- 1Department of Animal Nutrition and Management, Swedish University of Agricultural Sciences, Umeå, Sweden
- 2Department of Animal Nutrition and Management, Swedish University of Agricultural Sciences, Uppsala, Sweden
The objective was to evaluate whether methane (CH4) production from stored feces of cows previously supplemented with Asparagopsis taxiformis (AT) in their diet was lower compared with the feces of cows not supplemented with AT. We also investigated the possibility of further reducing CH4 production by adding AT to the stored feces of cows. Fecal samples were provided from a feeding trial (during two different periods) of four cows divided into two different groups. One group was supplemented with AT at a level of 0.5% of the total organic matter intake, and the other group was not supplemented with AT. A 2 × 2 factorial design was set in the laboratory for the incubation of feces. Fecal samples from the two groups of cows were divided into two subsamples receiving either no addition of AT or the addition of AT at a level of 0.5% of OM incubated. This resulted in four treatments with two replicates per period. The same design was repeated during period two. In total, 400 g of fresh fecal samples were incubated in 1 L serum bottles for 9 weeks at 39°C in a water bath. CH4 and total gas production were measured on days 1, 4, and 7 and subsequently every 2nd week until the end of the incubation period. Enteric CH4 production showed a significant reduction (61%) when AT was supplemented in the diet of dairy cows. We found that CH4 production from the feces of dairy cows supplemented with AT in their diet was only numerically lower (P = 0.61). Adding AT to the feces of dairy cows significantly reduced CH4 production from the feces by 44% compared with feces without AT. There were no differences observed in the bacterial and archaeal community profiles of fecal samples between cows fed AT and those not fed AT. This study concludes that the addition of AT to stored feces can effectively reduce CH4 production from the feces of dairy cows.
Introduction
There have been many efforts to decrease the carbon footprint from human activities to slow down climate change around the globe. Within the livestock sector, enteric methane (CH4) emissions from the ruminant sector play a key role. Enclosed by ruminants, the dairy sector is one of the most important contributors to CH4 emissions. It is estimated that ruminant livestock emits around one-third of anthropogenic CH4 (Jackson et al., 2020). Most CH4 produced by a dairy cow is from enteric fermentation, but the second largest source of CH4 is the decomposition of cows' feces (United States Environmental Protection Agency, 2006). Manure stored in the cool-temperate European climate is estimated to be responsible for ~12% of total CH4 emissions from the dairy system (Hindrichsen et al., 2015). However, manure is also used for biogas production, which is beneficial for the environment through the production of renewable energy (Huhtanen et al., 2021). The contribution of manure to greenhouse gas (GHG) emissions depends on many factors, such as the chemical composition of manure, the conditions under which manure is stored, and the temperature (Hassanat and Benchaar, 2019). For instance, incubated feces from cows that are fed vs. not fed rapeseed oil produced 3.45 and 3.85 L, respectively, of CH4 per kg of dry matter of incubated material (Ramin et al., 2021). Dietary strategies, such as high-concentrate feeding, will decrease enteric production of CH4 while increasing fecal output of potentially digestible organic matter (pdOM; Huhtanen et al., 2021). This will increase the production of CH4 from manure as the amount of fermentable substrate ending up in the feces also increases (Møller et al., 2014). In Sweden and some other Nordic countries, the Tier 2 methodology of the IPCC is used to predict CH4 production from manure (Intergovernmental Panel on Climate Change, 2006). The prediction equations developed by the IPCC do not take into account the effects of using inhibitory compounds or inducing changes in the composition of feces due to diet changes. This will lead to an overestimation/underestimation of CH4 production from manure.
Several strategies have been reported to decrease enteric CH4 production from dairy cows. Recently, a red macroalgae native from tropical areas, Asparagopsis taxiformis (AT), was identified as a natural enteric CH4 mitigator. The most exploited halogenated compound found in AT is bromoform (CHBr3). Bromoform mitigates CH4 production in the rumen by impairing the final step in methanogenesis (Duin et al., 2016). Stefenoni et al. (2021) showed a decrease of ~65% in CH4 production from dairy cows fed AT by the inclusion of AT at 0.5% of dry matter intake (DMI). However, there are concerns about using AT in the diet of dairy cows since traces of CHBr3 have been identified in the milk of dairy cows (Stefenoni et al., 2021), and a recent study showed inflammatory damages to the rumen wall of dairy cows supplemented with AT (Muizelaar et al., 2021). A more practical solution for using AT to reduce CH4 emissions could be the addition of AT to the manure of dairy cows. Only a few studies in the literature present strategies to decrease GHG from manure, and most of them are related to emissions of nitrous oxide (N2O).
The first objective of this study was to estimate CH4 production from stored feces of cows previously supplemented or not supplemented with AT in their diet. The second objective was to evaluate whether CH4 production could be mitigated by adding AT to the feces of dairy cows.
We hypothesized that feces from cows supplemented with AT in their diet would produce less CH4 than feces from cows not supplemented with AT and that the addition of AT to incubated cows' feces might impair methanogenesis during feces decomposition, resulting in decreased CH4 production.
