- 1Department of Soil Science, Punjab Agricultural University, Ludhiana, India
- 2Rajmata Vijayaraje Scindia Krishi Vishwa Vidyalaya, Gwalior, India
- 3Indian Institute of Soil Science, Bhopal, India
- 4Department of Chemistry, Punjab Agricultural University, Ludhiana, India
- 5Department of Botany and Microbiology, College of Science, King Saud University, Riyadh, Saudi Arabia
- 6Department of Botany and Plant Physiology, Faculty of Agrobiology, Food, and Natural Resources, Czech University of Life Sciences Prague, Prague, Czechia
- 7Division of Soil Science, Bangladesh Wheat and Maize Research Institute, Dinajpur, Bangladesh
Introduction: Micronutrients particularly Zn and Mn play a vital role in agricultural plants as well as human beings. Categorization of existing genotypes in wheat toward Zn and Mn application is extremely important to select the most efficient Zn as well as Mn genotype with higher yield and quality.
Methods: In this study, the differentiation of 14 genotypes was done for nutrient use efficiency characteristics through two methods to identify the most efficient Zn and Mn wheat genotypes. The categorization of genotypes was done based on efficiency and responsiveness under control and Zn + Mn treated conditions.
Results and discussion: The mean values of plant growth parameters, crop yield, nutrient concentration (Zn, Mn, N, P and K), quality parameters (crude fiber and protein content) and nutrient utilization efficiency were considerably greater in Zn + Mn treated plots signifying the higher response of traits toward Zn and Mn application. The interaction between genotype and Zn + Mn indicated that the application of Zn + Mn significantly affected the studied genotypes for different characteristics. The results of the study indicated that genotypes PBW-824, BWL-8855, PBW-872, PBW-869 and PBW Zn1 were efficient in terms of crop yield. Based on the quality parameters, genotypes PBW-824 and PBW-869 were the highest ranked among the studied genotypes. The identified Zn + Mn efficient genotypes would be valuable resources for higher crop production along with improved wheat grain quality.
1. Introduction
Greater production of staple food for the increasing population has reduced the nutritional quality of food and thus resulted in hidden hunger in living beings. Hidden hunger/micronutrient malnutrition refers to the insufficient intake of micronutrients which has severely affected human health as well as socio-economic growth. Thus, ending malnutrition by 2030 has been a set forward goal under the UN-Sustainable Development Goal 2 (UN-SDG2), which is insurmountable in the limited timeline. In this context, staple crops biofortification through scientific strategies such as agronomic approaches, conventional breeding and metabolic engineering, would gain great importance in the upcoming time. The biofortification process enables the augmentation of the nutrient levels in the edible part of crops, thus advocated as an efficient way to reduce micronutrient malnutrition by providing nutritionally enhanced crops. Economic efficiency along with the feasibility of using agronomically bio-fortified interventions have evolved as a valuable technique to reduce micronutrient deficiencies in developing countries as some studies have reported higher levels of micronutrients through biofortification. The other quality parameters of the crops have been found to increase in parallel with the nutrient content. However, the effectiveness of biofortification was primarily studied to increase the content of only one nutrient per crop.
Micronutrient deficiency in plants and soils, such as Zn (zinc) and Mn (manganese) has resulted in severe effects such as decreased yield and low micronutrient concentration in crops, leading to micronutrient malnutrition in humans and animals (Dhaliwal et al., 2020). Zinc is an important micronutrient in plants since it participates in a variety of physiological functions and activities of enzymes, including protein and auxin production, glucose metabolism, immune system development, cellular membrane maintenance, and pollen generation. Unfortunately, zinc deficiency affects nearly half of Indian soils (Chattha et al., 2017), causing visible anomalies in plants including stunted growth, chlorosis, reduced leaves, fungal infections and increased sensitivity to extreme light or temperature harm. Zinc has been listed as an imperative micronutrient for humans and is part of approximately 300 metalloenzymes involved in a series of metabolic reactions (Livingstone, 2015). In addition, it is known for its role in mitigating reactive oxygen species by elevating antioxidant metabolism in the body (Ram et al., 2016). Zinc intake has advocated the reduction in cancer cases, neurological disorders and autoimmune diseases (Chasapis et al., 2012). Besides Zn, Mn acts as an important micronutrient for the growth of humans and plants (Foy et al., 1978). Its crucial role in reproduction, carbohydrate/lipid metabolism, brain functioning and neurotransmitter synthesis is well-known (Greger, 1999; Golub et al., 2005). Manganese deficiency has shown adverse effects on plant carbohydrates, which further reduces crop yield and quality due to low pollen fertility and low carbohydrates during grain filling (Dhaliwal et al., 2023). The Mn deficiency significantly decreases the photosynthesis efficiency in plants, reducing plant productivity and dry matter yield (Rashed et al., 2021). The deficiency of Mn may result in severe health consequences in humans such as anorexia, weakness and apathy (Huang et al., 1989).
Micronutrient deficiencies in human are prevalent in regions with low plant-available concentrations of micronutrients (Cakmak, 2008; Cakmak et al., 2017). The existence of a strong relationship between soil and crop nutrient status along with human health has been already postulated (Welch et al., 2013; Cakmak and Kutman, 2018). Wheat contains lower nutrient content to satisfy human nutritional needs (Cakmak, 2008; Cakmak and Kutman, 2018). Therefore, the extreme consumption of monotonous wheat leads to micronutrient malnutrition. Hence, there is a dire need to increase the micronutrient content of wheat grain, particularly in developing countries where there is a lack of diet diversity. In recent years, work has been done to increase the micronutrient concentration in grain in particular wheat genotypes, but this study focuses on the comparative evaluation of 14 wheat genotypes through Zn and Mn fertilization for various growth parameters, proximate composition analysis, micro- and macro-nutrient concentrations.
2. Materials and methods
2.1. Field specification
The present experiment was conducted in split plot design (SPD) involving three replications, throughout both the Rabi seasons (November – April) of 2020–21 and 2021–22 at Research Farm, Department of Soil Science, PAU, Ludhiana (30° 56’ N, 75° 52′ E and 247 m above mean sea level). The texture of the soil under investigation was sandy loam exhibiting pH, EC and soil organic carbon values of 7.30, 0.35dS m−1 and 0.38%, respectively (Jackson, 1973; Dhaliwal et al., 2021a). The original content of micronutrients, i.e., Zn, Cu, Fe and Mn in soil was calculated using AAS (Varian AAS-FS 240 model) (Lindsay and Norvell, 1978) and the values were 1.20, 0.88, 5.32 and 4.17 mg kg−1, respectively. Whereas, N, P and K values were estimated to be 0.084%, 26.52 Kg ha−1 and 7.95 Kg ha−1 using alkaline KMnO4, the Olsen extractable P and neutral ammonium acetate methods, respectively (Merwin and Peech, 1950; Jackson, 1973). The climatic condition of the area was subtropical as well as hot and rainy summer followed by a drier winter. Annually the rainfall ranged between 400 and 600 mm where the highest level of rain, i.e., 70% was observed from July to September (Dhaliwal et al., 2021b).
2.2. Experimental details
In the present study, the wheat genotypes were screened through the foliar application with four treatments, i.e., control, ZnSO4.7H2O (0.5%), MnSO4.7H2O (0.5%) and ZnSO4.7H2O (0.5%) + MnSO4.7H2O (0.5%), respectively as main plots. The 14 wheat genotypes, i.e., WH-1105, HD-3086, PBW-550 (U), PBW-725, PBW-343 (U), PBW Zn 1, PBW-826, PBW-824, PDW-291, WHD-943, BWL-8855, PBW-872, PDW-274, PBW-869, procured from Punjab Agricultural University, Ludhiana, India were taken as subplots. As per treatments total of four foliar sprays were given, i.e., the first spray was applied 2–3 days before first irrigation (30 days after sowing; for effective absorption of zinc and manganese through plant foliage), the second spray at 15 days after first spray, the third spray before flowering and the fourth spray at 10 days after flowering, respectively. The land was prepared by sowing wheat with a seed-cum-fertilizer drill method at a depth of 4–6 cm. The sowing of genotypes was done in November with a row-to-row spacing of 15–20 cm. The recommended doses of fertilizers (RDF) in wheat crop, while the whole nitrogen (urea fertilizer) @ N at 125 kg ha−1 was applied as three splits and whole phosphorus (diammonium phosphate fertilizer) was applied @ P2O5 at 62.5 kg ha−1 as a basal dose. At sowing, half and the full dose of N and P were used, respectively, whereas the remaining half dose N was divided into two equal doses applied at the first as well as second irrigations.
2.3. Growth attributes
At the stage of physiological maturity, growth parameters including plant height, number of tillers and chlorophyll content were noted as the average value of five plants in each plot. The height of the plant from base to tip was noted by using a meter scale and the mean height was expressed in cm. The total tillers at maturity were calculated manually. The chlorophyll content in plant samples was measured using a chlorophyll meter (SPAD 502). It is a device developed by the soil and plant analysis department (SPAD) of Minolta Camera Co., Japan for computing chlorophyll content in leaves. The readings of the SPAD meter were recorded for the second or third fully opened leaves from the top of the main stem. The mean value of the 10 plants per plot was reported as chlorophyll meter (SPAD 502) value.
