- 1Guangdong Provincial Key Laboratory of Utilization and Conservation of Food and Medicinal Resources in Northern Region/Henry Fok College of Biology and Agriculture/Shaoguan Aromatic Plant Engineering Research Center, Shaoguan University, Shaoguan, China
- 2Guangdong Province Key Laboratory of Postharvest Science of Fruits and Vegetables/Key Laboratory of Biology and Germplasm Enhancement of Horticultural Crops in South China, Ministry of Agriculture, College of Horticulture, South China Agricultural University, Guangzhou, China
Fresh-cut taros are prone to browning even though they are stored at low temperatures, which leads to the quality deterioration and markedly limits the shelf life. The browning control of fresh-cut food with natural substances has received much attention. This study evaluated anti-browning potential of peppermint extracts in fresh-cut taro during cold storage. The results showed that both peppermint leaf and shoot extract (LE and SE) treatments could effectively retard the browning of fresh-cut taros. Furthermore, LE and SE treatments reduced the activities of phenylalanine ammonialyase and lipoxygenase, the contents of total phenolic compound (TPC), total flavonoids, soluble quinones, malondialdehyde and hydrogen peroxide, enhanced peroxidase activity and antioxidant capacity in taro slices. The TPC contents were reduced by 4.05 and 17.71%, and the T-AOC was enhanced by 28.73 and 28.82% as compared to the control at 12 d. These results together suggested that peppermint extract treatments reduced phenolic biosynthesis but enhanced antioxidant activity. However, LE and SE treatments did not inhibit polyphenol oxidase (PPO) activity, suggesting that peppermint extract treatments reduced taro browning was not through inhibition of phenolic oxidation. More than 240 phenolic-related compounds including caffeic acid, ferulic acid and p-Coumaric acid were identified in peppermint extracts through UPLC–MS/MS analysis, suggesting that phenolics may be bioactive substances reducing taro browning. In conclusion, this study demonstrates that peppermint extract is a promising natural anti-browning ingredient and phenolic compounds may function on taro browning inhibition.
Introduction
Taro (Colocasia esculenta), a member of the Araceae family, is cultivated worldwide, including in Africa, Asia, Oceania, and Americans (Xiao et al., 2022). Taro is a kind of staple food or root vegetable; the corm is the major edible part (Wang et al., 2022b). In 2021, the global production of taro corms amounted over 12 million tons, with China was one of the largest producers in the world and the total production in China exceeded 1.9 million tons a year (FAOSTAT, 2021). In addition to providing dietary energy by being rich in starch, fresh taro and its processed products are also an excellent source of amino acids, polyphenols, dietary fibers, polysaccharides, and minerals (Bellinger et al., 2020), which have numerous beneficial effects on human health.
Due to its convenient preparation, the market demand for fresh-cut taro is increasing annually (Xiao et al., 2022). However, the fresh-cut taro is prone to decay after peeling and cutting, even storage or consumption under low temperatures. The brought undesirable side effects by peeling and cutting mainly include surface browning, stale taste, cellular content leakage, and harmful microbe growth on the cut surface (Padrón-Mederos et al., 2020). Among those negative effects, surface browning is the most significant issue in preserving and consuming fresh-cut taro (Wang et al., 2022a), which significantly reduces its shelf life and affects consumer acceptance. Therefore, it is an urgent task to develop more effective technologies to prevent the browning of fresh-cut taro.
The browning mechanisms of fresh-cut products were suggested to be related to several enzymes (Arnold and Gramza-Michałowska, 2022). Phenylalanine ammonia lyase (PAL), peroxidase (POD) and polyphenol oxidase (PPO), are considered as three major enzymes involving in enzymatic browning reactions (Chen et al., 2021). PAL is a key enzyme in the regulation of the biosynthesis of phenolics and flavonoids through the phenylpropanoid pathway (Wang et al., 2021). The enhanced PAL activity may accelerate the phenolic biosynthesis and contribute to the browning in fresh-cut products (Remorini et al., 2015). POD and PPO are oxidoreductase enzymes that promotes the oxidation of phenolic compounds to produce dark-pigment products, quinones and other melanins, when oxygen is present in fresh-cut foods (Sikora et al., 2020). In addition, the browning of fresh-cut products may be caused by the peroxidation of membrane lipid triggered by reactive oxygen species (ROS), and the enhancement of ROS scavenging activity could restrain browning development (Pan et al., 2020; Zhu et al., 2022). These studies indicate that the modification of the activity of enzymes related to browning via postharvest treatments could regulate the browning process of fresh-cut products.
Postharvest treatments that could restrict the browning process of fresh-cut produces mainly include physical and chemical methods (Oms-Oliu et al., 2010). Although physical methods have some advantages, such as being cost-effective and offering high security, their ability to inhibit enzymatic browning is unsatisfactory due to their inability to directly inactivate the activity of enzymes related to browning. More importantly, a large number of physical treatments result in unwanted changes in color, flavor, and texture (Moon et al., 2020; Xu et al., 2022). Compared with physical treatments, chemical methods used to inhibit browning are more effective and stable. For example, sulfite, one of the extensively used PPO inhibitors, has been well demonstrated that it could effective inhibit the browning of most fresh-cut products (Pundir and Rawal, 2013). However, chemical anti-browning agents may cause some harmful effects such as asthma, hypersensitivity and neurological damages on human health (Ullah et al., 2022). In this regard, consumer demands for new food options are increasing, especially those containing natural and biologically active ingredients (Pateiro et al., 2021). Therefore, developing natural browning inhibitors with additional health benefits is of great interest to researchers in the food industry.
It is known that plant extracts contain high levels of phenolic compounds (Soysal, 2009). It has been reported that the application of several plant extracts especially those rich in compounds to fresh-cut foods is effective for the browning inhibition (Liu et al., 2019; Dias et al., 2020; Sikora et al., 2020; Zhang et al., 2022). The phenolic compounds in plant extracts not only provide health benefits for the consumption of fresh-cut foods, but also contribute to the maintenance of color and flavor (Sukhonthara et al., 2016). Therefore, the use of plant extracts as browning inhibitors is a sustainable and suitable method while producing safe and nutritional fresh-cut products (Moon et al., 2020). Peppermint (Mentha piperita L.) is an edible wild herb in the family of Lamiaceae (mintfamily), which is generally used in traditional medicine for its antioxidant, anti-inflammatory, antitumor and other properties (Mahendran and Rahman, 2020). In addition, peppermint plants are condiments to be used in day-to-day cooking in many countries due to its characteristic flavor and odor (Vining et al., 2017). In most cases, peppermint plant is generally used to extract essential oil for industrial use. However, whether methanolic extracts of peppermint plant could serve as an anti-browning alternative to control fresh-cut food browning is unknown.
The main goal of the present study was to evaluate the inhibitory effects of peppermint methanolic extracts on enzymatic browning of fresh-cut taros during cold storage. In addition, possible mechanisms of peppermint extracts on taro browning inhibition were proposed and phenolic compounds in peppermint extracts were profiled using UPLC–MS/MS analysis. This study would provide a glimpse into the use of methanolic extracts from medicinal plant and the development of safe and natural additives in food, especially in ready-to-eat fruit and vegetables.
Materials and methods
Materials
Peppermint (Mentha piperita cv. hengjing gaoyou) was planted in a garden of Shaoguan University, China (Wang et al., 2023). About 2 month old peppermint plants were harvested in May 2021, and fresh plants were used for the preparation of peppermint extracts.
Taro (Colocasia esculenta cv. Binglang) corms at a commercially ripe stage were harvested (Xiao et al., 2022), and purchased from a local farm in Shaoguan, China. Taros were immediately transported to the lab (Guangdong Provincial Key Laboratory of Utilization and Conservation of Food and Medicinal Resources in Northern Region) within 1 hour. At this phase, the growth of taro corm halts entirely, while nutrients are entirely amassed, the leaves above the ground tend to yellow and some to wither. Taros used for investigations were selected based on the standard of uniform sizes (800 g–1,000 g for each corm), free of physical and biotic damages. Any putrid or broken corms were excluded.
Preparation of peppermint extracts
Peppermint leaf and shoot were separately collected and were freeze-dried using a vacuum freeze-dryer (Scientz-100F). The freeze-dried samples were milled with a mixer mill (MM 400, Retsch) and then screened past a 50 mesh sieve. 1 g lyophilized powders were homogenized with 10 mL 70% methanol solution by using an overhead stirrer at 200 × g, 4°C overnight. The homogenate was then centrifuged at 11000 × g, 4°C for 10 min. The supernatants were collected and defined as leaf extract (LE) or shoot extract (SE) and chlorophyll in the extracts was removed by method of coprecipitation with molysite before treating fresh-cut taros. LE and SE were used for evaluating inhibitory effects on the browning of taro slices, and were stored at 4°C before further treatment and analysis.
