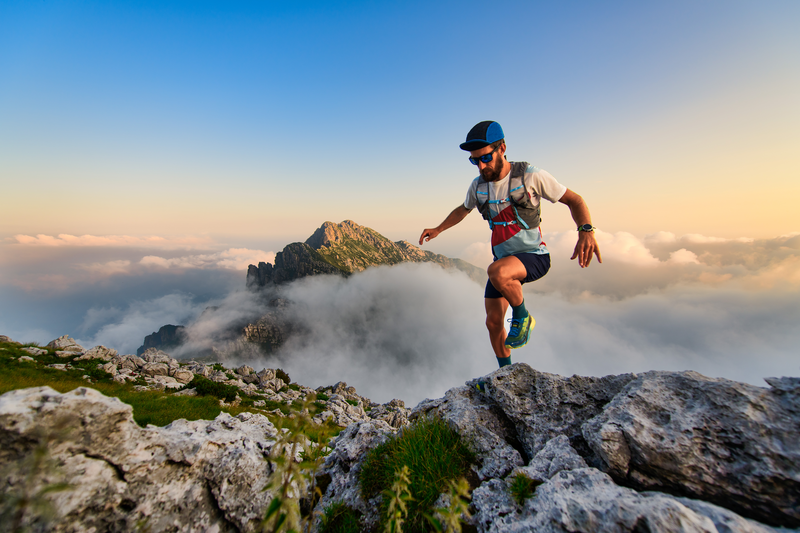
94% of researchers rate our articles as excellent or good
Learn more about the work of our research integrity team to safeguard the quality of each article we publish.
Find out more
ORIGINAL RESEARCH article
Front. Sustain. Food Syst. , 09 August 2023
Sec. Crop Biology and Sustainability
Volume 7 - 2023 | https://doi.org/10.3389/fsufs.2023.1185805
This article is part of the Research Topic Interrelationships Between Food Security, Crop Field Management and Technology View all 9 articles
Soil compaction under repetitive tillage and surface flood method of irrigation (SFMI) are significant hurdles for sustaining crop production in India, necessitating the adoption of efficient soil and water management strategies. Hence, a 3-year field study was conducted at two diverse agro-climatic locations (Abohar and Faridkot) to investigate the impact of subsurface drip (SUSD) fertigation on crop and water productivity of cotton-wheat cropping system (CWCS), over traditional practice (TP) (conventional tillage with SFMI and manual application of nutrients). The experiment was conducted in a factorial randomized complete block design with three levels of subsurface drip irrigation (SUSDI) [100, 80, and 60% of crop evapotranspiration (ETc)] and two fertigation levels [75% recommended dose of nutrients (RDN) and 100% RDN], where TP and surface drip (SD) fertigation at 80% ETc coupled with 100% RDN (Control 2), served as two control treatments. Cotton was raised through reduced tillage, while zero till drill was used for sowing wheat. The results revealed that, barring SUSDI at 60% ETc, both crops exhibited improved yield under all drip combinations of reduced or zero tillage over TP. Better mass and higher length of cotton roots in drip fertigation were evident due to improved steady-state infiltration rates (SSIR) and reduced bulk density (BD) under conservation tillage. When 100% RDN was applied, the 100% and 80% ETc SUSDI resulted in 26.7% and 24.7% higher seed cotton yield (SCY) than TP. Similarly, wheat yield with 100% RDN was improved by 10.5% and 14.4% under SUSDI of 80% and 100% ETc, respectively, over the TP. The results indicated that SUSD can be clubbed with reduced tillage for better soil health, improved crop yield, and higher apparent water productivity. The improved benefit to cost (B:C) owing to enhanced monetary returns over TP also substantiated that reduced tillage with SUSD is a viable and remunerative practice for CWCS. The study elucidated that reduced tillage exercised a beneficial effect on physical soil properties by lowering BD and improving SSIR. At the same time, SUSD could save huge amounts of irrigation water besides enhanced input use efficiency leading to higher crop productivity.
The development of a hard crust in the rhizosphere due to soil compaction and faulty irrigation through the surface flood method of irrigation (SFMI) are among the emerging problems affecting crop productivity in northwestern India (NWI). Bulky mechanical activities or repeated tillage are the prime reason behind soil compaction in farm fields, which is further aggravated by SFMI. After the rice-wheat cropping system (RWCS), the cotton-wheat cropping system (CWCS) is the most prominent in NWI. Together, the CWCS covers nearly 3.22 m ha of both crops in the states of Punjab, Haryana, and Rajasthan (Anonymous, 2020a). The CWCS is the major cash and grain cropping system in NWI and ensures food and fiber security and financial stability (Singh et al., 2022b). Therefore, improved farming practices, such as novel irrigation plans, can be suitably coupled with conservation tillage to realize practical benefits under CWCS. We have discussed and summarized the research work conducted under tillage and described the improvised irrigation techniques in the following paragraphs.
Asgari et al. (2014) observed higher soil BD with increased soil compaction and reported that such undesirable effects may be eliminated by reducing tillage operations. Since cotton and wheat are susceptible to stagnant water, their productivity is adversely affected in fields possessing drainage issues or under heavy precipitation, leading to soil compaction. Continuous tillage-induced soil compaction in China has decreased water use efficiency (WUE) besides poor crop yields owing to low porosity, poor water infiltration rate, and reduced availability of essential nutrients (Jin et al., 2007). Nevertheless, appreciable studies on the tillage effect on corn (Chaudhary et al., 1985), winter wheat (Gajri et al., 1991), oilseeds (Arora et al., 1993), sunflower crop (Gajri et al., 1997), soybean (Arora et al., 2011), and cotton (Singh et al., 2019) are well-documented in the Indian context. However, focused research on reduced tillage in CWCS has not been documented enough. Das et al. (2014) advocated CWCS over RWCS under irrigated conditions in NWI due to higher profitability, productivity, and resource conservation. To address soil degradation, reducing tillage operations seem indispensable in irrigated and arid cropping systems (Omololu et al., 2020). Many workers have reported the beneficial effect of reduced tillage on various soil characteristics in cotton production systems (Yoo and Touchton, 1989; Wright et al., 2008).
Improved irrigation systems reduce nutrient loss either by leaching or volatilization, consequently increasing their availability to plants (Brar et al., 2021). Furthermore, fertilizer application in split doses through fertigation facilitates quick nutrient absorption by crops with minimum fixation issues (Bharath et al., 2015). Drip irrigation has better application efficiency (80–90%) by directly providing water near the root zone besides reduced evaporation from surface soil; low runoff and infiltration loss (Wolff et al., 2017); better productivity and improved quality (Kennedy et al., 2013; Yan et al., 2020); and efficient use of nutrients.
Currently, subsurface drip (SUSD) fertigation is attaining significance owing to the efficient use of nutrients and water as surface runoff is minimal and there is low evaporation from the ground surface since laterals are placed below the soil layer at regular intervals (Mchugh et al., 2008). Roopashree et al. (2016) observed that SUSD could be greatly exploited for efficient water management, specifically in arid and semi-arid zones, compared to surface drip irrigation (SDI) in cotton. Ayars et al. (2015) also attributed the poor adoption rate of SDI to the excessive labor requirement for the layout and removal of drip laterals after each crop season. SUSD can improve the acceptance of drip irrigation in high-intensity cropping patterns (Ayars et al., 1999; Zhang et al., 2019). It reduces water loss by lowering soil surface evaporation and helps in the direct supply of water and fertilizer near the root zone, resulting in the economical and efficient usage of applied inputs (Barbosa et al., 2017). In addition, nitrogen loss through leaching and volatilization is reduced, owing to split and uniform fertigation application (Hagin et al., 2003; Lamm and Trooien, 2003). Besharat et al. (2020) reported that SUSD resulted in 34% higher root development in corn, which facilitated the uptake of water and consequently higher grain yield over conventional methods. Coltro et al. (2017) elucidated that, in Brazil, despite 50% lower water use, 90% lower power, and 66% lower greenhouse gas (GHG) emissions using the SUSD system, paddy productivity was 15% higher than SFMI. Sidhu et al. (2019) reported that SUSD lowered labor costs and enhanced net returns besides permitting direct seeding under zero-tillage in a RWCS. Hence, SUSD systems customized to CWCS must be evaluated for enhancing agricultural production and profit margins with sustained resource use.
The present literature reasonably supports that conservatory tillage and fertigation systems can be explored as a potential tool to improve productivity and reduce the soil, water, and nutrient losses in NWI. Being characterized by arid and semi-arid areas with brackish groundwater, the NWI region is an ideal niche for exploiting conservation strategies (Singh et al., 2020). Das et al. (2014) studied CWCS under assured irrigation, while Jalota et al. (2008) evaluated the impact of tillage and residue management on the yield of CWCS. Except for these two studies, any kind of documented information on the productivity of CWCS is limited to north India.
Hence, the present study was planned to investigate the effect of reduced tillage and SUSD on crop yield, physical soil parameters, and productivity parameters in CWCS. This study hypothesizes that reduced tillage and SUSD would help save water and nutrients and sustain the productivity of CWCS through improved soil characteristics over traditional practice (TP, field preparation by conventional tillage followed by SFMI, and application of fertilizer through manual broadcasting).
