- 1Department of Botany, University of Agriculture, Faisalabad, Pakistan
- 2Institute of Soil and Environmental Sciences, University of Agriculture, Faisalabad, Pakistan
Iron (Fe) is a vital element that is equally important for plants, animals, and humans. High Fe concentrations in wheat grains have reliance on plant roots, the hidden half of the plant with a role in nutrient mining. Enhanced grain Fe content of wheat can positively mitigate Fe malnutrition in poor populations. In the present study, 100 wheat varieties were studied to identify the root architectural characteristics in relation to grain Fe concentration. Germinated seeds were transplanted in a rhizobox kept in a standard nutrient solution and were harvested 12 days after transplanting. Roots were scanned and the images were processed using smart root software. A total of 12 wheat varieties, which had a vigorous and weak root system architecture (RSA), in combination with higher and lower Fe grain concentrations, were selected using principal component analysis. The uptake and translocation of Fe from root to shoot were determined through a pot experiment conducted for the above-mentioned 12 wheat varieties, with or without Fe fertilizer applied as FeSO4 to the soil. The data obtained from the pot experiment revealed that Dharabi-11 with vigorous RSA exhibited the highest grain Fe concentration (57.20 mg kg−1), low phytate concentration (6.50 mg kg−1), and maximum 1,000 grain weight, whereas Ujala-16 with weaker RSA had the lowest grain Fe concentration (13.33 mg kg−1), highest phytate concentration (9.07 mg kg−1), and lowest 1,000 grain weight. There were also varieties showing higher grain Fe concentrations with weaker RSA and vice versa. Although it is indicated that vigorous RSA leads to high grain Fe concentration, it is not the sole factor in high grain Fe concentration. Nevertheless, the results demonstrate that large genetic diversity is available among indigenous wheat germplasm in terms of grain Fe concentration and RSA. This information may be utilized in the development of new varieties through conventional and marker-assisted breeding programs using RSA traits for Fe biofortification in wheat, leading to the mitigation of Fe malnutrition.
1. Introduction
An adequate supply of micronutrients and macronutrients is as important for plants as it is for humans and animals. The deficiency of any micronutrient in the soil can reduce plant growth and yield. However, a number of factors contribute to the sufficient supply of nutrients, like availability, rate of absorption, and transport within the plant (Riaz et al., 2017). Fertilizers are applied to crops to provide these nutrients, but nutrient uptake ability in crops varies depending upon various types of root systems (Timilsena et al., 2015), nutrient mobilizing exudates, soil chemistry and nutrient movement processes (Marschner and Rengel, 2023). Iron is an indispensable nutrient contributing to the development and growth of plants. It plays a critical role in many metabolic phenomena, such as chlorophyll synthesis, which is a major contributor in the redox reactions of photosynthesis (Jeong et al., 2017). Plants follow two strategies for the acquisition of Fe. Strategy I (reduction based) is adopted by non-graminaceous plants, where protons are secreted by roots H+-ATPases that lower pH in the rhizosphere and reduction of Fe3+ takes place. Strategy II is adopted by graminaceous plants where plant roots release phytosiderophores that chelate with Fe3+, which passes through the root cell membrane via transporters (Rehman et al., 2021). Plant roots secrete several types of mugineic acids under Fe stress conditions. Since the distribution of Fe is heterogeneous in soil, roots adapt themselves to cope with this heterogeneity through morphological and physiological adaptive responses (Li et al., 2016). Wheat varieties reduce or increase root attributes to adjust plant Fe levels under Fe-sufficient and -deficient conditions, respectively. However, a variable extent of response to higher root-to-shoot ratio and root length has been witnessed among different varieties (Divte et al., 2019). The optimal root system architecture (RSA) is needed for efficient nutrient acquisition from the rhizosphere (Alahmad et al., 2019). Wheat has a fibrous root system, comprising nodal, primary, and lateral roots (Ober et al., 2021). Studies show that RSA has changed over time in historical wheat varieties. A positive change (1965–1916) was identified in unique RSAs associated with yield, phenology, and variation in the expression of functional genes among wheat cultivars belonging to different release years. Evaluations indicated that wheat cultivars released after 1965 (green revolution period) showed significant increases in root depth and length. However, cultivars released after 2000 showed a significant increase in root depth, diameter, number of roots, and surface area (Maqbool et al., 2022).
Genotype and environmental interaction have a significant effect on quality-related traits of wheat (Amiri et al., 2018). Wheat screening has revealed that primitive wheat varieties have the highest grain Fe content. In recent years, dwarf high-productive wheat varieties have been introduced (Mamrutha et al., 2014), which have shown low grain Fe content. It is also evident that most of the staple crops have genetic diversity for micronutrient density in their grains. Enhanced concentrations of micronutrients not only fulfill micronutrient deficiency problems but can also lead to better productivity, even in micronutrient-deficient soils (Welch and Graham, 2004).
Despite the fact that agriculture has always, traditionally, been a source of food and nutrition, hunger and hidden hunger are becoming a worldwide challenge. Researchers are trying to relieve micronutrient deficiencies by adopting food diversification, supplementation, fortification, and biofortification. However, biofortification is the more appropriate solution to reduce mortality and improve food security, yield, and quality of life (Wakeel et al., 2018). The agronomic biofortification of cereal crops with essential elements, i.e., Fe and Zinc (Zn), has been proven as a sustainable approach to improving the nutritional status of people in developing countries (Kiran et al., 2021). Desired wheat varieties can be selected by establishing genetic variation by screening wheat varieties on the basis of levels of Fe, Zn, phenols, and vitamins growing under various environmental conditions (Amiri et al., 2015). The Harvest Plus Challenge Program has approved the target of increasing the concentration of Fe to approximately 25 mg kg−1 in order to acquire quantifiable outcomes, for which the Fe level will have to be increased from 35 to 60 mg kg−1 (Welch and Graham, 2004).
Iron plays an important role in human metabolism, and its deficiency causes anemia and increases the risk of psychosis, anxiety, depression, and sleep disorders. Globally, 29.4% of women are reproductive, and 40% of pregnant women and 30% of non-pregnant women have anemia (Wu et al., 2020). According to the World Health Organization estimates, more than 27% of the world’s population may suffer from Fe deficiency, leading to anemia (Owaidah et al., 2020). It is also evident that the main staple foods of the world’s 2 billion Fe-deficient people are wheat-based and wheat products (Cakmak et al., 2010).
This study was designed to identify the relationship between RSA and grain Fe concentration to select wheat cultivars for productivity and Fe concentration improvement to be used in further breeding programs. The study of RSA is difficult, time-consuming, and expensive, which is why it is not usually included in breeding programs (Rufo et al., 2020). Nevertheless, rhizobox/rhizotron-based experiments have made it feasible to study RSA. Rhizoboxes are useful tools for understanding the hidden half of plants (Maskova and Klimes, 2020). It has been hypothesized that high grain Fe concentration depends on the vigorous RSA of wheat.
2. Materials and methods
This study was designed to find the correlation between grain iron content and root system architecture in wheat. Seeds of 100 wheat varieties were collected from the National Agriculture Research Center (NARC), Islamabad, Pakistan to perform two experiments in this study (Supplementary Table S1 includes pedigree information and year of release). In the first experiment, the association of RSA with a grain Fe content-based experimental system (under Fe-deficient conditions in a rhizobox) was conducted in a growth room situated in the Department of Botany, at the University of Agriculture Faisalabad Pakistan. The grain Fe content of all wheat varieties was determined using an atomic absorption spectrophotometer (Estefan et al., 2013). A rhizobox-based experiment was conducted to study RSA traits. A rhizobox consists of two plexiglass plates spaced 1.0 cm apart, with pores on the bottom side (Photo 1). The sand was washed, dried, and used to fill in the boxes. The rhizoboxes were adjusted in a tank, and nutrient solution without an Fe source was supplied in the tank, assuring the depth of solution to be 3 cm only (this depth is sufficient to raise the nutrient solution to the top of the rhizobox).
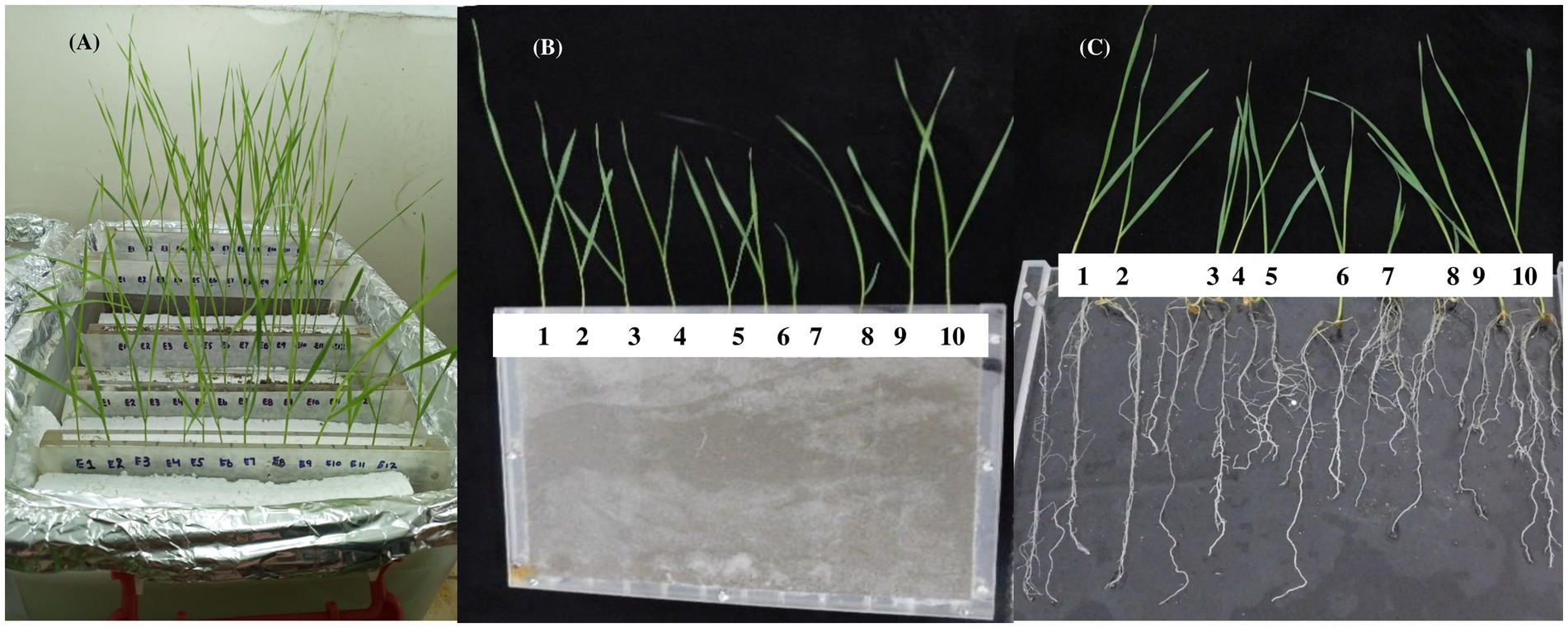
PHOTO 1. (A) Rhizobox setup (B) sand-filled rhizobox (closed) 10 wheat seedlings (C) sand-filled rhizobox (opened) with 10 wheat seedlings.
