- 1ICAR-Indian Agricultural Research Institute, New Delhi, India
- 2ICAR-Central Potato Research Institute, Shimla, Himachal Pradesh, India
- 3ICAR-National Research Centre for Banana, Tiruchirappalli, Tamil Nadu, India
- 4Vasantrao Naik Marathwada Krishi Vidyapeeth, Parbhani, Maharashtra, India
- 5ICAR-Indian Agricultural Research Institute, Regional Station, Karnal, Haryana, India
- 6ICAR-Indian Institute of Soil and Water Conservation, Dehradun, Uttarakhand, India
System of Wheat Intensification (SWI) can outperform conventional wheat cultivation. However, the manual planting (dibbling) of two seeds in each hill, with hills laid out in a 20 × 20 cm square pattern essential for SWI, is a laborious task and requires more time, manpower, energy, and monetary expenditure. To deal with these constraints, a scientific team of Agricultural Engineers and Agronomists at ICAR-Indian Agricultural Research Institute, New Delhi, developed a single-row manual SWI-planter (SRMSWIP) to make SWI planting easier, faster, and more economical. The SRMSWIP was field-evaluated for wheat var. HD-2967 during the winter season of 2015–2016 in a randomized complete design block thrice-replicated study with eight treatments. These treatments comprised (i) sowing with SRMSWIP using treated seeds (MSWIT); (ii) sowing with SRMSWIP using non-treated seed (MSWINT); (iii) manual sowing with SWI-management using treated seeds (MLSWIT); (iv) manual sowing with SWI-management using non-treated seeds (MLSWINT); (v) recommended planting with SWI-management using treated seeds (RPSWIT); (vi) recommended planting with No-SWI management using non-treated seeds (RPNoSWIT); (vii) check-row with SWI-management using treated (CRSWIT), and (viii) check-row with SWI-management using non-treated seeds (CRSWINT). The results showed that SWI-management increased the grain yield by 0.61–1.52 t/ha, with an overall average increase of 1.12 t/ha over control plots. The greatest increase (~25%) in grain yield was achieved with the mechanical seeder and seed treatment. At the same time, straw yield was lower by 0.09–0.66 t ha−1 under SWI-management, with one treatment exception. The increase in grain yield is attributable to SWI methods that led to an increase in the harvest index, i.e., in the wheat plant phenotypes. The water productivity and production efficiency were higher under SWI compared to conventional seed drilling with or without SWI-management. The gross returns using SWI planting ranged between Rs.123,526/ha in MLSWINT to Rs. 139,210/ha in MSWIT vs. Rs. 117,113/ha in RPNoSWIT. However, SWI with manual sowing (MLSWIT and MLSWINT) increased the cost of cultivation (COC) by Rs. 9,623/ha over RPNoSWIT. This increase in COC was lowered to Rs. 5,475/ha when planting was performed using SRMSWIP. Thus, owing to higher gross returns, SWI planting and management, barring MLSWINT, resulted in net returns of an increment of Rs. 7,615–16,372/ha over conventional planting without SWI-management. The B:C in MSWIT and MSWINT was significantly higher than RPSWI. The monetary efficiency (ME) was highest with MSWIT (Rs. 702/ha/day). Overall, the SRMSWIP may facilitate wheat sowing using SWI methods, with greater feasibility and economic viability achieved. The promotion of this low-cost SRMSWIP may be a boon in enhancing the adoptability of SWI-technology among wheat growers, particularly small and marginal farmers in India and adjoining regions.
1. Introduction
Wheat (Triticum aestivum L.) constitutes the staple food for over 35% of the world’s population and more than 50% of the people in India (Kushwaha et al., 2018), and thus, it holds the key to world food security. However, the input-dependent production of wheat and the high labor required has made its cultivation costlier due to escalating prices of labor, fossil fuels, energy, seeds, fertilizers, and other agro inputs; thus, becoming less profitable to farmers, with the agro inputs further causing soil, water, and air pollution. The negative ecological impacts of current cultivation methods and the fact that they are also becoming lesser remunerative led us to consider the adoption of more eco-friendly strategies that could reduce the environmental footprint without compromising crop yield. Efforts were already made in this direction by Fr. Henri de Laulanié, working with farmers in Madagascar for over 30 years to improve their output and efficiency of rice production. From this work came a set of practices and principles known as the System of Rice Intensification (SRI; Uphoff et al., 2011). Over the past decade and a half, the basic concepts of SRI have been extended first to rainfed rice, and then to improving the yields of a variety of other crops, starting with wheat and finger millet and then extrapolated to mustard, maize, teff, barley, legumes, sugarcane, and other crops (Uphoff, 2012). The adaptation of SRI principles and practices to raise the productivity of wheat crop has been given the name ‘System of Wheat Intensification (SWI).’ This involves modifying practices such as seed rate, sowing seeds at regular and optimum spacing, control of water in the main field, and inter-cultivation to control weeds and aerate the topsoil. These practices evoke more tillering from each plant and the growth of larger root systems, increasing the number of effective tillers/hill, enhancing spike length and producing bolder grains, with the cumulative result of enhancing the grain yield (Dhar et al., 2015).
Because SWI radically reduces plant population/m2, it requires only 25 kg of seed/ha, thereby saving ~75–100 kg of wheat seed/ha. Wider plant spacing (20–25 cm) between and within rows, the use of more organic manures to improve soil quality and functioning, and the treatment of seeds with organic biofertilizers all contribute to a higher yield. The wider spacing of plants and optimized plant density create conditions that enhance aeration and sunlight availability within the crop canopy and enhance moisture and nutrient availability in the soil. These practices facilitate vigorous growth and proper development of plant root systems at the initial stage of crop growth (Khadka and Raut, 2012; Dass et al., 2016, 2017). Growth and yield attributes as well as root traits were observed to be higher in SWI compared to conventional planting (Dhar et al., 2015; Kumar et al., 2015; Reddy et al., 2021). Moreover, the selection and use of healthy seeds, treated before sowing with a nutrient- and beneficial-microbes-rich mixture of cow urine, vermicompost, and jaggery (raw sugar) enhances the physiological and agronomic potential of SWI plants, leading to greater growth and yield. Dhar et al. (2015) reported that using SWI technology can greatly improve wheat productivity. Under the semi-arid conditions of India, SWI significantly outperformed standard methods of wheat cultivation, demonstrating ~46% yield advantage in a climatically stressful season, with improved nutrient uptake by the plants and maintained or improved soil fertility (Dhar et al., 2015). Moreover, SRI, SWI, and other crop intensification methods impart crop resilience to resist the hazards of climate change (Thakur et al., 2009; Zhao et al., 2009; Dass et al., 2017). Due to potential yield gains from SWI technology in terms of higher grain production per drop of water, per unit of land, and per unit of external input applied, the SWI technology has reached several states of India quickly, including Bihar, Chhattisgarh, Madhya Pradesh, Odisha, Punjab, and Uttar Pradesh (Rana et al., 2017); however, its rate of adoption is still very slow. SWI is not finding favor with farmers mainly because the planting of two seeds in each hill at a specified plant spacing (usually 20 × 20 cm is optimal) is a tedious, time-consuming, and costly operation.
However, SWI principles can be applied effectively by the mechanization of important operations (Sharif, 2011). Moreover, it has been observed that once farmers become more comfortable and skilled with the new methods, SWI can be a labor-saving technology with infrastructural support, particularly farm mechanization (Satyanarayana et al., 2007; Sharif, 2011). The requirement of human labor for planting can be reduced by devising and using suitable seed drills and cono-weeders instead of tedious line sowing and manual weeding, respectively (Sharif, 2011). Thus, the research team of the Division of Agricultural Engineering and the Division of Agronomy at ICAR-IARI, New Delhi, India developed a single-row manual SWI-planter (SRMSWIP) to make SWI-planting easier, faster, and more economical. Before taking the newly designed and developed machines to the end-users, they should be field validated to ascertain the performance in field conditions. The present study was thus conducted in the field to evaluate the performance of SRMSWIP and to compare the performance of SRMSWIP-sown wheat with conventionally sown and SWI wheat manually sown in a semi-arid climate.