The main objectives of this study were to evaluate the impact of reduced tillage and SUSD on (i) growth and yield attributes of cotton and wheat crops in comparison to TP, (ii) physical properties of soil and input use efficiency, and (iii) conduct monetary evaluation and identify ideal tillage plus SUSD combination, for CWCS to be recommended.
Field experiments on CWCS were conducted for 3 years (2018–2020) at University Research Stations located at Faridkot and Abohar. The research stations are 96 km apart in two diverse agro-climatic zones of southwestern Punjab. The research station of Faridkot falls under agro-climatic zone-IV (30° 40′ latitude N and 74° 44′E longitude), while the Abohar research station falls in agro-climatic zone-V (30° 08′N latitude and 74 ° 12′ E longitudes). Both stations lie in the trans-Gangetic plain zone having a sub-tropical climate accompanied by a semi-arid environment possessing a hot, dry summer period from April to June but cool, dry winters during November-February that typically represent NWI. The mean annual precipitation of both research stations ranges from 300–400 mm, of which 75% is primarily received during the monsoon period of July-September. During winter, northwestern disturbances occasionally yield some showers.
The study sites of Faridkot and Abohar have loamy and sandy loam textures, respectively. The initial soil characteristics are mentioned in Table 1. Depth-wise moisture holding capacity (at field capacity and permanent wilting point (PWP)) of soil from both research sites for the 0–100 cm soil profile is presented in Table 2. The average field capacity of the soil profile (determined through the pressure plate apparatus method by Richards and Weaver, 1943) from 0–100 cm was 17.7% and 16.2% v/v for Faridkot and Abohar, respectively. The average BD of the soil profile from 0–100 cm was 1.59 and 1.64 g cm−3 for Faridkot and Abohar, respectively (Table 2).
The experiment was laid out in a factorial randomized complete block design with three replications. A total of seven treatments for both crops (i.e., RCH773 BGII cotton hybrid in summer and PBW725 wheat cultivar in winter season) were evaluated in 2018, while eight treatment combinations were studied in 2019 and 2020. Figure 1 shows the schematic flowchart of the work model and the relevant methodology of the present investigation.
The experiment on cotton had six treatments with SUSD combinations from three subsurface drip irrigation (SUSDI) levels [i.e., 100, 80, and 60% of ETc (crop evapotranspiration)] and two nitrogen fertigation levels [i.e., 100% of recommended nitrogen (RDN) (112 kg N ha−1) and 75% of RDN (84 kg N ha−1)]. Additionally, two extra control treatments were kept. Traditional practice (conventional tillage combined with SFMI and 150 kg N ha−1 applied by manual application of urea) constituted the first control (Control 1) treatment. Surface drip fertigation (SDI) at 80% ETc along with 112 kg N ha−1 was the second control treatment (Control 2), included in the later years. Thus, a total of eight treatments included T1: Control 1 (TP); T2: Control 2; T3: SUSDI at 60% ETc and DF of 75% RDN; T4: SUSDI at 60% ETc and DF of 100% RDN; T5: SUSDI at 80% ETc and DF of 75% RDN; T6: SUSDI at 80% ETc and DF of 100% RDN; T7: SUSDI at 100% ETc and DF of 75% RDN; and T8: SUSDI at 100% ETc and DF of 100% RDN. Except for Control 1, all plots received reduced pre-sowing preparatory tillage by a single round of tractor-operated rotavator. However, Control 1 (TP) received conventional tillage (i.e., field preparation by tractor-operated cultivator and disc harrow twice, followed by single planking) during all crop growing seasons.
The experiment on wheat crops also comprised six treatments having SUSD combinations of three levels of SUSDI [60, 80, and 100% of ETc and two fertigation levels-−75% of recommended nitrogen and phosphorus (NP) fertilizer (75 kg N + 37.5 kg P2O5 ha−1) and 100% of recommended NP fertilizer (100 kg N + 50 kg P2O5 ha−1)]. There were two extra control treatments. The first control treatment (Control 1) was TP, where conventional tillage was followed by SFMI and 100% of recommended NP application (125 kg N + 62.5 kg P2O5 ha−1) through manual broadcasting (Anonymous, 2022–23). Here, wheat sowing was performed using a conventional seed cum fertilizer drill, and a full dose of phosphorus was drilled along with the seed. However, nitrogen (urea) application was done in two equal splits. Sowing of wheat through zero till drill and recommended SD (irrigation at 80% ETc along with DF of 100 kg N + 50 kg P2O5 ha−1) constituted the second control (Control 2). The treatments studied in this experiment included T1: Control 1 (TP); T2: Control 2; T3: SUSDI at 60% ETc and DF of 75% RD NP; T4: SUSDI at 60% ETc and DF of 100% RD NP; T5:SUSDI at 80% ETc and DF of 75% RD NP; T6: SUSDI at 80% ETc and DF of 100% RD NP; T7: SUSDI at 100% ETc and DF of 75% RD NP; and T8: SUSDI at 100% ETc and DF of 100% RD NP. Except for Control 1(TP), the wheat crop in all treatments was sown with zero till drill. It should be clarified that Control 2 (T2) treatment was not studied in 2018 for either of the crops but was included in the later years of the study after it was recommended by the university (Anonymous, 2020b).
After harvesting the wheat at the end of April 2018, the experimental sites at both locations were plowed two times using a disc harrow and cultivator. Then, in a leveled field, subsurface drip systems were laid in lines at 20 cm depth with lateral to lateral spacing at 67.5 cm and emitters at 20 cm distance (discharge rate of 2.2 l h−1) by employing a tractor-run SUSD laying machine. After the layout, pre-sowing irrigation (PSI) of ~75 mm (rauni) was done at both sites to fill the ditches and level the field uniformly. However, in the case of Control 2, SDI laterals (having emitters at 20 cm spacing with an emitter discharge rate of 2.2 l h−1) were manually laid out on the ground surface along the cotton rows at 67.5 cm distance just after cotton emergence. Water meters were installed among various plots to provide a measured water supply. Besides, strong bunds and buffer areas were provided around all plots to minimize variation across different treatments owing to differential water applications. It also provided a passage of implements and machinery in various plots.
The experimental sites at both locations had remained under CWCS for the previous 5 years. After the installation of various SD and SUSD treatments, experimental plots were fixed under CWCS for a 3-year period. After the pre-sowing irrigation (PSI), the whole experimental area (except for Control 1) at optimum field capacity was prepared by a single round of tractor-operated rotavators during all cotton cropping seasons. In May, Bt cotton seed was manually sown by dibbling two seeds hill−1 at 5 cm depth. A population of 19,752 plants per ha was maintained under a planting geometry of 67.5 x 75 cm.
During the winter season, a PSI of 75 mm prior to wheat seeding was applied at the end of October in a standing cotton field (after the first manual picking of seed cotton) in all study years. After the second cotton picking in the first week of November, cotton stalks were eliminated by manual chopping. Then, wheat sowing was done using a tractor-driven zero till seed drill in rows (22.5 cm wider) to visualize the impact of zero tillage and drip fertigation on growth, yield attributes, and grain yield of wheat continuously for 2 subsequent years at both sites. However, Control 1 received conventional tillage prior to wheat sowing. A uniform seed rate of 100 kg ha−1was used for sowing wheat crop. The details of the site, cropping seasons, and study years are mentioned in Table 3. Similarly, detail of crops, planting patterns, genotypes, sowing times, and picking dates are provided in Table 4.
Irrigation of wheat and cotton crops started at 21 and 35 days after sowing (DAS), respectively, and as per treatments of SDI or SUSDI, it was provided at 5–7 days intervals. The quantity of irrigation water that was applied varied as per protocol based on the values of evapotranspiration. In TP, irrigation water was applied using the surface flood method. The amount of water applied during each irrigation was added to calculate the total irrigation amount. To obtain ETc values, the reference evapotranspiration (ETo) was worked out using a calculator developed by the Food and Agriculture Organization (FAO) from site-specific weather parameters (i.e., temperature, humidity, wind velocity, and sunshine period) and multiplied with crop coefficient (Kc). The ETo explains the evaporative strength of the environment and remains unaffected by the crop type (Kamali et al., 2015). In the case of cotton, the Kc was 0.75 until the end of June, 1.15 for July to August, and 0.70 after August. However, for wheat crops, the Kc was 0.39 (at the early phase) and 1.26 from mid-season until maturity (Kaur et al., 2017).
