- 1Mill Industries, San Bruno, CA, United States
- 2School of Veterinary Medicine, University of California, Davis, Davis, CA, United States
- 3Graduate Group in Epidemiology, University of California, Davis, Davis, CA, United States
- 4Department of Animal Science, University of California, Davis, Davis, CA, United States
- 5Department of Food Science and Technology, University of California, Davis, Davis, CA, United States
Introduction: New poultry feed valorization pathways for recovered household food could be enabled by commercially available household devices that dry uneaten food material, arrest spoilage, and preserve nutrient content. However, the nutrient composition, safety, and feed incorporation potential of dried recovered household food (DRHF) is presently unknown.
Methods: Thirty-eight households spanning 31 states participated in a 4-to-6-week survey to generate and collect food residues that were dried using an in-home device. The DRHF samples were evaluated for chemical composition, digestibility of energy and amino acids, and safety to determine their potential for inclusion in chicken feed.
Results and discussion: The DRHF had average levels of 15.9% crude protein, 13.3% crude fat, and 22.6% neutral detergent fiber, and 3.18 kcal/g of nitrogen-adjusted true metabolizable energy (by dry weight). The Windows User-Friendly Feed Formulation 2.1 modeler was used to perform linear programming and develop chicken feed rations for broilers and layers that incorporated DRHF alongside conventional feed ingredients, including corn, soybean meal, dicalcium phosphate, limestone, synthetic amino acids, salt, vitamin premix, and mineral premix. The feed formulation results showed that, on average, DRHF incorporation rates of up to 33 and 37% (by weight) are predicted to avoid any nutrient deficiencies or electrolyte imbalances in the broiler and layer rations, respectively. In the broiler ration, DRHF displaced corn, soybean meal, and limestone to varying degrees, while corn, soybean meal, animal fat, dicalcium phosphate, and limestone were substantially displaced in the layer rations. Addition of vitamin premix was predicted as necessary to facilitate DRHF inclusion in the layer rations. Furthermore, foodborne pathogens, mycotoxins, and heavy metals were either absent or below United States regulatory threshold levels. Measured levels of biogenic amines and fat/oil oxidation were consistent with prior research showing compatibility with chickens. These results can inform future in vivo feeding trials to validate the use of DRHF with varying properties in poultry feed.
1. Introduction
Currently, the majority of non-industrial food waste in the United States is disposed of in landfills (55.9% of total non-industrial food waste; US Environmental Protection Agency, 2018) with minor fractions routed to animal feed (2.9%), composting (4.1%), and biofuel production (8.3%; US Environmental Protection Agency, 2018). Landfilling of food waste is associated with deleterious environmental effects, such as the emission of methane, a greenhouse gas with 80.8x the warming power of carbon dioxide over the first 20 years following its generation (Arias et al., 2021). Additional emissions stem from the collection, transportation, storage, and processing of food waste (Lam et al., 2018). In 2015, United States landfills were estimated to contribute 115.7 MT of carbon dioxide equivalent emissions to the atmosphere, representing the third largest source of national greenhouse gas emissions (US Environmental Protection Agency, 2017). Among the organic wastes deposited in landfills, food waste contributes most to the degradable carbon that drives greenhouse gas emissions (Lee et al., 2017).
In light of the risks of landfilling organic waste, legislation has started to mandate diversion of such wastes, including food waste, from landfills in some states. For example, California (Senate Bill 1,383), Maryland (House Bill 264), New Jersey (Assembly 2,371), and Vermont (Act 148) have set limits on the amount of food waste sent to landfills. While mitigating generation of food waste and routing excess edible food to humans for consumption are paramount, solutions are required for the residual food that will remain as part of the post-consumer food system (Jaglo et al., 2021). Feeding recovered household food to poultry has deep historical roots (Barber, 2018) and remains relevant today in United States urban, suburban, and rural regions (Blecha and Leitner, 2014; Elkhoraibi et al., 2014). However, this practice is primarily confined to non-commercial flocks kept by individual households (Blecha and Leitner, 2014; Elkhoraibi et al., 2014).
For commercial chicken production, feed production is a major determinant of overall environmental impact (Schader et al., 2015; Skunca et al., 2018). The use of land and other resources to produce animal feed can also lead to competition with human food production (Breewood and Garnett, 2020). Accordingly, replacement of feed ingredients that have high environmental impact with lower impact alternatives that avoid feed-food competition is an important tool for improving overall production sustainability (Di Paola et al., 2017; Searchinger et al., 2018; Skunca et al., 2018; Benavides et al., 2020). Research has indicated that aggregated residual food from food service facilities and restaurants can be processed into a dewatered and pelletized poultry feed (Siddiqui et al., 2021), which suggests there are opportunities for consolidating post-consumer recovered food for feed applications. Such strategies could create circular food economies where recovered food enables community and commercial production of poultry, linking agricultural and consumer-level sustainability practices. Assessment of this approach at community scales has suggested that utilization of aggregated recovered household food for chicken feed could have pronounced economic, energy efficiency, and environmental benefits for poultry meat and egg production (Hall et al., 2014; Trainer et al., 2019). Furthermore, data suggest that adoption of sustainable production practices could spark consumer interest in the resulting food products (Verain et al., 2012). In particular, younger consumers are increasingly recognizing the importance of sustainable food systems and factoring sustainability into their food purchasing decisions (Su et al., 2019).
As interest in promoting food system sustainability grows, new household appliances have entered the market that allow recovered food to be milled and dried into a stable material. Manufacturers of these devices sometimes promote the dried material as a soil amendment. However, such dried recovered household food (DRHF) materials may also be a promising animal feed due to their being stabilized in the home soon after the food residues are generated. In this way, the nutrient content and safety of the original food residues may be retained. Should the DRHF be a viable animal feed ingredient, it could motivate new pathways to collect and utilize the dried material in sustainable animal production. Previous work has examined incorporation of post-consumer and retail-level food residues, including dried residues, in pig diets (Rivas et al., 1994; Myer et al., 1998; Westendorf et al., 1998; Myer et al., 1999; Altizio et al., 2000; Westendorf, 2000; Adedokun et al., 2015; Dou et al., 2018). Much of this work has focused on scraps and wasted edible food from restaurants. However, the authors are aware of no studies that have evaluated the properties of household food residues that have been stabilized through in-home drying or the incorporation of such materials in conventional commercial poultry feed. Accordingly, the goals of this study were to assess the nutritional and safety properties of food residue samples from households using food residue dehydrators and conduct an initial assessment of their suitability for animal feed. Specifically, a survey was conducted to collect DRHF from households across the contiguous United States. The DRHF samples were screened for toxicological and microbiological hazards and nutritional properties, which informed linear programming to model the incorporation of DRHF into chicken feed rations. Both broiler finisher and layer rations were considered. The results are useful for informing future strategies to aggregate dried recovered household food and develop scalable valorization approaches to convert them into chicken feed.
2. Materials and methods
2.1. Participant selection for DRHF survey
In March 2022, a survey was conducted to identify a group of households across the United States that met the following criteria: household size greater than or equal to two, annual household income greater than $100,000, and a willingness to spend money to do something good for the environment or try new product solutions to help address climate change. The survey platform dscout (222 N La Salle St Ste. 650, Chicago, IL, United States) was used for recruitment and over 3,000 individuals submitted applications. Participants were not informed of the investigators’ identities to remove any potential biases. All communications were managed via the dscout platform, entries were submitted via text, photo, or video uploads, and responses were reviewed asynchronously. Of the total applicants, 40 were selected based on their availability to participate in a 4 to 6 week long survey, a qualitative assessment of their ability to provide thorough responses, and their state of residence.
While 40 participants accepted the invitation to join the survey and received the necessary materials, 2 participants stopped responding after the first week. The remaining 38 completed the full 4-to-6-week survey. The selected participants ranged in age from 28 to 54, with an average age of 39.3, and represented a diverse set of industry backgrounds (public utility, financial services, aerospace, apparel and accessories, non-profit, telecommunication, education, entertainment and leisure, manufacturing, health care, consulting, transportation, government and politics, service, design consulting, legal, customer services, managed care, technology, retail and wholesale, banking, and defense). All participants reported an education level of at least ‘college-level’, with 21 participants reporting ‘postgraduate coursework’. 34 of the 38 participants self-reported as ‘employed full time (30 h +)’, with 1 participant self-reporting as working ‘part-time’, and 3 participants self-reporting as ‘homemaker’. For household ethnicity 28 of the 38 participants self-identified as ‘White’, 3 self-identified as ‘Black or African American’, 2 self-identified as ‘White, Hispanic or Latinx’, 1 self-identified as ‘Hispanic or Latinx’, 2 self-identified as ‘Asian’, and 2 preferred not to say. Household size included adults and children, with 37 of the 38 participants reporting living with a partner or spouse, 29 of which also reported having at least one child under the age of 18. The participant who did not report living with a partner or spouse reported living with a child under the age of 18. All participants reported an annual household income of at least $100,000, with 6 households earning between $125,000 - $149,999 and 22 households earning greater than $150,000. The households represented 31 unique states (AZ, CA, CO, FL, GA, IL, IN, KS, MA, MD, MI, MN, MO, NC, NH, NJ, NV, NY, OH, OK, OR, PA, RI, SC, TN, TX, UT, VA, WA, WI, and WV) and the District of Columbia, with two households represented each of the following 6 states: CA, CO, GA, IL, NY, and TX.
2.2. Processing and collection of DRHF
Each household received a FoodCycler™ FC-50 (Vita-Mix Corporation, Olmsted Falls, OH) dryer unit, a 5-gallon screw-top pail (ULINE, Pleasant Prairie, WI), and a cardboard tab-locking box with a compostable bag (Better Packaging Co., Auckland, NZ) for lining. Participants were asked to use the dryer for 4 to 6 weeks, which involved adding all of the compatible food residues generated by their household each day, and running a cycle when the device bucket (2.5 L capacity) filled up. Participants were prompted to follow guidance provided by the device manufacturer in the user manual. The manual advised against the addition of items ‘larger than the size of a human palm’ unless they were cut down to a smaller size to reduce stress on the device. Large bones (such as pork or beef bones), pits, candy, gum, nuts, and hard shells were specifically listed as potential hazards that may cause damage to the bucket and/or cause the motor to overload. Oil, water, and any flammable materials or compounds were also listed as items to avoid adding to the device. Supplemental instructions were also provided to participants. All vegetables, fruits, herbs, meats, dairy products, eggs, frozen food, chicken and fish bones, coffee grounds, filters, tea bags (no staple), fiber-based food packaging, and food-soiled paper towels and napkins were listed on an ‘Ok to add’ list. The following items were highlighted in the ‘Do not add’ list: liquids, oils, grease, compostable plastic, large bones, hard shells (e.g., clam and oyster), wire ties, rubber bands, or other packaging. Participants were asked to follow the guidance to the best of their ability and report any items they were unsure about.
