10.3389/fpls.2022.930484
Li, Y. Z., Song, D. P., Dang, P. F., Wei, L., Qin, X. L., and Siddique, K. H. M. (2020). Combined ditch buried straw return technology in a ridge–furrow plastic film mulch system: implications for crop yield and soil organic matter dynamics. Soil Tillage Res. 199:104596. doi: 10.1016/j.still.2020.104596
Liu, S., Zhang, X. Y., Yang, J. Y., and Drury, C. F. (2013). Effect of conservation and conventional tillage on soil water storage, water use efficiency and productivity of corn and soybean in Northeast China. Acta Agric. Scand. Sect. B-Soil Plant Sci. 63, 383–394. doi: 10.1080/09064710.2012.762803
Norris, J., Chen, G., and Li, C. (2020). Dynamic amplification of subtropical extreme precipitation in a warming climate. Geophys. Res. Lett. 47:2020GL087200. doi: 10.1029/2020GL087200
Orfanou, A., Pavlou, D., and Porter, W. M. (2019). Maize yield and irrigation applied in conservation and conventional tillage at various plant densities. Water. 11:1726. doi: 10.3390/w11081726
Peng, Z. K., Wang, L. L., Xie, J. H., Li, L. L., Coulter, J. A., Zhang, R. Z., et al. (2020). Conservation tillage increases yield and precipitation use efficiency of wheat on the semi-arid loess plateau of China. Agric. Water Manag. 231:106024. doi: 10.1016/j.agwat.2020.106024
Rawls, W. J., Pachepsky, Y. A., Ritchie, J. C., Sobecki, T. M., and Bloodworth, H. (2003). Effect of soil organic carbon on soil water retention. Geoderma 116, 61–76. doi: 10.1016/S0016-7061(03)00094-6
Ren, X. L., Jia, Z. K., and Chen, X. L. (2008). Rainfall concentration for increasing corn production under semiarid climate. Agric. Water Manag. 95, 1293–1302. doi: 10.1016/j.agwat.2008.05.007
Rusinamhodzi, L., Corbeels, M., van Wijk, M. T., Rufino, M. C., Nyamangara, J., and Giller, K. E. (2011). A meta-analysis of long-term effects of conservation agriculture on maize grain yield under rain-fed conditions. Agron. Sustain. Dev. 31, 657–673. doi: 10.1007/s13593-011-0040-2
Shao, Y. H., Xie, Y. X., Wang, C. Y., Yue, J. Q., Yao, Y. Q., Li, X. D., et al. (2016). Effects of different soil conservation tillage approaches on soil nutrients, water use and wheat-maize yield in rainfed dry-land regions of North China. Eur. J. Agron. 81, 37–45. doi: 10.1016/j.eja.2016.08.014
Turmel, M. S., Speratti, A., Baudron, F., Verhulst, N., and Govaerts, B. (2015). Crop residue management and soil health: a systems analysis. Agric. Syst. 134, 6–16. doi: 10.1016/j.agsy.2014.05.009
Vita, P. D., Paolo, E. D., Fecondo, G., and Fonzo, N. D. (2007). Pisante M. no-tillage and conventional tillage effects on durum wheat yield, grain quality and soil moisture content in southern Italy. Soil Tillage Res. 92, 69–78. doi: 10.1016/j.still.2006.01.012
Wang, Z., Sun, J., Du, Y. D., and Niu, W. Q. (2022). Conservation tillage improves the yield of summer maize by regulating soil water, photosynthesis and inferior kernel grain filling on the semiarid loess plateau. China. J. Sci. Food Agric. 102, 2330–2341. doi: 10.1002/jsfa.11571
Wang, P. J., Wu, D. R., Yang, J. Y., Ma, Y. P., Feng, R., and Huo, Z. G. (2020). Summer maize growth under different precipitation years in the Huang-Huai-Hai plain of China. Agric. For. Meteorol. 285–286:107927. doi: 10.1016/j.agrformet.2020.107927
Wang, Y. Q., Zhang, Y. H., Zhou, S. L., and Wang, Z. M. (2018). Meta-analysis of no-tillage effect on wheat and maize water use efficiency in China. Sci. Total Environ. 635, 1372–1382. doi: 10.1016/j.scitotenv.2018.04.202