Each surface or subsurface PVC drip lateral pipe provided water to a single cotton row (67.5 cm wide rows) in the summer (Kharif ) season but watered four wheat rows (22.5 cm wide rows) during the winter season (Figure 2). Irrigation application through the SD or SUSD system was initiated when cotton was 35 days old and wheat was 21 days old. Subsequent water applications were made at intervals of 5 and 7 days for cotton and wheat crops according to treatments by calculating cumulative crop ETc values for prior dry days. Drip irrigation for wheat was given at 7-day intervals till mid of February and thereafter at 5-day intervals until crop maturity. Urea fertilizer was applied to the cotton crop to deliver nitrogen for SD and SUSD treatments starting from 35 days after sowing (DAS) as per protocol and provided through ten similar doses after 10-day intervals. Application of P to cotton was omitted as the recommended dose of P was given to the previous season's wheat crop as per university recommendations (Anonymous, 2020b). In Control 1 (TP), a recommended irrigation schedule for SFMI was followed, and urea was given in two similar doses (half at thinning and the remaining half at blooming). The complete fertilizer dose for wheat was fertigated in ten equal doses at weekly intervals starting from 21 DAS. Urea and mono ammonium phosphate were used as nitrogen and phosphorus sources for fertigation in wheat. The irrigation and fertilizer application schedule for both crops in the case of TP is provided in Table 5.
Table 5. Recommended dose of nutrients (RDN) and schedule for nutrients and conventional flood irrigation application in Control 1 (TP).
Soil water content was estimated using time domain reflectometry after calibrating with the Gravimetric method. Layer-wise soil moisture content (0–100 cm profile) was measured after a 3-day gap to determine soil water storage during all experimental periods. Actual crop evapotranspiration (ETactual) during the crop growth period was calculated using the equation (Allen et al., 1998) mentioned below.
where IW = irrigation water applied, Peff. = effective precipitation,
Rs = surface runoff, ΔSW = difference of stored soil profile moisture between planting and crop harvesting, and D = deep drainage in the root zone.
The surface run-off was nil as ridges/buffers were provided all around. Deep drainage was also considered nil when the moisture storage in the soil profile was lower than the field capacity and whenever soil moisture storage exceeded the field capacity after each irrigation or precipitation. Deeper drainage was calculated as the difference between field capacity, soil moisture storage, and rainfall or irrigation. Upward flux from groundwater was ignored as the water table of both experimental locations was below 4 m. Apparent water productivity (AWP) was calculated using the equation below (Sahoo et al., 2018).
where CY is crop yield (kg ha−1), ETa is the actual crop evapotranspiration (m3 ha−1), and nitrogen use efficiency (NUE) was calculated with the help of the formula mentioned below.
Steady-state infiltration rate (SSIR) for cotton and wheat growing season was recorded during July and February months, respectively, by employing an in-situ twin ring infiltrometer by Bouwer (1986), while soil BD was recorded by the Core technique (Black and Hartage, 1986). Initial BD values were recorded at both locations from soil layers of 0–10, 10–20, 20–30, 30–40, 40–60, and 60–100 cm. However, further periodic BD of various experimental plots was recorded from the soil layers of 0–30 and 30–60 cm in all crop growing seasons. Root length (cm) and fresh and dry root weight per plant were observed from 95- to 100-day-old plants from both locations during all growing seasons.
Cotton growth and yield parameters viz. plant height, sympodial (reproductive) branches plant−1, boll weight, and bolls plant−1 were recorded from 10 randomly selected plants plot−1. Seed cotton yield (SCY) from both the manual pickings was added and is expressed in kg ha−1(Singh et al., 2022a). In the case of wheat, crop height, spike length, and grains spike−1 were measured from 10 plants taken at random from every plot. After manual harvesting, the wheat crop was mechanically thrashed to record grain yield and expressed in kg ha−1.
The total cultivation cost spent for growing cotton and wheat crops was worked out from the enterprise budget (2022) for Kharif and Rabi crops as per university recommendations (https://www.pau.edu). The cost incurred for the SUSDI system was calculated considering a life span of 10 years. The benefit-cost (B:C) ratio was calculated to assess the financial viability of various treatments as per Singh et al. (2023).
Statistical analysis of variance was performed for randomized complete block design employing Proc GLM (SAS software 9.3) to compare various treatments. The difference among mean values was compared with Fisher's least significant difference (LSD) at a probability level of p equal to 0.05.
Various weather parameters were measured from the agro-meteorological observatories at Faridkot (Figure 3A) and Abohar (Figure 3B). In Faridkot, total rainfall was lowest at 390.7 mm in 2019, preceded by 424.5 mm in 2018, while, in 2020, it received the highest total rainfall of 667.8 mm. Similarly, the total rainfall at Abohar was 194, 396.2, and 573.4 mm in 2018, 2019, and 2020, respectively. The data showed that Abohar received lower rainfall during the 3 years than Faridkot, while the mean minimum temperature always remained higher at Abohar.
Figure 3. Weather data of experimental site during crop growth period at Abohar (A) and Faridkot (B). Where: RHm, maxiumum relative humidity; RHe, minimum relative humidity; Tmax, maximum temperature; Tmin, minimum temperature.
Numerical variation existed among studied treatments for SSIR during the first cropping cycle of CWCS at both study sites (Figures 4A, D). However, after the first cropping cycle, significant effects among treatments were evident during subsequent cotton seasons in 2019 (Figure 4B) and 2020 (Figure 4C). Reduced tillage in the case of drip fertigated cotton plots enhanced SSIR over Control 1 (TP) in agreement with Lindstrom et al. (1981). The SSIR improved irrespective of the water provided through surface or subsurface drip irrigation over the TP. For instance, during the 2019 cotton season at Abohar, the SSIR under all drip fertigated plots ranged from 2.47-2.73 mm h−1. However, Control 1 exhibited the lowest SSIR (1.87 mm h−1) due to continuous flood irrigation and repetitive tillage (Figure 4B). Though, in later study years, a gradual improvement for SSIR continued with every succeeding cropping season (Figures 4C, E) in agreement with Karamanos et al. (2004), who elucidated that zero and minimum tillage improve soil water status and seed cotton yield in comparison to the conventional tillage. Singh et al. (2019) reported that soil compaction reduced SSIR, and for higher cotton productivity from problematic fields, sub-soiling is required. Baumhardt et al. (1993) also demonstrated that a higher infiltration rate enhanced the available water for cotton and wheat crops in the semiarid south plains of Texas. The data further indicated a higher SSIR of 3.00 mm h−1 and 3.03 mm h−1 at Faridkot and Abohar, respectively, in 2020 at the end of the third cotton cropping cycle (Figure 4C). Despite zero-tilled wheat in all seasons and reduced tillage for cotton, SSIR could improve significantly only during the second cropping season over the Control 1, while slight improvement continued until the third cropping cycle (Figures 4C, E).
Figure 4. Steady-state infiltration rate under different treatments for cotton (A–C) and wheat (D, E) crop at experimental sites. Treatments: T1: SFMI with 100% RDF; T2: SDF at 80% ETc with 100% RDF; T3: SSD at 60% ETc and 75% RDF; T4: SSD at 60% ETc and 100% RDF; T5: SSD at 80% ETc and 75% RDF; T6: SSD at 80% ETc and 100% RDF; T7: SSD at 100% ETc and 75% RDF; T8: SSD at 100% ETc and 100% RDF. Observation time: (A) July 18; (B) July 19; (C) July 20; (D) Feb 19; (E) Feb 20.
Trivial differences existed among the different treatments for soil BD irrespective of soil depth until the first cropping cycle of cotton (Figures 5A, B) and wheat (Figures 5E, F). In contrast, significant differences were evident in subsequent cycles. At both sites, BD under Control 1 (TP) remained higher among all treatments, irrespective of depths. The BD for the topsoil layer (0–30 cm) was invariably higher over 30–60 cm depth, regardless of the seasons and locations. Another measurable trend was that BD values gradually increased with each successive season compared to their initial value for both test locations in the case of TP for cotton (Figures 5C, D, I, J) and wheat (Figures 5G, H). These findings are supported by Omololu et al. (2020), who observed increasing soil compaction irrespective of tillage treatments from the beginning to the end of the cotton crop season. Coates (2001) also reported increased soil penetration resistance from sowing to maturity in irrigated cotton, indicative of enhanced BD in line with present findings. However, the trend was reversed for the rest of the treatments, where BD tended to exhibit a declining response with few abrasions. Higher BD of 2.17 g cm−3 and 2.19 g cm−3 was observed at a depth of 30–60 cm under TP at Abohar and Faridkot, respectively (Figure 5H). The data indicated that, while moving away from conventional tillage toward reduced or zero tillage, the BD values tended to decrease under all drip fertigated plots in accordance with Asgari et al. (2014), who elucidated that BD continues to rise with either level of soil compaction and such negative effects could be nullified by reduced tillage operations.
Figure 5. Soil bulk density under different treatments for cotton (A–D, I, J) and wheat (E–H) crop at experimental sites. Treatments: T1: SFMI with 100% RDF; T2: SDF at 80% ETc with 100% RDF; T3: SSD at 60% ETc and 75% RDF; T4: SSD at 60% ETc and 100% RDF; T5: SSD at 80% ETc and 75% RDF; T6: SSD at 80% ETc and 100% RDF; T7: SSD at 100% ETc and 75% RDF; T8: SSD at 100% ETc and 100% RDF. Observation time: (A, B) July 18; (C, D) July 19; (E, F) Feb 19; (G, H) Feb 20; (I, J) July 20.