2. Materials and methods
2.1. Single-row manual SWI-planter
A view of the SRMSWIP developed by the scientists in the Division of Agricultural Engineering and the Division of Agronomy, ICAR-IARI, New Delhi is shown in Plate 1. In this SRMSWIP, a metering system for wheat sowing using SWI methods was designed and fabricated by employing a new 3D printing concept. The basic components of the CAD design used are shown in Plate 2. The metering unit of the planter was evaluated on a testing setup equipped with a sticky belt at different speeds to assess its distribution uniformity in terms of delivering the desired number of seeds/hills. It was observed that the metering unit delivered 2, 3, and 1 seed(s)/hill for 56%, 20%, and 20% hills, respectively. A total of 4%–5% of hills did not receive any seed, thus requiring gap-filling. During the forward movement of the SRMSWIP, the ground wheels begin to rotate, which drives the seed-metering plate of the metering unit, which, in turn, delivers seeds through a 3D-printed cell-type cup to the final delivery unit. The specially designed curved delivery unit and metering roller are set and fixed in such a way that only 2–3 seeds settle into specifically designed seed cells at the time at which the ground wheel is almost one-eighth of the way through its full rotation. The seeds roll from the seed cell into the tube and are delivered to the bottom of the furrow at a predetermined depth. As soon as the furrow opener leaves the position due to forward motion, the seed-firming device closes the furrow and presses the seeds into the furrowed soil. Overall, germination of seeds and plant growth were significantly better in machine-sown plots compared to conventional or manually sown SWI; the germination of the seeds was found to be 22–24 per m2 and the distribution of plants per hill was observed to be 69% double, 23% single, 5% triple, and 3% nil. Details on the design, fabrication, and laboratory evaluation of the single-row manual SWI-planter have been presented in the publication by Kushwaha et al. (2018). The machine can be operated by using a human draft or a single bullock (Plate 3).
2.2. Climate during the experiment
The climate during the experimental crop seasons was variable. The daily maximum temperature ranged from 13.9°C (21 January 2016) to 40.6°C (16 April 2016, harvest date). The maximum temperature remained between 14°C and 25°C during the vegetative stage to ear emergence (mid-December 2015 to 3rd week of February 2016). The minimum temperature for the corresponding period was between 2.2°C and 15.5°C, except for the lowest temperatures of 0.4°C and 0.7°C recorded on 22 January 2016 and 23 January 2016, respectively. The minimum temperature rose to 23 ᴼC during maturation of the crop. The daily mean temperature ranged from 8.2°C during the vegetative stage to 30.4°C during crop maturation (Figure 1). The total rainfall during the crop period was 19.8 mm, occurring on 3 rainy days during the 2nd and 3rd weeks of March 2016. The daily mean relative humidity ranged between 49% and 93%. The total number of bright sunshine hours experienced by the crop was 702 h.
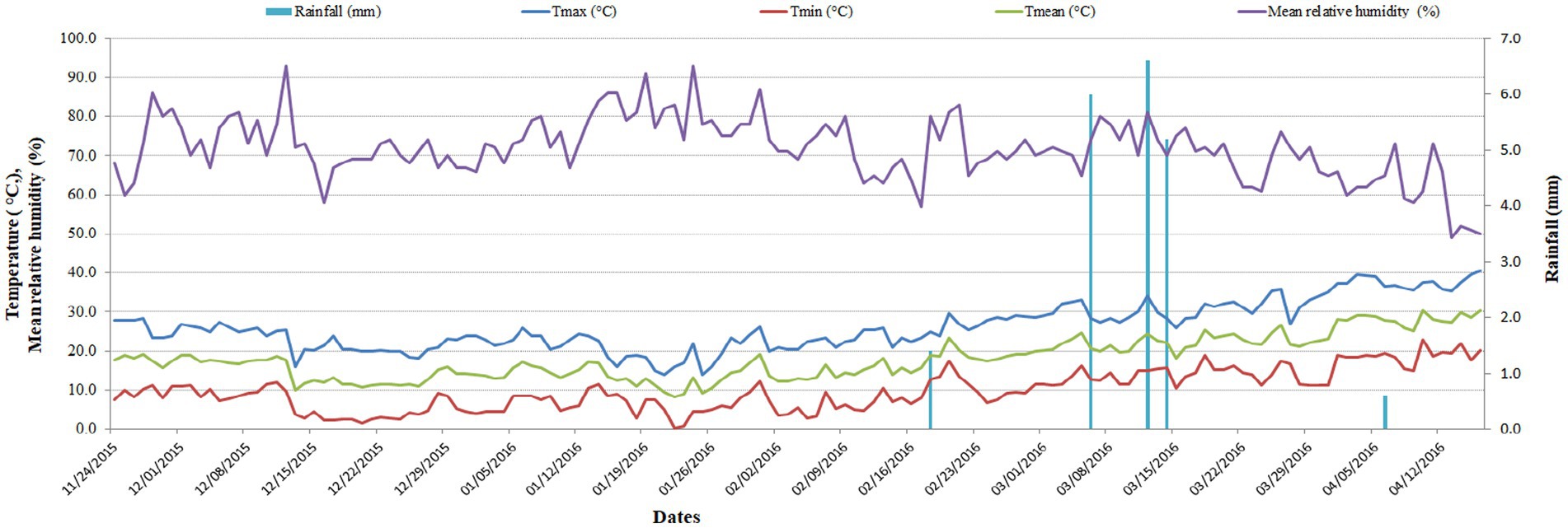
Figure 1. Maximum, minimum, and mean temperatures, relative humidity, and rainfall during the wheat crop period of 2015–2016 in the north-plain zone of India.
2.3. Treatment details and experimental design
Field evaluation of the SRMSWIP was performed by conducting a three-time replicated field experiment comprising eight treatments, as shown in Table 1. The experiment was conducted during the winter season of 2015–2016, following a randomized complete block design at the research farm of ICAR-Indian Agricultural Research Institute, New Delhi (28° 40′ N,77° 12′ E, and 228.6 m above mean sea level). There was a total of 24 experimental plots (dimensions: 8 × 10 m each). The soil of the experimental field was sandy loam with medium organic carbon (0.58%; Walkley and Black, 1934), low available N (232 kg/ha; Subbaiah and Asija, 1956), and medium available phosphorus (18.9 kg/ha; Olsen et al., 1954), and potassium (268 kg/ha; Black, 1971) contents and a pH of 7.3 (Rana et al., 2014).
2.4. Seed treatment and sowing protocols, and crop management
One day before seed sowing, the seeds were treated as per the SWI-management. Firstly, wheat seeds (var. HD-2967) were placed in a 20% saltwater solution; seeds that floated on the surface of the water were removed and the healthy and bold seeds that settled at the bottom of the container were retained. For seed treatment, a mixture of 10 L warm water (60°C), 2 kg of well-decomposed vermicompost, 3 L cow urine, and 2 kg jaggery (unrefined sugar) was prepared in an earthen pot. After mixing, 5 kg of seeds were dipped into the mixture in successive lots and left for 8 h. The seeds were then recovered from the mixture by filtration and washed with clean water. Treated seeds were stored in shade for 12 h, which enabled the seeds to sprout. Plates 4, 5 provide a view of the experimental crop at different growth stages. Before sowing, the soil was thoroughly prepared to a fine friable tilth by performing three tillage operations; first with a disc plough, followed by two secondary tillage operations using a cultivator and planking after each ploughing. Soil preparation was common for both SWI and conventional planting. For check-row planting, all plants from 20 cm row lengths were manually removed at intervals of 20 cm (row length with all plants) 20 days after sowing (DAS) to obtain crop plants in 20 cm row lengths alternated with 20 cm blank spaces. For recommended sowing, the crop was planted at a row spacing of 22.5 cm, while for SWI planting, a row-to-row and hill-to-hill spacing of 20 cm was maintained. Two seeds (sprouted seeds) were planted at each hill in SWI, and gaps were filled within 15 DAS (Table 1). Wheat was sown on 24 November 2015 and harvested on 16 April 2016.
