- 1Perdue Agribusiness, Salisbury, MD, United States
- 2Department of Animal Sciences, University of Florida, Gainesville, FL, United States
- 3Department of Animal Sciences, University of São Paulo, Piracicaba, Brazil
Dairy cows convert low-quality feedstuffs unsuitable for human consumption into milk, a high-quality protein source for humans. Even under grazing conditions of well-managed pastures, dietary energy limits the efficiency of animal production, contributing to the excretion of nutrients to the environment. Thus, our study aimed to assess the effect of supplying sources and levels of supplemental fat to grazing dairy cows during early lactation on nutrient excretion and recovery of human-edible (HE) nutrients in milk throughout the lactation cycle. Two experiments were conducted with grazing Jersey × Holstein dairy cows. In Experiment 1, five dairy cows were used in a 5 × 5 Latin Square design. Treatments were a control diet (concentrate without supplemental fat) or diets supplemented with calcium salts of either soybean fatty acids (CSSO) or palm fatty acids (CSPO) fed at two levels of supplementation (400 g/day or 700 g/day) for 24 days. In general, the level of supplemental fat had a minor effect on production parameters and methane emission and intensity. Feeding cows with CSPO increased milk yield by 8.1%, milk energy output by 20%, and milk fat yield by 35.2% compared with CSSO. A tendency was observed for CSPO to decrease methane per unit of milk energy compared with CSSO. In experiment 2, 24 dairy cows were used in a randomized block design. Treatments were a control diet or diets supplemented with calcium salts of either CSSO or CSPO fed at 400 g/day for 13 weeks. The carryover effect of the diets was evaluated until 42 weeks of lactation. During the supplementation period, feeding CSPO improved the recovery of HE energy, protein, and indispensable amino acids compared with control and CSSO. Interestingly, we demonstrated that feeding CSPO improved the recovery of HE energy, protein, and indispensable amino acids compared with control over the lactation cycle. Overall, our results suggested that the dose of supplemental fat used in our study had a minor influence on production and rumen fermentation parameters. We demonstrated that feeding dairy cows with a fatty acid source based on palm oil under tropical grazing conditions reduced nutrient excretion and improved the recovery of HE nutrients throughout the lactation cycle.
1. Introduction
Increasing the production of safe and nutritious food is a growing challenge to satisfy the demands of a global population projected to reach close to 10 billion by 2050. The solution is more complex than enhancing the cultivated area and the number of animals, given the increasingly limited land and resources available and concerns over the environmental impact. Instead, efforts should be directed at enhancing the production efficiency per unit of nutrients and decreasing the environmental footprint of the production systems. An increase in calorie availability to humans by almost 50% could be achieved by changing crop production away from activities, such as bioenergy, livestock feed, and other non-food applications (Foley et al., 2011). Nonetheless, the global concerns related to food security extend beyond caloric needs as the increase in energy consumption from refined grains has been negatively correlated with health outcomes (Vega Mejía et al., 2018). Additionally, in several developing nations, protein quality is a fundamental issue due to the lower digestibility and amino acid profile of grain-based diets compared with animal-based products (Ritchie et al., 2018). In this regard, dairy cows are able to transform feeds unsuitable for human consumption into milk, which is a high-quality protein-rich product, and also provides energy, essential fatty acids, vitamins, and minerals (Górska-Warsewicz et al., 2019).
In tropical areas, dairy milk production relies on the use of grazing systems because forage is an affordable source of nutrients in the diet. A higher potential for forage mass accumulation is observed in tropical forages (Santos et al., 2014), and the development of management strategies based on light interception improved the forages' nutritional value and milk production (Voltolini et al., 2010; Da Silva et al., 2013). Furthermore, using a grazing interval based on light interception resulted in reductions of 40% in nitrous oxide and 20% in methane per unit of milk when compared to the conventional method (Congio et al., 2018, 2019). Nevertheless, improving the grazing management strategy is not enough to match the energy needs of grazing dairy cows, and the use of energy-dense concentrates is required to meet the potential milk production. Concentrates rich in cereal grains are commonly used to increase cows' energy intake in pasture-based systems (Douglas et al., 2021). However, consuming large amounts of starch-based supplements may decrease fiber digestibility, the efficiency of nutrient utilization, and animal performance (Hills et al., 2015; Humer et al., 2018). Supplemental fat could be an alternative to increase dairy cows' energy intake and production efficiency as fat is energetically denser than carbohydrates. We previously demonstrated that feeding a palm oil-based fat supplement to grazing dairy cows enhanced the recovery of milk human-edible (HE) energy and protein as well as indispensable amino acids compared with extensively processed corn grain (Batistel et al., 2021). The use of a palm oil-based supplement reduced the environmental impact of the dairy production system by decreasing nitrogen excretion and methane output per unit of milk (Batistel et al., 2021). Another environmental advantage of incorporating fat supplements into ruminant diets is the reduction in waste generation, as most fat supplements are manufactured from byproducts generated by food and biodiesel industries.
Lately, the impact of the source of fatty acids and fatty acid profile on production responses and efficiency has been demonstrated on cows receiving total mixed rations. For instance, cows receiving a diet with palmitic acid (2% of the diet) improved feed efficiency and the energy was partitioned toward milk synthesis, whereas cows receiving a diet supplemented with soybean oil partitioned more energy toward body tissue gain (Liu et al., 2020). A dose-dependent response was previously reported to feeding increasing levels of palmitic acid replacing soyhulls indicating the need to determine optimal feeding doses that maximize animal performance (Rico et al., 2017). However, there is a lack of information regarding the ideal level of inclusion and fatty acid profile of supplemental fats to dairy cattle grazing tropical pastures. This information is especially important for grazing animals because high levels of fat and unsaturated fatty acids may disrupt rumen function and consequently decrease fiber digestion and animal production (Palmquist and Jenkins, 2017). Furthermore, there is a critical need for long-term studies to determine the potential effect of nutritional strategies implemented during the early lactation phase on the lactation cycle of grazing dairy cows. A carryover effect on the lactation cycle can enhance the efficiency of the production system and farm profitability.
Here, our first objective was to determine the ideal level of inclusion of calcium salts of palm and soybean oil (400 or 700 g/day) on rumen fermentation parameters and animal performance of dairy cows grazing tropical pastures. Subsequently, we investigated the effect of supplying grazing dairy cows with calcium salts of palm or soybean oil (400 g/day) during the early-lactation period on nitrogen excretion and recovery of HE nutrients in milk throughout the lactation cycle. We hypothesized that lower levels of supplemental fat and calcium salts of palm oil would positively influence the production efficiency of grazing dairy cows. Additionally, we postulated that feeding calcium salts of palm oil during early lactation would (a) enhance the recovery of HE nutrients, (b) decrease nitrogen excretion, and (c) enhance the milk production efficiency throughout the lactation cycle.
2. Materials and methods
Experiments were conducted at the University of São Paulo in Piracicaba, São Paulo, Brazil. The Ethical Committee for Animal Research of the University of São Paulo approved all procedures involving the animals.
2.1. Experiment 1
2.1.1. Experiment description
Five multiparous Jersey × Holstein dairy cows (rumen-cannulated) were used in a 5 × 5 Latin Square design. Cows at the start of the experiment had 428 ± 14 kg of body weight (mean ± standard deviation), milk yield of 16.5 ± 3.1 kg, and 115 ± 8.1 days in milk. Each experimental period lasted 24 days: 14 days for treatment adjustment and 10 days for sampling. The dietary treatments were as follows: (1) control (concentrate with no supplemental fat); (2) concentrate with a low dose of calcium salts of soybean fatty acids (CSSO; 400 g/day; Megalac-E, Química Geral do Nordeste, Rio de Janeiro, Brazil); (3) concentrate with a high dose of CSSO (700 g/day; Megalac-E); (4) concentrate with a low dose of calcium salts of palm fatty acids (CSPO; 400 g/day, Magnapac, Tectron Inc., Toledo, Parana, Brazil); and (5) concentrate with a high dose of CSPO (700 g/day, Magnapac). For the fat supplement dose, assuming an intake of 17.5 kg/day, we aimed to provide 2.0 and 3.5% of fatty acids of diet dry matter for low and high doses, respectively (NRC, 2001). We provided the treatments as a concentrate supplement (8 kg dry matter/day) fed twice a day individually before milking at 0400 and 1600 h. Grazing and cow management are described in detail elsewhere (de Souza et al., 2017). In summary, all cows were allocated daily to a new paddock (2,000 m2) grazing elephant grass (Pennisetum purpureum cv. Cameroon) with free access to natural shade and fresh water. The pasture was managed in a rotational grazing system based on canopy height. The entry height was 103 cm (Voltolini et al., 2010), and cows grazed the canopy down to 62 ± 2.2 cm. This procedure was adopted to avoid a reduction in short-term forage intake by sward structure, and therefore, animals should graze the canopy down to a maximum of 40% of the entry height (Fonseca et al., 2013). The ingredients and nutritional constituents of the concentrates and forage are presented in Supplementary Table 1.
2.1.2. Sample collection and analysis
During the sampling period, we recorded milk yield as a sum of both milkings. We collected milk samples from both milkings every 2 days and preserved them with a bronopol preservative pill. The contents of fat, protein, lactose, and milk urea nitrogen were determined in the milk samples using infrared procedures (Foss 4000; Foss North America, Eden Prairie, MN).
Composite forage, concentrate, and fecal samples were analyzed for dry matter, ash (AOAC, 2005), total nitrogen content by the Dumas combustion method using an N analyzer (Leco FP-2000 N Analyzer; Leco Instruments Inc., St. Joseph, MI), neutral detergent fiber (NDF; Van Soest et al., 1991) with sodium sulfite and heat-stable α-amylase for concentrate samples, acid detergent fiber (ADF) and lignin (AOAC, 2005), and gross energy by bomb calorimeter (Parr Instrument Inc., Moline, IL).