As a result of zero or reduced tillage and application of water through drip irrigation, reduced BD values compared to Control 1 (TP) were evident from the second and third cropping seasons, in line with the study of Evan et al. (1996). The present findings were confirmed by Gao and Li (1995), who found that low soil BD by 0.1 g cm−3 up to a depth of 0–50 cm under reduced tillage was evident compared to conventional tilth. The BD under TP was higher by 24.6–36.4% for 0–30 cm and 31.2–37.2% for 30–60 cm over the rest of the treatments at Abohar. Similarly, at Faridkot, the BD value for Control 1 (TP) was higher by 33.8–39.7% for 0–30 cm and 33.9–40.1% for 30–60 cm compared to other treatments. Jerzy and Leszek (2014) found that tilled soil has a unique “coherence” force that causes soil particles to adhere, leading to an unrealistic and dense layering of the adhered soil, which increased layer density and soil compaction, thus supporting the current findings.
Cotton plants exhibited the longest root length, i.e., 136.3, 151.6, and 119.1 cm in 2018, 2019, and 2020 respectively, under T8 at Abohar, and it was statistically better than Control 1 (TP) (Figures 6A–C). These findings are in line with Hodgson et al. (1990), who evaluated the length of cotton root in SDI, SUSDI, and furrow irrigation methods in Australian clayey soils and recorded statistically long roots under SUSDI. A similar trend was observed at Faridkot, except for the fact that the root length values were relatively lower. Significantly shorter root lengths were observed in plots receiving irrigation at 60% ETc at both sites, in line with Luo et al. (2015). This might be because the water supply in these plots remained sub-optimal throughout the crop growth period and, consequently, the root growth of cotton was drastically affected. The findings of Hamza and Anderson (2005) indicated that, if the core index threshold value for cotton exceeded 2,000 kPa, root growth was severely affected by compaction as a result. Contrarily, higher root parameters under T8 might be due to a regular supply of water throughout the crop growth period. Lowered BD values (Figures 5C, D, I, J) coupled with improved SSIR (Figures 4C, E) under reduced tillage and SUSD might have positively affected cotton root growth, leading to significant improvement over TP. Besides, drip fertigated plots receiving irrigation at 100% ETc always received the highest water supply compared to 60% or 80% ETc might have also contributed to improved root growth parameters in conformity with the findings of Min et al. (2014). The observation by Zhang et al. (2017) that the root system of cotton was better developed and suffered less stress under SDI than SFMI due to the availability of relatively stable water content supports current findings.
Figure 6. Root length (A–C), fresh root weight (D–F), and dry root weight (G–I) of cotton under different treatments. Treatments: T1: SFMI with 100% RDF; T2: SDF at 80% ETc with 100% RDF; T3: SSD at 60% ETc and 75% RDF; T4: SSD at 60% ETc and 100% RDF; T5: SSD at 80% ETc and 75% RDF; T6: SSD at 80% ETc and 100% RDF; T7: SSD at 100% ETc and 75% RDF; T8: SSD at 100% ETc and 100% RDF.
Thus, cotton root system under drip fertigation suffered less stress than TP, where the soil experienced water deficit before irrigation and waterlogging after irrigation (Sampathkumar et al., 2012; Lv et al., 2015). Consequently, fresh (Figures 6D–F) and dry root weight (Figures 6G–I) exhibited significantly higher values in plots receiving irrigation at 100% ETc over the TP. The present findings are well supported by Rao et al. (2016), who recorded lesser root spread (42.3 cm), and poor root dry mass (16.1 g plant−1) of furrow irrigated cotton as compared to drip irrigation (having a root spread and root dry mass of 46.9 cm and 17.5 g plant−1) under arid conditions of sub-tropical India. Singh et al. (2019) also reported better root length and fresh and dry root weight of cotton plants in chiseled over the conventionally tilled plots owing to lower BD and higher SSIR values.
Significant reduction in plant height and sympodial branches plant−1 was observed under a SUSDI of 60% ETc (T3 and T4 treatments) at all locations over the years (Table 6). This might be because, among all drip fertigated plots, the lowest amount of irrigation water (i.e., 263.9, 154.3, and 240.0 mm at Abohar in 2018, 2019, and 2020 with corresponding values of 210.4, 251.2, and 233.0 mm at Faridkot) for plant usage was applied here leading to sub-optimal plant growth (Table 8). Contrarily, the improved vigor of plants in the case of T5-T8 treatments over T1 (Control 1) conforms with Yadav and Chauhan (2016), where taller and more vigorous plants under drip irrigation were observed. Poor crop growth in the case of T3 and T4 further substantiated that applied nitrogen might be poorly utilized due to reduced water supply, and thus, sub-optimal crop growth was evident (Prajapati and Subbaiah, 2018). Better plant height and improved sympodial branches plant−1 were recorded under T6-T8 treatments in accordance with Ayyadurai and Manickasundaram (2014). Data further revealed that, though bolls plant−1 continued to increase with an enhanced quantum of applied water and nutrients, significant improvement could be observed only up to a SUSDI of 80% ETc (T6) with a non-significant increase for 100% ETc (T7-T8 treatments). The mean of 3-year data revealed the lowest boll count under T3 (48.2), while it was highest under T8 (65.4) for the reasons specified above. Higher boll weight under T2 (4.12 g), while lowest for T3 (3.65 g) was primarily due to reduced water supply.
Seed cotton was also significantly affected under different treatments in all study years. In 2018, T6 recorded better SCY at Abohar (3,579 kg ha−1) and Faridkot (3,503 kg ha−1), as compared to Control 1 (TP) and T3-T5 but remained at par with T7 and T8. In 2019, SCY under T8 was significantly higher at Abohar (3,599 kg ha−1) and Faridkot (3,502 kg ha−1) over the respective Control 1 (TP) but remained at par with T6. In 2020, better SCY was recorded under T6 at Abohar (3,461 kg ha−1) and Faridkot (3,393 kg ha−1), which remained at par with T2, T7-T8 but superior to the rest all other treatments in agreement with Kakade et al. (2017). SCY under T3-T5 was significantly reduced due to a low supply of inputs (water and nitrogen) compared to T2 and T6-T8 treatments. Significantly reduced yield parameters and seed cotton were observed under T3, where the lowest levels of water and nitrogen were applied.
The mean of 3 years revealed the lowest SCY under Control 1 (2,791 kg ha−1) and highest under T8 (3,540 kg ha−1), closely followed by T6 (3,484 kg ha−1) in line with Aladakatti et al. (2012), who observed at par SCY at SDI of 80% and 100% ETc but a significantly lower yield at 60% ETc and SFMI. Improved yield and yield parameters like bolls plant−1 in SUSDI of 80% and 100% ETc over TP are supported by Neelakanth et al. (2019), who observed a yield improvement of 12% in SUSDI over the flood method. Improved SCY by 23% and 19% over SFMI under an SDI of 80% and 100% ETc also endorses present findings (Singh et al., 2018).
It is pertinent to mention that reduced tillage practices led to favorable improvement in SSIR (Figures 4C, E) and lowered BD values (Figures 5C, D, I, J), which had a beneficial effect on cotton growth and yield parameters over the TP. However, in the case of TP, the frequency of unsaturated soil pores might be decreased post-SFMI, which could have hindered the gaseous exchange between the soil and atmosphere and suffocated the cotton root respiration (Yu et al., 2015). Consequently, the huge quantity of water applied through SFMI reduced the normal activity of cotton roots, resulting in poor yield levels (Hulugalle et al., 2015). Nevertheless, the beneficial effect of improved soil properties through reduced tillage and SUSD contributed to the harvesting of higher SCY. Better boll weight and higher bolls plant−1 in the case of SUSD was the major reason for improved SCY compared to TP. As a result, bolls plant−1 remained higher by 24.8% and 28.9% under T6 and T8, respectively, over TP. Consequently, T6 and T8 recorded 24.7% and 26.7% higher seed cotton than TP (2,791 kg ha−1).
These results indicate that reduced tillage and SUSD play a vital role in enhancing the yield parameters and seed cotton compared to TP, which was in close agreement with the findings of Zhang et al. (2019). The present study's findings reveal that both tillage and input supply (nitrogen and water) primarily regulate the SCY. While reducing tillage operations on one side but improving the water and N level through fertigation on the other side improved SCY over TP. The data also proved that, under reduced tillage, when 100% RDN was applied, SUSDI either at 80% ETc (T6) or 100% ETc (T8) increased SCY significantly by 692 kg ha−1 and 748 kg ha−1, respectively, over TP. This demonstrated that the yield tended to optimize between irrigation levels of 80–100% ETc in addition to responding positively to reduced tillage and SUSD.
The data indicated that plant height, ear length, and grains ear −1 were significantly affected under different treatments at Faridkot during all study years, while differences at Abohar were not significant (Table 7). However, wheat yield was significantly affected at all locations except Abohar in 2018–19. During 2018–19, the highest wheat yield at Faridkot was recorded under T8 (6,193 kg ha−1), though statistically on par with T6 and T7, but significantly better than all other treatments. A similar trend was observed during 2019–20 at Faridkot, where T8 again revealed the highest grain yield (6,340 kg ha−1), though at par with T5-T7, but was significantly better than other treatments. The data further revealed that wheat yield at Faridkot under T6 was higher by 21.0% and 6.1% over the TP during 2018–19 and 2019–20, respectively, in close agreement with Chen et al. (2015). Although wheat yield at Abohar also remained higher under T8 during 2018–19 (4,792 kg ha−1) and 2019–20 (4,407 kg ha−1), the effect was significant only in later years. Wheat yield under T6 was significantly higher by 18.4% at Abohar in 2019–20 over the TP. The overall mean of the 2-year data showed that wheat yield under T6 and T8 was higher by 10.5% and 14.4% over the TP.