Wheat seeds were germinated in petri plates separately for 24 h and then germinated seeds were sown in sand-filled rhizoboxes, which were irrigated with the nutrient solution, as in Photo 1. Each rhizobox contained 10 wheat varieties. Each variety had four replicates (in separate rhizoboxes). Seedlings were harvested after 12 days and images of the roots were taken immediately after harvesting using a camera (Nikon Coa-PTX L840) through an optimized image-taking system. The optimized image system consisted of a case with the dimensions 30 cm × 30 cm fitted with a camera on the top at the height of 60 cm. The plants to be imaged were placed on a scaled hard sheet, which was adjusted at the bottom. Images of roots were subjected to the software Smart Root (Lobet et al., 2011), and data for RSA attributes, i.e., primary root length (PRL), total lateral root length (TLRL), total root length (TRL), lateral root number (LRN), and lateral root density (LRD), were recorded. Data were arranged in ascending order for grain Fe contents and formed an index to check the frequency of varieties for root traits.
The second experiment was conducted, in polyvinyl chloride (PVC) pots, to evaluate the Fe uptake and translocation to shoot/grain in selected wheat varieties (with different combinations of RSA and grain Fe content). These combinations were developed from analyzing the data of the first experiment, through principal component analysis, and three varieties from each of the four combinations were selected for the previously mentioned pot study, i.e., high grain Fe content and vigorous RSA, high grain Fe content and weak RSA, low grain Fe content and vigorous RSA, low grain Fe content and weak RSA (Supplementary Table S2).
Pots were filled with an 8 kg sieved mixture of soil and sand at a 60:40 ratio. Nitrogen, phosphorus, and potassium fertilizers were applied at a rate equivalent to 150:100:60 kg ha−1 in the form of urea, single super phosphate, and potassium sulfate. Potassium and phosphorous were applied as a basal dose, while nitrogen was applied in three equal splits. The first split was applied as a basal dose, while the second and third were applied at the tillering and heading stages, respectively. Iron was applied as soil application at a rate equivalent to 20 kg ha−1 at the time of first irrigation using FeSO4.7H2O in one treatment, while the other treatment was not supplied with Fe fertilizer.
Standard crop management practices were followed during the whole experiment. At harvest, three plants were selected from each pot, then the number of spikelets on each spike and the number of grains were counted. The average was calculated considering each replicate. The grains of the harvested plants were weighed for the determination of yield.
2.1. Estimation of grain iron
The grain Fe content of all the wheat varieties was determined using an atomic absorption spectrophotometer following the atomic absorption method (Estefan et al., 2013). All grain samples were oven-dried at 60–70°C for 2 h. Sample powder (0.25 g) was digested in 2.5 mL of (2:1) nitric acid and perchloric acid mixture in the digestion flask. Samples were allowed to stand overnight. These samples were heated on a hot plate (180°C) until white fumes appeared and the digest was transparent. They were then cooled down, and deionized water was added to make the volume up to 50 mL. The samples were filtered and preserved for further analysis. The same process was carried out for the preparation of the blank solution. Iron analysis of the samples was conducted by Atomic Absorption Spectrophotometer (Hitachi Polarized Zeeman Z-5200 A A Spectrophotometer, Hitachi, Japan).
The localization of Fe in wheat grain was determined using the Perls Prussian blue staining method (Shobhana et al., 2013), for which 2% Prussian blue solution was utilized for the determination of the localization of grain Fe content. Grain samples were dipped in a stain solution for 2 h. Seed grains became imbibed and stained. Then, cross-sections of grains were taken, slides were observed under a microscope (Stereo Blue Euromex model), and images were taken.
Statistical analysis was carried out using the R studio R × 64 3.6.1 software. The selection of wheat varieties from the rhizobox experiment was done by applying principal component analysis (PCA). Analysis of variance was applied to the pot experiment conducted in a completely randomized design (CRD). Comparison of treatment was carried out using the mean of least square difference (LSD) at a probability level of 0.05.
3. Results
3.1. Study I association of RSA with grain Fe content under Fe-deficient conditions in rhizobox-based experimental system
3.1.1. Grain Fe concentration
A grain Fe concentration histogram exhibited a right-skewed distribution. The distribution peak is toward the right-side limits from the center, and the tail stretches away from the center. A high number of varieties (~80 varieties) were found to have grain Fe content within the range of 0–10 mg kg−1. The highest grain Fe concentrations were 39.84 mg kg−1, 37.18 mg kg−1, and 35.52 mg kg−1, which were detected in Dharabi-11, Punjab-11, and Faisalabad-2008, respectively, and the lowest grain Fe concentrations were determined in Ujala-16, Lasani-08, and Miraj-08, which were 0.16 mg kg−1, 0.13 mg kg−1, and 0.08 mg kg−1, respectively (Figure 1A).
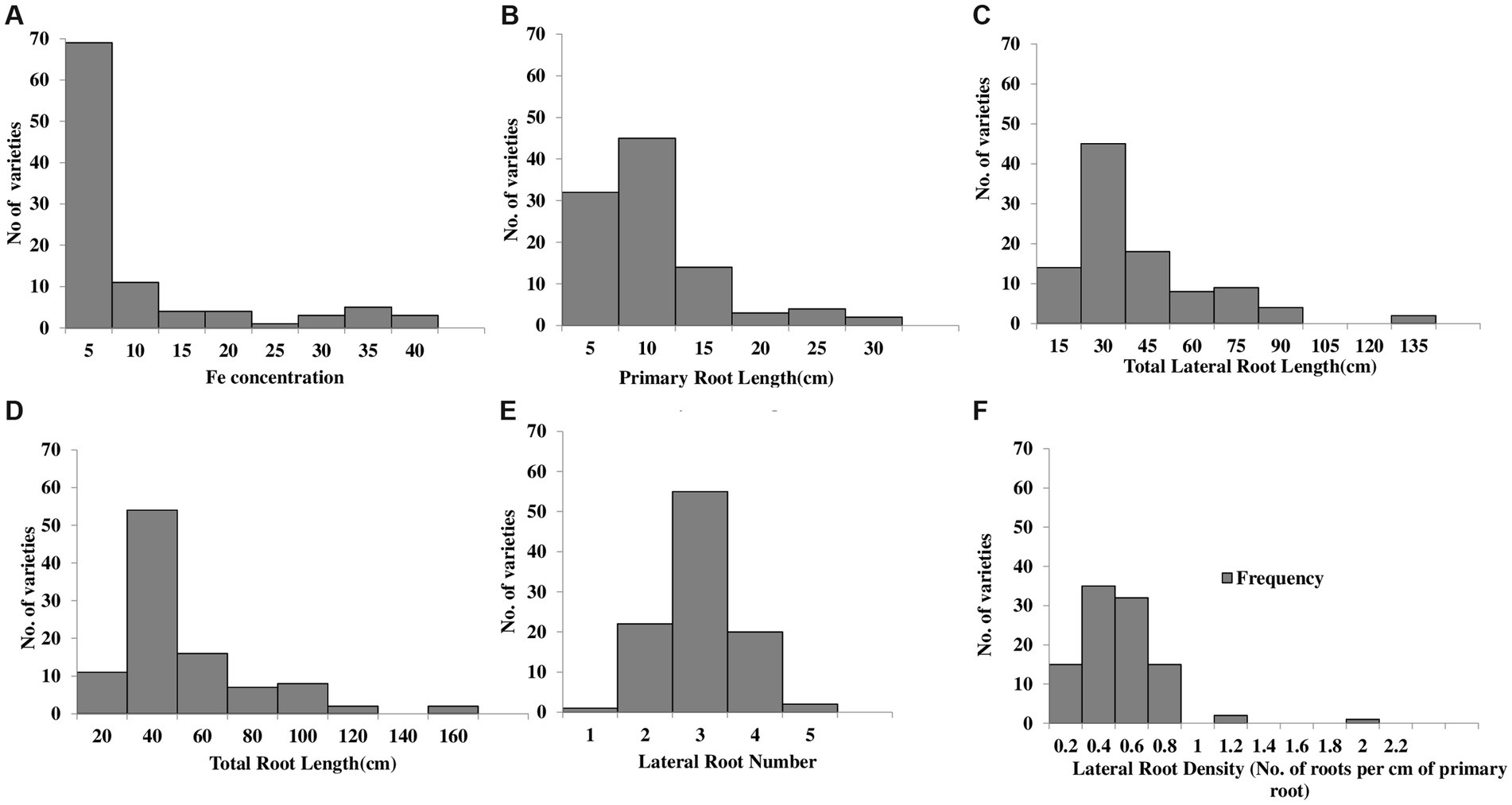
Figure 1. Histogram representation of the frequency distribution of 100 wheat varieties vs root system architecture: (A) grain Fe content (B) primary root length (C) total lateral root length (D) total root length (E) lateral root number (F) lateral root density.
Primary root length, total lateral root length, total root length, and lateral root density of wheat varieties shown skewed distribution toward the right side of the histogram. Most of the varieties lie within the range of 5–10 cm for PRL. Among the varieties, the longest PRL was observed in Dharabi-11 (27.05 cm), while the shortest PRL was found in Lasani-08 (1.95 cm; Figure 1B). Total lateral root length ranging from 15 cm to 30 cm was observed in a maximum number of varieties. The longest TLRL was detected in Dharabi-11 (132.06 cm), whereas the shortest TLRL was found in Lasani-08 (4.03 cm; Figure 1C). The TRL of most of the varieties was between 20 cm and 40 cm, whereas the longest and the shortest TRL was observed in Dharabi-11 (159.12 cm) and Lasani-08 (5.98 cm), respectively (Figure 1D).