Nutrient management under the SWI protocol included fertilization with Trichoderma-treated (2.5 kg/t) compost at 2.0 t/ha + 68 kg di-ammonium phosphate (DAP) and 33 kg/ha muriate of potash (MOP) before sowing; 68 kg urea/ha on the 16th day; vermicompost at 500 kg/ha + PSB culture at 6.25 kg/ha on the 20th day; 34 kg urea/ha and 34 kg MOP/ha on the 36th day; and vermicompost at 500 kg/ha on the 40th day. Under conventional management (non-SWI), the crop was fertilized with 150 kg N, 60 kg P2O5, and 40 kg K2O/ha. N was sourced from urea and DAP, P from DAP, and K from MOP. A whole amount of P and K and a one-third quantity of N were incorporated in the soil just before sowing. The remaining N was applied in two equal splits; the first split at first irrigation and the second at second irrigation.
Under SWI management, seven irrigations were applied, including one pre-sowing irrigation; the first irrigation to the crop was applied at 10 days after sowing (DAS) and the remaining five irrigations were applied at 20-day intervals. The number of irrigations for conventional planting and conventional management was six, including one pre-sowing irrigation. The first irrigation to the crop was applied at crown root initiation (21 DAS) and the remaining four irrigations were applied at 20–25-day intervals (corresponding to critical stages). Each irrigation had a uniform depth of 5.5 cm and the method of irrigation was check basin (surface method). Weeds were managed by three weedings using a cono-weeder at 20, 30, and 40 DAS under SWI management and by post-emergence application of sulfosulfuron at 25 g/ha in 750 L of water and one hand weeding under the conventional method of crop management.
2.5. Growth observations
To determine the growth characteristics of wheat, all plants in 1 m row lengths from the sampling rows in each plot were cut at the ground level using a sharp-edged knife, placed in polythene bags, and brought to the laboratory immediately in a fresh condition (Rana et al., 2014). All the leaves were detached from the shoots and the leaf area was determined using a leaf area meter (Model LI-COR-3100). The leaf area index was computed as the total leaf area of all plants/total ground area occupied by the plants. All tillers/shoots were counted simultaneously while detaching the leaves from the stems. For determining dry matter accumulation (DMA), all leaves and shoots were dried in a hot-air oven at 70°C ± 2°C until constant weights were obtained. Plant height was recorded by measuring the height of five representative plants from each plot (Rana et al., 2014). Photosynthetically active radiation (PAR) was measured using an AccuPAR LP-80 ceptometer. The intercepted PAR was computed by deducting the transmitted PAR from the incident PAR. The matured crop was harvested plot-wise and dried in the sun for 4 days. After sun drying, the biological yield (grain + straw) was recorded and converted to t/ha. The crop was threshed using a Pullman thresher, and the weight of grains was recorded and converted to t/ha. The grain yield was deducted from the biological yield to obtain the straw yield (Rana et al., 2014).
2.6. Input use-efficiencies
The water productivity (WP) was calculated by dividing the grain yield by the total water received by the crop (irrigation + effective rainfall). The economics of the treatments was determined based on prevailing market prices of inputs and outputs. The production efficiency (PE) of each treatment (kg/ha/day) was computed using the following expression (Rana et al., 2014):
The monetary efficiency (ME) of each treatment (INR ha−1 day−1) was computed using the following expression (Rana et al., 2014):
2.7. Statistical analysis
The data were analyzed using analysis of variance (ANOVA) techniques, as applicable for randomized complete block design (Gomez and Gomez, 1984; Rana et al., 2014). The significance of treatment means was tested using an F-test at a 5% level of probability. The critical difference (p = 0.05) was determined to evaluate differences between treatment means.
3. Results
3.1. Growth parameters, chlorophyll content index
At the tillering stage (45 DAS), the plants were of significantly shorter height in the machine-planted and manually planted SWI treatments (MSWIT, MSWINT, MLSWIT, and MLSWINT) compared to the recommended practice of wheat sowing with and without SWI management (RPSWIT and RPNoSWINT; Table 2; Figure 2). Check-row planting with and without SWI management was intermediate between SWI and recommended planting. The same trend continued until the boot leaf stage (75 DAS). However, as the growth progressed, the gap in plant height between SWI and non-SWI treatments reduced. The differences between the treatments became non-significant at grain filling (105 DAS). The differences further narrowed down to 4–5 cm at maturity. As a result of shorter plant height and lower number of shoots/m2, the LAI and DMA were lower in SWI at 45 DAS, though at later stages (75 and 105 DAS), the differences were non-significant both for LAI and DMA. At maturity, DMA was highest in MSWIT, which was significantly higher than MLSWINT, RPNoSWINT, and CRSWINT.
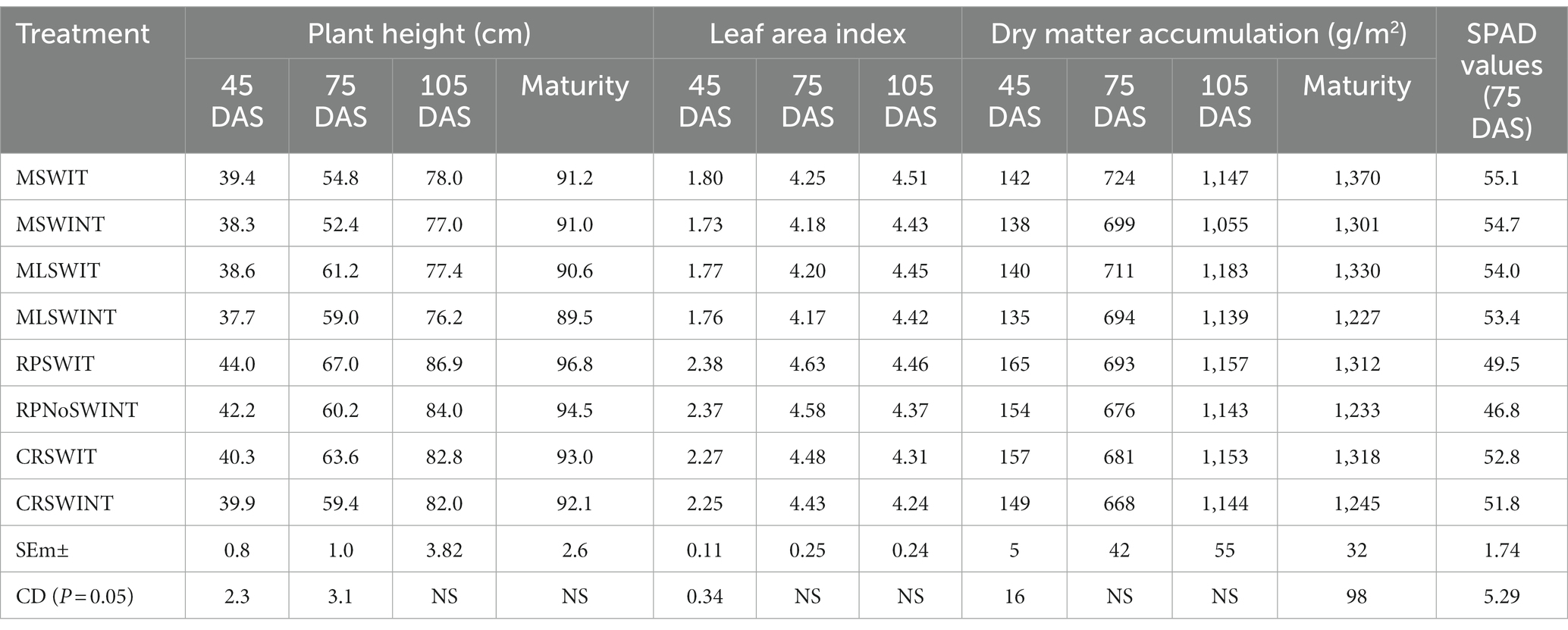
Table 2. Plant height, leaf area index, and dry matter accumulation of wheat under manual and machine-sown SWI and conventional planting.