2.4. Yield study
Net plot area was used to calculate the wheat yield where the border rows were ignored and this was further written in t ha−1. The samples (grain and straw), were made to dry in a hot air oven for 3 days at 65°C and were grounded in a Lab Willey Grinder (Model Arthur H. Thomas type) manufactured by Laboratory Equipments, Pune, Maharashtra, India. The powdered samples of straw (1.0 g) and grain (0.5 g) were digested using a mixture of diacids (HNO3 and HClO4 in a ratio of 3:1; Kumar and Dhaliwal, 2021). After digestion, the samples were analyzed for Zn and Mn content using an atomic absorption spectrophotometer (Model AAS 240 FS, Varian, Germany Limited).
2.5. Estimation of certain quality parameters
Total ash, crude fiber, total soluble solids (TSS) and protein content in wheat grain were determined in the present study where the results were given as mean of triplicates.
2.5.1. Moisture content
The moisture content was calculated through the method given by AOAC (1995) where 2.0 g sample was weighed in an aluminum dish and was allowed to dry properly in an oven at 130 ± 3°C for 1 h until a constant weight was achieved. Loss in weight after cooling the sample was determined using the following formula.
M1 is mass of empty dish; M2 is mass of dish + sample (before drying); M3 is mass of dish + sample (after drying)
2.5.2. Ash content
Total ash in the sample was recorded by AOAC (1995) method where 5.0 g sample in a dish was burnt on a hot plate until blackened. The sample was allowed to cool at room temperature followed by heating in a muffle furnace at 550°C to get gray ash which was further allowed to cool and its weight was noted. The remaining weight was noted, and ash content was calculated as follows:
M1 is mass of dish before burning; M2 is mass of dish + sample after burning; M is the sample mass
2.5.3. Crude fiber
The sample (2.0 g) was added to the beaker having 200 mL of H2SO4 (1.25%) which was then heated for 30 min (AOAC, 1995). After heating, filtration of the solution was done using Whatman filter paper No. 54 and the remains were washed with hot distilled water. The residue was transferred to sodium hydroxide solution (1.25%) and heated for another 30 min which was then filtered and again washed with distilled water. The contents on filter paper were allowed to dry in an oven at 105°C until a constant weight was obtained. The contents were cooled in a desiccator and weighed. The drop in weight was attributed to the crude fiber present in the sample which was calculated using the following formula:
M1 is mass of filter paper; M2 is mass of residue + filter paper.
2.5.4. Total soluble solids
Abbe’s refractometer was used to calculate the total soluble solids in wheat grain by the index of refraction which was referred to as the degrees brix. A drop of extract from wheat grain prepared in ethyl acetate through ultra-sonication was placed on a clean and dry prism and calibration was done at zero line on the scale. Then the samples of wheat grain were analyzed for their TSS value by reading the line of demarcation on the scale of a refractometer (AOAC, 1995).
2.5.5. Protein content
Protein percentage was recorded by multiplying N content (noted by carrying out the digestion of samples using H2SO4 where total N was calculated through micro Kjeldahl’s method as reported by Jackson, 1967) by multiplying it with a factor 6.25.
Total Protein (%) = N content in the sample x 6.25
2.6. Categorization of genotypes
Categorization of efficient genotypes was done based on the background of each wheat genotype along with combined foliage application of Zn + Mn in terms of yield, quality attributes and nutrient content. Thus, the categorization of 14 genotypes showing variation in characteristics was done under Zn + Mn treatment as E (Efficient), M (Moderately efficient) and I (Inefficient).
2.6.1. Method 1
The wheat genotypes were categorized (μ) and the standard deviation (SD) of every factor under control and fertilizer application was noted (Osborne and Rengel, 2002; Aziz et al., 2017). Based on the response to Zn and Mn, genotypes showing E (Efficient), M (Moderately efficient) and I (Inefficient) characteristics had values>μ + SD (Efficient), ranging between μ + SD to μ-SD (Moderately efficient), and < μ - SD (Efficient), respectively. Like the scores 3 (E), 2 (ME), and 1(I) were assigned to E, M and I denote response to genotypes, respectively and were further ranked based on the cumulative scores.
2.6.2. Method 2
The genotypes were also categorized for efficiency and responsiveness to Zn and Mn application based on the crop yield (grain and straw) and nutrient use efficiency (NUE) (Rawal et al., 2022). Nutrient use efficiency was computed through the formula given by Manske et al. (2002):
The genotypes having higher crop yield as compared to the average yield of studied genotypes have been classified as efficient and those exhibiting higher NUE in comparison to average NUE were classified as responsive genotypes. Thus, the classification of genotypes was done under four categories, i.e., (i) efficient and responsive (ER), (ii) efficient and non-responsive (ENR) (iii) inefficient and responsive (IR) and (iv) inefficient and non-responsive (INR) as decribed by Kosar et al. (2003).
2.7. Statistical analysis
Statistical analysis of the results was done by using the SPSS package, version 16.0 (SPSS Inc., Chicago, United States of America). All attributes were calculated using two ways analysis of variance (ANOVA) for obtaining minima, maxima, mean, standard deviation (SD), standard error (SE), the mean sum of squares, F-value, P-significance along with the coefficient of variation (CV).
3. Results and discussion
3.1. Statistical analysis and study of variance for measured parameters
3.1.1. Effect of fertilizers on some growth parameters of different wheat genotypes
The descriptive statistics of plant growth parameters of 14 studied genotypes under different fertilizer applications, i.e., control, Zn application, Mn application and Zn + Mn application are presented in Table 1.
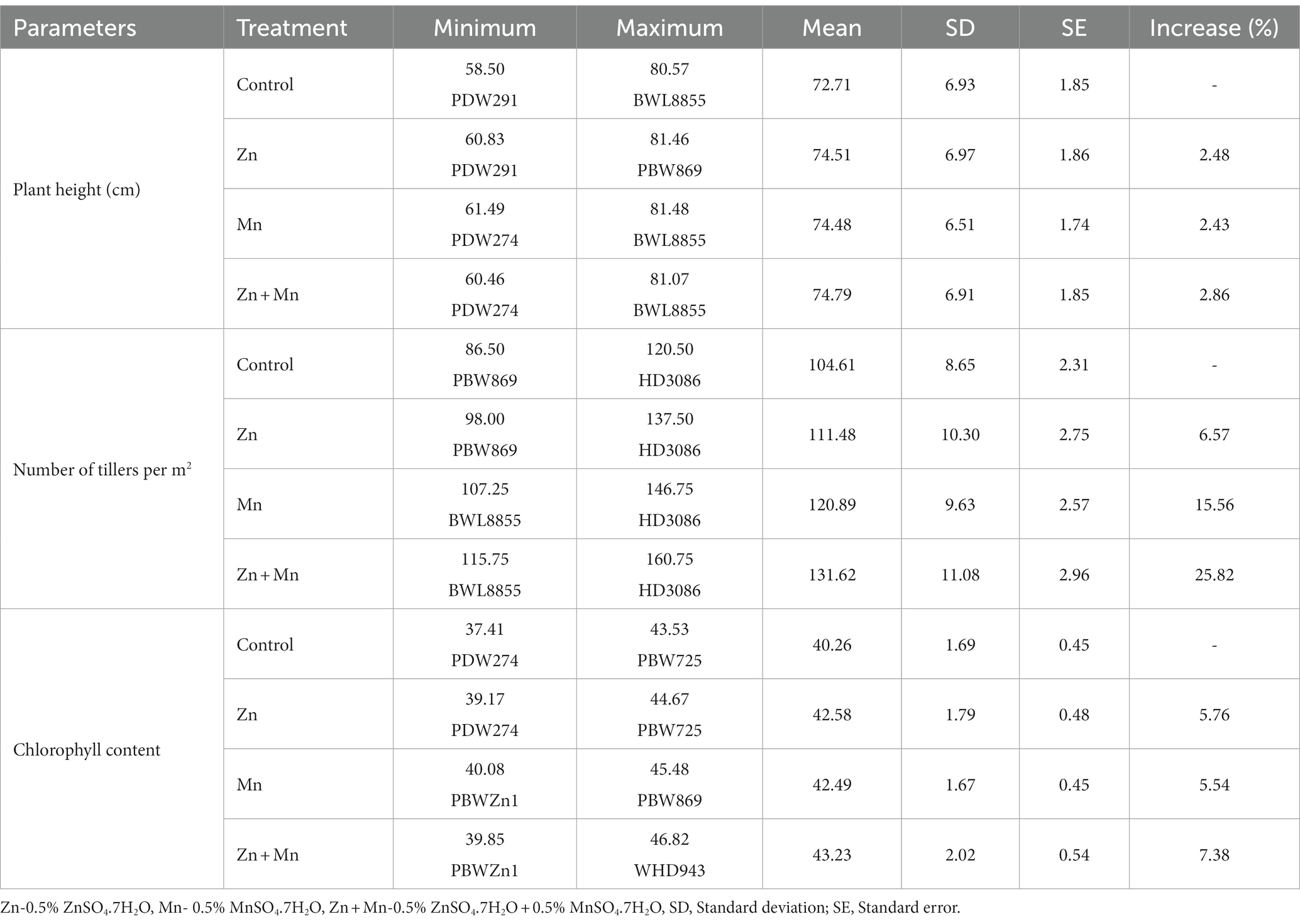
Table 1. Statistical analysis of growth parameters in wheat genotypes with different fertilizer applications (Mean data of both seasons).