The LRN is shown to have a normal distribution trend in the histogram, and most of the varieties were observed to produce three lateral roots, although the maximum of four lateral roots was observed in Punjab-11, and the minimum LRN was in Lasani-08 (Figure 1E). The lateral root density was found to range from 0.1 to 2 roots per cm (Figure 1F).
Comparison of RSA attributes with grain Fe content by correlation matrix predicted that grain Fe content has a positive correlation with PRL (R2 = 0.26), TLRL (R2 = 0.16), and TRL (R2 = 0.19) and a negative correlation with LRN (R2 = −0.01) and LRD (R2 = −0.000). Similarly, RSAIN is also shown to have a positive correlation with grain Fe content (Figures 2A,B).
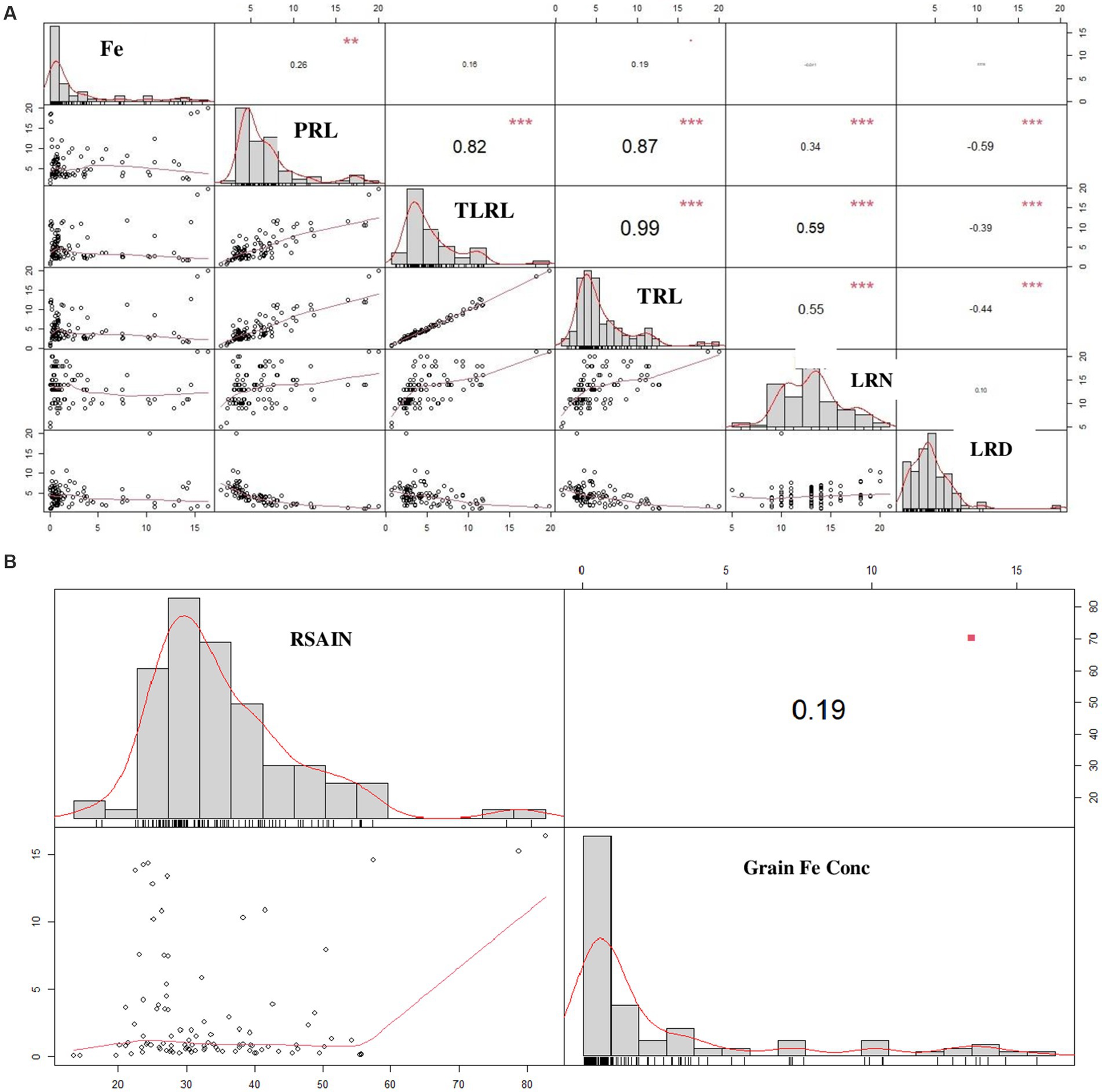
Figure 2. Pearson correlation matrix for the comparison of root system architecture with grain Fe content in 100 wheat varieties (A) correlation between individual root attributes vs grain Fe content (B) correlation between total root attributes vs grain Fe content. Diagonal represents attributes trend, upper portion of diagonal denotes coefficient of R2, lower portion of diagonal indicates scatter plot with trend line. PRL, primary root length; LRL, lateral root length; TRL, total root length; LRN, lateral root number; LRD, lateral root density; Fe, iron concentration; RSAIN, root system architecture index.
3.1.2. Screening of wheat varieties with reference to root system architecture and grain Fe content
Principal component analysis explored four types of trends in wheat varieties on the basis of the correlation of root system architecture with grain Fe content, i.e., 6% of varieties were found to have vigorous RSA and higher grain Fe content; amongst these varieties, Dharabi-11 (V77), Punjab-11 (V73), and Faisalabad-08 (V126) were found to be the most efficient. However, 7% of the varieties showed higher grain Fe content and weak RSA, amongst which, BARS-09 (V58), NARC-09 (V55), and Jauhar-78 (V69) were more efficient. Similarly, 9% of varieties represented lower grain Fe content and vigorous RSA with PirSabak-2013 (V82), Pakistan-2013 (V86), and NARC-2011 (V78) on top. Approximately 78% of varieties were found to have low grain Fe content and weak RSA. At the same time, Ujala-16 (V117), Miraj-08 (V50), and Lasani-08 (V49) exhibited the weakest attributes (Figure 3).
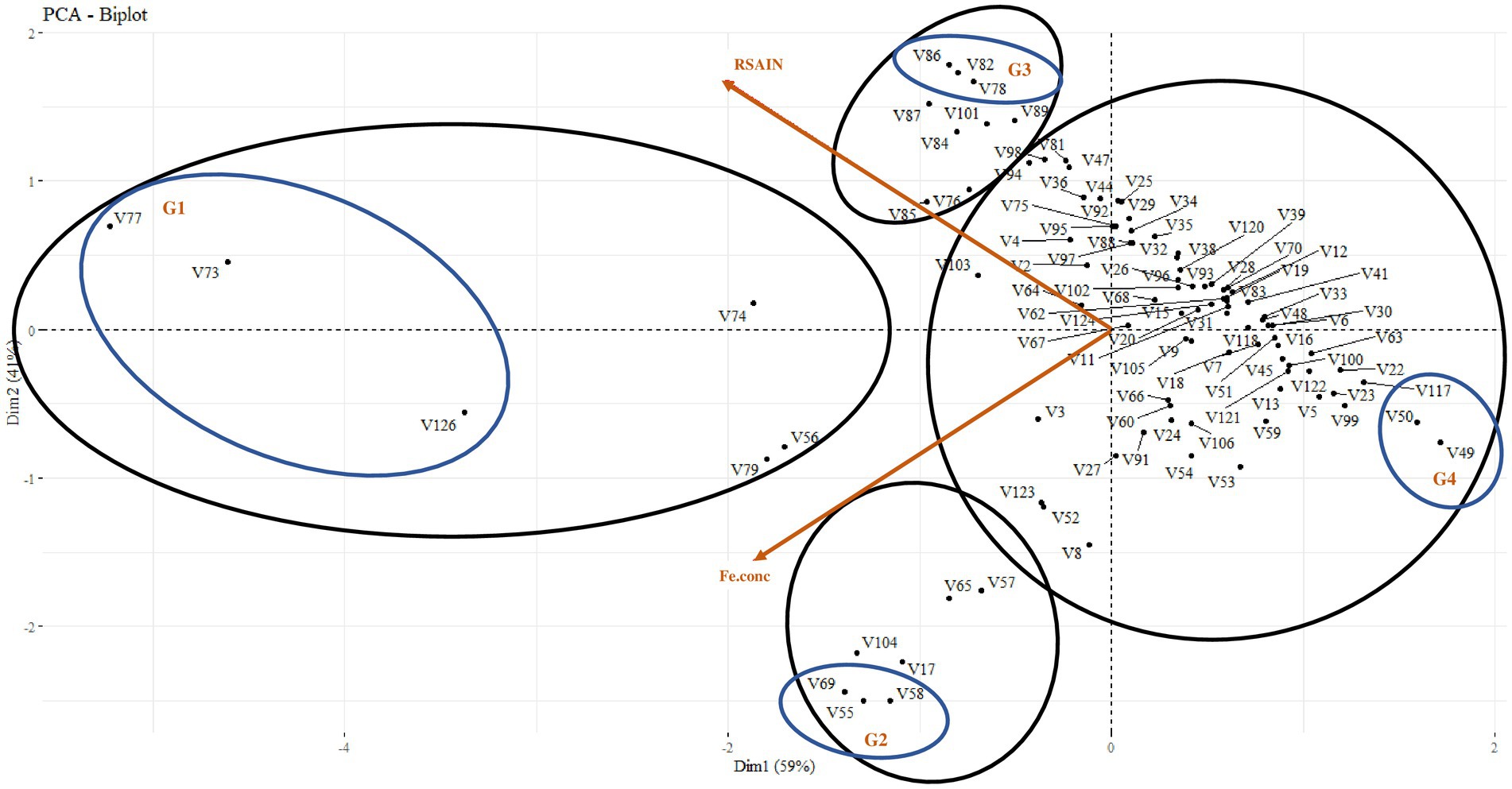
Figure 3. Principal component analysis between root system architecture and grain Fe content in 100 wheat varieties for the selection of 12 varieties. G1: efficient root system architecture vs higher grain Fe content, G2: weaker root system architecture vs higher grain Fe content, G3: efficient root system architecture vs lower grain Fe content, G4: weaker root system architecture vs lower grain Fe content.
