- 1Department of Animal Source Food Technology, Faculty of Agriculture, University of Belgrade, Belgrade, Serbia
- 2Department of Industrial Microbiology, Faculty of Agriculture, University of Belgrade, Belgrade, Serbia
- 3Department of Chemistry and Biochemistry, Faculty of Agriculture, University of Belgrade, Belgrade, Serbia
- 4Department of Food Safety and Quality Management, Faculty of Agriculture, University of Belgrade, Belgrade, Serbia
- 5The Institute for Science Application in Agriculture, Belgrade, Serbia
Two groups of market samples were collected: four samples of whey produced in small scale facilities, and four samples produced in large scale dairy factories. The additional two groups: acid (a) and sweet whey(s) were collected in laboratory from cheeses produced from differently heated goat milk (A—65°C/30 min, B—80°C/5 min and C—90°C/5 min). Gross composition (dry matter content, fat content, protein content), pH, protein, mineral composition and microbial counts were determined. Obtained results for laboratory and market whey samples were analyzed by two-way and one-way ANOVA, respectively. Visualization of quantitative relationships within market and laboratory whey samples has been done by principal component analysis (PCA). Comparison of the protein composition of market samples with those from the laboratory suggested that the majority of goat whey from the market originated from milk heated between 65 and 80°C. While heat treatment of milk affected protein composition, coagulation type determined mineral composition of whey. The amount of Ca content was almost four times higher, while the amount of Zn is more than 15 times higher in acid than in sweet goat whey. The lack of influence of heat treatment on the Ca and Mg content in whey has been detected. Such behavior is the opposite of cow milk behavior, in which with the subsequent increase in heating temperature, the amount of soluble Ca and Mg decreases. For all analyzed samples, dry matter content was in agreement with the legally required minimum level (5.5%). Although legal requirements for safety and quality of small scale dairy products are more flexible than that of the large counterparts, there was not a single characteristic that differed significantly between small scale and large scale market goat whey.
1. Introduction
Whey represents the serum phase of milk comprised of about 55% of milk nutrients, and 85–95% of milk volume. It is usually classified into acid whey and sweet whey, based on a milk coagulation mechanism conducted during cheese production. Sweet whey is obtained mostly from rennet coagulated cheeses, while collection of acid whey implies cheese making that involves either the addition of organic acids to milk or acid coagulation/fermentation conducted by the activity of lactic acid bacteria. As a result of different production processes, these two sub varieties of whey have different functional characteristics and composition (Rocha-Mendoza et al., 2021).
Serbian legislation does not distinguish between sweet and acid liquid whey. According to the Regulation (2014a) whey is described as a by-product from cheese and casein production, produced in accordance with the manufacturer’s specification. The only quality requirement is, that it contains no less than 5.5% of dry matter. High flexibility of legislation implies the hypothesis, that both acid and sweet whey, significantly different in composition and functionality (Nishanthi et al., 2017), would be found on the Serbian market.
Together with a growing demand and popularity of goat dairy products in general (Zhang et al., 2022), a growing demand for liquid goat whey has also been observed in Serbia. Liquid whey that appeared on the market recently, originated from both farm and industry cheese production. Four farm producers (small scale), and four factory producers (large scale) were involved in the present study. The important difference between these two production scales (besides capacity and market opportunities) is that, according to the Regulation (2017) small scale dairy producers in Serbia have less stringent legal requirements. Thus, it is of high importance both for producers and for consumers, to determine whether physico-chemical and microbiological quality of whey from small scale production could be comparable with the one from large scale production.
For producers, and especially for small scale manufacturers, further whey processing for obtaining powdered whey products is an expensive and challenging process (Mehra et al., 2021). Due to the high content of lactic acid and calcium phosphate, in case of acid whey, drying process is even more challenging (Rocha-Mendoza et al., 2021). For those reasons, and many more, goat whey is mostly utilized as food supplement for pigs, as agricultural land fertilizer, but unfortunately it is often spilled into sinks or dumped into rivers, although it is considered as one of the most pollutant by-products in food industry (Moreno-Indias et al., 2009; Barukčić et al., 2014). However, the simple and acceptable solution could be production of whey based beverages (Barukčić et al., 2019). For that purpose, it is highly important to have a deep insight into the constituents of whey from different streams.
Heat treatment is the main milk processing step used in cheese manufacturing (Nishanthi et al., 2017). Heating regimes higher than the conventional pasteurization, promote denaturation of whey proteins, and their covalent interaction with κ-casein at the micelle surface (Lucey, 2016). As a result, acid-coagulated milk products from high heat treated milk tend to be firmer and more viscous, have a lower tendency to syneresis, and are smoother than the corresponding products from conventionally pasteurized milk (Fox et al., 2017). On the contrary, the increase in whey protein denaturation is paralleled by a marked deterioration of rennet-induced gelation properties of cow milk. Such negative effect may be off-set somewhat by higher milk protein content, pre-acidification and/or addition of CaCl2 (Guinee, 2021).
High heat treatments affect goat milk components differently compared to cow milk, in a way that it enables the application of such heating regimes for the production of rennet-coagulated goat cheese without any modification of cheese making process (Hovjecki et al., 2022). Moreover, it has been reported that high pasteurization regimes (90°/5 min) improve sensorial quality and yield of rennet coagulated goat white brined cheese (Miloradovic et al., 2020). Consequently, they affect also the composition of the corresponding whey. The way that heat treatments of milk affect the sweet goat whey composition has been previously studied in brief by the authors of the current study. It has been reported that protein and fat content decreases with the increase of heating temperature. Altering the heat treatment of milk resulted in whey of different gross and protein composition, and thus different biological and functional properties (Miloradovic et al., 2016). Previous studies have also dealt with variations in whey protein composition of cow whey from different streams (sweet, acid, salty and natural whey; Nishanthi et al., 2017). However no study up till the present one aimed to assess properties of goat whey affected by a combination of heat treatment of milk and the type of coagulation (rennet and acid) from which they were yielded. The described assessment was carried out prior to market whey collection and analysis. It aimed to set a framework for the interpretation of Serbian market whey characteristics, but hopefully it could also serve for all future market whey characterizations. After setting the framework, the objective of the present study was to estimate the category in which liquid goat whey from the Serbian market belongs, and also, to compare the quality of whey that originated from small scale production, with the quality of those from large scale production.
2. Material and methods
Raw milk was collected from a commercial flock of 30 Alpine goats, in two consecutive weeks, in May 2022, and processed on the same day it has been produced. Written informed consent was obtained from the flock owners for the participation of their animals in this study.
2.1. Laboratory whey samples collection
Laboratory whey samples were collected after the production of rennet and acid coagulated cheese. Raw milk (15 L) has been portioned into three identical 5 L lots. The range of milk heating regimes (65°C/30 min—A, 80°C/5 min—B, and 90°C/5 min—C) has been kept identical to in the previously published studies (Miloradovic et al., 2016, 2017, 2020).