The average data of plant height, tillers per plant along with chlorophyll content were higher with the Zn + Mn application compared to the control, Zn application and Mn application. The highest percentage increase was noticed for the number of tillers/plant (25.82%) followed by chlorophyll content (7.38) and plant height (2.86) under Zn + Mn application. Among the 14genotypes, the plant height ranged from 58.50(PDW291) to 80.57 cm (BWL8855) and 60.46(PDW274) to 81.07 (BWL8855) under control and Zn + Mn application, respectively, whereas the number of tillers/plant ranged from 86.50 (PBW869) to 120.50(HD3086) and 115.75 (BWL8855) to 160.75 (HD3086) under control and Zn + Mn application, respectively among the tested genotypes. Likewise, chlorophyll content varied from 37.41 (PDW274) to 43.53 (PBW725) and 39.85 (PBWZn1) to 46.82 (WHD941) under control and Zn + Mn application, respectively. The study of variance for measured parameters suggested substantial differences among various genotypes (Table 2) where the interaction between genotypes and fertilizer type was significant.
The beneficial effects of Zn fertilization on wheat have been recorded by significant improvement in plant growth attributes such as increased plant height, number of tillers per plant as well as chlorophyll content. The results were corroborated through a study by Singh et al. (2019) on wheat. The trend could be due to the higher Zn availability which favors the metabolic functions of the crop during different growth stages including chlorophyll synthesis, carbohydrate metabolism and ribosomal functioning (Kumar et al., 2019; Zulfidar et al., 2020). A significant enhancement in green gram height under Zn treatment has been observed by Muindi et al. (2020). Jatav and Singh (2018) recorded a greater improvement in plant height, chlorophyll content and number of tillers per plant of hybrid rice with Zn application.
Also, the results of the present support the hypothesis that Mn is also an essential nutrient for plant growth. The improvement in the height of plant, the number of tillers and chlorophyll content reflected the crucial role of Mn in various metabolic processes. The probable reason for the enhanced height of the plant with Mn application was because of its role in stem elongation (Marschner, 1995). Similar results with Mn application on plant height of cotton have been observed by Dordas (2009). Further, the promoting effects of Mn in chlorophyll synthesis might be due to its role in photosynthesis and as an activator of many enzymatic reactions (Kabata-Pendias and Pendias, 1999). The present study results were in agreement with the results of Shahi and Srivastava (2018) where it was stated that foliar spray of Mn enhanced the chlorophyll content in the crop. Thus, the integrated application of Zn + Mn showed a maximum increase in plant growth parameters due to their combined beneficial effects.
3.1.2. Effect of fertilizers on some quality parameters of different wheat genotypes
Different wheat genotypes were studied for their proximate composition. The descriptive statistics of five measured traits, i.e., moisture content, ash content, crude fiber, total soluble solids (TSS) and protein content among 14 studied genotypes of wheat through Zn, Mn and Zn + Mn fertilization are presented in Table 3.
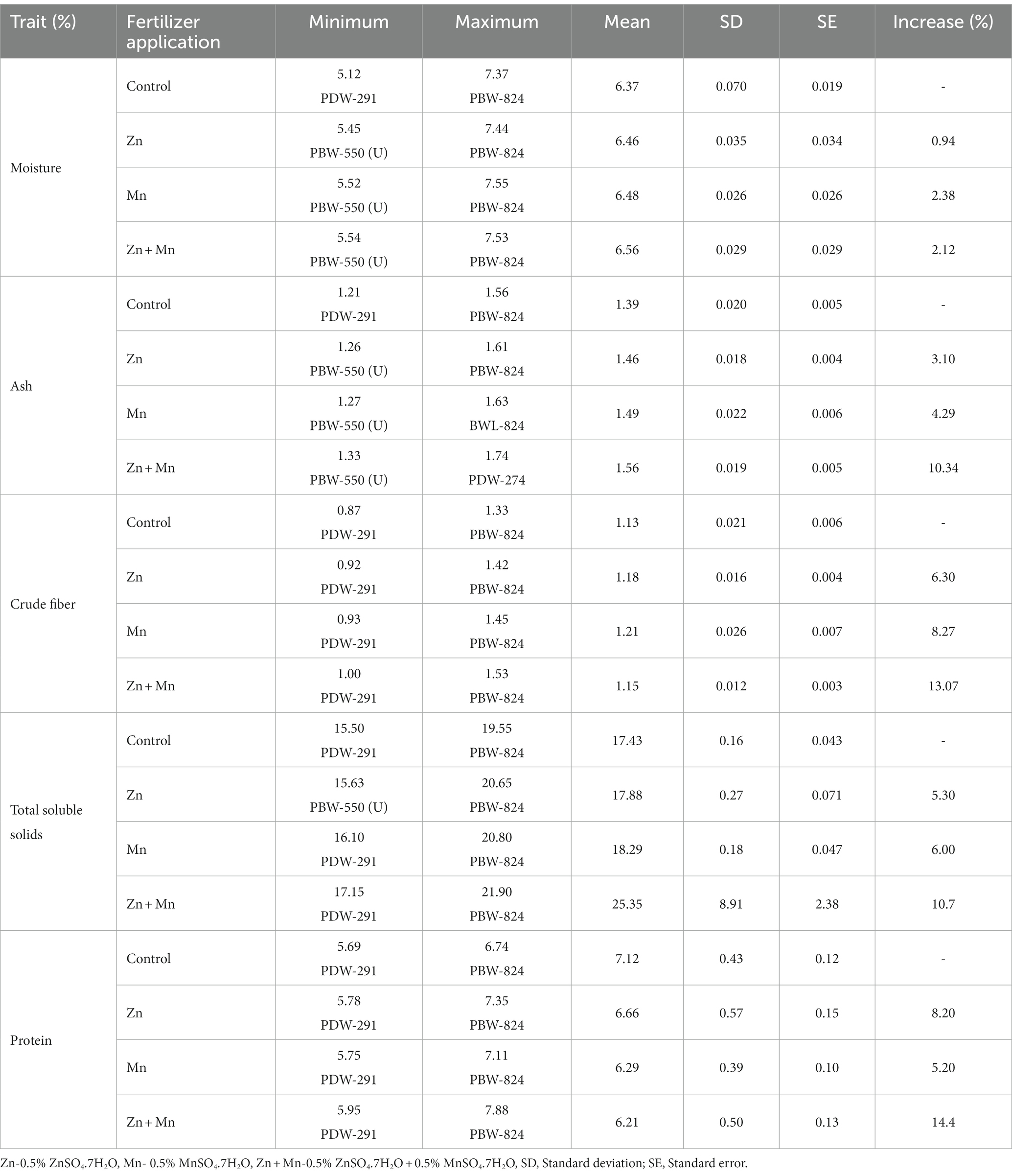
Table 3. Descriptive statistics of the moisture, ash, crude fiber, TSS and protein contents in wheat genotypes under different fertilizer applications.
The average data of moisture (%), ash (%), crude fiber (%), TSS (%) and protein content (%) were higher in the combined application of Zn and Mn with maximum values of 7.53% in PBW-824, 7.44% in PDW-274, 1.53 in PBW-824, 21.90 in PBW-824 and 7.88 in PBW-824 genotypes, respectively. The highest percentage increase of 14.4% was observed in protein content for the collective use of Zn as well as Mn in comparison to control. Further, crude fiber and TSS showed an increase of 13.07 and 10.7%, respectively. Overall, moisture, ash, crude fiber, TSS and protein contents varied from 5.12 to 7.53%, 1.21 to 1.74%, 0.87 to 1.53%, 15.50 to 21.90% and 5.69 to 7.88%, respectively with minimum values observed in HD-3086 genotype. The analysis of variance (ANOVA) for measured parameters showed substantial differences among various genotypes (Table 4) where the interaction between genotypes and fertilizer type was significant.