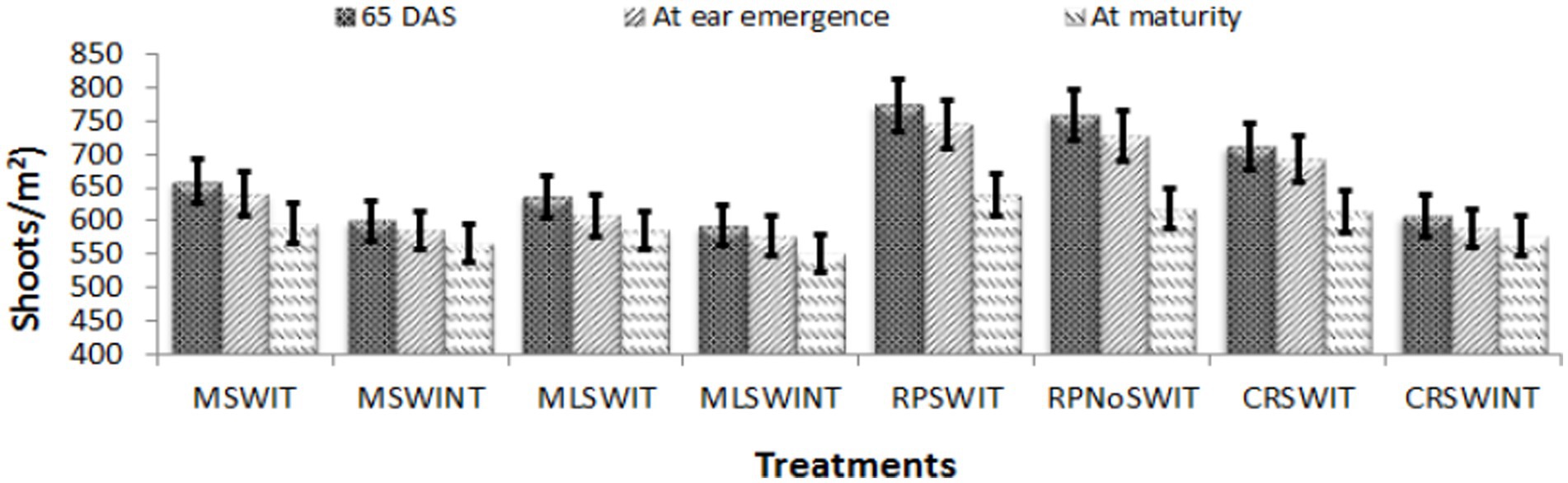
Figure 2. Growth stage-wise number of shoots/m2 of wheat under manual and machine-sown SWI and conventional planting (line above bars indicate critical difference values at p = 0.05).
3.2. PAR interception
Conventional planting with SWI management (RPSWIT) resulted in the highest interception of PAR, which was significantly higher than all other treatments, barring MSWIT and CRSWIT; the trend was reversed for the amount of transmitted PAR. The treatment recording the lowest intercepted PAR (MLSWINT) exhibited the highest amount of transmitted PAR. A similar trend was observed for the proportion of intercepted PAR. The PAR interception or transmittance depends on the leaf area of the crop, which in turn depends on plant height and tillers/m2. All of these plant parameters were higher in RPSWIT, closely followed by RPNoSWIT (Figure 3).
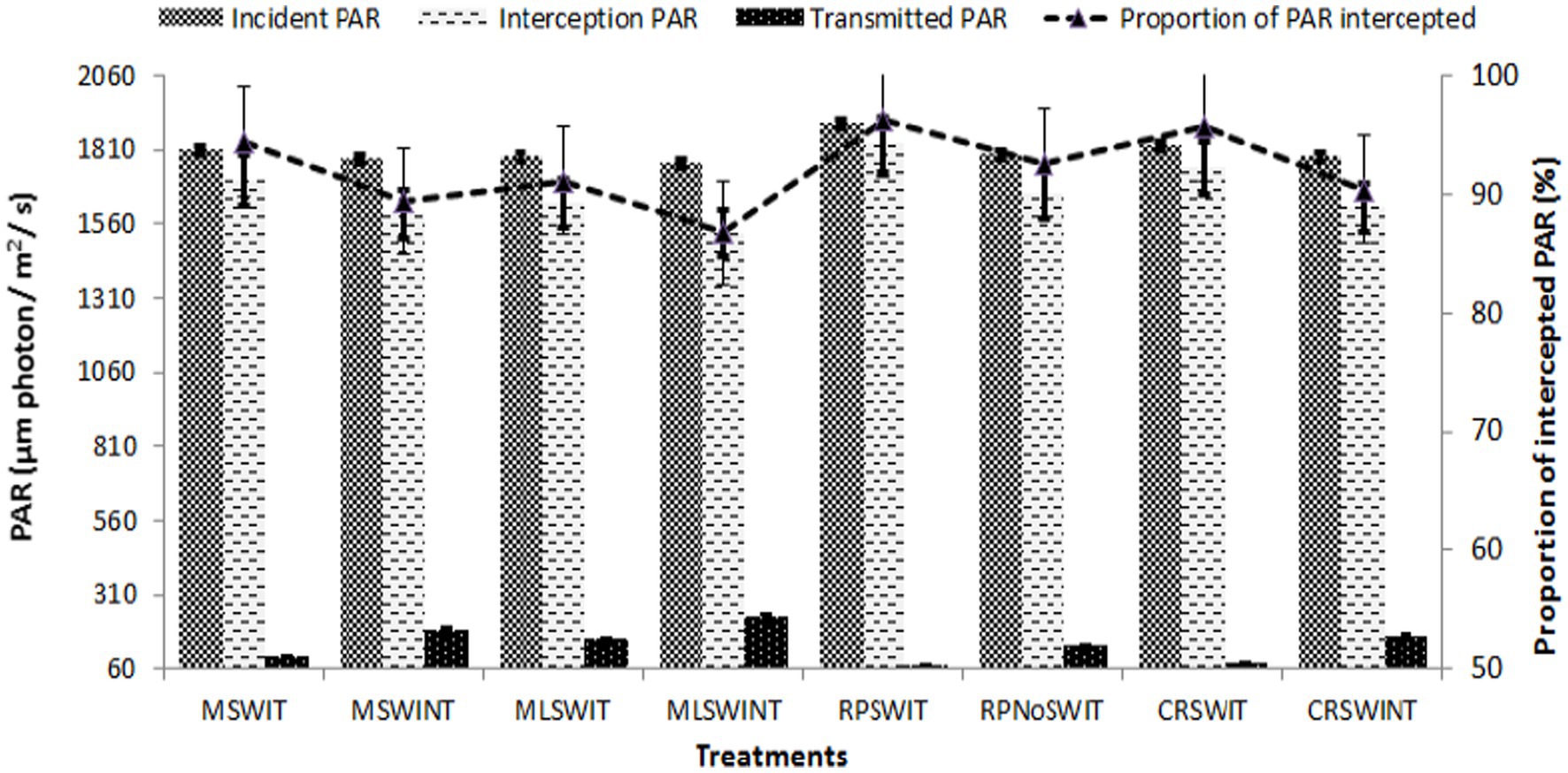
Figure 3. PAR interception characteristics of wheat under manual and machine-sown SWI and conventional planting (line above bars indicate critical difference values at p = 0.05).
3.3. Yield attributes
The results showed that the average number of spikelets per spike varied between 16.3 (RPNoSWINT) and 21.8 (MSWIT), with a mean standard error of 0.81 (Table 3). The average number of grains per spike ranged from 46.3 (RPNoSWINT) to 58.3 (MSWIT), with a standard error of 1.87. The 1,000-grain weight ranged from 41.2 g (RPNoSWINT) to 43.8 g (MSWIT), with a standard error of 1.27. The grain weight per spike varied from 1.54 g (RPNoSWINT) to 2.17 g (MSWIT), with a standard error of 0.1.