3.2. Study-II: evaluation for Fe uptake and translocation to shoot/grain and yield parameters in wheat varieties with varying RSA
3.2.1. Yield under Fe-sufficient and -deficient conditions
Data related to yield parameters were recorded at the time of anthesis. Statistical analysis of the data revealed that the number of spikelets was increased under Fe-sufficient conditions. The number of spikelets ranged from 11 to 21 among various varieties. The maximum number of spikelets (i.e., 21) was found in Dharabi-11 in Fe-sufficient conditions, whereas the minimum number of spikelets (i.e., 11) was found in Ujala-16 under Fe-deficient conditions. The mean value of the number of grains ranged between 36 in (Ujala-16) and 61 in Dharabi-11 (Table 1). Grain yield and 1,000 grain weight (showing the vigor of grains) were higher in Fe-sufficient conditions. The maximum 1,000 grain weight was found for Dharabi-11 (i.e., 47.00 g) and the minimum 1,000 grain weight was recorded in Ujala-16 (29.90 g; Figures 4A,B). Data regarding leaf area, plant height, and total biomass are presented in Supplementary Table S3.
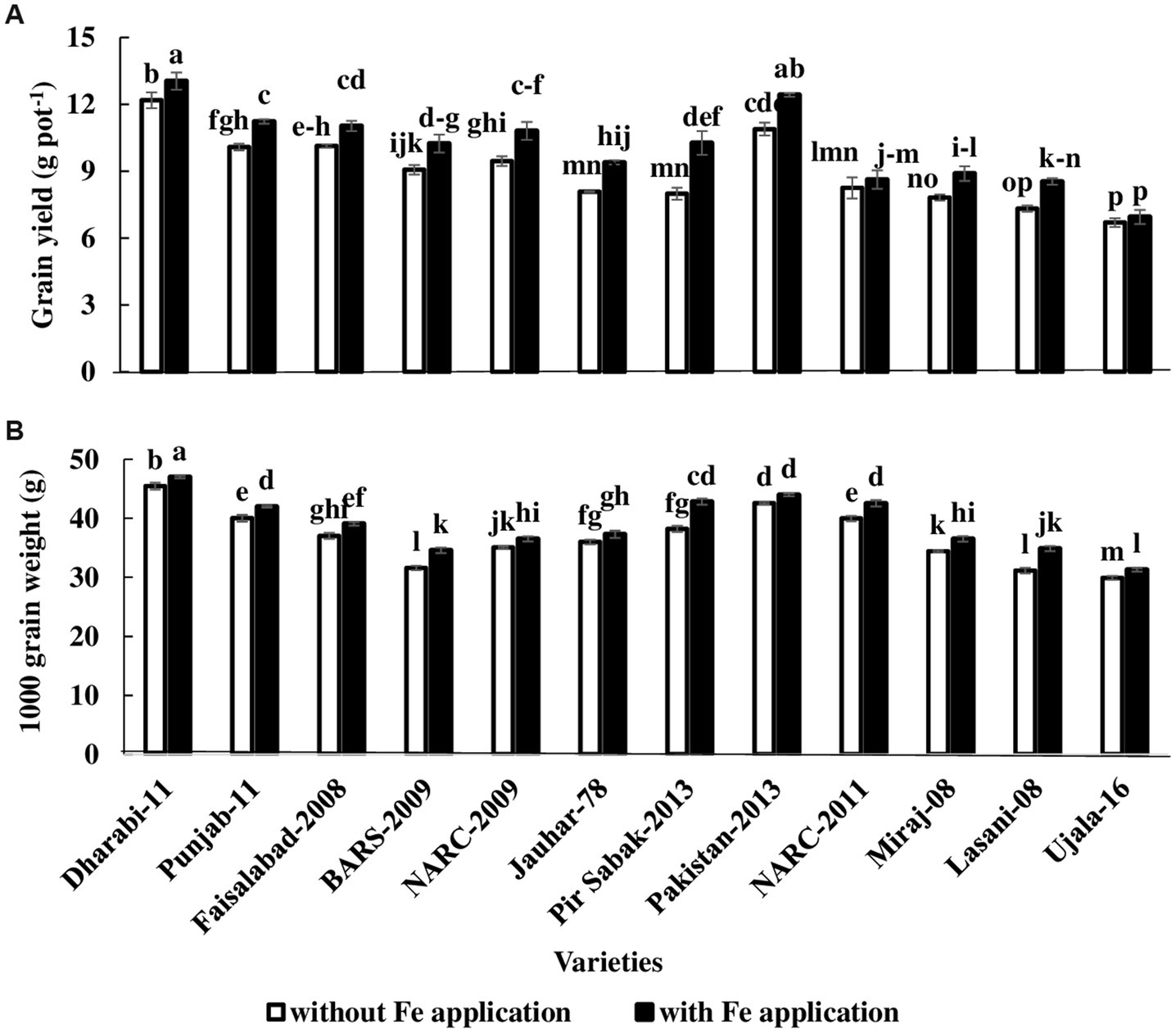
Figure 4. Effect of Fe application on yield attributes of various varieties of wheat in pot conditions. Treatment: Without Fe application and with Fe application at a rate of 20 kg ha−1. (A) 1,000 grain weight (B) Grain yield. The value in the column shows the mean of four replications, the filled column denotes with Fe application, and the unfilled column denotes without Fe application. The bars show the standard error, and column sharing letters are not significantly different at p < 0.05.
3.2.2. Evaluation for Fe concentration, uptake, and translocation
Roots proliferate in the rhizosphere for the acquisition and uptake of Fe. In order to explore the translocation, Fe concentration was analyzed in roots, shoots, husks, and grains. The highest grain Fe concentration, 57.20 mg kg−1, was found in Dharabi-11, and the lowest Fe concentration 13.33 mg kg−1 was observed in Ujala-16 (Figure 5A). Phytate content has significant importance as it reduces the phytoavailability of Fe. The highest concentration of phytate was 9.07 mg kg−1 and was found in Ujala-16, and the lowest concentration, 5.75 mg kg −1, was observed in Faisalabad-08 (Figure 5B).
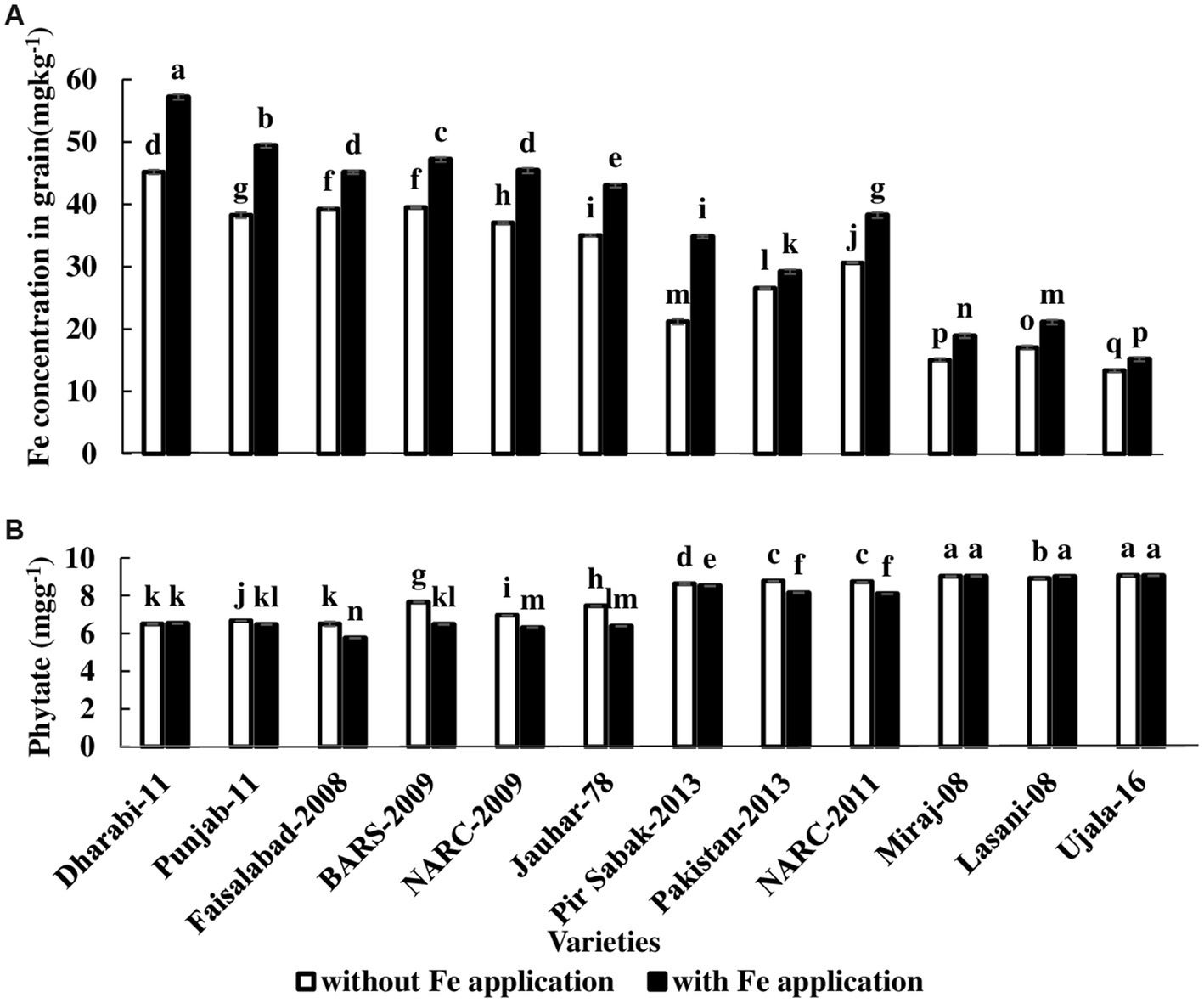
Figure 5. Effect of Fe application on grain Fe concentration: (A) Fe concentration in grain (B) Phytate concentration in grain. The value in the column shows the mean of three replications, the filled column denotes without Fe application, and the unfilled column denotes with Fe application. The bars show the standard error, and column not sharing letters are significantly different at p < 0.05. LSD value. Grain Fe: 0.65, phytate: 0.02.
