- 1Department of Food Sciences, Faculty of Animal Productions Engineering and Management, University of Agronomic Sciences and Veterinary Medicine of Bucharest, Bucharest, Romania
- 2Department of Biotechnologies, Bioengineering Faculty of Animal Resources, Banat University of Agricultural Science and Veterinary Medicine “King Michael I of Romania”, Timisoara, Romania
Introduction: One key factor contributing to microbial resistance is the deliberate and inappropriate use of antibiotics in human and animal health management. Recent studies point out various ways to tackle this controversy to mitigate the unnatural rapid evolution of pathogenic bacteria. Chicken meat remains at the top of Romanian consumers' preferences, being the most consumed type of meat, desired for its nutritional and dietetic attributes.
Methods: This research was conducted in 2022, aiming to evaluate the antibiotic residues (quinolones, aminoglycosides, tetracycline, and sulfonamides) in broiler chicken meat with various trade strategies [retail market (RM), n = 40, traditional market (TM), n = 185, and door-to-door vendors (DTD), n = 121] during two seasonal periods, spring (March–April) and summer (July–September). An efficient and precise protocol was employed for determining the meat organoleptic attributes, qualitative screening, and quantitative assay antibiotic of six antibiotics (enrofloxacin, marbofloxacin, streptomycin, oxytetracycline, doxycycline, and sulfamethoxazole), consumers' antibiotic exposure (estimated daily intake), and potential risk assessment (hazard quotient).
Results: The antibiotic quality assessment revealed an overall antibiotic residue presence in groups TM (75%) and DTD (82%), while no antibiotic residues were detected in the RM group. Our results show that 32% (n = 110) of the total chicken meat samples were free of antibiotic residues, 4.5% (n = 16) contained antibiotic residues belonging to one class of antibiotics, 40% (n = 139) had two antibiotics groups, 22% (n = 77) had three antibiotics groups, and 1.5% of the chicken meat samples presented four groups of antibiotics.
Discussion: During the spring season, the enrofloxacin antibiotic residue present in the meat samples was higher, showing an 84% presence in TM meat samples group when compared with the DTD meat sampled group (75%). The analysis data processing showed a strong correlation between the antibiotic residue's meat samples origin (trade market and door-to-door traded meat antibiotic residue variations) and seasonal variations. As a result of the hazard quotient assay, the meat antibiotic residue levels had subunit values, indicating the meat quality was proper for consumption. It is mandatory to strengthen the level of knowledge by continuously monitoring and providing updated information to each group of farmers to increase their understanding of and adherence to the proper handling of antibiotics when growing chickens. Regarding the use of prohibited growth-promoting antibiotics in chicken-rearing systems, local authorities should increase the guard level, at antibiotic supplier and end user levels.
1. Introduction
Chicken meat has been by far the most appreciated dietary animal protein among Romanian consumers (Balan et al., 2022), placing the poultry sector on top of the meat-producing industries in the last year, with around 550kt of meat sold (as carcass) (INSSE, 2023). Moreover, the chicken meat-producing systems' trend toward environmentally friendly and high-quality products (Barbut and Leishman, 2022), while recirculating waste and increasing productivity (Boumans et al., 2022), has also increased their popularity. However, extrinsic quality parameters (production, processing, and marketing) present a major role in perception, expectation, and consumers' purchase decisions. The Romanian consumer's purchase decision behavior (in the retail market and out of it) regarding poultry meat quality is affected by two main factors, first is the employed farming techniques (Pirvutoiu and Popescu, 2013) and the second is the affordability of meat price (Caratus Stanciu, 2020). Poultry meat that originates from small-size farming (semi-intensive rearing systems) is often merchandised in traditional markets and local fairs at a much higher price than conventionally reared chickens, having a specific customer-targeted group of young people and the elderly (Voinea et al., 2020) due to their high-quality attributes (Grigore et al., 2023). Another available marketing source of broiler chicken meat is individual producers either via door-to-door vendors or internet advertisement, generally offering “home-made” chicken meat (extensive reared system). While conventional, semi-intensive, or extensive farming systems are facing common challenges such as prolonged infectious threats (Pandey and Kumar, 2021), it is difficult to establish the boundary between welfare, health safety practices, and consumer risks (Berg, 2001). A common practice, often employed in broiler rearing systems, is antibiotic meta phylactic treatments against seasonal infectious bronchitis and viruses (de Mesquita Souza Saraiva et al., 2022). Antibiotic usage continues to be the most cost-effective measure while guaranteeing welfare principles and intervention effectiveness (Nanda et al., 2022). In broiler rearing systems, antibiotic drugs [aminoglycosides (streptomycin) (Mak et al., 2022), sulfonamides (sulfamethoxazole) (Divala et al., 2022), quinolones (enrofloxacin, marbofloxacin) (Haeili et al., 2022), and tetracycline (oxytetracycline and doxycycline) (Duga, 2018)] are approved by the EU and USA (Mader et al., 2022) and are included in the farm health protocol management. Moreover, antibiotic utilization in chickens is strictly regulated, for a therapeutic and preemptive purpose only (Mader et al., 2022), and slaughtering is permitted only after the antibiotic withdrawal period. Current data points out the antibiotic residue in meat and other poultry products (Ramatla et al., 2017; Oyedeji et al., 2019; Verma et al., 2020; Mak et al., 2022; Mohammadzadeh et al., 2022; Fei et al., 2023). The widespread curative and preemptive usage of antibiotics (quinolone, aminoglycosides, tetracyclines, and sulfonamides) for both humans and livestock, as sole active bacteriostatic agents, are contributing to the antimicrobial resistance phenomenon, with implications on the environment and human health (Gržinić et al., 2023). Nowadays, antibiotic resistance infections are more prevalent and often associated with high morbidity (Srisuwananukorn et al., 2021) and mortality (1.27 million cases globally) (Murray et al., 2022) among both adults and infants (de Kraker et al., 2016; Malik et al., 2019; Murray et al., 2022).