Three different heating regimes were applied to each lot of raw milk. After heating and cooling milk to 40°C, each lot was further portioned in two equal 2.5 L sub-lots: one to be subjected to rennet coagulation and the other to acid coagulation.
In order to collect sweet whey laboratory samples that originate from different milk heating regimes (As, Bs, and Cs) along with the identical heating regimes, cheese making procedure similar to previously published studies has been applied (Miloradovic et al., 2016; Miloradovic et al., 2017). The single modification was the absence of starter culture in the current experiment.
In order to collect acid way laboratory samples (Aa, Ba, and Ca) the identical cheese making procedure has been applied as in the previously published study (Miloradovic et al., 2018).
2.2. Market whey samples collection
Market whey samples were collected from supermarkets/hypermarkets and local specialized shops. Four collected samples were produced in small scale – dairy households (S1—Dis Todorović, Ražanj; S2—Miloš Erceg, Kucura; S3—Ćuk, Banatsko Karađorđevo, and S4—Dobrivoj, Bela Reka), while another four were produced in large scale - dairy factories (L1—Delhaize Serbia, Beograd; L2—Stara Planina, Dimitrovgrad; L3—Carpe Diem, Krušedol and L4—Select Milk, Inđija).
2.3. Compositional analysis of raw milk and whey samples
Basic composition of raw milk, laboratory whey samples and market whey samples were analyzed using the following methods: standard drying method (FIL-IDF, 1987) for total solids content; Gerber method (FIL-IDF, 1981) for fat content; Kjeldahl method (FIL-IDF, 1993) for total nitrogen content. Protein content was calculated by multiplying the value for total nitrogen with the factor of 6.38. Digital pH meter was used to measure pH value of all samples.
2.4. Protein characterization
Samples of milk and whey were prepared as described by Miloradovic et al. (2016). Briefly, the raw milk and the whey samples were mixed in mass ratio1:9 and 1:1, respectively, with sodium dodecyl sulphate polyacrylamide gel electrophoresis (SDS-PAGE) sample buffer. Samples were applied to the gel in equal volumes of 5 μL.
The SDS-PAGE was carried out according to the method of Laemmli, (1970). Samples were run with 4% stacking gel of pH 6.80, and 12.5% resolving gel pH 8.85. The running buffer was Tris–glycine buffer containing 0.05 M TRIS, 0.19 M glycine, and 0.1% (w/v) SDS. Before the analysis, all the samples were heated in boiling water for 5 min. Aliquots of 5 μL supernatant and precipitate samples were loaded per well. Electrophoresis was performed using 20.5 × 10 cm TV200YK twin-plate electrophoresis unit (Consort, Turnhout, Belgium) together with Electrophoresis Power Supply EV202 of the same producer. The power supply was set at constant current of 80 mA and the maximum voltage of 300 V was applied for 1.5 h. Following electrophoresis, the gels were fixed and stained for 1 h at the temperature of 45°C, in 250 mL Coomassie blue dye solution containing 0.23% (w/v) Coomassie brilliant blue R250, 3.9% (w/v), trichloroacetic acid, 6% (v/v) acetic acid and 17% (v/v) methanol. The staining was followed by three destaining steps, with a destaining solution containing 8% (v/v) acetic acid and 18% (v/v) methanol. The gels were scanned using HP Scanjet G2710 (Hewlett Packard, California, United States).
2.5. Determination of macro and micro elements content
Prior to determination of the content of analyzed elements, the homogenized sample (2.0 mL) was placed in PTFE vessel, followed by addition of 4 mL 65% HNO3 and 1 mL 30% H2O2. The mixture was digested in microwave oven (CEM Mars 5, United States) at a temperature of 200°C using pressure controlled program. Firstly, pressure was raised up to 1.36 atm (20 psi; 3 min ramp) and held for 1 min. The pressure was raised up to 2.72 atm (40 psi) during 8 min, and held for 5 min. Samples were cooled to room temperature, made up to 25 mL with deionized water and conserved at 4°C until it is analysis with ICP.
The content of macro and micro elements were determined by ICP-OES, model iCAP 6,500 Duo (Thermo Scientific, United Kingdom). The calibration curves were constructed by dilution of PE-CAL4-ASL-1, ICP-41 W-1, ICP-56 W-1 and MES-13-1 standard solution.
2.6. Microbiological analysis
Goat whey samples were serially diluted with sodium chloride (0.9% (w/v)) and appropriate dilution was plated on Nutrient agar (NA; Merck, Darmstadt, Germany) for the enumeration of total aerobic count, MRS agar (Merck, Darmstadt, Germany) for the enumeration of Lactobacillus spp. and M17 agar (Merck, Darmstadt, Germany) supplemented with 0.5% glucose was used for the enumeration of Lactococcus spp. MRS plates were incubated anaerobically (GasPak Jar, BBL, Kansas, United States) at 37°C for 48 h, while NA and GM17 plates were incubated at 30°C during 48 h.
Number of coagulase positive Staphylococcus ssp. was determined according to the EN ISO 6888-2, while number of Escherichia coli and Listeria monocytogenes was examined according to ISO 16649-2 and EN ISO 11290-2, respectively.
2.7. Statistical analysis
Whey samples collection was undertaken in duplicate, while all applied analyses were carried out in triplicate.
Two-way analysis of variance (ANOVA), with general linear model (GLM) and post-hoc LSD test was conducted to determine the effects of coagulation mechanism, heat treatment and their interaction on analyzed characteristics of laboratory whey. One-way ANOVA was performed for analysis of the effect of production site scale (small vs. large) on analyzed characteristics of market whey. Statistical significance level was set at 0.05.
A principal components analysis (PCA) was run on 14 samples (6 laboratory and 8 market whey samples) and 26 items that characterized all samples (items are listed in Table 1). Prior to analysis, suitability of PCA was assessed. The overall Kaiser–Meyer–Olkin (KMO) measure was 0.6 with individual KMO measures all greater than 0.9. Bartlett’s test of sphericity was statistically significant (p < 0.0005), indicating that the data was likely factorable. A Varimax orthogonal rotation was employed to aid interpretability. The purpose of PCA was to enable clear visualization of quantitative relationships in terms of composition within market and laboratory whey samples.
For all calculations, IBM SPSS 21 (IBM Corp., Chicago, IL, United States) software was used.
3. Results and discussion
3.1. Properties of laboratory whey
3.1.1. Basic composition
It has been previously reported that heat treatment affects fat content of sweet goat whey (Miloradovic et al., 2016), and also that sweet whey has higher fat content when compared to acid whey (Mehra et al., 2021). The current study showed no significant effect of either of these two factors. As it could be seen from Table 2, and as it will be discussed later for market samples, fat content appears to be the whey component with the widest variations. It is highly dependent on cheese making technology—curd cutting process in a first place (Moreno-Indias et al., 2009).