These findings suggest that, apart from saving water and nutrients under drip irrigation, yield advantages in wheat were also ensured when SUSD was combined with zero tillage compared to TP. The beneficial effect of improved soil properties (i.e., reduced BD and improved SSIR) achieved through zero tillage sowing of wheat coupled with SUSD might have attributed to harvesting higher wheat yield (Singh et al., 2019). Nevertheless, when irrigated at 60% ETc (i.e., 40% lesser irrigation), both cotton and wheat experienced water stress, as evidenced by significantly reduced bolls plant−1 in cotton (Table 6) and reduced ear length as well as grains ear−1 in wheat (Table 7). The current observations are supported by Chen et al. (2020), who reported poor productivity of drip-irrigated wheat crop owing to a low quantity of applied water. In general, wheat yield is governed by ear length, grains ear −1, and test weight. However, in this study, grains ear −1 and ear length were highly prone to water stress, and wheat yield was negatively affected in accordance with Denčić et al. (2000). The grains ear −1 declined with any reduction in the amount of irrigated water, owing to the limited supply of water and its negative impact on assimilate translocation leading to poor yield.
A comparison of water applied and saved (%) under different schedules in cotton and wheat crops is provided in Table 8. Water saving in SUSDI cotton (i.e., over a range of 60–100% ETc) at Faridkot ranged from 32.1–52.0, 52.4–61.4, and 29.8–49.4% for 2018, 2019, and 2020, respectively, over the TP. The corresponding values for Abohar were 40.2–55.7, 36.0–54.3, and 19.7–41.7%, respectively (Table 8). Similarly, water saving under wheat raised through SUSDI over TP ranged from 49.9 to 62.0 and from 54.5 to 66.0% at Faridkot and from 42.6 to 57.5 and from 27.9 to 46.8% at Abohar during 2018–19 and 2019–20, respectively (Choudhary et al., 2014). This data indicated a considerable saving of water under drip fertigated treatments for both crops, in close agreement with Li et al. (2018).
Table 8. Comparison of water applied and saving (%) under different schedules in cotton and wheat crops.
The mean of 3-year data over the two locations revealed the highest apparent water productivity (AWP) of 1.361 kg m−3 in cotton under T6 treatment (Table 9). The TP recorded significantly lower values for AWP in both crops. A mean value of 0.596 and 1.324 kg m−3 was observed for cotton and wheat, respectively, which revealed the inferiority of TP as compared to drip irrigated treatments. Reduced AWP under TP as compared to T6 indicated it to be less efficient in conformity with Singh et al. (2018), who found the least water productivity (0.95 kg m−3) under a SDI of 60% ETc. The amount of irrigated water under various drip treatments was sufficiently lower to avoid deeper infiltration besides minimizing nutrient loss over TP (Ning et al., 2015). Better AWP under T6 and T8 was supported by Shruti and Aladakatti (2017), where DI at 80% ETc remained superior over other irrigation levels.
Table 9. Apparent water productivity (kg m−3) of cotton and wheat as influenced by various treatments.
Nitrogen use efficiency (NUE) was lowest in cotton (26.6 kg SCY kg−1 of N) and wheat (38.0 kg grain kg−1 of N) grown under TP (Jayakumar et al., 2015). Though NUE was higher under T7 for cotton (38.5) and wheat (68.5), the AWP values were relatively lower (Table 10). This indicated T7 to be slightly inferior to T6, where both exhibited better magnitudes. Except for T4 in cotton (SUSD at 60% ETc with DF of 100% RDN), all drip fertigation treatments in both crops revealed higher NUE, which revealed the superiority of drip treatments due to reduced losses of applied nutrients.
Table 10. Economics and Nitrogen use efficiency (NUE) of cotton, wheat and CWCS as influenced by various treatments.
Owing to improved yield attributes, the productivity of the drip-fertigated CWCS under T6 (80% ETc along with 100% RDN) was higher by 19.6% (in terms of cotton equivalent yield) as compared to TP (Table 10). Similarly, the productivity of CWCS at 80% ETc was improved by 6.48% when both crops (cotton and wheat) were fertigated using 75% in comparison to 100% RDN. Increasing the fertigation rate from 75% to 100% RDN improved the yield attributes and overall yield, suggesting that a higher fertigation rate can lead to increased productivity. However, only minimal improvement in productivity was observed at both levels of nutrition when applied with higher water application of 100% ETc (T7-T8). This established T6 (SUSDI at 80% ETc along with 100% RDN) to be the optimal level of fertigation. Though few studies have revealed that using just 40-80% of the conventional dose through drip fertigation is better for optimum productivity (Feng et al., 2017), in this study, SCY continued to improve up to 100% RDN. However, in the case of wheat, grain yield under SUSD was highest at 100% RDN (i.e., 80% of RDN under TP), in line with Bai et al. (2020), who found that increasing fertigation rates above 70% of conventional dose would not enhance wheat yield. However, in the present study, the application of 100% RDN through fertigation (i.e., 80% RDN of TP in the case of wheat and 100% RDN of TP in the case of cotton) resulted in better crop productivity owing to higher NUE (Table 10).
Higher gross returns ($4271.6 ha−1), net returns ($2793.6 ha−1), and B:C ratio (1.89) were observed under T8 (SUSD of 100% ETc with fertigation of 100% RDN) for CWCS (Table 10). However, T6 (SUSD of 80% ETc with fertigation of 100% RDN) exhibited slightly low net returns of $2,676.5 ($117.2 ha−1 less as compared to T8) but saved 20% of irrigation water (Table 8) and higher AWP (Table 9). Lowest gross returns ($3155.3 ha−1), net returns ($1724.7 ha−1), and B:C (1.21) were recorded for SUSDI at 60% ETc along with 75% RDN. This indicated T3 as the least remunerative among drip treatments. Reduced water supply remained the primary reason for poor economic returns. Nevertheless, T6 recorded higher net returns over TP by 31.3%. A reduced B:C ratio (1.45) under TP further substantiated that conservatory tillage and fertilizer application through SUSD was much more rewarding under NWI conditions in conformity with Neelakanth et al. (2019), who observed 16% better net returns for SUSDI over conventional methods. These findings are well supported (Kang et al., 2012; Pawar et al., 2015).
Soil compaction under repetitive tillage and the continuous decline of non-replenishable water sources is a major challenge for the agrarian sector that requires urgent attention to sustainable alternative options. Therefore, the need to optimize efficient technologies like conservation tillage and SUSD in CWCS while maintaining the optimum yield with better management of water and fertilizer resources is much sought after. Our findings indicated that, by adopting conservatory tillage, seed cotton and wheat yield increased by 692 and 499 kg ha−1, respectively, under a SUSDI of 80% ETc with 100% RDN over TP. Higher AWP for cotton (1.361 kg m−3) and wheat (2.907 kg m−3) established T6 (SUSD of 80% ETc with 100% RDN) as the most efficient and best-suited option for CWCS. Reduced tillage exercised a beneficial effect on physical soil properties by lowering BD and improving SSIR. At the same time, SUSD could save huge amounts of irrigated water besides enhanced input use efficiency, leading to higher food and fiber yields. The improved benefit:cost ratio owing to enhanced monetary returns over TP also substantiated that conservation tillage with SUSD can be a viable and remunerative practice. This study elucidated that SUSD, when coupled with reduced tillage in CWCS, improved soil health besides significantly saving irrigation water compared to TP. Therefore, in addition to saving huge quantities of irrigation water, implementing reduced tillage and SUSD in CWCS would greatly benefit in supporting healthy soil to sustain crop productivity.
The present study elucidated that reduced tillage coupled with SUSD in the CWCS is a potential tool for addressing future water scarcity expected in NWI due to the continuously depleting groundwater table as an alternative to RWCS. Policymakers should, therefore, advocate alternatives to assist agriculturists in adopting economic and efficient water usage techniques rather than disbursing monetary help through subsidies for groundwater pumping. For instance, the Direct Benefit Transfer (DBT) on electric power saving might be notified by the state/federal governments to support farmers in their minimal use of water for agrarian purposes. In this context, a fine combination of SUSD and alternate CWCS would be beneficial. Besides, the integration of reduced tillage and SUSD in a CWCS might serve to be a complementary approach for policy as well as in technological aspects. After installation, SUSD could be utilized for a fairly longer period (about 10 years) than surface drip owing to the non-removal of laterals as needed for surface drip after each harvest and before the next season's cropping. Furthermore, saving fuel and manpower on the preparatory tillage of CWCS under SUSD would improve farmers' profit margins considerably without any yield penalty. Such additional benefits would reduce cultivation costs and may support the government to cut the subsidies on free power and surface drip. Comprehending the challenge of receding water resources, forecasted market upheaval, and rice procurement issues in NWI, sustaining CWCS with optimum SUSD fertigation could be an environmentally effective and economically viable alternative to replace unsustainable RWCSs.