Fresh-cut taro preparation and experiment design
Taro corms were peeled and cut into 1 cm thickness. The taro slices were washed with cool water and then were sterilized with ozone for 30 min. The sterilized taro slices were subsequently placed in a plastic basket to remove residual water.
The prepared slices were immersed in 2 and 5% LE or SE for 30 min at ambient temperature. The control slices were immersed in sterilized distilled water (DW, pH 7.0) for 30 min. After treatment, fresh-cut taro slices were left to drain naturally for 10 min and were hermetically wrapped with plastic bags (0.02 mm thickness). The packages with taro slices were stored at 90 to 95% RH and 5°C for 12 days.
Browning degree evaluation
The browning degree on the surface of fresh-cut taros was quantitatively evaluated using a CR-400 Chroma meter (KONICA MINOLTA, Japan). Four color parameters (L*, a*, b*, and ∆E) were employed. In which, ∆𝐸 = [(∆L*)2 + (∆a*)2 + (∆b*)2]1/2. Moreover, browning index (BI) was also used as an indicator to evaluate the browning severity of fresh-cut taros. The BI formula was described in our former study (Wang et al., 2022b).
Determination of enzyme activity
The PAL activity was determined according to the generation of trans-cinnamate (Xiao et al., 2020). 1.0 g taro samples (derived from three slices) were ground with 5.0 mL borate buffer (pH 7.8, 0.2 M) in a mortar. Then the homogenates were centrifuged at 12000 × g, 4°C for 10 min. The supernatants were collected for the measurement of PAL activity. After centrifugation, 0.5 mL supernatants were reacted with 1.5 mL 0.1 mM sodium borate (pH 8.8), 0.5 mL 0.02 M L-phenylalanine and 0.5 mL 5.0 mM dithiothreitol for 2 h at ambient temperature. At last, the absorbance of mixtures was recorded at 290 nm to determine PAL activity.
The crushed extracts were prepared with 0.05 M sodium phosphate buffer (SPB, pH 7.0). 1.0 g taro samples (derived from three slices) were ground with 5.0 mL phosphate buffer (pH 7.8, 0.1 M) in a mortar. The homogenized samples were centrifuged at 12000 × g, 4°C for 10 min. The supernatants were regarded as crushed extracts and were used to determine enzyme activity.
Enzyme activities were assayed according to the methods we described previously (Xiao et al., 2021). Here, one unit of POD, PPO, and lipoxygenase (LOX) were defined as an increase in A470, A398, and A234 of per minute, respectively.
Antioxidant capacity assay
1.0 g taro samples were first grind with 5.0 mL normal saline in a mortar. Then, the homogenate was centrifuged at 12,000 × g for 10 min at 4°C. The supernatants were collected into a new tube to assay antioxidant capacity.
The scavenging effect of taro samples for hydroxyl radical (OH) was determined with the Fenton reaction (Gutteridge, 1987). The scavenging activity of taro samples for 1,1-Diphenyl-2-picrylhydrazyl free radical (DPPH) were monitored according to the method of the previous report (Duan et al., 2016). Scavenging capacity of taro samples for superoxide anion radical (O2−) was determined with pyrogallic reagent (Zhang et al., 2016).
Total antioxidant capacity (T-AOC) of taro samples was evaluated using the ferric reducing antioxidant power (FRAP) method (Zhu et al., 2022). T-AOC was calculated with a standard curve generated by different concentrations of trolox, and the results were expressed as mg kg−1 fresh weight (FW).
Measurement of total phenolics, total flavonoids, soluble quinones, malondialdehyde, and hydrogen peroxide contents
The contents of total phenolic compound (TPC), total flavonoid (TF), soluble quinone (SQ), malondialdehyde (MDA), and hydrogen peroxide (H2O2) in fresh-cut taro slices were detected using the method that we described previously (Wang et al., 2022a). TPC content was calculated with a standard curve produced by different concentration of gallic acid. TPC content was expressed as milligrams of gallic acid equivalent (GAE) per kilogram of fresh samples.
UPLC–MS/MS analysis
Peppermint extracts were filtrated through a 0.22 μm membrane filter (SCAA-104, ANPEL, China) before LC–MS analysis. Chemical compounds in the filtrates were analyzed using an UPLC-ESI-MS/MS system (UPLC, SHIMADZU Nexera X2; MS, Applied Biosystems 4,500 Q TRAP).
The filtrates were separated at 40°C and the flow rate of 0.35 mL/min with an Agilent SB-C18 UPLC column (1.8 μm, 2.1 mm × 100 mm). The injection volume was 4 μL. Solvent A (pure water with 0.1% formic acid) and solvent B (acetonitrile with 0.1% formic acid) composed the mobile phase. The gradient program was started at conditions of 95% A, 5.0% B. A linear gradient to 5.0% A, 95% B was programmed within 9 min, and a composition of 5.0% A, 95% B was hold for 1 min. Then, a composition of 95% A, 5.0% B was adjusted within 70 s, hold for 2.9 min. The effluent was alternatively connected to an ESI-triple quadrupole-linear ion trap (Q TRAP)-MS.
(Q TRAP)-MS analysis was carried out according to the procedures and conditions described previously by a former study (Qu et al., 2021).
Statistical analysis
The experiment was randomly designed. All values were reported as mean ± standard deviation (SD) (n = 3). Statistically significant differences (p ≤ 0.05) between the control and treatments were detected by Duncan’s multiple range tests in the SPSS software (version 25). The statistical differences were marked in all figures using different lowercase letters above the bars.
Results
Effects of peppermint extracts on the browning performance of fresh-cut taros
Instrumental color analysis using CIE L*, a*, and b* system indicates a process of product browning (Gao et al., 2017). As shown in Figure 1A, L* values were decreased with the evaluation of storage duration regardless of treatments. L* values in the control (DW) were steady decreased from 88.33 at 0 d to 82.02 at 12 d. Treatments of peppermint extracts (LE and SE) significantly repressed the reduction of L* values, and L* values in LE and SE treatments were 2.06–2.60% higher than that in the control after 12 days of cold storage. L* values between different concentrations of peppermint extract treatments did not show significant differences (Figure 1A).
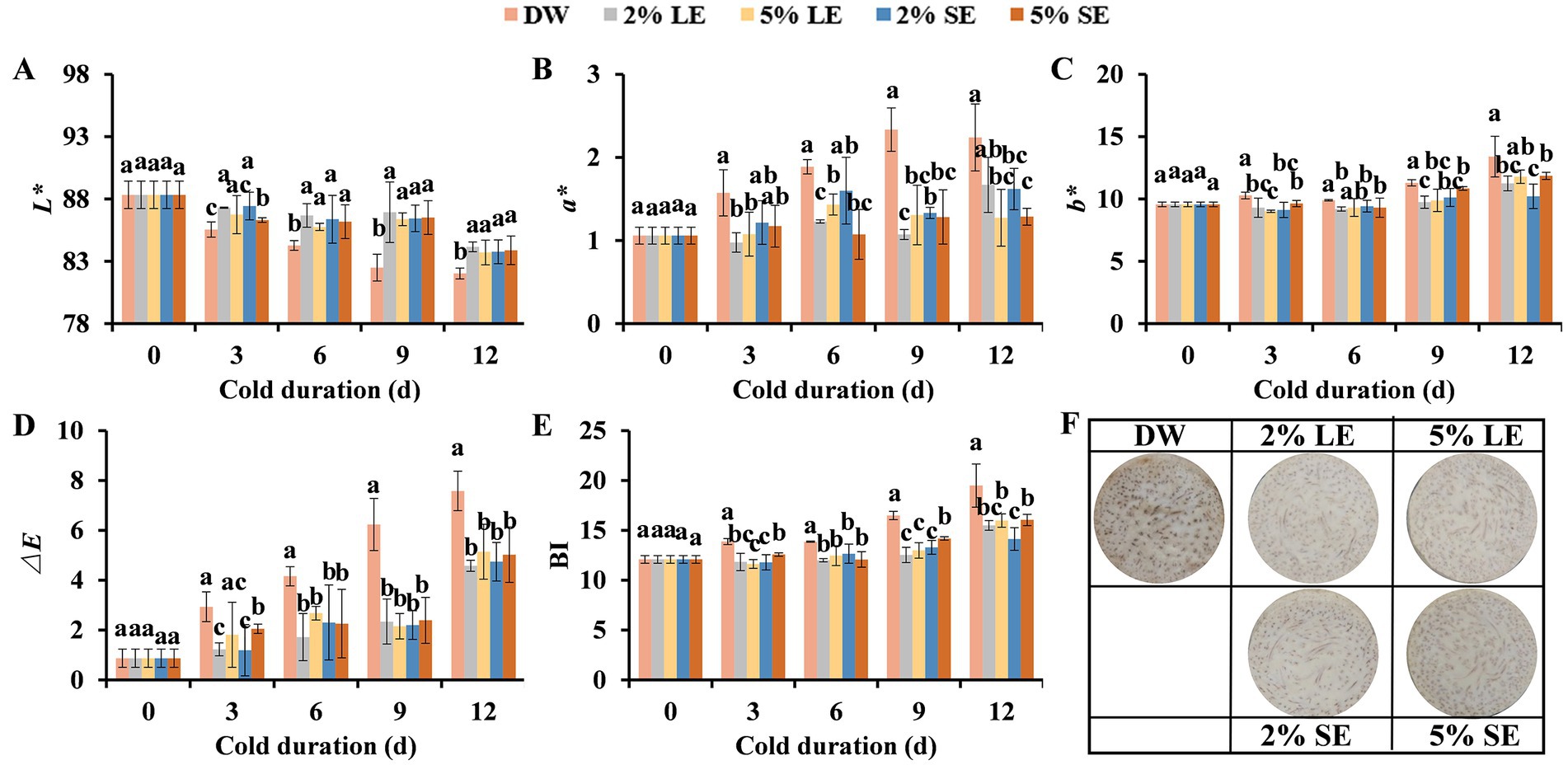
Figure 1. Effects of different concentrations of peppermint extract treatments on the surface browning of fresh-cut taro during cold storage. (A) L* value, (B) a* value, (C) b* value, (D) ∆𝐸 value, (E) browning index (BI), and (F) the representative images of fresh-cut taro stored at 5°C for12 days. DW, distilled water; LE, leaf extract; SE, shoot extract. Each value is presented as mean ± standard deviation (SD, n = 3). Statistical differences (p ≤ 0.05) between treatments at the same time point are analyzed and indicated using lower case letters above the bars.