The 4-to-8-h drying and grinding cycle achieved temperatures of 60.0 to 80.5°C in the food material and transformed the wet food residues into a dried and ground output material. Participants were instructed to empty the output material into the 5-gallon pail. As soon as the 5-gallon pail was full, participants sent a message via the dscout platform and a courier pickup was requested on their behalf. If participants had not filled their pails after approximately 4 weeks, they were instructed to send their partially full pail in and continue collecting DRHF in the tab-locking box with the compostable bag liner. Each participant’s DRHF were aggregated upon reception depending on how many containers they filled. Additionally, a portion of each participant’s DRHFwas sieved to reject particles greater than 9.5 mm. Aliquots of 875 g of sieved DRHF from each participant were stored in opaque, airtight containers under ambient conditions until further use.
2.3. Chemical and microbiological analyses
Sieved samples from each participant’s collected DRHF were aliquoted into plastic bags, sealed, and shipped to external labs for chemical composition, lipid peroxidation, and microbiological analyzes. All samples were shipped at ambient temperature using two-day shipping. The various analyzes followed the standardized methodologies summarized in Supplementary material S1. Totox (total oxidation) number (Shahidi and Wanasundara, 2002) was calculated as Totox = 2 × peroxide value + p-anisidine value. The available phosphorus in each sample was estimated as 40% of the total phosphorus value. Although available phosphorus data are not available for DRHF in chicken feed, this conversion factor is similar to the available P:total P ratio found in several grains used in swine feed (which, like chickens, are non-ruminant animals; National Research Council, 1994).
2.4. Calculated and measured nitrogen-corrected true metabolizable energy
Metabolizable energy (ME) was calculated based on the following formula for protein-rich poultry feed (Nascimento, 2007; Alvarenga et al., 2011):
where EE is ether extract (g/kg) and NDF is neutral detergent fiber (g/kg).
Additional DRHF samples underwent empirical measurement of true metabolizable energy corrected to zero nitrogen retention (TMEn; n = 5 cages) using a previously described method (Parsons et al., 1982). The DRHF samples for TMEn analysis were generated by pooling DRHF samples of approximately equal mass from all participants and then randomly sampling from different locations of the bulk material to compile the 1 kg of DRHF required for the analysis. The ratio between the average predicted ME based on the composition data and the average empirically measured TMEn was used to create a conversion factor that could adjust the calculated ME values for each DRHF sample to better reflect expected TMEn values.
2.5. Digestible amino acids
Samples of aggregated DRHF (prepared as described in the preceding section) were processed for empirical measurement of digestible amino acids (n = 5 cages) using an existing method (Engster et al., 1985). The average measured digestibility for each amino acid was multiplied by the total level for each amino acid in each DRHF sample to predict the sample’s digestible amino acid content.
2.6. Chicken feed formulation
The Windows User-Friendly Feed Formulation 2.1 (WUFFDA2.1) modeling tool was used to determine DRHF incorporation rates into various chicken feed rations (Alhotan and Pesti, 2016). WUFFDA2.1 uses the solver function in Microsoft Excel software to perform linear programming and combine a user-specified list of feed ingredients to match a user-specified ration nutrient profile while minimizing the cost of the final ration. Specifically, DRHF inclusion in Hy-Line W-36 layer and Cobb 500 broiler rations was considered. Both breeds utilize indoor production systems and have been used commercially for decades. The layer ration corresponded to hens at 85–89% of peak egg production, while the broiler ration represented a finisher diet for chickens 23–42 days old. The nutrient criteria for each ration met or exceeded the nutrient requirements specified by the National Research Council (1994) and breeders’ recommendations (Hy-Line International, 2020; Cobb-Vantress, 2022) and are given in Table 1. The composition data for each DRHF sample were entered into the modeler to generate a series of corresponding feed formulations. Corn, soybean meal, animal fat, dicalcium phosphate, limestone, DL-methionine, L-lysine, L-threonine, common salt (sodium chloride), and vitamin premix (including choline and folate) were designated as possible ingredients to complement DRHF in the rations. Compositional properties for these conventional feed ingredients were obtained from the National Research Council (1994, 2012) and the National Animal Nutrition Program (2022). The default conventional ingredient costs in WUFFDA2.1 were used, which, despite continuously shifting market conditions, reflect the general differences in ingredient cost magnitude: corn, $176/ton; soybean meal, $445/ton; animal fat, $540/ton; dicalcium phosphate, $960/ton; limestone, $160/ton; DL-methionine, $4,800/ton; L-lysine, $2,700/ton; L-threonine, $3,700/ton; common salt, $50/ton; vitamin premix, $3,600/ton. To maximize the inclusion of DRHF, the cost of DRHF was set at $0 in WUFFDA2.1. Additionally, all feed ingredients were set to have upper limits of 100% in the final ration formulation to allow the modeler to find a solution that best satisfied the nutrient requirements without constraining ingredient selection. The resultant feed formulations were used to identify the upper limits of DRHF inclusion in each ration and to discover potential excess nutrients in the rations. In cases where nutrients or dietary electrolyte balance (DEB) levels (Mongin, 1981) were found to exceed chicken tolerances, appropriate maximum limits were added to WUFFDA2.1 and the feed rations were reformulated for each DRHF sample. Specifically, to agree with recommended poultry limits for sodium (0.23%; Cobb-Vantress, 2022) and DEB (150–250 mEq/kg with tolerance up to 350 mEq/g; Gezen et al., 2005; Ahmad et al., 2009; Mushtaqi et al., 2013), maximum limits of 0.23 and 1.11% for sodium and potassium, respectively, were imposed and rations were reformulated. WUFFDA2.1 was also used to generate feed rations without DRHF inclusion to facilitate comparison of rations with DRHF against rations containing only conventional feed ingredients.
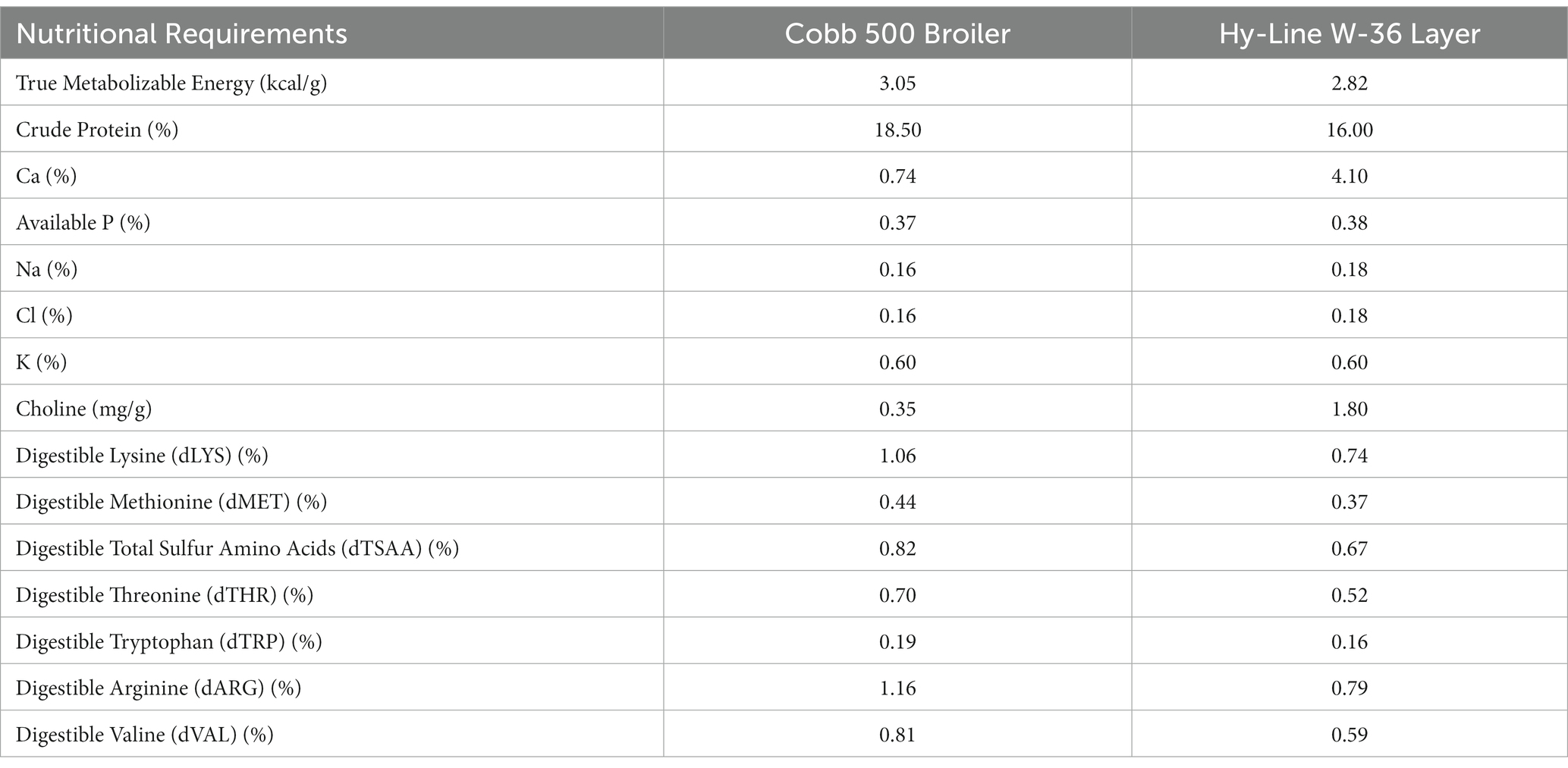
Table 1. Nutritional requirements in feeds for Cobb 500 broilers (for broilers from 23 to 42 days of age) and Hy-Line W-36 layers (targeted at 85–89% peak egg production).
2.7. Statistical analysis
Multivariate correlation analysis of the ingredients in the feed ration formulations was performed in JMP Pro 16 software (version 16.2.0, SAS Institute Inc.).
3. Results
3.1. Chemical composition and dietary properties of DRHF
The complete set of measured values for the assays outlined in Supplementary material S1 are provided in Supplementary material S2. Select properties are summarized in Figure 1A. The drying process resulted in DRHF with an average dry matter content of 90.5%. Average values for compositional properties required for ration formulation in WUFFDA2.1 included 3.2% calcium, 0.72% chloride, 15.9% crude protein, 1.1% potassium, 0.46% sodium, and 0.33% total phosphorus (yielding an estimate of 0.13% available phosphorus).