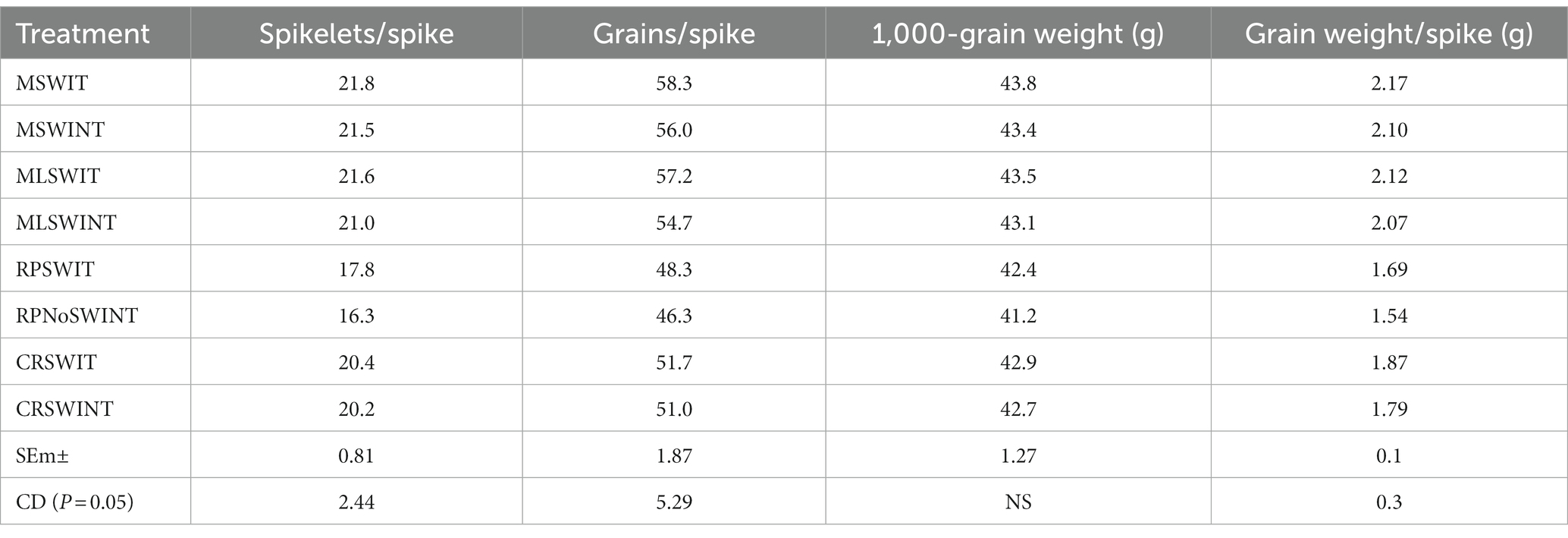
Table 3. Yield attributes of wheat as affected by manual and machine-sown SWI and conventional planting.
A critical difference (CD) test was conducted at a significance level of 0.05. The robust and stout wheat plant shoots in the SWI method produced larger spikes with a significantly greater number of spikelets and grains/spike compared to RPNoSWIT. All SWI treatments stood on par with one other both for spikelets and grains/spike. However, MSWIT showed a marginal edge over manual- or machine-sown SWI. CRSWIT and CRSWINT were also significantly better than RPNoSWIT. Although there was an improvement in the 1,000-grain weight due to SWI management over non-SWI treatments, the differences were non-significant. All SWI treatments (MSSWIT, MSWINT, MLSWIT, and MLSWINT) showed significant enhancements in grain weight/spike over RPSWIT and RPNoSWIT. Spikes in CRSSWIT were also significantly heavier than those in RPNoSWIT (Table 3). The results indicated that the differences in the number of spikelets/spike and grain weight/spike were significant among the treatments, whereas the differences in 1,000-grains weight were not significant. Overall, the results of this experiment showed that the different treatments had a significant impact on the spikelets/spike and grain weight/spike.
3.4. Grain and straw yields
SWI-management increased the grain yield by ~0.61–1.52 t/ha over RPNoSWIT, with the overall average increase being 1.12 t/ha (Figure 4). The greatest increase in wheat grain yield was with MSWIT (~25%), closely followed by MLSWIT and MSWINT. Even check-row planting with SWI-management increased the grain yield by ~0.63 t/ha. However, the straw yield dropped by ~0.09–0.66 t/ha under SWI-management, barring MSWIT, which recorded a similar straw yield to RPNoSWIT.
3.5. Water productivity and production efficiency
The water productivity was higher in SWI planting and management compared to conventional drilling of seeds with or without SWI-management; however, the difference was significant between MSSWIT and RPSWIT and between MSSWIT and CRNOSWIT only (Table 4). Treatments involving SWI planting and management did not differ significantly among one another, though machine-planted SWI exhibited a small edge over manually planted SWI mainly due to a higher grain yield. The production efficiency (PE) was significantly greater in MSWIT, MSWINT, and MLSWIT compared to RPNoSWIT due to higher grain yield. However, the former three treatments gave similar results. The remaining treatments involving SWI (MLSWINT) or check-row planting (CRSWIT, CRSWINT) did not vary significantly from conventional planting (RPNoSWIT, RPSWIT) in terms of PE.
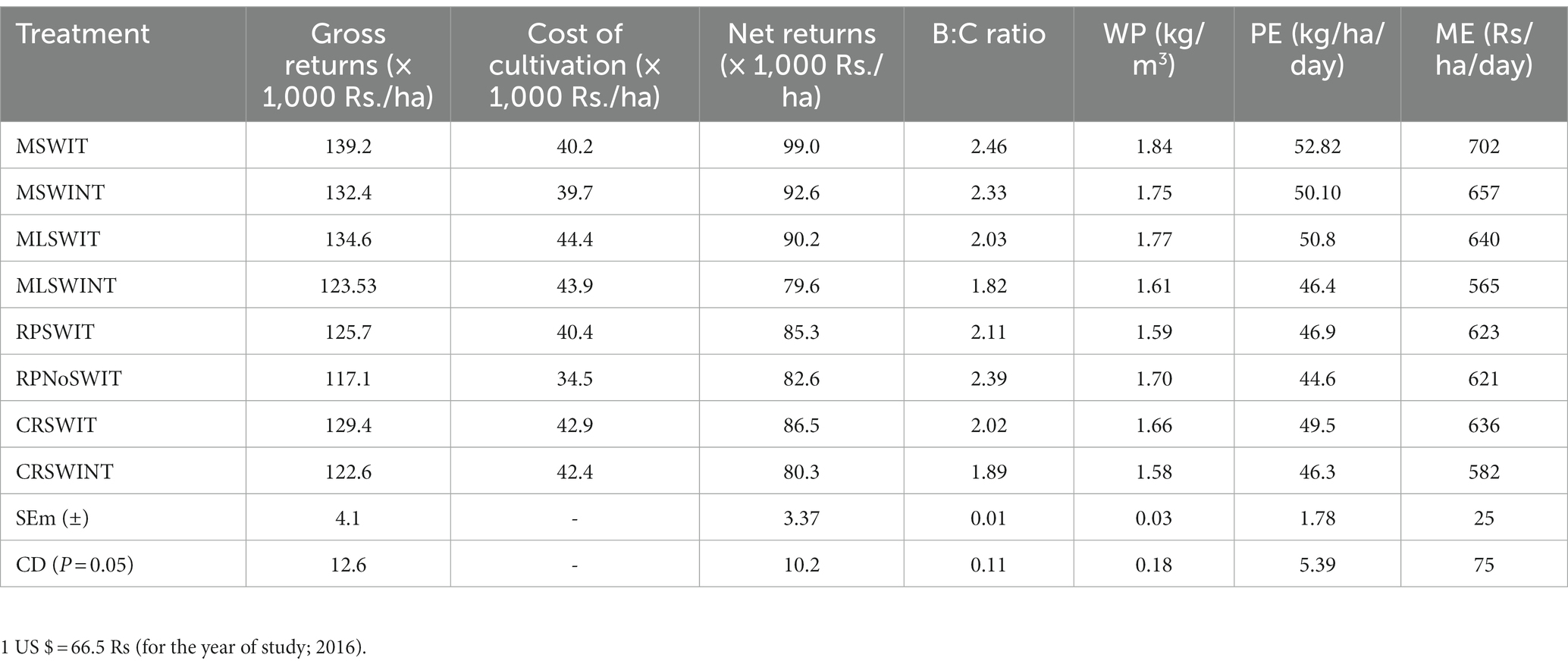
Table 4. Economics, water productivity (WP), production efficiency (PE), and monetary efficiency (ME) of wheat under manual and machine-sown SWI and conventional planting.