Certain limitations exist in the current research, which offers scope for further study. Though the higher costs of installing micro irrigation systems could be a major bottleneck in the widespread acceptance of SUSD at the level of farmers, incentivizing sustainable water-savvy techniques could be a better option coupled with the promise of future dividends. The SUSD and conservative tillage also deserve special appraisal in water-starved areas for efficient utilization of preserved rainwater in attaining national water security in addition to food and fiber security and improved soil health.
The authors wish to extend this investigation toward the cotton-rapeseed/mustard cropping system, which is a healthy alternative to replace the traditional rice-wheat cropping system. Though the success of reduced tillage clubbed with SUSD systems for CWCS has been proven to be economical in NWI, for comprehensive output, a long-term evaluation of this method at farmers' fields is equally essential and might lead toward new challenges and opportunities for further refinement of this technology.
The original contributions presented in the study are included in the article/supplementary material, further inquiries can be directed to the corresponding authors.
All authors listed have made a substantial, direct, and intellectual contribution to the work and approved it for publication.
This work was funded by the Punjab Agricultural University, Ludhiana, India and also by Researchers Supporting Project (No. RSP2023R390), King Saud University, Riyadh, Saudi Arabia.
The authors extend their appreciation to Punjab Agricultural University, Ludhiana, India, and the Researchers Supporting Project Number (RSP2023R390), King Saud University, Riyadh, Saudi Arabia.
The authors declare that the research was conducted in the absence of any commercial or financial relationships that could be construed as a potential conflict of interest.
All claims expressed in this article are solely those of the authors and do not necessarily represent those of their affiliated organizations, or those of the publisher, the editors and the reviewers. Any product that may be evaluated in this article, or claim that may be made by its manufacturer, is not guaranteed or endorsed by the publisher.
AWP, apparent water productivity; BD, bulk density; CA, conservation agriculture; CWCS, cotton-wheat cropping system; DAS, days after sowing; DF, drip fertigation; ETc, crop evapotranspiration; ETo, reference evapotranspiration; NUE, nitrogen use efficiency; NWI, north-western India; PSI, pre-sowing irrigation; RWCS, rice-wheat cropping system; RDN, recommended dose of nutrients; RCBD, randomized complete block design; SCY, seed cotton yield; SD, surface drip; SUSD, subsurface drip; SUSDI, subsurface drip irrigation; SSIR, steady state infiltration rate; SFMI, surface flood method of irrigation; TP, traditional practice; WUE, water use efficiency.
Aladakatti, Y. R., Hallikeri, S. S., Nandagavi, R. A., Shivamurthy, D., and Malik, R. (2012). Precision irrigation and fertigation to enhance the productivity and economic returns of Bt cotton in vertisols. Proc 3rd Agro-Informat Precision Agric. Hyderabad, India, 341–43.
Allen, R. G., Pereira, L. S., Raes, D., and Smith, M. (1998). Crop Evapotranspiration: Guidelines for Computing Crop Water Requirements – FAO Irrigation and Drainage Paper 56. Rome: Food and Agriculture Organization of the United Nations.
Anonymous (2020a). Agricultural Statistics at a Glance 2019: Government of India- Ministry of Agriculture and Farmers Welfare. Department of Agriculture, Cooperation and Farmers Welfare: Directorate of Economics and Statistics. Available online at: https://eands.dacnet.nic.in/PDF/At%20a%20Glance%202019%20Eng.pdf.Pp:51-78 (accessed January 15, 2023).
Anonymous (2020b). Package of practices for crops of Punjab - kharif (2020). Available online at: https://www.pau.edu/content/ccil/pf/pp_kharif.pdf (accessed January 5, 2023). Pp:34. Punjab Agricultural University, Ludhiana, India
Anonymous. (2022–23). Package of Practices for Crops of Punjab—Rabi 2022. (Ludhiana, India: Punjab Agricultural University), 1–22. Available online at: https://www.pau.edu/content/ccil/pf/pp_rabi.pdf (accessed January 20, 2023).
Arora, V. K., Gajri, P. R., and Chaudhary, M. R. (1993). Effects of conventional and deep tillage on mustard for efficient water and nitrogen use in coarse textured soils. Soil Tillage Res. 26, 327–340. doi: 10.1016/0167-1987(93)90004-9
Arora, V. K., Singh, C. B., Sidhu, A. S., and Thind, S. S. (2011). Irrigation, tillage and mulching effects on soybean yield and water productivity in relation to soil texture. Agric. Water Manage. 98, 563–568. doi: 10.1016/j.agwat.10004
Asgari, H. R., Ghiami, A., Zahra, S., and Farshid, G. (2014). Effect of subsoil compaction constraints on some morphological, physiological and agronomic properties of wheat (Triticum aestivum L.) under rain-fed farming. Int. J. Adv. Biol. Biom. Res. 2, 1657–1669.
Ayars, J. E., Fulton, A., and Taylor, B. (2015). Subsurface drip irrigation in California—Here to stay? Agric. Water Manage. 157, 39–47. doi: 10.1016/j.agwat.01001
Ayars, J. E., Phene, C. J., Hutmacher, R. B., Davis, K. R., Schoneman, R. A., Vail, S. S., et al. (1999). Subsurface drip irrigation of row crops: a review of 15 years research at the water management research laboratory. Agric. Water Manage. 42, 1–27. doi: 10.1016/S0378-3774(99)00025-6
Ayyadurai, P., and Manickasundaram, P. (2014). Growth, nutrient uptake and seed cotton yield as influenced by foliar nutrition and drip fertigation in cotton hybrid. Int. J. Agric. Sci. 10, 276–279.
Bai, S., Kang, Y., and Shuqin, W. (2020). Drip fertigation regimes for winter wheat in the North China Plain. Agric. Water Manage. 228, 105885. doi: 10.1016/j.agwat.2019.105885
Barbosa, E. A. A., Matsura, E. E., dos Santos, L. N. S., Gonçalves, I. Z., Nazário, A. A., Feitosa, D. R. C., et al. (2017). Water footprint of sugarcane irrigated with treated sewage and freshwater under subsurface drip irrigation in Southeast Brazil. J. Clean. Prod. 153, 448–456. doi: 10.1016/j.jclepro.01167
Baumhardt, R. L., Keeling, J. W., and Wendt, C. W. (1993). Tillage and residue effects on infiltration into soils cropped to cotton. Agron. J. 85, 379–383. doi: 10.2134/agronj1993.00021962008500020038x
Besharat, S., Barão, L., and Cruz, C. (2020). New strategies to overcome water limitation in cultivated maize: eesults from subsurface irrigation and silicon fertilization. J. Environ. Manage. 263, 110398. doi: 10.1016/j.jenvman.2020.110398
Bharath, R., Joshi, M., and Vishaka, G. V. (2015). Effect of surface fertigation on nutrient uptake, fertilizer use efficiency and economics of inter-specific hybrid Bt cotton. Univ. J. Agric. Res. 3, 46–48. doi: 10.13189/ujar.2015.030202
Black, G. R., and Hartage, K. H. (1986). “Bulk density,” in Methods of Soil Analysis. Part I. Physical and Mineralogical Methods. Am. Soc. Agron. Soil Sci. Soc. Am, ed Klute, A. (WI: Madison), pp. 363–375. doi: 10.2136./sssabookser5.1.2ed.c13
Bouwer, H. (1986). “Intake rate: cylinder infiltrometer,” in Methods of Soil Analysis. Part I. Physical and Mineralogical Methods. Am. Soc. Agron. Soil Sci. Soc. Am, ed Klute, A. (WI: Madison), pp. 825–844. doi: 10.2136./sssabookser5.1.2ed.c32
Brar, A. S., Buttar, G. S., Singh, M., Singh, S., and Vashist, K. K. (2021). Improving biophysical and economic water productivity of menthol mint (Mentha arvensis L.) through drip fertigation. Irri. Sci. 39, doi: 10.1007/s00271-021-00722-6
Chaudhary, M. R., Gajri, P. R., Prihar, S. S., and Romesh, K. (1985). Effect of deep tillage on soil physical properties and maize yield on coarse textured soils. Soil Tillage Res. 6, 31–44. doi: 10.1016/0167-1987(85)90004-2
Chen, R., Cheng, W., Cui, J., Liao, J., Fan, H., Zheng, Z., et al. (2015). Lateral spacing in drip irrigated wheat: the effects on soil moisture, yield, and water use efficiency. Field Crops Res. 179, 52–62. doi: 10.1016/j.fcr.03021
Chen, X., Thorp, K. R., van Oel, P. R., Xu, Z., Zhou, B., Li, Y., et al. (2020). Environmental impact assessment of water-saving irrigation systems across 60 irrigation construction projects in northern China. J. Clean. Prod. 245, 118883. doi: 10.1016/j.jclepro.2019.118883
Choudhary, R. A. K. E. S. H., Jat, M. L., Nandal, D. P., Sidhu, H. S., Singh, Y. A. D. V. I. N. D. E. R., Jat, H. S., et al. (2014). Conservation Agriculture in irrigated cotton-wheat system of the western Indo-irrigation plains: crop and water productivity and economic profitability. Field Crops Res. 158, 24–33. doi: 10.1016/j.fcr.12017
Coates, W. E. (2001). Reduced tillage systems for irrigated cotton: is soil compaction a concern? Appl. Eng. Agric.17, 273–279. doi: 10.13031/2013.6207
Coltro, L., Marton, L. F. M., Pilecco, F. P., Pilecco, A. C., and Mattei, L. F. (2017). Environmental profile of rice production in Southern Brazil: a comparison between irrigated and subsurface drip irrigated cropping systems. J. Clean. Prod. 153, 491–505. doi: 10.1016/j.jclepro.09207
Das, T. K., Bhattacharyya, R., Sudhishri, S., Sharma, A. R., Saharawat, Y. S., Bandyopadhyay, K. K., et al. (2014). Conservation agriculture in an irrigated cotton-wheat system of the western Indo-Gangetic Plains: Crop and water productivity and economic profitability. Field Crops Res. 158, 24–33. doi: 10.1016/j.fcr.2013.12.017
Denčić, S., Kastori, R., Kobiljski, B., and Duggan, B. (2000). Evaluation of grain yield and its components in wheat cultivars and landraces under optimal and drought conditions. Euphytica, 113, 43–52. doi: 10.1023/A:1003997700865
Evan, S. D., Lindstrom, W. B., Voorhees, W. B., Moncrief, J. F., and Nelson, G. A. (1996). Effect of subsoiling and subsequent tillage on soil bulk density, soil moisture, and corn yield. Soil Tillage Res. 38, 35–46. doi: 10.1016/0167-1987(96)01020-3
Feng, Z., Wan, S., Kang, Y., and Liu, S. (2017). Drip fertigation regime for potato on sandy soil. Emirates J. Food and Agric. 29, 476–484. doi: 10.9755/ejfa.2017-02-275
Gajri, P. R., Gill, K. S., Chaudhary, M. R., and Rachpal, S. (1997). Irrigation of sunflower (Helianthus annuus) in relation to tillage and mulching. Agric. Water Manage. 34, 149–160. doi: 10.1016/S0378-3774(97)00009-7
Gajri, P. R., Prihar, S. S., Cheema, S. S., and Kapoor, A. (1991). Irrigation and tillage effect on root development, water use and yield of wheat on coarse texture soils. Irri. Sci. 12, 161–168. doi: 10.1007/BF00192288
Gao, H. W., and Li, H. W. (1995). A testing study of dryland subsoiling. Agric. Res. Arid Areas 4, 126–133.