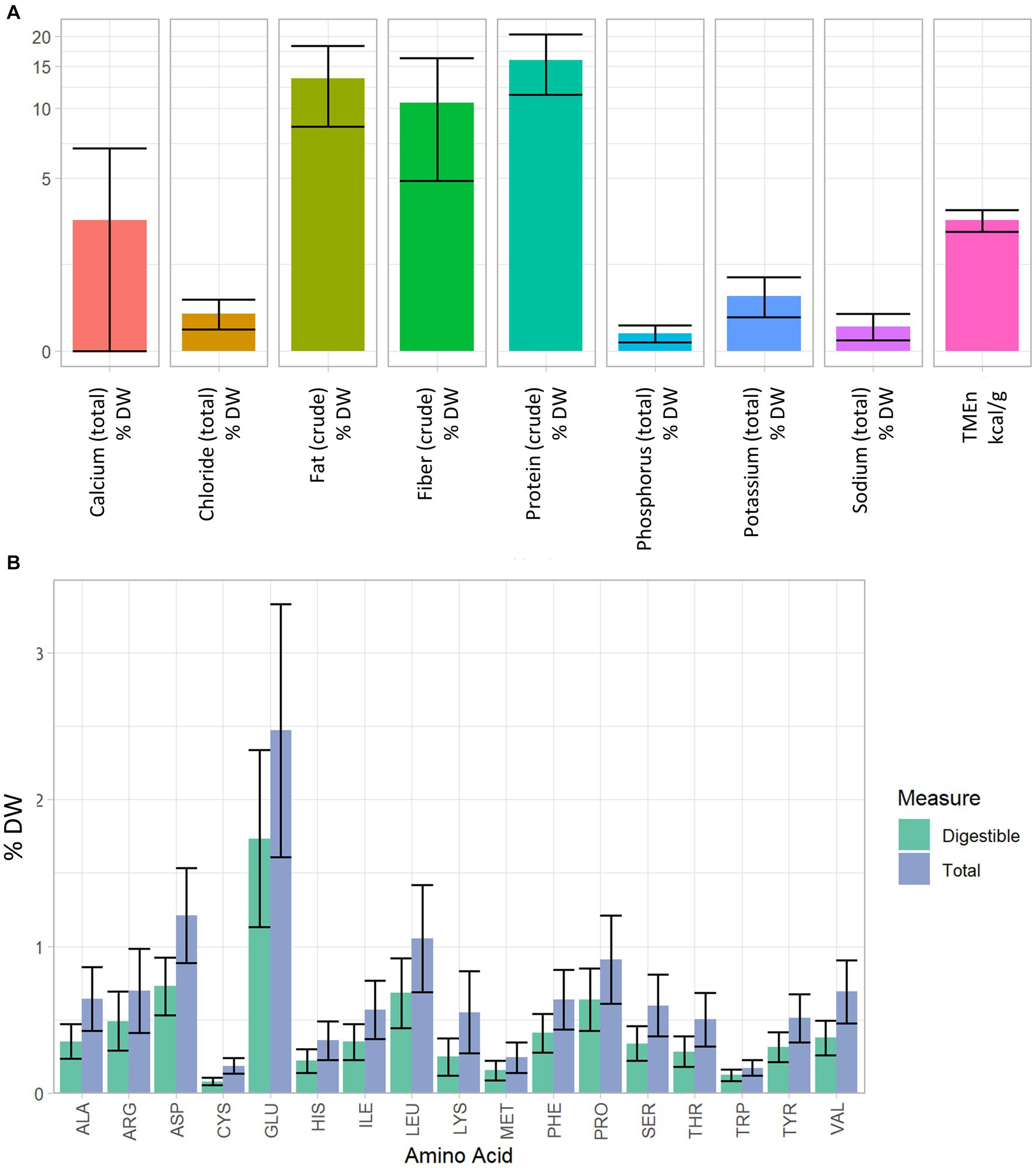
Figure 1. Compositional properties of DRHF collected from participant households. Values show select proximate characteristics, elements, and TMEn (A) and total and digestible levels of amino acids (B). Error bars indicate one standard deviation. AR, value given in material as-received; DW, values given on dry weight basis.
The mean values for crude fat and NDF were 13.3 and 22.6%, respectively. Individual crude fat and NDF values were used in conjunction with Equation 1 to calculate ME values for each DRHF sample. The mean calculated ME value based on DRHF composition data was 3.12 kcal/g whereas the mean in vivo- measured TMEn value was 3.18 kcal/g (Supplementary material S3). Accordingly, each calculated ME value was multiplied by a correction factor of 1.02 [(3.18 Kcal/g; 3.12 Kcal/g)−1] to obtain the TMEn values inputted to WUFFDA2.1. For each DRHF sample, the total values for each amino acid were multiplied by the corresponding average measured in vivo digestibility values (Supplementary material S4) to obtain estimates of digestible amino acid concentrations in each DRHF sample (Figure 1B).
3.2. Toxicological and microbiological properties of DRHF
Complete results for microbiological, heavy metal, biogenic amine, mycotoxin, and lipid oxidation assays are given in Supplementary material S2. There was no detection of heavy metals, Staphylococcus aureus, Escherichia coli, Listeria, or Salmonella in any DRHF samples. Additionally, most mycotoxins were not detected in any samples (specifically, aflatoxins B1, B2, G1, G2; deoxynivalenol; fumonisin B2, B3; T-2 toxin; zearalenone). There was one sample out of 38 that showed detectable fumonisin B1 (0.3 ppm) and 2 of 38 had measurable ochratoxin (2.6 to 5.8 ppb).
Biogenic amines 2-phenylethylamine, cadaverine, histamine, spermine, and tryptamine were not detected in any DRHF samples. Spermidine was detected in 2 of 38 samples at levels ranging 5.5 to 6.1 mg/kg. Putrescine was found in 4 out of 38 samples at levels spanning 6.8 to 11.6 mg/kg. Tyramine was detected in 8 out of 38 samples at levels ranging 5.3 to 21.2 mg/kg.
Thirty-one of the 38 DRHF samples contained measurable peroxides. For samples with non-zero values, the values ranged 2.8 to 21.6 and the mean value was 8.4 meq/kg fat. P-anisidine values averaged 52.2 across all 38 samples (with values ranging 14 to 165). Totox values across all samples had a range of 17 to 191 with a mean value of 65.9.
3.3. Chicken feed rations containing DRHF
Broiler and layer rations were formulated without DRHF inclusion to provide baseline diets for comparison against rations containing DRHF (Figure 2). Both formulations contained corn as the primary ingredient, representing 70% broiler ration and 55% of the layer ration. Soybean meal content was similar in both rations (25–27%). The layer rations required 5–10% of animal fat, dicalcium phosphate, and limestone, while the broiler rations contained comparatively less or no amounts of these ingredients. Additionally, the layer ration uniquely contained 0.56% vitamin premix. Conversely, the broiler rations uniquely contained L-lysine (0.22%) and L-threonine (0.07%). Both rations contained similar levels of DL-methionine (0.20–0.27%) and common salt (0.34–0.37%). Both baseline rations provided 100% of the necessary TME, crude protein, calcium, available phosphorus, and sodium, but contained an excess of chloride (145–150% of target values) and potassium (119–137% of target values). For the layer ration, 100% of the required choline was provided while an excess of choline (439% of target) was delivered in the broiler ration. Digestible amino acids (dLYS, dMET, dTHR, dTRP, dARG, and dVAL) were supplied at levels ranging 100 to 135% of the target values in both rations.
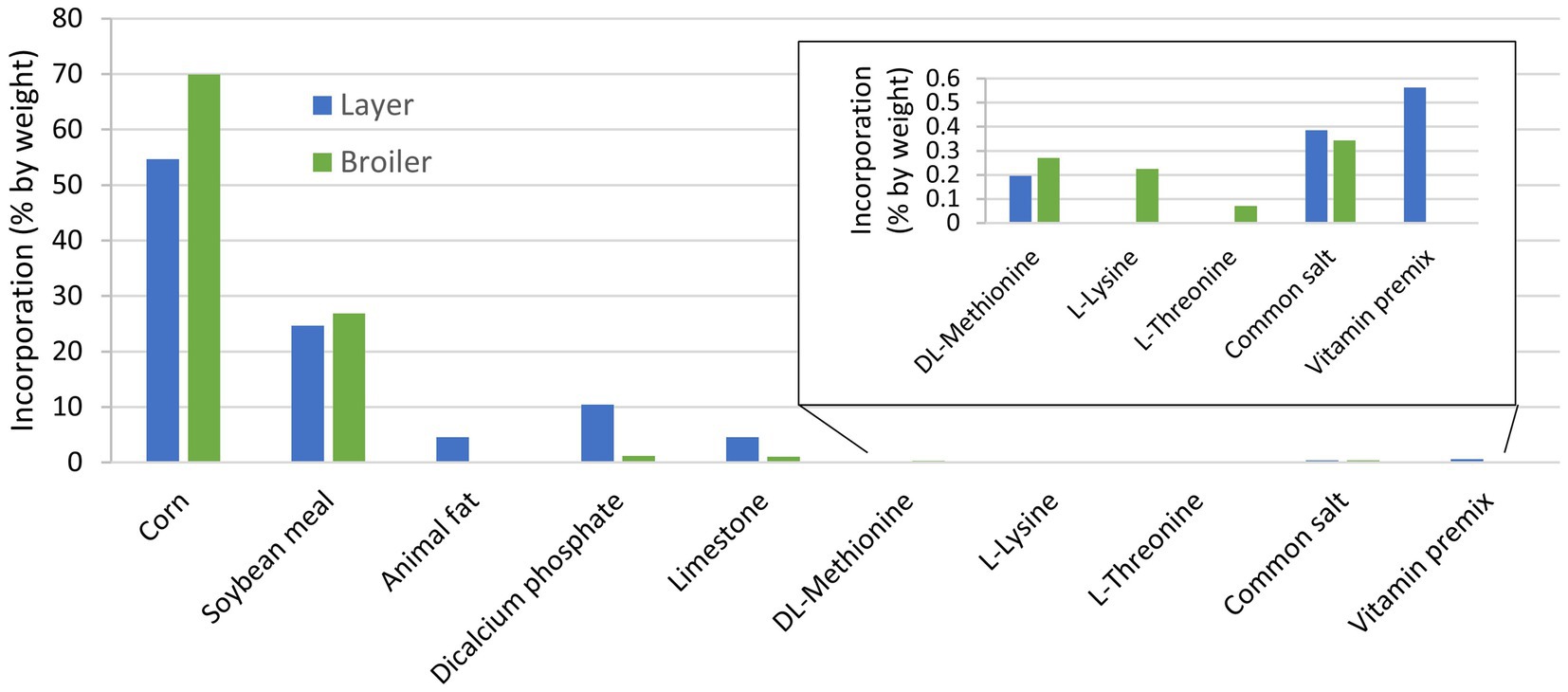
Figure 2. Composition of baseline feed rations (i.e., lacking inclusion of DRHF) for broilers and layers.