Comparative analysis of the uptake of Fe and its translocation to shoots, husks, and grains disclosed that each variety acquired different concentrations of Fe from soil containing the same amount of Fe. The uptake of total Fe by the roots influenced the concentration of Fe to be translocated to the shoots, husks, and grains. Moreover, the application of Fe enhanced its availability in the rhizosphere and improved the uptake of Fe. Varieties with efficient uptake of Fe by the roots generally translocate higher amounts of Fe to the shoots, husks, and grains. The amount of Fe to be translocated toward the furthest sink grains from the roots correlates with the amount of Fe existing in the shoots, husks, and grains. Varieties with higher Fe content in the roots, shoots, and husks translocated higher amounts to grains (Table 2).
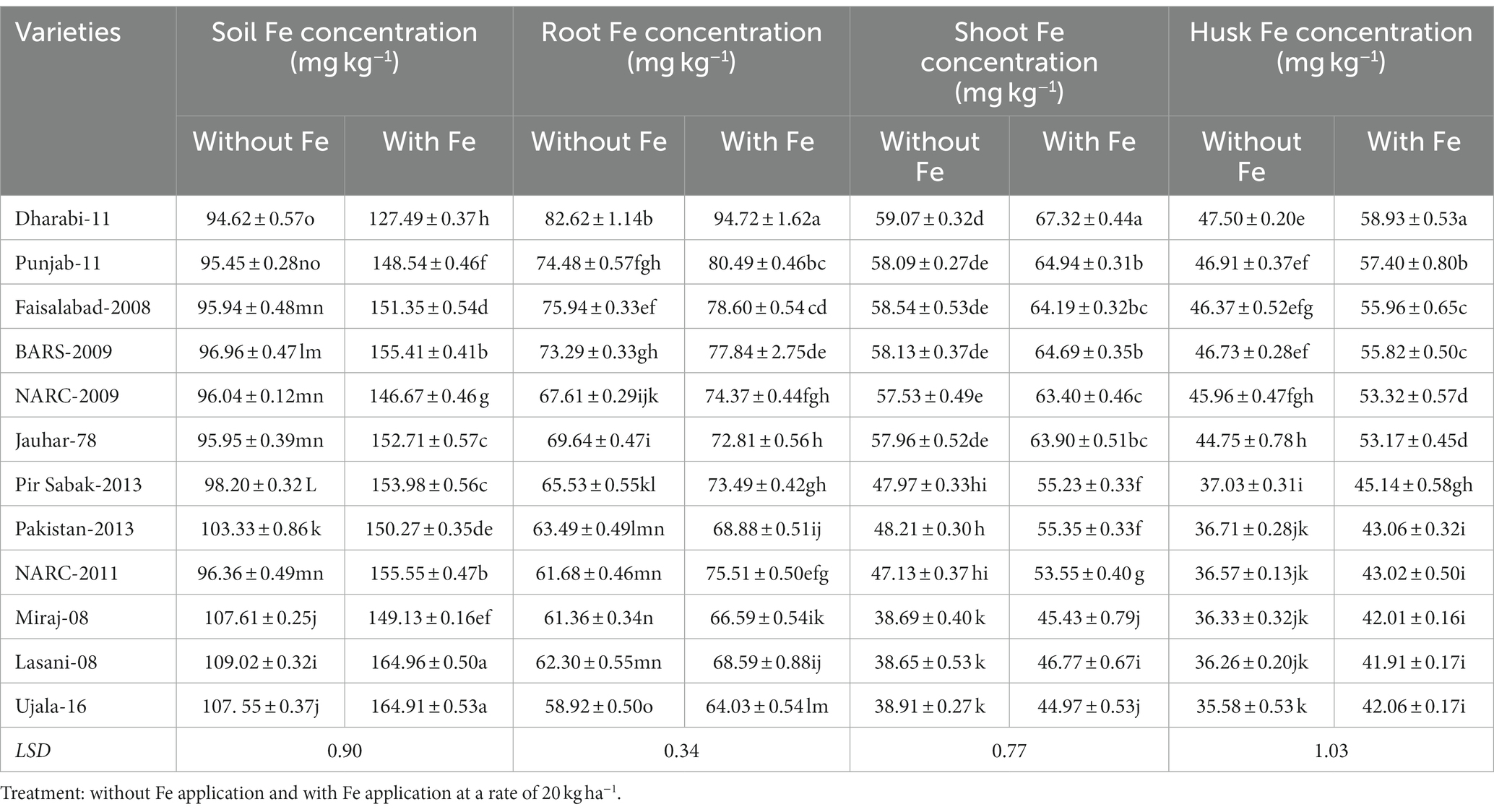
Table 2. Effect of Fe application on uptake and translocation of Fe in various varieties of wheat in greenhouse conditions.
Although only a small amount of Fe was translocated to the grains, varieties with higher uptake potential by the roots translocated higher concentrations of Fe to grains. The order of uptake of Fe by the roots was Dharabi-11 > Faisalabad-2008 > Punjab-11 > BARS-2009 > NARC-2009 > Jauhar-78 > NARC-2011 > Pakistan-2013 > Pir Sabak-2013 > Lasani-08 > Miraj-08 > Ujala-16. Similarly, the varieties can be arranged in increasing order of grain Fe content as follows: Dharabi-11 > Punjab-11 > BARS-2009 > NARC-2009 > Faisalabad-2008 = Jauhar-78 > NARC-2011 > Pir Sabak-2013 > Pakistan-2013 > Lasani-08 > Miraj-08 > Ujala-16 (Figure 6).
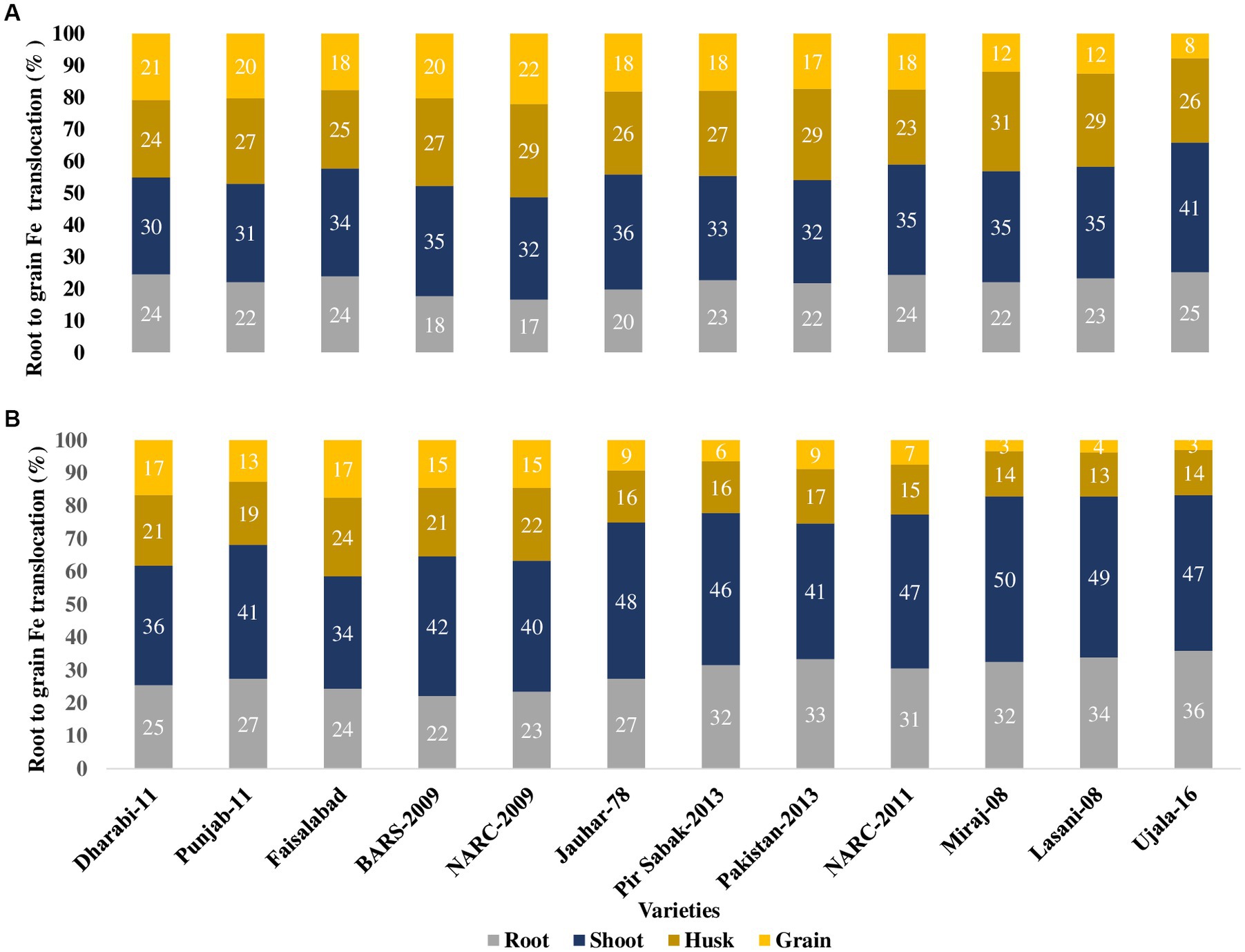
Figure 6. Uptake and translocation of Fe by various wheat varieties grown in conditions with soil application of Fe in the pot experiment. Fe application at a rate of 20 kg ha−1. Fe uptake and translocation are displayed on the clustered stacked column chart, giving a visual comparison of Fe content from root to grains, where the numeric value in individual stacks represents the Fe percentage in the respective plant part. Numeric values on the Y axis represent total Fe uptake by the plant. (A) Uptake and translocation of Fe by various wheat varieties grown in conditions with soil application of Fe in the pot experiment (B) uptake and translocation of Fe by various wheat varieties grown in conditions without soil application of Fe in the pot experiment.
3.2.3. Fe localization in wheat grains
Visualization of Fe localization by staining showed that the parts of the grains with a higher concentration of Fe appeared darker in color compared to the parts of the grains with a lower concentration of Fe. The aleuronic layer and embryo part of the seed stained darker than the endosperm (Photo 2).