Hagin, J., Sneh, M., and Lowengart-Aycicegi, A. (2003). PI Research Topics No. 23: Fertigation—Fertilization Through Irrigation. Basel, Switzerland: International Potash Institute.
Hamza, M. A., and Anderson, W. K. (2005). Soil compaction in cropping systems—A review of the nature, causes and possible solutions. Soil Tillage Res. 82, 121–145. doi: 10.1016/j.still.08009
Hodgson, A. S., Constable, G. A., Duddy, G. R., and Daniells, I. G. (1990). A comparison of drip and furrow irrigated cotton on a cracking clay soil. 2. Water use efficiency, water logging, root distribution and soil structure. Irri. Sci. 11, 143–148. doi: 10.1007/BF00189451
Hulugalle, N. R., Broughton, K. J., and Tan, D. K. Y. (2015). Fine root production and mortality in irrigated cotton, maize and sorghum sown in vertisols of northern New South Wales, Australia. Soil Tillage Res. 146, 313–322. doi: 10.1016/j.still.10004
Jackson, M. L. (1967). Soil Chemical Analysis. Prentice Hall of India. (New Delhi: Private Limited), 234–46.
Jalota, S. K., Buttar, G. S., Sood, A., Chahala, G.B.S., Ray, S.S., and Panigrahy, S. (2008). Effects of sowing date, tillage and residue management on productivity of cotton (Gossypium hirsutum L.)–wheat (Triticum aestivum L.) system in northwest India. Soil Tillage Res. 99, 76–83. doi: 10.1016/j.still.01005
Jayakumar, M., Surendran, U., and Manicksundram, P. (2015). Drip fertigation program on growth, crop productivity, water and fertilizer use efficiency of Bt cotton in semi arid tropical region of India. Comm. Soil Sci. Plant Anal. 46, 293–300. doi: 10.1080/00103624.2014.969403
Jerzy, B., and Leszek, S. (2014). Effect of moisture content on soil density—Compaction relation during soil compacting in the soil bin. Ann. WaAboharw Univ. Life Sci. SGGW, Agricult. 64, 5–13.
Jin, H., Li, H., Wang, X., McHugh, A. D., Wenying, L., Huanwen, G., et al. (2007). The adoption of annual sub-soiling as conservation tillage in dry land maize and wheat cultivation in northern China. Soil Tillage Res. 94, 493–502. doi: 10.1016/j.still.10005
Kakade, S., Bhale, V., Deshmukh, J., and Wadatkar, S. (2017). Growth, nutrient uptake and seed cotton yield as influenced by split application of nutrients through fertigation in Bt cotton. Int. J. Curr. Microbiol. App. Sci. 6, 2982–2990. doi: 10.20546/ijcmas.609366
Kamali, M. I., Nazari, R., Faridhosseini, A., Ansari, H., and Eslamian, S. (2015). The determination of reference evapotranspiration for spatial distribution mapping using geostatistics. Water Res. Manage. 29, 3929–3940. doi: 10.1007/s11269-015-1037-4
Kang, Y. H., Wang, R. S., Wan, S. Q., Hu, W., Jiang, S. F., Liu, S. P., et al. (2012). Effects of different water levels on cotton growth and water use through drip irrigation in an arid region with saline ground water of Northwest China. Agric. Water Manage. 109, 117–126. doi: 10.1016/j.agwat.02013
Karamanos, A. J., Bilalis, D., and Sidiras, N. (2004). Effects of reduced tillage and fertilization practices on soil characteristics, plant water status, growth and yield of upland cotton. J. Agron. Crop. Sci. 190, 262–276. doi: 10.1111/j.1439-037X.2004.00101.x
Kaur, H., Huggins, D. R., Rupp, R. A., Abatzoglou, J. T., Stöckle, C. O., Reganold, J. P., et al. (2017). Agro-ecological class stability decreases in response to climate change projections for the Pacific Northwest, USA. Front. Ecol. Environ. 5, 74. doi: 10.3389/fevo.2017.00074
Kennedy, T. L., Suddick, E. C., and Six, J. (2013). Reduced nitrous oxide emissions and increased yields in California tomato cropping systems under drip irrigation and fertigation. Agriculture, Ecosyst. Environ. 170, 16–27. doi: 10.1016/j.agee.02002
Lamm, F. R., and Trooien, T. P. (2003). Subsurface drip irrigation for corn production: a review of 10 years of research in Kansas. Irri. Sci. 22, 195–200. doi: 10.1007/s00271-003-0085-3
Li, J., Xu, X., Lin, G., Wang, Y., Liu, Y., Zhang, M., et al. (2018). Micro-irrigation improves grain yield and resource use efficiency by co-locating the roots and N-fertilizer distribution of winter wheat in the North China Plain. Sci. Total Environ. 643, 367–377. doi: 10.1016/j.scitotenv.06157
Lindstrom, M. J., Voorhees, W. B., and Randall, G. W. (1981). Long term tillage effects on inter row runoff and infiltration. Soil Sci. Soc. Am. J. 45, 945–948.
Luo, H. H., Tao, X. P., Hu, Y. Y., Zhang, Y. L., and Zhang, W. F. (2015). Response of cotton root growth and yield to root restriction under various water and nitrogen regimes. J. Plant Nutr. Soil Sci. 178, 384–392. doi: 10.1002/jpln.201400264
Lv, G. H., Song, J. Q., Bai, W. B., Wu, Y. F., Liu, Y., Kang, Y. H., et al. (2015). Effects of different irrigation methods on micro-environments and root distribution in winter wheat fields. J. Integr. Agric. (14, 1658–1672. doi: 10.1016/S2095-3119(14)60927-8
Mchugh, A. D., Bhattarai, S., and Midmore, D. J. (2008). Effects of subsurface drip irrigation rates and furrow irrigation for cotton grown on a vertisol on off-site movements of sediments, nutrients and pesticides. Agric. Sustain. Dev. 28, 507–519. doi: 10.1051/agro:2008034
Merwin, H. D., and Peech, M. (1950). Exchangeability of soil potassium in sand, silt and clay fractions as influenced by the nature of complementary exchangeable cations. Section II—Soil Chemistry. Soil Sci. Soc. Am. J. 15, 125–128. doi: 10.2136/sssaj1951.036159950015000C0026x
Min, W., Guo, H. J., Zhou, G. W., Zhang, W., Ma, L. J., Ye, J., et al. (2014). Root distribution and growth of cotton as affected by drip irrigation with saline water. Field Crops Res. 169, 1–10. doi: 10.1016/j.fcr.09002
Neelakanth, J. K., Rajkumar, S., Gundlur, S. S., and Dasar, G. V. (2019). Effect of surface and subsurface drip irrigation system on seed cotton in vertisols of Malaprabha command in northern Karnataka. J. Pharmacogn. Phytochem. 8, 956–958.