Ration formulations with maximal DRHF inclusion resulted from linear programming with no upper limit restrictions for any target nutrient. Under these criteria, DRHF incorporation ranged 51–85% in the broiler rations, with a mean of 70%, and 22–79% in the layer rations, with a mean of 64% (Figures 3A, 4A). Compared to the baseline rations, the near total displacement of corn was the primary effect of DRHF inclusion (Figures 3C, 4C). The rations developed for broilers and layers met or exceeded all required nutrient levels (Figures 3E, 4E). However, ration formulations with maximal inclusion of DRHF often showed elevated sodium and DEB levels. As a result, limits on sodium, potassium, and DEB were imposed in WUFFDA2.1 as described in the methods and rations were reformulated.
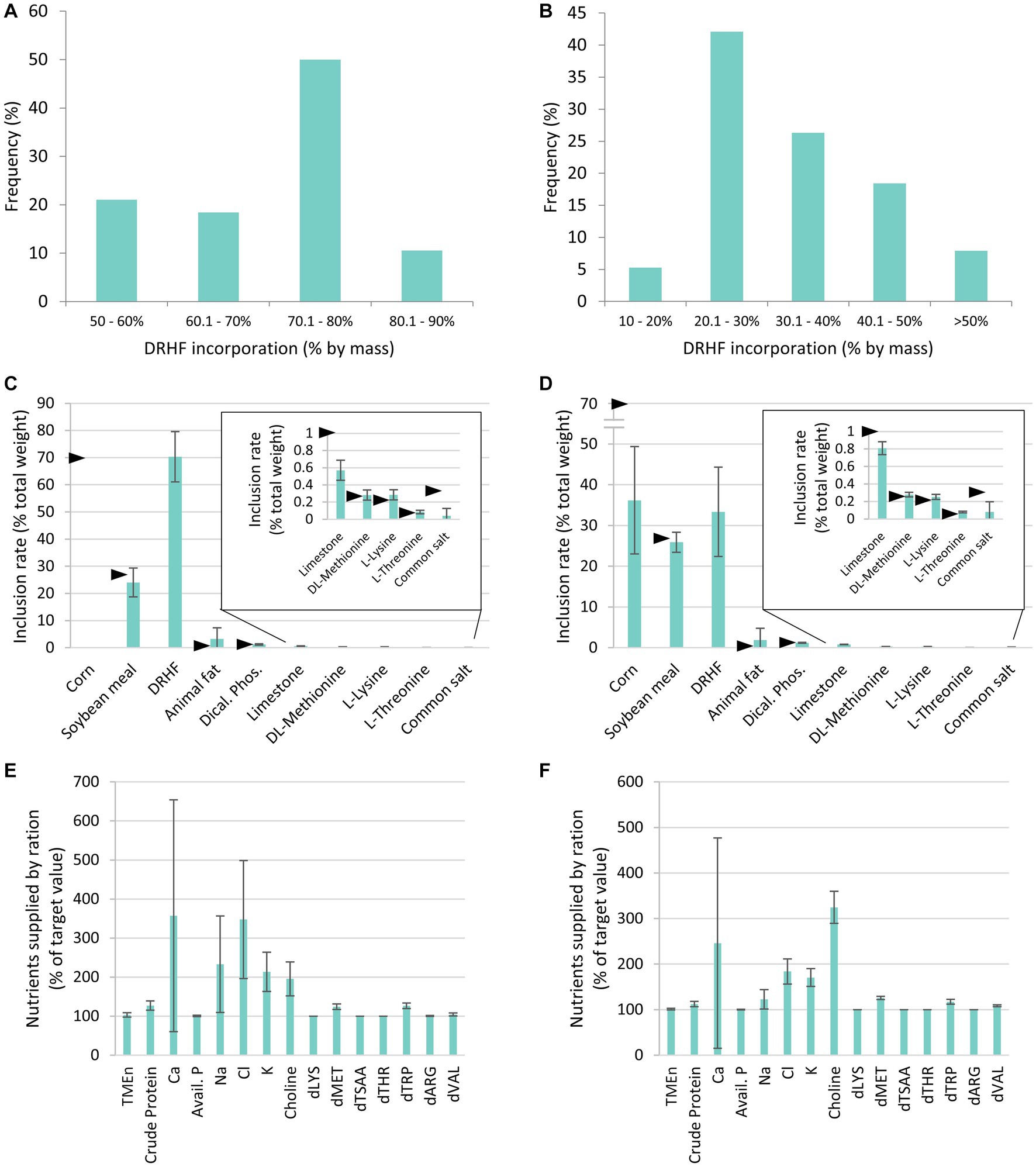
Figure 3. Incorporation of DRHF into Cobb 500 broiler finisher feed rations. Results represent DRHF inclusion without upper limit constraints for ration nutrient targets (A,C,E) and with maximum sodium and potassium limits of 0.23 and 1.11% imposed, respectively (B,D,F). Data show a histogram of DRHF inclusion in the formulated rations (A,B), the ingredient distribution in the ration formulations (C,D), and agreement with ration nutrient targets (E,F). Error bars represent one standard deviation. Arrows in C and D indicate the level of each ingredient in the baseline ration that lacks DRHF.
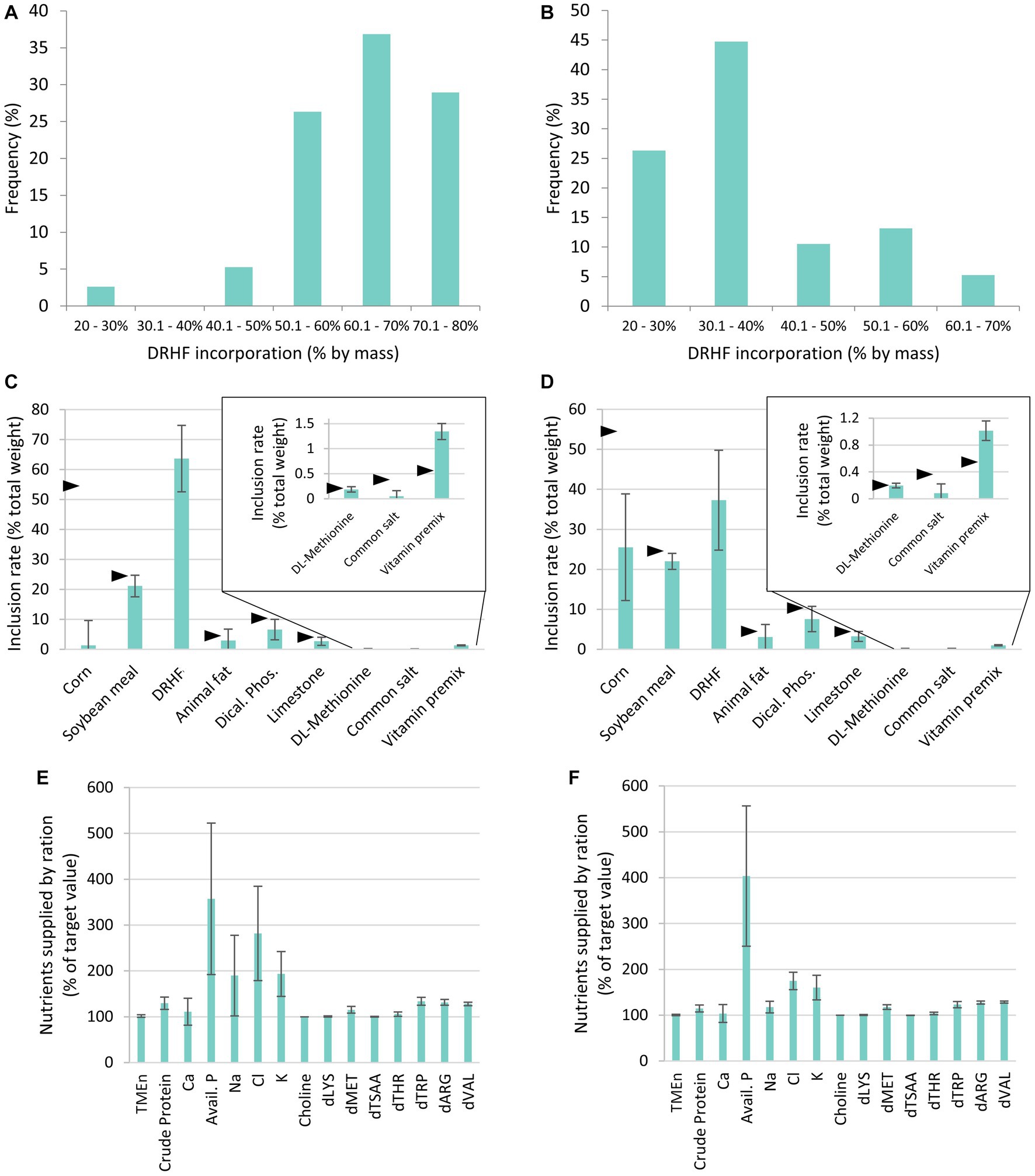
Figure 4. Incorporation of DRHF into Hy-Line W36 layer 3 feed rations. Results represent DRHF inclusion without upper limit constraints for ration nutrient targets (A,C,E) and with maximum sodium and potassium limits of 0.23 and 1.11% imposed, respectively (B,D,F). Data show a histogram of DRHF inclusion in the formulated rations (A,B), the ingredient distribution in the ration formulations (C,D), and agreement with ration nutrient targets (E,F). Error bars represent one standard deviation. Arrows in C and D indicate the level of each ingredient in the baseline ration that lacks DRHF.
The reformulated rations satisfied all nutritional requirements while maintaining tolerable sodium levels and electrolyte balance. For the broiler rations, DRHF incorporation spanned 15–68% with a mean value of 33% (Figure 3B). The broiler rations contained similar average levels of corn and DRHF with an average soybean meal content of 26% (Figure 3D). The rations satisfied all nutrient criteria, with levels close to the target values for most nutrients (Figure 3F). Calcium levels in the rations were most variable. Sodium, chloride, and potassium levels remained higher than the target values; however, the reformulation criteria maintained a tolerable average DEB of 264 ± 31 mEq/kg, with none of the individual formulations exceeding a DEB of 300 mEq/kg. Multivariate analysis of the various broiler ration compositions revealed significant correlations between DRHF incorporation and other ingredient levels (Figure 5). Namely, there were significant negative relationships between DRHF and corn levels (p < 0.0001) and DRHF and limestone levels (p < 0.0001).
