- College of Life Science, Yangtze University, Jingzhou, Hubei, China
In this study, alkali-soluble polysaccharide from wampee (ASP-W) was prepared and its effect on chitosan-based composite film was investigated. Preliminary characterization of ASP-W showed it was a kind of typical homogeneous polysaccharide, with a molecular weight of 1.5 × 103 KDa. Infrared spectroscopy proved the successful combination of ASP-W with chitosan. Meanwhile, composite film exhibited increased thickness, water solubility percentage, and swelling ratio and decreased moisture content. In terms of optical transmittance, composite films showed excellent UV barrier capability below 400 nm. Mechanical tests demonstrated tensile strength up to 24.84 MPa at ASP-W 1.5% and elongation at break up to 27.93% at ASP-W 4.5%. Composite films also possessed better barrier properties. Differential scanning calorimeter (DSC) results also indicated better thermostability of composite films. DPPH radical scavenging reached 46.84% at ASP-W 1.5% and hydroxyl radical scavenging peaked at 97.16% at ASP-W 3%. In summary, ASP-W can improve optical, mechanical, barrier, and thermal stability and antioxidant properties of chitosan film, which provided a certain reference for the development and utilization of polysaccharide in edible packaging film.
1. Introduction
Edible film is a kind of porous and compact film with specific mechanical properties and selective permeability, which takes natural polymers as the main base material and mixes it with edible plasticizer (Galus and Kadzińska, 2015; Nešić et al., 2019). Generally, edible film is a packaging film consisting of a layer of edible material in the form of wrapping, coating, impregnating, or spraying that covers the internal interface of the food, which can isolate air, prevent the loss of moisture and nutrients, etc., and avoid lipid oxidation and microbial decomposition during transportation and preservation, thus ensuring the safety of the food (Tavassoli-Kafrani et al., 2016). Based on matrix, edible film can be classified as lipid edible films, protein edible films, polysaccharide edible films, and composite edible films. Compared with the traditional plastic packaging film, edible film has the advantages of harmlessness, biodegradability, reproducibility, and having a barrier and water barrier (Zareie et al., 2020; Kumar et al., 2021). Compared to single films, composite films contain a variety of material components that complement each other’s functions, maintaining the advantages of each film-forming substrate and making up for the shortcomings of a single edible film. At the same time, composite edible films have other excellent properties, such as preventing the growth of microorganisms and improving food quality (Wang et al., 2020).
Polysaccharides are naturally occurring macromolecular polymers widely distributed in plants, animals, algae, and microorganisms, formed by the polymerization of more than 10 monosaccharides through alpha- or beta-glycosidic bonds. Polysaccharide is not only the structural material of plant cells, but also the source of energy (Huang et al., 2021). Plant polysaccharides have increasingly attracted attention because of their extensive pharmacological activities, such as anti-tumor, immunomodulation, antioxidant, and anti-inflammatory effects (Cho et al., 2015). Polysaccharides are hydrophilic, stable, safe, nontoxic, and naturally biodegradable, and some of them are widely used in food packaging, pharmaceutical preparations, and various sustainable and renewable commodities in the biomedical industry (Tudu and Samanta, 2022). Studies have shown that, compared with other polysaccharides, alkaline soluble polysaccharides have more potential. The alkali-soluble polysaccharide of dendrobium candida has a proliferation effect on lactic acid bacteria (Xing et al., 2021). Alkaline and ultrasound-assisted alkaline extraction were used to obtain polysaccharide from papaya, and it was found that the alkali-soluble papaya polysaccharide exhibited higher water-holding, oil-holding, and swelling capacities (Zhang et al., 2017). Recently, polysaccharides have been used as one of the sustainable materials in coatings and edible film.
Chitosan, also known as deacetylated chitin, is a natural polymer made from chitin by deN-acetylation (≥55%) under alkaline conditions (Negm et al., 2020; Xie et al., 2022). It is the second most alkaline polysaccharide in nature after cellulose, and has good film formation, biocompatibility, biodegradability, and other excellent properties (Martínez-Camacho et al., 2010). As a result, chitosan can be used in the development of edible film and is gaining attention in the food packaging sector. Although chitosan film has certain film-forming properties, tensile properties, and antibacterial properties, single chitosan edible film has not been widely used because of some defects in properties, such as weak mechanical strength, poor oxygen permeability, light transmittance, moisture resistance, and affecting the preservation effect of perishable food (Ferreira et al., 2016). It is often compounded with other materials to make up for its defects (Jamróz and Kopel, 2020; Mohammadi Sadati et al., 2022). Proteins and polysaccharides have some excellent film-forming characteristics, so they are widely used as film-forming substrates (Ren et al., 2017). Previous research suggested that chitosan had good compatibility with some polysaccharides, and the preparation of films by mixing chitosan with these polysaccharides could improve the properties of blended films and reduce the production cost of enterprises (Garcia et al., 2006; Fonseca-García et al., 2021).
Clausena lansium (Lour.) Skeels, also called wampee, belongs to Rutaceae and is mainly distributed in the Guangxi, Yunnan, and Guangdong provinces of China and southeast Asian countries (Shen et al., 2012; Song et al., 2022). Wampee can be eaten raw when ripe, or processed into sauces, preserves, and drinks; it is known as the “treasure in fruit.” The chemical constituents of wampee have been reported as polysaccharide, alkaloids, terpenoids, vitamins, organic acids, and coumarins (Wu et al., 2013; Fu et al., 2022; Song et al., 2022), which can treat coughs and relieve pain, and have anti-inflammatory, antioxidant, and anti-cancer properties, among other things (Liu et al., 2012; Shen et al., 2018). Domestic and international research on wampee bark has focused on chemical composition, antioxidant properties, and other aspects (Liu et al., 2012; Chang et al., 2018; Shen et al., 2018). Previous studies also investigated the properties of water-soluble wampee polysaccharides (Shen et al., 2018). More and more attention has been paid to the extraction and utilization of natural active compounds from by-products of food processing (Castro-Muñoz et al., 2016). Many domestic and international scholars have studied chitosan and protein substances, lipids, and polysaccharide composite film, clearly pointing out that the addition of these substances can improve the disadvantages of chitosan membranes, especially polysaccharides which have many favorable properties, such as good antioxidant and barrier properties (Bakshi et al., 2020). However, the use of alkali-soluble polysaccharide from wampee (ASP-W) for the production of edible films has rarely been reported. In this paper, alkali-soluble polysaccharide from wampee was extracted from wampee residue and chitosan was used as the matrix to prepare a composite film with ASP-W. The effects of different additions of ASP-W on the structure, mechanical properties, barrier properties, thermostability, and antioxidant properties of composite film were studied, in order to expand the development and application of ASP-W and broaden the research ideas of plant polysaccharides in the packaging industry.
2. Materials and methods
2.1. Experimental material and reagents
Wampee was obtained from the Jiaxiangwei Store in Maoming of Guangdong, China. Uniform fruits (length: 30 ± 5 mm, weight: 10 ± 1 g, maturity: ripe) with no diseases were used in experiments. Chitosan (deacetylation degree: 90%) was supplied by Shanghai yuanye Bio-Technology Co., Ltd. (Shanghai, China). Potassium bromide was of spectral purity. All other chemicals and reagents were of analytical grade.