Forage intake and total dry matter intake were estimated from feed indigestibility values and total fecal excretion. To determine fecal output, we dosed cows twice daily with titanium dioxide (20 g/cow per day) during the last 15 days of each experimental period (de Souza et al., 2015). Fecal samples were collected during the last 5 days after milkings and then stored at −20°C. Subsequently, samples were thawed, dried at 55°C in a forced-air oven, ground through a 1-mm screen (Wiley mill, Thomas Scientific), and composited by the cow. Fecal samples were analyzed for titanium concentration according to Myers et al. (2004). We determined the indigestible NDF (iNDF) content of forage, concentrate, and fecal samples according to Huhtanen et al. (1994). The iNDF contents of forage and concentrate were divided by the iNDF content in the feces of each cow to calculate the indigestibility of feeds for each animal. Total fecal excretion, fecal excretion coming from the concentrate, forage intake, and total dry matter intake were calculated as previously described (de Souza et al., 2015).
On day 20 of each period, we collected samples of ruminal fluid from three locations in the ventral rumen sac. Samples were taken at 0 (relative to morning concentrate feeding), 2, 4, 6, 8, 12, 18, and 24 h. Samples of ruminal fluid were then preserved by adding 1 mL of 50% sulfuric acid and stored at −20°C. The ruminal fluid samples were thawed and processed by centrifugation (15,000 × g, 4°C, 30 min); the supernatant was analyzed for short-chain fatty acids (SCFA) by gas chromatography (Erwin et al., 1961) and ammonia nitrogen by the phenol-hypochlorite procedure (Chaney and Marbach, 1962). We calculated methane using SCFA stoichiometry using the equations generated by Williams et al. (2019).
2.1.3. Statistical analyses
We analyzed the data using the Mixed procedure of SAS 9.4 (SAS Institute) in a linear model that included the fixed effect of treatment and the random effect of cow and period. The data were checked for normality of the residuals using normal probability and box plots and for homogeneity of variances with plots of residuals vs. predicted values. For rumen variables that were collected through the day of sampling, a repeated procedure was used. We used the variance components matrix as the covariate structure for repeated-measures analysis based on the lowest Bayesian information criterion. Three preplanned orthogonal contrasts were tested to assess the overall effect of supplemental fat (CON vs. FAT), the effect of source of fatty acids (CSSO vs. CSPO), and the effect of supplement dose (low vs. high). Treatment differences were determined at P ≤ 0.05 and tendencies at P ≤ 0.10.
2.2. Experiment 2
2.2.1. Experiment description
For this study, we used a subset of cows from a previously published study (de Souza et al., 2017). In a previous publication, we presented results for nutrient digestibility production responses and milk fatty acid profile. A subset of 24 multiparous Jersey × Holstein dairy cows, with 490 ± 40 kg of body weight and 18 ± 2.0 days in milk, were used in a randomized complete block design. The experiment lasted 39 weeks, with supplementation and carryover periods lasting 13 and 26 weeks, respectively. Cows were blocked by previous lactation milk production, and blocks were balanced by body condition score and parity. Treatment diets were offered for 13 weeks during the supplementation period (from 3 to 16 weeks postpartum). The dietary treatments were as follows: (1) control (concentrate with no supplemental fat); (2) concentrate with CSSO (400 g/day; Megalac-E); and (3) concentrate with CSPO (400 g/day, Magnapac). All diets were formulated to meet predicted requirements (NRC, 2001). During the treatment period, all cows rotationally grazed elephant grass (Pennisetum purpureum cv. Cameroon) and the treatments were added to a concentrate supplement. The treatments were fed twice a day at 0400 and 1600 h individually before milking. Grazing management practices were the same as described in Experiment 1. From 17 to 42 weeks postpartum (carryover period), all cows received a common diet (without fat supplement) fed as a total mixed ration once a day at 0900 h.
2.2.2. Sample collection and analysis
We collected a weekly sample of concentrate and forage throughout the study. These samples were analyzed for dry matter, crude protein, NDF, ADF, lignin, ash, and fatty acids using the same methods described in Experiment 1. Starch was measured by an enzymatic method (Karkalas, 1985) after samples were gelatinized with sodium hydroxide, glucose concentration was measured with glucose oxidase, and absorbance was determined with a spectrophotometer. Cows were milked twice a day, and milk yield was recorded daily. Milk samples from both milkings were collected once a week and analyzed using the same methods described in Experiment 1.
We determined forage and total dry matter intakes on weeks 4, 8, 12, 24, and 36 from fecal excretion and feed indigestibility. We dosed titanium dioxide during 15 days of each period and collected fecal samples in the last 5 days. The methods and procedures to determine feed intake were the same as described in Experiment 1.
Urine samples were collected from each cow on weeks 4, 8, 12, 24, and 36. The samples were acidified with sulfuric acid (pH of urine < 5) and stored at −20°C until further analysis. We determined creatinine, allantoin, and uric acid using an HPLC instrument (Pimpa et al., 2001). Total nitrogen was measured by the micro-Kjeldahl procedure (AOAC, 1990). We estimated the total urine yield using the creatinine content of each sample, accounting for a constant excretion of creatinine at 0.213 mmol/kg of body weight (Chizzotti et al., 2008). We considered the intake of concentrate and pasture and their respective nitrogen contents to calculate N intake. The excretion of urinary nitrogen was calculated from total urine yield multiplied by its nitrogen content. Milk nitrogen efficiency (assuming no retention or mobilization of body nitrogen) calculated by dividing milk nitrogen output (milk crude protein/6.34) by total nitrogen intake. Fecal nitrogen excretion was calculated from fecal nitrogen × fecal output.
2.2.3. Recovery of HE nutrients
We estimated HE nutrient recovery using the approach proposed by Takiya et al. (2019). Based on sugar, starch, true protein, and fat concentrations in corn grain, soybean meal products, and fat supplements, we estimated HE metabolizable energy and protein contents of diets. During the supplementation period, we postulated two situations to calculate HE nutrient recovery in milk: First, we considered corn, soybean meal, and fat supplements as HE feeds and the pasture as unedible (Pasture Scenario); and in the second scenario, we considered that the land used for pasture production could be used to grow soybeans and corn (Land Scenario). For the land scenario, we estimated the HE metabolizable energy and protein using a blend of corn and dehulled soybeans (82 and 18% on a dry matter basis, respectively) in place of forage (elephant grass). Thus, we could grow 0.70 ha of corn and 0.30 ha of soybeans for every hectare used for elephant grass production. The annual yields (on a dry matter basis) of corn (5,319 kg/ha) and soybeans (3,032 kg/ha), retrieved from CONAB 2021/2022 Annual Crop Brazilian Production Summary (CONAB, 2022), were used to determine the potential HE energy and protein displaced by pasture production. We discounted the yield of soybean by 7.3% (Perkins, 1995) as soyhulls is not HE. Based on the yield of pasture leaves for elephant grass we calculated the total forage yield as previously reported (de Souza et al., 2017). To calculate the recovery of HE nutrients during the whole lactation, we used the average input and output of the pasture scenario plus the calculated input and output during the carryover period.
To account for amino acid recovery, we utilized the standardized ileal digestibility (SID) library and estimated the HE digestible amino acid inputs and outputs using the feed's total amino acid content. Recoveries of HE nutrients in milk were calculated as the ratio between nutrients secreted in milk and the corresponding HE nutrient intake by cows. To calculate the recovery of HE digestible AA during the whole lactation, we used the average input and output of the pasture scenario plus the calculated input and output during the carryover period.
2.2.4. Statistical analyses
We analyzed the data using the Mixed procedure of SAS 9.4 (SAS Institute) in a linear model including the fixed effect of treatment, time, and its interaction, and the random effect of the block. For the data accounting for the recovery of nutrients for the whole lactation, a reduced model without the time effect was used. The data were checked for normality of the residuals using normal probability and box plots and for homogeneity of variances with plots of residuals vs. predicted values. For rumen variables that were collected over the day of sampling, a repeated procedure was used. We used the variance components matrix as the covariate structure for repeated-measures analysis based on the lowest Bayesian information criterion. Treatment differences were determined at P ≤ 0.05 and tendencies at P ≤ 0.10.
3. Results
3.1. Experiment 1
Animal performance was affected by the fatty acid source of the supplemental fat (Figures 1A–I). Feeding cows with CSPO increased milk yield by 8.1% compared with CSSO (P = 0.02). Although intake was not affected by treatments, CSPO increased milk energy output by 20% compared with CSSO (P < 0.01). This result was associated with the CSPO treatment increasing milk fat content by 25.6% (P < 0.01) and milk fat yield by 35.2% (P < 0.01) when compared with feeding CSSO. Similarly, CSPO increased the yield of milk protein by 8.2% (P = 0.03) and milk lactose by 8.4% (P = 0.03) compared with CSSO. In general, the level of supplemental fat had a minor effect on production parameters. We observed a tendency for feeding the high dose of supplemental fat to decrease milk lactose content (P = 0.07) and milk fat yield (P = 0.10) compared with the low dose.