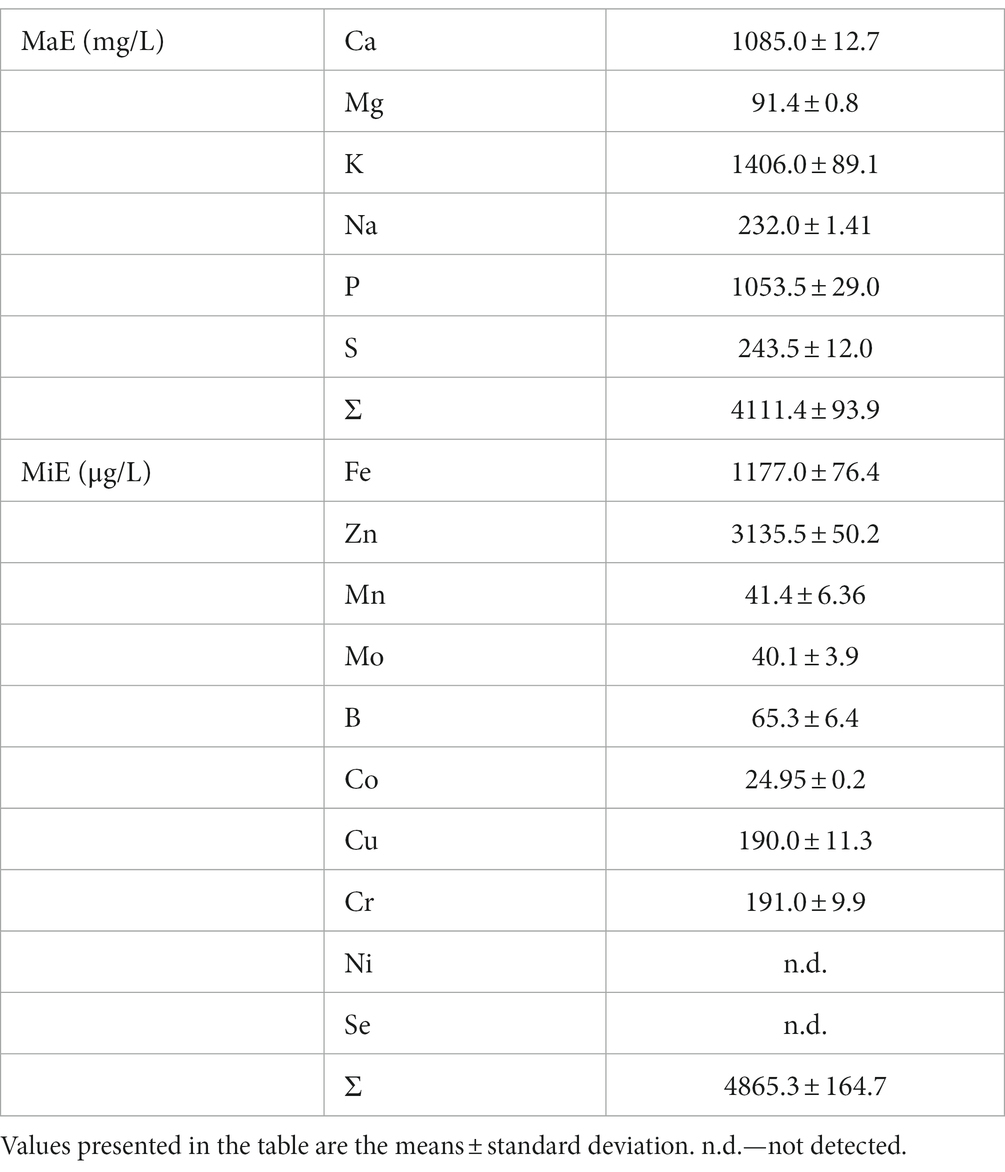
Table 2. Basic composition of sweet (s) and acid (a) whey obtained from differently heated milk A (65°C/30 min), B (80°C/5 min) and C (90°C/5 min).
As reported previously (Rocha-Mendoza et al., 2021) and therefore expected, the type of coagulation affected the pH value of whey. However, only pH values of acid whey were affected by heat treatment of milk: samples Ba and Ca had a lower pH than Aa (Table 2). The current result represents a demonstration of how high pasteurization is lowering a buffering capacity of cheese milk (Salaün et al., 2005).
3.1.2. Protein composition
High temperature treatments of milk, depending on severity, may result in a number of changes, including denaturation of whey proteins (Guinee, 2021). Heating of milk to temperatures higher than 70°C results in unfolding of whey proteins, exposure of reactive side-chain groups that are normally buried within the native protein conformation, and interaction with other whey proteins and/or κ-CN through disulphide, hydrophobic and electrostatic interactions (Cho et al., 2003). High temperature treatment conditions can be varied to alter the proportions of native whey proteins in the resultant cheese whey, thereby enabling the provision of whey streams more suited to the manufacture of whey protein ingredients for specific applications (Guinee, 2021).
In literature, one could come across the claim that sweet whey has a higher total protein and whey protein content when compared to acid whey (Nishanthi et al., 2017; Rocha-Mendoza et al., 2021). However, as Nishanthi et al. (2017) have suggested, and the present study confirmed it is not the coagulation type responsible for such a difference in protein content, but the heat treatment of milk. The increase in heating temperature leads to the decrease in whey proteins (Table 2; Figure 1). The ability of goat milk to coagulate upon the action of rennet without diminished renneting properties, even if it has been subjected to a range of pasteurization regimes, is highly positive from the perspective of cheese production (Miloradovic et al., 2017), but it also leads to the whey depleted of proteins.
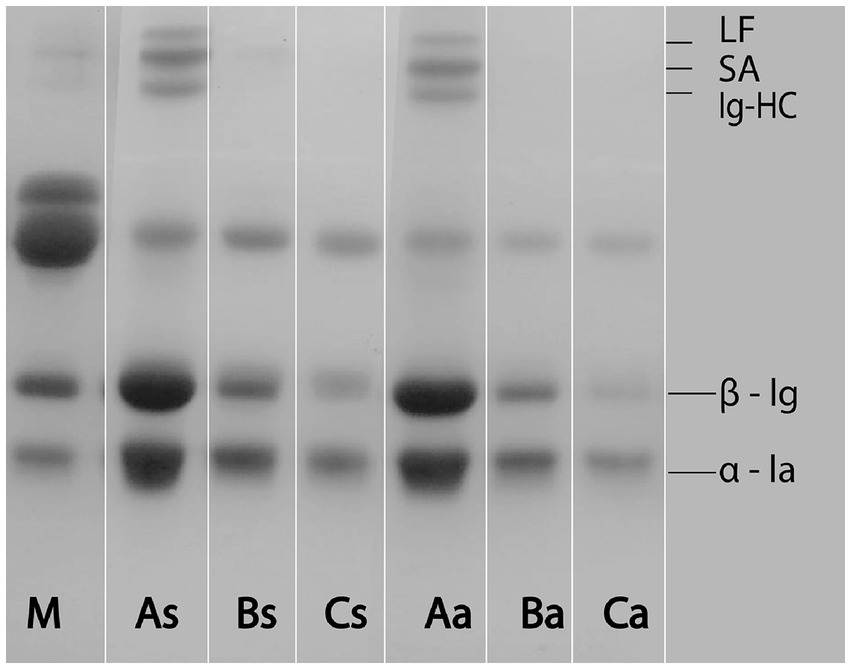
Figure 1. SDS-PAGE electrophoretogram of raw milk (M), sweet (s) and acid (a) whey obtained from differently heated milk A (65°C/30 min), B (80°C/5 min) and C (90°C/5 min). LF, lactoferrin; SA, serum albumin; Ig-HC, immunoglobulin heavy chain; β-lg, β-lactoglobulin; α-la, α-lactalbumin.
