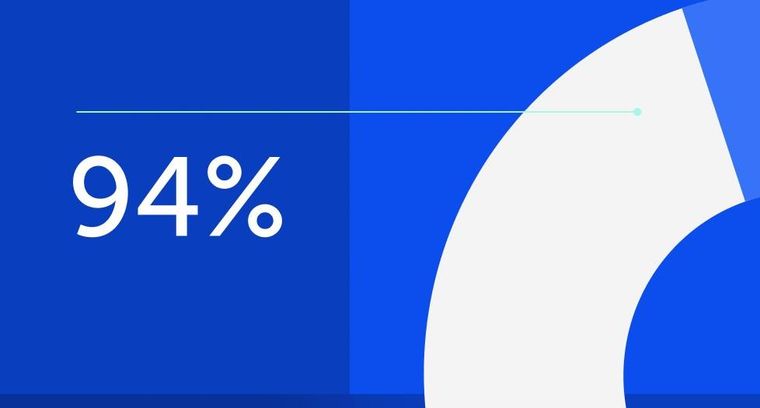
94% of researchers rate our articles as excellent or good
Learn more about the work of our research integrity team to safeguard the quality of each article we publish.
Find out more
ORIGINAL RESEARCH article
Front. Sustain. Food Syst., 28 March 2023
Sec. Agroecology and Ecosystem Services
Volume 7 - 2023 | https://doi.org/10.3389/fsufs.2023.1155458
This article is part of the Research TopicCrop Pest Control and Pollination, volume IIView all 14 articles
Insects are key pollinators to ecosystem function, but much work remains to determine the most cost-effective, reliable scheme to monitor them. Pan traps (PT) and flight interception traps (FIT) are two of the most popular insect sampling methods used. However, their relative sampling performance and cost is poorly known for agroecosystems in China. We conducted a study across 18 oilseed rape fields in smallholder farmland in Zhejiang, China using these two traps. Our results showed that a single FIT had a greater sampling efficiency (more individuals and higher species richness) than a single PT, but controlling for cost, four PTs (the cost for four PTs is close to one FIT) showed a greater sampling efficiency than FITs. PTs collected more small-bodied individuals while FITs and PTs did not significantly differ in terms of monitoring pollinator insects with large body size. When exploring whether semi-natural habitat embedded in the agricultural landscape affected these results, results from both trap types shows that semi-natural habitat had a significant positive impact on wild pollinator diversity and rarefied species richness. Future studies that examine the effects of agricultural landscape on the wild pollinator community should combine PTs with netting or other active methods for long-term wild pollinator monitoring strategies.
Insect pollinators provide important ecological services for crops globally (Klein et al., 2007; Aizen et al., 2009), but recent reports of decline make their future uncertain (Potts et al., 2010, 2016; Rhodes, 2018; LeBuhn and Vargas Luna, 2021). The decline of pollinators in the agroecosystems may lead to yield losses in pollinator-dependent crops (Steffan-Dewenter et al., 2005). To better conserve insect pollinators and maintain their pollination services, it is necessary to monitor their population dynamics and community composition using solid sampling methods (Howlett et al., 2009; Westerberg et al., 2021), as otherwise recommendations and subsequent conservation actions could prove ineffective.
Insect monitoring is generally done through a combination of active and passive collection methods. Active methods are relatively straightforward, involving either direct specimen collection or observation, and are best paired with passive sampling to ensure a full accounting of local biodiversity (Gibbs et al., 2017; Templ et al., 2019; Portman et al., 2020; Prendergast et al., 2020). Passive methods are more complicated, carried out through various types of traps (Prendergast et al., 2020). Notably, it is exceedingly important to test passive traps across settings, to understand their biases and also ensure that they are used in a responsible manner that does not potentially cause decline of local susceptible species (Gibbs et al., 2017; Portman et al., 2020).