The current paper aims to increase public awareness and provide valuable supporting information for future policies to meat producers and consumers about the effects of antibiotic administration practices leading to accelerated extensive antibiotic resistance, thus contributing to the vast and contra-balanced health risks associated with infections.
The main objective was to evaluate the chicken-meat antibiotic residue content, group-specific antibiotic residue content, and consumers' antibiotic exposure (antibiotic-estimated daily intake) and hazard quotient.
2. Materials and methods
2.1. Chicken meat sampling
Chicken meat was sampled from March to April 2022 and July to September 2022, in twelve different areas (Figure 1) of Romania (n = 6 cities, and their rural surroundings), collecting a total of 348 chicken breast meat samples (Figure 2), from different provenances: 12% (n = 42) from commercial center origin, such as retail markets (RM, based on conventional intensive farming systems, sold in hypermarkets and supermarkets), 53% (n = 185) from traditional markets and local fairs (TM, individual producers or small-sized farms semi-intensive rearing systems), and 35% (n = 121) from door-to-door vendors via the internet market origin [DTD, exclusively extensive production systems (individual agricultural holdings, authorized as family farms, merchandising via the internet]. The collected breast meat samples were individually weighed (1 ± 0,120kg/sample), with a technical balance (Axis AZT 320, Poland), and evaluated for water content (SR ISO 1442:2010). The organoleptic evaluation of the chicken meat samples (ISO 5492:2008) was conducted, with nine naïve assessors (ISO 8586:2023), mixed-sexes, aged between 23 ± 2 years old, evaluated for the traded raw meat organoleptic standardized characteristics: carcass appearance, meat aroma, meat odor, meat consistency, and meat juiciness (EC 543/2008). There is a 1–5 hedonic scale response as for consumers purchasing choice (1 – unpleasant, 2 – satisfactorily, 3 – neither pleasant nor unpleasant, 4 – pleasant, 5 – very pleasant).
2.2. Meat antibiotic residue qualitative assessment
The qualitative screening methodology (de Kraker et al., 2016) employed the detection of antibiotic and sulfamide residues through their direct inhibitory action against Geobacillus stearothermophilus (GS). GS is an important bioindicator for the presence of antibiotics in meat and milk samples. The analysis protocol was previously developed by Liofilchem (2011), focusing on the meat antibiotic residues inhibition on the GS growth. The inoculation takes place with preincubation (30 min at 20°C) of the mixture meat extract-GS media. The quick germination and proliferation of the GS is expressed at the final step of incubation (at 64 ± 0.4°C, during 3.5h). Due to the sensitivity of Geobacillus stearothermophilus to antimicrobial agents, such as beta-lactams, tetracyclines, macrolides, lincosamides, aminoglycosides, sulphamides, aminoglycosides, sulphamides, sulfanilamides, benzyl pyrimidine, and quinolones, it is commonly used in veterinary medicine and with relevant MRL (Maximum Residue Limits) ranges valid in Europe – regulation 37/2010 EC.
2.3. Chemicals, reagents, and apparatus
The purified water (18,2MΩ cm, Adrona SIA CB-2303, Latvia), meat antibiotic residue quick detection test (MeRA test, Liofilchem Diagnostici, Roseto, Italy), the analytic grade reagents such as methanol, acetonitrile, dimethyl sulfoxide (Merk KGaA, Darmstadt, Germany), and ammonium acetate, and the antibiotic standard reference enrofloxacin (ENR)marbofloxacin (MAR), streptomycin sulfate (STM), oxytetracycline (OTC), doxycycline (DOX), and sulfamethoxazole (SXT), were provided by the University of Agronomic Sciences and Veterinary Medicine for Bucharest, the Faculty of Animal Productions Engineering and Management, Food Industry Department.
2.4. Apparatus instrumental conditions and software
The HPLC-ELSD meat antibiotic residue analysis was performed using the Agilent 1260 Infinity II system. The Agilent 1260 Infinity II G7104C Flexible pump, Agilent 1260 Infinity II G7129 vial autosampler, and Agilent 1260 Infinity II G7116A multicolumn thermostat were employed. Compound separation was achieved using the ZORBAX Eclipse Plus C18 column 4.6 mm × 75 mm × 3.5 μ (Agilent, USA). For instrumental, and chemical analyte detection was employed the Agilent 1260 Infinity II ELSD G4260 detector, followed by quantification tool system Agilent Open Lab CDS for LC and LC/MS. The mobile phase had three components: ultrapure water, acetonitrile, and Tri fluoric acid; 900: 99: 1, v/v/v. The flow rate was 200 μl/min−1, the sample injection volume was 2 μl, and the column temperature was 28°C. The auxiliary equipment was represented by a pH meter (WTW Multi 310, Germany), with an electrode (SenTix 41, WTW, Germany, stored in KCl 3M), a laboratory centrifuge (Boeco C28-A, Germany), vortex-mixer (Vortex Genie 2 mixer, USA), ultrasonic bath (Elmasonic S 50 R, Germany), and a rotary evaporator (Heidolph Rotary Evaporator Laborota 4000, Germany).