2.2. Extraction of alkali-soluble polysaccharide from wampee
ASP-W was extracted from wampee according to a previously reported method with slight modifications (Chen et al., 2019). To begin, 500 g of wampee flesh was pulped in a juicer and absolute ethanol (solid–liquid ratio of 1:4, w: v) was added to degrease with stirring for 24 h. After centrifugation at 11,100 g for 15 min, the precipitate was collected and dried at 40°C. To adequately remove water-soluble polysaccharide from wampee flesh, the dried granules were extracted with distilled water at 80°C three times (each for 2 h) and the combined supernatants through centrifugation were discarded. Then the residue was extracted by 0.5 mol/L NaOH solution (solid–liquid ratio of 1:4, w: v) at 50°C three times (each for 2 h). Similarly, the supernatant was combined and its pH value was adjusted to 7.0. Afterwards, the supernatant was concentrated by rotary evaporator and then precipitated four times of absolute ethanol overnight at 4°C. After centrifugation for 15 min at 11,100 g several times, the precipitate was re-dissolved in water and dialyzed (Mw cut off 100,000 Da; Width: 77 cm) against distilled water for 72 h. Finally, the alkali-soluble polysaccharide from wampee (ASP-W) was obtained by lyophilization.
2.3. Chemical composition analysis of ASP-W
The total sugar content was determined by the phenol-sulfuric acid method, and the reducing sugar content was determined by 3,5-dinitrosalicylic acid (DNS) method (Huang and Huang, 2020; Zhao et al., 2020). The polysaccharide content was equal to the former minus the latter (Abou Zeid et al., 2014; Wu et al., 2020). The content of soluble protein was determined by Coomassie brilliant blue G-250 method (Bao et al., 2016).
2.4. Characterization of ASP-W
2.4.1. Determination of molecular weight distribution
The molecular weight distribution of ASP-W was conducted according to the method reported (Wu et al., 2020). A certain amount of polysaccharide (2.0 mg) was dissolved in ultrapure water (1.0 mL) and measured by Aligent 1,200 (Waters, America) high performance liquid chromatograph matched with TSK-GEL G4000PWxl chromatographic column. The mobile phase was ultrapure water and its flow rate was 0.6 mL/min. The temperature of the column and differential refraction index detector (RID) was 30°C and 35°C, respectively.
2.4.2. Ultraviolet and visible (UV–vis) spectra
Polysaccharide was dissolved in distilled water with a final concentration of 0.02 mg/mL and scanned from 200 to 400 nm by ultra-violet-visible spectrophotometer (UV-1800, China), detecting whether it contains impurities such as protein and nucleic acid.
2.4.3. Fourier transform infrared spectroscopy
FT-IR of the polysaccharide was studied by BRUKER FTIR-VECTOR-22. Polysaccharide (1 mg) and dry KBr (150 mg) were fully ground and pressed into a thin disk for infrared spectrometer testing with scanning range of 4,000 ~ 400 cm−1.
2.5. Preparation of ASP-W/chitosan composite film
ASP-W/chitosan composite film were prepared in accordance with Ren et al. (2017) with slight modifications. Chitosan powder (1 g) was dissolved in 100 mL of acetic acid solution (0.35 mol/L) to prepare 1% (w/v) chitosan solution. Then ASP-W (0.0, 1.5, 3.0, 4.5, and 6%) and 30% (w/w) of glycerin were added into the solution. After the solution was evenly stirred with 600 rpm for 60 min at 60°C and degassed, it was cast on a PP board and dried at 30°C and 53% relative humidity for 24 h. Finally, the film was uncovered and balanced in a constant humidity dryer for 24 h, then all indexes were determined.
2.6. Fourier transform infrared spectroscopy of composite film
The composite film (1 mg) and dry KBr (150 mg) were fully ground and pressed into a thin disk for infrared spectrometer testing with scanning range of 4,000 ~ 400 cm−1.
2.7. Film thickness, moisture content, water solubility percentage, and swelling ratio
Film thickness was measured by a thickness gauge by randomly selecting different positions on the film and taking an average. The moisture content of the composite film was measured using the method described by Arifin et al. (2022). The composite film was dried at 105°C in an oven to a constant weight, and moisture content was calculated by the mass difference of film before and after drying.
The water solubility percentage and swelling ratio of the composite film was measured using the method described by Sun et al. (2017). To determine water solubility percentage, the composite film with initial mass M0, was soaked in distilled water for 24 h. Then the remaining water on the film was absorbed with filter paper before drying at 60°C for 24 h, and its mass was weighed as M1. Water solubility percentage was calculated by the mass difference of film before (M0) and after drying (M1). To determine swelling ratio, the film was first soaked in NaCl solution (0.1 mol/L) for 30 min. Then the water on its surface was wiped off with filter paper and its weight was marked as M2. Swelling ratio of composite film was calculated by the mass difference of M0 and M2.
2.8. Optical transmittance
The composite film was cut into strips and adhered to the inside of the cuvette glossy surface. The ultraviolet spectrophotometer was used to scan continuously from 200 nm to 800 nm, and the optical transmittance was recorded.
2.9. Mechanical properties of composite film
The mechanical properties were based on the methods described by Li et al. (2020). The mechanical properties of composite film including tensile strength (TS) and elongation at break (EAB) were measured by a texture analyzer-TMS-PRO (Food Technology Corporation (FTC), United States). The film was cut into 1.5 cm × 6.0 cm strips and fixed on the device. Then the strip was vertically stretched and shook slowly (with a displacement speed of 0.5 mm/s). When the film was broken, the test was stopped. TS and EAB was calculated by equations (1) and (2).
Where TS (MPa) was the tensile strength of film, F(N) was the maximum force of film when broken, and b and d was the width (mm) and thickness (mm) respectively.
Where EAB was the elongation at break of film, l0 was the length of the film before test, and l1 was the length of film when broken.
2.10. Water vapor and oil permeability of composite film
Water vapor permeability (WVP) was based on the method described by Peng and Li (2014) with some modifications. Anhydrous calcium chloride was put in a weighing bottle that was sealed by composite film. Then the bottle was placed in a desiccator with saturated sodium chloride solution at the bottom. The weight of the whole bottle was recorded every 24 h and continuously for 72 h. Water vapor permeability (WVP) was calculated according to the following formula (3):
Where A was the area for fixing the film (m2), Δt was the time variation (s), d was the film thickness (mm), and Δp was the partial pressure difference of vapor between film (KPa).
The oil permeability was measured in accordance with Liu et al. (2015). 5 mL of blend oil was added into the test tube which was sealed by composite film. Then it was placed on a piece of filter paper at 25°C and the mass of the whole test tube was measured after 48 h. Oil permeability (PO) was calculated according to the following formula (4):
where ΔW was the mass change of test tube (g), d was the thickness of film (mm), S was the area for fixing the film (m2), and T was the test time (d).
2.11. Differential scanning calorimeter of composite film
Differential scanning calorimeter (DSC) was measured in accordance with Mittal et al. (2021) with some modifications. The composite film was accurately weighed, sealed in an aluminum pan, and analyzed by differential scanning calorimeter from 20°C to 200°C at a heating rate of 20°C/min. Pure nitrogen at 20 mL/min were used for cooling and purge medium. The melting point (Teo) and maximum transition temperature (Tmax) of composite film was recorded in the thermogram and the denaturation enthalpy (H) was determined by measuring the area of the corresponding endothermic peak.
2.12. Antioxidant activity of composite film
2.12.1. DPPH radical-scavenging activity
DPPH was determined according to the method of Gao et al. (2019). The composite film (0.2 g) was completely dissolved in 6 mL 0.5% acetic acid and then the solution (0.8 mL) was mixed with 4.0 mL of DPPH-ethanol solution (0.1 mmol/L). The absorbance at 517 nm was measured after the solution was reacted in the dark for 30 min. The absorbance was set to zero with ethanol, and DPPH-ethanol solution without samples was used as control.