Materials and methods
We collected fecal samples in connection to a feeding trial conducted at the Röbäcksdalen experimental farm of the Swedish University of Agricultural Sciences in Umeå (63°45′ N, 20°17′ E) that aimed to evaluate the effect of supplementing AT to a grass silage-based diet fed to dairy cows. More detail on the feeding trial can be found in the study by Krizsan et al. (2023). In brief, six Nordic Red dairy cows at 122 ± 13.7 (mean ± SD) days in milk, parity 2.7 ± 0.52, and producing 36 ± 2.5 kg milk/day at the start of the experiment were assigned to a Latin square change-over design comprising two dietary treatments (diet supplemented and not supplemented with AT). Cows were kept in an insulated free-stall barn with free access to fresh water. The cows were fed a basal diet consisting of grass silage and a commercial concentrate at a ratio of 60:40 on a dry matter basis. The basal diet was either not supplemented or supplemented with 0.5% of AT on a total organic matter (OM) intake basis. The diets were fed to the cows as a total mixed ration (TMR) ad libitum. The diets were mixed using the TMR mixer (Nolan A/S) and delivered to the feed troughs three times per day by an automatic feeding wagon. Feed intake was monitored with Roughage Intake Control feeders (Insentec B.V., Marknesse, the Netherlands).
Enteric CH4 production was measured using the GreenFeed system (C-Lock Inc., Rapid City, SD, USA), as described by Huhtanen et al. (2015). The GreenFeed was set to allow all six cows in the experiment (previously trained) to visit the system at 5 h intervals. To attract the animals to visit the GreenFeed regularly, eight drops of ~50 g (each drop) of pelleted concentrate, the same commercial concentrate (Komplett Amin 180; Lantmännen Lantbruk AB, Malmö, Sweden) as in the TMR, were given to the cows. The interval set between each drop was 40 s.
Fecal samples were collected during two periods from two cows not supplemented with AT (control) and from two cows supplemented with AT at a level of 0.5% of OM intake. We collected fecal samples from only two cows per treatment since we had limited space in the laboratory for measurements of fecal CH4 production. A 2 × 2 factorial design was set for the incubation of feces in the laboratory. Each fecal sample (four in total) was divided into two subsamples. Each subsample received one of the two treatments; no addition of AT to the fecal sample (control) or addition of AT to the fecal sample at a level of 0.5% of OM incubated. This resulted in four treatments with two replicates per treatment per period. The same design was repeated during the second period. The experimental design applied to the incubation of feces to measure fecal CH4 production is shown in Figure 1.
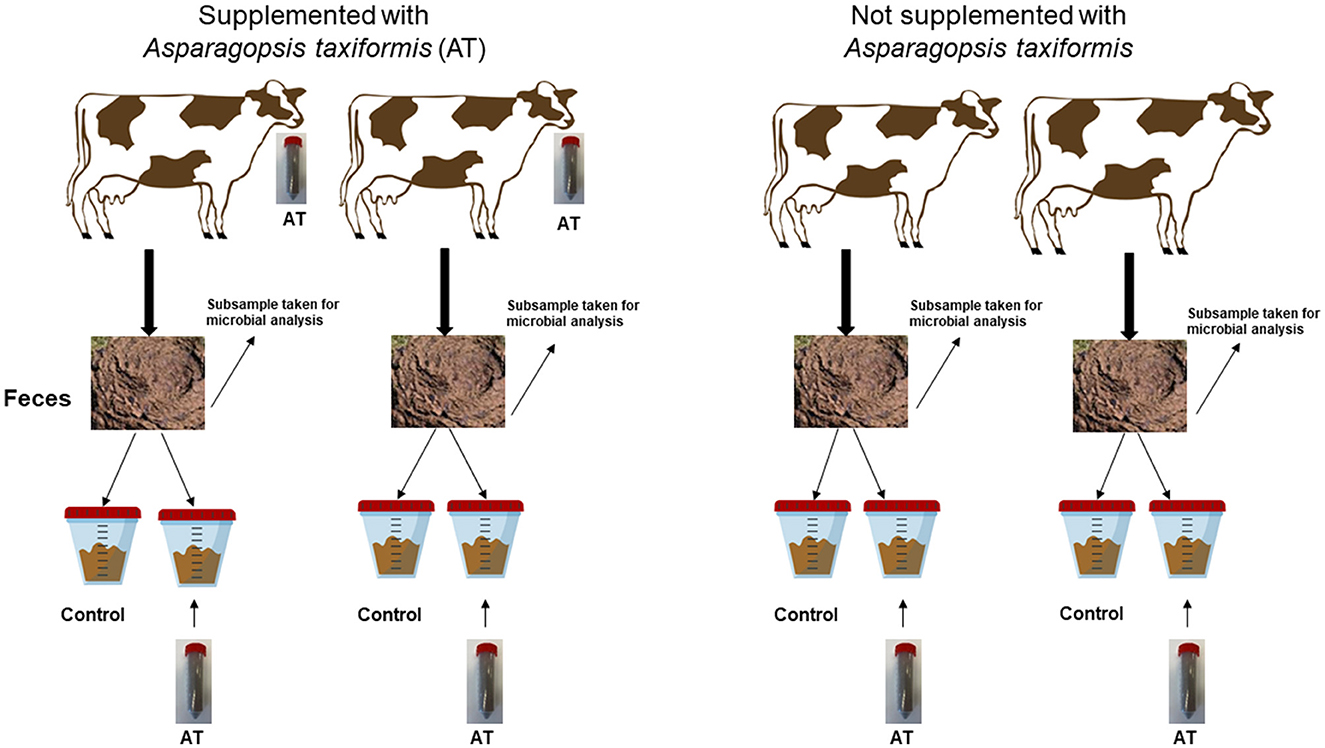
Figure 1. A schematic view of the experimental design applied to the incubation of feces to measure fecal CH4 production.
The method described by Ramin et al. (2021) was used for measuring CH4 production from feces. In brief, 400 g of fresh fecal sample collected from the cows was incubated in eight 1 L serum bottles for 9 weeks at 39°C in a water bath. Asparagopsis taxiformis was added to four of the bottles (Figure 1). Bottles were gently shaken three times per week. The 1 L serum bottles were connected to gastight inert foil sampling bags (SupelTM-Supelco, USA). Methane and total gas production were measured on days 1, 4, and 7 and then every 2nd week until the end of the incubation.