Yang, H. S., Feng, J. X., Zhai, S. L., Dai, Y. J., Xu, M. M., Wu, J. S., et al. (2016). Long-term ditch-buried straw return alters soil water potential, temperature, and microbial communities in a rice-wheat rotation system. Soil Tillage Res. 163, 21–31. doi: 10.1016/j.still.2016.05.003
Yerli, C., Sahin, U., Ors, S., and Kiziloglu, F. M. (2023). Improvement of water and crop productivity of silage maize by irrigation with different levels of recycled wastewater under conventional and zero tillage conditions. Agric. Water Manag. 277:108100. doi: 10.1016/j.agwat.2022.108100
Zhang, P., Chen, X., Wei, T., Yang, Z., Jia, Z. K., Yang, B. P., et al. (2016). Effects of straw incorporation on the soil nutrient contents, enzyme activities, and crop yield in a semiarid region of China. Soil Tillage Res. 160, 65–72. doi: 10.1016/j.still.2016.02.006
Zhang, K. P., Li, Y. F., Wei, H. H., Zhang, L., Li, F. M., and Zhang, F. (2022a). Conservation tillage or plastic film mulching? A comprehensive global meta-analysis based on maize yield and nitrogen use efficiency. Sci. Total Environ. 831:154869. doi: 10.1016/j.scitotenv.2022.154869
Zhang, S. L., Lövdahl, L., Grip, H., Tong, Y. A., Yang, X. Y., and Wang, Q. J. (2008). Effects of mulching and catch cropping on soil temperature, soil moisture and wheat yield on the loess plateau of China. Soil Tillage Res. 102, 78–86. doi: 10.1016/j.still.2008.07.019
Zhang, Q., Wang, S. L., Sun, Y. G., Zhang, Y. H., Li, H. Y., Liu, P. Z., et al. (2022b). Conservation tillage improves soil water storage, spring maize (Zea mays L.) yield and WUE in two types of seasonal rainfall distributions. Soil Tillage Res. 215:105237. doi: 10.1016/j.still.2021.105237
Zhang, Y. J., Wang, S. L., Wang, H., Wang, R., Wang, X. L., and Li, J. (2018). Crop yield and soil properties of dryland winter wheat-spring maize rotation in response to 10-year fertilization and conservation tillage practices on the loess plateau. Field Crop Res. 225, 170–179. doi: 10.1016/j.fcr.2018.07.003
Keywords: conservation tillage, precipitation variability, maize, yield, soil moisture
Citation: Niu L, Qin W, You Y, Mo Q, Pan J, Tian L, Xu G, Chen C and Li Z (2023) Effects of precipitation variability and conservation tillage on soil moisture, yield and quality of silage maize. Front. Sustain. Food Syst. 7:1198649. doi: 10.3389/fsufs.2023.1198649
Received: 01 April 2023; Accepted: 10 July 2023;
Published: 24 July 2023.
Edited by:
Adnan Mustafa, Brno University of Technology, CzechiaReviewed by:
Xiao Sun, Nanjing Agricultural University, ChinaCopyright © 2023 Niu, Qin, You, Mo, Pan, Tian, Xu, Chen and Li. This is an open-access article distributed under the terms of the Creative Commons Attribution License (CC BY). The use, distribution or reproduction in other forums is permitted, provided the original author(s) and the copyright owner(s) are credited and that the original publication in this journal is cited, in accordance with accepted academic practice. No use, distribution or reproduction is permitted which does not comply with these terms.
*Correspondence: Zhou Li, emxpOEBnenUuZWR1LmNu
†These authors have contributed equally to this work and share first authorship
Disclaimer: All claims expressed in this article are solely those of the authors and do not necessarily represent those of their affiliated organizations, or those of the publisher, the editors and the reviewers. Any product that may be evaluated in this article or claim that may be made by its manufacturer is not guaranteed or endorsed by the publisher.
Research integrity at Frontiers
Learn more about the work of our research integrity team to safeguard the quality of each article we publish.