An opposite trend was observed for a*, b*, ∆E, and BI values during cold storage in relative to L* trend (Figures 1B–E). The values of these four parameters were increased over storage time. During 12 days of cold storage, these four color parameters (a*, b*, ∆E, and BI) increased faster in the control than that in LE and SE treatments. At 12 d, the values of four color parameters in LE and SE treatments were significantly lower than that in the control, and no significant differences between different concentrations (2 and 5%) of LE and SE treatments were detected (Figures 1B–E). To go along with this, LE- and SE-treated taros remained brighter than the control (Figure 1F). As the conditions of the control and extract treatments were same excepting that the concentration of peppermint extracts was different, suggesting that the different color changes of the control and extract treatments were caused by peppermint extracts.
To confirm inhibitory effects of LE and SE solutions on taro browning, a repeated experiment was conducted using 5% LE and 5% SE solutions. The results showed that both LE and SE treatments resulted in significantly higher L* values, but lower b*, ∆E, and BI values when compared with the control (Figures 2A–D). The browning process on the surface of fresh-cut taros in LE and SE treatments was evidently slower than that in the control. Some slight, visible browning symptoms on the surface were observed at day 6 in the control, but no obvious browning appeared in the LE- and SE-treated samples at the same time. At day 12, the control displayed severe browning symptoms on taro surface, while the browning was slight in both SE and LE treatments (Figure 2E). Therefore, it is reasonable to believe that peppermint extracts have functions on browning inhibition in fresh-cut taro, and the results suggest that peppermint extracts may be a good source of natural anti-browning agents.
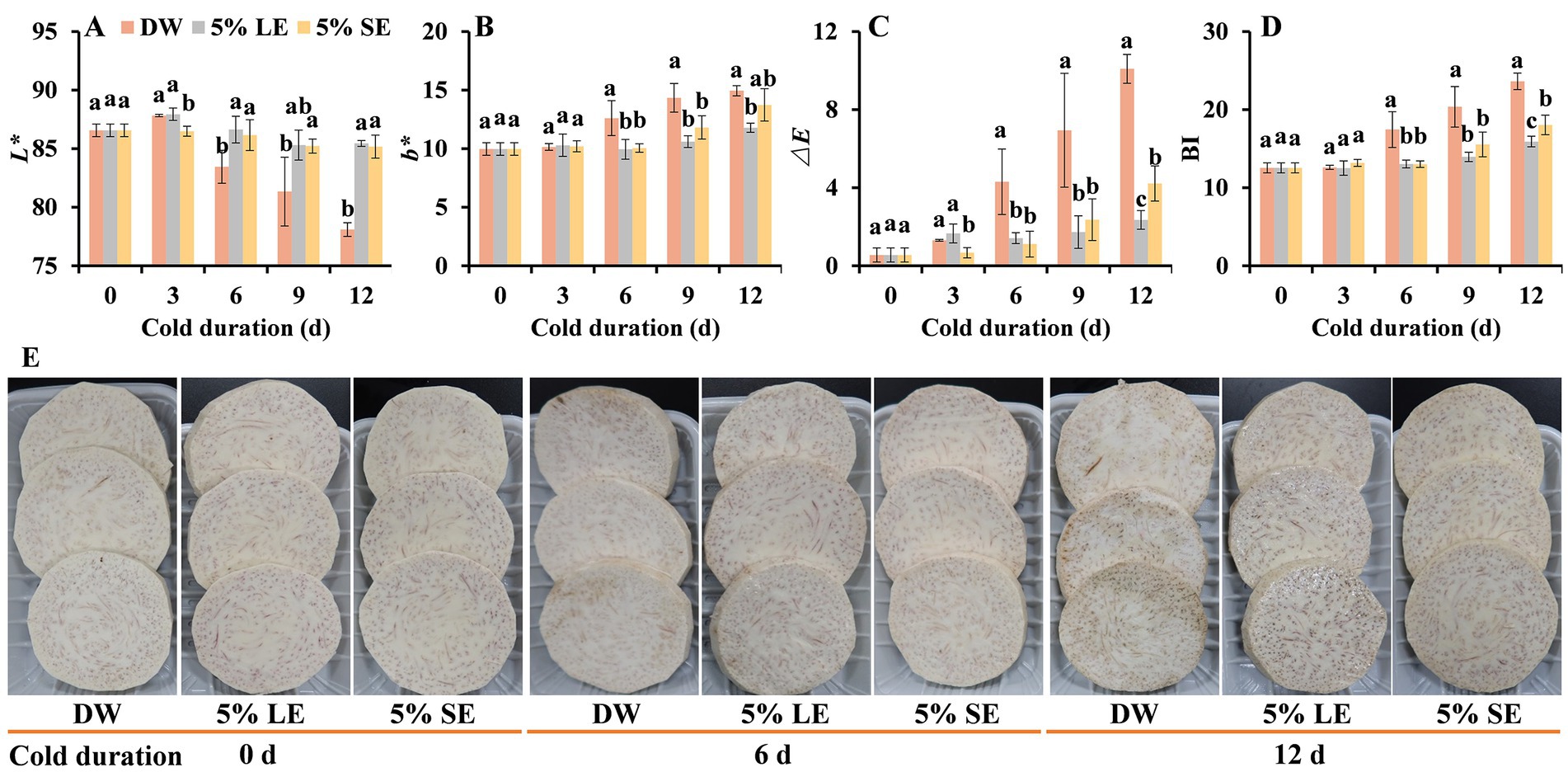
Figure 2. Effects of 5% leaf extract (LE) and 5% shoot extract (SE) treatments on the surface browning of fresh-cut taro during cold storage. (A) L* value, (B) b* value, (C) ∆𝐸 value, (D) browning index (BI), and (E) the development of browning symptoms of fresh-cut taro during cold storage at 5°C. DW, distilled water. Each value is presented as mean ± standard deviation (SD, n = 3). Statistical differences (p ≤ 0.05) between treatments at the same time point are analyzed and indicated using lower case letters above the bars.
Effects of peppermint extracts on the biosynthesis and oxidation of phenolics in fresh-cut taros
As the biosynthesis and oxidation of phenolics may contribute to the discoloration of fresh-cut products (Siddiqui et al., 2020; Chen et al., 2022). We further analyzed the effects of 5% LE and 5% SE treatments on the biosynthesis and oxidation of phenolics in fresh-cut taros during cold storage.
PAL activity in the control progressively increased with the extension of storage time, whereas that in LE and SE treatments increased much less during the entire cold storage (Figure 3A). The PAL activities in LE and SE treatments were significantly lower than that in the control with an exception at 3 d. After 12 d cold storage, PAL activities in LE and SE treatments were reduced by 11.20 and 13.52%, respectively, as compared to the control (Figure 3A). These results suggested that peppermint extract treatments had inhibitory effects on PAL activity.