Cumulative CH4 production was calculated based on the sum of CH4 production at each time point as follows:
where HSCH4 is the headspace CH4 concentration; bag CH4 is the CH4 concentration in the sampling bag; and 600 is the headspace volume in the serum bottles. The concentration of CH4 in the gas sample was determined by injecting 0.2 ml of gas withdrawn from the headspace/sampling bag into a gas chromatograph using a gastight syringe, as described by Ramin and Huhtanen (2012).
Fecal samples from the four cows selected for the fecal incubations were dried at 105°C for 16 h. Thereafter, samples were milled using a cutter mill (SM 300, Retsch Ltd., Haan, Germany) to pass through a 1 mm sieve for neutral detergent fiber (NDF) and OM analysis. Subsamples were also milled to pass a 2 mm sieve for indigestible NDF (iNDF) analysis. Ash was determined on dried fecal samples by combustion at 500°C for 4 h (AOAC International, 2000). Organic matter was calculated as 1,000—ash. The NDF concentration of fecal samples was determined using heat stable α-amylase and sodium sulfite (Mertens, 2002) in an Ankom200 Fiber Analyzer (Ankom Technology Corp., Macedon, NY, USA). Indigestible NDF was determined as duplicate samples of 2 g which were weighed into polyester bags of 11 μm pore size (Krizsan et al., 2015). The bags were incubated for 288 h in the rumen of three cannulated cows that were fed a grass silage-based diet, and iNDF was expressed on an ash-free basis (Huhtanen et al., 1994). The potentially digestible NDF (pdNDF, g/kg DM) was calculated as the difference between NDF and iNDF. Neutral detergent soluble (NDS) was calculated as the difference between OM and NDF. The pdOM was calculated as the sum of NDS and pdNDF.
Subsamples of 15 g from the feces of cows supplemented with and without AT in the diet were collected, frozen at −80°C, and sent to Novogene, UK, for microbial community analysis. Community DNA was isolated using the TIAN amp Stool DNA Kit, as per the manufacturer's instructions with slight modifications. The extracted DNA from the feces was subjected to 16S rRNA gene (V4 region) amplification, targeting both Bacteria and Archaea using the primer pair 515F (GTGBCAGCMGCCGCGGTAA)/805R (GACTACHVGGGTATCTAATCC). The amplified product was further used for meta-barcoding sequencing using the PE250 strategy on the Illumina sequencing platform (Hi Seq 2500), and the raw sequencing reads were processed using USEARCH version 11 (Edgar, 2010), according to Pal et al. (2022). The OTU files were further used for classification using RDP classifier version 2.11, database release 11.5 (Cole et al., 2014), and the final image was rendered in MEGAN-LR (Huson et al., 2018). OTUs representing archaeal taxa were extracted using MEGAN-LR for the graphical representation (Huson et al., 2018).
Dry matter intake, enteric hydrogen, and enteric CH4 production data were subjected to the analysis of variance using the PROC GLM procedure in SAS (SAS Inc. 2002–2003, Release 9.4 SAS Inst. Inc., Cary, NC, USA) by applying the following model:
where μ = the overall mean, Di = the effect of dietary treatment (supplemented with AT or not), Pj = the effect of period, Ck = the effect of cow, and εijk = random residual error.
We adopted the following model (PROC GLM) using the statistical package of SAS (9.4) for analyzing CH4 and total gas production from stored feces:
where μ = the overall mean, Fi = the effect of level i of factor F (cow) supplemented with AT to diet or not, Aj = the effect of level j of factor A adding AT to feces or not, Pk = the effect of level k of the period, (FA)ij = the effect of the interaction between F and A, and εijk = random residual error.
Results
The chemical composition of the feces of cows with or without AT supplementation in their diet is presented in Table 1. The pdOM content in the feces of cows supplemented with AT was rather similar to the feces of cows not supplemented with AT (Table 1). Supplementing AT to the diet of dairy cows decreased daily enteric CH4 production, g/d (P = 0.01) by 61% and DMI (P = 0.03) by 3.7 kg/d (Table 2). Methane yield also decreased (P = 0.02) from cows supplemented with AT (9 g/kg DMI lower) than from cows not supplemented with AT (Table 2). As a consequence of reduced CH4 production from cows supplemented with AT in their diet, hydrogen production was greater (P = 0.02) for cows supplemented with AT (5.02 vs. 1.07 g/d, respectively; Table 2). Methane production was numerically lower (P = 0.61) in the feces of cows supplemented with AT in their diet compared to feces of cows not supplemented with AT in their diet, 3.73 vs. 4.13 L/kg DM, respectively (Table 3). Adding AT to the feces of cows reduced (P < 0.01) CH4 production from the feces by 44% compared with feces without the addition of AT, 2.84 vs. 5.04 L/kg DM (Table 3). Total gas production was also lower (P = 0.03) in feces with the addition of AT than in feces without the addition of AT (Table 3).
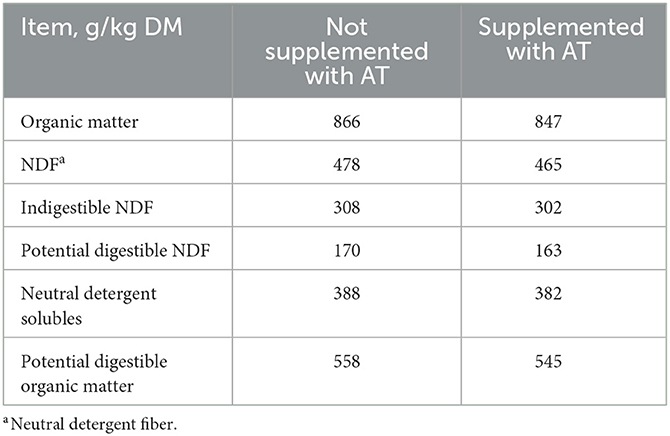
Table 1. Chemical composition of feces of cows (n = 4) not supplemented or supplemented with Asparagopsis taxiformis (AT) in their diet.