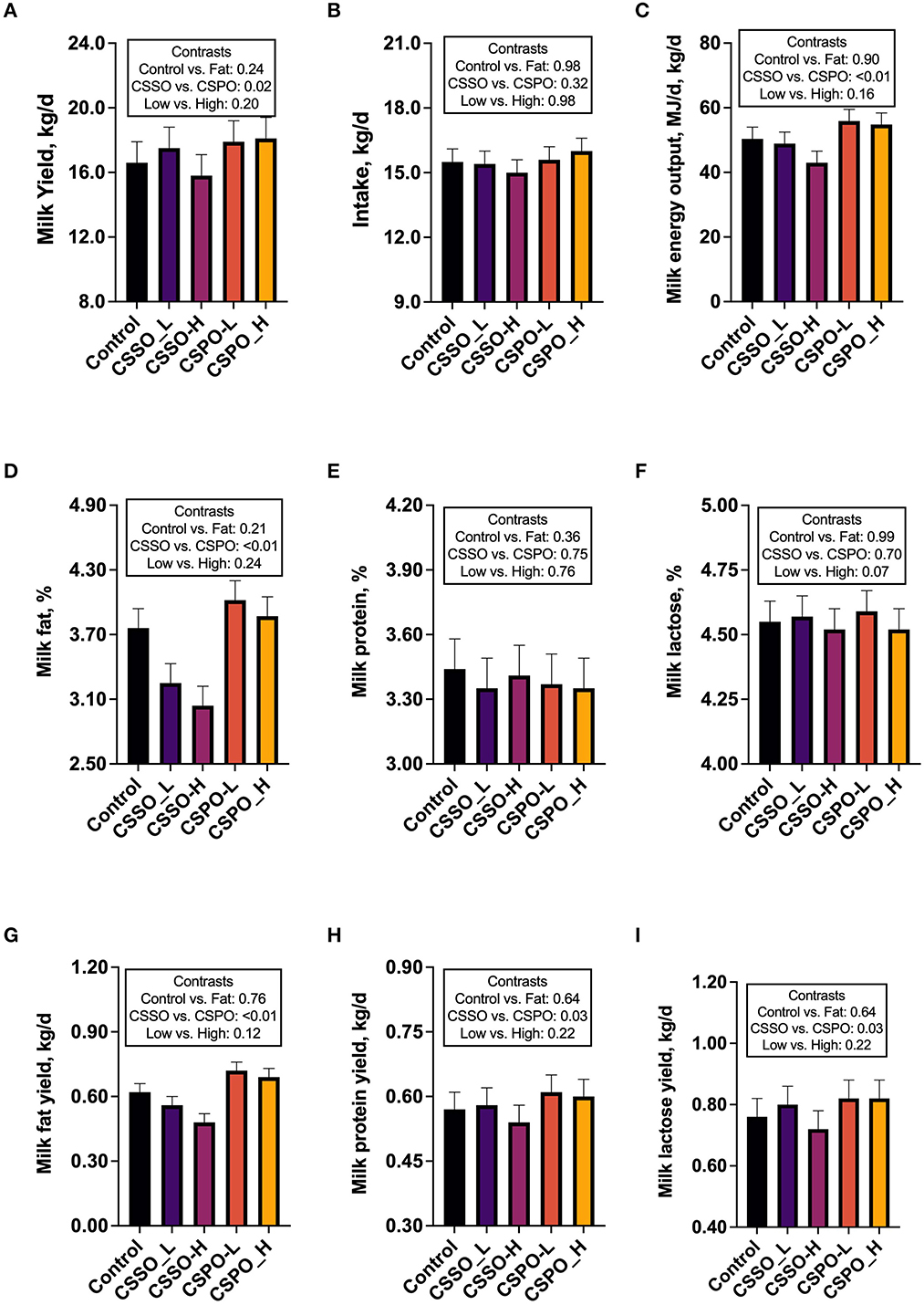
Figure 1. Milk production parameters (A–I) in cows that grazed elephant grass and received treatments as a concentrate supplement (Experiment 1). Treatments were a control diet (concentrate without supplemental fat) or diets supplemented with calcium salts of either soybean fatty acids (CSSO) or palm fatty acids (CSPO) fed at two levels of supplementation (L: 400 g/day; H: 700 g/day).
Compared with the control, feeding supplemental fat decreased the rumen concentration of isovalerate (P = 0.02; Table 1). There was no effect of fat supplementation on methane emission and intensity. CSPO increased rumen total SCFA production (P = 0.02) and acetate (P = 0.02) compared to CSSO. Furthermore, CSPO tended to increase butyrate by 5.0% (P = 0.09) and isovalerate by 4.3% (P = 0.10) and decrease methane per unit of milk energy by 16.3% (P = 0.07) compared with CSSO. Feeding a high dose of supplemental fat tended to reduce total SCFA production (P = 0.10) and acetate (P = 0.06) compared to a low dose.
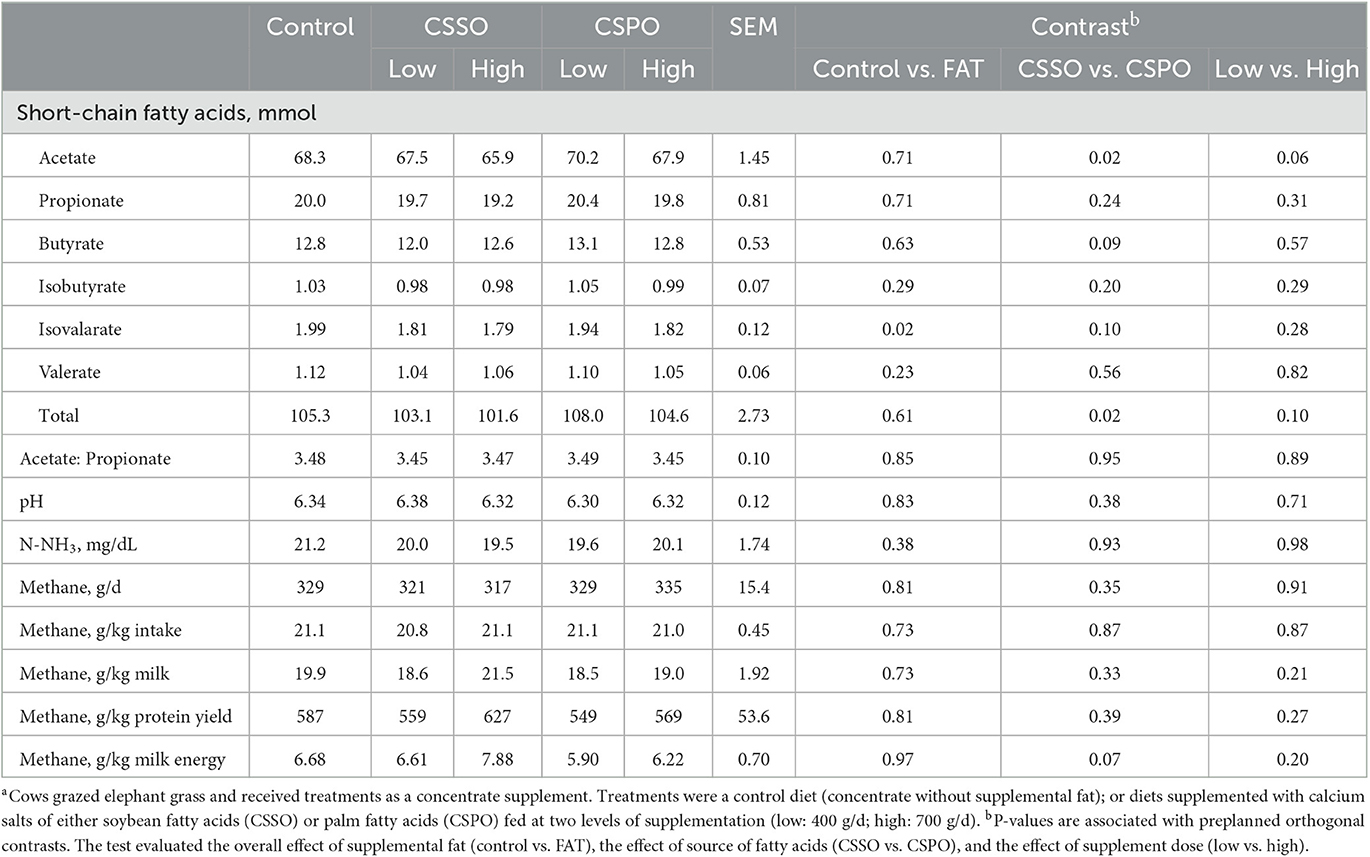
Table 1. Rumen fermentation parameters and methane estimates of dairy cows receiving the treatment dietsa (Experiment 1).
3.2. Experiment 2
In the pasture scenario during the supplementation period, feeding both CSSO and CSPO increased HE energy intake compared with control (P = 0.01; Table 2). Regarding the land scenario, supplemental fat did not impact intakes of energy and protein (P > 0.10). Cows fed CSPO had greater milk energy (P < 0.01) and protein output (P = 0.04) compared with control and CSSO. For total lactation, in both scenarios, we did not observe a treatment effect for HE intakes of energy and protein. In contrast, CSPO increased total lactation energy (P < 0.01) and protein output (P = 0.02) compared with CSSO and control.