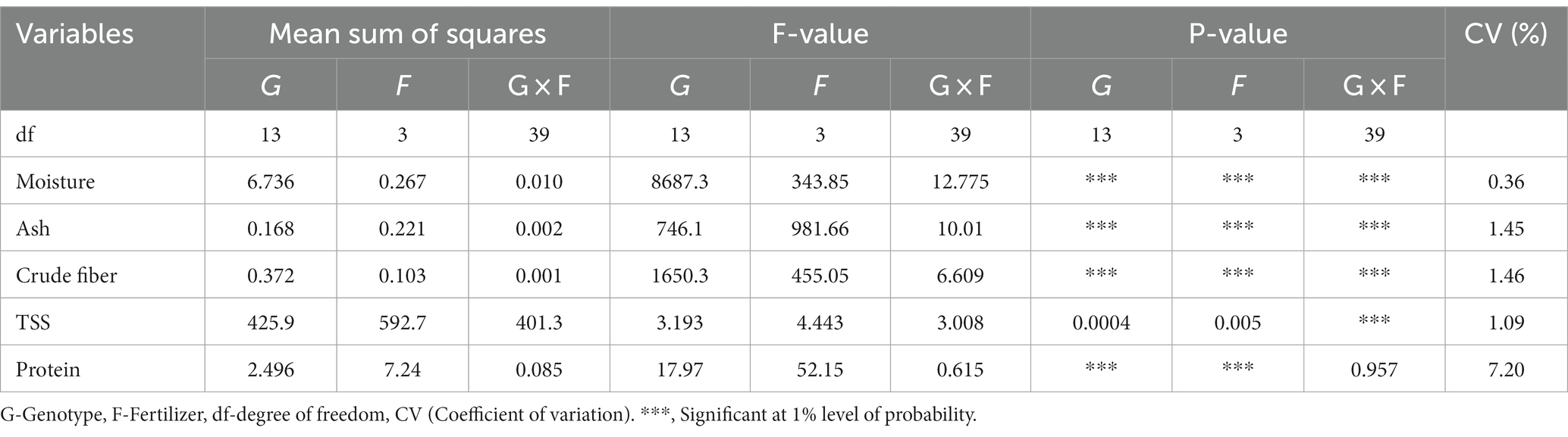
Table 4. Analysis of variance for moisture, ash, crude fiber, TSS and protein content under different fertilizer applications.
The coefficient of variation varied from 0.36% (moisture content) to 7.20% (protein content) among the traits investigated. The results pertaining to the moisture content in 14 genotypes of wheat undertaken in the present study revealed the superiority of combined Zn and Mn application over other treatments. The outcomes were in agreement with the results obtained by Punia et al. (2019) where the moisture content ranged from 7.79 to 9.45% among different genotypes. These variations in moisture contents among various wheat genotypes with collective use of Zn and Mn were major because of variations in levels of sun drying for crops when harvested. Moreover, the moisture content could also be determined through the genetic composition of grain along with variations in climate (Maity et al., 2016). Two aspects are mainly associated with grain moisture content. Primarily, the increased moisture may result in enhanced kernel weight. Furthermore, it may reduce the total storage period of grain (Abramson et al., 2005). Similar results were observed by Asim et al. (2018) for a percentage of moisture in different wheat genotypes. Whereas, the percentage of ash in the present experiment was in accordance with the results observed by Amir et al. (2013). Various environmental conditions like fertility of soil, temperature and type of fertilizer used majorly contribute toward the variation in ash content for different genotypes. The percentage increase in crude fiber with Zn and Mn application was because of the important role of Zn in metabolic reactions and synthesis of protein that ultimately affected the properties of fiber (Nofal et al., 2011). In one study, the percentage of fiber varied between 0.79 to 0.93% in wheat which was lesser in comparison to the present study (Punia et al., 2019).
Additionally, the TSS level increased with Zn and Mn application as compared to the control. An increase in chlorophyll levels in leaves through Zn application resulted in improved rates of photosynthesis which further improved the synthesis of sugars as well as carbohydrates (Hussain et al., 2020). Increased TSS was also related to the higher photosynthate translocation to wheat grain over other sections of the plant (Borah et al., 2021). Also, the increased content of protein in wheat grain under Zn and Mn treatment was because of enhanced nitrogen fixation along with greater levels of available nitrogen in soil as well as improved uptake of nitrogen by plants. Zinc is responsible for protein metabolism involving various enzyme systems and translocating protein to wheat grain enhancing the overall percentage of protein in wheat (Amir et al., 2020). Different factors affecting protein content include drought stress, fertility of the soil, thermal stress and practices related to agriculture (Li et al., 2013; Mutwali et al., 2016). Wheat genotypes exhibited different levels of protein content which ultimately resulted in a significant enhancement in its quality which added nutritional value to various genotypes.
3.1.3. Impact of fertilizers on grain and straw yield in different wheat genotypes
Among 14 wheat genotypes, PBW-824 produced significantly highest grain yield (5.14 t ha−1for control, 5.25 t ha−1 for Zn treatment, 5.48 t ha−1 under Mn treatment and 5.42 t ha−1 under Zn + Mn treatment, respectively) and the lowest grain yield was recorded in HD 3086 genotype (Table 5). Whereas, the maximum values of straw yield for PBW-824 were observed to be 8.60 t ha−1for control, 8.87 t ha−1 for Zn treatment, 8.83 t ha−1 under Mn treatment and 9.11 t ha−1 under Zn + Mn treatment, respectively (Table 6). For the control treatment, the minimum value of straw yield was observed in HD 3086 genotype, whereas, under Zn, Mn and Zn + Mn treatments minimum straw yield was obtained in PBW-550 (U) genotype.
The enhanced grain, as well as straw yield observed through combined fertilization of Zn, was due to their favorable effect on wheat productivity and the role of Zn as a catalyst or stimulant in many physiological as well as metabolic processes. Also, Zn acts as a metal activator of enzymes which help in the synthesis of carbohydrates and proteins. Further, the improved levels of growth attributes like chlorophyll, leaf area index, height of plant and better root system of wheat were observed through Zn supplementation (Hussain et al., 2020). The role of Zn in the synthesis of carbohydrates, and lipids along with nucleic acid also improved the wheat yield due to their importance in growth and plant development (Khan et al., 2002). Indeed, Zn also plays a significant role in the functioning of pollen, fertilization as well as germination (Aravind and Prasad, 2003; Pandey et al., 2006; Cakmak, 2008) that automatically leads to enhanced crop performance (Shojaei and Makarian, 2015). Comparable results were reported in the literature where ZnSO4 foliar application improved seed and straw yield in field pea (Shankar et al., 2004; Verma et al., 2018; Borah et al., 2021). On the other hand, Mn holds the central position in the oxygen-evolving complex during photosystem II which confirms its importance as compared to other nutrients in photosynthesis. Improved yield parameters observed in the present investigation might be related to enhanced Zn and Mn supply which resulted in proper growth and development in wheat (Ullah et al., 2017).
3.1.4. Effect of fertilizers on micro–and macro-nutrient concentrations in different wheat genotypes
The statistical analysis of the nutrient content of 14 studied genotypes under different fertilizer applications showed that nutrient content significantly increased with the application of fertilizers (Table 7).
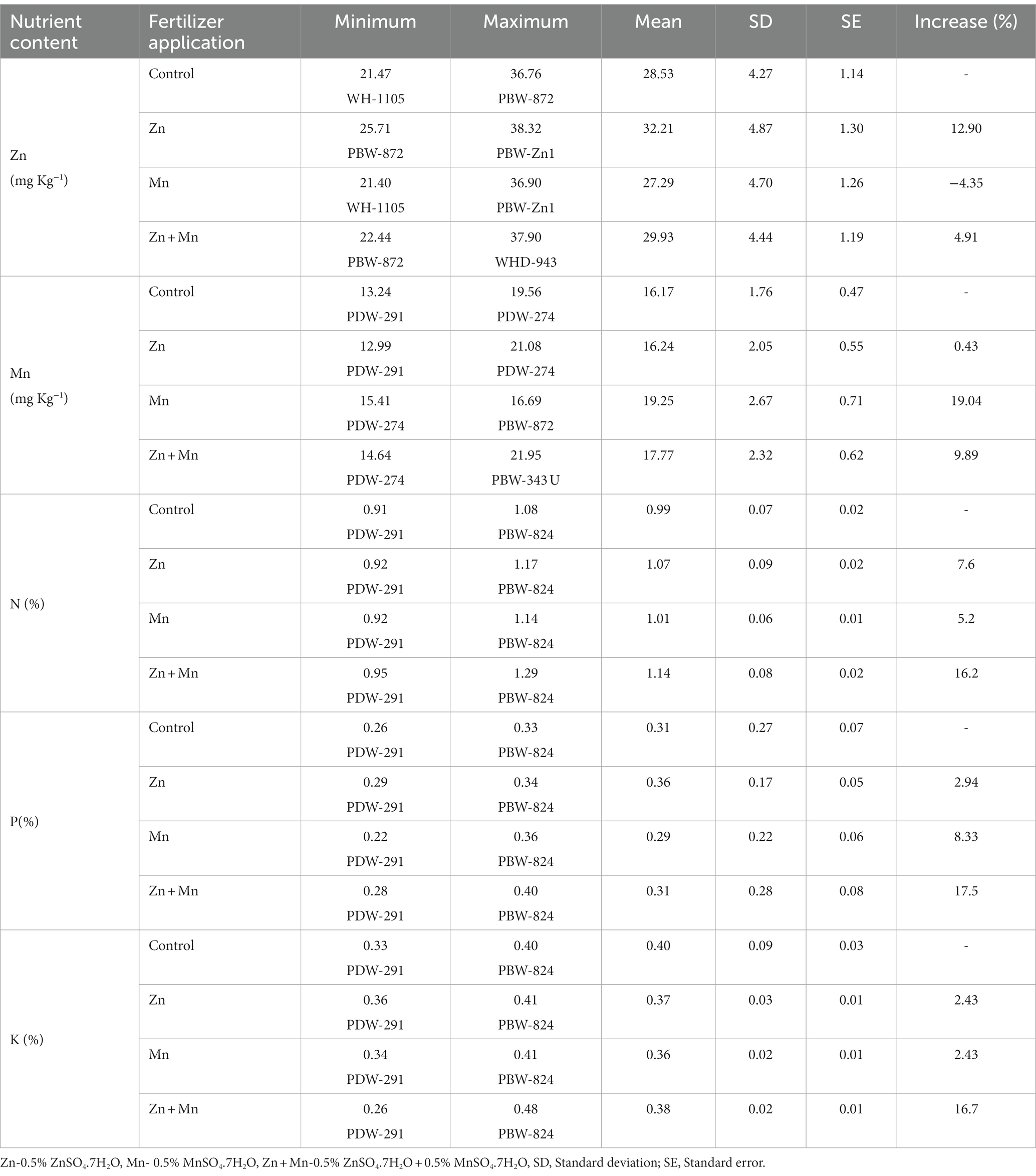
Table 7. Descriptive statistics of the Zn, Mn, N, P and K concentrations in wheat genotypes under different fertilizer applications.