Table 2. Effect of supplementation of Asparagopsis taxiformis (AT) to the diet of dairy cows (n = 4) on dry matter intake (DMI) and enteric methane (CH4) production.
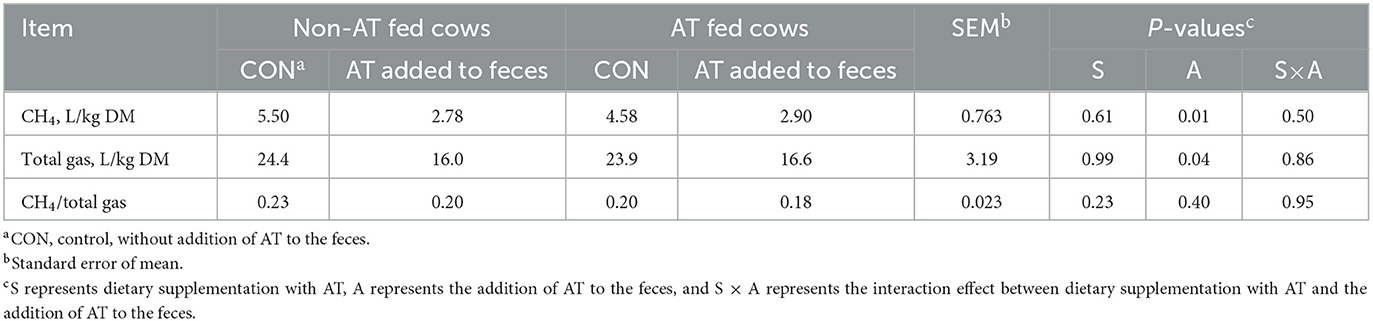
Table 3. Methane (CH4) production from stored feces (n = 8) of cows supplemented or not supplemented with Asparagopsis taxiformis (AT) in their diet and with or without the addition of AT to feces.
In eight samples, a total of 823,839 clean reads were observed with an average read length of 102,980. A total of 19,638 OTUs were observed, among which 61 OTUs were classified as Archaea and 19,181 OTUs as Bacteria. No significant variation was observed in the archaeal and bacterial community profiles of feces samples from cows supplemented with AT and non-AT supplemented diets. Firmicutes and Bacteroidetes were the most dominant bacterial phyla, followed by Proteobacteria, Actinobacteria, and Acidobacteria, constituting over ~95% of total OTU relative abundance (Figure 2A). In addition, Gemmatimonadetes, Synergistetes, and Planctomycetes were only observed in non-AT samples. Euryarchaeota was the only observed archaeal phyla in both AT and non-AT samples. At the genus level, Methanobrevibacter, Methanosphaera, and Methanocorpusculum (within the phylum Euryarchaeota) were present in both AT and non-AT samples (Figure 2B). The average species richness (Chao-1) was greater in the AT-fed group compared with the non-AT-fed group, while no contrasting differences were observed in alpha diversity (Simpson and Shannon) and beta diversity (data not shown).
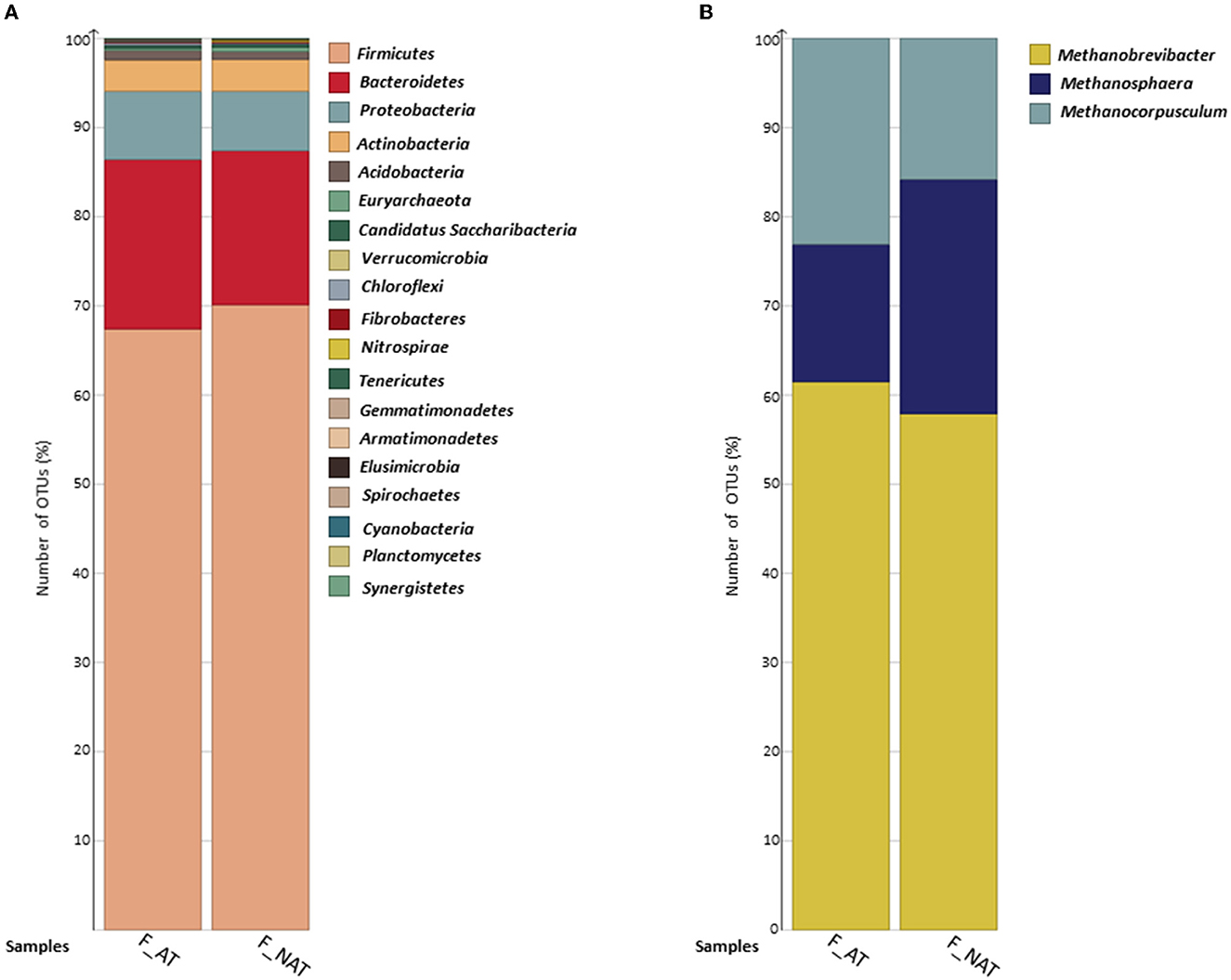
Figure 2. Bar chart represents the percent distribution of (A) bacterial and (B) archaeal OTUs at phylum and genus levels, respectively. AT (n = 4) and NAT (n = 4) samples. Archaeal OTUs were extracted from the total observed OTUsfor better visualization. AT, feces from cows supplemented with Asparagopsis taxiformis in their diet; NAT, feces from cows not supplemented with Asparagopsis taxiformis in their diet.
Discussion
We showed that supplementing AT to the diet of Nordic dairy cows significantly reduces DMI and enteric CH4 production. We also found that adding AT to the feces of dairy cows further decreases CH4 production. Methane production from the feces of cows that have previously been supplemented with AT in their diet is not significantly decreased compared with the feces of cows not supplemented with AT. However, the addition of AT to feces showed that around 44% of CH4 production could be reduced from the feces of dairy cows.
To date, AT is the most promising naturally derived inhibitor of CH4 production from dairy cows (Wasson et al., 2022). The most reported CH4 inhibitor active compound in AT is CHBr3, and it acts by inhibiting the enzyme methyl-coenzyme M reductase (MCR), which catalyzes the final step in methanogenesis in rumen archaea (Duin et al., 2016). Since the main compound reducing CH4 in AT is CHBr3, as shown by Stefenoni et al. (2021) in dairy cows, the addition of other halogenated compounds is a feasible method for reducing CH4 production from manure as well. Inhibiting CH4 production from anaerobic digesters is a relevant concern nowadays for the livestock sector that intends to utilize the manure in their fields (Wasson et al., 2022). In addition to CH4, N2O is another GHG