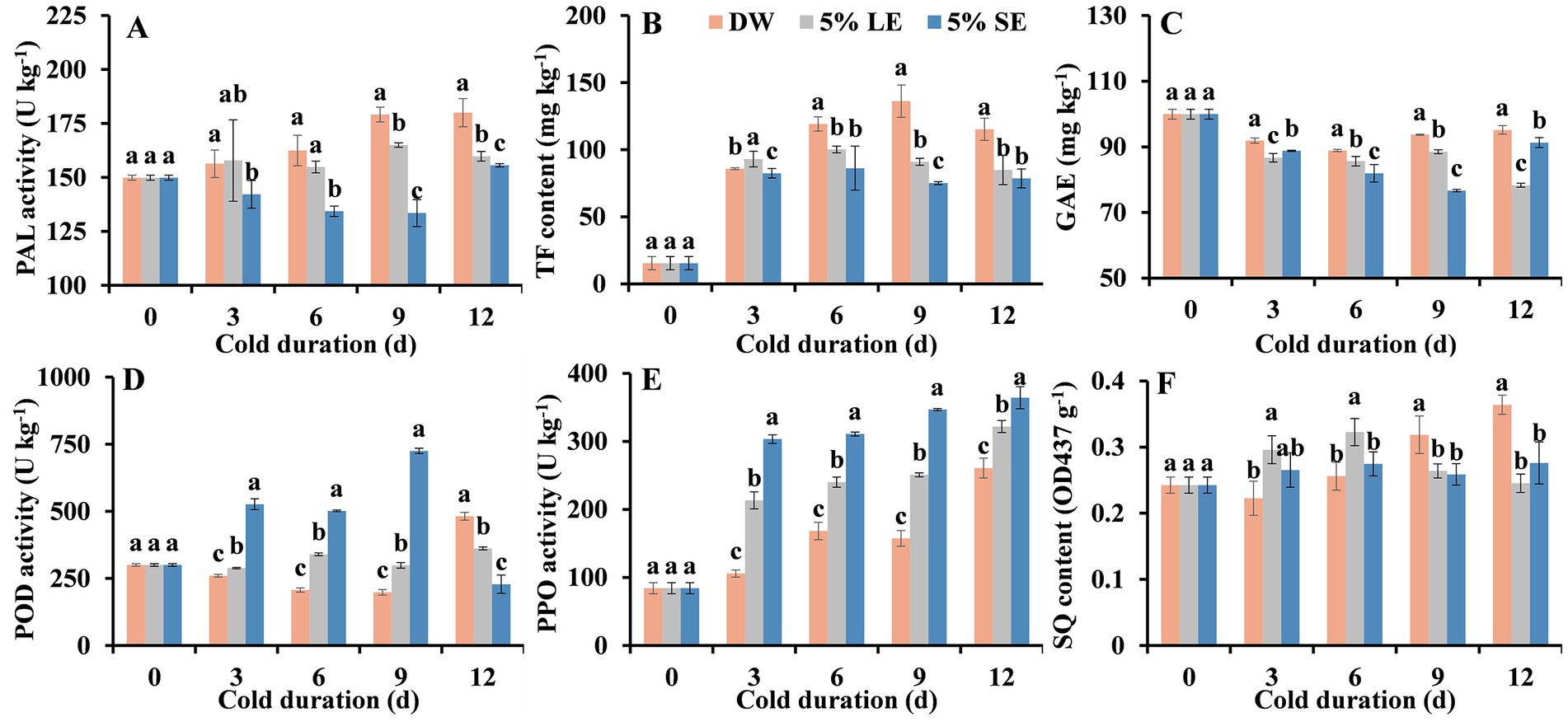
Figure 3. Effects of 5% leaf extract (LE) and 5% shoot extract (SE) treatments on the biosynthesis and oxidation of phenolics in the fresh-cut taros cold-stored at 5°C. (A) PAL activity, (B) total flavonoid (TF) content, (C) total phenolic content, (D) POD activity, (E) PPO activity, and (F) soluble quinone (SQ) content. Each value is presented as mean ± standard deviation (SD, n = 3). Statistical differences (p ≤ 0.05) between treatments at the same time point are analyzed and indicated using lower case letters above the bars.
TF contents in all groups were markedly increased at 3 d (Figure 3B), suggesting that minimal processing (fresh-cut and peeling) induced TF biosynthesis. TF contents in all treatments first increased and then declined. TF contents in the control increased to a peak at 9 d whereas that in both LE and SE treatments, at 6 d. TF contents in LE and SE treatment groups were significantly lower than that in the control during the last 9 days of storage (Figure 3B). When compared with the control, LE and SE treatments reduced TF contents by 26.32 and 31.73%, respectively, at 12 d (Figure 3B).
TPC contents in all groups showed an overall downward trajectory during cold storage (Figure 3C). TPC contents in the control decreased much less than that in the extract treatments. Compared with the control, TPC contents in both LE and SE treatments were significantly lower than that in the control during the entire cold storage period (Figure 3C).
POD activity in the control gradually decreased, while that in LE and SE treatments increased during the first 9 days of cold storage (Figure 3D). As a consequence, POD activity in LE and SE treatments was significantly higher than that in the control with an exception at 12 d. These results suggested that peppermint extract treatment enhanced POD activity in fresh-cut taros under cold condition. In addition, the induction intensity for POD activity by SE treatment was stronger than other treatments (Figure 3D).
PPO activity in all groups progressively rose along with the elevation of storage duration (Figure 3E). Compared with the control, both LE and SE treatments caused higher PPO activity in taro slices, suggesting that LE and SE treatments could not inhibit PPO activity. SQ contents in the control increased over storage time, while that in LE treatment only increased at first 6 days and that in SE treatment did not significantly changed when compared with pre-storage (0 d) (Figure 3F). At day 12, SQ contents in LE and SE treatments were 32.60 and 24.18% lower than that in the control slices (Figure 3F).
Effects of peppermint extracts on the antioxidant activity of fresh-cut taros
Oxidative damage caused by minimal processing was also suggested to be related to the browning occurrence of fresh-cut products (Zhu et al., 2022). Therefore, representative antioxidant-related parameters were analyzed. LOX activity, MDA and H2O2 contents in all treatments showed similar increased trend during cold storage (Figures 4A–C). LOX activity, MDA, and H2O2 contents in both extract treatments were significantly lower than that in the DW during storage, with an exception at 6 d for LOX activity (Figures 4A–C). They did not show significant differences between two extract treatments.
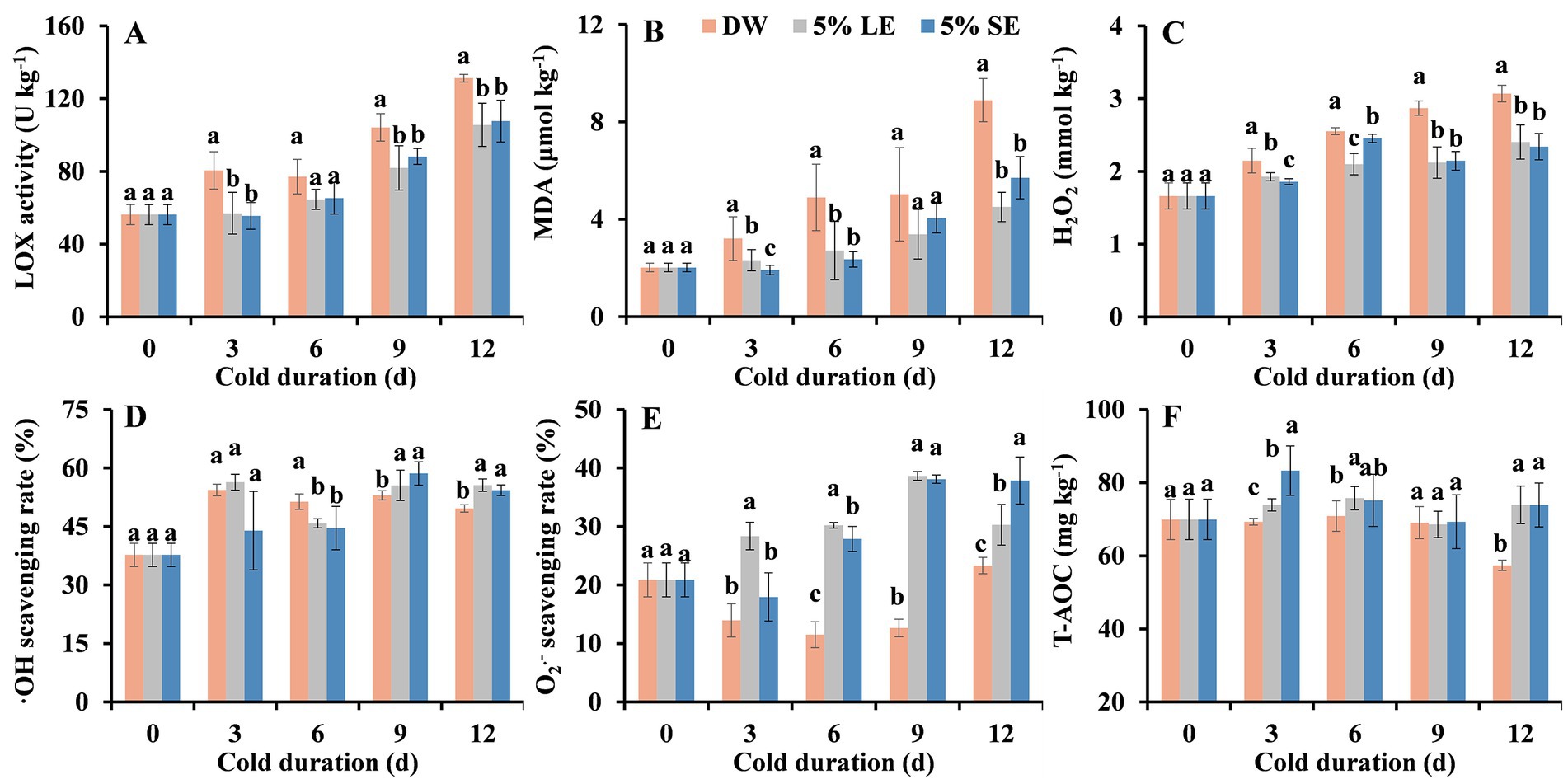
Figure 4. Effects of 5% leaf extract (LE) and 5% shoot extract (SE) treatments on antioxidant capacity of fresh-cut taros during cold storage. (A) LOX activity, (B) malondialdehyde (MDA) content, (C) hydrogen peroxide (H2O2) content, (D) scavenging activity of hydroxyl radical (·OH), (E) scavenging activity of superoxide anion radical (O2−), and (F) total-antioxidant capacity (T-AOC). Each value is presented as mean ± standard deviation (SD, n = 3). Statistical differences (p ≤ 0.05) between treatments at the same time point are analyzed and indicated using lower case letters above the bars.