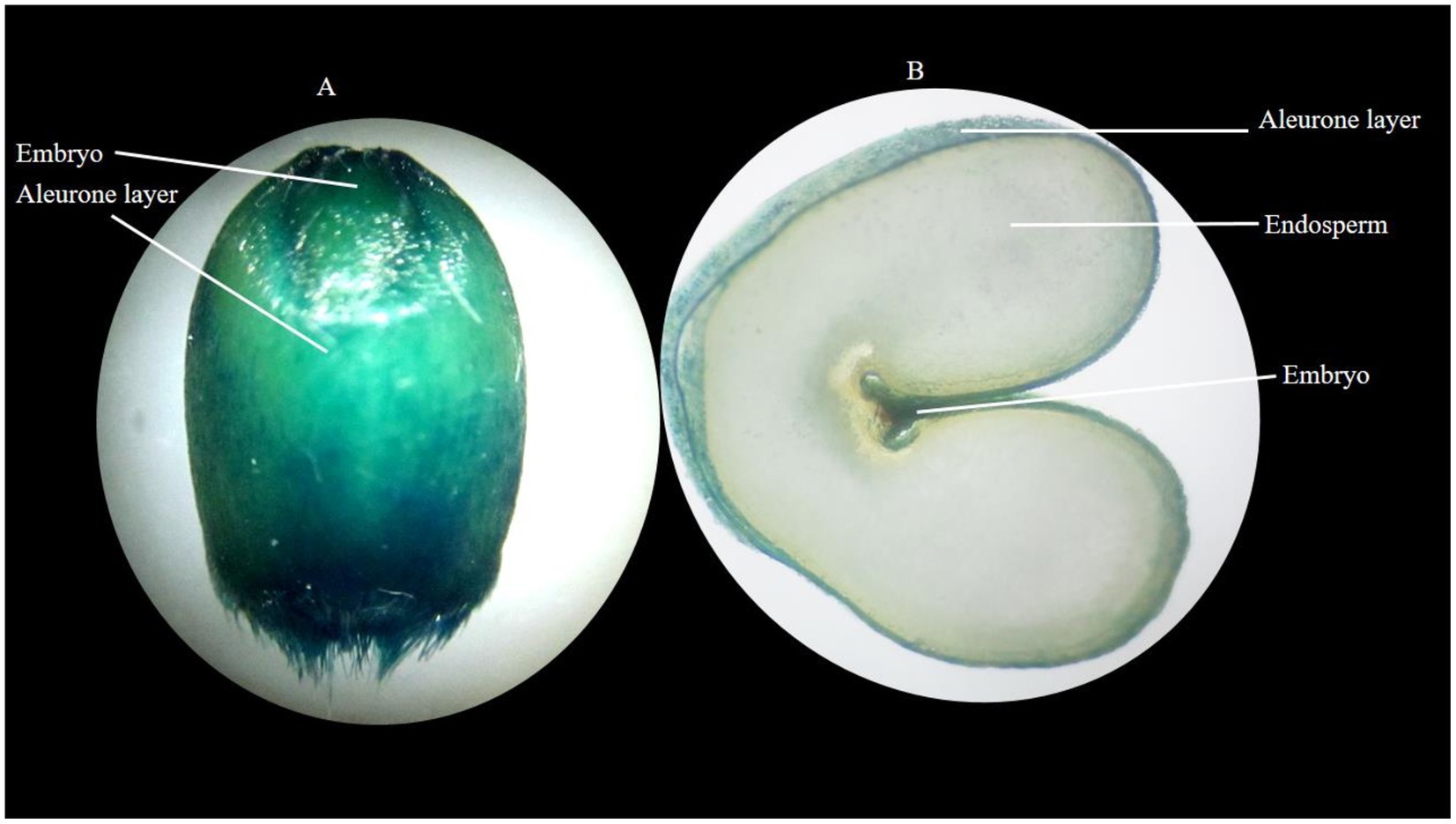
PHOTO 2. Localization of Fe in wheat grain (A) whole stained grain (B) cross-section of stained grain, where the stained aleurone layer represents the existence of Fe in aleurone.
3.2.4. Physiological attributes
Evaluation of physiological parameters is of significant importance as physiological attributes influence the photosynthetic yield. The physiological activity was improved when wheat varieties were grown in Fe-sufficient conditions. The maximum improvement in physiological attributes was observed in Dharabi-11, Faisalabad-2008, Punjab-11, and Pakistan 2013, respectively. As in these varieties, photosynthetically active radiation, fluorescent transient, electron transport rate, and effective quantum yield of photosystem II exhibited a positive correlation. However, the minimum improvement was observed in Ujala-16, Lasani-08, and Miraj-08. NARC-2009, Pir Sabak-2013, Jauhar-78, BARS-2009, and NARC-2011 showed moderate behavior, where a negative correlation was observed in physiological attributes (Figure 7).
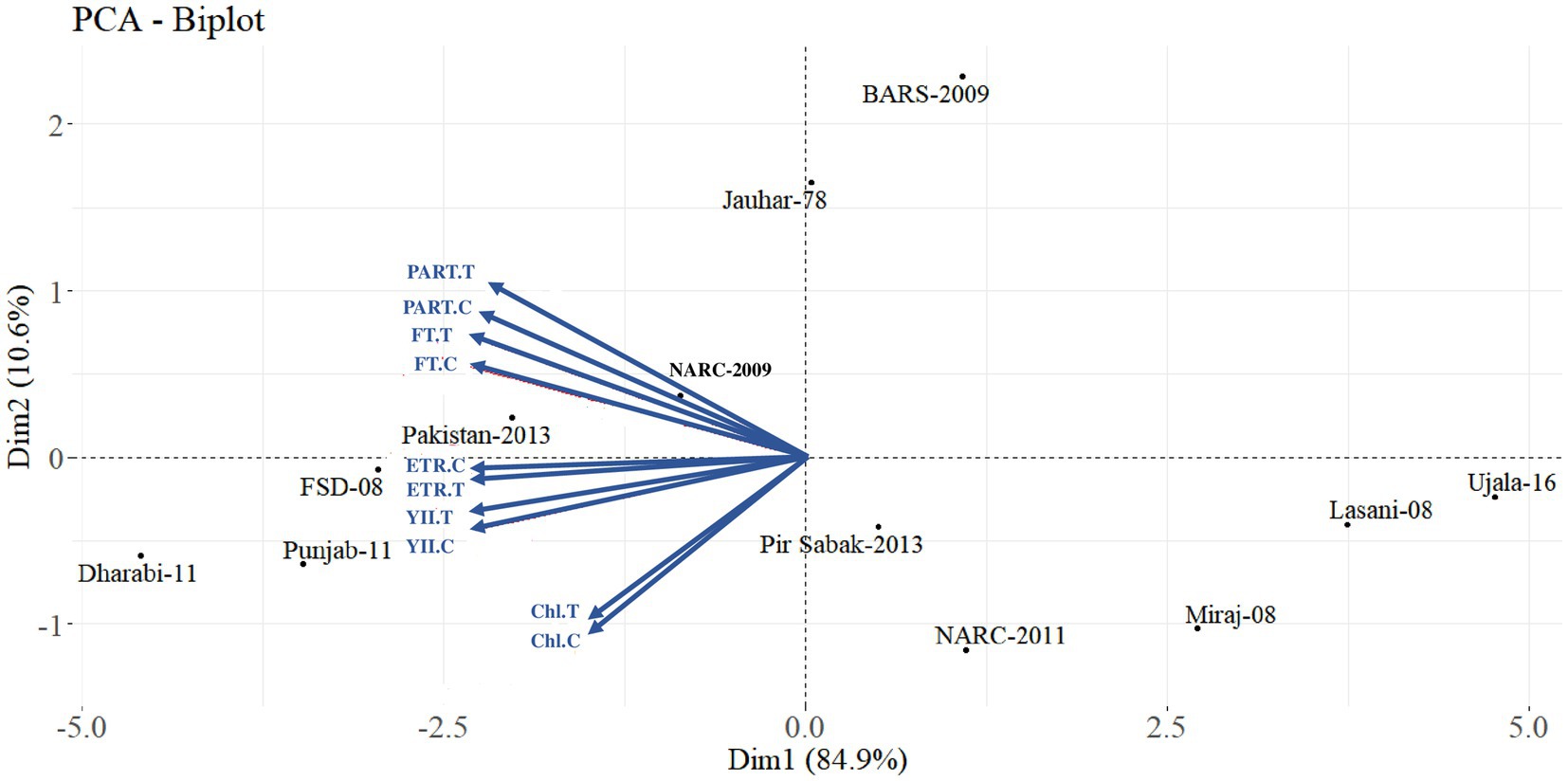
Figure 7. Effect of Fe application on physiological parameters of various varieties of wheat in pot conditions. PAR, photosynthetically active radiation [PAR (μmol m−1 s−1)]; YII, effective quantum yield photosystem II; ETR, electron transport rate [ETR (umole−2 s−1)]; FT, fluorescent transient; Treatment, without Fe application (C) and with Fe application (T) at a rate of 20 kg ha−1.
4. Discussion
Fe deficiency is one of the most prevalent problems leading not only to a risk of early death but also to long-term negative consequences related to health and growth. One of the major factors contributing to Fe malnutrition is the low bioavailable Fe content in staple foods such as wheat, which is inherently low in Fe (Wakeel et al., 2018; Hassan et al., 2021). The current study was conducted to identify the root characteristics responsible for high Fe content in wheat grain to mitigate Fe malnutrition in developing countries where wheat is used as a staple food.
Often, breeding is carried out considering the grain yield trait, which is a late-stage and multifaceted trait influenced by a number of factors, including root system architecture (RSA). Roots’s vigor at an early growth stage can be useful for screening efficient genotypes for future breeding programs (Kiran et al., 2019). Bontpart et al. (2020) stated that phenotypic analysis of RSA can assess the soil resources acquired by the roots.
Most of the varieties with higher grain Fe content were found to produce vigorous root systems, and several varieties with lower grain Fe content formed weaker root systems. According to Oliveira et al. (2018), Fe accumulation in grain is affected by the uptake potential of root system architecture. However, some varieties with low grain Fe concentration developed long roots and vice versa. Low grain Fe concentration may develop long roots because root growth is stimulated at low rhizospheric Fe concentration to struggle for Fe acquisition (Giehl and Lima, 2012). A high grain Fe concentration producing a weaker root system might be due to metabolic reprogramming for the uptake of Fe by making phenotypic changes in roots, as elaborated by Yan et al. (2020). Plants absorb or restrict Fe acquisition by modifying their RSA (Brumbarova et al., 2015). Moreover, Fe transporters IRTI are also stimulated in roots under Fe-deficient conditions in order to regulate Fe homeostasis (Zhang et al., 2019). It reveals an excellent genotypic variation considering RSA and grain Fe content (Liu et al., 2021).