The highest increase in Zn and Mn content was observed under the sole application of Zn (12.90%) and Mn (19.04%), respectively in comparison to the combined Zn + Mn application. The concentration of Zn ranged from 21.47 (WH-1105) to 36.76 (PBW-872) mg kg−1 in control and 25.71 (PBW-872) to 38.32 (PBW-Zn1) mg kg−1 under Zn application, whereas Mn content ranged from 13.24 (PDW-291) to 19.56 (PDW-274) mg kg−1 in control and 15.41 (PDW-274) to 23.59(PBW-872) mg kg−1under Mn application. Whereas, the results pertaining to the concentrations of N, P and K showed that their mean values were higher in the combined application of Zn and Mn with maximum values of 1.29, 0.40 and 0.48% for N, P and K in PBW-824 cultivar. The highest percentage increase of 17.50% was observed in P content for the collective use of Zn and Mn in comparison to control followed by K content of 16.70%. Overall, N, P and K content ranged between 0.91 to 1.29%, 0.26 to 0.40% and 0.33 to 0.48%, respectively, with minimum values observed in PDW-291 wheat cultivar. The study of variance for measured parameters suggested substantial differences among various genotypes (Table 8) where the interaction between genotypes and fertilizer type was significant. Whereas, the coefficient of variation between Zn, Mn, N, P and K ranged from 7.20% (N content) to 15.23% (Zn content) among the investigated traits.
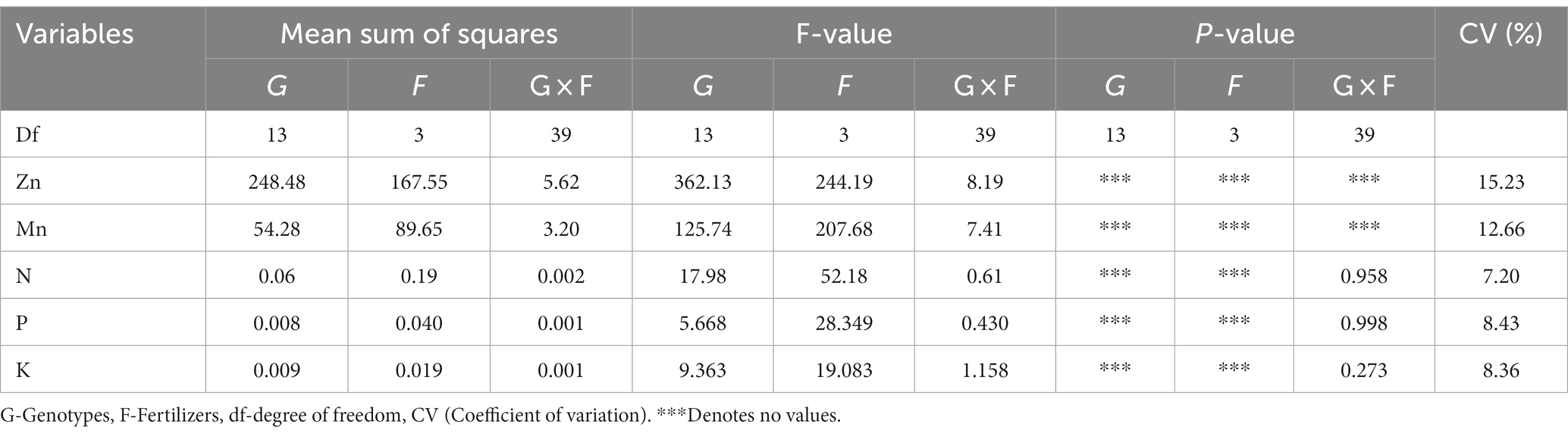
Table 8. Study of variance for Zn, Mn, N, P and K concentrations under different fertilizer applications.
The results pertaining to the macronutrient contents in 14 genotypes of wheat undertaken in the present study predicted the superiority of combined Zn and Mn application through sprays on plant foliage as compared with other treatments. Utilization of Zn and Mn mineral elements by a plant varies with the hereditability of each genotype (Sadeghi et al., 2021). Among different varieties, the significant differences in Zn and Mn concentrations and their variable response toward nutrient application have also been reported in previous studies (Mathpal et al., 2015). This difference might be due to variations in accumulation, translocation and utilization mechanisms because of variable genetic makeup (Fageria and Baligar 2003). Remarkable improvement in grain Zn and Mn concentration with Zn and Mn application, respectively, was due to the effective absorption of nutrients on plant leaves and its mobility in wheat through crease phloem, and transported to endosperm (Cakmak et al., 2010). A similar trend of increased Zn content on Zn applications has been reported in previous studies. In line with the present study, Dhaliwal et al. (2021a) reported a significant upsurge in Zn concentration in chickpeas under the Zn application. Similar improvement in Zn concentration in linseed has also been recorded with Zn application (Dhaliwal et al., 2022). The lower Zn and Mn concentration in Zn + Mn treated plants as compared to the sole application of nutrients might be due to the dilution effect.
In the present experiment, the Zn and Mn application significantly increased N, P and K concentrations in wheat grains as compared to the control. Increased consumption, as well as absorption of Zn and Mn in plants, positively affected their concentrations in wheat. Zn and Mn not only enable their own absorption, but they also help in improving the absorption of nutrients such as N, P and K in the plant. The leaf epidermis absorbs fertilizer-applied Zn and Mn through stomata as well as trichomes (DomõÂnguez et al., 2011). This accelerates the macronutrient content and their uptake especially N as compared to P and K in wheat genotypes by increasing the level of amino acids needed for the synthesis of protein (Mohammad and Naseem, 2006). Similarly, the increase in Zn and N content through Zn fertilization was reported by Ghasal et al. (2017). Also, Pooniya and Shivay (2011, 2013) noted a significant enhancement in N, P and K concentrations with Zn application.
3.1.5. Categorization of wheat genotypes for nutrient use efficiency
Under method 1, the different genotypes displayed significant variations in quality attributes like protein, crude fiber, TSS and nutrient concentration considered for ranking in both control and Zn + Mn treatment (Table 9).