The results from the present study confirmed that, as in the previously published paper (Miloradovic et al., 2016), in a goat whey Lactoferrin (LF) and Immunoglobulin heavy chain (Ig-HC) could be detected only after the heating regime A. In line with the previous study, Serum albumin (SA) has been detected in both As and Aa samples (Figure 1). In our previous study (Miloradovic et al., 2016) the SA band has been clearly detected in whey from milk heated to 80°C/5 min. Presumably as a result of the lower amount of this protein fraction in currently used milk, only a shallow band of SA occurred in sweet whey from the same heating regime—Bs (Figure 1). However no SA bend has been detected in the acid whey counterpart (Ba), suggesting that acidification process contributed to the SA incorporation in cheese matrix. Major whey proteins decreased gradually upon the range of more and more severe heat treatments (Figure 1). The current study revealed that the identical pattern could be recognized for acid whey Aa, Ba and Ca.
It is noteworthy that, to the opposite of the previously published paper (Miloradovic et al., 2016), in the present experiment a faint bands of Glycomacropeptide (GMP) oligomer could be detected in sweet whey samples after the first step of gel distaining, but after complete distaining, they faded completely. For the reason that has already been pointed out by Nishanthi et al. (2017), that there is a low affinity between GMP and Coomassie Brilliant Blue dye, SDS-PAGE might be somewhat limited method for differentiation between proteins of sweet and acid whey.
3.1.3. Mineral composition of raw milk and whey
Although minerals represent minor fraction of milk compared to the protein or lipid fraction, they play an important role from the point of view of structural stability of casein, but also from the point of view of dairy product biological value. Thorough investigations of mineral composition - both major (macro elements—MaE) and trace (micro elements—MiE) in dairy products is attracting the attention of scientists for over 70 years (Gaucheron, 2005). Macro and micro elements have an important role in many biological functions. Compared to milk, their bioavailability in whey is higher (Zamberlin et al., 2011). Data on mineral content of whey is important in it is use as an ingredient for powdered products, whey based beverages, or as an alternative to inorganic fertilizer (Wong et al., 1978; Mehra et al., 2021). By comparison of mineral composition of goat milk (Table 3) and whey (Table 4) the distribution of macro and micro elements between cheese and whey could be followed.
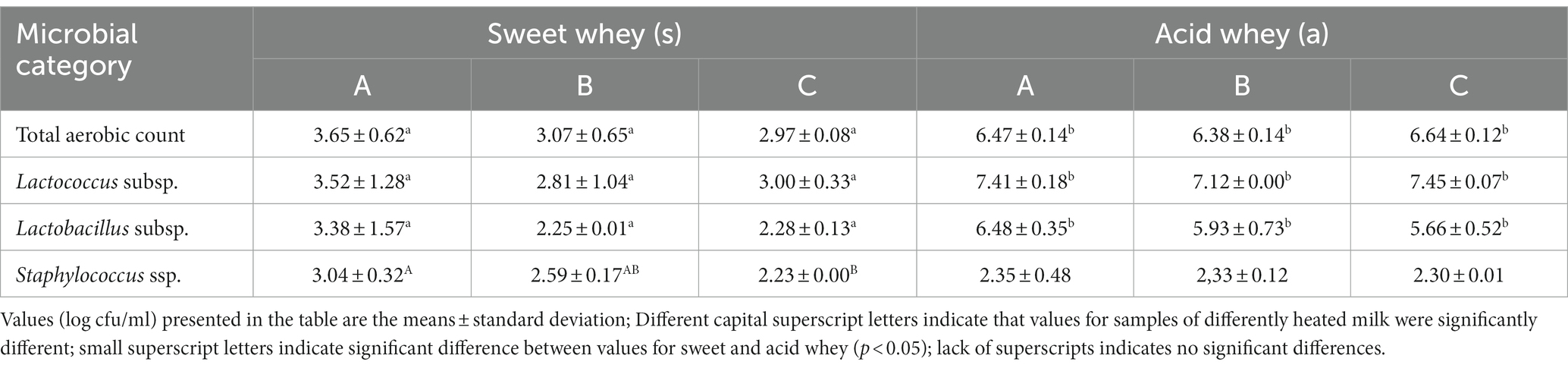
Table 4. Mineral composition (macroelements—MaE and microelements—MiE) in sweet (s) and acid (a) whey obtained from differently heated milk A (65°C/30 min), B (80°C/5 min), and C (90°C/5 min).
3.1.3.1. Mineral composition of raw goat milk
In general, goat milk is similar to cow milk with regard to the content of Na, Fe, Zn, and Mo, but has higher amounts of Ca, K, Mg, P, and Mn (Zhao et al., 2020). Raw milk used in the present experiment (Table 3) is comparable with the milk analyzed in several studies in terms of Ca, P, Zn, and K content. However, it has lower Na and Mg content and higher Fe and Zn content (Soliman, 2005; Zamberlin et al., 2011). The reason for such differences could be explained by the influence of many factors such as soil composition, feeding method, animal breed, lactation stage, health condition of animals (Zamberlin et al., 2011).
3.1.3.2. Macro elements in whey
The obtained sweet whey was significantly lower in a sum of both MaE and MiE compared to acid whey, across the range of heating regimes applied (Table 4). Such results are in line with the ones concluding that generally, due to the release of calcium from casein micelle during acid coagulation, sweet cow whey is lower in total mineral content, when compared to acid cow whey (Mehra et al., 2021; Rocha-Mendoza et al., 2021). The results of the present study showed that aside from calcium, significantly higher amount of Mg and P could be found in the acid whey compared to sweet whey. Wong et al. (1978) reported that calcium content was three times as great in acid as in sweet whey, while in the same study magnesium content was ≈50% lower in sweet than in the acid whey. The results of the present study showed a comparable pattern for Mg, while calcium content in acid whey was almost four times higher than in sweet whey.