3.6. Economics and monetary efficiency
The gross returns under SWI planting ranged from Rs. 123,526 in MLSWINT to Rs. 139,210 in MSWIT (Table 4). Barring MLSWINT, all other SWI planting treatments showed a significant edge over RPNoSWIT (Rs. 117,113). Conventional planting with SWI-management (RPSWIT) also exhibited a more than Rs. 8,000/ha increase in gross returns over RPNoSWIT; however, the difference was statistically non-significant. Within SWI planting, MSWIT recorded significantly higher gross returns than MLSWINT. Check-row planting with SWI management did not differ significantly from RPNoSWIT. SWI-management with manual sowing (MLSWIT and MLSWINT) increased the cost of cultivation (COC) by Rs. 9,623/ha over conventional cultivation of wheat (RPNoSWIT). This increase in the COC was lowered to Rs. 5,475/ha when planting was performed using the single-row-SWI-planter. Check-row planting with SWI-management enhanced the COC by Rs. 8,109/ha. These results showed that the higher COC issue can be addressed by using the newly developed single-row-SWI-planter. Due to higher gross returns, SWI planting and management, barring MLSWINT, resulted in Rs. 7,615–16,372/ha higher net returns than conventional planting without SWI management, though the increase was significant for MSWIT only. MLSWINT exhibited a decrease of Rs. 2,960/ha in net returns compared to RPNoSWIT due to the higher COC. Moreover, CRSWINT exhibited reduced net returns by Rs. 2,338/ha over RPNoSWIT because the increase in yield and the resultant increase in gross returns did not commensurate with the increase in the COC. CRSWIT showed increased net returns by approximately Rs. 3,918/ha over RPNoSWIT. The B:C ratio in MSWIT and MSWINT was significantly higher than that in RPSWI due to higher net returns; however, the B:C ratio in RPNoSWIT was statistically similar to those in MSWIT and MSWINT. The ME followed the net returns trend, with MSWIT (Rs. 702/ha/day) recording the highest ME, followed by MSWINT (Rs. 657/ha/day) and MLSWIT (Rs. 640/ha/day). These three treatments were, however, statistically at par with each other but significantly superior to all other treatments.
4. Discussion
4.1. Growth parameters, chlorophyll content index
Under the SWI protocol, seeding was performed at a spacing interval of 20 × 20 cm and due to this, the plants were exposed to four times greater space to expand horizontally, producing a much larger number of tillers/hill, and thus, remained significantly shorter in height until the mid-growth period, compared to recommended conventional seeding at 22.5 × 4–5 cm spacing. However, greater competition for light among closely growing plants under RPSWIT and RPNoSWINT resulted in the upward growth of the plants, thus attaining more height. Evidence shows that herbaceous plants elongate the stem and grow taller to avoid shade under a dense plant stand and high leaf index (Smith, 1982; Weiner et al., 1990; Weiner and Thomas, 1992). In SWI planting, the number of planting hills/m2 having healthy plants was below 25 as compared to more than 110 plants/m2 in conventional planting. Thus, despite the much larger number of tillers or shoots/plant, the number of shoots/m2 was significantly lower in SWI planting. However, when the wheat seeds are sown wider apart under good soil conditions, the roots of the resultant plants have ample space to proliferate; therefore, they do not compete with one another, enabling the wheat plants to produce more healthy tillers along with a high volume of roots per hill due to greater access to both oxygen and water under aerobic conditions (Chopra and Sen, 2013). Thus, in our study, a wider square spacing, reinforced with a better rooting system, and with better nutrition from both organic and inorganic sources, allowed the soil microbiome to be invigorated, enhancing the nutrient bioavailability in the root-zone soil. On top of this, root aeration through hoeing and weeding, greater exposure of widely spaced plants to solar radiation and circulating air, and additional irrigation favored a higher net photosynthetic rate. This was continued for an additional period of more than 1 week, leading to the continuous growth of plants and resulting in a convergence of the differences between SWI and conventional sowing regarding plant height, leaf area index, and dry matter production, particularly at later crop growth stages (Thakur et al., 2010; Dass and Chandra, 2013; Dhar et al., 2015; Dass et al., 2017).
4.2. Photosynthetically active radiation
A higher chlorophyll content in the leaf, as reflected by larger SPAD values (Dwyer et al., 1991; Piekielek and Fox, 1992; Dass et al., 2014), was also found under SWI-management, resulting in better physiological processes and improved plant growth. This study found that the conventional planting method with SWI-management (RPSWIT) resulted in the highest interception of PAR. This means that the crop was able to capture more light energy from the sun, which is an important factor for plant growth and productivity. The treatment that recorded the lowest intercepted PAR (MLSWINT) exhibited the highest amount of transmitted PAR, which suggests that the crop was not effectively capturing and utilizing the available light energy. The trend observed in PAR interception and transmittance depends on the leaf area of the crop, which is influenced by the plant height and number of tillers/m2 (Miralles and Slafer, 1997; Reynolds et al., 2005; Gautam et al., 2019). In this study, RPSWIT had the highest plant parameters, followed closely by RPNoSWIT. These results indicate that the conventional planting method with SWI-management has the potential to produce crops with higher light interception efficiency, which can contribute to higher crop productivity.
4.3. Yield attributes and yield
The significant improvements in all yield attributes due to SWI-management is a reflection of the significantly positive effect of SWI-management on plant growth and development, which translated into yield-forming components. In northern India, yield enhancement of 35%–67% due to SWI methods has been reported (Adhikari et al., 2017). A robust, erect plant architecture facilitates better percolation of solar radiation and circulation of air in the plant’s micro-environment as well as larger root system thus resisting plant lodging in the widely spaced SWI-planting regime, resulting in a higher grain yield compared to non-SWI treatments (Dass and Dhar, 2014; Dass et al., 2015). Moreover, germination of seeds and the grain yield were significantly better in machine-sown plots compared to conventional or manually sown SWI/non-SWI plots. The final yield in machine-sown SWI (7.26 t/ha) was greater than that for recommended sowing with no-SWI management non-treated (RPNoSWI) planting of wheat by 22.3%. The increase in grain yield due to SWI mainly came from the significant improvement in the harvest index (approximately 45% in SWI and approximately 40% in RPNoSWI-sown wheat; Kushwaha et al., 2018). Partitioning of dry matter in favor of grains in SWI treatments is another reason for the higher grain yield under SWI since the total dry matter production at harvest was comparable for SWI and non-SWI treatments. The greater straw yield in non-SWI treatments is attributed to greater plant height and a larger number of tillers/m2. Biomass production depends on the ability of the crop to intercept PAR, which is a function of the leaf area index (Gautam et al., 2019); both were higher in non-SWI treatments; thus, larger straw yields were recorded.
4.4. Water productivity and production efficiency
The water productivity, defined as the ratio of crop output to water input, is a critical aspect of sustainable agriculture. The wheat crop in this study was irrigated seven times, including a pre-sowing irrigation in SWI management, and six times in conventional management. The depth of irrigation was maintained uniformly at 5.5 cm. The check basin (surface) method of irrigation was used in this experiment. All SWI treatments used 4,048m3 of water and conventional planting (non-SWI) used 3,498 m3, including both irrigation water and rainfall. Although the water use was higher in SWI management, it was associated higher yields. Thus, the use of SWI-management resulted in a significant improvement in the water productivity, mainly due to higher yields, which reflects its positive effect on plant growth and development (Dass et al., 2016; Kushwaha et al., 2018). The higher water productivity of wheat in SWI-planter–sown SWI crops, as compared to manual dibble–sown SWI crops, stemmed mainly from the greater yield under the former.
However, studies conducted by Ibrahim (2009) have shown that the use of SWI methods can reduce water use by ~25%–30% over normally sown wheat. However, this was not true in our study. The upright and robust plant behavior in the SWI planting system, with wider spacing, allows for better absorption of solar radiation, better circulation of air, and a larger root system, which helps resist lodging, leading to higher grain yield and promotes better water productivity and production efficiency as compared to non-SWI treatments (Choudhary and Suri, 2018; Choudhary et al., 2022). Some published reports suggested that the SWI method can increase the wheat yield by up to 13%–15%, with 25%–30% higher water productivity than non-SWI–sown wheat (Sunaratiya and Banik, 2022). The results showed that machine planting resulted in higher grain yields, improved crop growth and development, and a higher water productivity as compared to manual planting. The higher production efficiency of SWI-planter–sown and manual dibbling–sown SWI crops than conventionally sown SWI or conventionally sown non-SWI crops was a consequent of the higher grain yield in the former two treatments.