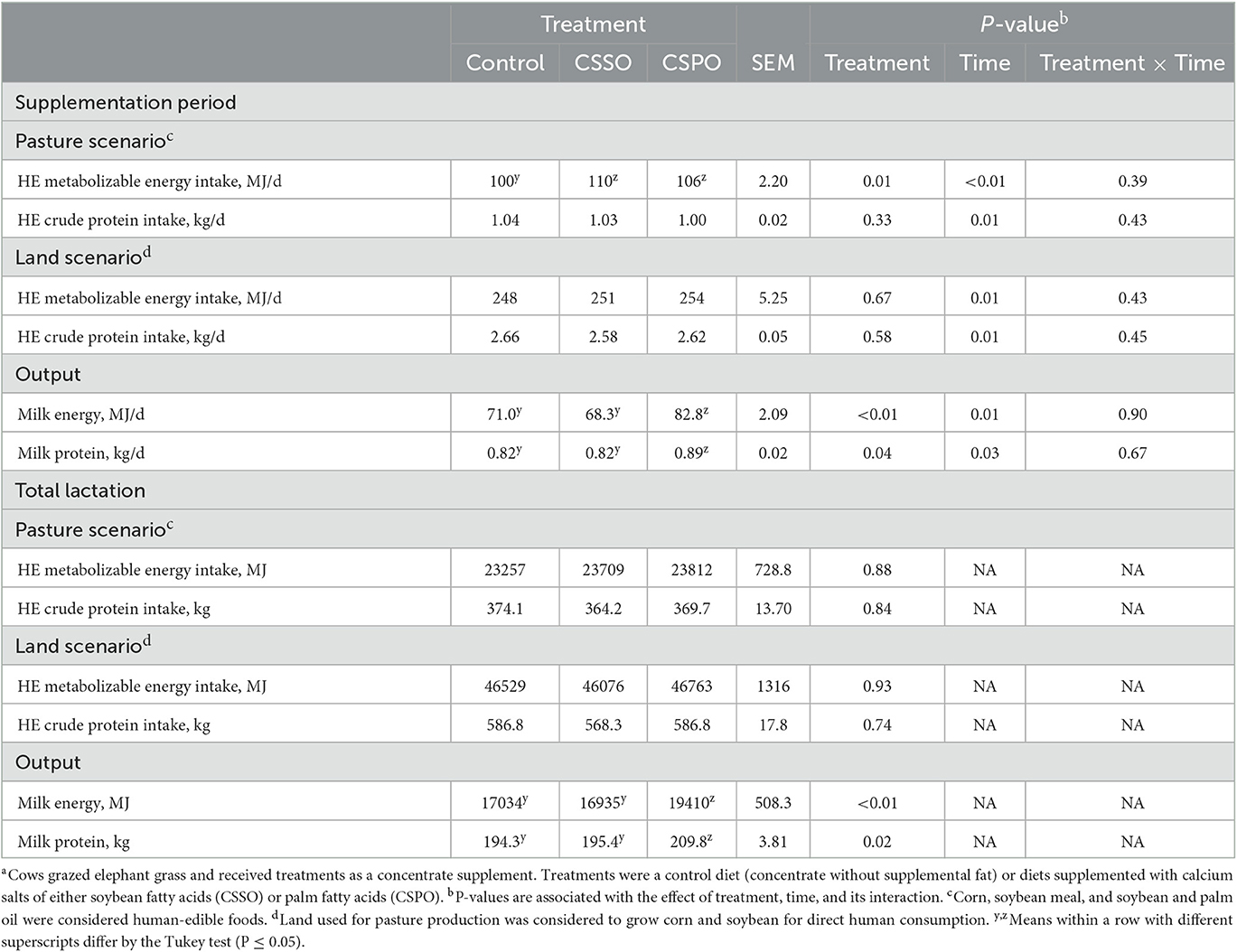
Table 2. Human-edible (HE) nutrient inputs and outputs of dairy cows receiving the treatment diets during the supplementation period and total lactationa (Experiment 2).
Regarding the pasture scenario during the supplementation period, feeding CSPO improved the recovery of HE energy by 10 and 25% compared with control and CSSO, respectively (P < 0.01; Figure 2A). Similarly, CSPO enhanced the recovery of HE protein by 14 and 12% compared with control and CSSO, respectively (P < 0.01; Figure 2B). Feeding cows with CSSO reduced the recovery of HE energy (P < 0.01) but did not affect the recovery of protein compared to the control. The HE energy (P < 0.01) and protein (P < 0.01) recoveries decreased in the land scenario when compared to the pasture scenario. In the land scenario during the supplementation period, CSPO improved the recovery of HE energy (P < 0.01; Figure 2C) and protein (P < 0.01; Figure 2D) compared with other treatments. For total lactation, in both scenarios feeding CSPO increased (P < 0.01) the recovery of HE energy and tended to increase (P ≤ 0.10) the recovery of HE protein compared with other treatments.
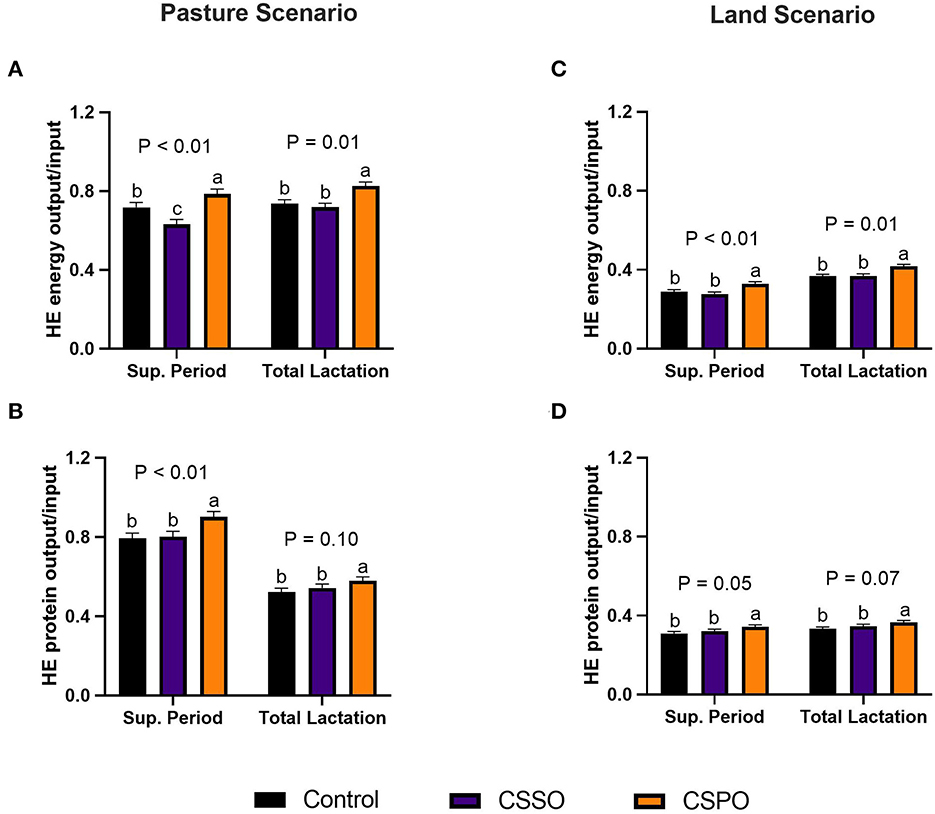
Figure 2. Human-edible (HE) metabolizable energy and protein conversion rate in cows that grazed elephant grass and received treatments as a concentrate supplement in a pasture scenario (A, B) or land scenario (C, D) during the supplementation period and total lactation. The concentrate contained a control diet (not supplemented with fat) or supplemented with calcium salts of either soybean fatty acids (CSSO) or palm fatty acids (CSPO) fed during the supplementation period. The letters a, b, and c indicate “Means within different superscripts differ by the Tukey test (P ≤ 0.05)”.
We did not observe treatment differences for nitrogen excretion in the feces in both the supplementation period and total lactation (Figure 3A; P > 0.10). During the supplementation period, feeding CSPO reduced the excretion of nitrogen in urine by 4% compared with CSSO and control (Figure 3B; P = 0.01). Feeding CSPO tended to increase milk nitrogen efficiency by 7 and 13% compared with CSSO and control, respectively, in both the supplementation period and total lactation (Figure 3C; P = 0.09). We also calculated the recovery of amino acids considering the pasture scenario. Feeding cows with CSPO increased the recovery of all indispensable amino acids evaluated by 27 and 14% compared with control and CSSO, respectively (P < 0.05; Figure 4). Also, cows fed with CSSO had greater recovery of all amino acids evaluated (between 9 and 11%) compared with the control (P < 0.05). Overall, CSPO tended to increase the recovery of all indispensable amino acids evaluated by 23% over control during total lactation (P < 0.10).
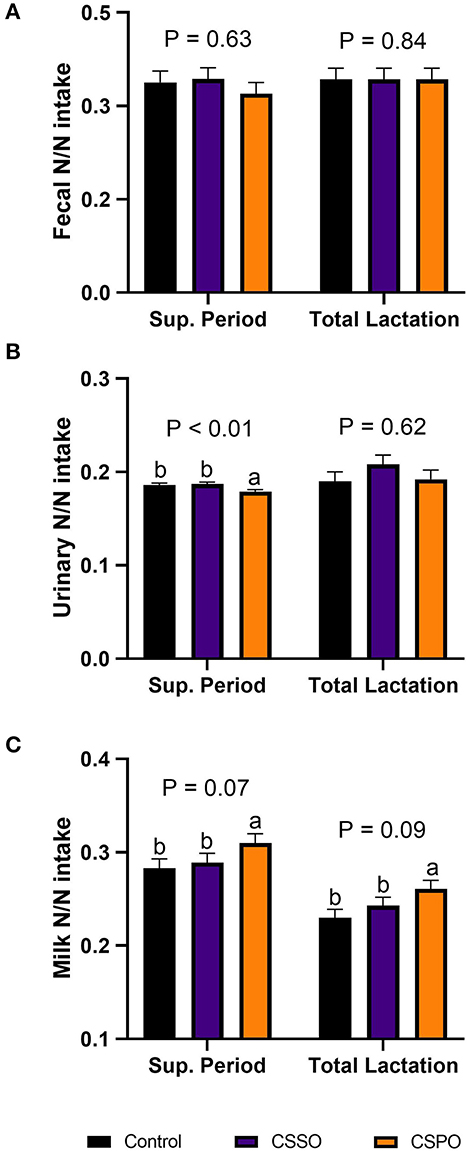
Figure 3. Apparent nitrogen digestion (A) and nitrogen output in urine (B) and milk (C) in cows that grazed elephant grass and received treatments as a concentrate supplement during the supplementation period and total lactation. The concentrate contained a control diet (not supplemented with fat) or supplemented with calcium salts of either soybean fatty acids (CSSO) or palm fatty acids (CSPO) fed during the supplementation period. The letters a, b, and c indicate “Means within different superscripts differ by the Tukey test (P ≤ 0.05)”.