Ning, S. R., Shi, J. C., Zuo, Q., Wang, S., and Ben-Gal, A. (2015). Generalization of the root length density distribution of cotton under film mulched drip irrigation. Field Crops Res. 177, 125–136. doi: 10.1016/j.fcr.03,012.
Olsen, S. R., Cole, C. V., Waternade, F. S., and Dean, L. A. (1954). Estimation of available phosphorous in soil by extraction with sodium bicarbonate. USDA Circ. 939, 1–19.
Omololu, J. I., Sifat, S., Murali, D., Leslie, B., Robert, S., Mohammed, O., et al. (2020). Tillage effects on cotton performance and soil quality in an irrigated arid cropping system. Agriculture 10, 531. doi: 10.3390/agriculture10110531
Pawar, N., Bishnoi, D. K., Singh, M., and Dhillon, A. (2015). Comparative economic analysis of drip irrigation vis-a-vis flood irrigation system on productivity of Bt cotton in Haryana. Agric. Sci. Digest. 35, 300–303. doi: 10.18805/asd.v35i4.6863
Prajapati, G. V., and Subbaiah, R. (2018). Combined response of irrigation system regimes and mulching on productivity of Bt Cotton. J. Agrometeorol. 20, 47–51.
Rao, S. S., Tanwar, S. P. S., and Regar, P. L. (2016). Effect of deficit irrigation, phosphorous inoculation and cycocel spray on root growth, seed cotton yield and water productivity of drip irrigated cotton in arid environment. Agric. Water Manage. 169, 14–25. doi: 10.1016/j.agwat.02008
Richards, L. A., and Weaver, L. R. (1943). Fifteen-atmosphere percentage as related to the permanent wilting percentage. Soil Sci. 56, 331–339. doi: 10.1097/00010694-194311000-00002
Roopashree, M., Rajkumara, S., and Neelakanth, J. K. (2016). Effect of surface and subsurface drip irrigation at different ETc levels on growth and yield of Bt cotton (Gossypium hirsutum L.). J. Farm Sci. 29, 456–460.
Sahoo, P., Brar, A. S., and Sharma, S. (2018). Effect of methods of irrigation and sulphur nutrition on seed yield, economic and bio-physical water productivity of two sunflower (Helianthus annus L.) hybrids. Agric. Water Manage.2 06, 158–164. doi: 10.1016/j.agwat.05009
Sampathkumar, T., Pandian, B. J., and Mahimairaja, S. (2012). Soil moisture distribution and root characters as influenced by deficit irrigation through drip system in cotton–maize cropping sequence. Agric. Water Manage. 103, 43–53. doi: 10.1016/j.agwat.10016
Shruti, M. Y., and Aladakatti, Y. R. (2017). Effect of drip irrigation and fertigation on yield, economics and water use efficiency of intra-hirsutum Bt cotton. J. Farm Sci. 30, 185–89.
Sidhu, H. S., Jat, M. L., Singh, Y., Sidhu, R. K., Gupta, N., Singh, P., et al. (2019). Subsurface drip fertigation with conservation agriculture in a rice-wheat system: a breakthrough for addressing water and nitrogen use efficiency. Agric. Water Manage. 216, 273–283. doi: 10.1016/j.agwat.02019
Singh, K., Brar, A. S., and Singh, H. P. (2018). Drip fertigation improves water and nitrogen use efficiency of Bt cotton. J. Soil Water Cons. 73, 549–557. doi: 10.2489/jswc.73.5.549
Singh, K., Choudhary, O. P., Singh, H. P., Singh, A., and Mishra, S. K. (2019). Sub-soiling improves productivity and economic returns of cotton-wheat cropping system. Soil Tillage Res. 189, 131–139. doi: 10.1016/j.still.01013
Singh, K., Mishra, S. K., Singh, M., Singh, K., and Brar, A. S. (2022b). Water footprint assessment of surface and subsurface drip fertigated cotton-wheat cropping system—A case study under semi-arid environments of Indian Punjab. J. Clean. Prod. 365, 132735. doi: 10.1016/j.jclepro.2022.132735
Singh, K., Mishra, S. K. P., and Rathore Kumar, A. (2022a). Reduced dose coupled with improved method of neem-coated urea application and foliar sprays of KNO3 enhance cotton yield and fiber parameters under semi-arid climates. J. Plant Nutri. 3, 4368. doi: 10.1080/01904167.2022.2144368
Singh, K., Rathore, P., and Mishra, S. K. (2023). Osmoprotectants improve cotton yield by alleviating moisture stress under semi-arid conditions. Agron. J. 2, 1238. doi: 10.1002/agj2.21238
Singh, K., Singh, H. P., and Mishra, S. K. (2020). Irrigation module and sowing dates affect seed cotton yield, quality, productivity indices and economics of cotton in north-western India. Comm. Soil Sci. Plant Annal. 51, 1–13. doi: 10.1080/00103624.2020.1744633
Subbiah, B. V., and Asija, G. L. (1956). A rapid procedure for the estimation of available nitrogen in soils. Current Sci.25, 259–260.
Walkley, A., and Black, C. A. (1934). An examination of the Degtjareff method for determination of soil organic matter and a proposed modification of chromic acid titration method. Soil Sci. 37, 29–38. doi: 10.1097/00010694-193401000-00003
Wolff, M. W., Hopmans, J. W., Stockert, C. M., Burger, M., Sanden, B. L., Smart, D. R., et al. (2017). Effects of drip fertigation frequency and N-source on soil N2O production in almonds. Agric. Ecosyst. Environ. 238, 67–77. doi: 10.1016/j.agee.08001
Wright, A. L., Hons, F. M., Lemon, R. G., McFarland, M. L., and Nichols, R. L. (2008). Microbial activity and soil C sequestration for reduced and conventional tillage cotton. Appl. Soil Ecol. 38, 168–173. doi: 10.1016/j.apsoil.10006
Yadav, B. S., and Chauhan, R. P. S. (2016). Drip fertigation technology for enhancing water and nutrient use efficiency in arid agro-ecosystem of irrigated northwestern Rajasthan. Annals Arid Zone. 55, 139–145.
Yan, S., Wu, Y., Fan, J., Zhang, F., Paw, U., Zheng, K. T., et al. (2020). A sustainable strategy of managing irrigation based on water productivity and residual soil nitrate in a no-tillage maize system. J. Clean. Prod. 262, 121279. doi: 10.1016/j.jclepro.2020.121279
Yoo, K. H., and Touchton, J. T. (1989). Runoff and soil loss by crop growth stage under three cotton tillage systems. J. Soil Water Cons. 44, 225–228.
Yu, Y. X., Zhao, C. Y., Zhao, Z. M., Yu, B., and Zhou, T. H. (2015). Soil respiration and the contribution of root respiration of cotton (Gossypium hirsutum L.) in arid region. Acta Ecol. Sin. 35, 17–21. doi: 10.1016/j.chnaes.04001
Zhang, H., Liu, H., Sun, C., Gao, Y., Gong, X., Sun, J., et al. (2017). Root development of transplanted cotton and simulation of soil water movement under different irrigation methods. Water 9, 503. doi: 10.3390/w9070503
Keywords: apparent water productivity, bulk density, drip fertigation, reduced tillage, seed cotton yield, steady state infiltration rate
Citation: Singh K, Singh M, Mishra SK, Soufan W, Habib-ur-Rahman M and El Sabagh A (2023) Reduced tillage and subsurface fertigation improve productivity and economic benefits in the cotton-wheat cropping system. Front. Sustain. Food Syst. 7:1185805. doi: 10.3389/fsufs.2023.1185805
Received: 14 March 2023; Accepted: 07 July 2023;
Published: 09 August 2023.
Edited by:
Adnan Mustafa, Brno University of Technology, CzechiaReviewed by:
Pravin Kumar Upadhyay, Indian Agricultural Research Institute (ICAR), IndiaCopyright © 2023 Singh, Singh, Mishra, Soufan, Habib-ur-Rahman and El Sabagh. This is an open-access article distributed under the terms of the Creative Commons Attribution License (CC BY). The use, distribution or reproduction in other forums is permitted, provided the original author(s) and the copyright owner(s) are credited and that the original publication in this journal is cited, in accordance with accepted academic practice. No use, distribution or reproduction is permitted which does not comply with these terms.
*Correspondence: Kulvir Singh, a3VsdmlyQHBhdS5lZHU=; Ayman El Sabagh, YXltYW5lbHNhYmFnaEBnbWFpbC5jb20=
Disclaimer: All claims expressed in this article are solely those of the authors and do not necessarily represent those of their affiliated organizations, or those of the publisher, the editors and the reviewers. Any product that may be evaluated in this article or claim that may be made by its manufacturer is not guaranteed or endorsed by the publisher.
Research integrity at Frontiers
Learn more about the work of our research integrity team to safeguard the quality of each article we publish.