4.5. Economics and monetary efficiency
The results showed that the gross returns of all SWI planting treatments (MSWIT, MLSWIT, and CRSWIT) were higher than that of conventional planting without SWI-management (RPNoSWIT), which led to improved economy of the SWI-system (Choudhary and Suri, 2018; Choudhary et al., 2022). Among the SWI treatments, MSWIT recorded the highest gross returns, which were significantly higher than those of MLSWINT. The COC was higher for the manual planting treatments (MLSWIT and MLSWINT) compared to conventional planting; however, it was lower for SRMSWIP. Check-row planting with SWI-management (CRSWIT) increased the COC and reduced the net returns compared to conventional planting.
The major component costs of agronomic management that differed between SWI and non-SWI treatments included the cost of seed (Rs. 625/ha for SWI manual dibbling, Rs. 750/ha for SWI-planter, and Rs. 2,500/ha for conventional sowing), the cost of sowing (Rs. 7,280/ha, Rs. 3,120/ha, and Rs. 2,760/ha for SWI-manual dibbling, SWI-planter, and conventional sowing, respectively); the cost of fertilizers and manures/composts (SWI treatments: Rs. 9,666/ha; non-SWI treatment: Rs. 7,092/ha), weed control (SWI treatments: Rs. 6,240/ha; non-SWI treatment: Rs. 3,430/ha), and irrigation (SWI treatments: Rs. 7,240/ha; non-SWI treatment Rs. 5,780/ha). There was an approximately four times reduction in the cost of seed; however, the cost of planting was 2.6 times higher for SWI-manual dibbling compared to conventional drilling of seeds using a tractor-drawn seed drill. However, the use of the SWI-planter drastically reduced the cost of SWI plating; the difference between conventional drilling of seed and SWI-planter sowing was reduced to Rs. 360/ha. Moreover, SWI management increased the expenses spent on fertilizer and manure/compost, weed control, and irrigation by 36%, 81.9%, and 25.3%, respectively, over conventional sowing, which in turn increased the overall cost of cultivation for SWI. However, the use of the SWI-planter reduced the cost of sowing using SWI by 57.1% compared to SWI manual dibbling.
The results indicated that the use of the SRMSWIP can address the issue of higher COC to a considerable extent, leading to higher net returns (Kushwaha et al., 2018). The SWI treatments, except for MLSWINT, resulted in higher net returns than conventional planting, with MSWIT exhibiting the highest net returns. The benefit–cost ratio (B:C) was highest in MSWIT and MSWINT, reflecting the higher net returns. Earlier studies, although they did not use machines, have also demonstrated significantly and convincingly higher net returns and B:C using SWI methods compared to conventional planting of wheat both under adequate and limited water availability conditions (Chopra and Sen, 2013; Rana et al., 2017).
5. Conclusion
A single-row manual system of wheat intensification planter has been developed by scientists at the ICAR-Indian Agricultural Research Institute, New Delhi to make the labor- and resource-intensive process of manual planting in SWI easier, faster, and more economical. The SRMSWIP was field tested on a winter wheat crop during 2015–2016, which showed that SWI-management significantly increased the grain yield over conventional wheat cultivation. Within SWI treatments, SRMSWIP–sown wheat produced an approximately 1 t/ha higher yield over manual dibbling. Consequently, the water productivity, production efficiency, and net returns were also higher in SRMSWIP–sown wheat compared to conventional planting and manual dibbling in SWI. The cost of sowing was also reduced by Rs. 4,160/ha when the SWI-planter was used. The SRMSWIP is, therefore, a useful tool for making SWI planting more feasible and economical for wheat growers. Overall, the findings of the study suggest that SWI planting treatments may lead to improved gross and net returns and monetary efficiency in wheat, particularly when using SRMSWIP. The study provides valuable insights for farmers and policymakers in developing sustainable and economically viable farm tools for crop production. Overall, this low-cost SRMSWIP may be a boon in enhancing the adoptability of SWI-technology and wheat productivity among wheat growers, particularly small and marginal farmers in south-Asia.
Data availability statement
The original contributions presented in the study are included in the article/supplementary material, further inquiries can be directed to the corresponding authors.
Author contributions
AD: conceptualization of SWI research, designing specifications of single-row SWI planter, field experiments, data collection, analyses, review of literature, and writing and editing of manuscript. HK: conceptualization, design and fabrication of single-row SWI planter, and field experiments. PS: conceptualization of single-row SWI planter and supervision of experiment. SD: conceptualization of SWI technology and data analyses. AC: field experiments, data collection, and editing of manuscript. TK: design and fabrication of single-row SWI planter and editing of manuscript. SB: review of literature and polishing of manuscript. AS: data analyses, review of literature, and editing of manuscript. IM: conceptualization, design and fabrication of single-row SWI planter. MK: review of literature and preparation of manuscript. RK: field evaluation of SWI planter and data collection. DY: review of literature and editing of manuscript. All authors contributed to the article and approved the submitted version.
Funding
This research was funded by ICAR-Indian Agricultural Research Institute, New Delhi under its in-house research program.
Conflict of interest
The authors declare that the research was conducted in the absence of any commercial or financial relationships that could be construed as a potential conflict of interest.
Publisher’s note
All claims expressed in this article are solely those of the authors and do not necessarily represent those of their affiliated organizations, or those of the publisher, the editors and the reviewers. Any product that may be evaluated in this article, or claim that may be made by its manufacturer, is not guaranteed or endorsed by the publisher.
References
Adhikari, P., Araya, H., Aruna, G., Balamatti, A., Banerjee, S., Baskaran, P., et al. (2017). System of crop intensification for more productive, resource-conserving, climate-resilient, and sustainable agriculture: experience with diverse crops in varying agro-ecologies. Int. J. Agric. Sust. 16, 1–28. doi: 10.1080/14735903.2017.1402504
Chopra, R., and Sen, D. (2013). Golden wheat becomes more Golden—extending SRI to wheat. LEISA India 15, 30–32.
Choudhary, A. K., Sood, P., Rahi, S., Yadav, D. S., Thakur, O. C., Siranta, K. R., et al. (2022). Rice productivity, Zn-biofortification and nutrient-use efficiency as influenced by Zn-fertilization under conventional transplanted-rice and the system of rice intensification. Front. Environ. Sci. 10:869194. doi: 10.3389/fenvs.2022.869194
Choudhary, A. K., and Suri, V. K. (2018). System of rice intensification in short duration rice hybrids under varying bio-physical regimes: new opportunities to enhance rice productivity and rural livelihoods in NW Himalayas under a participatory-mode technology transfer program. J. Plant Nutr. 41, 2581–2605. doi: 10.1080/01904167.2018.1510515
Dass, A., and Chandra, S. (2013). Irrigation, spacing and cultivar effects on net photosynthetic rate, dry matter partitioning and productivity of rice under SRI in Mollisols of northern India. Exp. Agric. 49, 504–523. doi: 10.1017/S0014479713000252
Dass, A., Chandra, S., Choudhary, A. K., Singh, G., and Sudhishri, S. (2016). Influence of field re-ponding pattern and plant spacing on rice root-shoot characteristics, yield, and water productivity of two modern cultivars under SRI management in Indian Mollisols. Paddy Water Environ. 14, 45–59. doi: 10.1007/s10333-015-0477-z
Dass, A., Chandra, S., Uphoff, N., Choudhary, A. K., Bhattacharyya, R., and Rana, K. S. (2017). Agronomic fortification of rice grains with secondary and micronutrients under differing crop management and soil moisture regimes in the north Indian Plains. Paddy Water Environ. 15, 745–760. doi: 10.1007/s10333-017-0588-9
Dass, A., and Dhar, S. (2014). Irrigation management for improving productivity nutrient uptake and water-use efficiency in SRI: a review. Ann. Agric. Res. 35, 107–122.