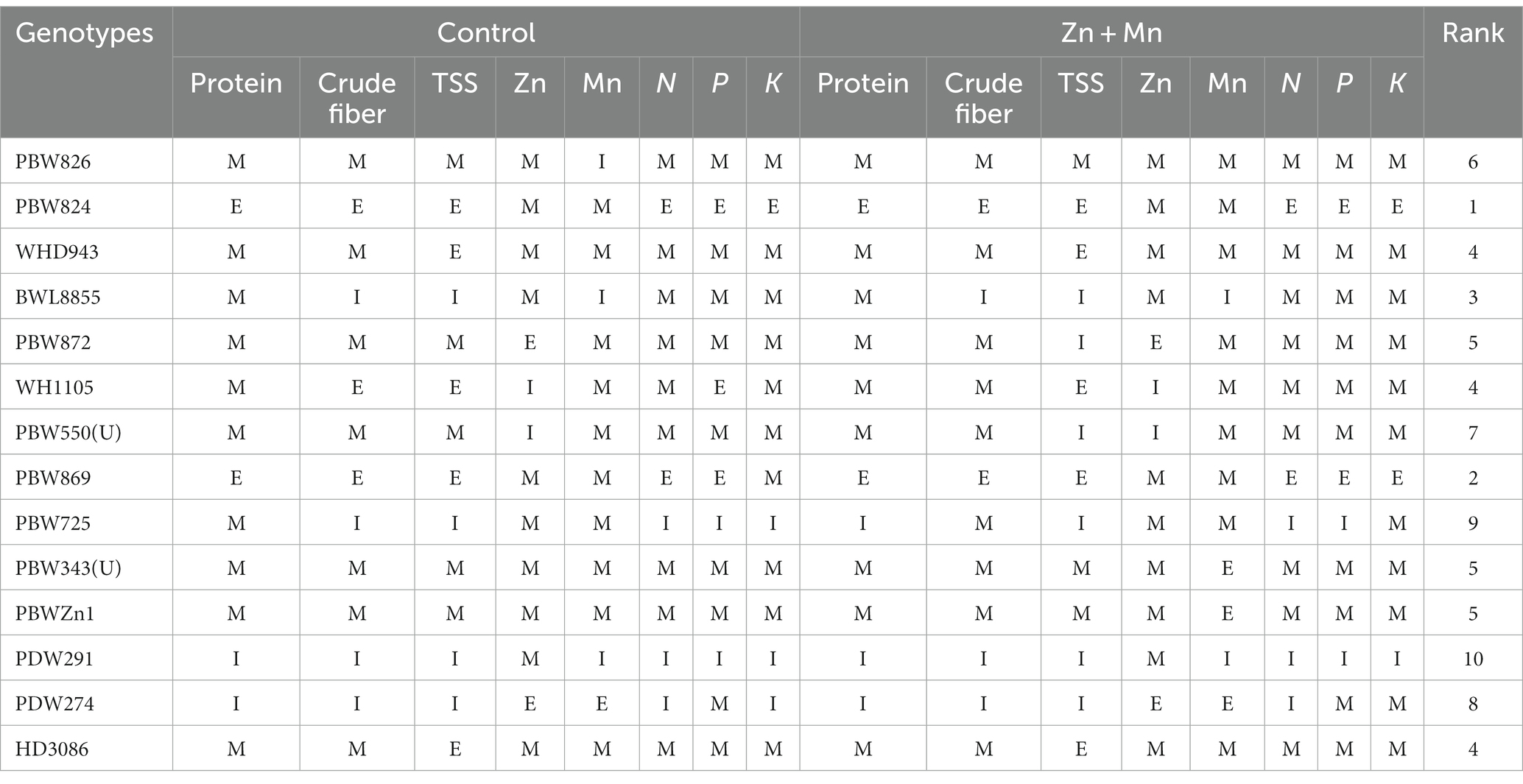
Table 9. Genotype classifications into efficient (E), medium (M) and inefficient (I) based on various traits measured under control treatment.
The genotypes PBW-824 recorded the highest rank overall followed by PBW-869. On the other hand, PDW-274, PDW-725 and PDW-291 recorded the lowest ranks among the studied genotypes. Further, the studied genotypes under method 2 were classified into four groups based on total dry mass as well as nutrient use efficiency in Zn + Mn treatment (Figure 1). The genotypes PBW 872, PDW-869 and PBW-Zn1 were classified into ER group. Further, the genotypes WHD 943, WH 1105, PBW 725, PBW 343 and PDW-291 were classified in INR group. Additionally, the genotypes PBW 824 and BWL 8855 were categorized in ENR group with the fertilizer treatment of Zn + Mn. both P conditions. Whereas, genotypes PBW-826, PBW 550(U), PDW 274 and HD 3086 were categorized in the IR group under the Zn + Mn treatment.
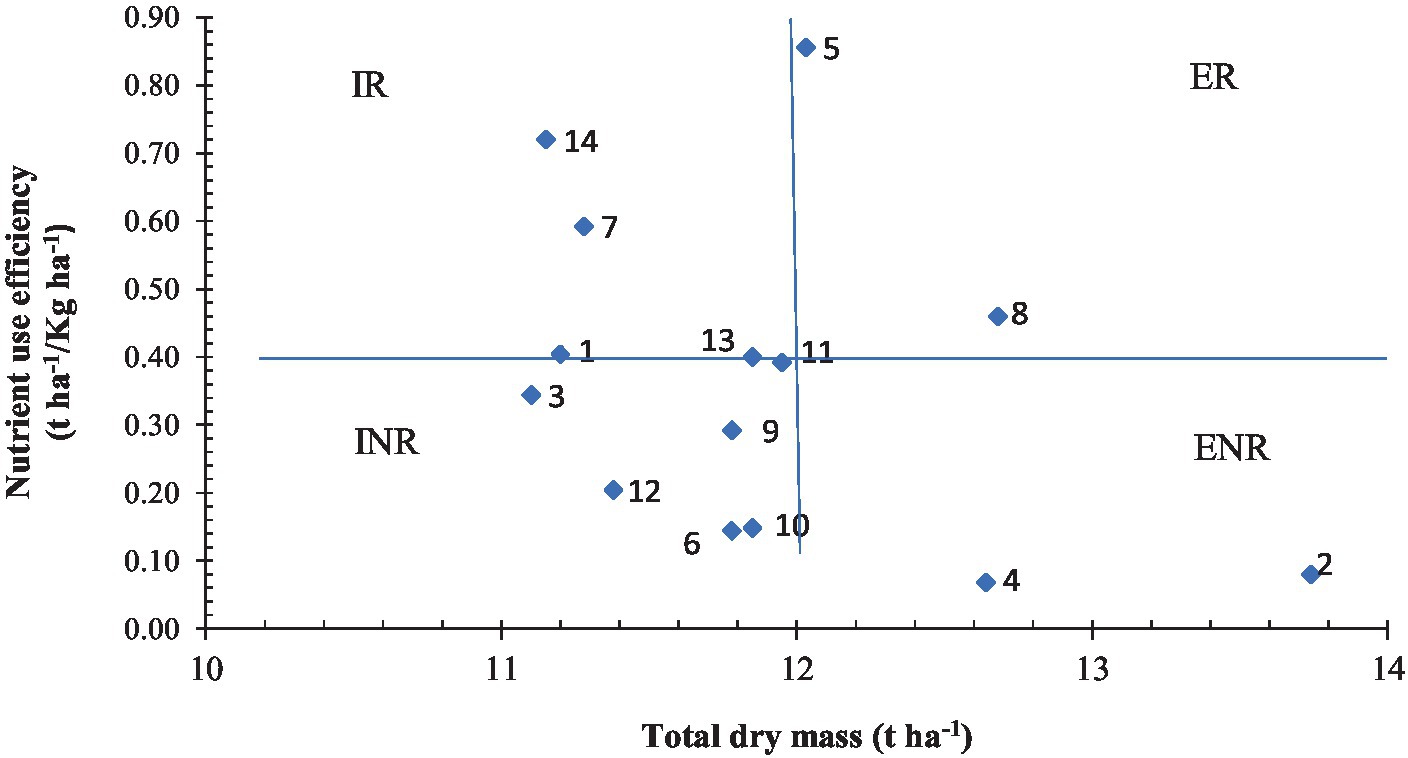
Figure 1. Genotype classifications based on nutrient use efficiency and total dry mass. This divides genotypes into four groups such as efficient with responsive (ER), inefficient with responsive (IR), efficient with non-responsive (ENR) and inefficient with non-responsive (INR).(1) PBW-826, (2) PBW-824, (3) WHD-943. (4) BWL-8855, (5) PBW-872, (6) WH-1105, (7) PBW-550 (U), (8) PBW-869, (9) PBW-725, (10) PBW-343 (U), (11) PBW Zn1, (12) PDW-291, (13) PDW-274 and (14) HD-3086.
4. Conclusion
The present study concluded that the studied 14 wheat genotypes differ significantly toward yield, nutrient content and other quality parameters. The genotypes were categorized for yield and quality parameters using two different techniques under control and Zn + Mn treatment. The plant growth parameters, crop yield, nutrient content and quality parameters significantly increased under Zn + Mn treated conditions. The genotypes PBW-824 and BWL-8855 produced a higher yield under control conditions, whereas PBW-872, PBW-869 and PBW Zn1 showed a higher yield under Zn + Mn treatment. Further, PBW-824 and PBW-869 were ranked higher in terms of quality parameters. Thus, these genotypes could be further studied for higher crop production to reduce micronutrient malnutrition in humans.
Data availability statement
The original contributions presented in the study are included in the article/supplementary material, further inquiries can be directed to the corresponding authors.
Author contributions
SD, VS, AS, VV, MK, and PS: conceptualization, investigation, methodology, resources, validation, and writing–original draft. SD, SB, SA, MS, and AH: data curation and writing–review and editing. SD, SB, SA, MS, and AH: formal analysis and software. SD, SB, and AS: supervision. SD, SB, SA, AS, and AH: funding acquisition. SB: resources. All authors contributed to the article and approved the submitted version.
Funding
This research was funded by ICAR- Indian Institute of Soil Science, Bhopal, 462038, Madhya Pradesh, India with the support of the Department of Soil Science, Punjab Agricultural University, Ludhiana, India. This study was also funded by the Researchers Supporting Project number (RSP2023R194), King Saud University, Riyadh, Saudi Arabia.
Acknowledgments
The authors are thankful to the Indian Council for Agricultural Research (ICAR), Indian Institute of Soil Science (IISS), Bhopal. The authors would like to extend their sincere appreciation to the Researchers Supporting Project number (RSP2023R194), King Saud University, Riyadh, Saudi Arabia.
Conflict of interest
The authors declare that the research was conducted in the absence of any commercial or financial relationships that could be construed as a potential conflict of interest.