For radical scavenging capacity, ·OH scavenging rate in LE and SE treatments were significantly higher that in the control during the last 6 days, O2- scavenging rate in LE and SE treatments were significantly higher that in the control during the last 9 days, T-AOC in LE and SE treatments were significantly higher than that in the control at 3 d, 6 d, and 12 d of storage (Figures 4D–F). However, the DPPḤ scavenging rate between treatments did not have significant differences (Supplementary Figure S2). These results suggest that peppermint extract treatment enhanced the radical scavenging capacity of taro slices, especially ·OH and O2− radicals.
The compounds in peppermint leaf and shoot extracts
As the results mentioned above, peppermint extracts could significantly restrain the browning of cold-stored fresh-cut taros. However, which compound functions on browning inhibition and what compounds contained in the peppermint extracts are unknown. Therefore, we profiled the components in peppermint leaf and shoot extracts using UPLC–MS/MS analysis.
Three principal components PC1, PC2, and PC3 (74.95, 7.94, and 6.58%) were extracted in both leaf and shoot extract, respectively (Supplementary Figure S1), indicating the reproducibility and reliability of the data. A total of 732 metabolites in peppermint tissues were identified and they were classed into 34 classes based on HMDB_taxonomy (Figure 5). Prenol lipids (60.00% in LE and SE, respectively) were the most abundant compounds, followed by flavonoids (7.00% in LE, 8.00% in SE) and phenols (7.00% in LE, 6.00% in SE) in two extracts (Figure 5).
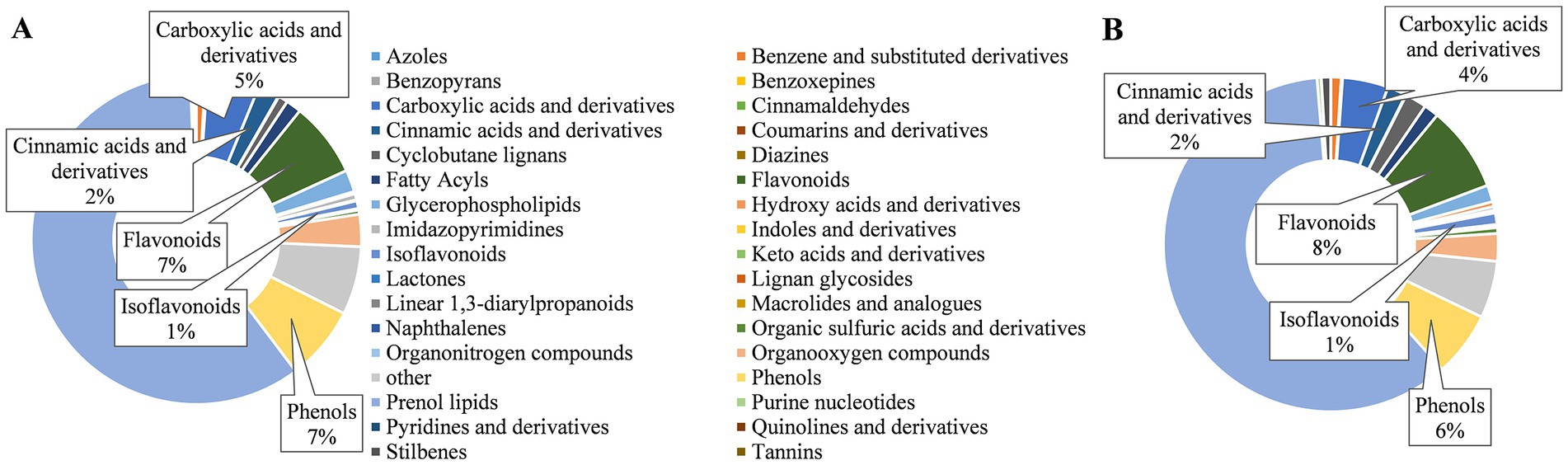
Figure 5. Classes of chemical compounds in leaf extracts (A) and shoot extracts (B). Chemical compounds were identified by UPLC–MS/MS analysis. The classification of chemical compounds was implemented in HMDB database (https://hmdb.ca).
Some studies have demonstrated that phenolic-related compounds are the major components in plant extracts inhibiting the browning development of fresh-cut food (Sukhonthara et al., 2016; Liu et al., 2019; Zhang et al., 2022). Therefore, we laid special stress on analyzing phenolic-related compounds in peppermint extracts.
A total of 107 carboxylic acids and derivatives, 103 flavonoids, 20 cinnamic acids and derivatives, 8 phenols and 7 isoflavonoids were found in peppermint extracts, respectively (Supplementary Data 1). Among 107 carboxylic acids and derivatives composition, L-Serine, L-Valine, succinic acid, pipecolic acid, L-Glutamic acid, L-Tyrosine, isocitric acid, and citric acid have relative high amount. Diosmetin, salvigenin, jaceosidin, 5-Hydroxy-6,7,3′,4′-tetramethoxyflavone, retusin and acerosin were most abundant among those 103 flavonoids. Ethyl rosmarinate, caftaric acid and 2-(7-Dihydroxyl)-benzofuranyl-ferulic acid were the three most abundant compositions in the identified cinnamic acids and derivatives. Prunetin and sodium valproate were the major components in isoflavonoids and phenols, respectively (Supplementary Data 1). These results suggested that phenolic-related compounds were rich in peppermint extracts, and the inhibitory effects of extracts on taro browning were probably due to phenolics.
Total phenolic and total flavonoid contents in fresh peppermint leaves and shoots
We also detected total phenolic and total flavonoid contents in fresh peppermint leaves and shoots. The results were shown in Figure 6. Total phenolic contents in fresh leaves and shoots were about 183.07 mg/kg and 216.58 mg/kg, respectively (Figure 6A). Total flavonoid contents in fresh leaves and shoots were about 90.00 mg/kg and 121.89 mg/kg, respectively (Figure 6B). Both total phenolic and flavonoid contents in shoots were higher than that in leaves. These results again demonstrated that peppermint tissues contained a relative high amount of phenolic and flavonoid compounds, which signifies that peppermint may be a source of natural anti-browning medicaments.