2.5. Meat sample chromatography preparation
The organoleptic quality appropriate meat samples were individually minced (using a conventional blender (Bosch, Hausgeräte GmbH, Germany) and weighed (10 ± 0.02 g) using an analytical balance (Kern ABJ 220–4M, Kerk & Sohn GmbH, Germany). An individual aliquot of each chicken meat sample was weighed (1 g), mixed with 2 g of anhydrous sodium sulfate, and saturated with 500 μl of acetonitrile. A repetitive double-cycle extraction in 25 ml acetonitrile was developed by ultrasonication for 20 min. The meat sample supernatants were collected in conical 50 ml Eppendorf tubes after the centrifugation (3000 rpm, 5 min) and frozen (−20°C) for lipid content removal. The meat sample products were filtered (0.45 nm, unsterile syringe filters) and finally concentrated by evaporation to 1 ml (45 °C, 200 mmHg, 80 rpm), and stored at −12°C, over 24 h, for further HPLC analysis.
2.6. Meat antibiotic quality assurance and quantitative assessment
Standard reference substances as powder or crystals [enrofloxacin (ENR, Fluka, China), marbofloxacin (MAR, Sigma Aldrich, Merk, Switzer-land), streptomycin sulfate (STR, Sigma Aldrich, Merk, Switzerland), oxytetracycline (OTC, European Pharmacopeia reference standard, Europe Council), doxycycline (DOX, Sigma Aldrich, Switzerland), and sulfamethoxazole (SMX, Merk, Italy)] were individually soluted as per producers' requirements, using the ultrasonication bath (15 min), reaching a final concentration of ~1 mg/ml. The quality assurance for the analytical procedure was developed for accuracy, precision, sensitivity limit of detection and of quantification, working range, selectivity and specificity, presence of antibiotic recovery, ruggedness and robustness, and possible interferences. A calibration curve was developed, for each antibiotic analyte, spiked in 0, 5, 10, 50, 100, 250, and 500 μg/ml, taking into consideration the maximum antibiotic residue limits (FAO, 2015). For the simultaneous antibiotic residue determination, an aliquot (100 μl) of each stock solution was diluted to a total volume of 900 μl (600 μl analytes mixture + 400 μl mobile phase).
2.7. Health risk assessment
The consumer's antibiotic exposure was assessed for each of the four classes of antibiotics: quinolones, aminoglycosides, tetracyclines, and sulfonamides. The daily intake of antibiotics was estimated based on the individual antibiotic concentration and the chicken breast meat average daily consumption (27.8 kg/capita/year) (FAO., 2020). The daily antibiotic dose ingested through chicken breast meat was calculated, with the formula [1].
EDI, Estimated antibiotic residues daily intake; ACR, the antibiotic residue concentration, in this study (μg/kg fresh meat); MDI, the mean of meat daily intake, expressed as kg/person; ABW, The adult consumer average body weight reference, 70 kg (Aggarwal et al., 2022).
After calculating the EDI, the hazard quotient was estimated using the calculation formula [2].
HQ, hazard quotient; EDI, Estimated antibiotic residue daily intake; ADI, Acceptable antibiotic residue daily intake (Medicines V., 2002; Assessment and Mebendazole, 2010; EMEA., 2015; FAO, 2015; Bahmani et al., 2020; Kyriakides et al., 2020); interpretation: if HQ < 1.0 – Acceptable, receptors are not exposed to the contaminant, if not (HQ > 1.0) – Not acceptable, the receptors are exposed to the contaminant.
2.8. Data processing and statistics
Agilent Open Lab CDS LC&LC/MS software (Agilent Technology Inc. USA) was used for processing and data acquisition, and the SPSS (version 25, IBM, USA) software was used for descriptive statistics and the Pearson correlation. The dimensionally reduction method of principal component analysis (PCA) was employed using the two components, F1 and F2, to explain all linear combinations with large variations.
3. Results and discussions
3.1. Organoleptic meat properties
At the start of the experiment (during the sampling procedure), all chicken breast meat samples (n = 348) were evaluated for organoleptic characteristics. Evaluating the organoleptic attributes of the broiler carcass and breast meat of TM and DTD groups did not indicate significant differences, except for two broiler carcasses belonging to the RM group. The two altered chicken carcasses also presented an unacceptable level of water addition, so the meat samples were excluded from the study, with the motivation of the meat quality being unfit for human consumption. The organoleptic characteristics of the chicken raw meat refer to the conditions that domestically produced poultry meat must meet to be marketed (Comunit C., 2008). Poorly and improperly processed meat has the potential to carry a zoonotic disease (Georganas et al., 2022), thus causing pathogenic contamination (French, 2023), and posing the highest risk for both human and animal health (Amore et al., 2022).