Diversification of RSA is necessary for plants to adapt to the complex rhizosphere (Kiran et al., 2019). Furthermore, it has been observed that varieties with smaller root lengths produce a greater number of lateral roots compared to varieties with larger root lengths. The unavailability of Fe triggers plants to produce more lateral roots in order to acquire the optimum quantity of Fe for growth (Zhang et al., 2019). However, it can be influenced by genotype and soil environment (Hodgkinson et al., 2017). Root system architecture index (RSAIN) considering all the RSA attributes showed a correlation with grain Fe concentration (Figure 2). In this study, the principal component analysis (PCA) shown in Figure 3 revealed that screening at the initial seedling stage by an association of grain Fe concentration and root system architecture can be a way forward for the selection of wheat varieties. Amongst the 15% of wheat varieties with vigorous root system architecture, 40% of varieties showed high grain Fe concentration. Out of the 85% of varieties with weaker root system architecture, 92% of varieties exhibited low grain Fe concentration.
Among the 12 varieties categorized by PCA, Dharabi-11 and Faisalabad-08 have Pastor-97 as a common ancestor in parentage, which also has a high grain Fe content, although grain Fe has been found to decline in modern varieties by 0.06 mg kg−1 year−1 (Shaukat et al., 2021). Dharabi-11 and Faisalabad 2008 are also drought- and disease-resistant, high-yielding varieties with longer roots (Hussain et al., 2009; Tariq et al., 2013). The variation in Fe uptake can be attributed to the variation in plant requirements and utilization because high Fe concentration in the root, shoot, husk, and grains led to a higher root, shoot, husk, and grain dry weight. Produce yield and quality can be improved by the application of micronutrient fertilizers (Kiran et al., 2022). Moreover, grain Fe concentration was enhanced under Fe-sufficient conditions compared to Fe-deficient conditions, as also documented in previous studies (Kiran et al., 2021). This indicates that soil Fe content has an ultimate impact on grain Fe concentration in wheat regardless of genetic variation, which endorses that the application of Fe fertilizer to soil is a successful strategy to biofortify wheat grain with Fe (Wakeel et al., 2018).
Higher grain weight exhibited higher grain Fe concentrations, while wheat varieties with lower grain weight showed lower concentrations of Fe, with some varieties showing deviation from this trend (Figures 4, 5), as observed in another study (Kiran et al., 2021). This may be due to the genotype influence and environmental conditions that make phytoavailable mineral content in wheat (Ishfaq et al., 2021). Conferring to another study, membrane transporters regulate the uptake and translocation of Fe. Under nutrient constraints inside plants, the consequence of signal conduction and regulation of H+-ATPase facilitated H+ efflux from root to soil takes place. The H+ efflux-induced acidification of the rhizosphere reduces ferric to ferrous ions, and Fe is transported inside the root cells through IRT transporters. Therefore, RSA variation in wheat varieties has an indirect effect on Fe uptake through H+ release from the roots (Conte and Walker, 2011; Zhang et al., 2019). Other factors include the release of phytosidrophores that chelate with Fe3+ and cross the root cell membrane via transporters (Rehman et al., 2021). Furthermore, enzymes nicotianaminsynthase (NAS) and nicotianamintranferase (NAAT) are involved in the translocation of Fe. The terminal destination of Fe translocation is considered the seed, where Fe is stored to be used for germination. Iron is sequestered in a seed vacuole in the form of ferritin (Fe storage proteins) via vacuolar iron transporters (Connorton et al., 2017; He et al., 2020).
The variable responses of the varieties, despite an equivalent dose of Fe being applied, may be due to the presence of different phytate contents in grain, which influences the Fe concentration of grain. Wheat varieties with higher grain Fe concentration exhibited lower phytate content. In the current study, Dharbi-11 exhibited the highest grain Fe concentration, extensive RSA, and lowest phytate content whereas, Ujala-16 showed the lowest grain Fe concentration, weaker RSA, and the highest phytate content (Figure 6). Since phytate is a captivating inhibitor of Fe, it decreases the bioavailability of Fe (Bilgrami et al., 2018). Similarly, Fe was localized in the aleurone layer and embryonic region (Photo 2), as was evident in the previous research conducted by Kiran et al. (2021).
It has also been observed that variations exist in grain yield among different varieties with the same concentration of Fe. It was found that an increase in chlorophyll content, photosynthetically active radiation absorption, and photosynthetic yield of photosystem II were supportive for enhanced yield. Photosynthetic efficiency enhances the yield potential of cereal crops since photosynthetic apparatus to light is also genotype-dependent (Yousef et al., 2022). Therefore, Fe fertigation alone does not provide an adequate solution to the problem of Fe deficiency in wheat; instead, the selection and cultivation of Fe-efficient wheat cultivars is the appropriate practice to cope with this matter (Wakeel et al., 2018). This work will be helpful for the selection of wheat varieties to develop Fe-biofortified wheat varieties with efficient Fe uptake and grain Fe content.
5. Conclusion
The current study has revealed that genetic variability in root system architecture (RSA) exists in wheat varieties cultivated in Pakistan during the last 40 years. The existence of a large genetic pool for grain Fe and RSA content in the pedigree was also observed in ancestors from which high grain Fe decendents arose. Although Fe transport systems are also variable in different varieties, as mentioned in previous studies, the impact of variable RSA is also obvious. Nevertheless, a vigorous root architecture system is not always efficient for Fe uptake. Approximately 77% of varieties showed weaker RSA and lower grain Fe content, indicating that a vigorous root system is significant in enhancing Fe uptake. Furthermore, it is also clear that vigorous RSA has not only increased shoot Fe concentration but also grain Fe content, and it has also improved yield as 90% of varieties with extensive RSA gave better yield. Based on the RSA study, the varieties Dharabi-11, Punjab-11, and Faisalabad-08 are identified as having the potential for Fe biofortification as well as high grain yield and can be used for further molecular studies to identify the genes responsible for it without jeoperdizing of yield and can be included in both conventional and molecular marker-based breeding programs.
Data availability statement
The original contributions presented in the study are included in the article/Supplementary material, further inquiries can be directed to the corresponding authors.
Author contributions
AK and AW: supervision and conceptualization. AK, AW, MS, and RS: methodology and draft reviewed and edited. RS: execution of the experimental work and first draft preparation. All authors contributed to the article and approved the submitted version.
Acknowledgments
We would like to thank Tariq Aziz Depalpur Campus University of Agriculture Faisalabad for providing access to the research facilities to conduct the Fe analysis.
Conflict of interest
The authors declare that the research was conducted in the absence of any commercial or financial relationships that could be construed as a potential conflict of interest.
Publisher’s note
All claims expressed in this article are solely those of the authors and do not necessarily represent those of their affiliated organizations, or those of the publisher, the editors and the reviewers. Any product that may be evaluated in this article, or claim that may be made by its manufacturer, is not guaranteed or endorsed by the publisher.
Supplementary material
The Supplementary material for this article can be found online at: https://www.frontiersin.org/articles/10.3389/fsufs.2023.1156728/full#supplementary-material
References
Alahmad, S., Hassouni, E. K., Bassi, F. M., Bassi, F. M., Dinglasan, E., Youssef, C., et al. (2019). A major root architecture QTL responding to water limitation in durum wheat. Front. Plant Sci. 10, 1–18. doi: 10.3389/fpls.2019.00436
Amiri, R., Bahraminejad, S., Sasani, S., Honarmand, J. S., and Fakhri, R. (2015). Bread wheat genetic variation for grain’s protein, iron and zinc concentrations as uptake by their genetic ability. Eur. J. Agron. 67, 20–26. doi: 10.1016/j.eja.2015.03.004
Amiri, R., Sasani, S., Honarmand, J. S., Rasaei, A., Seifolahpour, B., and Bahraminejad, S. (2018). Genetic diversity of bread wheat genotypes in Iran for some nutritional value and baking quality traits. Physiol. Mol. Biol. Plants 24, 147–157. doi: 10.1007/s12298-017-0481-4
Bilgrami, S. S., Houshmand, S., Kadivar, M., Fakheri, B., Zandi, P., Shariati, V., et al. (2018). Phytic acid, iron and zinc content in wheat ploidy levels and amphiploids: the impact of genotype and planting seasons. Arch. Agron. Soil Sci. 64, 331–346. doi: 10.1080/03650340.2017.1352085
Bontpart, T., Concha, C., Giuffrida, M. V., Robertson, I., Admkie, K., Degefu, T., et al. (2020). Affordable and robust phenotyping framework to analyze root system architecture of soil-grown plants. Plant J. 103, 2330–2343. doi: 10.1111/tpj.14877
Brumbarova, T., Bauer, P., and Ivanov, R. (2015). Molecular mechanisms governing Arabidopsis iron uptake. Trends Plant Sci. 20, 124–133. doi: 10.1016/j.tplants.2014.11.004
Cakmak, I., Pfeiffer, W. H., and McClafferty, B. (2010). Biofortification of durum wheat with zinc and iron. Cereal Chem. 87, 10–20. doi: 10.1094/CCHEM-87-1-0010
Connorton, J. M., Balk, J., and Rodríguez-Celma, J. (2017). Iron homeostasis in plants. Metallomics 9, 813–823. doi: 10.1039/c7mt00136c
Conte, S. S., and Walker, E. L. (2011). Transporters contributing to iron trafficking in plants. Mol. Plant 4, 464–476. doi: 10.1093/mp/ssr015
Divte, P., Yadav, P., Jain, K. P., Paul, S., and Singh, B. (2019). Ethylene regulation of root growth and phytosiderophore biosynthesis determines iron deficiency tolerance in wheat (Triticum spp). Environ. Exp. Bot. 162, 1–13. doi: 10.1016/j.envexpbot.2019.01.01
Estefan, G., Sommer, R., and Ryan, J. (2013). Methods of soil, plant and water analysis. nt. Cent. Agric. Res. Dry Areas. 3rd edition, 1–244. https://hdl.handle.net/20.500.11766/7512
Giehl, R. F. H., and Lima, J. E. (2012). Localized iron supply triggers lateral root elongation in Arabidopsis by altering the AUX1-mediated auxin distribution. Plant Cell 24, 33–49. doi: 10.1105/tpc.111.092973
Hassan, M. U., Aamer, M., Nawaz, M., Rehman, A., Aslam, T., Afzal, U., et al. (2021). Agronomic bio-fortification of wheat to combat zinc deficiency in developing countries. Pak. J. Agric. Res. 34, 201–217. doi: 10.17582/journal.pjar/2021/34.1.201.217
He, L. Z., Yue, C., Chen, C., Li, J., and Sun, Z. (2020). Enhancing iron uptake and alleviating iron toxicity in wheat by plant growth-promoting bacteria: theories and practices. Int. J. Agric. Biol. 23, 190–196. doi: 10.17957/IJAB/15.1276
Hodgkinson, L., Dodd, I. C., Binley, A., Ashton, R. W., White, R. P., Watts, C. W., et al. (2017). Root growth in field-grown winter wheat: some effects of soil conditions, season and genotype. Eur. J. Agron. 91, 74–83. doi: 10.1016/j.eja.2017.09.014
Hussain, M., Hussain, M., Khan, S., Anwar, J., and Akbar, M. (2009). Faisalabad-08: a new high yielding and disease resistant wheat variety. J. Agric. Res. 47, 365–374.