2.12.2. Hydroxyl radical-scavenging activity
The composite film (0.25 g) was completely dissolved in 7.5 mL 0.5% acetic acid and then the solution (1 mL) was mixed with 1 mL of 9 mmol/L FeSO4 solution, 1 mL of 9 mmol/L salicylic acid-ethanol solution, and 1 mL of 8.8 mmol/L H2O2 solution to react in water bath at 37°C for 30 min. The absorbance at 510 nm was measured, and mixed solution without samples was used as control.
2.13. Statistical analysis
Data were expressed as means ± standard deviation (SD) obtained from triplicate experiments and analyzed by SPSS version 13.0 for Windows.
3. Results and discussion
3.1. Preliminary characterization of ASP-W
In the present study, ASP-W was obtained from wampee by alkali extraction and its yield was 5.39%. The contents of protein, polysaccharide, and reducing sugar were 6.27, 70.79, and 1.53%, respectively. Figure 1A shows the HPGPC chromatogram of ASP-W, and it can be seen that ASP-W had only a single symmetrical peak, demonstrating it was a kind of homogeneous polysaccharide. Based on the calibration with standard dextrans, the estimated average molecular weight of ASP-W was 1.5 × 103 KDa. UV–vis spectra of ASP-W in Figure 1B shows that there were no absorption peaks at 260 nm and 280 nm, which proved the absence of protein or nucleic acid in ASP-W.
Infrared spectrum of ASP-W is depicted in Figure 2. The bands at 3407.26 cm−1 and 2931.14 cm−1 were associated with O-H and C-H stretching (Zhou et al., 2021), while the two bands at 1636.34 cm−1 and 1419.85 cm−1 were the characteristic absorption peak of C=O bending and vibration of C-H bending, respectively (Zhao et al., 2022). The above four peaks were the characteristic absorption of polysaccharide. The absorption peak at 1320.93 cm−1 was caused by symmetric and asymmetric C-H bending (Gao et al., 2019). The band at 1260.32 cm−1 may be caused by the stretching vibration of C=O. The two peaks at 1078.05 cm−1 and 1047.26 cm−1 indicated the existence of pyranose. The weak band at 898.76 cm−1 was ascribed to β-glucosidic bond, suggesting that β-configurations existed in ASP-W (Fonseca-García et al., 2021).
3.2. FT-IR of ASP-W/chitosan composite film
FT-IR is a useful tool for detecting the possible interactions between chitosan and ASP-W. The effect of ASP-W on the infrared spectrum of composite film is exhibited in Figure 2. The composite film had a strong broad absorption peak at around 3,500 cm−1, which was due to the stretching of -OH and-NH2 (Huang and Huang, 2020). It was found that the red-shift of composite film was more obvious than the control group. The frequency of absorption peaks varied greatly due to the difference of strength and bond length of hydrogen bond (Augusto et al., 2018). The peak at 2933 cm−1 was attributed to the C–H stretching vibration of polysaccharides. Compared with chitosan film, the wavenumber of composite film had little change. This was because chitosan groups also contained many C-H bonds. The peaks at 1650 ~ 1,660 cm−1 were assigned to the contraction vibration of C=O and the angular vibration of O-H in the ASP-W (Zhao et al., 2022). The maximum absorption peak at 1550 ~ 1,560 cm−1 was N-H deformation amplitude. At about 1,421 cm−1, it corresponded to the strong C-O and C-N stretching (Gao et al., 2019). Compared with chitosan film, slight shift and strong absorption in composite films were observed at this position, which indicated that ASP-W and chitosan had a close connection. Pinzon et al. (2018) pointed out that the hydrogen bond formed between chitosan and polysaccharides was strong, which could reduce the original chemical bond constant between polysaccharides and lead to the change of absorption frequency. At 1160 cm−1, the absorption peak was the result of C-C stretching vibration bending and C-O-C stretching vibration of ASP-W (Peng et al., 2022). The above results showed that there was strong interaction between ASP-W and chitosan.
3.3. Film thickness, moisture content, water solubility percentage, and swelling ratio
Table 1 summarized the thickness, moisture content, water solubility percentage, and swelling ratio of composite film after the addition of ASP-W. The thickness of chitosan film was 28.25 μm, and when the addition of ASP-W was 4.5%, the film thickness reached 46.50 μm. The film thickness results were between chitosan/starch (Bof et al., 2015) and chitosan/pectin polysaccharide (Gao et al., 2019). However, this is contrary to the results of Li′s Bletilla polysaccharide/chitosan composite film (Li, 2021). It showed that the interaction between ASP-W and chitosan had an effect on the film thickness. It was probable that the existence of interaction between ASP-W and chitosan enhanced the network structure of chitosan molecular chains. The greater the addition of ASP-W, the greater the degree of compatibility between ASP-W/chitosan film, at the same time the ductility and flexibility and plasticity of the film were enhanced, which had an important influence network on improving the mechanical energy of the active film.
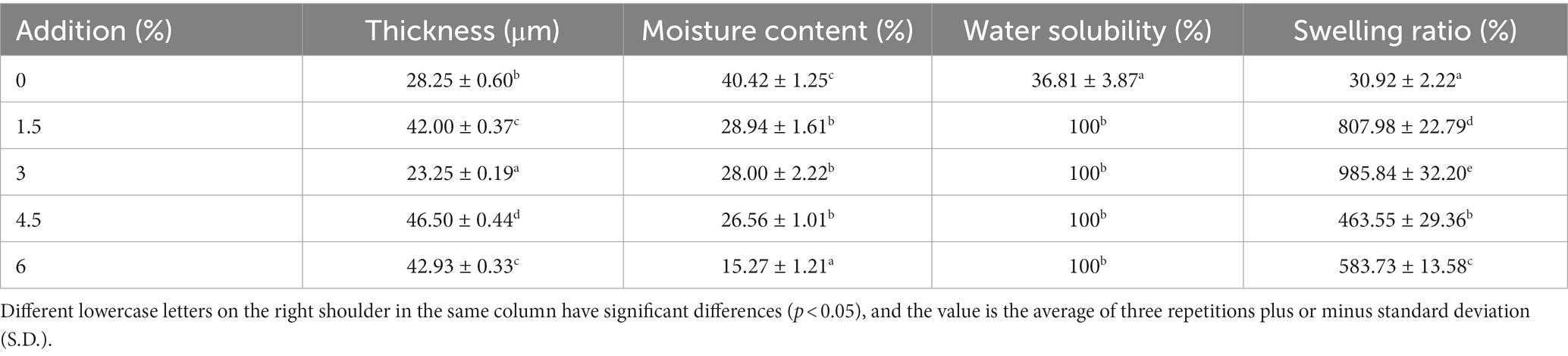
Table 1. Thickness, moisture content, water solubility percentage, and swelling ratio of composite film.
In addition, with the increasement of ASP-W addition, the moisture content of composite film gradually decreased. The moisture content of film with the addition of 6% ASP-W was the lowest (15.27%) and approximately two times lower than that of control. This phenomenon was probably due to the interaction of hydroxyl and carboxyl groups in ASP-W with the hydrophilic groups in chitosan, which in turn decreased the interaction between chitosan and water molecules (Khazaei et al., 2014).
In terms of water solubility percentage, it was 36.81% in control group while other sample groups all reached 100%. That is to say, when different amounts of ASP-W were added, composite films were completely dissolved in water. In the preservation of fruits and vegetables, in order to maintain the structural integrity, low water solubility percentage films were needed. However, in other applications, such as edible films for candy, films with high water solubility percentage can be selected. The addition of ASP-W increased the water solubility percentage of the composite film, and the water solubility percentage of ASP-W in water was very high. The increased water solubility percentage of the composite films was attributed to the interaction between the amino group in chitosan and the hydroxyl group of ASP-W. Also in this work, the addition of 30% glycerol (w/w) as a plasticizer for the composite film played an important role in weakening the interactions between the polymer molecular chains and increasing the free volume between the chains, and the addition of glycerol promoted the diffusion of water molecules into the film matrix, thereby increasing the water solubility percentage of the composite film, which confirmed the results in FTIR that intermolecular forces exist between ASP-W and chitosan (Fonseca-García et al., 2021). Similar to water solubility percentage, swelling ratio of composite films were significantly (p < 0.05) enhanced and modified after the addition of ASP-W compared with control group. The increased swelling ratio may be attributed to the interaction of the hydrophilic groups of the polysaccharides with water molecules, and the addition of ASP-W increased the ability of water to bind to the matrix and increased swelling, leading to increased water uptake by the polysaccharide molecules (Zhang et al., 2017).