3.2. Meat antibiotic residue qualitative assessment
None of the 40 samples collected and analyzed originating from the retail market were confirmed positive for any category of antibiotics (Figure 3). Only nine chicken breast meat samples suspected of antibiotic residue presence were identified (false positive results) and kept for further quantitative antibiotic residue assessment. The results concerning the chicken breast meat samples from the TM group (during all experimental trials) presented an overall 75% (n = 137) antibiotic residue presence. Meanwhile, the total breast meat chicken collected from the DTD group (n = 22) showed a much higher antibiotic residue presence (82% of the DTD meat samples were positive for antibiotic residues). A potential explanation for the difference in antibiotic presence in the chicken breast meat samples' origin could be attributed to the antibiotic administration without respecting the drug withdrawal periods (Györke et al., 2013; Verma et al., 2020) and rushing the slaughtering protocols without considering the potential harmful effects. Generally, in extensive and intensive rearing management, the antibiotics have specific prophylaxis procedures, regarded as health-related mandatory actions, which are more focused on prevention and less therapeutic intervention, and strictly impose the drug withdrawal period before slaughtering (Mohammadzadeh et al., 2022), promoting product biosecurity and consumer safe.
3.3. Meat antibiotic quantitative assessment
The data presented in Table 1 highlight the most antibiotic-contaminated chicken breast meat was in the TM and DTD groups, and the antibiotic residues belonged to the quinolone antibiotics group, recommended for veterinary use and administered with restriction (EMA., 2019). Enrofloxacin was detected in 84% of samples from traditional markets and local fairs, in the first part of the year, with a maximum concentration of 12.80 μg/kg and a minimum concentration of 2.02 μg/kg. During the summer, the reported enrofloxacin residues in TM-origin broiler meat were higher (65% of total TM broiler meat samples) when compared with the DTD provenance meat samples (12%). Furthermore, in the spring season the enrofloxacin antibiotic residue present in the meat samples was higher, showing an 84% presence in the TM meat samples group when compared with the DTD meat samples group (75%). Current results point out the fact that among individual producers (DTD group), both the frequency of quinolone contamination and the maximum residue concentration of enrofloxacin is significantly lower when compared with the TM group. Regarding the variation of enrofloxacin concentration according to the months of the year, increased residue concentrations are found in chicken breasts sold in traditional markets and door-to-door in the colder months of the year, which also correspond to high atmospheric humidity when compared to the summer months. Similar situations have been reported internationally by other researchers (Panzenhagen et al., 2016; Moghadam et al., 2018; Kang et al., 2021; Foreign et al., 2022). Tetracycline residues were mainly detected in the spring months of the year, while oxytetracycline residues were detected in 42% of the total DTD-origin chicken breast meat samples during the spring season in 2022. The highest concentration of oxytetracycline was 11.20 μg/kg (spring season) and 8.02 μg/kg (summer season), with an average concentration of 9.83 μg/kg chicken breast from the DTD group in the spring and 6.93 μg/kg in the summer months. Similar results to our findings were previously reported by Salama et al. (2011) and Bahmani et al. (2020). Moreover, cross-contamination has been reported (Figure 4), indicating a multi-drug therapeutic intervention, although most of the antibiotics such as tetracyclines and aminoglycosides (Li, 2022) share the same group of targeted pathogens, so no justification could be attributed, and thus antibiotics are still used for the growth promotive effects. However, in this study, the largest group of antibiotic contaminants was sulfonamides (SMX). During the spring months, almost 82% of the total meat samples of the DTD group presented SMX contamination (7.15–18.20 μg/kg), followed by the TM-origin chicken breast meat samples (5.80–7.88 μg/kg) in the same seasonal conditions. SMX is a synthetically developed substance with broad bacteriostatic action against susceptible bacteria, interfering directly in their folic acid synthesis. In the current study, the maximum concentrations of antibiotic residues detected (ENR, MBX, STR, OTC, DOX, and SMX) in the broiler breast meat samples (Table 1) for TM and DTD in spring and summer conditions did not exceed the regimented limits (Europene CU., 2006).
3.4. Health risk assessment
The antibiotic residue contaminant exposure of the population was assessed for each of the four classes of antibiotics: quinolones, aminoglycosides, tetracyclines, and sulfonamides. The daily intake of antibiotics was calculated based on the concentration of antibiotics in the chicken meat consumed, and the daily consumption of chicken breast (27.8 kg/capita/year). The current results were compared with the MRL values (Table 2), and no analyzed chicken breast meat sample exceeded the MRL values, indicating the meat is safe and suitable for human consumption. The estimated maximum daily intake (Table 3) for the antibiotic analytes ranged from 2.932 ng/kg-bw (body weight)/day of MBX in summer to 194.544 ng/kg-bw/day of STR for adults during the summer period. However, chicken meat is often recommended for consumption to children of all ages, starting at 6 months of age, although safe limits for antibiotic residues in foods consumed by children are not currently available. This might represent a considerable risk due to children's immune system issues and gastric imbalances. An avoidance strategy might be represented by approaching a diet with a diverse range of protein sources that meets the daily nutritional requirements for children. The hazard index had a subunit value (Table 3) in adults, which is why we can say that the detected levels of antibiotic residues in the chicken breast could not be considered a public health threat regarding these veterinary antimicrobial substances presence (Oyedeji et al., 2019). Recent studies in China (Fei et al., 2023) and Greece (Stavroulaki et al., 2022) present similar results concerning consumer safety and chicken meat antibiotic contaminants exposure.