Ishfaq, M., Wakeel, A., Shahzad, M. N., Kiran, A., and Li, X. (2021). Severity of zinc and iron malnutrition linked to low intake through a staple crop: a case study in east-Central Pakistan. Environ. Geochem. Health 43, 4219–4233. doi: 10.1007/s10653-021-00912-3
Jeong, J., Merkovich, A., Clyne, M., and Connolly, E. L. (2017). Directing iron transport in dicots: regulation of iron acquisition and translocation. Curr. Opin. Plant Biol. 39, 106–113. doi: 10.1016/j.pbi.2017.06.014
Kiran, A., Wakeel, A., Mahmood, K., Mubaraka, R., and Haefele, S. M. (2022). Biofortification of staple crops to alleviate human malnutrition: contributions and potential in developing countries. Agronomy 12:452. doi: 10.3390/agronomy12020452
Kiran, A., Wakeel, A., Snowdon, R., and Friedt, W. (2019). Genetic Dissection of Root Architectural Traits by QTL and Genome - Wide Association Mapping in Rapeseed (Brassica napus) [M. Phil Thesis]. University of Giessen. 184–192.
Kiran, A., Wakeel, A., Sultana, R., Khalid, A., Ain, Q., Mubaraka, R., et al. (2021). Concentration and localization of Fe and Zn in wheat grain as affected by its application to soil and foliage. Bull. Environ. Contam. Toxicol. 106, 852–858. doi: 10.1007/s00128-021-03183-x
Li, G., Kronzucker, H. J., and Shi, W. (2016). The response of the root apex in plant adaptation to iron heterogeneity in soil. Front. Plant Sci. 7:344. doi: 10.3389/fpls.2016.00344
Liu, H., Fiorani, F., Jack, O., Colombi, T., Nagel, K. A., and Weih, M. (2021). Shoot and root traits underlying genotypic variation in early vigor and nutrient accumulation in spring wheat grown in high-latitude light conditions. Plan. Theory 10, 1–18. doi: 10.3390/plants10010174
Lobet, G., Pages, L., and Draye, X. (2011). A novel image-analysis toolbox enabling quantitative analysis of root system architecture. Plant Physiol. 157, 29–39. doi: 10.1104/pp.111.179895
Mamrutha, H. M., Kumar, R., Venkatesh, K., Sharma, P., Kumar, R., Tiwari, V., et al. (2014). Genetic transformation of wheat – present status and future potential. J. Wheat Res. 6, 107–119.
Maqbool, S., Ahmad, S., Kainat, Z., Khan, M. I., Maqbool, A., Hassan, M. A., et al. (2022). Root system architecture of historical spring wheat cultivars is associated with alleles and transcripts of major functional genes. BMC Plant Biol. 22, 590–515. doi: 10.1186/s12870-022-03937-7
Marschner, P., and Rengel, Z. (2023). “Nutrient availability in soils” in Marschner's Mineral Nutrition of Plants (Netherlands: Academic Press), 499–522.
Maskova, T., and Klimes, A. (2020). The effect of rhizoboxes on plant growth and root: shoot biomass partitioning. Front. Plant Sci. 10, 1–5. doi: 10.3389/fpls.2019.01693
Ober, E. S., Alahmad, S., Cockram, J., Forestan, C., Hickey, L. T., Kant, J., et al. (2021). Wheat root systems as a breeding target for climate resilience. Theor. Appl. Genet. 134, 1645–1662. doi: 10.1007/s00122-021-03819-w
Oliveira, G. A. L., Chander, S., Ortiz, R., Menkir, A., and Gedil, M. (2018). Genetic basis and breeding perspectives of grain iron and zinc enrichment in cereals. Front. Plant Sci. 9:937. doi: 10.3389/fpls.2018.00937
Owaidah, T., Numair, N., Suliman, A., Zolaly, M., Hasanato, R., Zahrani, F., et al. (2020). Iron deficiency and iron deficiency anemia are common epidemiological conditions in Saudi Arabia: report of the national epidemiological survey. Anemia. 2020:6642568. doi: 10.1155/2020/6642568
Rehman, A., Masood, S., Ullah, N., Abbasi, E., Hussain, Z., and Ali, I. (2021). Molecular basis of iron biofortification in crop plants; a step towards sustainability. Plant Breed 140, 12–22. doi: 10.1111/pbr.12886
Riaz, A., Huda, N., Abbas, A., and Raza, S. (2017). Biofortification of wheat with iron. Int. J. Adv. Sci. Res. 3, 69–76. doi: 10.7439/ijasr.v3i7.4275
Rufo, R., Salvi, S., Royo, C., and Soriano, J. M. (2020). Exploring the genetic architecture of root-related traits in Mediterranean bread wheat landraces by genome-wide association analysis. Agronomy 10:613. doi: 10.3390/agronomy10050613
Shaukat, M., Sun, M., Ali, M., Mahmood, T., Naseer, S., Maqbool, S., et al. (2021). Genetic gain for grain micronutrients and their association with phenology in historical wheat cultivars released between 1911 and 2016 in Pakistan. Agronomy 11:1247. doi: 10.3390/agronomy11061247
Shobhana, V. G., Senthil, N., Kalpana, K., Abirami, B., Sangeetha, J., Saranya, B., et al. (2013). Comparative studies on the iron and zinc contents estimation using atomic absorption spectrophotometer and grain staining techniques (prussian blue and Dtz) in maize germplasms. J. Plant Nutr. 36, 329–342. doi: 10.1080/01904167.2012.744419
Tariq, M., Mahmood, A., Mian, M. A., Cheema, N. M., Sabar, M., and Ihsan, M. (2013). Dharabi-11: a new high yielding drought and disease tolerant wheat variety. Int. J. Agric. Biol. 15, 701–706.
Timilsena, Y. P., Adhikari, R., Casey, P., Muster, T., Gill, H., and Adhikari, B. (2015). Enhanced efficiency fertilisers: a review of formulation and nutrient release patterns. J. Sci. Food Agric. 95, 1131–1142. doi: 10.1002/jsfa.6812
Wakeel, A., Farooq, M., Bashir, K., Ozturk, L. H., Anwar, M., Kamiya, T., et al. (2018). “Micronutrient malnutrition and biofortification: recent advances and future perspectives”. in Plant micronutrient use efficiency: Molecular and genomic perspectives in crop plants. eds. Hossain, Anwar, M., Kamiya, Takehiro, Burritt, David, J. and Tran, Phan, L.S and Fujiwara, Toru (Elsevier, UK, USA: Academic Press), 225–243.
Welch, R. M., and Graham, R. D. (2004). Breeding for micronutrients in staple food crops from a human nutrition perspective. J. Exp. Bot. 55, 353–364. doi: 10.1093/jxb/erh064
Wu, Y., Ye, H., Liu, J., Ma, Q., Yuan, Y., Pang, Q., et al. (2020). Prevalence of anemia and sociodemographic characteristics among pregnant and non-pregnant women in Southwest China: a longitudinal observational study. BMC Pregnancy Childbirth 20, 1–10. doi: 10.1186/s12884-020-03222-1
Yan, L., Li, P., Zhao, X., Ji, R., and Zhao, L. (2020). Physiological and metabolic responses of maize (Zea mays) plants to Fe3O4 nanoparticles. Sci. Total Environ. 718:137400. doi: 10.1016/j.scitotenv.2020.137400
Yousef, A. F. M. M., Ali, H. M., Rizwan, S. A., Tadda, H. M., Kalaji, H., Yang, M. A. A., et al. (2022). Photosynthetic apparatus performance of tomato seedlings grown under various combinations of LED illumination. Plos one. 16:e0249373. doi: 10.1371/journal.pone.0249373
Keywords: root traits, rhizobox, soil iron, adaptability potential, grain Fe content, Fe localization
Citation: Sultana R, Kiran A, Sanaullah M and Wakeel A (2023) Exploring the linkage between root system architecture and grain iron content in wheat (Triticum aestivum L.). Front. Sustain. Food Syst. 7:1156728. doi: 10.3389/fsufs.2023.1156728
Edited by:
Manosh Kumar Biswas, University of Leicester, United KingdomReviewed by:
Zina Flagella, University of Foggia, ItalyFaisal Nadeem, University of Agriculture, Dera Ismail Khan, Pakistan
Copyright © 2023 Sultana, Kiran, Sanaullah and Wakeel. This is an open-access article distributed under the terms of the Creative Commons Attribution License (CC BY). The use, distribution or reproduction in other forums is permitted, provided the original author(s) and the copyright owner(s) are credited and that the original publication in this journal is cited, in accordance with accepted academic practice. No use, distribution or reproduction is permitted which does not comply with these terms.
*Correspondence: Aysha Kiran, YXlzaGEua2lyYW5AdWFmLmVkdS5waw==; Abdul Wakeel, YWJkdWwud2FrZWVsQHVhZi5lZHUucGs=