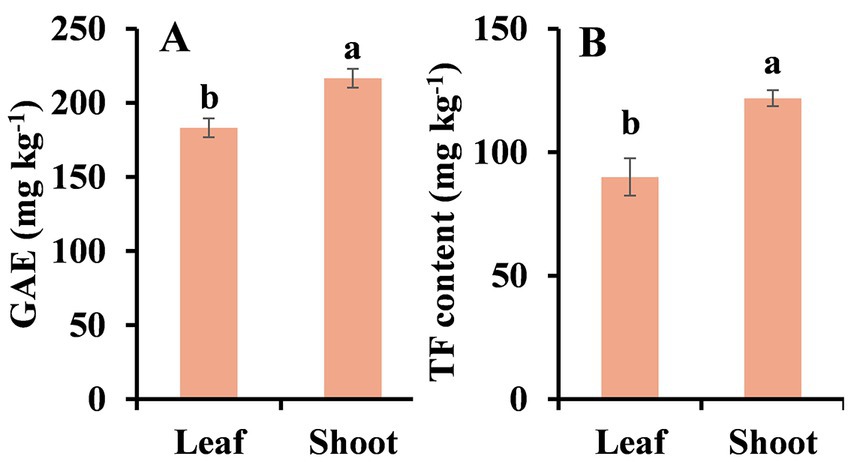
Figure 6. Total phenolic and flavonoid contents in fresh peppermint leaf and shoot. (A) total phenolic content and (B) total flavonoid (TF) content. Each value is presented as mean ± standard deviation (SD, n = 3). Statistical differences (p ≤ 0.05) between leaf and shoot are analyzed and indicated using lower case letters above the bars.
Discussion
The cell structure and integrity of fresh-cut foods were severely destroyed after peeling and cutting, which sent direct contact between enzymes and substrates, accelerated the respiration rate, and increased the activity of endogenous metabolism (Yu et al., 2021). Thus, fresh-cut taros brown rapidly after processing and during storage (Wang et al., 2022a). Study on brown stain prevention techniques has received a lot of attention in recent years. However, due to the low operability or potential hazard concerns, the applications of some chemical methods in the fresh-cut food industry are largely limited (Moon et al., 2020). Hence, the development of browning inhibitors with natural, safe, low-cost, and health properties is of great interest for researchers in the food industry. It has been reported that many plant extracts such as ginger extract (Weerawardana et al., 2020), apple pomace, and peel extracts (Dias et al., 2020) and potato peel extract (Venturi et al., 2019) have strong anti-browning effects. In this study, the browning of fresh-cut taros were significantly reduced after the addition of peppermint leaf and shoot extracts, as compared to the control (Figures 1, 2). These results suggest that peppermint extracts could act as a reliable and effective natural anti-browning substance. The application of peppermint extracts to fresh-cut food may be a promising and sustainable preservation method in the food industry attributing to its natural and health properties.
Due to the higher sensitivity, UPLC–MS/MS analysis has been recognized as a powerful way to identify the metabolites in plant (Zhan et al., 2023). The compounds contained in the extracts actually are peppermint metabolites. So, UPLC–MS/MS analysis was employed to identify what compounds contained in peppermint extracts in this study. The results presented here showed that more than 730 metabolites were identified in peppermint extracts and phenolic-related compounds were abundant in them (Figure 5; Supplementary Data 1). In a previous study, caffeic acid, chlorogenic acid, gallocatechin, and gallic acid were identified as the major polyphenols in Hibiscus acetosella leaves (Lyu et al., 2020). The TPC and TFC levels in peppermint leaves and shoots ranged from 183.07 to 216.58 mg/kg, and 90.00 to 121.89 mg/kg, respectively, with the highest level in shoots (Figure 6). A former study on peppermint young plant, investigating cadmium stress response under hydroponic conditions, reported lower TPC and TFC levels in peppermint leaves (Wang et al., 2023). These results suggest that their contents are varied by plant species, plant organs or tissues, cultivation conditions and harvesting time. With the increase in evidences linking health, phenolic rich foods have emerged as possessing potential health benefits (Veiga et al., 2020). Therefore, the addition of peppermint extracts into fresh-cut taros could additionally improve the nutritional quality.
The phenolics in various plant extracts play a major role in the browning inhibition of fresh-cut fruit and vegetables (Zhang et al., 2022). It has been reported that phenolic treatments show strong inhibitions on fresh-cut product browning (Jiang and Penner, 2022). In previous studies, we demonstrated that the application of ferulic acid and cinnamic acid, two cinnamic acids, could effectively reduce the browning of fresh-cut taro during cold storage (Xiao et al., 2022; Wang et al., 2022a). The addition of p-Coumaric acid, a cinnamic acid, significantly inhibited the browning of potato juice (Zolghadri et al., 2019). Dipping fresh-cut apples into citric acid, a arboxylic acid, improved the color appearance during 7 days of storage at 4°C (Adam et al., 2022). In this study, ferulic acid, 2-Hydroxycinnamic acid and p-Coumaric acid, and other cinnamic acids and derivatives were all abundant in peppermint extracts (Supplementary Data 1). These results suggested that phenolic-related compounds, especially cinnamic acids and derivatives, were bioactive compounds in peppermint extracts. In addition to cinnamic acids and derivatives, numerous other phenolic compounds including carboxylic acids and derivatives, flavonoids, isoflavonoids and phenols were successfully identified in the extracts (Supplementary Data 1). It is necessary to clear whether other phenolic compounds have anti-browning effects.
The biosynthesis of phenolics and flavonoids may be one of important mechanisms of surface discoloration in fresh-cut produces (Chen et al., 2022). PAL involves in the biosynthesis of free phenolics, and its activity was positively correlated with the surface browning of fresh-cut products (Nguyen et al., 2003). In this study, the application of two kinds of peppermint extracts to fresh-cut taros significantly reduced PAL activity, the contents of TPC and TF in fresh-cut taro slices (Figures 3A–C), suggesting the extracts reduced the de novo biosynthesis of phenolics and flavonoids thus resulting in a reduction on taro browning. Phenolic compounds synthesized through the phenylpropanoid pathway have feedback regulations on the phenolic biosynthesis. For instance, caffeic acid in sugarcane plants is a feedback inhibitor on PAL activity (de Armas et al., 2007). It is demonstrated that cinnamic acid treatment could significantly reduce taro browning and PAL activity at both protein and gene levels (Xiao et al., 2022). These results suggested that peppermint extracts reduced phenolic biosynthesis via a feed-forward manner.
ROS is an important factor causing the browning of fresh-cut products (Dou et al., 2021). Peeling and cutting operations induce ROS burst and destroy the balance in intracellular ROS metabolism, and the reduction of ROS level in the body is crucial to the functions of biological macromolecules (Mittler et al., 2022). In this study, LOX activity and contents of MDA and H2O2 were significantly reduced in peppermint extract treatments, suggesting that the treatment reduced membrane lipid peroxidation of taro slices. Moreover, the extract treatments showed stronger ·OH and O2− scavenging capacity and T-AOC (Figure 4). These results together suggested that the treatment reduced oxidative damages on taro cell by directly scavenging ROS, especially ·OH and O2− radicals. Phenolic compounds in plant extracts possess strong radical scavenging ability (Zhang et al., 2022). For instance, the antioxidant activity of the extracts of Hibiscus acetosella varied from the lowest value of 57.47% to the highest values of 84.02% in different cultivars, and the authors supposed that the antioxidant effects of the extracts were probably attributed to flavonoids and anthocyanins (Lyu et al., 2020). These results suggest that the enhancement of antioxidant activity in fresh-cut taros was attributed to a large number of phenolic compounds in peppermint extracts, and thus resulting in a slight browning in taro slices.
Many studies demonstrate that the discoloration of fresh-cut products is mainly caused by enzymatic browning (Zhang et al., 2022). In the enzymatic browning reaction, phenolase catalyze phenols to form quinones under aerobic condition. PPO and POD are two key phenolases in the oxidation process of phenols (Tinello and Lante, 2018), and restricting PPO and POD activities could reduce the browning performance of fresh-cut foods in many cases. For example, in the previous studies, postharvest applications of several chemicals that could inactive PPO or POD activities effectively reduced the browning of fresh-cut taros under low temperature condition (Xiao et al., 2022; Wang et al., 2022a, b). However, compared with the control, PPO and POD activities were not reduced by LE and SE treatments although SQ contents were significantly reduced (Figures 3D–F). These results suggested that peppermint extract treatments reduced taro browning through other mechanisms, rather than through the inhibition of phenolic oxidation. The current results indicated that the suppressed browning may mainly attribute to strong antioxidant activity of peppermint extracts. In addition to being involved in the phenolic oxidation, POD also plays a pivotal role in intracellular ROS balance (Sellami et al., 2022). The enhanced POD activity may also contribute to lower levels of H2O2 in taro slices. However, the mysterious roles of POD in taro browning needs in-depth investigations, because the actions of postharvest treatments that could reduce taro browning during cold storage on POD activity are contradictory in our previous studies.
Conclusion
The application of different concentrations of peppermint extracts significantly reduced the browning of fresh-cut taros during cold storage, suggesting that peppermint extracts could be a natural browning inhibitor. Peppermint extract treatments enhanced antioxidant capacity, reduced the contents of TPC, TF, SQ, MDA, and H2O2, reduced the activities of PAL and LOX, but did not reduce the activities of POD and PPO in taro slices, suggesting that peppermint extract treatment reduced taro browning through restricting phenolic biosynthesis and improving ROS scavenging activity to alleviate oxidative damages. LC–MS analysis showed the presence of phenolic-related compounds in abundance in two kinds of extracts, suggesting that peppermint extracts reduced taro browning and enhanced antioxidant activity of taro slices partly given the credit to rich phenolic compounds.