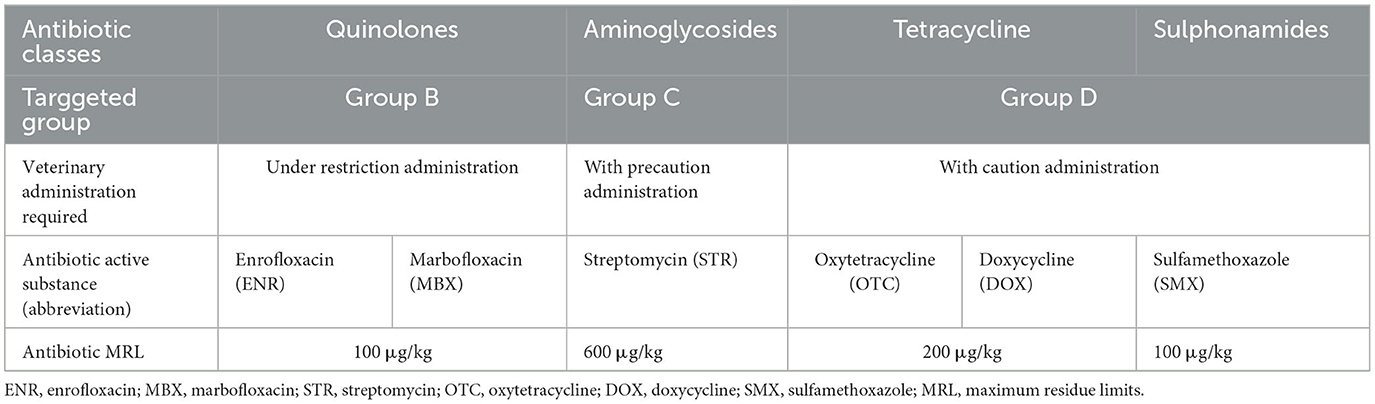
Table 2. Maximum residue limits of antibiotics (Salama et al., 2011).
3.5. Correlations
The total variance (97.86%) is explained by the PCA analysis plot (Figure 5) (F1= 16.01% and F2 = 81.85%). Strong correlations were obtained between the meat antibiotic residues among the studied trade systems and seasonal differences. The residues of ENR – DOX (groups 1, 5, 7, 11, 13, 17, and 19) and OTC - SMX (groups 4, 6, 10, 12, 16, 18, 20, and 22) were clustered and might represent a cause of similar antibiotic administration among both commercial groups in both seasons. The STR antibiotic residue meat presence plotted dispersion appears different from all other antibiotic residues (Verma et al., 2020; Pandey and Kumar, 2021; Barbut and Leishman, 2022; Haeili et al., 2022), suggesting their targeted administration strategy among the studied antibiotic residue groups have low levels on the studied meat traded samples. The analysis processing data showed the correspondence among the antibiotic residue's meat samples origin (trade market and door-to-door traded meat antibiotic residues variations) and the seasonal variations (Table 4). The first dimension (F1) explains 81.85% and the second one (F2) 16.10% of the total inertia.
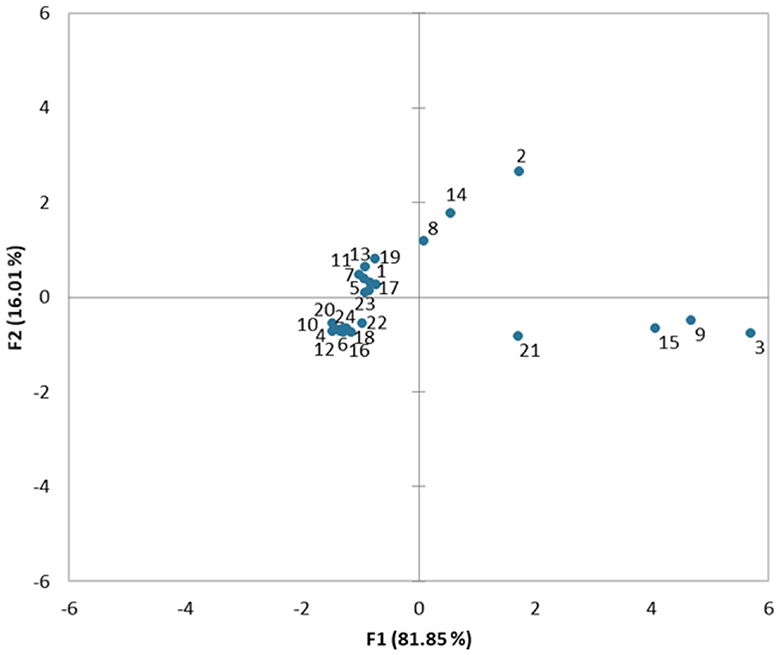
Figure 5. Principal component analysis plot. Traditional market trade meat: 1, Enrofloxacin residues encountered during spring; 2, Marbofloxacin residues encountered during spring; 3, Streptomycin residues encountered during spring; 4, Oxytetracycline residues encountered during spring; 5, Doxycycline residues encountered during spring; 6, Sulfamethoxazole residues encountered during spring; 7, Enrofloxacin residues encountered during summer; 8, Marbofloxacin residues encountered during summer; 9, Streptomycin residues encountered during summer; 10, Oxytetracycline residues encountered during summer; 11, Doxycycline residues encountered during summer; 12, Sulfamethoxazole residues encountered during summer. Door-to-door via Internet traded meat: 13, Enrofloxacin residues encountered during spring; 14, Marbofloxacin residues encountered during spring; 15, Streptomycin residues encountered during spring; 16, Oxytetracycline residues encountered during spring; 17, Doxycycline residues encountered during spring; 18, Sulfamethoxazole residues encountered during spring; 19, Enrofloxacin residues encountered during summer; 20, Marbofloxacin residues encountered during summer; 21, Streptomycin residues encountered during summer; 22, Oxytetracycline residues encountered during summer; 23, Doxycycline residues encountered during summer; 24, Sulfamethoxazole residues encountered during summer.