Heat treatment of milk, however, did not have a significant effect on the total sum of either MaE or MiE content in whey (Table 4). Comparison of individual MaEs revealed that only sulfur (S) and phosphor (P) were affected by milk heating regime. The lower content of S in both sweet and acid whey heated above 80°C could be explained by involving cysteine thiol groups of denatured whey proteins in disulfide interactions with casein and thus by it is inclusion in cheese mass/exclusion from whey (Guinee, 2021).
Phosphorus was not affected by heat treatment of milk when sweet whey is considered, but only in case of acid whey (Table 4). The highest P content could be found in the sample Aa, then Ca and the lowest in Ba. In milk and dairy products, phosphorus occur in numerous forms including inorganic and organic salts, ions, as a part of proteins, fats, carbohydrates, nucleic acid etc. (Zamberlin et al., 2011). Hence, upon heat treatment of milk, and subsequent different types of coagulation, it undergoes complex transformations. Along with the two investigated factors that affected mentioned transformations, it could be hypothesized that duration of heat treatment also plays a significant role.
Some authors cited by Guinee (2021) reported the progressive decrease in concentration of soluble Ca, P, and Mg with gradual increase in milk heating temperature, due to their transfer to the colloidal or sedimentable phase of cow milk. However, in case of goat milk, there is no significant decrease in Ca or Mg in either sweet or acid whey across the range of milk heating regimes applied in the study. It could, at least in a part, explain why rennet coagulation of goat milk is less impeded by prior heat treatments (Miloradovic et al., 2020).
Sodium and potassium are found in milk almost entirely in ionic form (Zamberlin et al., 2011). Therefore as expected, neither heat treatment of milk, nor milk coagulation type affected significantly their content in whey. The lack of significant difference between Na and K content in milk and whey are clearly showing that those two elements were just transferred from serum phase of milk to whey.
3.1.3.3. Micro elements in whey
Micro elements included in the present study are just a small group of those that occur in milk. However, aside from the determined ones, the others such as lithium, bromine, aluminum, silver, lead, etc., are not nutritionally important (Zamberlin et al., 2011).
Micro element that was the most dramatically changed by the influence of the treatments applied is Zn. The amount of Zn in acid whey was more than 15 times higher than in sweet whey (Table 4). Data from the literature report an even greater difference—20 times more Zn in acid whey (Wong et al., 1978). Zinc is one of the MiE associated with caseinate in the high extent (≈ 90%), while only about 10% of it is in serum phase (Zamberlin et al., 2011). It could be suggested that the similar phenomenon occurs for Mo and Cr. The reason why only heating regime of 80°/5 min made a difference between coagulation types, should be the subject to further investigation.
3.1.4. Microbiological quality
Sweet laboratory goat whey has been obtained from rennet coagulated cheese. Coagulation has been conducted with the absence of starter culture. Therefore the presented counts of lactic acid bacteria in sweet whey could be considered as non-starter microbiota (Table 5).
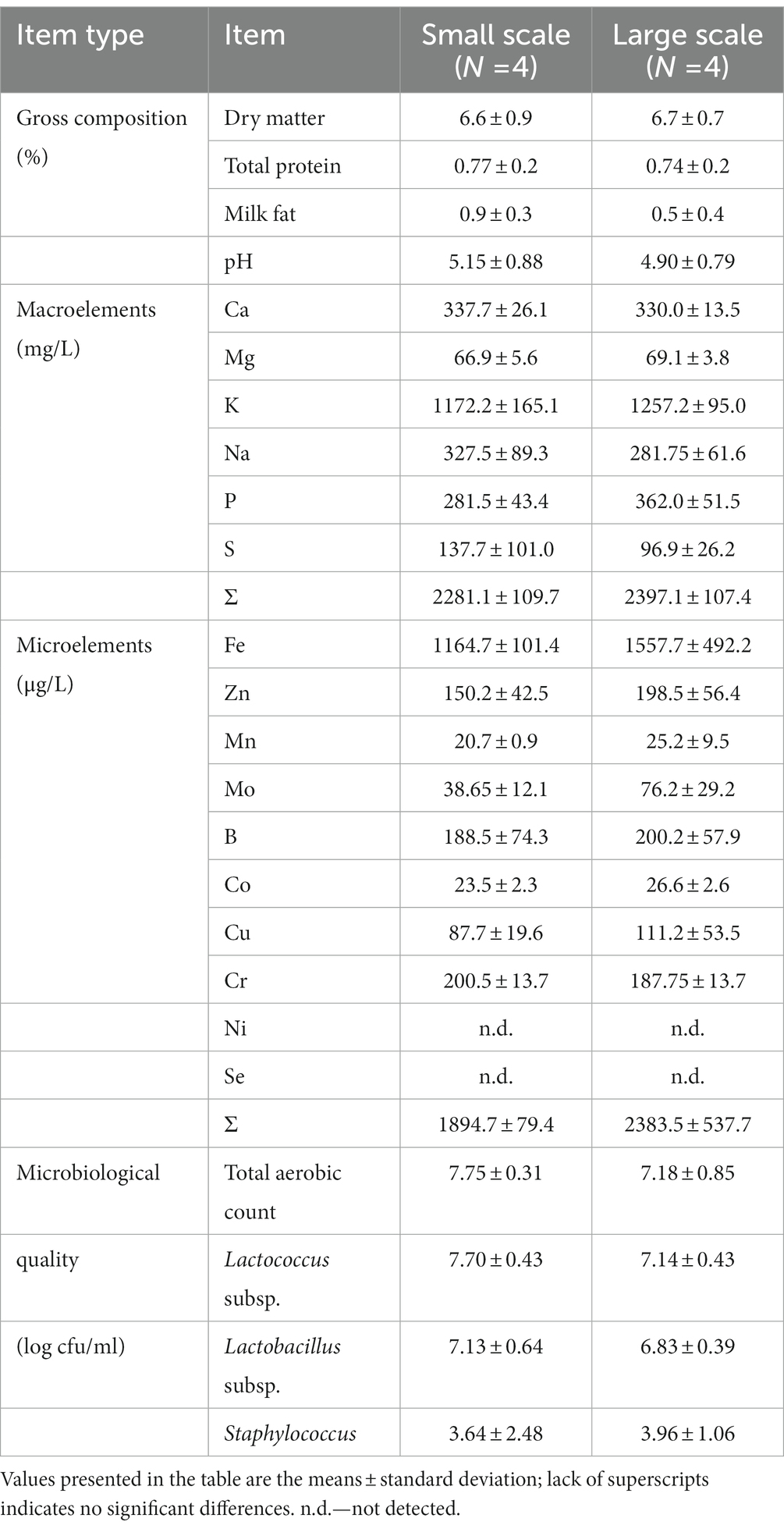
Table 5. Microbiological quality of sweet(s) and acid (a) goat whey obtained from differently heated milk A (65°C/30 min), B (80°C/5 min) and C (90°C/5 min).