Data availability statement
The original contributions presented in the study are included in the article/Supplementary material, further inquiries can be directed to the corresponding authors.
Author contributions
XY and BT: methodology, data curation, and investigation. YW, YJ, and JH: writing and editing. GW: project administration, writing-editing, and review. PY: investigation. BW: conceptualization, validation, visualization, writing-editing, and review. All authors contributed to the article and approved the submitted version.
Funding
This work was financially supported by the Open Fund of the Guangdong Provincial Key Laboratory of Utilization and Conservation of Food and Medicinal Resources in Northern Region (Grant number: FMR2022001M), Guangdong Basic and Applied Basic Research Foundation (Grant number: 2023A1515010494), and Special Fund for Scientific and Technological Innovation Cultivation of Guangdong College Student (Grant number: pdjh2023a0470).
Conflict of interest
The authors declare that the research was conducted in the absence of any commercial or financial relationships that could be construed as a potential conflict of interest.
Publisher’s note
All claims expressed in this article are solely those of the authors and do not necessarily represent those of their affiliated organizations, or those of the publisher, the editors and the reviewers. Any product that may be evaluated in this article, or claim that may be made by its manufacturer, is not guaranteed or endorsed by the publisher.
Supplementary material
The Supplementary material for this article can be found online at: https://www.frontiersin.org/articles/10.3389/fsufs.2023.1191396/full#supplementary-material
References
Adam, A. M., Jeganathan, B., Vasanthan, T., and Roopesh, M. S. (2022). Dipping fresh-cut apples in citric acid before plasma-integrated low-pressure cooling improves Salmonella and polyphenol oxidase inactivation. J. Sci. Food Agric. 102, 3425–3434. doi: 10.1002/jsfa.11690
Arnold, M., and Gramza-Michałowska, A. (2022). Enzymatic browning in apple products and its inhibition treatments: a comprehensive review. Compr. Rev. Food Sci. Food Saf. 21, 5038–5076. doi: 10.1111/1541-4337.13059
Bellinger, M. R., Paudel, R., Starnes, S., Kambic, L., Kantar, M. B., Wolfgruber, T., et al. (2020). Taro genome assembly and linkage map reveal QTLs for resistance to Taro leaf blight. G3 (Bethesda) 10, 2763–2775. doi: 10.1534/g3.120.401367
Chen, C., Jiang, A. L., Liu, C., Wagstaff, C., Zhao, Q. Q., Zhang, Y. H., et al. (2021). Hydrogen sulfide inhibits the browning of fresh-cut apple by regulating the antioxidant, energy, and lipid metabolism. Postharvest Biol. Tec. 175:111487. doi: 10.1016/j.postharvbio.2021.111487
Chen, Z., Xu, Y. H., Lu, Y., Miao, Z. Y., Yi, Y., Wang, L. M., et al. (2022). Effect and mechanism of eugenol on storage quality of fresh-peeled Chinese water chestnuts. Front. Plant Sci. 13:965723. doi: 10.3389/fpls.2022.965723
de Armas, R., Santiago, R., Legaz, M., and Vicente, C. (2007). Levels of phenolic compounds and enzyme activity can be used to screen for resistance of sugarcane to smut (Ustilago scitaminea). Australas. Plant Path. 36, 32–38. doi: 10.1071/AP06077
Dias, C., Fonseca, A. M. A., Amaro, A. L., Vilas-Boas, A. A., Oliveira, A., Santos, S. A. O., et al. (2020). Natural-based antioxidant extracts as potential mitigators of fruit browning. Antioxidants 9, 1–20. doi: 10.3390/antiox9080715
Dou, Y., Chang, C. M., Wang, J., Cai, Z. P., Zhang, W., Du, H. Y., et al. (2021). Hydrogen sulfide inhibits enzymatic browning of fresh-cut Chinese water chestnuts. Front. Nutr. 8:652984. doi: 10.3389/fnut.2021.652984
Duan, X. J., Zhang, W. W., Li, X. M., and Wang, B. G. (2016). Evaluation of antioxidant property of extract and fractions obtained from a red alga. Food Chem. 95, 37–43. doi: 10.1016/j.foodchem.2004.12.015
FAOSTAT (2021). Crops and livestock products. Food and Agriculture Organization of the United Nations (FAO). Available at: https://www.fao.org/faostat/en/#data/QCL
Gao, H., Chai, H. K., Cheng, N., and Cao, W. (2017). Effects of 24-epibrassinolide on enzymatic browning and antioxidant activity of fresh-cut lotus root slices. Food Chem. 217, 45–51. doi: 10.1016/j.foodchem.2016.08.063
Gutteridge, J. M. (1987). Ferrous-salt-promoted damage to deoxyribose and benzoate. The increased effectiveness of hydroxyl radical scavengers in the presence of EDTA. Biochem. J. 243, 709–714. doi: 10.1042/bj2430709
Jiang, S., and Penner, M. H. (2022). The effect of p-Coumaric acid on browning inhibition in potato polyphenol oxidase-catalyzed reaction mixtures. Foods 11:577. doi: 10.3390/foods11040577
Liu, X., Yang, Q., Lu, Y. Z., Li, Y., Li, T. T., Zhou, Y. B., et al. (2019). Effect of purslane (Portulaca oleracea L.) extract on anti-browning of fresh-cut potato slices during storage. Food Chem. 283, 445–453. doi: 10.1016/j.foodchem.2019.01.058
Lyu, J. I., Ryu, J., Jin, C. H., Kim, D. G., Kim, J. M., Seo, K. S., et al. (2020). Phenolic compounds in extracts of Hibiscus acetosella (cranberry hibiscus) and their antioxidant and antibacterial properties. Molecules 25:4190. doi: 10.3390/molecules25184190
Mahendran, G., and Rahman, L. U. (2020). Ethnomedicinal, phytochemical and pharmacological updates on peppermint (Mentha × piperita L.)—a review. Phytother. Res. 34, 2088–2139. doi: 10.1002/ptr.6664
Mittler, R., Zandalinas, S. I., Fichman, Y., and Van Breusegem, F. (2022). Reactive oxygen species signalling in plant stress responses. Nat. Rev. Mol. Cell Biol. 23, 663–679. doi: 10.1038/s41580-022-00499-2
Moon, K. M., Kwon, E. B., Lee, B., and Kim, C. Y. (2020). Recent trends in controlling the enzymatic browning of fruit and vegetable products. Molecules 25:2754. doi: 10.3390/molecules25122754
Nguyen, T. B. T., Ketsa, S., and Doorn, W. G. V. (2003). Relationship between browning and the activities of polyphenoloxidase and phenylalanine ammonia lyase in banana peel during low temperature storage. Postharvest Biol. Technol. 30, 187–193. doi: 10.1016/S0925-5214(03)00103-0
Oms-Oliu, G., Ma, R. G., González, L. A., Varela, P., Soliva-Fortuny, R., Hernando, M., et al. (2010). Recent approaches using chemical treatments to preserve quality of fresh-cut fruit: a review. Postharvest Biol. Tec. 57, 139–148. doi: 10.1016/j.postharvbio.2010.04.001
Padrón-Mederos, M., Rodríguez-Galdón, B., Díaz-Romero, C., LoboRodrigo, M. G., and Rodríguez-Rodríguez, E. M. (2020). Quality evaluation of minimally fresh-cut processed pineapples. LWT Food Sci. Technol. 129:109607. doi: 10.1016/j.lwt.2020.109607
Pan, Y. F., Chen, L., Pang, L. L., Chen, X. T., Jia, X. Y., and Li, X. H. (2020). Ultrasound treatment inhibits browning and improves antioxidant capacity of fresh-cut sweet potato during cold storage. RSC Adv. 10, 9193–9202. doi: 10.1039/c9ra06418d
Pateiro, M., Gómez-Salazar, J. A., Jaime-Patlán, M., Sosa, M. E., and Lorenzo, J. M. (2021). Plant extracts obtained with green solvents as natural antioxidants in fresh meat products. Antioxidants 10:181. doi: 10.3390/antiox10020181
Pundir, C. S., and Rawal, R. (2013). Determination of sulfite with emphasis on biosensing methods: a review. Anal. Bioanal. Chem. 405, 3049–3062. doi: 10.1007/s00216-013-6753-0
Qu, S. S., Li, M. M., Wang, G., Yu, W. T., and Zhu, S. (2021). Transcriptomic, proteomic and LC–MS analyses reveal anthocyanin biosynthesis during litchi pericarp browning. Sci. Hortic. 289:110443. doi: 10.1016/j.scienta.2021.110443