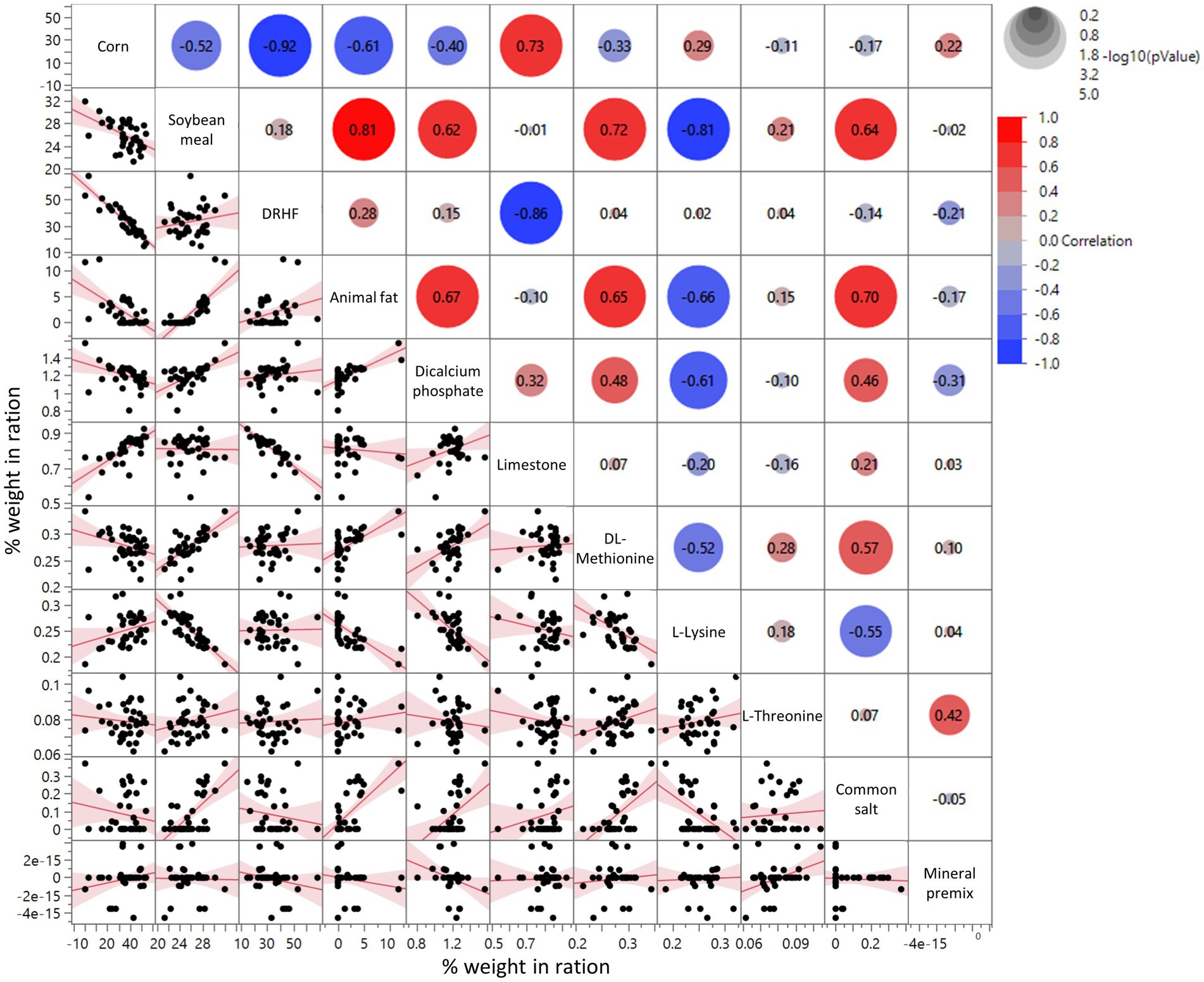
Figure 5. Scatterplot matrix for feed ingredients in formulated Cobb 500 broiler finisher rations. Numbers within colored circles denote the Pearson correlation coefficients while the circle diameters relate to the p-values for the correlations. The intensity of the blue and red coloring in the circles indicate the degree of positive and negative correlation, respectively. Shaded areas around regression lines show 95% confidence intervals.
Similar to the broiler rations, the reformulated layer rations contained an average DRHF incorporation rate of 37% based on individual rates that spanned 21–69% (Figure 4B). Corn was the next greatest ingredient at 26%, followed by soybean meal at 22% (Figure 4D). Most nutrient levels in the rations were close to the target values, with available phosphorus being the exception, showing high variability and a mean level of 400% of the target value (Figure 4F). Chloride and potassium were also elevated, but, as with the broiler rations, the DEB was in the tolerable range, having a mean value of 249 ± 38 mEq/kg. Multivariate analysis indicated that DRHF level had significant negative correlations with corn (p < 0.0001), limestone (p = 0.002), and dicalcium phosphate (p = 0.006; Figure 6). Additionally, the level of DRHF exhibited a significant positive relationship with the vitamin premix level (p < 0.0001).
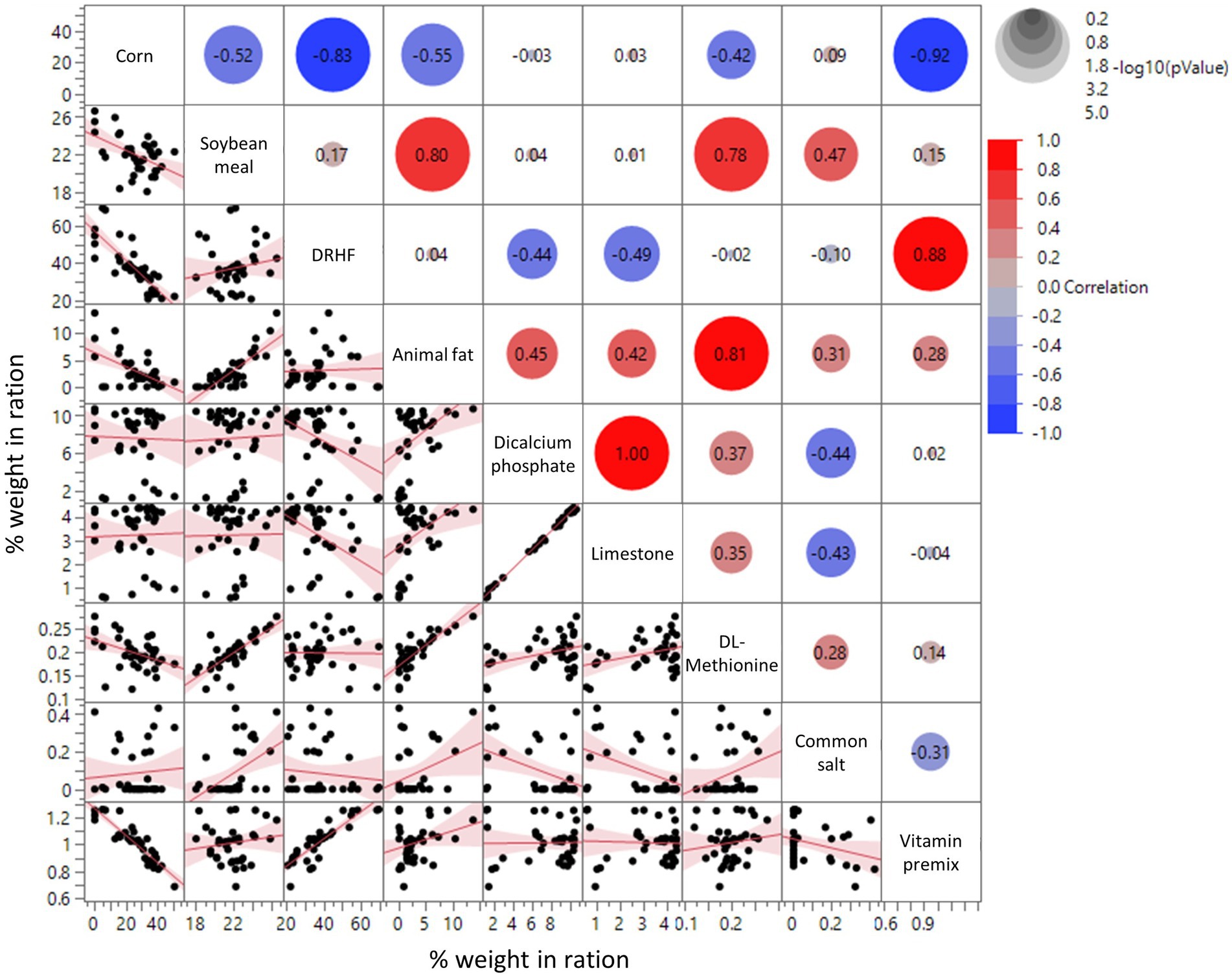
Figure 6. Scatterplot matrix for feed ingredients in formulated Hy-Line W-36 layer 3 rations. Numbers within colored circles denote the Pearson correlation coefficients while the circle diameters relate to the p-values for the correlations. The intensity of the blue and red coloring in the circles indicate the degree of positive and negative correlation, respectively. Shaded areas around regression lines show 95% confidence intervals.
4. Discussion
Compositional data for DRHF indicated that they contain more protein, fat, calcium, sodium and chloride than corn, with TMEn comparable to corn but higher than that in soybean meal. As a result, the DRHF have the potential to be a suitable energy ingredient in poultry feed (National Research Council, 1994). Specifically, formulated broiler and layer rations containing DRHF satisfied the minimum nutrient requirements as specified by poultry genetics companies (e.g., Cobb and Hyline) and the National Research Council (1994). By comparing the baseline rations and formulated rations with DRHF (Figures 1, 3D, 4D), it was observed that the DRHF displaced approximately 50% of the corn required in both broiler and layer rations on average. Additionally, the inclusion of DRHF was predicted to partially replace soybean meal (average of 3.7 to 10.8% decrease depending on ration), limestone (average of 20.2 to 28.6% decrease depending on ration), and common salt (76.2 to 78.0% decrease depending on ration) in broiler and layer rations. Unique to the layer ration formulations, DRHF partially displaced animal fat (33% decrease) and dicalcium phosphate (27% decrease), but required additional inputs of vitamin premix (80% increase) and methionine (1.3% increase). The increase in vitamin premix stemmed from the absence of choline data for DRHF; additional vitamin premix was required to compensate for the lost choline in the corn displaced by DRHF. Future measurement of choline in DRHF may show that less choline supplementation is needed, particularly if DRHF choline levels are comparable to corn. Conversely, DRHF inclusion in the broiler ration was predicted to require additional animal fat (1,800% increase); while a notable fold-change, this represents an increase of 0.1 to 1.8% animal fat in the ration. Additionally, the broiler rations with DRHF were predicted to require increased supplementation with methionine, lysine, and threonine, with average increases in these ingredients spanning 3 to 12%. This is primarily due to the higher protein required in a broiler diet as opposed to a layer diet, which requires higher energy relative to protein.