that has a significant effect on global warming with a 265 times higher global warming potential than carbon dioxide (Intergovernmental Panel on Climate Change, 2014). Compared to CH4 production, N2O could be mitigated more easily from manure since dietary optimization of protein consumption will lead to improved nitrogen utilization and result in lower nitrogen loss to the manure (Novak and Fiorelli, 2010). However, mitigating enteric CH4 production from dairy cows seems to be more challenging, since it might affect the productivity of the animals as well. In addition, it might be easier to reduce the emissions of CH4 from manure, although a lower amount of CH4 is produced from the manure compared with enteric fermentation. We should also take into account that supplementing dairy cows with AT and the related environmental benefits will depend on the producer's acceptance of the current cost of AT (Wasson et al., 2022).
Other halogenated compounds could also be tested and used, e.g., 3-nitrooxypropanol (3NOP). We used AT as the CH4 inhibitor in this study since 3NOP and AT both have the same mode of action and block the enzyme in the fermentation process in the methanogens, producing CH4 in the rumen. Using 3NOP could be a cheaper option for both feeding the animal and adding manure to mitigate CH4 production. The effect of 3NOP on CH4 inhibition in dairy cows is, however, smaller than the effect of AT. In a study conducted by Hristov et al. (2015), enteric CH4 production was reduced by ~30% from dairy cows supplemented with 3NOP compared with the control group. Supplementing dairy cows with AT also has potentially negative effects on their production performance. We found that DMI was significantly reduced, similar to the findings by Stefenoni et al. (2021). In addition, they found that milk yield decreased, which is also supported by the findings of Krizsan et al. (2023), who showed a decrease in energy-corrected milk yield from cows supplemented with AT. The use of AT in the diet might also limit the market adoption if negative side effects of AT supplementation are observed (Wasson et al., 2022). In a recent study, Muizelaar et al. (2021) reported that feeding AT to dairy cows showed signs of inflammation in the rumen.
One assumption is that dietary strategies that reduce enteric CH4 production are compensated by increased CH4 production from the manure, which is called a trade-off between enteric and fecal CH4 production. This could be due to the presence of soluble carbohydrates (volatile solids) in the feces, which are later fermented and converted into CH4. Benchaar et al. (2015) found that the addition of linseed oil to dairy cows fed a corn silage-based diet caused a decrease in enteric CH4 production (−26%). However, Hassanat and Benchaar (2019) found that CH4 production (L/kg OM) from feces was greater from cows fed a corn silage-based diet supplemented with linseed oil because of the bypass of fermentable substrates into the feces. However, in this study, pdOM (could also be referred to as volatile solids) in the feces was rather similar between the group of cows supplemented with AT in their diet and the control group of cows not supplemented with AT. This is also in line with the non-significant differences in CH4 production from feces between the same two groups of cows.