Dass, A., Kaur, R., Choudhary, A. K., Pooniya, V., Rana, D. S., Raj, R., et al. (2015). System of rice (Oryza sativa L.) intensification for higher productivity and resource-use efficiency–a review. Indian. J. Agron. 60, 1–19.
Dass, A., Vyas, A. K., and Kaur, R. (2014). Nitrogen management in precision farming by soil-plant analyses development meter. Indian Farming 63, 33–35.
Dhar, S., Barah, B. C., Vyas, A. K., and Uphoff, N. T. (2015). Comparing SWI with standard recommended practices in the north-western plain zone of India. Arch. Agron. Soil Sci. 62, 994–1006. doi: 10.1080/03650340.2015.1101518
Dwyer, L. M., Tollenaar, M., and Houwing, L. (1991). A nondestructive method to monitor leaf greenness in corn. Can. J. Plant Sci. 71, 505–509. doi: 10.4141/cjps91-070
Gautam, P., Lal, B., Nayak, A. K., Raja, R., Panda, B. B., Tripathi, R., et al. (2019). Inter-relationship between intercepted radiation and rice yield influenced by transplanting time, method, and variety. Int. J. Biometeorol. 63, 337–349. doi: 10.1007/s00484-018-01667-w
Gomez, K. A., and Gomez, A. A. (1984). Statistical procedures for agricultural research. New York: John Wiley & Sons.
Ibrahim, H. (2009). The system of wheat intensification (SWI) initial test by farmers in Goundam and dire, Timbuktu, Mali, Africa. Africare, Mali, USAID. Available at: http://sri.ciifad.cornell.edu/countries/mali/Mali_SWI_ Africare09.pdf (Accessed 8 February 2023).
Khadka, R. B., and Raut, P. (2012). System of wheat intensification (SWI): A new concept on low-input technology for increasing wheat yield in marginal land. Kathmandu. In: Paper for European Union Food Facility Project & Forum for Awareness and Youth Activity, Nepal.
Kumar, A., Raj, R., Dhar, S., and Pandey, U. C. (2015). Performance of system of wheat intensification (SWI) and conventional wheat sowing under north eastern plain zone of India. Ann. Agric. Res. New Series 36, 258–262.
Kushwaha, H. L., Dass, A., Khura, T. P., Sahoo, P. K., Singh, M. K., and Mani, I. (2018). Design, development and performance evaluation of manual planter for system of wheat intensification. Indian J. Agric. Sci. 89, 678–687. doi: 10.56093/ijas.v89i4.88867
Miralles, D. J., and Slafer, G. A. (1997). Radiation interception and radiation use efficiency of near-isogenic wheat lines with different height. Euphytica 97, 201–208. doi: 10.1023/A:1003061706059
Olsen, S. R., Cole, C. V., Watanabe, F. S., and Dean, L. A. (1954). Estimation of available phosphorus in soils by extraction with sodium bicarbonate. USDA Circul 939:19.
Piekielek, W., and Fox, R. H. (1992). Use of a chlorophyll meter to predict side dress nitrogen requirements for maize. Agron. J. 84, 59–65. doi: 10.2134/agronj1992.00021962008400010013x
Rana, L., Banerjee, H., Ray, K., and Sarkar, S. (2017). System of wheat intensification (SWI) – a new approach for increasing wheat yield in small holder farming system. J. Appl. Nat. Sci. 9, 1453–1464. doi: 10.31018/jans.v9i3.1384
Rana, K. S., Choudhary, A. K., Sepat, S., Bana, R. S., and Dass, A. (2014). Methodological and analytical agronomy. IARI, New Delhi, India.
Reddy, J. R., Singh, R., Singh, R. K., and Singh, E. (2021). Performance of wheat (Triticum aestivum L.) cultivars under system of wheat intensification and conventional methods of sowing. Int. J. Curr. Microbiol. App. Sci. 10, 3389–3394. doi: 10.20546/ijcmas.2021.1002.373
Reynolds, M. P., Pellegrineschi, A., and Skovmand, B. (2005). Sink-limitation to yield and biomass: a summary of some investigations in spring wheat. Ann. Appl. Biol. 146, 39–49. doi: 10.1111/j.1744-7348.2005.03100.x
Satyanarayana, A., Thiyagarajan, T. M., and Uphoff, N. (2007). Opportunities for water saving with higher yield from the SRI. Irr. Sci. 25, 99–115. doi: 10.1007/s00271-006-0038-8
Sharif, A. (2011). Technical adaptations for mechanized SRI production to achieve water saving and increased profitability in Punjab, Pakistan. Paddy Water Environ. 9, 111–119. doi: 10.1007/s10333-010-0223-5
Smith, H. (1982). Light quality, photoperception, and plant strategy. Annu. Rev. Plant Physiol. 33, 481–518. doi: 10.1146/annurev.pp.33.060182.002405
Subbaiah, B. V., and Asija, G. L. (1956). A rapid procedure for the determination of available-N in soil. Curr. Sci. 25, 259–262.
Sunaratiya, A., and Banik, R. (2022). System of wheat intensification: a novel approach. Agri. Food: E-Newsletter 4, 297–300. doi: 10.5281/zenodo.7803544
Thakur, A. K., Choudhari, S. K., Singh, R., and Kumar, A. (2009). Performance of rice varieties at different spacing grown by the SRI in eastern India. Indian J. Agric. Sci. 79, 443–447.
Thakur, A. K., Rath, S., Roychowdhury, S., and Uphoff, N. (2010). Comparative performance of rice with SRI and conventional management using different plant spacings. J. Agron. Crop Sci. 196, 146–159. doi: 10.1111/j.1439-037X.2009.00406.x
Uphoff, N. (2012). Raising smallholder food crop yields with climate-smart Agroecological practices. Booklet prepared for World Bank Institute seminar. Available at: http://sri.ciifad.cornell.edu/aboutsri/othercrops/Other_Crops_Brochure_Uphoff101012.pdf (Accessed 10 October 2012).
Uphoff, N., Kassam, A., and Harwood, R. (2011). SRI as a methodology for raising crop and water productivity: productive adaptations in rice agronomy and irrigation water management. Paddy Water Environ. 9, 3–11. doi: 10.1007/s10333-010-0224-4
Walkley, A., and Black, I. A. (1934). An examination of Degtjareff method for determining soil organic matter and a proposed modification of the chromic acid titration method. Soil Sci. 37, 29–38. doi: 10.1097/00010694-193401000-00003
Weiner, J., Berntson, G. M., and Thomas, S. C. (1990). Competition and growth form in a woodland annual. J. Ecol. 78, 459–469. doi: 10.2307/2261124
Weiner, J., and Thomas, S. C. (1992). Competition and allometry in three species of annual plants. Ecology 73, 648–656. doi: 10.2307/1940771
Keywords: SWI planter, yield, water productivity, monetary-efficiency, net returns, SWI-technology, wheat
Citation: Dass A, Kushwaha HL, Sahoo PK, Dhar S, Choudhary AK, Khura TK, Babu S, Singh A, Mani I, Kumar M, Kumar R and Yadav D (2023) Comparative analysis of machine-planted and manual-planted wheat on crop and water productivity, and profitability under system of wheat intensification management. Front. Sustain. Food Syst. 7:1187647. doi: 10.3389/fsufs.2023.1187647
Edited by:
Pankaj Kumar Arora, Babasaheb Bhimrao Ambedkar University, IndiaReviewed by:
Jiban Shrestha, Nepal Agricultural Research Council, NepalAmit Anil Shahane, Central Agricultural University, India
Copyright © 2023 Dass, Kushwaha, Sahoo, Dhar, Choudhary, Khura, Babu, Singh, Mani, Kumar, Kumar and Yadav. This is an open-access article distributed under the terms of the Creative Commons Attribution License (CC BY). The use, distribution or reproduction in other forums is permitted, provided the original author(s) and the copyright owner(s) are credited and that the original publication in this journal is cited, in accordance with accepted academic practice. No use, distribution or reproduction is permitted which does not comply with these terms.
*Correspondence: Anchal Dass, anchal_d@rediffmail.com; H. L. Kushwaha, hlkushwaha@gmail.com