4. Conclusions
Chicken meat antibiotic residues are a current global challenge and present severe consequences concerning consumer health safety. In our study, the chicken meat from traditional markets and local fairs and individual producers selling via the internet contained more antibiotic residues when compared with the meat from retail markets, most probably because individual farmers' antibiotic resistance awareness is still quite low. Generally, more than 40% of total analyzed chicken meat samples were positive for two classes of antibiotics, most of them belonging to the traditional markets and local fairs merchandised group. The higher antibiotic contamination rate in samples taken from small-size farms and individual farmers suggest a need for a more rigorous control of drugs and antibiotics. Moreover, our results proved that local individual and small-size farming has increased the need for knowledge and education about antibiotic drugs used to ensure prudent approaches toward preventing health hazards and ensuring durable and sustainable meat products. There is a lack of information and national and local level protocols and campaigns about proper antibiotic management and respecting the withdrawal periods concerning consumer safety.
Data availability statement
The original contributions presented in the study are included in the article/supplementary material, further inquiries can be directed to the corresponding authors.
Author contributions
EP: conceptualization, methodology, and investigation. D-MG: writing—original draft, investigation, and software. DI, DP, and GB: investigation. DP and IP: validation. IP, ND, and D-MG: conceptualization, writing—review and editing, and supervision. All authors contributed to the article and approved the submitted version.
Funding
This paper was funded by the USAMV Bucharest Internal Research Projects Competition, 2021, Contract No. 2021-003/30.07.2021—Assessment of consumer exposure to antibiotics coming from animal products in Romania.
Conflict of interest
The authors declare that the research was conducted in the absence of any commercial or financial relationships that could be construed as a potential conflict of interest.
Publisher's note
All claims expressed in this article are solely those of the authors and do not necessarily represent those of their affiliated organizations, or those of the publisher, the editors and the reviewers. Any product that may be evaluated in this article, or claim that may be made by its manufacturer, is not guaranteed or endorsed by the publisher.
References
Aggarwal, R., Bibbins-Domingo, K., Yeh, R. W., Song, Y., Chiu, N., Wadhera, R. K., et al. (2022). Diabetes screening by race and ethnicity in the united states: equivalent body mass index and age thresholds. Ann. Intern. Med. 175, 765–773. doi: 10.7326/M20-8079
Amore, G., Boelaert, F., Gibin, D., Papanikolaou, A., Rizzi, V., Stoicescu, A., et al. (2022). Zoonoses and foodborne outbreaks guidance for reporting 2021 data. EFSA Support Publ. 19, 7131. doi: 10.2903/sp.efsa.2022.EN-7131
Assessment, R., and Mebendazole, R. (2010). Food safety commission of japan risk assessment report Mebendazole (veterinary medicines). Food Safety Comm. Japan. 2010, 2–3.
Bahmani, K., Shahbazi, Y., and Nikousefat, Z. (2020). Monitoring and risk assessment of tetracycline residues in foods of animal origin. Food Sci. Biotechnol. 29, 441–448. doi: 10.1007/s10068-019-00665-x
Balan, I. M., Gherman, E. D., Gherman, R., Brad, I., Pascalau, R., Popescu, G., et al. (2022). Sustainable nutrition for increased food security related to romanian consumers' behavior. Nutrients 14, 892. doi: 10.3390/nu14224892
Barbut, S., and Leishman, E. M. (2022). Quality and processability of modern poultry meat. Animals 12, 766. doi: 10.3390/ani12202766
Berg, C. (2001). Health and welfare in organic egg production. Acta. Vet. Scand. 95, 37–45. doi: 10.1186/1751-0147-43-S1-S37
Boumans, I. J. M. M., Schop, M., Bracke, M. B. M., de Boer, I. J. M., Gerrits, W. J. J., Bokkers, E. A. M., et al. (2022). Feeding food losses and waste to pigs and poultry: Implications for feed quality and production. J. Clean Prod. 378, 134623. doi: 10.1016/j.jclepro.2022.134623
Caratus Stanciu, M. (2020). Research regarding consumers attitude, in relation with poultry meat purchase and consumption. case study Sibiu, Romania. Sci. Pap. Manag. Econ. Eng. Agric. Rural Dev. 20, 125–130.
Comunit C. (2008). Europene I. L 157/46. Available online at: https://eur-lex.europa.eu/legal-content/RO/TXT/HTML/?uri=CELEX:32008R0543 (accessed September 29, 2023).
de Kraker, M. E. A., Stewardson, A. J., and Harbarth, S. (2016). Will 10 million people die a year due to antimicrobial resistance by 2050? PLoS Med. 13, e1002184. doi: 10.1371/journal.pmed.1002184
de Mesquita Souza Saraiva, M., Lim, K., do Monte, D. F. M., Givisiez, P. E. N., and Alves, L. B. R. (2022). Antimicrobial resistance in the globalized food chain: a One Health perspective applied to the poultry industry. Braz. J. Microbiol. 53, 465–486. doi: 10.1007/s42770-021-00635-8
Divala, T. H., Lewis, J., Bulterys, M. A., Lutje, V., Corbett, E. L., Schumacher, S. G., et al. (2022). Missed opportunities for diagnosis and treatment in patients with TB symptoms: a systematic review. Pub. Health Action 12:10–17. doi: 10.5588/pha.21.0022
EMEA. (2015). Summary of the scientific discussion for the establishment of MRLs. Eur. Agency Eval. Med. Prod. Commit. Vet. Med. Prod. 44, 1–7.