Studies looking into the archaeal and bacterial community in the feces of cows supplemented with AT in their diet are rare. One of the aims of the current study was to evaluate whether the population of the bacterial and archaeal communities will differ between the feces of cows supplemented with AT and the feces of cows not supplemented with AT. At the phyla level, Firmicutes was the most abundant group followed by Bacteroidetes, which is in accordance with previous studies where microbial communities in feces from dairy cows have been examined (Callaway et al., 2010; Hagey et al., 2019; Williamson et al., 2022). In the study by Williamson et al. (2022), there were clear differences between the microbial community in the rumen and the microbial community in the feces, and the main difference was related to an increase in Firmicutes in relation to Bacteroidetes. However, for the bacterial community, no differences were observed in feces from cows supplemented with AT or not supplemented with AT. Henderson et al. (2015) conducted a study with 742 rumen samples of foregut microbial community composition from 35 countries, and they found that similar bacteria and archaea dominated in nearly all samples.
The effect of AT is mainly related to the inhibition of methanogenesis in the archaeal community, and further effects on the bacterial population are not well-studied. In this study, archaea were only represented by Euryarchaeota at the phyla level, which is in line with the study by Jin et al. (2017). Most of the methanogens in liquid manure originate from the animal's gut, and Methanobrevibacter is, in general, the most abundant genus in dairy cows, both in the rumen and feces (Henderson et al., 2015; Jin et al., 2017). In this study, Methanobrevibacter was found to be the most abundant genus in the feces of cows, regardless of whether their diets were supplemented with AT or not. The similarity observed between treatments in the microbial community of manure aligns with the lack of an effect on CH4 production (L/kg DM) from feces.
It is anticipated that there will be a noticeable distinction in treatments as a result of inhibiting methanogenesis in methanogens within the rumen, subsequently leading to observable changes in the feces. Nevertheless, it has been shown that the CH4-inhibiting compound present in AT, CHBr3, is quickly metabolized in the rumen (Romero et al., 2022) and that it is not excreted in the manure of the animals (Muizelaar et al., 2021). Due to the quick degradation of CHBr3 in the rumen (Muizelaar et al., 2021), an effect on active methanogens in the feces might not be present.
Our results showed that the inhibitory effect of supplementing dairy cow diets with AT was smaller on CH4 production from feces compared with the effect on CH4 production from enteric fermentation. Asparagopsis taxiformis produces a wide range of different halogenated compounds (Woolard et al., 1979), and the potency of the inhibitory effect of these different compounds varies (Lanigan, 1972). Our results revealed that CH4 production from the feces of cows supplemented with AT in their diet was numerically lower compared with the feces of cows not supplemented with AT. However, we should note that this study was a pilot study; it brought new insights into reducing CH4 production from manure. More research is needed with a greater number of cows in future experiments to confirm the results of this study. Methane production could be further reduced by the addition of AT to the manure. Nonetheless, more studies are also required to be conducted to elucidate the interactions between the halogenated compounds of ATs and the microbiome of feces.
Conclusion
This study concluded that daily enteric CH4 production and CH4 yield were reduced when AT was supplemented to the diet of Nordic Red dairy cows. It should, however, be noted that DMI was also affected and reduced by dietary AT supplementation. Methane production from feces could be reduced by up to 44% with the addition of AT to the stored feces. No differences were observed in the distribution of the bacterial and archaeal communities between the feces of cows that were supplemented with AT in their diet and those that were not. Further studies are necessary to investigate the interactions between the halogenated compounds of ATs and the fecal microbiome, in order to provide a deeper understanding of their effects.
Data availability statement
The datasets presented in this study can be found in online repositories. The names of the repository/repositories and accession number(s) can be found at: https://www.ncbi.nlm.nih.gov/genbank/, PRJNA906285.
Ethics statement
The handling of animals in this experiment was approved by the Swedish Ethics Committee on Animal Research (Dnr A 32-16), represented by the Court of Appeal for Northern Norrland in Umeå, and the experiment was carried out in accordance with laws and regulations governing experiments performed with live animals in Sweden.
Author contributions
MR: conceptualization, formal analysis, funding acquisition, methodology, project administration, resources, supervision, and writing the manuscript. JC: methodology and helped in writing the manuscript. YP: methodology, microbial analysis, and writing the manuscript. RD: microbial analysis and edited the manuscript. PF: statistical analysis and writing and editing of the manuscript. SK: conceptualization and writing the manuscript. All authors contributed to the article and approved the submitted version.
Funding
This study was partly funded by the RumenPredict Project (FACCE ERA-GAS) as part of the EU Horizon 2020 Research and Innovation Program and Carl Tryggers Stiftelsen, Sweden (project number: 24837801).
Acknowledgments
We acknowledge the staff working on the farm who helped with the in vivo trial and the laboratory staff for their support in conducting this study.
Conflict of interest
The authors declare that the research was conducted in the absence of any commercial or financial relationships that could be construed as a potential conflict of interest.
Publisher's note
All claims expressed in this article are solely those of the authors and do not necessarily represent those of their affiliated organizations, or those of the publisher, the editors and the reviewers. Any product that may be evaluated in this article, or claim that may be made by its manufacturer, is not guaranteed or endorsed by the publisher.