From an economic perspective, least cost feed formulation within NRC guidelines is the primary incentive for the poultry industry. Considering the quantity of each conventional ingredient displaced by DRHF, along with any additional ingredient supplementation, a coarse estimate of the value of DRHF can be calculated. Using the default ingredient costs in WUFFDA2.1, the net cost displaced by DRHF was $159.25/ton DRHF and $226.05/ton DRHF for the broiler and layer rations, respectively. At the time of this writing in December 2022, corn prices have risen to $319.72/ton and soybean meal sells at $489.97/ton [United States dollars, values are averages from monthly price data spanning January to October, 2022; data sourced from the Federal Reserve Economic Data (2022)]. Keeping all other ingredient prices at their WUFFDA2.1 defaults, current prices for corn and soybean meal would raise the minimum value of DRHF to $305.97/ton DRHF and $341.60/ton DRHF when included in broiler and layer rations, respectively.
Notably, the formulated rations showed highly variable levels of calcium (broiler rations) or available phosphorus (layer rations), owing to the differences in diet requirements and the variability in the levels of these elements within DRHF. Research has shown that the ratio of calcium to available phosphorus, rather than calcium levels alone, can influence broiler growth performance (Gautier et al., 2017). For formulated broiler rations, the average calcium:available phosphorus ratio was 4.91 (1.82% Ca/0.37% available P). Prior work has shown that a ratio of 4.28 (0.77% Ca/0.18% available P) did not significantly affect the growth of finishing broilers (Rousseau et al., 2012). Conversely, another study observed that a ratio of 4.07 (1.1% Ca/0.27% available P) resulted in decreased growth, but that ratios in the 1.2 to 2.2 range did not show a negative growth effect (Li et al., 2012). Examination of the Ca:available P distribution for the formulated rations containing DRHF showed that approximately one fourth of the formulations had Ca:available p values in the 1.9 to 2.2 range (Figure 7). Approximately, 40% of broiler rations containing DRHF had ratios below 3. As a result, only a subset of DRHF with suitably low Ca may be appropriate for broiler rations. Alternatively, DRHF with higher Ca may require blending with lower Ca DRHF to achieve desired Ca:available P levels in the final ration. For layers, the ratio of calcium to available phosphorus in the rations containing DRHF (2.78,1) has not been tested in feeding trials. Previous research has examined calcium to available phosphorus ratios down to 3.87:1 and found no significant impact on body weight, feed conversion, egg production, or egg properties (Hassan and Al Aqil, 2015). Additionally, excess phosphorus can be excreted by the kidneys but may require careful management of the excreta to avoid eutrophication (Waldroup, 1999). Nevertheless, DRHF are compositionally unique relative to other feed ingredients and in vivo feed trials will be required to determine if additional management of the calcium levels is needed.
Based on the results of microbiological, heavy metal, biogenic amine, and mycotoxin analyzes, minimal concerns were identified for the use of DRHF in chicken feed. The fumonisin B1 concentration (0.3 ppm) in the sole DRHF sample that contained a detectable quantity was considered acceptable in animal feeds based on FDA guidance (US Food and Drug Administration, 2001). The highest measured ochratoxin level in the DRHF samples was 5.8 ppb. Although the US FDA has not established guidance for ochratoxin levels in animal feed (US Food and Drug Administration, 2016), the highest observed ochratoxin level was lower than the limit established for ochratoxin in the European Union (100 ppb; European Commission, 2016).
Biogenic amines are metabolites that can be formed during the putrefaction of organic matter. The DRHF did not show detectable levels of certain biogenic amines known to affect poultry health. Specifically, there was an absence of histamine and cadaverine, which have been observed to cause reduced food intake or malabsorption of nutrients in chickens (Saadoun et al., 1997; Barnes et al., 2001). Spermine was not detected in any DRHF samples but is known to be linked to both toxicity and growth promoting effects in chickens, depending on concentration (Sousadias and Smith, 1995). Similar effects have been detected for spermidine and putrescine in chicken feed. For spermidine, inclusion at 0.05% in the diet was found to significantly increase growth rate in chicks while levels at or above 0.4% inhibited growth (Smith et al., 1996). However, for the 2 out of 38 DRHF samples with detectable spermidine, the levels (approximately 0.0006%) were orders of magnitude below the concentrations associated with either effect. Similarly, low inclusion of putrescine (0.03%) in the feed of growing broilers improved the energy efficiency ratio (Hashemi et al., 2014) whereas high levels (≥0.2%) led to significantly impaired feed consumption and egg mass in layers (Chowdhury and Smith, 2001). In the 4 of 38 DRHF samples that showed measurable putrescine, the greatest observed level was 0.0012%, well below the known inhibitory level. Tyramine was the most frequently observed biogenic amine (detected in 8 of 38 samples), with levels ranging 5.3 to 21.2 ppm in positive samples. There is limited research about the animal health effects of tyramine in chicken feed. However, as it is a product of meat putrefaction, tyramine has been studied in fermented carcasses that may be used to generate feed additives. In this work, 550 ppm was proposed as the limit for animal feed to avoid toxicity (Tamim and Doerr, 2000). The highest observed DRHF tyramine level, 21.2 ppm, was over an order of magnitude below this limit. Combined, these results indicate that the in-home food residue drying strategy was successful in stabilizing the food residues before excessive putrefaction and biogenic amine generation could occur.
Peroxide values measure the concentrations of peroxides and hydroperoxides, while p-anisidine values reflect the concentration of aldehydes, the oxidation products in fat. Both metrics are commonly used to determine the oxidative status of fat and oil in animal feed (Danowska-Oziewicz and Kaprińska-Tymoszcyk, 2005; Shurson et al., 2015). However, p-anisidine value is considered to be the more useful metric since it directly captures the oxidative products. The Totox value is a function of both the peroxide and p-anisidine values. Freshly refined oils usually have a peroxide value lower than 1 meq/kg oil; values above 10 meq/kg oil are considered to be oxidized (Gunstone, 2009; Frankel, 2012). Fresh oils and fats typically have p-anisidine values lower than 4. Oxidized fats and oils are indicated by p-anisidine values above 6 (Esfarjani et al., 2019). The mean peroxide, p-anisidine, and Totox values observed in the DRHF were consistent with those found in heavily used cooking oil (Sebastian et al., 2014), indicating a high level of oxidation. Presently, it is unclear if the oxidation was present in the original food material, perhaps owing to cooking, or was introduced through the foods residue heating and drying process. However, researchers have highlighted that no single metric can encompass all effects of oil and fat oxidation and their relevance to animal health (Liu et al., 2014). While broilers fed diets including highly oxidized oil exhibited decreased growth after 2 weeks of feeding (Enberg et al., 1996), the peroxide value (156 meq/kg fat) was considerably greater than the average value in DRHF (6.9 meq/kg fat across all samples). Another study showed that, broilers fed diets that included heavily oxidized corn oil (p-anisidine values up to 150) showed a 2.5 to 4.2% decrease in apparent ME but there was no measurable effect on body weight gain, feed intake, feed efficiency, or abdominal fat pad weight when compared against chickens fed a control diet (Ehr et al., 2015).
While nutritional and toxicological compatibility are crucial requirements for realizing commercial use of DRHF in poultry feed, there are additional drivers and targets for future work that can help to highlight the sustainability and food security benefits of utilizing DRHF in poultry production. Existing life cycle assessments show that conversion of recovered food into dry animal feed can deliver pronounced reductions in greenhouse gas emissions compared to landfill disposal. Emissions reductions were on par with, or in some cases exceeded, reductions achieved through diversion from landfilling to composting (Shurson, 2020). Routing of DRHF into poultry feed may lower emissions compared to landfilling by decreasing the water weight and thus the fuel needed to transport the recovered food as well as avoiding methane production from anaerobic digestion in landfills. Displacing a fraction of conventional poultry feed ingredients can also avoid the embedded emissions associated with their cultivation, processing, and distribution. The energy use to dry recovered household food is a determinant of the carbon footprint for DRHF production. As a result, future work to optimize the efficiency of the drying process and understand the impact of the local electricity grid energy profile (specifically, the mix of renewable versus non-renewable sources) on the embedded emissions during drying will be important for maximizing environmental benefits. Moreover, while this study focused on DRHF generated from households across the United States, the DRHF-to-poultry feed pathway also warrants investigation in developing countries as a potential mitigating strategy for conventional feed supply chain disruptions, which can severely affect poultry production in these regions (Attia et al., 2022).
5. Conclusion
This study provides the first detailed report on the proximate, chemical, toxicological, and digestibility properties of recovered food from households that has been processed using commercially available in-home drying devices. Here, the data were used to assess compatibility of DRHF with chicken feed. However, these data may also enable ration formulations for other livestock as well as facilitate research into other bioconversion strategies to valorize DRHF.
The feed modeling results presented in this study can inform future in vivo feeding studies to investigate the performance of chicken feed rations containing DRHF. The results of this work suggest that DRHF is a suitable nutrient source for poultry. Specifically, linear programming to formulate chicken feeds that include DRHF alongside conventional feed ingredients indicated that average DRHF inclusion rates up to 33 to 37% (by dry weight) can be achieved in broiler finisher and layer diets, meeting nutritional requirements while avoiding excessive sodium and electrolyte balance issues. Predicted calcium and available phosphorus levels in the formulated broiler and layer rations, respectively, were highly variable. For available phosphorus, the average level was outside the concentrations examined in prior feeding studies. DRHF screening and aggregation strategies, along with consideration of additional formulation algorithms, may help to control the variability of these nutrients in rations. These strategies could include measuring calcium and available phosphorus levels in individual batches of DRHF and either rejecting those with excessive levels or selectively aggregating them with other DRHF batches that are lower in calcium and phosphorus to achieve acceptable levels.
This initial assessment of feed potential focused on major dietary nutritional requirements; however, a complete assessment of available micronutrients (vitamins and trace minerals) should also be performed for DRHF ahead of in vivo feed trials. Additionally, while the ration formulations focused on Cobb 500 broilers and Hy-Line W-36 layers, these breeds have similar nutrition requirements to other popular breeds (e.g., Hy-Line W-80 and Hy-Line Brown for layers, Cobb 700 for broilers). As a result, the developed rations provide insight into the potential to incorporate DRHF into a broad range of chicken feed rations.