Coagulase positive staphylococci represents a microbiological safety concern (mainly for the enterotoxin production) when the detected values exceed 5 log cfu/ml (Regulation, 2018). In laboratory samples, in case of sweet goat whey, milk heat treatment had a significant effect on number of Coagulase positive Staphilococci—the counts decreased significantly with the severity of heat treatment of milk. However, in line with the mentioned regulation, the level of coagulase positive Staphilococci were acceptable for all laboratory samples. Esherichia coli was below the level of quantification and Listeria monocytogenes was not detected. Obtained results indicated that high heat treatments of milk had a positive effect on microbiological quality of sweet whey.
Significantly higher counts of Lactococcus subsp. in acid whey could be well explained by the presence of (LL) Lactococcus lactis subsp. lactis, (LLC) Lactococcus lactis subsp. cremoris and Lactococcus lactis subsp. lactis biovar. Diacetylactis (LLD) in Flora Danica starter culture, used for the experiment. However, during acid coagulation/fermentation which lasted for 20 h, Lactobacillus subsp. have grown, exhibiting a significantly higher count in acid whey compared to sweet whey.
3.2. Comparison of the quality of small and large scale market whey
Each market sample, from both small and large scale fulfilled the requirement set by the Regulation (2014a), for the limit of dry matter content (5.5%), and also for the microbiological quality (Regulation, 2018).
As presented in the Table 1, the average for milk fat content from small scale whey samples was almost double (0.9) than in those from large scale (0.5), however, as a result of high standard deviation, no statistical difference has been observed. The assumption is that in small scale cheese production, the ineffective recovery of fat from milk to cheese occasionally occurs. Significantly higher fat content in farm whey compared to factory whey has been determined by Moreno-Indias et al. (2009), with a similar explanation as given here.
The scientific literature reports some data on poor microbiological quality of small scale dairy products (Şanlıbaba et al., 2018). The average price of the large scale whey samples from the current study was more than 35% higher compared to the price of those from small scale producers (data not shown). And yet, not a single quality parameter, including microbiological, differed significantly between whey samples originating from farm producers (small scale) and those from dairy factories (large scale). It is obvious that regardless of flexibility of safety and quality legal requirements, small scale produced whey from the Serbian market does not have any quality shortage compared to the large scale produced whey. It is clear that this branch represents an undeniable potential to be supported and promoted, especially bearing in mind that in Serbia, consumers perceive small scale foods as superior from the aspects of both health and quality, compared to their large scale counterparts (Miloradovic et al., 2021).
3.3. Estimation of heat treatment of milk and coagulation type of market goat whey samples
Principal component analysis (PCA) has been applied in order to estimate whether market whey samples belonged to a group of acid or sweet whey. PCA revealed two components that had eigenvalues greater than one and which explained 85.1 and 12.9% of the total variance, respectively. The two-component solution explained 98.0% of the total variance.
As expected, it could be seen from Figure 2 that as a result of numerous differences in quality parameters, acid and sweet whey from the laboratory, formed two distinct clusters. Such grouping revealed that from the two factors analyzed, milk coagulation type was the predominant factor affecting the quality of liquid goat whey. Furthermore, it is evident that all market samples shared high degree of similarity with sweet whey samples, meaning that it could be hypothesized that sweet whey is the category to which they belong.
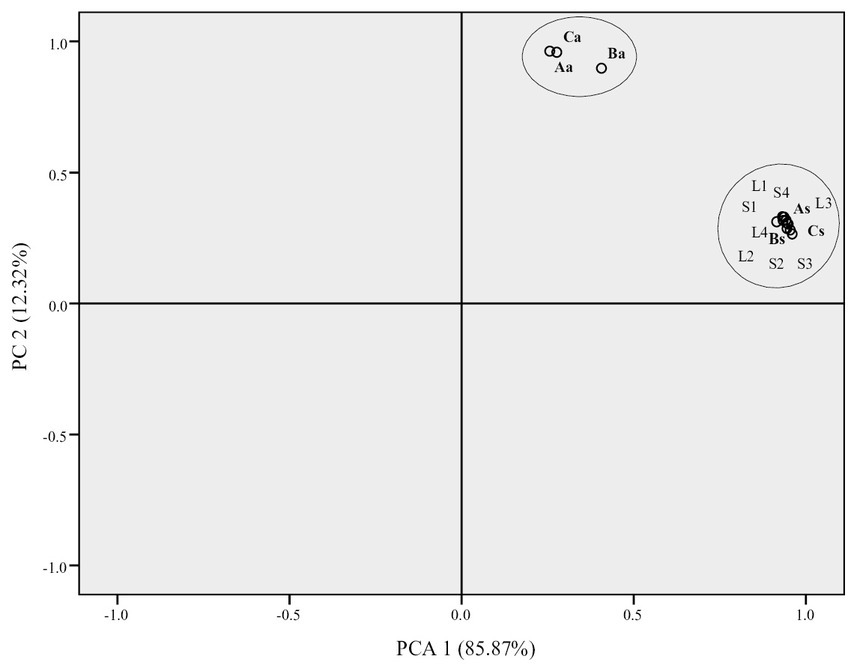
Figure 2. Loading map obtained by principal component analysis of physico-chemical and microbiological parameters of liquid whey collected from laboratory cheese production (Aa, Ba, and Ca—acid whey and As, Bs, and Cs—sweet whey) obtained from differently heated milk A (65°C/30 min), B (80°C/5 min), and C (90°C/5 min), and small scale (S1–S4) and large scale (L1–L4) liquid goat whey from the Serbian market.
In order to estimate the milk heating regime of market whey, SDS-PAGE has been engaged (Figure 3). When compared to the same analysis of laboratory samples, it could be inferred that with one exception (large scale sample L2), all other market whey samples showed a visible bands of LF and Ig-HC, meaning that they originated from milk heated to less than 80°C.
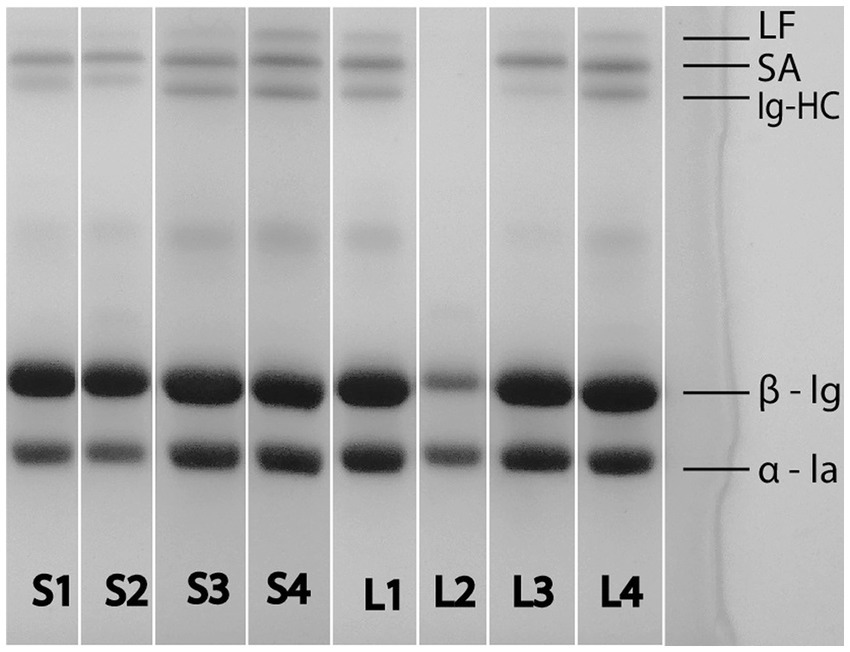
Figure 3. SDS-PAGE electrophoretogram of small scale (S1–S4) and large scale (L1–L4) liquid goat whey from the Serbian market. LF, lactoferrin; SA, serum albumin; Ig-HC, immunoglobulin heavy chain; β-lg, β-lactoglobulin; α-la, α-lactalbumin.