References
Benchaar, C., Hassanat, F., Martineau, R., and Gervais, R. (2015). Linseed oil supplementation to dairy cows fed red clover silage- or corn silage-based diets: effects on methane production, rumen fermentation, nutrient digestibility, N balance, and milk production. J. Dairy Sci. 98, 7993–8008. doi: 10.3168/jds.2015-9398
Callaway, T. R., Dowd, S. E., Edrington, T. S., Anderson, R. C., Krueger, N., Bauer, N., et al. (2010). Evaluation of bacterial diversity in the rumen and feces of cattle fed different levels of dried distillers grains plus solubles using bacterial tag-encoded FLX amplicon pyrosequencing. J. Anim. Sci. 12, 3977–3983. doi: 10.2527/jas.2010-2900
Cole, J. R., Wang, Q., Fish, J. A., Chai, B., McGarrell, D. M., Sun, Y., et al. (2014). Ribosomal Database Project: data and tools for high throughput rRNA analysis. Nucleic Acids Res. 42, D633–D642. doi: 10.1093/nar/gkt1244
Duin, E. C., Wagner, T., Shima, S., Prakash, D., Cronin, B., Yáñez-Ruiz, D. R., et al. (2016). Mode of action uncovered for the specific reduction of methane emissions from ruminants by the small molecule 3-nitrooxypropanol. Proc. Natl. Acad. Sci. U. S. A. 113, 6172–6177. doi: 10.1073/pnas.1600298113
Edgar, R. C. (2010). Search and clustering orders of magnitude faster than BLAST. Bioinformatics 26, 2460–2461. doi: 10.1093/bioinformatics/btq461
Hagey, J. V., Bhatnagar, S., Heguy, J. M., Karle, B. M., Price, P. L., Meyer, D., et al. (2019). Fecal microbial communities in a large representative cohort of California dairy cows. Front. Microbiol. 10, 1093. doi: 10.3389/fmicb.2019.01093
Hassanat, F., and Benchaar, C. (2019). Methane emissions of manure from dairy cows fed red clover or corn silage based diets supplemented with linseed oil. J. Dairy. Sci. 102, 11766–11776. doi: 10.3168/jds.2018-16014
Henderson, G., Cox, F., Ganesh, S., Jonker, A., Young, W., Census, G. R., et al. (2015). Rumen microbial community composition varies with diet and host, but a core microbiome is found across a wide geographical range. Sci. Rep. 5, 14567. doi: 10.1038/srep14567
Hindrichsen, I. K., Wettstein, H. R., Machmüller, A., Jörg, B., and Kreuzer, M. (2015). Effect of the carbohydrate composition of feed concentrates on methane emission from dairy cows and their slurry. Environ. Mont. Asses. 107, 329–350. doi: 10.1007/s10661-005-3008-3
Hristov, A. N., Oh, J., Giallongo, F., Frederick, T., Harper, M., Weeks, H., et al. (2015). An inhibitor persistently decreased enteric methane emission from dairy cows with no negative effect on milk production. Proc. Natl. Acad. Sci. U. S. A 112, 10663–10668. doi: 10.1073/pnas.1504124112
Huhtanen, P., Cabezas-Garcia, E. H., Utsumi, S., and Zimmerman, S. (2015). Comparison of methods to determine methane emissions from dairy cows in farm conditions. J. Dairy Sci. 98, 3394–3409. doi: 10.3168/jds.2014-9118
Huhtanen, P., Kaustell, K., and Jaakkola, S. (1994). The use of internal markers to predict total digestibility and duodenal flow of nu-triens in cattle given six different diets. Anim. Feed Sci. Technol. 48, 211–227. doi: 10.1016/0377-8401(94)90173-2
Huhtanen, P., Krizsan, S. J., and Ramin, M. (2021). Modelling faecal output and nutrient composition, and maximal methane emission from manure of dairy cows. Anim. Feed Sci. Tech. 282, 115120. doi: 10.1016/j.anifeedsci.2021.115120
Huson, D. H., Albrecht, B., Bagci, C., Bessarab, I., Górska, A., Jolic, D., et al. (2018). MEGAN-LR: new algorithms allow accurate binning and easy interactive exploration of metagenomic long reads and contigs. Biol. Direct. 13, 6. doi: 10.1186/s13062-018-0208-7
Intergovernmental Panel on Climate Change (2006). “Chapter 10 emissions from livestock and manure management,” in IPCC Guidelines for National Greenhouse Gas Inventories, Vol 4. Agriculture, Forestry and Other Land Use, Prepared by the National Greenhouse Gas Inventories Programme, eds S. Eggleston, L. Buendia, K. Miwa, T. Ngara, and K. Tanabe (IGES). Available online at: http://www.ipcc-~nggip.iges.or.jp/public/2006gl/vol4.html (accessed February 25, 2023).
Intergovernmental Panel on Climate Change (2014). “AR5. Climate change 2014: mitigation,” in Contribution of Working Group III to the Fifth Assessment Report of the Intergovernmental Panel on Climate Change, eds O. Edenhofer, et al. (New York, NY: Cambridge University Press). Available online at: https://www.ipcc.ch/report/ar5/wg3/ (accessed November 7, 2022).