The toxicological screening results indicated that there were no detectable levels of heavy metals, common foodborne pathogens, and most major mycotoxins in rations containing DRHF. Sparsely detected levels of fumonisin B1 and ochratoxin were below concentrations that are expected to be hazardous. Biogenic amines were largely absent in the DRHF and those that were detected were present at levels that are not expected to impact chicken growth or performance. Indicators of fat and oil oxidation were consistent with levels in the literature that have not affected broiler performance. As a result, it is likely that DRHF can be safely included in poultry feed.
Based on the net cost displaced by the DRHF in each ration, the minimum potential value of the DRHF is expected to fall between that of corn and soybean meal. To better understand the economic incentives associated with DRHF incorporation into chicken feed, additional analysis is warranted to examine the minimum potential value of DRHF in relation to the conventional feed ingredients that it displaces, considering previously observed or anticipated changes for the prices of these ingredients. Understanding more granular economic decision making, including regional pricing, spot markets and alternative ingredients, will be necessary to better evaluate the true economic value of this alternative feed. The displacement of conventional feed ingredients also has sustainability implications that may enhance the value of DRHF. The feed formulations identified in this work can inform life cycle assessments to quantify changes in land use, resource inputs, and environmental impacts due to the inclusion of DRHF in animal feed in lieu of other cultivated ingredients, such as corn. Together, the results of the present work and those of future related studies may motivate policy and industry action to promote the use of DRHF in animal feed.
Data availability statement
The original contributions presented in the study are included in the article/Supplementary material, further inquiries can be directed to the corresponding author.
Ethics statement
The requirement of ethical approval was waived by the UC Davis Institutional Review Board for the studies involving humans. The studies were conducted in accordance with the local legislation and institutional requirements. The participants provided their written informed consent to participate in this study.
Author contributions
GH and AS designed and conducted the survey of DRHF and managed measurement of DRHF properties. YL, MP, CS, and ZT conducted the DRHF safety and feed nutrition assessments. JG performed the DRHF cost assessment. YL, MP, AS, and CS wrote the manuscript. All authors contributed to the article and approved the submitted version.
Funding
This work was funded by a grant from Mill Industries, Inc. (previously known as Chewie Labs, Inc.) (UC Davis project #A23-2260).
Acknowledgments
The authors thank Gerald Shurson for advice on selecting chemical and biological assays that are relevant to inclusion of DRHF in animal feed, and Alexandra Niedzwiecki for assistance with coordinating processing and analysis of DRHF samples. The authors also thank Jennifer van de Ligt, Janet Snow, Hilary Pavlidis, Scott Andrews, Kristi Smedley, and Nicole Civita for editorial guidance on framing poultry feed and food waste issues.
Conflict of interest
AS and GH were employed by Mill Industries, Inc. CS serves as a consulting scientific advisor to Mill Industries, Inc.
The remaining authors declare that the research was conducted in the absence of any commercial or financial relationships that could be construed as a potential conflict of interest.
Publisher’s note
All claims expressed in this article are solely those of the authors and do not necessarily represent those of their affiliated organizations, or those of the publisher, the editors and the reviewers. Any product that may be evaluated in this article, or claim that may be made by its manufacturer, is not guaranteed or endorsed by the publisher.
Supplementary material
The Supplementary material for this article can be found online at: https://www.frontiersin.org/articles/10.3389/fsufs.2023.1180249/full#supplementary-material
References
Adedokun, S. A., Jaynes, P., Payne, R. L., and Applegate, T. J. (2015). Standardized ileal amino acid digestibility of corn, corn distillers’ dried grains with solubles, wheat middlings, and bakery by-products in broilers and laying hens. Poult. Sci. 94, 2480–2487. doi: 10.3382/ps/pev226
Ahmad, T., Mushtaq, T., Khan, M. A., Babarm, M. E., Yousaf, M., Hasan, Z. U., et al. (2009). Influence of varying dietary electrolyte balance on broiler performance under tropical summer conditions. J. Anim. Physiol. Anim. Nutr. 93, 613–621. doi: 10.1111/j.1439-0396.2008.00840.x
Alhotan, R., and Pesti, G. (2016). Windows user-friendly feed formulation 2.1. Athens, GA: The University of Georgia.
Altizio, B. A., Westendorf, M. L., and Schoknecht, P. A. (2000). Comparison of a corn/soybean diet versus a dried, recycled food waste product in swine. The Professional Animal Scientist 16, 254–260. doi: 10.15232/S1080-7446(15)31708-3
Alvarenga, R. R., Rodrigues, P. B., Zangeronimo, M. G., Freitas, R. T. F., Lima, R. R., Bertechini, A. G., et al. (2011). Energetic values of feedstuffs for broilers determined with in vivo assays and prediction equations. Anim. Feed Sci. Technol. 168, 257–266. doi: 10.1016/j.anifeedsci.2011.04.092
Arias, P., Bellouin, N., Coppola, E., Jones, R., Krinner, G., Marotzke, J., et al. (2021). Climate change 2021: the physical science basis. Contribution of working Group14 I to the sixth assessment report of the intergovernmental panel on climate change; Technical Summary.
Attia, Y. A., Rahman, M. T., Hossain, M. J., Basiouni, S., Khafaga, A. F., Shehata, A. A., et al. (2022). Poultry production and sustainability in developing countries under the COVID-19 crisis: lessons learned. Animals 12:644. doi: 10.3390/ani12050644
Barnes, D. M., Kirby, Y. K., and Oliver, K. G. (2001). Effects of biogenic amines on growth and the incidence of proventricular lesions in broiler chickens. Poult. Sci. 80, 906–911. doi: 10.1093/ps/80.7.906
Benavides, P. T., Cai, H., Wang, M., and Bajjalieh, N. (2020). Life-cycle analysis of soybean meal, distiller-dried grains with solubles, and synthetic amino acid-based animal feeds for swine and poultry production. Anim. Feed Sci. Technol. 268:114607. doi: 10.1016/j.anifeedsci.2020.114607
Blecha, J., and Leitner, H. (2014). Reimagining the food system, the economy, and urban life: new urban chicken-keepers in US cities. Urban Geogr. 35, 86–108. doi: 10.1080/02723638.2013.845999
Breewood, H., and Garnett, T. (2020). What is feed-food competition. Foodsource: Building blocks. Oxford, UK: Food Climate Research Network, University of Oxford.
Chowdhury, S. R., and Smith, T. K. (2001). Effects of dietary 1, 4-diaminobutane (putrescine) on eggshell quality and laying performance of older hens. Poult. Sci. 80, 1208–1214. doi: 10.1093/ps/80.8.1208
Cobb-Vantress, L. L. C. . (2022). Cobb500 broiler performance and nutrition supplement (2022). Available at: https://www.cobb-vantress.com/assets/Cobb-Files/product-guides/5502e86566/2022-Cobb500-Broiler-Performance-Nutrition-Supplement.pdf. (Accessed December 2022).
Danowska-Oziewicz, M., and Kaprińska-Tymoszcyk, M. (2005). Quality changes in selected frying fats during heating in a model system. J. Food Lipids 12, 159–168. doi: 10.1111/j.1745-4522.2005.00014.x
Di Paola, A., Rulli, M. C., and Santini, M. (2017). Human food vs. animal feed debate. A thorough analysis of environmental footprints. Land Use Policy 67, 652–659. doi: 10.1016/j.landusepol.2017.06.017
Dou, Z., Toth, J. D., and Westendorf, M. L. (2018). Food waste for livestock feeding: feasibility, safety, and sustainability implications. Glob. Food Sec. 17, 154–161. doi: 10.1016/j.gfs.2017.12.003
Ehr, I. J., Kerr, B. J., and Persia, M. E. (2015). Effects of peroxidized corn oil on performance, AMEn, and abdominal fat pad weight in broiler chicks. Poult. Sci. 94, 1629–1634. doi: 10.3382/ps/pev131
Elkhoraibi, C., Blatchford, R. A., Pitesky, M. E., and Mench, J. A. (2014). Backyard chickens in the United States: a survey of flock owners. Poult. Sci. 93, 2920–2931. doi: 10.3382/ps.2014-04154
Enberg, R. M., Lauridsen, C., Jensen, S. K., and Jakobsen, K. (1996). Inclusion of oxidized vegetable oil in broiler diets. Its influence on nutrient balance and on the antioxidative status of broilers. Poult. Sci. 75, 1003–1011. doi: 10.3382/ps.0751003
Engster, H. M., Cave, N. A., Likuski, H., McNab, J. M., Parsons, C. A., and Pfaff, F. E. (1985). A collaborative study to evaluate a precision-fed rooster assay for true amino acid availability in feed ingredients. Poult. Sci. 64, 487–498. doi: 10.3382/ps.0640487
Esfarjani, F., Khoshtinat, K., Zargaraan, A., Mohammadi-Nasrabadi, F., Salmani, Y., Saghafi, Z., et al. (2019). Evaluating the rancidity and quality of discarded oils in fast food restaurants. Food Sci. Nutr. 7, 2302–2311. doi: 10.1002/fsn3.1072
European Commission (2016). Commission recommendation (EU) 2016/1319 of 29 July 2016 amending recommendation 2006/576/EC as regards deoxynivalenol, zearalenone and ochratoxin a in pet food. Off. J. Eur. Union 208, 58–60.
Federal Reserve Economic Data . (2022). Federal Reserve Bank of St. Louis. Available at: https://fred.stlouisfed.org/. (Accessed December 2022).
Frankel, E. N. (2012). “Methods to determine extent of oxidation” in Lipid oxidation. ed. E. N. Frankel . 2nd ed (Sawston, UK: Woodhead Publishing Limited), 99–127.
Gautier, A. E., Walk, C. L., and Dilger, R. N. (2017). Influence of dietary calcium concentrations and the calcium-to-non-phytate phosphorus ratio on growth performance, bone characteristics, and digestibility in broilers. Poult. Sci. 96, 2795–2803. doi: 10.3382/ps/pex096
Gezen, S. S., Eren, M., and Deniz, G. (2005). The effect of different dietary electrolyte balances on eggshell quality in laying hens. Rev. Med. Vet. 156, 491–497.