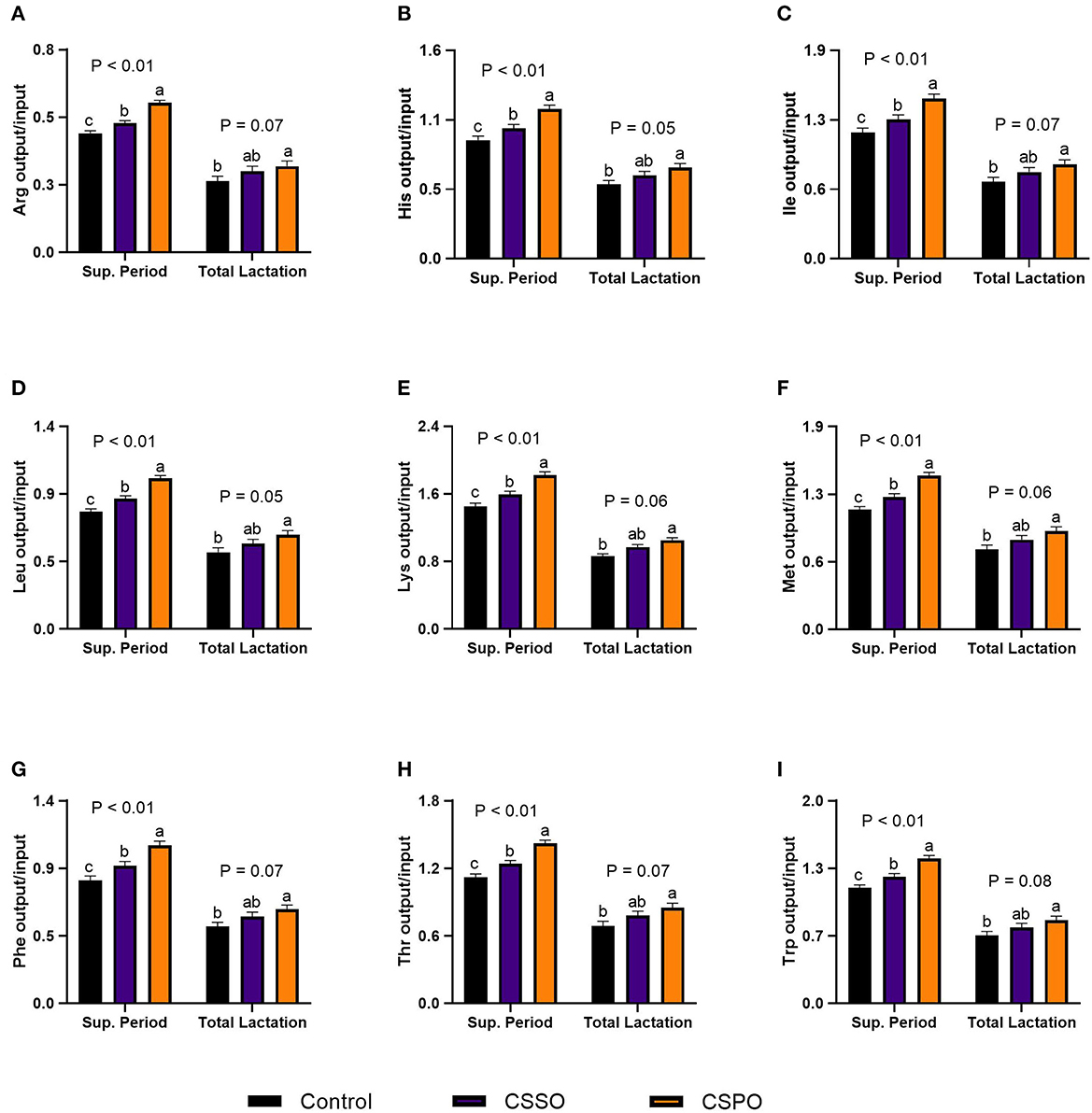
Figure 4. Milk recovery of indispensable amino acids (A–I) in cows that grazed elephant grass and received treatments as a concentrate supplement during the supplementation period and total lactation. The concentrate contained a control diet (not supplemented with fat) or supplemented with calcium salts of either soybean fatty acids (CSSO) or palm fatty acids (CSPO) fed during the supplementation period. The letters a, b, and c indicate “Means within different superscripts differ by the Tukey test (P ≤ 0.05)”.
4. Discussion
Recently, considerable advances in forage management practices have been made to improve the nutritional value of tropical grasses (Da Silva et al., 2013). Nevertheless, energy is the major constraint to improving dairy cattle production efficiency in tropical pasture-based systems. Currently, concentrates rich in cereal grains are commonly used to increase cows' energy intake in pasture-based systems (Douglas et al., 2021). However, feeding large amounts of starch-based supplements may decrease fiber digestibility, the efficiency of nutrient utilization, and animal performance (Hills et al., 2015; Humer et al., 2018). Additionally, the use of this type of concentrate represents a direct competition of food ingredients for humans. We previously demonstrated that adding a supplemental fat of palm oil was more efficient than cereal grains to improve the efficiency of recovery of nutrients in milk and to reduce methane emissions and nitrogen excretion in tropical grazing conditions during the supplementation period (Batistel et al., 2021). However, there is a lack of information regarding the ideal level of inclusion and fatty acid profile of supplemental fats to enhance dairy cattle production efficiency and decrease nitrogen excretion in tropical grazing systems. Furthermore, the long-term impacts of feeding strategies during early lactation on production efficiency are poorly understood. Thus, our first objective was to determine the ideal level of inclusion of calcium salts of palm and soybean oil (400 or 700 g/day) on rumen fermentation and animal performance of grazing dairy cows. Our follow-up objective was to investigate the effect of supplying calcium salts of palm or soybean oil to dairy cows grazing tropical pastures during the early-lactation period on nitrogen excretion and recovery of HE nutrients in milk throughout the lactation cycle.
Interestingly, animal production, rumen fermentation, and methane emissions were primarily influenced by the fatty acid source in the diet but not by the feeding level. It has been previously established that the fatty acid profile of a fat supplement is most likely the primary factor affecting production responses to it (Lock and de Souza, 2016). Additionally, determining the response to different sources of fat is essential, considering the sustainability aspects of different agricultural activities. Lately, sustainability and deforestation concerns have been raised over the utilization of palm oil (Teng et al., 2020; Murphy et al., 2021). We observed that feeding CSPO increased milk yield by 8.1% compared with CSSO indicating a major influence of the fatty acid profile on milk yield responses. Feeding fat supplements not only increases the dietary energy density but also increases energetic efficiency in lactating cows due to increased ATP efficiency compared to SCFA and by direct utilization of long-chain fatty acids for milk fat synthesis (Palmquist and Jenkins, 2017). Additionally, fatty acids may spare glucose utilization as the downregulation of de novo fatty acids synthesis may reduce the need for reducing equivalents for milk fat synthesis (Storry et al., 1973). In our prior study, both fat supplements decreased de novo milk fatty acids (< 16 carbon) (de Souza et al., 2017), so the mechanism mentioned above may be at least in part responsible for these results. Feeding CSPO increased the yield of milk fat and energy output by 35 and 20%, respectively, when compared to CSSO. Improved milk yield and yield of milk components when fat supplements contain more saturated fatty acids have been previously reported (de Souza et al., 2018). A recent study indicated increased milk fat output (22%) and milk energy output (8%) partially due to changes in insulin metabolism when feeding a palmitic acid supplement rather than soybean oil in dairy cows (Liu et al., 2020). In our study, we fed the supplements as calcium salts to reduce the negative effect of feeding fat to the rumen microbial population. However, ruminal pH and the degree of unsaturation of the fat source majorly influence the stability of calcium salts (Sukhija and Palmquist, 1990). In general, diet-induced milk fat depression is commonly detected in cows receiving plant or fish oil supplements and/or fed with rapidly degradable carbohydrates (Dewanckele et al., 2020). Under specific dietary conditions, altered ruminal biohydrogenation of unsaturated fatty acids may lead to the formation of biohydrogenation intermediates that inhibit fat synthesis in the mammary gland (Bauman et al., 2011). Furthermore, CSSO supplementation tended to reduce acetate, butyrate, and total SCFA in the rumen. Acetate and butyrate are major sources of energy generated by rumen fermentation and building blocks for de novo milk fat synthesis (Palmquist and Harvatine, 2020). Similar to our results, a previous study feeding CSSO to grazing cows also observed a negative effect on several isoacids and parameters of rumen fermentation (Macedo et al., 2016). A decrease in microbial growth can be postulated as the reduced concentration of these isoacids in the rumen fluid of CSSO-supplemented cows may result in a reduction in the population of acetate-producing cellulolytic bacteria. Altogether, our results indicated that fat supplements based on palm oil improved production outcomes compared to supplements based on soybean oil.
Knowledge about the optimal dietary inclusion level of fat supplements with specific fatty acid profiles brings the advantage of optimizing animal performance and farm profitability. In our study, feeding increasing levels of supplemental fat did not influence feed intake, milk yield, and the yield of milk components. Replacing a corn-based concentrate with 400 g/day of CSPO increased the yields of milk and energy-corrected milk and did not affect feed intake in the first 16 weeks postpartum in grazing cows (Batistel et al., 2017). However, the production response to different doses of fat inclusion in the diet has been variable. A previous study reported a dose-dependent response to feeding increasing levels of palmitic acid replacing soyhulls in the diet of dairy cows with animal performance peaking when the supplement was fed at 420 g/day (Rico et al., 2017). Increasing the level of a CSPO supplement in the diet (from 200 to 600 g/d) of early lactating dairy cows grazing a tropical pasture linearly increased milk yield and the yield of milk components (dos Santos Neto et al., 2022). One reason for the lack of results in our study is the possibility that the high feeding level was over the optimal level. Additionally, the inconsistency in production responses due to different levels of supplemental fat may be related to the total dietary fat, nutrient replaced (fiber vs. starch), and stage of lactation. In our study, we also did not observe major effects of feeding levels on rumen fermentation parameters as only acetate concentration was reduced as the feeding level increased. Acetate is the major SCFA produced in rumen, and it is strongly related to fiber digestion. In general, based on our results, feeding supplemental fat levels over 400 g/day did not influence rumen fermentation and animal performance of grazing cows. As fat supplements are generally more expensive than cereal grains, the lack of production responses precludes feeding higher doses of supplemental fat.