Remorini, D., Landi, M., Tardelli, F., Lugani, A., Massai, R., Graziani, G., et al. (2015). Effect of chlorine dioxide and ascorbic acid on enzymatic browning and shelf life of fresh-cut red delicious and granny smith apples. J. Food Process. Pres. 39, 2925–2934. doi: 10.1111/jfpp.12544
Sellami, K., Couvert, A., Nasrallah, N., Maachi, R., Abouseoud, M., and Amrane, A. (2022). Peroxidase enzymes as green catalysts for bioremediation and biotechnological applications: a review. Sci. Total Environ. 806:150500. doi: 10.1016/j.scitotenv.2021.150500
Siddiqui, M. W., Homa, F., Nayyer, M. A., Ghatak, A., Yousuf, B., Aftab, M. A., et al. (2020). Browning metabolism and quality of fresh-cut drumstick (Moringa oleifera) as influenced by acidulant treatments. J. Food Sci. Technol. 57, 2010–2016. doi: 10.1007/s13197-019-04233-3
Sikora, M., Złotek, U., and Świeca, M. (2020). Effect of basil leaves and wheat bran water extracts on enzymatic browning of shredded storage iceberg lettuce. Int. J. Food Sci. Tech. 55, 1318–1325. doi: 10.1111/ijfs.14406
Soysal, C. (2009). Effects of green tea extract on “golden delicious” apple polyphenoloxidase and its browning. J. Food Bio. Chem. 33, 134–148. doi: 10.1111/j.1745-4514.2008.00201.x
Sukhonthara, S., Kaewka, K., and Theerakulkait, C. (2016). Inhibitory effect of rice bran extracts and its phenolic compounds on polyphenol oxidase activity and browning in potato and apple puree. Food Chem. 190, 922–927. doi: 10.1016/j.foodchem.2015.06
Tinello, F., and Lante, A. (2018). Recent advances in controlling polyphenol oxidase activity of fruit and vegetable products. Innov. Food Sci. Emerg. 50, 73–83. doi: 10.1016/j.ifset.2018.10.008
Ullah, H., Hussain, Y., Santarcangelo, C., Baldi, A., Di Minno, A., Khan, H., et al. (2022). Natural poly phenols for the preservation of meat an d dairy products. Molecules 27:1906. doi: 10.3390/molecules27061906
Veiga, M., Costa, E. M., Silva, S., and Pintado, M. (2020). Impact of plant extracts upon human health: a review. Crit. Rev. Food Sci. Nutr. 60, 873–886. doi: 10.1080/10408398.2018.1540969
Venturi, F., Bartolini, S., and Chiara, S. (2019). Potato peels as a source of novel green extracts suitable as antioxidant additives for fresh-cut fruits. Appl. Sci. 9:2431. doi: 10.3390/app9122431
Vining, K. J., Johnson, S. R., Ahkami, A., Lange, I., Parrish, A. N., Trapp, S. C., et al. (2017). Draft genome sequence of mentha longifolia and development of resources for mint cultivar improvement. Mol. Plant 10, 323–339. doi: 10.1016/j.molp.2016.10.018
Wang, B., Huang, Y. Y., Zhang, Z. M., Xiao, Y. H., and Xie, J. (2022a). Ferulic acid treatment maintains the quality of fresh-cut taro (Colocasia esculenta) during cold storage. Front. Nut. 9:884844. doi: 10.3389/fnut.2022.884844
Wang, B., Lin, L. N., Yuan, X., Zhu, Y. N., Wang, Y. K., He, J. M., et al. (2023). Low-level cadmium exposure induced hormesis in peppermint young plant by constantly activating antioxidant activity based on physiological and transcriptomic analyses. Front. Plant Sci. 14:1088285. doi: 10.3389/fpls.2023.1088285
Wang, B., Wang, Y. K., Jiang, Y. Y., Huang, Y. Y., He, J. M., and Xiao, Y. H. (2022b). Anti-browning effects of citronellal on fresh-cut taro (Colocasia esculenta) slices under cold storage condition. Front. Sustain. Food Syst. 6:1001362. doi: 10.3389/fsufs.2022.1001362
Wang, B., Wu, C. S., Wang, G., He, J. M., and Zhu, S. J. (2021). Transcriptomic analysis reveals a role of phenylpropanoid pathway in the enhancement of chilling tolerance by pre-storage cold acclimation in cucumber fruit. Sci. Hortic. 288:110282. doi: 10.1016/j.scienta.2021.110282
Weerawardana, M. B. S., Thiripuranathar, G., and Paranagama, P. A. (2020). Natural antibrowning agents against polyphenol oxidase activity in Annona muricata and Musa acuminata. J. Chem. 2020, 1–6. doi: 10.1155/2020/1904798
Xiao, Y. H., He, J. M., Zeng, J., Yuan, X., Zhang, Z. M., and Wang, B. (2020). Application of citronella and rose hydrosols reduced enzymatic browning of fresh-cut taro. J. Food Biochem. 44:e13283. doi: 10.1111/jfbc.13283
Xiao, Y. H., Xie, J., Wu, C. S., He, J. M., and Wang, B. (2021). Effects of melatonin treatment on browning alleviation of fresh-cut foods. J. Food Biochem. 45:e13798. doi: 10.1111/jfbc.13798
Xiao, Y. H., Zhang, J. L., Jiang, Y. Y., Yuan, Y., Xie, J., and He, J. M. (2022). Cinnamic acid treatment reduces the surface browning of fresh-cut taro. Sci. Hortic. 291:110613. doi: 10.1016/j.scienta.2021.110613
Xu, Y., Wang, D., Zhao, W., Zheng, Y., Wang, Y., Wang, P., et al. (2022). Low frequency ultrasound treatment enhances antibrowning effect of ascorbic acid in fresh-cut potato slices. Food Chem. 380:132190. doi: 10.1016/j.foodchem.2022.132190
Yu, K., Zhou, L., Sun, Y. F., Zeng, Z. C., Chen, H. W., Liu, J. P., et al. (2021). Anti-browning effect of Rosa roxburghii on apple juice and identification of polyphenol oxidase inhibitors. Food Chem. 359:129855. doi: 10.1016/j.foodchem.2021.129855
Zhan, Q., Thakur, K., Feng, J. Y., Zhu, Y. Y., Zhang, J. G., and Wei, Z. J. (2023). LC–MS based metabolomics analysis of okara fermented by Bacillus subtilis DC-15: insights into nutritional and functional profile. Food Chem. 413:135656. doi: 10.1016/j.foodchem.2023.135656
Zhang, X. Y., Meng, W. B., Chen, Y. L., and Peng, Y. (2022). Browning inhibition of plant extracts on fresh-cut fruits and vegetables-a review. J. Food Process. Pres. 46:46. doi: 10.1111/jfpp.16532
Zhang, Q. A., Wang, X., Song, Y., Fan, X. H., and García Martín, J. F. (2016). Optimization of pyrogallol autoxidation conditions and its application in evaluation of superoxide anion radical scavenging capacity for four antioxidants. J. AOAC Int. 99, 504–511. doi: 10.5740/jaoacint.15-0223
Zhu, L. J., Hu, W. F., Murtaza, A. S., Iqbal, A., Li, J. X., Zhang, J., et al. (2022). Eugenol treatment delays the flesh browning of fresh-cut water chestnut (Eleocharis tuberosa) through regulating the metabolisms of phenolics and reactive oxygen species. Food Chem. 14:100307. doi: 10.1016/j.fochx.2022.100307
Keywords: antioxidant activity, natural anti-browning agent, postharvest treatment, surface discoloration, fresh-cut food
Citation: Yuan X, Tang B, Wang Y, Jiang Y, He J, Wang G, Yang P and Wang B (2023) Inhibitory effects of peppermint extracts on the browning of cold-stored fresh-cut taro and the phenolic compounds in extracts. Front. Sustain. Food Syst. 7:1191396. doi: 10.3389/fsufs.2023.1191396
Edited by:
Lourdes Maria Correa Cabral, Brazilian Agricultural Research Corporation (EMBRAPA), BrazilReviewed by:
Noor Liyana Yusof, Putra Malaysia University, MalaysiaQisen Xiang, Zhengzhou University of Light Industry, China
Copyright © 2023 Yuan, Tang, Wang, Jiang, He, Wang, Yang and Wang. This is an open-access article distributed under the terms of the Creative Commons Attribution License (CC BY). The use, distribution or reproduction in other forums is permitted, provided the original author(s) and the copyright owner(s) are credited and that the original publication in this journal is cited, in accordance with accepted academic practice. No use, distribution or reproduction is permitted which does not comply with these terms.
*Correspondence: Guang Wang, wguang@scau.edu.cn; Bin Wang, b_wang@sgu.edu.cn
‡ORCID: Bin Wang, https://orcid.org/0000-0002-6812-235X
†These authors have contributed equally to this work