Europene CU. (2006). Jurnalul oficial al uniunii europene. 2, 64–117. Available online at: https://eur-lex.europa.eu/legal-content/RO/TXT/HTML/?uri=CELEX:32009R0470 (accessed September 29, 2023).
FAO, OMS, CODEX. (2015). Maximum Residue Limits (MRLs) and Risk Management Recommendations (RMRs) for Residues of Veterinary Drugs in foods CAC/MRL 2-2015. Available online at: https://www.fao.org/fao-who-codexalimentarius/home/en/ (accessed September 29, 2023).
Fei, Z., Song, S., Gao, J., Song, Y., Xiao, X., Yang, X., et al. (2023). Antibiotic residues in chicken meat in China: occurrence and cumulative health risk assessment. J. Food Compos. Anal. 116, 105082. doi: 10.1016/j.jfca.2022.105082
Foreign, N., Investment, D., States, U., and Be, F. (2022). Federal Register. 87, 11–22. Available online at: https://www.govinfo.gov/content/pkg/FR-2022-09-29/pdf/2022-21290.pdf (accessed September 29, 2023).
French, N. (2023). “Chapter 57 - Whole-genome sequencing for food safety,” in Popping BBT-PK, eds M. E. Knowles, L. E. Anelich, A. R. Boobis (New York, NY: Academic Press), 854–870.
Georganas, A., Giamouri, E., Pappas, A. C., Papadomichelakis, G., Fortatos, S., Manios, T., et al. (2022). Redefining the future of catering waste application in animal diets – A review on the minimization of potential hazards in catering waste before application in animal diets. Anim. Feed Sci. Technol. 289, 115334. doi: 10.1016/j.anifeedsci.2022.115334
Grigore, D. M., Mironeasa, S., Ciurescu, G., Ungureanu-Iuga, M., Batariuc, A., Babeanu, N. E., et al. (2023). Carcass yield and meat quality of broiler chicks supplemented with yeasts bioproducts. Appl. Sci. 13, 1607. doi: 10.3390/app13031607
Gržinić, G., Piotrowicz-Cieślak, A., Klimkowicz-Pawlas, A., Górny, R. L., Ławniczek-Wałczyk, A., Piechowicz, L., et al. (2023). Intensive poultry farming: a review of the impact on the environment and human health. Sci. Total Environ. 858, 160014. doi: 10.1016/j.scitotenv.2022.160014
Györke, A., Pop, L., and Cozma, V. (2013). Prevalence and distribution of Eimeria species in broiler chicken farms of different capacities. Parasite 20, 52. doi: 10.1051/parasite/2013052
Haeili, M., Salehzeinali, H., Mirzaei, S., Pishnian, Z., and Ahmadi, A. (2022). Molecular characterization of quinolone resistance and antimicrobial resistance profiles of Klebsiella pneumoniae and Escherichia coli isolated from human and broiler chickens. Int. J. Environ. Health Res. 32, 1382–1392. doi: 10.1080/09603123.2021.1885632
INSSE. (2023). Sacrificările de animale şi păsări şi producţia de. Available online at: https://insse.ro/cms/sites/default/files/com_presa/com_pdf/carne04r23.pdf (accessed September 29, 2023).
Kang, J., Hossain, M. A., Park, H. C., Jeong, O. M., Park, S. W., Her, M., et al. (2021). Cross-contamination of enrofloxacin in veterinary medicinal and nutritional products in Korea. Antibiotics 10, 1–6. doi: 10.3390/antibiotics10020128
Kyriakides, D., Lazaris, A. C., Arsenoglou, K., Emmanouil, M., Kyriakides, O., Kavantzas, N., et al. (2020). Dietary exposure assessment of veterinary antibiotics in pork meat on children and adolescents in Cyprus. Foods 9, 1–14. doi: 10.3390/foods9101479
Li, J. J. (2022). Eravacycline (Xerava), a novel and completely synthetic fluorocycline antibiotic. Curr. Drug Synthesis 12, 8–100. doi: 10.1002/9781119847281.ch5
Liofilchem. (2011). MeRA Test. 2. Available online at: https://www.liofilchem.com/images/brochure/MeRA_Test.pdf (accessed September 29, 2023).
Mader, R., Demay, C., Jouvin-Marche, E., Ploy, M. C., Barraud, O., Bernard, S., et al. (2022). Defining the scope of the European antimicrobial resistance surveillance network in veterinary medicine (EARS-Vet): a bottom-up and one health approach. J. Antimicrob. Chemother. 77, 816–826. doi: 10.1093/jac/dkab462
Mak, P. H. W., Rehman, M. A., Kiarie, E. G., Topp, E., and Diarra, M. S. (2022). Production systems and important antimicrobial resistant-pathogenic bacteria in poultry: a review. J. Anim. Sci. Biotechnol. 13, 1–20. doi: 10.1186/s40104-022-00786-0
Malik, S. J., Saleemi, M. A., and Danquah, C. A. (2019). Antimicrobials, Antibiotic Resistance, Antibiofilm Strategies and Activity Methods. Norderstedt: BoD–Books on Demand.