Several targets of mitigation practices for the dairy industry have been discussed lately, and the reduction of enteric methane production is one of the main goals (Hristov et al., 2013). Therefore, to improve the sustainability and profitability of dairy production systems, nutritional strategies to mitigate enteric methane emissions are warranted (Shetty et al., 2017). In our study, we utilize SCFA stoichiometry to estimate methane output rather than directly measuring it. This technique has been used previously due to the strong correlation between rumen fermentation profile and methane output (Cabezas-Garcia et al., 2017; de Souza et al., 2020). Importantly, different methods of measuring methane have different degrees of uncertainty and variability in methane measurements (Hristov et al., 2018). In this study, we observed a tendency for CSPO to decrease methane per unit of milk energy output by 16.3% compared with CSSO with no differences in feed intake between treatments. Similarly, our previous study with grazing dairy cows indicated a reduction in methane per unit of milk (-31%) and per unit of milk energy output (-29%) when CSPO was fed compared with non-fat control diet (Batistel et al., 2021). Feeding supplemental fat has been shown to decrease methane yield in cattle and sheep (Grainger and Beauchemin, 2011). The effect of supplemental fat on methane yield may depend on the fatty acid profile and form of the additional fat. For example, feeding a diet supplemented with calcium salts of palm plus linseed oil decreased methane yield and methane per unit of milk compared with linseed oil and a non-fat control diet (Kliem et al., 2019). Also, the recent publication of NASEM (2021) suggested that intake, fiber digestibility, and dietary fat levels as factors that influence enteric methane production in dairy cows. It also proposes a linear decrease in methane production as dietary fat level increases (NASEM, 2021). Reduction in methane yield may be achieved by strategies that reduce feed intake. Nonetheless, reducing feed intake will also decrease animal performance, increasing methane intensity by a unit of milk, which is not desired. Our data suggest that feeding CSPO can decrease methane intensity mostly due to the increased performance of cows receiving CSPO without influencing intake.
Increasing the efficiency of livestock production has been proposed to reduce the environmental impact of the livestock sector (Patel et al., 2017). Recently, Wilkinson (2011) proposed a new method of measuring efficiency considering edible feeds to relate HE nutrient inputs and outputs in animal products. A decrease in the utilization of pesticides, soil erosion, and greenhouse emissions was suggested in a food system model assuming minimal HE feeds used for feeding animals (feeding animals with a large amount of grass and by-products) (Schader et al., 2015). As a result, supplying animals with ingredients inedible for human consumption, such as by-products and grasses, will have a positive impact on global food production and environmental impact. However, there is a role for strategically using HE feeds to increase the efficiency of milk production because forage-only systems are associated with relatively low output per head (Wilkinson and Lee, 2018). In our second experiment, the recovery of HE nutrients was calculated under two scenarios. First, we assumed soybean meal, corn, and fat supplement as HE feeds and the pasture as inedible (Pasture scenario); and then, we assumed that the land used for pasture production could be used to grow soybeans and corn (Land Scenario). In the pasture scenario, during the supplementation period feeding CSPO improved the recovery of HE energy by 10 and 25% compared with control and CSSO, respectively. Similarly, CSPO enhanced the recovery of HE protein by 14 and 12% compared with control and CSSO, respectively. Corroborating our results, a previous study with grazing cows indicated enhanced recovery of HE energy (+20%) and protein (+17%) when the fat supplement was provided in diets (Batistel et al., 2021). We observed a decrease in the recovery of HE energy and protein between the pasture and land scenarios (-40 and−51%, respectively); however, feeding CSPO also improved the recovery of HE energy and protein compared with other treatments regardless of the scenario. It is essential to point out that non-arable land can be used to produce forage, but our model considered that all land would be used for crop production rather than forage production. Altogether, our results suggest that partial replacement of cereal grains (corn) by supplemental fatty acids improved HE energy and protein recoveries, but the magnitude of response varies by the fatty acid profile of the supplemental fat.
Although some studies suggested a positive effect on food supply and climate impact of reducing consumption of animal protein (Foley et al., 2011; González et al., 2011), it is important to consider that ruminants can convert poor quality nitrogen that is inedible to human consumption into a high-quality protein. In many developing nations, accounting for protein quality is essential when comparing protein sources as protein consumption is generally limited (Ritchie et al., 2018). When considering HE feed conversion efficiency, the nutritional value of animal protein is twice greater than plant protein (Patel et al., 2017). In our study, we accounted for protein quality based on the value of milk-digestible amino acids. On average, we observed that the recovery of HE indispensable amino acids varied from 47 to 187%. Recovery rates over 100% were observed for lysine (162%), methionine (127%), isoleucine (129%), and tryptophan (127%). Recovery of over 100% of the supply of indispensable amino acids contributes to food security as these are essential nutrients to human diets. Enhancing the recovery of lysine and methionine is important as these amino acids are considered limited and are presented in suboptimal proportions for human needs in grain-based diets (Mariotti and Gardner, 2019). In addition, our results indicated that during the supplementation period feeding cows with CSPO increased the recovery of all amino acids evaluated by 27 and 14% compared to control and CSSO, respectively. Also, cows fed with CSSO had higher recovery of all amino acids (between 9 and 11%) compared with the control. Thus, our data suggest that it is possible to improve the efficiency of recovery of HE nutrients, especially indispensable amino acids, by manipulating fatty acid sources in the dairy cows' diet.
In this study, we first demonstrate improving the recovery of HE nutrients over the course of a whole lactation cycle by strategically altering animal nutrition strategies during a specific period (early lactation). The use of supplementation with HE feeds strategically to increase the efficiency of milk production in forage-based systems is important (Wilkinson and Lee, 2018). We target supplemented grazing cows with CSSO and CSPO during early lactation (over 13 weeks) but assess its impact over the lactation (42 weeks). Interestingly, we demonstrated that feeding CSPO improved the recovery of HE energy (+13%), protein (+11%), and indispensable amino acids (+23%) compared with control over the lactation period. Previous studies have demonstrated that early lactation feeding programs may have a carryover effect (Jørgensen et al., 2016). Usually, each extra kilogram of milk secreted at peak production results in approximately 200 kg of milk subsequent during lactation (Roche et al., 2013), suggesting that supplementation during early lactation that increases milk production at peak could have positive effects even after supplementation ceased. Therefore, increased response beyond the supplementation period not only improved the efficiency of nutrients recovered in milk but also may have a positive impact on the economic viability of this strategy. We also indicated an improvement in milk nitrogen efficiency by 13% over the lactation period when feeding CSPO compared with the control. Improving nitrogen efficiency is a central goal of livestock production as excess nitrogen can contribute to air, water, and soil pollution. Although several nutrition strategies have been proposed to improve nitrogen efficiency, their utilization may negatively influence production and reproduction performance (Hristov et al., 2013; Dijkstra et al., 2018). In agreement with our results, previous studies reported that feeding fatty acids supplements enhanced milk nitrogen efficiency by 8 to 17% in dairy cows under confinement or grazing systems (Nichols et al., 2018; Batistel et al., 2021). Fatty acids do not directly contribute to glucose synthesis. However, providing dietary fatty acids may spare glucose as preformed fatty acids may be incorporated into milk fat, reducing the need for glucose associated with de novo synthesis (Rico et al., 2017). Therefore, providing energy precursors and preformed fatty acids may reduce the use of amino acids for gluconeogenesis or energy-yielding oxidative processes favoring the utilization of indispensable amino acids for milk protein synthesis (Rius et al., 2010). Our results indicated that supplemental fat based on palm oil can improve nitrogen efficiency not only during the supplementation period suggesting a key role of energy in nitrogen metabolism. Importantly, in our study, the improvement in the recovery of HE nutrients is associated with a greater nutrient output instead of a reduction in input. Altogether, our results suggest that target supplementation of fat supplements can improve the recovery of HE nutrients and reduce nitrogen excretion throughout lactation in dairy cows.
5. Conclusion
In this study, we demonstrated that changing the source of supplemental fat in the diet of grazing dairy cows reduced nitrogen excretion and methane emissions in dairy systems. Production, rumen fermentation, and methane emissions were largely influenced by the source of fatty acid in the diet but not by the feeding level. Furthermore, grazing dairy cows are efficient at using human-edible foods yielding a net increase in digestible indispensable amino acids. Our results suggest that partial replacement of cereal grains (corn) by supplemental fatty acids improves the recovery of human-edible energy and protein, but the magnitude of response varies by the fatty acid profile of supplemental fat. Furthermore, we first demonstrate improving the recovery of human-edible nutrients over the course of a whole lactation cycle by strategically altering nutrition during early lactation. We also demonstrated that feeding dairy cows with a fatty acid source based on palm oil under tropical grazing conditions reduced nutrient excretion and improved the recovery of HE nutrients over the lactation cycle.
Data availability statement
The raw data supporting the conclusions of this article will be made available by the authors, without undue reservation.
Ethics statement
The animal study was reviewed and approved by Ethical Committee for Animal Research of the University of São Paulo, Piracicaba.
Author contributions
FB, JS, and FS contributed to the overall design of the study. FB and JS carried out the experiments, acquisition of data, analyses, and drafted and revised the first version of the manuscript. All authors reviewed and approved the final manuscript.
Funding
This study was supported by the State of São Paulo Research Foundation (São Paulo, SP, Brazil) project 12/09535-1.