The most consumed cheese in Serbia is white brined cheese (Miloradovic et al., 2021). The same type is also the most abundant variety in Serbian tradition. It is characterized by low pasteurization of milk, followed by rennet coagulation (Miocinovic and Miloradovic, 2019). So, even if goat milk offers the possibility of rennet coagulation after high heat treatment of milk (Miloradovic et al., 2016), and even if legal requirement of 5.5% of dry matter is achievable with both acid and rennet coagulation type, local producers evidently hold to the tradition. The current study showed that sweet liquid whey derived from goat milk heated to less than 80°C/5 min is what Serbian market predominantly offers at the present moment.
4. Conclusion
The type of coagulation is a predominant factor affecting all quality parameters of whey (especially mineral composition), while heat treatment predominantly determines protein composition. The present study detected the lack of influence of heat treatment on the Ca and Mg content in goat whey. Such behavior is the opposite of cow milk behavior, in which with the subsequent increase in heating temperature, the amount of soluble Ca and Mg decreases. Deeper investigation would be needed to determine how transformation of calcium upon the range of heating regimes influences rheological parameters of goat milk during rennet coagulation.
Despite the non-stringent legal requirement for liquid whey quality (only 5.5% of dry matter minimum), according to the principal component analysis of physico-chemical and microbiological characteristics, all samples of market whey were grouped together with sweet laboratory whey samples. According to this result, and to the results of SDS-PAGE, it could be hypothesized that whey producers in Serbia apply low pasteurization of milk followed by rennet coagulation. Although legal requirements for safety and quality of small scale dairy products are more flexible than that of the large counterparts, there was not a single characteristic that differed significantly between small scale and large scale market goat whey.
Further popularization of goat whey, supported by scientific studies, would be needed. From the environmental point of view, it would be a path to turn a hazardous waste into a popular healthy food product. Goat whey is increasingly used as a functional food in the context of a healthy lifestyle or as a means to compensate for an unhealthy one. However it is highly questionable whether it is as functional as consumers perceive it. Therefore, it would be of great importance to study bioactivity of both liquid and lyophilized goat whey from different streams. In addition to that, it could be suggested that more investments are needed in finding out what goat whey can contribute to individual and public health in relation to the claims made by manufacturers.
Since liquid goat whey and goat dairy products in general represent a niche in the dairy industry, there is only a limited number of both small scale and large scale whey on the market, which represents the limitation of the present study.
Data availability statement
The original contributions presented in the study are included in the article/Supplementary material, further inquiries can be directed to the corresponding author.
Author contributions
ZM and JM: conceptualization and work designing. MH, MM, and II: methodology. AS and NB: formal analysis. ZM: writing the original draft. NS, JM, MM, and RJ: revision of the final article. All authors have read and agreed to the final version of the manuscript.
Funding
This paper is a result of the research conducted according to the contract on the implementation and financing of scientific research in 2023, between the Institute for Science Application in Agriculture, Belgrade and the Ministry of education, science and technological development of the Republic of Serbia, contract number: 451-03-47/2023-01/200116.
Conflict of interest
The authors declare that the research was conducted in the absence of any commercial or financial relationships that could be construed as a potential conflict of interest.
Publisher’s note
All claims expressed in this article are solely those of the authors and do not necessarily represent those of their affiliated organizations, or those of the publisher, the editors and the reviewers. Any product that may be evaluated in this article, or claim that may be made by its manufacturer, is not guaranteed or endorsed by the publisher.
References
Barukčić, I., Jakopović, K. L., and Božanić, R. (2019). Valorisation of whey and buttermilk for production of functional beverages-an overview of current possibilities. Pregled mogućih načina iskorištenja sirutke i mlaćenice u proizvodnji funkcionalnih napitaka 57, 448–460. doi: 10.17113/ftb.57.04.19.6460
Božanić, R., Barukčić,., Jakopić, K. L., and Travnik, L. J. (2014). Possibilities of Whey Utilisation. Austin of Food Nutr. Food Sci. 2, 7.
Cho, Y., Singh, H., and Creamer, L. K. (2003). Heat-induced interactions of β-lactoglobulin a and κ-casein B in a model system. J. Dairy Res. 70, 61–71. doi: 10.1017/s0022029902005642
FIL-IDF (1981). “Milk” in Determination of Fat Content. Gerber Butyrometers (Brussels, Belgium: International Dairy Federation).
FIL-IDF (1987). “Milk, cream and evaporated milk” in Determination of Total Solids Content (Brussels, Belgium: International Dairy Federation).
FIL-IDF (1993). “Milk” in Determination of Nitrogen Content (Kjeldahl method) (Brussels, Belgium: International Dairy Federation).
Fox, P. F., Guinee, T. P., Cogan, T. M., and McSweeney, P. L. H. (2017). Fundamentals of Cheese Science (2nd edn., Chapt. 7). New York, NY: Springer Science Business Media LLC.