Jackson, R. B., Saunois, M., Bousquet, P., Canadell, J. G., Poulter, B., Stavert, A. R., et al. (2020). Increasing anthropogenic methane emissions arise equally from agricultural and fossil fuel sources. Environ. Res. Lett 15, 1002. doi: 10.1088/1748-9326/ab9ed2
Jin, D., Kang, K., Wang, H., Wang, Z., Xue, B., Wang, L., et al. (2017). Effects of dietary supplementation of active dried yeast on fecal methanogenic archaea diversity in dairy cows. Anaerobe 44, 78–86. doi: 10.1016/j.anaerobe.2017.02.007
Krizsan, S. J., Ramin, M., Chagas, J. C. C., Halmemies-Beauchet-Filleau, A., Singh, A., Schnürer, A., et al. (2023). Effects on rumen microbiome and milk quality of dairy cows supplemented the macroalga Asparagopsis taxiformis in a grass silage-based diet. Front. Anim. Sci. Anim. Nutr. 2023, 1112969. doi: 10.3389/fanim.2023.1112969
Krizsan, S. J., Rinne, M., Nyholm, L., and Huhtanen, P. (2015). New recommendations for the ruminal in situ determination of indigest-ible neutral detergent fibre. Anim. Feed Sci. Technol. 205, 31–41. doi: 10.1016/j.anifeedsci.2015.04.008
Lanigan, G. (1972). Metabolism of pyrrolizidine alkaloids in the ovine rumen. IV. Effects of chloral hydrate and halogenated methanes on rumen methanogenesis and alkaloid metabolism in fistulated sheep. Crop. Pasture Sci. 23, 1085–1091. doi: 10.1071/AR9721085
Mertens, D. R. (2002). Gravimetric determination of amylase-treated neutral detergent fiber in feeds with refluxing in beakers or crucibles: collaborative study. J. AOAC Int. 85, 1217–1240.
Møller, H. B., Moset, V., Brask, M., Weisbjerg, M. R., and Lund, P. (2014). Feces composition and manure derived methane yield from dairy cows: influence of diet with focus on fat supplement and roughage type. Atmos. Environ. 94, 36–43. doi: 10.1016/j.atmosenv.2014.05.009
Muizelaar, W., Groot, M., van Duinkerken, G., Peters, R., and Dijkstra, J. (2021). Safety and transfer study: transfer of bromoform present in Asparagopsis taxiformis to milk and urine of lactating dairy cows. Foods 10, 584. doi: 10.3390/foods10030584
Novak, S. M., and Fiorelli, J. L. (2010). Greenhouse gases and ammonia emissions from organic mixed crop-dairy systems: a critical review of mitigation options. Agron. Sustain. Dev. 30, 215–236. doi: 10.1051/agro/2009031
Pal, Y., Mayilraj, S., and Krishnamurthi, S. (2022). Exploring the distinct distribution of archaeal communities in sites contaminated with explosives. Biomolecules 12, 489. doi: 10.3390/biom12040489
Ramin, M., Chagas, J. C., Smidt, H., Gomez Exposito, R., and Krizsan, S. (2021). Enteric and fecal methane emissions from dairy cows fed grass or corn silage diets supplemented with rapeseed oil. Animals 11, 1322. doi: 10.3390/ani11051322
Ramin, M., and Huhtanen, P. (2012). Development of an in vitro method for determination of methane production kinetics using a fully automated in vitro gas system—A modeling approach. Anim. Feed Sci. Technol. 174, 190–200. doi: 10.1016/j.anifeedsci.2012.03.008
Romero, P., Belanche, A., Hueso, R., Ramos-Morales, E., Salwen, J. K., Kebreab, E., et al. (2022). “In vivo rumen microbial degradation of bromoform and the impact on rumen fermentation,” in 8th Annual Global Greenhouse Gas & Animal Agriculture Conference, (Orlando, FL), 291.
Stefenoni H. A. Räisänen S. E. Cueva S. F. Wasson D. E. Lage C. F. A. Melgar A. . (2021). Effects of the macroalga Asparagopsis taxiformis and oregano leaves on methane emission, rumen fermentation, and lactational performance of dairy cows. J. Dairy Sci. 104, 4157–4173. doi: 10.3168/jds.2020-19686
United States Environmental Protection Agency (2006). Global Anthropogenic Non-CO2 Greenhouse Gas Emissions: 1990-2020, EPA 430-R-06-003. Washington DC: United States Environmental Protection Agency.
Wasson, D. E., Yarish, C., and Hristov, A. N. (2022). Enteric methane mitigation through Asparagopsis taxiformis supplementation and potential algal alternatives. Front. Anim. Sci. 3, 999338. doi: 10.3389/fanim.2022.999338
Williamson, J. R., Callaway, T. R., Lourenco, J. M., and Ryman, V. E. (2022). Characterization of rumen, fecal, and milk microbiota in lactating dairy cows. Front. Microbiol. 13, 984119. doi: 10.3389/fmicb.2022.984119
Keywords: climate, methane from manure, dairy cows, seaweed, red algae
Citation: Ramin M, Chagas JCC, Pal Y, Danielsson R, Fant P and Krizsan SJ (2023) Reducing methane production from stored feces of dairy cows by Asparagopsis taxiformis. Front. Sustain. Food Syst. 7:1187838. doi: 10.3389/fsufs.2023.1187838
Received: 16 March 2023; Accepted: 09 June 2023;
Published: 13 July 2023.
Edited by:
Arif Reza, Stony Brook University, United StatesReviewed by:
Wenbing Tan, Chinese Research Academy of Environmental Sciences, ChinaAshish Kumar Das, Jashore University of Science and Technology, Bangladesh
Copyright © 2023 Ramin, Chagas, Pal, Danielsson, Fant and Krizsan. This is an open-access article distributed under the terms of the Creative Commons Attribution License (CC BY). The use, distribution or reproduction in other forums is permitted, provided the original author(s) and the copyright owner(s) are credited and that the original publication in this journal is cited, in accordance with accepted academic practice. No use, distribution or reproduction is permitted which does not comply with these terms.
*Correspondence: Mohammad Ramin, bW9oYW1tYWQucmFtaW5Ac2x1LnNl