Publisher’s note
All claims expressed in this article are solely those of the authors and do not necessarily represent those of their affiliated organizations, or those of the publisher, the editors and the reviewers. Any product that may be evaluated in this article, or claim that may be made by its manufacturer, is not guaranteed or endorsed by the publisher.
References
Abramson, D., Hulasare, R., York, R. K., White, N. D. G., and Jayas, D. S. (2005). Mycotoxins, ergosterol, and odor volatiles in durum wheat during granary storage at 16 and 20% moisture content. J. Stored Prod. Res. 41, 67–76. doi: 10.1016/j.jspr.2003.11.002
Amir, R. M., Anjum, F. M., Khan, M. I., Khan, M. R., Pasha, I., and Nadeem, M. (2013). Application of Fourier transform infrared (FTIR) spectroscopy for the identification of wheat varieties. J. Food Sci. Technol. 50, 1018–1023. doi: 10.1007/s13197-011-0424-y
Amir, R. M., Hussain, M. N., Ameer, K., Ahmed, A., Ahmad, A., Nsdeem, M., et al. (2020). Comprehensive assessment and evaluation of selected wheat varieties for their relationship to chapatti quality attributes. Food Sci. Technol. 40, 436–443. doi: 10.1590/fst.31619
AOAC. (1995). Official methods of analysis, 16th Edn.. Washington, DC: Association of Official Analytical Chemists.
Aravind, P., and Prasad, M. N. V. (2003). Zinc alleviates cadmium-induced oxidative stress in Ceratophyllum demersum L.: a free floating freshwater macrophyte. Plant Physiol. Biochem. 41, 391–397. doi: 10.1016/S0981-9428(03)00035-4
Asim, S. M., Ahmed, A., Amir, R. M., and Nadeem, M. (2018). Comprehensive identification and evaluation of selected wheat cultivars for their relationship to pan bread quality. J. Food Proc. Preserv. 42:e13670. doi: 10.1111/jfpp.13670
Aziz, M. M., Palta, J. A., Siddique, K. H., and Sadras, V. O. (2017). Five decades of selection for yield reduced root length density and increased nitrogen uptake per unit root length in Australian wheat varieties. Plant Soil 413, 181–192. doi: 10.1007/s11104-016-3059-y
Borah, L., Saikia, J., and Basumatary, A. (2021). Effect of foliar application of zinc on growth and yield of garden pea (Pisum sativum L.) in Assam condition. Int. J. Chem. Stud. 9, 869–872. doi: 10.22271/chemi.2021.v9.i2m.11927
Cakmak, I. (2008). Enrichment of cereal grains with zinc: agronomic or genetic biofortification? Plant Soil 302, 1–17. doi: 10.1007/s11104-007-9466-3
Cakmak, I., and Kutman, U. B. (2018). Agronomic biofortification of cereals with zinc: a review. Eur. J. Soil Sci. 69, 172–180. doi: 10.1111/ejss.12437
Cakmak, I., Mclaughlin, M. J., and White, P. (2017). Zinc for better crop production and human health. Plant Soil 411, 1–4. doi: 10.1007/s11104-016-3166-9
Cakmak, I., Pfeiffer, W. H., and McClafferty, B. (2010). Biofortification of durum wheat with zinc and iron. Cereal Chem. 87, 10–20. doi: 10.1094/CCHEM-87-1-0010
Chasapis, C. T., Loutsidou, A. C., Spiliopoulou, C. A., and Stefanidou, M. E. (2012). Zinc and human health: an update. Arch. Toxicol. 86, 521–534. doi: 10.1007/s00204-011-0775-1
Chattha, M. U., Hassan, M. U., Khan, I., Chattha, M. B., Mahmood, A., Chattha, M. U., et al. (2017). Biofortification of wheat cultivars to combat zinc deficiency. Front. Plant Sci. 8, 1–8. doi: 10.3389/fpls.2017.00281
Dhaliwal, S. S., Sharma, V., and Shukla, A. K. (2022). Impact of micronutrients in mitigation of abiotic stresses in soils and plants–a progressive step toward crop security and nutritional quality. Adv. Agron. 173, 1–78. doi: 10.1016/bs.agron.2022.02.001
Dhaliwal, S. S., Sharma, V., Shukla, A. K., Kaur, J., Verma, V., Kaur, M., et al. (2023). Differential response of diverse wheat genotypes to Mn application for yield and quality parameters under Mn deficient soil of North-Western India. J. Plant Nutr. 46, 3697–3714. doi: 10.1080/01904167.2023.2211596
Dhaliwal, S. S., Sharma, V., Shukla, A. K., Verma, V., Behera, S. K., Singh, P., et al. (2021b). Comparative efficiency of mineral, chelated and nano forms of zinc and iron for improvement of zinc and iron chickpea (Cicer arietinum L.) through biofortification. Agronomy 11:2436. doi: 10.3390/agronomy11122436
Dhaliwal, S. S., Sharma, V., Taneja, P. K., Shukla, A. K., Kaur, L., Verma, G., et al. (2021a). Effect of cadmium and ethylenediaminetetraacetic acid supplementation on cadmium accumulation by roots of Brassica species in cd spiked soil. Environ. Sci. Pollut. Res. 29, 1–10. doi: 10.1007/s11356-021-16084-7
Dhaliwal, S. S., Shukla, A. K., Sharma, V., Behera, S. K., Choudhary, O. P., Chaudhari, S. K., et al. (2020). Status of Sulphur and micronutrients in soils of Punjab-Blockwise atlas. Department of Soil Science, Punjab Agricultural University, Ludhiana and ICAR-Indian Institute of Soil Science, Bhopal, India, pp. 1–210.
DomõÂnguez, E., Heredia-Guerrero, J. A., and Heredia, A. (2011). The biophysical design of plant cuticles, an overview. New Phytol. 189, 938–949. doi: 10.1111/j.1469-8137.2010.03553.x
Dordas, C. (2009). Foliar application of manganese increases seed yield and improves seed quality of cotton grown on calcareous soils. J. Plant Nutr. 32, 160–176. doi: 10.1080/01904160802609013
Fageria, N. K., and Baligar, V. C. (2003). Methodology for evaluation of lowland rice genotypes for nitrogen use efficiency. J. Plant Nutr. 26, 1315–1333. doi: 10.1081/PLN-120020373
Foy, C. D., Chaney, R. L., and White, M. C. (1978). The physiology of metal toxicity in plants. Annu. Rev. Plant Physiol. 29, 511–566. doi: 10.1146/annurev.pp.29.060178.002455
Ghasal, P. C., Shivay, Y. S., Pooniya, V., Choudhary, M., and Verma, R. K. (2017). Zinc fertilization effect on macro and micro-nutrients concentrations and uptake in wheat (Triticum aestivum) varieties. Indian J. Agron. 62, 470–475.
Golub, M. S., Hogrefe, C. E., and Germann, S. L. (2005). Neurobehavioral evaluation of rhesus monkey infants fed cow’s milk formula, soy formula, or soy formula with added manganese. Neurotoxicol. Teratol. 27, 615–627. doi: 10.1016/j.ntt.2005.04.003
Greger, J. L. (1999). Nutrition versus toxicology of manganese in humans: evaluation of potential biomarkers. Neurotoxicology 20, 205–212.
Huang, C. C., Chu, N. S., and Lu, C. S. (1989). Chronic manganese intoxication. Arch. Neurol. 46, 1104–1106. doi: 10.1001/archneur.1989.00520460090018
Hussain, M., Kanwal, A., Khan, M. B., Naeem, M., Rizwan, M. S., and Hye, M. Z. U. (2020). Basal application of zinc to improve mung bean yield and zinc-grains-biofortification. Phyton 89, 87–96. doi: 10.32604/phyton.2020.07845
Jatav, S. S., and Singh, S. K. (2018). Evaluation of different methods of zinc application on growth and yield of hybrid rice (Oryza sativa L.) in inceptisol of Varanasi. J. Pharmacogn. Phytochem. 7, 1739–1744.
Kabata-Pendias, A., and Pendias, H. (1999).Biogeochemistry of trace elements. Warsaw, Poland: PanstowoweWydawnictwoNaukowe.
Khan, M. U., Qasim, M., and Jamil, M. (2002). Effect of different levels of zinc on the extractable zinc content of soil and chemical composition of rice. Asian J. Plant Sci. 1, 20–21.
Kosar, H., Gill, M., Aziz, T., and Tahir, M. (2003). Relative phosphorus utilization efficiency of wheat genotypes in hydroponics. Pak. J. Agric. Sci. 40:28–32.