Gaucheron, F. (2005). The minerals of milk. Reprod. Nutr. Dev. 45, 473–483. doi: 10.1051/rnd:2005030
Guinee, T. P. (2021). Effect of high-temperature treatment of milk and whey protein denaturation on the properties of rennet–curd cheese: a review. Int. Dairy J. 121:105095. doi: 10.1016/j.idairyj.2021.105095
Hovjecki, M., Miloradovic, Z., Barukčić, I., Blažić, M., and Miocinovic, J. (2022). Rheological properties of goat milk coagulation as affected by rennet concentration, pH and temperature. Fermentation 8:291. doi: 10.3390/fermentation8060291
Laemmli (1970). Cleavage of structural proteins during the assembly of the head of bacteriophage T4. Nature 227, 680–685. doi: 10.1038/227680a0
Lucey, J. A. (2016). “Acid coagulation of milk” in Advanced Dairy Chemistry. Vol. 1B. Proteins: Applied Aspects (4th edn., pp. 309e328). eds. P. L. H. McSweeney and J. A. O'Mahony (New York, NY: Springer Science Business Media)
Mehra, R., Kumar, H., Kumar, N., Ranvir, S., Jana, A., Buttar, H. S., et al. (2021). Whey proteins processing and emergent derivatives: an insight perspective from constituents, bioactivities, functionalities to therapeutic applications. J. Funct. Foods 87:104760. doi: 10.1016/j.jff.2021.104760
Miloradovic, Z., Blazic, M., Barukcic, I., Fonti Furnols, M., Smigic, N., Tomasevic, I., et al. (2021). Serbian, Croatian and Spanish consumers beliefs towards artisan cheese. Br. Food J. 124, 3257–3273. doi: 10.1108/BFJ-04-2021-0409
Miloradovic, Z., Kljajevic, N., Miocinovic, J., Levic, S., Pavlovic, V. B., Blažić, M., et al. (2020). Rheology and microstructures of rennet gels from differently heated goat Milk. Foods 9:283. doi: 10.3390/foods9030283
Miloradovic, Z., Kljajevic, N., Miocinovic, J., Tomic, N., Smiljanic, J., and Macej, O. (2017). High heat treatment of goat cheese milk. The effect on yield, composition, proteolysis, texture and sensory quality of cheese during ripening. Int. Dairy J. 68, 1–8. doi: 10.1016/j.idairyj.2016.12.004
Miloradovic, Z. N., Macej, O. D., Kljajevic, N. V., Jovanovic, S. T., Vucic, T. R., and Zdravkovic, I. R. (2016). The effect of heat treatment of caprine milk on the composition of cheese whey. Int. Dairy J. 58, 39–42. doi: 10.1016/j.idairyj.2016.01.016
Miloradovic, Z., Miocinovic, J., Kljajevic, N., Tomasevic, I., and Pudja, P. (2018). The influence of milk heat treatment on composition, texture, colour and sensory characteristics of cows and goats quark-type cheeses. Small Rumin. Res. 169, 154–159. doi: 10.1016/j.smallrumres.2018.09.012
Miocinovic, J., and Miloradovic, Z. (2019). “Production and characteristics of different types of Serbian cheeses” in Cheeses Around the World. eds. R. D. P. F. Guinee, P. M. D. R. Correia, and A. C. Ferrao (New York, NY: Nova Science Publishers), 183–203.
Moreno-Indias, I., Castro, N., Morales-dela Nuez, A., Sánchez-Macías, D., Assunção, P., Capote, J., et al. (2009). Farm and factory production of goat cheese whey results in distinct chemical composition. J. Dairy Sci. 92, 4792–4796. doi: 10.3168/jds.2009-2215
Nishanthi, M., Vasiljevic, T., and Chandrapala, J. (2017). Properties of whey proteins obtained from different whey streams. Int. Dairy J. 66, 76–83. doi: 10.1016/j.idairyj.2016.11.009
Regulation, S. (2014a). “Amendment on Sebian Regulation 2010 Quality of Dairy Products and Strarter Cultures” in 33/10 (Belgrade, Serbia: Official Bulletin of the Republic of Serbia).
Regulation, S. (2014b). "Ordinance on Quality of Milk Products and Starter Cultures (in Serbian)", in: 33/2010, 69/2010, 43/2013 and 34/2014. (ed.) Belgrade, Serbia: Official Gazzete of Republic of Serbia.
Regulation (2017). Ordinance on small quantities of primary products that serve to supply consumers, the area for carrying out these activities, as well as deviations related to small entities in the business of food of animal origin (in Serbian), in: 41/09 (ed.) (Belgrade, Serbia: Official Gazzete of Republic of Serbia.
Regulation (2018). “General and special conditions of food hygiene at any stage of production and circulation (in Serbian)”, in: 72/2010 and 62/2018 (ed.) O.b.o.t.R.o. Serbia. Belgrade, Serbia.
Rocha-Mendoza, D., Kosmerl, E., Krentz, A., Zhang, L., Badiger, S., Miyagusuku-Cruzado, G., et al. (2021). Invited review: acid whey trends and health benefits. J. Dairy Sci. 104, 1262–1275. doi: 10.3168/jds.2020-19038
Salaün, F., Mietton, B., and Gaucheron, F. (2005). Buffering capacity of dairy products. Int. Dairy J. 15, 95–109. doi: 10.1016/j.idairyj.2004.06.007
Şanlıbaba, P., Tezel, B. U., and Çakmak, G. A. (2018). Prevalence and antibiotic resistance of Listeria monocytogenes isolated from ready-to-eat foods in Turkey. J. Food Qual. 2018, 1–9. doi: 10.1155/2018/7693782
Soliman, G. Z. A. (2005). Comparison of chemical and mineral content of milk from human, cow, buffalo, camel and goat in Egypt. The Egyptian. J. Hosp. Med. 21, 116–130. doi: 10.21608/EJHM.2005.18054
Wong, N. P., LaCroix, D. E., and McDonough, F. E. (1978). Minerals in whey and whey fractions. J. Dairy Sci. 61, 1700–1703. doi: 10.3168/jds.S0022-0302(78)83790-4
Zamberlin, Š., Antunac, N., Havranek, J., and Samardžija, D. (2011). Mineral elements in milk and dairy products. Mljekarstvo 62, 111–125.
Zhang, X., Qiao, C., Fu, S., Jiao, Y., and Liu, Y. (2022). DNA-based qualitative and quantitative identification of bovine whey powder in goat dairy products. J. Dairy Sci. 105, 4749–4759. doi: 10.3168/jds.2021-21618
Keywords: goat whey, side streams, mineral composition, acid whey, sweet whey, zero waste
Citation: Miloradovic Z, Hovjecki M, Mirkovic M, Bajcetic N, Ignjatovic IS, Satric A, Smigic N, Maslovaric M, Jovanovic R and Miocinovic J (2023) Quality of liquid goat whey affected by heat treatment of milk and coagulation type: case study of the Serbian market. Front. Sustain. Food Syst. 7:1171734. doi: 10.3389/fsufs.2023.1171734
Edited by:
Zhipeng Li, Jimei University, ChinaReviewed by:
Gerardo Mendez-Zamora, Autonomous University of Nuevo León, MexicoZhi Wang, Zhengzhou University, China
Huipeng Liu, Pingdingshan University, China
Copyright © 2023 Miloradovic, Hovjecki, Mirkovic, Bajcetic, Ignjatovic, Satric, Smigic, Maslovaric, Jovanovic and Miocinovic. This is an open-access article distributed under the terms of the Creative Commons Attribution License (CC BY). The use, distribution or reproduction in other forums is permitted, provided the original author(s) and the copyright owner(s) are credited and that the original publication in this journal is cited, in accordance with accepted academic practice. No use, distribution or reproduction is permitted which does not comply with these terms.
*Correspondence: Zorana Miloradovic, em9yYW5hQGFncmlmLmJnLmFjLnJz