3.4. Optical transmittance of ASP-W/chitosan composite film
The most harmful effect of light on food was caused by UV light, and the light transmission of the packaging film affected the rate of oxidation of lipids in food, which could lead to food spoilage. Optical transmittance of chitosan-based composite film is shown in Figure 3. It can be seen that in the range of visible light (400 ~ 800 nm), the transmittance of film increased with the increasement of wavelength, and addition of ASP-W had no obvious effect on transmittance except for the addition of 3%. In the ultraviolet wavelength range (200 ~ 400 nm), the addition of ASP-W led to the overall decrease of optical transmittance of composite film, which reached the minimum at addition of 3% ASP-W. Although the transmittance of 3% ~ 4.5% has an upward trend, it is still lower than that of chitosan film. The transmittance of the film was almost zero at 200 ~ 215 nm. On the one hand, ASP-W was slightly yellowish brown, which affected the transparency of the film. It was also related to the diffuse reflection of polysaccharides in the composite film. On the other hand, it might also be due to the high light absorption capacity of ASP-W, which could effectively resist the damage of ultraviolet rays to food or prevent the formation of free radicals, thus avoiding the oxidization and decomposition by light irradiation (Zhao et al., 2022). The above results indicated that ASP-W/chitosan film can potentially retard UV-induced lipid oxidation in food products, which is beneficial for food preservation.
3.5. Mechanical and barrier properties of ASP-W/chitosan composite film
The tensile strength of the composite film can reflect the size of the force that the composite film can withstand; the higher the tensile strength, the greater the force that the composite film can withstand. The elongation at break of the composite film can reflect the flexibility of the composite film; the higher the elongation at break, the better the flexibility of the composite film. Chitosan composition, film network microstructure, and intermolecular forces played an important role in the mechanical properties of chitosan film. The effect of ASP-W on mechanical properties (including tensile strength and elongation at break of composite film) is shown in Figures 4A,B. With the increase of ASP-W addition, the tensile strength firstly increased and then gradually decreased, which is similar to the result trend of Auricularia auricular polysaccharide/chitosan edible composite film (Qin et al., 2021).
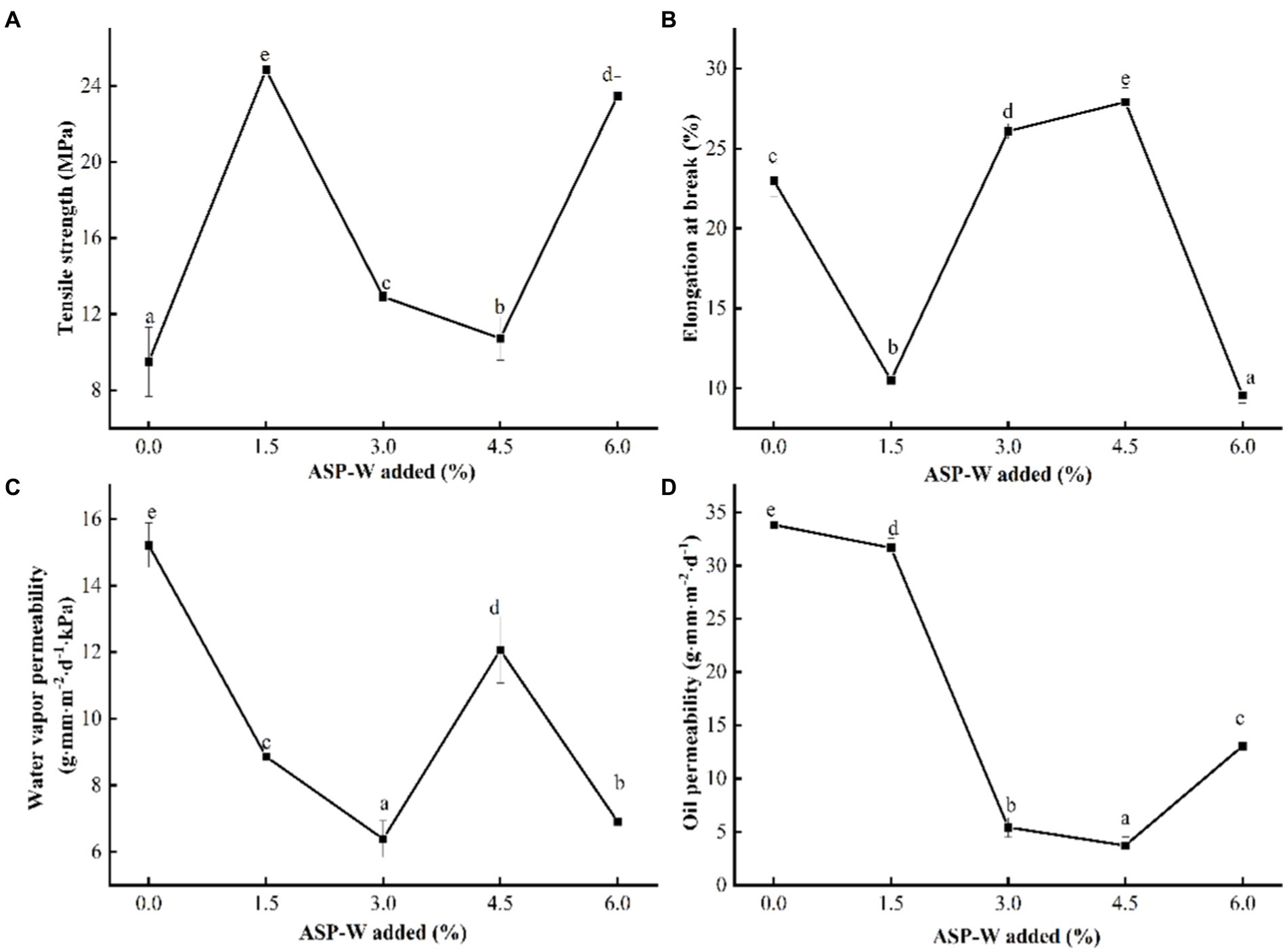
Figure 4. Effect of ASP-W on (A) tensile strength, (B) elongation at break, (C) water vapor permeability, and (D) oil permeability of composite film.
The tensile strength of film in control group was determined as 9.5 MPa; it was lower than the ASP-W/chitosan composite film and up to 24.84 MPa at ASP-W1.5%. On one hand, due to ASP-W being added, the number of molecules per unit volume increased. Consequently, free amino groups in chitosan had strong hydrogen bonds with hydroxyl groups in ASP-W, which improved the compactness of composite film, and further enhanced its tensile strength. In addition, glycerol acts as a plasticizer in ASP-W/chitosan films, which effectively reduced the cohesion between polymer chains and softened their structure (Sun et al., 2019). What is more, because ASP-W was added, the viscosity of the solution increased, which led to the deterioration of solution fluidity, the increase of tensile strength, and the decrease of elongation at break. When ASP-W was added continuously (1.5–4.5%), the elongation at break of composite film was enhanced and became firm because of excessive hydrogen bonds (Huang and Huang, 2020; Qin et al., 2021). The-NH2 in chitosan formed NH3+ in acetic acid, while a large number of hydroxyl groups existed in ASP-W, and the two easily formed hydrogen bonds, which made the structure of the composite film more continuous.