Kumar, B., and Dhaliwal, S. S. (2021). Zinc biofortification of dual-purpose cowpea [Vigna unguiculata (L.) Walp.] for enhancing the productivity and nutritional quality in a semi-arid region of India. Arch. Agron. Soil Sci. 68, 1034–1048. doi: 10.1080/03650340.2020.1868040
Kumar, D., Dhar, S., Kumar, S., Meena, D. C., and Meena, R. B. (2019). Effect of zinc application on yield attributes and yield of maize and wheat in maize-wheat cropping system. Int. J. Curr. Microbiol. App. Sci. 8, 1931–1941. doi: 10.20546/ijcmas.2019.801.203
Lindsay, W. L., and Norvell, W. A. (1978). Development of a DTPA soil test for zinc, iron, manganese, and copper. Soil Sci. Soc. Am. J. 42, 421–428. doi: 10.2136/sssaj1978.03615995004200030009x
Livingstone, C. (2015). Zinc: physiology, deficiency, and parenteral nutrition. Nutr. Clin. Pract. 30, 371–382. doi: 10.1177/0884533615570376
Li, Y., Wu, Y., Hernandez-Espinosa, N., and Peña, R. J. (2013). The influence of drought and heat stress on the expression of end-use quality parameters of common wheat. J. Cereal Sci. 57, 73–78. doi: 10.1016/j.jcs.2012.09.014
Maity, A., Vijay, D., Mukherjee, A., and Lamichaney, A. (2016). “Potential impacts of climate change on quality seed production: a perspective of hill agriculture” in Conservation agriculture. eds. J. K. Bisht, V. S. Meena, P. K. Mishra, and A. Pattanayak, vol. 5 (Singapore: Springer), 459–485.
Manske, G. G. B., Ortiz-Monasterio, J. I., Ginkel, R. M. V., Rajaram, S., and Vlek, P. L. G. (2002). Phosphorus use efficiency in tall, semi-dwarf and dwarf near-isogenic linesof spring wheat. Euphytica 125, 113–119. doi: 10.1023/A:1015760600750
Mathpal, B., Srivastava, P. C., Shankhdhar, D., and Shankhdhar, S. C. (2015). Improving key enzyme activities and quality of rice under various methods of zinc application. Physiol. Mol. Biol. Plants 21, 567–572. doi: 10.1007/s12298-015-0321-3
Merwin, H. D., and Peech, M. (1950). Exchangeability of soils potassium in the sand, silt and clay fractions as influenced by the nature of the complementary exchangeable cations. Soil Sci. Soc. Am. J. 15, 125–128. doi: 10.2136/sssaj1951.036159950015000C0026x
Mohammad, F., and Naseem, U. (2006). Effect of K application on leaf carbonic anhydrase and nitrate reductase activities, photosynthetic characteristics, NPK and NO contents, growth and yield of mustard. Photosynthetica 44, 471–473. doi: 10.1007/s11099-006-0053-2
Muindi, C. M., Ndiso, J. B., and Muindi, E. M. (2020). Effect of zinc application method on growth and yield of green grams.International journal of agriculture. Environ. Biores. 5, 1–10.
Mutwali, N. I., Mustafa, A. I., Gorafi, Y. S., and Mohamed-Ahmed, I. A. (2016). Effect of environment and genotypes on the physicochemical quality of the grains of newly developed wheat inbred lines. Food Sci. Nutr. 4, 508–520. doi: 10.1002/fsn3.313
Nofal, O. A., Zedian, M. S., and Bakry, B. A. (2011). Flax yield and quality traits as affected by zinc foliar application under newly reclaimed sandy soils. J. Appl. Sci. Res. 7, 1361–1367.
Osborne, L. D., and Rengel, Z. (2002). Genotypic differences in wheat for uptake and utilisation of P from iron phosphate. Aust. J. Agric. Res. 53, 837–844. doi: 10.1071/AR01080
Pandey, N., Pathak, G. C., and Sharma, C. P. (2006). Zinc is critically required for pollen function and fertilization in lentil. J. Trace Elem. Med. Biol. 20, 89–96. doi: 10.1016/j.jtemb.2005.09.006
Pooniya, V., and Shivay, Y. S. (2011). Effect of green manuring and zinc fertilization on productivity and nutrient uptake in basmati rice (Oryza sativa)–wheat (Triticum aestivum) cropping system. Indian J. Agron. 56, 28–34.
Pooniya, V., and Shivay, Y. S. (2013). Enrichment of basmati rice grain and straw with zinc and nitrogen through ferti-fortification and summer green manuring crops under indo-Gangetic Plains of India. J. Plant Nutr. 36, 91–117. doi: 10.1080/01904167.2012.733052
Punia, S., Sandhu, K. S., and Siroha, A. K. (2019). The difference in protein content of wheat (Triticum aestivum L.): effect on functional, pasting, colour and antioxidant properties. J. Saudi Soc. Agric. Sci. 18, 378–384. doi: 10.1016/j.jssas.2017.12.005
Ram, H., Rashid, A., Zhang, W., Duarte, A. P., Phattarakul, N., Simunji, S., et al. (2016). Biofortification of wheat, rice and common bean by applying foliar zinc fertilizer along with pesticides in seven countries. Plant Soil 403, 389–401. doi: 10.1007/s11104-016-2815-3
Rashed, M. H., Hoque, T. S., Jahangir, M. R., and Hashem, M. A. (2021). Manganese as a micronutrient in agricul-ture: crop requirement and management. J. Environ. Sci. Nat. Resour. 12, 225–242. doi: 10.3329/jesnr.v12i1-2.52040
Rawal, N., Pande, KR., Shrestha, R., and Vista, SP. (2022). Nutrient use efficiency (NUE) of wheat (Triticum aestivum L.) as affected by NPK fertilization. PLoS One. 17:e0262771. doi: 10.1371/journal.pone.0262771
Sadeghi, F., Rezeizad, A., and Rahimi, M. (2021). Effect of zinc and magnesium fertilizers on the yield and some characteristics of wheat (Triticum aestivum L.) seeds in two years. International Journal of Agronomy 2021, 1–6. doi: 10.1155/2021/8857222
Shahi, S., and Srivastava, M. (2018). Influence of foliar application of manganese on growth, pigment content, and nitrate reductase activity of Vigna radiata (L.) R. Wilczek under salinity. J. Plant Nutr. 41, 1397–1404. doi: 10.1080/01904167.2018.1454470
Shankar, M. A., Gajanana, G. N., Kumar, H. K. M., Ravi, R. J. M., and Roopadevi, V. D. (2004). Effect of zinc and boron on growth, yield and quality of finger millet-groundnut based cropping system for alfisoils of Karnataka. IFA International Symposium on Micronutrients, New Delhi, India, pp 23–25.
Shojaei, H., and Makarian, H. (2015). The effect of nano and non-nano zinc oxide particles foliar application on yield and yield components of mungbean (Vigna radiate L.) under drought stress. Iran. J. Field Crop Res. 12, 727–737.
Singh, P., Shukla, A. K., Behera, S. K., and Tiwari, P. K. (2019). Zinc application enhances superoxide dismutase and carbonic anhydrase activities in zinc-efficient and inefficient wheat genotypes. J. Soil Sci. Plant Nutr. 19, 477–487. doi: 10.1007/s42729-019-00038-7
Ullah, A., Farooq, M., Rehman, A., Arshad, M. S., Shoukat, H., Nadeem, A., et al. (2017). Manganese nutrition improves the productivity and grain biofortification of bread wheat in alkaline calcareous soil. Exp. Agric. 54, 744–754.
Verma, P. D., Swaroop, N., Upadhyay, Y., Swamy, A., and Dhruw, S. S. (2018). Role of phosphorus, zinc and rhizobium on growth and yield of field pea (Pisum sativum L) var Rachna. J. Pharmacogn. Phytochemistry 7, 1479–1492.
Welch, R. M., Graham, R. D., and Cakmak, I. (2013). Linking agricultural production practices to improving human nutrition and health.ICN2 second Rome, Italy: International conference on nutrition preparatory technical meeting, pp. 13–15.
Keywords: biofortification, wheat genotypes, Zn and Mn nutrition, grain quality, micronutrients efficient genotypes
Citation: Dhaliwal SS, Sharma V, Shukla AK, Behera SK, Verma V, Kaur M, Singh P, Alamri S, Skalicky M and Hossain A (2023) Biofortification of wheat (Triticum aestivum L.) genotypes with zinc and manganese lead to improve the grain yield and quality in sandy loam soil. Front. Sustain. Food Syst. 7:1164011. doi: 10.3389/fsufs.2023.1164011
Edited by:
Maryke T. Labuschagne, University of the Free State, South AfricaReviewed by:
V. S. Saravanan, Pondicherry University, IndiaAbdullah Al Mahmud, King Abdullah University of Science and Technology, Saudi Arabia
Copyright © 2023 Dhaliwal, Sharma, Shukla, Behera, Verma, Kaur, Singh, Alamri, Skalicky and Hossain. This is an open-access article distributed under the terms of the Creative Commons Attribution License (CC BY). The use, distribution or reproduction in other forums is permitted, provided the original author(s) and the copyright owner(s) are credited and that the original publication in this journal is cited, in accordance with accepted academic practice. No use, distribution or reproduction is permitted which does not comply with these terms.
*Correspondence: Arvind Kumar Shukla, YXJ2aW5kc2h1a2xhMmszQHlhaG9vLmNvLmlu; Akbar Hossain, YWtiYXJob3NzYWlud3JjQGdtYWlsLmNvbQ==