Hall, G., Rothwell, A., Grant, T., Isaacs, B., Ford, L., Dixon, J., et al. (2014). Potential environmental and population health impacts of local urban food systems under climate change: a life cycle analysis case study of lettuce and chicken. Agric. Food Secur. 3, 1–13. doi: 10.1186/2048-7010-3-6
Hashemi, S. M., Loh, T. C., Foo, H. L., Zulkifli, I., and Bejo, M. H. (2014). Effects of putrescine supplementation on growth performance, blood lipids and immune response in broiler chickens fed methionine deficient diet. Anim. Feed Sci. Technol. 194, 151–156. doi: 10.1016/j.anifeedsci.2014.05.008
Hassan, S. M., and Al Aqil, A. A. (2015). Effect of adding different dietary calcium to available phosphorus ratios on productive performance and eggshell quality of white egg layer hens. Int. J. Poult. Sci. 14, 103–111. doi: 10.3923/ijps.2015.103.111
Hy-Line International . (2020) W-36 commercial layers management guide. Available at: https://www.hyline.com/filesimages/Hy-Line-Products/Hy-Line-Product-PDFs/W-36/36%20COM%20ENG.pdf. (Accessed December 2022).
Jaglo, K., Kenny, S., and Stephenson, J. (2021). From farm to kitchen: The environmental impacts of US. Washington, DC: Food Waste EPA, Environmental Protection Agency.
Lam, C. M., Iris, K. M., Hsu, S. C., and Tsang, D. C. (2018). Life-cycle assessment on food waste valorisation to value-added products. J. Clean. Prod. 199, 840–848. doi: 10.1016/j.jclepro.2018.07.199
Lee, U., Han, J., and Wang, M. (2017). Evaluation of landfill gas emissions from municipal solid waste landfills for the life-cycle analysis of waste-to-energy pathways. J. Clean. Prod. 166, 335–342. doi: 10.1016/j.jclepro.2017.08.016
Li, J., Yuan, J., Guo, Y., Sun, Q., and Hu, X. (2012). The influence of dietary calcium and phosphorus imbalance on intestinal NaPi-IIb and calbindin mRNA expression and tibia parameters of broilers. Asian Australas. J. Anim. Sci. 25, 552–558. doi: 10.5713/ajas.2011.11266
Liu, P., Kerr, B. J., Chen, C., Weber, T. E., Johnston, L. J., and Shurson, G. C. (2014). Methods to create thermally oxidized lipids and comparison of analytical procedures to characterize peroxidation. J. Anim. Sci. 92, 2950–2959. doi: 10.2527/jas.2012-5708
Mongin, P. (1981). Recent advances in dietary anion-cation balance: applications in poultry. Proc. Nutr. Soc. 40, 285–294. doi: 10.1079/PNS19810045
Mushtaqi, M. M. H., Pasha, T. N., Mushtaqi, T., and Parvin, R. (2013). Electrolytes, dietary electrolyte balance and salts in broilers: an updated review on growth performance, water intake and litter quality. Worlds Poult. Sci. J. 69, 789–802. doi: 10.1017/S0043933913000810
Myer, R. O., Brendemuhl, J. H., and Johnson, D. D. (1999). Evaluation of dehydrated restaurant food waste products as feedstuffs for finishing pigs. J. Anim. Sci. 77, 685–692. doi: 10.2527/1999.773685x
Myer, R. O., Johnson, D. D., Boswick, K. K., and Brendemuhl, J. H. (1998). “Dehydration of restaurant food wastes produces a nutritious feedstuff for use in pig diets” in Beneficial co-utilization of agricultural, municipal and industrial by-products. eds. A. Brown, J. S. Angle, and L. Jacobs (Berlin: Springer), 397–403.
Nascimento, G. A. J. (2007). Prediction equations of the energetic values of poultry feedstuffs for utilizing the meta-analysis principle. PhD Thesis University Lavras, Minas Gerais, Brazil.
National Animal Nutrition Program . (2022). Feed composition database. Available at: https://animalnutrition.org/feed-composition-database-signup?destination=feed-composition-database. (Accessed November 2022).
National Research Council . (1994). Nutrient requirements of poultry. 9th Edn. Washington, DC: National Academy Press. p. 2114.
National Research Council . (2012). Nutrient requirements of swine. 11th Edn. Washington, DC: National Academy Press. p. 13298.
Parsons, C. M., Potter, L. M., and Bliss, B. A. (1982). True metabolizable energy corrected to nitrogen equilibrium. Poult. Sci. 61, 2241–2246. doi: 10.3382/ps.0612241
Rivas, M. E., Brendemuhl, J. H., Johnson, D. D., and Myer, R. O. (1994). Digestibility by swine and microbiological assessment of dehydrated edible restaurant waste. Res. Rep. Al-1994-3. College of Agriculture. Florida Agricultural Experiment Station. University of Florida, Gainesville.
Rousseau, X., Létourneau-Montminy, M. P., Meme, N., Magnin, M., Nys, Y., and Narcy, A. (2012). Phosphorus utilization in finishing broiler chickens: effects of dietary calcium and microbial phytase. Poult. Sci. 91, 2829–2837. doi: 10.3382/ps.2012-02350
Saadoun, A., Alvarez, A. F., and Cabrera-Saadoun, M. C. (1997). Effect of acute histamine and pentagastrin on voluntary food intake in growing chickens. Appl. Anim. Behav. Sci. 55, 129–136. doi: 10.1016/S0168-1591(97)00003-8
Schader, C., Muller, A., Scialabba, N. E. H., Hecht, J., Isensee, A., Erb, K. H., et al. (2015). Impacts of feeding less food-competing feedstuffs to livestock on global food system sustainability. J. R. Soc. Interface 12:20150891. doi: 10.1098/rsif.2015.0891
Searchinger, T. D., Wirsenius, S., Beringer, T., and Dumas, P. (2018). Assessing the efficiency of changes in land use for mitigating climate change. Nature 564, 249–253. doi: 10.1038/s41586-018-0757-z
Sebastian, A., Ghazani, S. M., and Marangoni, A. G. (2014). Quality and safety of frying oils used in restaurants. Food Res. Int. 64, 420–423. doi: 10.1016/j.foodres.2014.07.033
Shahidi, F., and Wanasundara, U. N. (2002). “Methods for measuring oxidative rancidity in fats and oils” in Food Science And Technology. eds. C. C. Akoh and D. B. Min (New York: Marcel Dekker), 465–488.
Shurson, G. C. (2020). “What a waste”—can we improve sustainability of food animal production systems by recycling food waste streams into animal feed in an era of health, climate, and economic crises? Sustainability 12:7071. doi: 10.3390/su12177071
Shurson, G. C., Kerr, B. J., and Hanson, A. R. (2015). Evaluating the quality of feed fats and oils and their effects on pig growth performance. J. Anim. Sci. Biotechnol. 6, 1–11. doi: 10.1186/s40104-015-0005-4
Siddiqui, Z., Hagare, D., Jayasena, V., Swick, R., Rahman, M. M., Boyle, N., et al. (2021). Recycling of food waste to produce chicken feed and liquid fertiliser. Waste Manag. 131, 386–393. doi: 10.1016/j.wasman.2021.06.016
Skunca, D., Tomasevic, I., Nastasijevic, I., Tomovic, V., and Djekic, I. (2018). Life cycle assessment of the chicken meat chain. J. Clean. Prod. 184, 440–450. doi: 10.1016/j.jclepro.2018.02.274
Smith, T. K., Mogridge, J. A. L., and Sousadias, M. G. (1996). Growth-promoting potential and toxicity of spermidine, a polyamine and biogenic amine found in foods and feedstuffs. J. Agric. Food Chem. 44, 518–521. doi: 10.1021/jf9503974
Sousadias, M. G., and Smith, T. K. (1995). Toxicity and growth-promoting potential of spermine when fed to chicks. J. Anim. Sci. 73, 2375–2381. doi: 10.2527/1995.7382375x
Su, C. H., Tsai, C. H., Chen, M. H., and Lv, W. Q. (2019). US sustainable food market generation Z consumer segments. Sustainability 11:3607. doi: 10.3390/su11133607
Tamim, N. M., and Doerr, J. A. (2000). Fermentation of broiler carcasses–foolproof or not? Poul. Perspect. 2, 6–7.
Trainer, T., Malik, A., and Lenzen, M. (2019). A comparison between the monetary, resource and energy costs of the conventional industrial supply path and the “simpler way” path for the supply of eggs. Biophys. Econ. Resource Qual. 4, 1–7. doi: 10.1007/s41247-019-0057-8
US Environmental Protection Agency . (2017). Inventory of US greenhouse gas emissions and sinks: 1990–2015. Washington, DC: US Environmental Protection Agency.
US Environmental Protection Agency . (2018). 2018 wasted food report. Available at: https://www.epa.gov/sites/default/files/2020-11/documents/2018_wasted_food_report.pdf
US Food and Drug Administration . (2001). Guidance for industry: Fumonisin levels in human foods and animal feeds. Washington DC: United States Food and Drug Administration.
US Food and Drug Administration . (2016). CVM annual report on mycotoxins in animal food. Washington DC: US Food and Drug Administration.
Verain, M. C., Bartels, J., Dagevos, H., Sijtsema, S. J., Onwezen, M. C., and Antonides, G. (2012). Segments of sustainable food consumers: a literature review. Int. J. Consum. Stud. 36, 123–132. doi: 10.1111/j.1470-6431.2011.01082.x
Waldroup, P. W. (1999). Nutritional approaches to reducing phosphorus excretion by poultry. Poult. Sci. 78, 683–691. doi: 10.1093/ps/78.5.683
Westendorf, M. L. (2000). Food waste as animal feed: an introduction. Food Waste Anim feed 2000, 3–16. doi: 10.1002/9780470290217.ch1
Keywords: circular economy, feed ration formulation, food byproducts, food residue valorization, poultry
Citation: Hill G, Sayadi A, Gendreau JD, Tobar Z, Liu Y, Pitesky ME and Simmons CW (2023) Assessment of the variation in nutritional composition and safety of dried recovered food from United States households and prospects for use in chicken feed. Front. Sustain. Food Syst. 7:1180249. doi: 10.3389/fsufs.2023.1180249
Edited by:
Sampat Ghosh, Andong National University, Republic of KoreaReviewed by:
Michael Westendorf, Rutgers, The State University of New Jersey, United StatesZhengxia Dou, University of Pennsylvania, United States
Copyright © 2023 Hill, Sayadi, Gendreau, Tobar, Liu, Pitesky and Simmons. This is an open-access article distributed under the terms of the Creative Commons Attribution License (CC BY). The use, distribution or reproduction in other forums is permitted, provided the original author(s) and the copyright owner(s) are credited and that the original publication in this journal is cited, in accordance with accepted academic practice. No use, distribution or reproduction is permitted which does not comply with these terms.
*Correspondence: Christopher W. Simmons, Y3dzaW1tb25zQHVjZGF2aXMuZWR1
†These authors have contributed equally to this work