Acknowledgments
The authors would like to thank the employees of the Department of Animal Sciences of the University of São Paulo and the internship students Katiéli Caroline Welter, Márcio Rezende Ribeiro Soares, and Mateus Mendes Vilanova e Silva (all from The University of São Paulo) for their assistance with the fieldwork.
Conflict of interest
JS is employed by the company Perdue Agribusiness.
The remaining authors declare that the research was conducted in the absence of any commercial or financial relationships that could be construed as a potential conflict of interest.
Publisher's note
All claims expressed in this article are solely those of the authors and do not necessarily represent those of their affiliated organizations, or those of the publisher, the editors and the reviewers. Any product that may be evaluated in this article, or claim that may be made by its manufacturer, is not guaranteed or endorsed by the publisher.
Supplementary material
The Supplementary Material for this article can be found online at: https://www.frontiersin.org/articles/10.3389/fsufs.2023.1186454/full#supplementary-material
References
Batistel, F., de Souza, J., and Santos, F. A. P. (2017). Corn grain-processing method interacts with calcium salts of palm fatty acids supplementation on milk production and energy balance of early-lactation cows grazing tropical pasture. J. Dairy Sci. 100, 5343–5357. doi: 10.3168/jds.2016-12503
Batistel, F., de Souza, J., Vaz Pires, A., and Santos, F. A. P. (2021). Feeding grazing dairy cows with different energy sources on recovery of human-edible nutrients in milk and environmental impact. Front. Sustain. Food Syst. 5, 1–16. doi: 10.3389/fsufs.2021.642265
Bauman, D. E., Harvatine, K. J., and Lock, A. L. (2011). Nutrigenomics, rumen-derived bioactive fatty acids, and the regulation of milk fat synthesis. Annu. Rev. Nutr. 31, 299–319. doi: 10.1146/annurev.nutr.012809.104648
Cabezas-Garcia, E. H., Krizsan, S. J., Shingfield, K. J., and Huhtanen, P. (2017). Between-cow variation in digestion and rumen fermentation variables associated with methane production. J. Dairy Sci. 100, 4409–4424. doi: 10.3168/jds.2016-12206
Chaney, A. L., and Marbach, E. P. (1962). Modified reagents for determination of urea and ammonia. Clin. Chem. 8, 130–132. doi: 10.1093/clinchem/8.2.130
Chizzotti, M. L., Valadares Filho, S.d.C, Valadares, R. F. D., Chizzotti, F. H. M., and Tedeschi, L.O. (2008). Determination of creatinine excretion and evaluation of spot urine sampling in Holstein cattle. Livest. Sci. 113, 218–225. doi: 10.1016/j.livsci.2007.03.013
CONAB (2022). Acompanhamento da Safra Brasileira de Graos. Available online at: https://www.conab.gov.br/ (accessed January 15, 2023).
Congio, G. F. S., Batalha, C. D. A., Chiavegato, M. B., Berndt, A., Oliveira, P. P. A., Frighetto, R. T. S., et al. (2018). Strategic grazing management towards sustainable intensification at tropical pasture-based dairy systems. Sci. Total Environ. 636, 872–880. doi: 10.1016/j.scitotenv.2018.04.301
Congio, G. F. S., Chiavegato, M. B., Batalha, C. D. A., Oliveira, P. P. A., Maxwell, T. M. R., Gregorini, P., et al. (2019). Strategic grazing management and nitrous oxide fluxes from pasture soils in tropical dairy systems. Sci. Total Environ. 676, 493–500. doi: 10.1016/j.scitotenv.2019.04.186
Da Silva, S. C., Gimenes, F. M. A., Sarmento, D. O. L., Sbrissia, A. F., Oliveira, D. E., Hernadez-Garay, A., et al. (2013). Grazing behaviour, herbage intake and animal performance of beef cattle heifers on marandu palisade grass subjected to intensities of continuous stocking management. J. Agric. Sci. 151, 727–739. doi: 10.1017/S0021859612000858
de Souza, J., Batistel, F., and Santos, F. A. P. (2017). Effect of sources of calcium salts of fatty acids on production, nutrient digestibility, energy balance, and carryover effects of early lactation grazing dairy cows. J. Dairy Sci. 100, 1072–1085. doi: 10.3168/jds.2016-11636
de Souza, J., Batistel, F., Welter, K. C., Silva, M. M., Costa, D. F., and Portela Santos, F. A. (2015). Evaluation of external markers to estimate fecal excretion, intake, and digestibility in dairy cows. Trop. Anim. Health Prod. 47, 265–268. doi: 10.1007/s11250-014-0674-6
de Souza, J., Leskinen, H., Lock, A. L., Shingfield, K. J., and Huhtanen, P. (2020). Between-cow variation in milk fatty acids associated with methane production. PLoS ONE 15:e0235357. doi: 10.1371/journal.pone.0235357
de Souza, J., Preseault, C. L., and Lock, A. L. (2018). Altering the ratio of dietary palmitic, stearic, and oleic acids in diets with or without whole cottonseed affects nutrient digestibility, energy partitioning, and production responses of dairy cows. J. Dairy Sci. 101, 172–185. doi: 10.3168/jds.2017-13460
Dewanckele, L., Toral, P. G., Vlaeminck, B., and Fievez, V. (2020). Invited review: Role of rumen biohydrogenation intermediates and rumen microbes in diet-induced milk fat depression: an update. J. Dairy Sci. 103, 7655–7681. doi: 10.3168/jds.2019-17662
Dijkstra, J., Bannink, A., Bosma, P. M., Lantinga, E. A., and Reijs, J. W. (2018). Modeling the effect of nutritional strategies for dairy cows on the composition of excreta nitrogen. Front. Sustain. Food Syst. 2. 1–16. doi: 10.3389/fsufs.2018.00063
dos Santos Neto, J. M., Silva, J. O., Meschiatti, M. A. P., de Souza, J., Negrão, J. A., Lock, A. L., et al. (2022). Increasing levels of calcium salts of palm fatty acids affect production responses during the immediate postpartum and carryover periods in dairy cows. J. Dairy Sci. 105, 9652–9665. doi: 10.3168/jds.2022-22337
Douglas, M. L., Auldist, M. J., Wright, M. M., Marett, L. C., Russo, V. M., Hannah, M. C., et al. (2021). Using estimated nutrient intake from pasture to formulate supplementary concentrate mixes for grazing dairy cows. J. Dairy Sci. 104, 4350–4361. doi: 10.3168/jds.2020-19383
Erwin, E. S., Marco, G. J., and Emery, E. M. (1961). Volatile fatty acid analyses of blood and rumen fluid by gas chromatography. J. Dairy Sci. 44, 1768–1771. doi: 10.3168/jds.S0022-0302(61)89956-6
Foley, J. A., Ramankutty, N., Brauman, K. A., Cassidy, E. S., Gerber, J. S., Johnston, M., et al. (2011). Solutions for a cultivated planet. Nature 478, 337–342. doi: 10.1038/nature10452
Fonseca, L., Carvalho, P. C. F., Mezzalira, J. C., Bremm, C., Galli, J. R., and Gregorini, P. (2013). Effect of sward surface height and level of herbage depletion on bite features of cattle grazing Sorghum bicolor swards1. J. Animal Sci. 91, 4357–4365. doi: 10.2527/jas.2012-5602
González, A. D., Frostell, B., and Carlsson-Kanyama, A. (2011). Protein efficiency per unit energy and per unit greenhouse gas emissions: Potential contribution of diet choices to climate change mitigation. Food Policy 36, 562–570. doi: 10.1016/j.foodpol.2011.07.003
Górska-Warsewicz, H. A.-O., Rejman, K. A.-O., Laskowski, W. A. O., and Czeczotko, M. (2019). Milk and dairy products and their nutritional contribution to the average polish diet. Nutrients 11, 1–19. doi: 10.3390/nu11081771
Grainger, C., and Beauchemin, K. A. (2011). Can enteric methane emissions from ruminants be lowered without lowering their production? Anim. Feed Sci.Technol. 166, 308–320. doi: 10.1016/j.anifeedsci.2011.04.021
Hills, J. L., Wales, W. J., Dunshea, F. R., Garcia, S. C., and Roche, J. R. (2015). Invited review: an evaluation of the likely effects of individualized feeding of concentrate supplements to pasture-based dairy cows. J. Dairy Sci. 98, 1363–1401. doi: 10.3168/jds.2014-8475
Hristov, A. N., Kebreab, E., Niu, M., Oh, J., Bannink, A., Bayat, A. R., et al. (2018). Symposium review: uncertainties in enteric methane inventories, measurement techniques, and prediction models. J. Dairy Sci. 101, 6655–6674. doi: 10.3168/jds.2017-13536
Hristov, A. N., Oh, J., Firkins, J. L., Dijkstra, J., Kebreab, E., Waghorn, G., et al. (2013). Special topics - Mitigation of methane and nitrous oxide emissions from animal operations: I. a review of enteric methane mitigation options. J. Anim Sci. 91, 5045–5069. doi: 10.2527/jas.2013-6583
Huhtanen, P., Kaustell, K., and Jaakkola, S. (1994). The use of internal markers to predict total digestibility and duodenal flow of nutrients in cattle given six different diets. Anim. Feed Sci.Technol 48, 211–227. doi: 10.1016/0377-8401(94)90173-2
Humer, E., Petri, R. M., Aschenbach, J. R., Bradford, B. J., Penner, G. B., Tafaj, M., et al. (2018). Invited review:Practical feeding management recommendations to mitigate the risk of subacute ruminal acidosis in dairy cattle. J. Dairy Sci. 101, 872–888. doi: 10.3168/jds.2017-13191
Jørgensen, C. H., Spörndly, R., Bertilsson, J., and Østergaard, S. (2016). Invited review: Carryover effects of early lactation feeding on total lactation performance in dairy cows. J. Dairy Sci. 99, 3241–3249. doi: 10.3168/jds.2014-9043
Karkalas, J. (1985). An improved enzymatic method for the determination of native and modified starch. J. Sci. Food Agric. 36, 1019–1027. doi: 10.1002/jsfa.2740361018
Kliem, K. E., Humphries, D. J., Kirton, P., Givens, D. I., and Reynolds, C. K. (2019). Differential effects of oilseed supplements on methane production and milk fatty acid concentrations in dairy cows. Animal 13, 309–317. doi: 10.1017/S1751731118001398
Liu, E., VandeHaar, M. J., and Lock, A. L. (2020). Effects of supplementing Holstein cows with soybean oil compared with palmitic acid-enriched triglycerides on milk production and nutrient partitioning. J. Dairy Sci. 103, 8151–8160. doi: 10.3168/jds.2019-18100
Lock, A. L., and de Souza, J. (2016). “Fatty acid digestibility and impacts on responses of dairy cows,” in Annual Florida Ruminant Nutrition Symposium, 78–89.