However, when the addition of ASP-W was 6%, the elongation at break became minimum. Elongation at break was up to 27.93% at ASP-W 4.5%. This might be because of the special structure of excessive ASP-W which improved the intramolecular or intermolecular hydrogen bonds, thus softening the rigid structure of composite film (Marangoni Júnior et al., 2021). The chitosan molecules and ASP-W may form hydrogen bonds within ASP-W rather than between chitosan molecules and ASP-W molecules. The number of NH3+ groups increased with the increase of chitosan concentration in the film-forming solution, and when the concentration of ASP-W exceeded the critical value, it is difficult to form a chitosan/ASP-W composite film with a uniform structure, which led to weak interactions at the boundaries and a decrease in tensile strength (Hopkins et al., 2015).
Figures 4C,D displayed the barrier properties of ASP-W/chitosan composite film. Water vapor permeability (WVP) was used to evaluate the capacity of edible film to allow water vapor to transfer from the surroundings to the packaged food (Sutharsan et al., 2022). It could be seen that WVP in control group was15.21 g·mm·m−2·d−1·kPa−1, higher than that of ASP-W addition groups and it reached the lowest 6.83 g·mm·m−2·d−1·kPa−1 at ASP-W 3%. As a whole, WVP firstly decreased and then increased. Water vapor permeability between composite film may be related to ASP-W. ASP-W contains more-OH, which had hydrogen bonds with the groups in chitosan molecules. Different to the binding degree with chitosan, this resulted in the change of water vapor permeability of composite film. With the addition of ASP-W, the number of free hydrophilic groups in the film-forming solution decreased. Therefore, the composite film was more tightly combined and the moisture resistance was improved. When the addition of ASP-W exceeded 3%, hydrogen bonds between polysaccharide and chitosan molecules were basically saturated. At this time, adding polysaccharide was equivalent to introducing a large number of hydrophilic groups, so the water resistance decreased. A similar phenomenon was also reported previously and it could be concluded that the more hydrophilic the composite film is might be due to the more hydroxyl groups in the polymer chain (Sivarajan et al., 2016).
In general, high oil permeability of edible films means poor oil resistance. With the addition of ASP-W, oil resistance of composite film firstly increased and then decreased. It could be seen that oil permeability in control group was 32.82 g·mm·m−2·d−1, higher than that of ASP-W addition groups. When the addition of ASP-W was 4.5%, oil permeability arrived at the lowest with 3.71 g·mm·m−2·d−1. With the addition of ASP-W, free groups form hydrogen bonds with hydroxyl groups in polysaccharide molecules, and the free oleophobic groups in edible films decrease, thus making the oil resistance of edible films worse. When the ASP-W continued to increase, the hydrogen bonds reached saturation, and the free oleophobic groups such as hydroxyl groups increased, thus enhancing the oil resistance.
3.6. DSC analysis of ASP-W/chitosan composite film
The thermostability of the composite film was analyzed by differential scanning calorimeter (DSC) and the interactions between chitosan and ASP-W was further investigated. It can be seen from Figure 5 that there was only one glass transition temperature (Tg) for the composite films. Tg of a single chitosan film was 100°C, while the Tg of 1.5, 3, 4.5, and 6% of composite film were all 110°C, which indicated that glycerol can play a plasticizing role. Films below Tg were hard and brittle, while films above Tg became flexible and bendable (Khazaei et al., 2014). The copolymers formed by chitosan and ASP-W had a tighter structure between them, but as the addition of ASP-W increased, they may form intra-molecular hydrogen bonds, making it difficult to form uniformly structured chitosan/ASP-W composite film again, and the low crystallinity of ASP-W made the dispersion easier as well as the disruption of the ordered structure, resulting in the Tg of the composite film being approximately the same, which was confirmed by the results of elongation at break and FT-IR.
A gradually broadening absorption peak was observed between 70°C and 100°C. The appearance of a peak was associated with the evaporation of free water, bound water, and acetic acid and the melting transition of the film (Ulrich and Faez, 2022), which appeared at higher temperatures in the composite film than the chitosan film. The results suggested that ASP-W improved the thermostability of composite film and confirmed the addition of ASP-W facilitated the interaction of chitosan with it. At around 160°C, the exothermic peaks appearing in the composite film belonged to the decomposition of glycosidic bonds. As the addition of ASP-W increased, the heat absorption peak gradually moved to a higher temperature, which indicated that the films of chitosan and ASP-W were partially crystalline, while the addition of ASP-W to chitosan generated intermolecular force and further emphasized the FTIR analysis results. Enthalpy H could reflect the stability of the film structure. The smaller the enthalpy, the more stable the composite film structure (Gong et al., 2020). Thermostability of the composite film was related to its inner structure and higher stability corresponded to higher thermal stability, as more energy (heat) was required to destroy the higher stable structure. It also confirmed that the thermostability of composite film was higher than that of single film.
3.7. Antioxidant activity of composite film
The effect of ASP-W on the antioxidant activity of composite film is shown in Figure 6. The scavenging ability on DPPH radicals of chitosan film was 24.09%. Compared with control, the ability of composite films to scavenge DPPH radicals significantly (p < 0.05) improved (Figure 6A), which was similar to a previous study (Qin et al., 2021). And when the addition of ASP-W was 6%, DPPH radical scavenging was the highest (48.49%). It was worth noting that the DPPH scavenging rate of composite films with different ASP-W addition were always higher than the control group. This phenomenon might be due to the strong hydrogen donating ability of hydroxyl groups in ASP-W, which prevented the reaction between free radicals, thus enhancing the free radical scavenging ability (Peng et al., 2022).
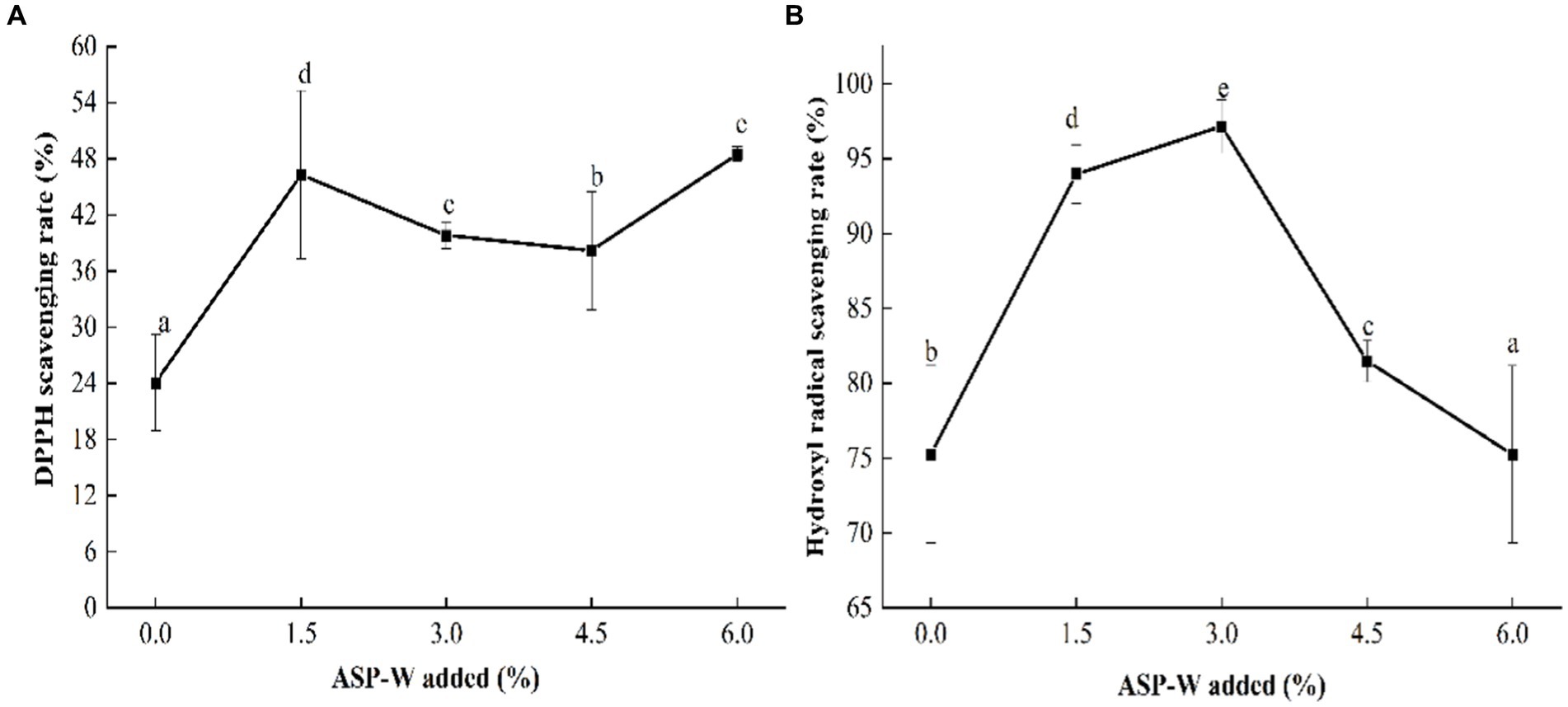
Figure 6. (A) DPPH scavenging rate and (B) hydroxyl radical scavenging rate of ASP-W/chitosan composite film.