Medicines V. (2002). Committee for Veterinary Medicinal Products Streptomycin Summary Report (3). Emea/Mrl/809/01-Final 3–8. Available online at: https://www.ema.europa.eu/en/documents/mrl-report/streptomycin-summary-report-3-committee-veterinary-medicinal-products_en.pdf (accessed May 25, 2023).
Moghadam, N. R., Arefhosseini, S. R., Javadi, A., Lotfipur, F., Ansarin, M., Tamizi, E., et al. (2018). Determination of enrofloxacin and ciprofloxacin residues in five different kinds of chicken tissues by dispersive liquid–liquid microextraction coupled with HPLC. Iran J. Pharm. Res. 17, 1182–1190.
Mohammadzadeh, M., Montaseri, M., Hosseinzadeh, S., Majlesi, M., Berizi, E., Zare, M., et al. (2022). Antibiotic residues in poultry tissues in Iran: a systematic review and meta-analysis. Environ. Res. 204, 112038. doi: 10.1016/j.envres.2021.112038
Murray, C. J., Ikuta, K. S., Sharara, F., Swetschinski, L., Robles Aguilar, G., Gray, A., et al. (2022). Global burden of bacterial antimicrobial resistance in 2019: a systematic analysis. Lancet 399, 629–655. doi: 10.1016/S0140-6736(21)02724-0
Nanda, P. K., Bhattacharya, D., Das, J. K., Bandyopadhyay, S., Ekhlas, D., Lorenzo, J. M., et al. (2022). Emerging role of biosensors and chemical indicators to monitor the quality and safety of meat and meat products. Chemosensors 10, 1–26. doi: 10.3390/chemosensors10080322
Oyedeji, A. O., Msagati, T. A. M., Williams, A. B., and Benson, N. U. (2019). Determination of antibiotic residues in frozen poultry by a solid-phase dispersion method using liquid chromatography-triple quadrupole mass spectrometry. Toxicol. Rep. 6, 951–956. doi: 10.1016/j.toxrep.2019.09.005
Panzenhagen, P. H. N., Aguiar, W. S., Gouvêa, R., de Oliveira, A. M. G., Barreto, F., Pereira, V. L. A., et al. (2016). Investigation of enrofloxacin residues in broiler tissues using ELISA and LC-MS/MS. Food Addit. Contam. Part A 33, 639–643. doi: 10.1080/19440049.2016.1143566
Pirvutoiu, I., and Popescu, A. (2013). Research on consumer behaviour in bucharest poultry meat market. Sci. Pap. Anim. Sci. Biotechnol. 46, 389.
Ramatla, T., Ngoma, L., Adetunji, M., and Mwanza, M. (2017). Evaluation of antibiotic residues in raw meat using different analytical methods. Antibiotics 6, 1–17. doi: 10.3390/antibiotics6040034
Salama, N. A., Abou-Raya, S. H., Shalaby, A. R., Emam, W. H., and Mehaya, F. M. (2011). Incidence of tetracycline residues in chicken meat and liver retailed to consumers. Food Addit. Contam. 4, 88–93. doi: 10.1080/19393210.2011.585245
Srisuwananukorn, A., Han, J., Raslan, R., Gowhari, M., Hussain, F., Njoku, F., et al. (2021). Antimicrobial resistance is a risk factor for mortality in adults with sickle cell disease. Haematologica 106, 1745–1748. doi: 10.3324/haematol.2020.267872
Stavroulaki, A., Tzatzarakis, M. N., Karzi, V., Katsikantami, I., Renieri, E., Vakonaki, E., et al. (2022). Antibiotics in raw meat samples: estimation of dietary exposure and risk assessment. Toxics 10, 1–15. doi: 10.3390/toxics10080456
Verma, M. K., Ahmad, A. H., Pant, D., Rawat, P., Sharma, S., Arya, N., et al. (2020). Screening of enrofloxacin and ciprofloxacin residues in chicken meat by high-performance liquid chromatography. J. Pharm. Res. Int. 5, 64–69. doi: 10.9734/jpri/2020/v32i2130753
Keywords: antibiotic resistance, food safety, meat antibiotic residue, public health, the withdrawal period
Citation: Pogurschi EN, Grigore D-M, Ianitchi D, Bahaciu G, Popa DC, Dragomir N and Pet I (2023) Screening and detection of antibiotic residues on broiler meat based on trade system variations, seasonal differences, and the impact on final consumer safety in Romania. Front. Sustain. Food Syst. 7:1198411. doi: 10.3389/fsufs.2023.1198411
Received: 01 April 2023; Accepted: 12 September 2023;
Published: 09 October 2023.
Edited by:
Jolieke Van Der Pols, Queensland University of Technology, AustraliaReviewed by:
Babak Pakbin, Technical University of Munich, GermanyWageh Darwish, Zagazig University, Egypt
Copyright © 2023 Pogurschi, Grigore, Ianitchi, Bahaciu, Popa, Dragomir and Pet. This is an open-access article distributed under the terms of the Creative Commons Attribution License (CC BY). The use, distribution or reproduction in other forums is permitted, provided the original author(s) and the copyright owner(s) are credited and that the original publication in this journal is cited, in accordance with accepted academic practice. No use, distribution or reproduction is permitted which does not comply with these terms.
*Correspondence: Daniela-Mihaela Grigore, ZGFuaWVsYS1taWhhZWxhLmdyaWdvcmUmI3gwMDA0MDt1c2Ftdi5ybw==; Ioan Pet, aW9hbnBldCYjeDAwMDQwO3VzYWItdG0ucm8=