Macedo, F. L., Batistel, F., de Souza, J., Chagas, L. J., and Santos, F. A. (2016). Supplementation with Ca salts of soybean oil interacts with concentrate level in grazing dairy cows: intake, ingestive behavior, and ruminal parameters. Trop. Anim. Health Prod. 48, 1593–1598. doi: 10.1007/s11250-016-1132-4
Mariotti, F., and Gardner, C. D. (2019). Dietary protein and amino acids in vegetarian diets-a review. Nutrients 11, 1–19. doi: 10.3390/nu11112661
Murphy, D. J., Goggin, K., and Paterson, R. R. M. (2021). Oil palm in the 2020s and beyond: challenges and solutions. CABI Agric. Biosc. 2, 1–39. doi: 10.1186/s43170-021-00058-3
Myers, W. D., Ludden, P. A., Nayigihugu, V., and Hess, B. W. (2004). Technical note: a procedure for the preparation and quantitative analysis of samples for titanium dioxide. J. Anim. Sci. 82, 179–183. doi: 10.2527/2004.821179x
NASEM (2021). Nutrient Requirements of Dairy Cattle: Eighth Revised Edition. Washington, DC: The National Academies Press.
Nichols, K., Bannink, A., Pacheco, S., van Valenberg, H. J., Dijkstra, J., and van Laar, H. (2018). Feed and nitrogen efficiency are affected differently but milk lactose production is stimulated equally when isoenergetic protein and fat is supplemented in lactating dairy cow diets. J. Dairy Sci. 101, 7857–7870. doi: 10.3168/jds.2017-14276
NRC (2001). Nutrient Requirements of Dairy Cattle. 7th rev. ed. Washington, DC: National Academic Press.
Palmquist, D. L., and Harvatine, K. J. (2020). “Origin of fatty acids and influence of nutritional factors on milk fat,” in Advanced Dairy Chemistry, Volume 2: Lipids, eds. P. L. H. McSweeney, P. F. Fox and J. A. O'Mahony. Cham: Springer International Publishing, 33–66. doi: 10.1007/978-3-030-48686-0_2
Palmquist, D. L., and Jenkins, T. C. (2017). A 100-year review: fat feeding of dairy cows. J. Dairy Sci. 100, 10061–10077. doi: 10.3168/jds.2017-12924
Patel, M., Sonesson, U., and Hessle, A. (2017). Upgrading plant amino acids through cattle to improve the nutritional value for humans: effects of different production systems. Animal 11, 519–528. doi: 10.1017/S1751731116001610
Perkins, E. G. (1995). Chapter 2 - Composition of Soybeans and Soybean Products, In Practical Handbook of Soybean Processing and Utilization, ed. D.R. Erickson. AOCS Press, 9-28. doi: 10.1016/B978-0-935315-63-9.50006-1
Pimpa, O., Liang, J. B., Jelan, Z. A., and Abdullah, N. (2001). Urinary excretion of duodenal purine derivatives in Kedah-Kelantan cattle. Anim. Feed Sci.Technol. 92, 203–214. doi: 10.1016/S0377-8401(01)00259-0
Rico, J. E., de Souza, J., Allen, M. S., and Lock, A. L. (2017). Nutrient digestibility and milk production responses to increasing levels of palmitic acid supplementation vary in cows receiving diets with or without whole cottonseed. J. Anim. Sci. 95, 436–446. doi: 10.2527/jas.2016.1089
Ritchie, H., Reay, D. S., and Higgins, P. (2018). Beyond calories: a holistic assessment of the global food system. Front. Sustain. Food Syst. 2, 1–12. doi: 10.3389/fsufs.2018.00057
Rius, A. G., Appuhamy, J. A. D. R.N., Cyriac, J., Kirovski, D., Becvar, O., Escobar, J., et al. (2010). Regulation of protein synthesis in mammary glands of lactating dairy cows by starch and amino acids. J. Dairy Sci. 93, 3114–3127. doi: 10.3168/jds.2009-2743
Roche, J. R., Kay, J. K., Rius, A. G., Grala, T. M., Sheahan, A. J., White, H. M., et al. (2013). Short communication: Immediate and deferred milk production responses to concentrate supplements in cows grazing fresh pasture. J. Dairy Sci. 96, 2544–2550. doi: 10.3168/jds.2012-4626
Santos, F.A.P., Dorea, J.R.R., de Souza, J., Batistel, F., and Costa, D.F. (2014). “Forage management and methods to improve nutrient intake in grazing cattle,” in 25th Annual Florida Ruminant Nutrition Symposium, 144–164.
Schader, C., Muller, A., Scialabba, N. E.-H., Hecht, J., Isensee, A., Erb, K.-H., et al. (2015). Impacts of feeding less food-competing feedstuffs to livestock on global food system sustainability. J. R. Soc. Interface 12:20150891. doi: 10.1098/rsif.2015.0891
Shetty, N., Difford, G., Lassen, J., Lovendahl, P., and Buitenhuis, A. J. (2017). Predicting methane emissions of lactating Danish Holstein cows using Fourier transform mid-infrared spectroscopy of milk. J. Dairy Sci. 100, 9052–9060. doi: 10.3168/jds.2017-13014
Storry, J. E., Hall, A. J., and Johnson, V. W. (1973). The effects of increasing amounts of dietary tallow on milk-fat secretion in the cow. J. Dairy Res. 40, 293–299. doi: 10.1017/S002202990001462X
Sukhija, P. S., and Palmquist, D. L. (1990). Dissociation of calcium soaps of long-chain fatty acids in rumen fluid1. J. Dairy Sci. 73, 1784–1787. doi: 10.3168/jds.S0022-0302(90)78858-3
Takiya, C. S., Ylioja, C. M., Bennett, A., Davidson, M. J., Sudbeck, M., Wickersham, T. A., et al. (2019). Feeding dairy cows with “leftovers” and the variation in recovery of human-edible nutrients in milk. Front. Sustain. Food Syst. 3, 1–16. doi: 10.3389/fsufs.2019.00114
Teng, S., Khong, K. W., and Che Ha, N. (2020). Palm oil and its environmental impacts: a big data analytics study. J. Clean. Prod. 274:122901. doi: 10.1016/j.jclepro.2020.122901
Van Soest, P. J., Robertson, J. B., and Lewis, B. A. (1991). Methods for dietary fiber, neutral detergent fiber, and nonstarch polysaccharides in relation to animal nutrition. J. Dairy Sci. 74, 3583–3597. doi: 10.3168/jds.S0022-0302(91)78551-2
Vega Mejía, N., Ponce Reyes, R., Martinez, Y., Carrasco, O., and Cerritos, R. (2018). Implications of the western diet for agricultural production, health and climate change. Front. Sustain. Food Syst. 2:88. doi: 10.3389/fsufs.2018.00088
Voltolini, T. V., Santos, F. A. P., Martinez, J. C. R.L C, Penati,I, M. A., et al. (2010). Productive and qualitative characteristics of elephant grass pasture grazed in fixed and intermittent intervals according to interception of active photosynthetic radiation. Rev. Bras. de Zootec. 39, 1002–1010. doi: 10.1590/S1516-35982010000500009
Wilkinson, J. M. (2011). Re-defining efficiency of feed use by livestock. Animal 5, 1014–1022. doi: 10.1017/S175173111100005X
Wilkinson, J. M., and Lee, M. R. F. (2018). Review: Use of human-edible animal feeds by ruminant livestock. Animal 5, 1014–1022. doi: 10.1017/S175173111700218X
Keywords: fat supplementation, nitrogen excretion, rumen fermentation, fatty acid profile, sustainability
Citation: de Souza J, Batistel F and Santos FAP (2023) Enhancing the recovery of human-edible nutrients in milk and nitrogen efficiency throughout the lactation cycle by feeding fatty acid supplements. Front. Sustain. Food Syst. 7:1186454. doi: 10.3389/fsufs.2023.1186454
Received: 14 March 2023; Accepted: 22 May 2023;
Published: 23 June 2023.
Edited by:
Reza Rastmanesh, Independent Researcher, Tehran, IranReviewed by:
Hani M. El-Zaiat, Sultan Qaboos University, OmanJuan J. Loor, University of Illinois at Urbana-Champaign, United States
Copyright © 2023 de Souza, Batistel and Santos. This is an open-access article distributed under the terms of the Creative Commons Attribution License (CC BY). The use, distribution or reproduction in other forums is permitted, provided the original author(s) and the copyright owner(s) are credited and that the original publication in this journal is cited, in accordance with accepted academic practice. No use, distribution or reproduction is permitted which does not comply with these terms.
*Correspondence: Flávio Augusto Portela Santos, ZmFwc2FudG9zQHVzcC5icg==; Fernanda Batistel, ZmVybmFuZGFiYXRpc3RlbEB1ZmwuZWR1