With the addition of ASP-W, the scavenging ability on OH radicals of composite film increased firstly and then decreased, reaching the peak value of 97.16% at the addition of 3% (Figure 6B). Although the scavenging ability of composite film at the addition of 3% ~ 4.5% had a decreased trend, it was still better than chitosan film. This phenomenon suggested that the addition of ASP-W improved the antioxidant activity of composite film, which was due to the radical quenching ability of hydroxyl groups in the molecule of ASP-W (Kumar et al., 2021). There was free NH3+ in the molecular chain of chitosan, which can interact electrostatically with the-OH radicals in ASP-W and adsorb them, and can prevent the reaction between free radicals, thus improving the free radical scavenging ability. Once the amount of ASP-W was too much, its scavenging rate of hydroxyl radicals would be reduced.
4. Conclusion
ASP-W/chitosan films with different ASP-W additions of 0, 1.5, 3, 4.5, and 6% were prepared successfully by casting. The structural characteristics of ASP-W and its effects on the physical and chemical properties, mechanical and barrier properties, thermostability, and antioxidant activity of ASP-W/chitosan films were comprehensively studied. The structural characterization of ASP-W, which was a typical β-glucosidic bond compound containing pyranose ring, showed that ASP-W contained no impurities, such as nucleic acid and protein. FT-IR suggested that the interactions between the hydroxyl groups of ASP-W and the amino groups of chitosan could affect the thermostability of composite film. The incorporation of ASP-W led to an increase in film thickness (up to about 1.5 times of the single CS film), water solubility percentage (completely dissolved.), and swelling ratio (up to 30 times of the single CS film), and a decrease in moisture content. Compared with chitosan film, ASP-W strengthened the structure of composite film and resulted in a composite film with superior tensile strength, flexibility, and barrier properties, as well as improved optical properties and antioxidant activity. The study confirmed that ASP-W, a natural substance with promising activity, can interact with chitosan to prepare composite films with excellent properties and high antioxidant activity. The above results demonstrated that ASP-W/chitosan composite film could be a potential edible film for food and pharmaceutical packaging. However, it was still necessary to optimize the preparation process of ASP-W/chitosan composite film, to further improve the properties and to study the antibacterial ability against microorganisms and the shelf life of the packaged food.
Data availability statement
The original contributions presented in the study are included in the article/Supplementary material, further inquiries can be directed to the corresponding author.
Author contributions
P-PS conceived and designed research. J-LF and Y-YR conducted experiments and wrote the manuscript. R-ZG contributed new reagents and analytical tools. Z-LX analyzed data. All authors contributed to editing and finalizing the manuscript.
Funding
This work was financially supported by the College Students Innovation and Entrepreneurship Training Program of Yangtze University (Yz2022233).
Conflict of interest
The authors declare that the research was conducted in the absence of any commercial or financial relationships that could be construed as a potential conflict of interest.
Publisher’s note
All claims expressed in this article are solely those of the authors and do not necessarily represent those of their affiliated organizations, or those of the publisher, the editors and the reviewers. Any product that may be evaluated in this article, or claim that may be made by its manufacturer, is not guaranteed or endorsed by the publisher.
References
Abou Zeid, A., Aboutabl, E. A., Sleem, A., and El-Rafie, H. (2014). Water soluble polysaccharides extracted from Pterocladia capillacea and Dictyopteris membranacea and their biological activities. Carbohydr. Polym. 113, 62–66. doi: 10.1016/j.carbpol.2014.06.004
Arifin, H. R., Djali, M., Nurhadi, B., Azlin-Hasim, S., Masruchin, N., Vania, P. A., et al. (2022). Corn starch-based bionanocomposite film reinforced with ZnO nanoparticles and different types of plasticizers. Front. Sustain. Food Syst. 6:248. doi: 10.3389/fsuf-s.2022.886219
Augusto, A., Dias, J. R., Campos, M. J., Alves, N. M., Pedrosa, R., and Silva, S. F. (2018). Influence of Codium tomentosum extract in the properties of alginate and chitosan edible films. Foods 7:53. doi: 10.3390/foods7040053
Bakshi, P. S., Selvakumar, D., Kadirvelu, K., and Kumar, N. S. (2020). Chitosan as an environment friendly biomaterial–a review on recent modifications and appl-ications. Int. J. Biol. Macromol. 150, 1072–1083. doi: 10.1016/j.ijbiomac.2019.10.113
Bao, H., You, S., Cao, L., Zhou, R., Wang, Q., and Cui, S. W. (2016). Chemical and rheological properties of polysaccharides from fruit body of Auricularia auricula-judae. Food Hydrocoll. 57, 30–37. doi: 10.1016/j.foodhyd.2015.1-2.031
Bof, M. J., Bordagaray, V. C., Locaso, D. E., and García, M. A. (2015). Chitosan molecular weight effect on starch-composite film properties. Food Hydrocoll. 51, 281–294. doi: 10.1016/j.foodhyd.2015.05.018
Castro-Muñoz, R., Yáñez-Fernández, J., and Fíla, V. (2016). Phenolic compounds recovered from agro-food by-products using membrane technologies: an overview. Food Chem. 213, 753–762. doi: 10.1016/j.foodchem.2-016.07.030
Chang, X., Ye, Y., and Pan, J. (2018). Comparative assessment of phytochemical profiles and antioxidant activities in selected five varieties of wampee (Clausena lansium) fruits. Int. J. Food Sci. Technol. 53, 2680–2686. doi: 10.1111/ijfs.13877
Chen, H., Sun, J., Liu, J., Gou, Y., Zhang, X., Wu, X., et al. (2019). Structural characterization and anti-inflammatory activity of alkali-soluble polysaccharides from purple sweet potato. Int. J. Biol. Macromol. 131, 484–494. doi: 10.1016/j.ijbiomac.2019.03.126
Cho, C. W., Han, C. J., Rhee, Y. K., Lee, Y. C., Shin, K. S., Shin, J. S., et al. (2015). Cheonggukjang polysaccharides enhance immune activities and prevent cyclophosphamide-induced immunosuppression. Int. J. Biol. Macromol. 72, 519–525. doi: 10.1016/j.ijbiomac.2014.09.010
Ferreira, A. R., Alves, V. D., and Coelhoso, I. M. (2016). Polysaccharide-based membranes in food packaging applications. Membranes 6:22. doi: 10.3390/membranes6020022
Fonseca-García, A., Jiménez-Regalado, E. J., and Aguirre-Loredo, R. Y. (2021). Preparation of a novel biodegradable packaging film based on corn starch-chitosan and poloxamers. Carbohydr. Polym. 251:117009. doi: 10.1016/j.c-arbpol.2020.117009
Fu, W., Liao, X., Zhang, Q., Zhu, Y., Mei, S., Li, Q., et al. (2022). Anti-melanogenesis effect from wampee fruit pectin via α-MSH/TRY pathway in A375 cells. BMC Complement. Med. Ther. 22, 1–13. doi: 10.1186/s1-2906-022-03646-6
Galus, S., and Kadzińska, J. (2015). Food applications of emulsion-based edible films and coatings. Trends Food Sci. Technol. 45, 273–283. doi: 10.1016/j.tifs.2015.07.011
Gao, H. X., He, Z., Sun, Q., He, Q., and Zeng, W. C. (2019). A functional polysaccharide film forming by pectin, chitosan, and tea polyphenols. Carbohydr. Polym. 215, 1–7. doi: 10.1016/j.carbpol.2019.03.029
Garcia, M. A., Pinotti, A., and Zaritzky, N. E. (2006). Physicochemical, water vapor barrier and mechanical properties of corn starch and chitosan composite films. Starch Stärke 58, 453–463. doi: 10.1002/star.200500484
Gong, G. Z., Di, F., and Jing, X. H. (2020). Preparation and properties of citrus peel cellulose/polyphosphoric acid ammonium/melamine/polyvinyl alcohol composite films. Plast. Ind. 48, 172–177. doi: 10.3969/j.issn.1005–5770.2020.06.034
Hopkins, E. J., Chang, C., Lam, R. S., and Nickerson, M. T. (2015). Effects of flaxseed oil concentration on the performance of a soy protein isolate-based emulsion-type film. Food Res. Int. 67, 418–425. doi: 10.1016/j.foodr-es.2014.11.040
Huang, G., Chen, F., Yang, W., and Huang, H. (2021). Preparation, deproteinization and comparison of bioactive polysaccharides. Trends Food Sci. Technol. 109, 564–568. doi: 10.1016/j.tifs.2021.01.038
Huang, H., and Huang, G. (2020). Extraction, separation, modification, structural characterization, and antioxidant activity of plant polysaccharides. Chem. Biol. Drug Des. 96, 1209–1222. doi: 10.1111/cbdd.13794
Jamróz, E., and Kopel, P. (2020). Polysaccharide and protein films with antimicrobial/ant-ioxidant activity in the food industry: a review. Polymers 12:1289. doi: 10.3390/polym12061289
Khazaei, N., Esmaiili, M., Djomeh, Z. E., Ghasemlou, M., and Jouki, M. (2014). Chara-cterization of new biodegradable edible film made from basil seed (Ocimum basilicum L.) gum. Carbohydr. Polym. 102, 199–206. doi: 10.1016/j.carbpol.2013.10.062
Kumar, N., Ojha, A., Upadhyay, A., Singh, R., and Kumar, S. (2021). Effect of active chitosan-pullulan composite edible coating enrich with pomegranate peel extract on the storage quality of green bell pepper. LWT 138:110435. doi: 10.1016/j.lwt.2020.110435
Li, Y. (2021). Study on preparation and properties of Bletilla polysaccharide/chitosan composite film and double-layer film. Southwest Minzu University, Chendu, China.
Li, S., Yi, J., Yu, X., Wang, Z., and Wang, L. (2020). Preparation and characterization of pullulan derivative/chitosan composite film for potential antimicrobial applications. Int. J. Biol. Macromol. 148, 258–264. doi: 10.1016/j.ijbiomac.2020.01.080
Liu, Y. H., Jiao, Y., and Li, M. T. (2015). Study on the properties of polysaccharide/flavone-chitosan/sodium alginate preservative film. North. Hortic. 20, 117–121. doi: 10.11937/bfyy.201520029
Liu, H., Li, C. J., Yang, J. Z., Ning, N., Si, Y. K., Li, L., et al. (2012). Carbazole alkaloids from the stems of Clausena lansium. J. Nat. Prod. 75, 677–682. doi: 10.1021/np200919a
Marangoni Júnior, L., Rodrigues, P. R., Da Silva, R. G., Vieira, R. P., and Alves, R. M. V. (2021). Sustainable packaging films composed of sodium alginate and hydrolyzed collagen: preparation and characterization. Food Bioprocess Technol. 14, 2336–2346. doi: 10.1007/s11947-021-02727-7
Martínez-Camacho, A., Cortez-Rocha, M., Ezquerra-Brauer, J., Graciano-Verdugo, A., Rodriguez-Félix, F., Castillo-Ortega, M., et al. (2010). Chitosan composite films: thermal, structural, mechanical and antifungal properties. Carbohydr. Polym. 82, 305–315. doi: 10.1016/j.carbpol.2010.04.069
Mittal, A., Singh, A., Benjakul, S., Prodpran, T., Nilsuwan, K., Huda, N., et al. (2021). Composite films based on chitosan and epigallocatechin gallate grafted chitosan: characterization, antioxidant and antimicrobial activities. Food Hydrocoll. 111:106384. doi: 10.1016/j.foodhyd.2020.106384
Mohammadi Sadati, S. M., Shahgholian-Ghahfarrokhi, N., Shahrousvand, E., Mohammadi-Rovshandeh, J., and Shahrousvand, M. (2022). Edible chitosan/cellulose nanofiber nanocomposite films for potential use as food packaging. Mater. Technol. 37, 1276–1288. doi: 10.1080/10667857.2021.1934367
Negm, N. A., Hefni, H. H., Abd-Elaal, A. A., Badr, E. A., and Abou Kana, M. T. (2020). Advancement on modification of chitosan biopolymer and its potential applications. Int. J. Biol. Macromol. 152, 681–702. doi: 10.1016/j.ijbiomac.2020.02.196
Nešić, A., Cabrera-Barjas, G., Dimitrijević-Branković, S., Davidović, S., Radovanović, N., and Delattre, C. (2019). Prospect of polysaccharide-based materials as advanced food packaging. Molecules 25:135. doi: 10.3390/molecules25010-135
Peng, J., Bu, Z., Ren, H., He, Q., Yu, Y., Xu, Y., et al. (2022). Physicochemical, structural, and functional properties of wampee [Clausena lansium (Lour.) Skeels] fruit peel pectin extracted with different organic acids. Food Chem. 386:132834. doi: 10.1016/j.foodchem.2022.13-2834
Peng, Y., and Li, Y. (2014). Combined effects of two kinds of essential oils on physical, mechanical and structural properties of chitosan films. Food Hydrocoll. 36, 287–293. doi: 10.1016/j.foodhyd.2013.10.013
Pinzon, M. I., Garcia, O. R., and Villa, C. C. (2018). The influence of Aloe vera gel incorporation on the physicochemical and mechanical properties of banana starch-chitosan edible films. J. Sci. Food Agric. 98, 4042–4049. doi: 10.1002/jsfa.8915
Qin, D. D., Wu, Q., Yang, B., Cao, H. X., and Liu, M. L. (2021). Preparation of Auricularia auricular polysaccharide/chitosan edible composite film and its preservative effect on fresh beef. Food Sci. 42, 246–251. doi: 10.7506/spkx1002-6630-20200312-195
Ren, L., Yan, X., Zhou, J., Tong, J., and Su, X. (2017). Influence of chitosan concentration on mechanical and barrier properties of corn starch/chitosan films. Int. J. Biol. Macromol. 105, 1636–1643. doi: 10.1016/j.ijbiomac.2017.02.008
Shen, D. Y., Chao, C. H., Chan, H. H., Huang, G. J., Hwang, T. L., Lai, C. Y., et al. (2012). Bioactive constituents of Clausena lansium and a method for discrimination of aldose enantiomers. Phytochemistry 82, 110–117. doi: 10.1016/j.phytochem.2012.06.019
Shen, S., Liao, Q., Huang, L., Li, D., Zhang, Q., Wang, Y., et al. (2018). Water soluble fraction from ethanolic extract of Clausena lansium seeds alleviates obesity and insulin resistance, and changes the composition of gut microbiota in high-fat diet-fed mice. J. Funct. Foods 47, 192–199. doi: 10.1016/j.jff.2018.05.057
Sivarajan, M., Chandra Mohan, C., Rakhavan, K., Babuskin, S., and Sukumar, M. (2016). Effect of spice incorporated starch edible film wrapping on shelf life of white shrimps stored at different temperature conditions. J. Sci. Food Agric. 96, 4268–4275. doi: 10.1002/jsfa.7638
Song, C., Huang, F., Liu, L., Zhou, Q., Zhang, D., Fang, Q., et al. (2022). Characterization and prebiotic properties of pectin polysaccharide from Clausena lansium (Lour.) Skeels fruit. Int. J. Biol. Macromol. 194, 412–421. doi: 10.1016/j.ijbiomac.2021.11.083
Sun, J., Du, Y., Ma, J., Li, Y., Wang, L., Lu, Y., et al. (2019). Transparent bionanocomposite films based on konjac glucomannan, chitosan, and TEMPO-oxidized chitin nanocrystals with enhanced mechanical and barrier properties. Int. J. Biol. Macromol. 138, 866–873. doi: 10.1016/j.ijbiomac.2019.07.170
Sun, L., Sun, J., Chen, L., Niu, P., Yang, X., and Guo, Y. (2017). Preparation and characterization of chitosan film incorporated with thinned young apple polyphenols as an active packaging material. Carbohydr. Polym. 163, 81–91. doi: 10.1016/j.carbpol.2017.01.016
Sutharsan, J., Boyer, C. A., and Zhao, J. (2022). Physicochemical properties of chitosan edible films incorporated with different classes of flavonoids. Carbohydr Polym Technol Appl 4:100232. doi: 10.1016/j.carp-ta.2022.100232
Tavassoli-Kafrani, E., Shekarchizadeh, H., and Masoudpour-Behabadi, M. (2016). Development of edible films and coatings from alginates and carrageenans. Carbohydr. Polym. 137, 360–374. doi: 10.1016/j.carbpol.2015.1-0.074
Tudu, M., and Samanta, A. (2022). Natural polysaccharides: chemical properties and application in pharmaceutical formulations. Eur. Polym. J. 184, 111801. doi: 10.1016/j.eurpolymj.2022.111801
Ulrich, G. D., and Faez, R. (2022). Thermal, mechanical and physical properties of composite films developed from seaweed polysaccharides/cellulose nanofibers. J. Polym. Environ. 30, 3688–3700. doi: 10.1007/s10924-022-02459-5
Wang, R., Li, X., Liu, L., Chen, W., Bai, J., Ma, F., et al. (2020). Preparation and characterization of edible films composed of Dioscorea opposita Thunb. Mucilage and starch. Polym. Test. 90:106708. doi: 10.1016/j.polymertesting.2020.106708
Wu, H., Min, T., Li, X., Li, L., Lai, F., Tang, Y., et al. (2013). Physicochemical properties and antioxidant activities of acidic polysaccharides from wampee seeds. Int. J. Biol. Macromol. 59, 90–95. doi: 10.1016/j.ijbiomac.2013.04.020
Wu, Q., Qin, D., Cao, H., and Bai, Y. (2020). Enzymatic hydrolysis of polysaccharide from Auricularia auricula and characterization of the degradation product. Int. J. Biol. Macromol. 162, 127–135. doi: 10.1016/j.ijbiomac.2020.06.098
Xie, Y., Wang, L., and Qi, B. (2022). Preparation and properties of crosslinked chitosan/montmorillonite composite films. China Plast 36:58. doi: 10.19491/j.issn.1001-9278.2022.03.010
Xing, L., Miao, Y., Li, N., Jiang, L., and Chen, J. Y. (2021). Molecular structure features and lactic acid fermentation behaviors of water-and alkali-soluble polysaccharides from Dendrobium officinale. J. Food Sci. Technol. 58, 532–540. doi: 10.1007/s13197-020-04564-6
Zareie, Z., Yazdi, F. T., and Mortazavi, S. A. (2020). Development and characterization of antioxidant and antimicrobial edible films based on chitosan and gamma-aminobutyric acid-rich fermented soy protein. Carbohydr. Polym. 244:116491. doi: 10.1016/j.carbpol.2020.116491
Zhang, W., Zeng, G., Pan, Y., Chen, W., Huang, W., Chen, H., et al. (2017). Properties of soluble dietary fiber-polysaccharide from papaya peel obtained through alkaline or ultrasound-assisted alkaline extraction. Carbohydr. Polym. 172, 102–112. doi: 10.1016/j.carbpol.2017.05.030
Zhao, S., Han, Z., Yang, L., Hong, B., and Zhu, H. (2020). Extraction, characterization and antioxidant activity evaluation of polysaccharides from smilacina japonica. Int. J. Biol. Macromol. 151, 576–583. doi: 10.1016/j.ijbiomac.2020.02.015
Zhao, S., Jia, R., Yang, J., Dai, L., Ji, N., Xiong, L., et al. (2022). Development of chitosan/tannic acid/corn starch multifunctional bilayer smart films as pH-responsive actuators and for fruit preservation. Int. J. Biol. Macromol. 205, 419–429. doi: 10.1016/j.ijbiom-ac.2022.02.101
Keywords: edible film, chitosan, polysaccharide, Wampee, properties, antioxidant activity
Citation: Ren Y-Y, Fang J-L, Gong R-Z, Xiang Z-L and Sun P-P (2023) Preparation of alkali-soluble polysaccharide from Clausena lansium (Lour.) Skeels and its effects on properties of chitosan-based edible film. Front. Sustain. Food Syst. 7:1185951. doi: 10.3389/fsufs.2023.1185951
Edited by:
Ravi Pandiselvam, Central Plantation Crops Research Institute (ICAR), IndiaReviewed by:
Nishant Rachayya Swami Hulle, Tezpur University, IndiaPravitha M., Central Institute of Agricultural Engineering (ICAR), India
Tejinder Pal Singh, Lala Lajpat Rai University of Veterinary and Animal Sciences, India
Pranav Vashisht, Tennessee State University, United States, in collaboration with reviewer TS
Copyright © 2023 Ren, Fang, Gong, Xiang and Sun. This is an open-access article distributed under the terms of the Creative Commons Attribution License (CC BY). The use, distribution or reproduction in other forums is permitted, provided the original author(s) and the copyright owner(s) are credited and that the original publication in this journal is cited, in accordance with accepted academic practice. No use, distribution or reproduction is permitted which does not comply with these terms.
*Correspondence: Peng-Peng Sun, cGVuZ3BzdW5AeWFuZ3R6ZXUuZWR1LmNu