Various passive sampling traps have been developed for pollinating insects, including pan trap (Cane et al., 2000; Westphal et al., 2008) and flight interception trap (Howlett et al., 2009). Pan traps (PTs) are colored containers filled with liquid that attract flower-visiting insects (Cane et al., 2000; Westphal et al., 2008); they have been widely applied in previous studies for pollinator biodiversity monitoring due to their cost-effectiveness (Zou et al., 2017; Wu et al., 2018; Larkin and Stanley, 2021; Shi et al., 2022a). However, as an attractant-based sampling trap, they have inherent biases (Cane et al., 2000). PT's sampling performance may be affected by surrounding flowering plants (Steven et al., 2003; Baum and Wallen, 2011; Westerberg et al., 2021), especially when deployed in mass-flowering crops, and tend to be biased toward pollinators with similar traits, for instance, ground nesters (Roulston et al., 2007), or those from the family Halictidae. Flight interception traps (FIT) are non-attractant traps and might result in different catches from PTs (Lamarre et al., 2012; Mesa et al., 2013). FITs are essentially transparent panes used to direct flying insects into collection traps held below (Knuff et al., 2019; González et al., 2020).
Some studies have previously compared PT and FIT efficacy, but these are largely outside of pollinator-dependent agroecosystems and have largely been conducted in just temperate areas (Kehinde and Samways, 2012; Cunningham et al., 2013; Rader et al., 2014). Additionally, sampling methods may be biased toward pollinators with different functional traits (Prendergast et al., 2020). Body size is important functional traits for pollinators and larger pollinators have been found to provide superior pollination services (Huda et al., 2015; Jauker et al., 2016). While some studies have explored the impacts of body size for trapping (McCravy et al., 2019; Krahner et al., 2021; Thompson et al., 2021), PTs and FITs have yet to be explicitly compared as such.
Habitat type can also strongly influence trapping results. In agricultural landscapes, semi-natural habitats (forest, shrub and grassland) can support the local wild pollinator communities through offering floral resources and nesting locations (Garibaldi et al., 2020; Raderschall et al., 2021; Shi et al., 2021). Consequently, it is necessary to account for habitat types in agroecosystems from a broad array of climates and crops, as otherwise the generality of best practices for trapping may be limited to overstudied temperate environments.
In this study, we collected wild pollinators using PTs and FITs in 18 oilseed rape (Brassica napus L.) fields in the smallholder agricultural landscapes in subtropical China. Oilseed rape requires insect pollination for optimal yield (pollinator-dependent) (Zou et al., 2017). Thus, monitoring pollinator diversity for oilseed rape is essential for agricultural production (Ouvrard et al., 2019). However, our knowledge of best practices for monitoring in oilseed rape is limited. In conducting long-term monitoring, financial (money to make traps) and labor costs (effort in assembly and deployment) must be considered. Our aims were to (1) assess the pollinator diversity for oilseed rape in these areas; (2) compare the sampling performance of these two trap types under the same budget, accounting for wild pollinator abundance and species richness. We further explore whether these two sampling methods are biased toward pollinators' body size; (3) To account for the effect of agricultural landscape, we also collected in a range of situations involving varying levels of semi-natural habitat. Thereby, we provide a better framework for understanding and monitoring crop pollinator diversity in the under-studied subtropics of East Asia.
This study was conducted in oilseed rape fields in Kaihua County, Zhejiang Province, China. Kaihua County was chosen for its small field sizes due to montane terrain (Lou et al., 2019). Thereby, these landscapes well represent smallholder plantations. In the early spring, oilseed rape is the most dominant crop there. Later in May, oilseed rape will be harvested and rice will be cultivated in the same field (oilseed rape-rice rotation). Oilseed rape production is essential for local smallholder farmers' livelihood since many farmers use oilseed rape as their cooking oil sources rather than for sale. Oilseed rape pollinators sampling started at the end of February and ended in late April in 2022, covering the whole flowering season of oilseed rape. In total, 18 research sites were selected with one field each (Figure 1). All of the fields were managed using smallholder farming practices (< 2 hectares) (Lowder et al., 2016). The minimum distance between two sampling sites was 1.9 km with montane terrain between them, exceeding the average foraging distance of many insect pollinators (Chifflet et al., 2011). Semi-natural habitat is defined as the habitat in the agricultural landscape where non-crop plants grow (Holland et al., 2016). Semi-natural habitats in this study include forest, shrub and grassland that embedded in the agricultural landscape and water bodies, like rivers and streams were not included in the analysis, as in prior studies in China (Zou et al., 2017; Shi et al., 2021, 2022b) and abroad (Papanikolaou et al., 2017; Zou et al., 2017; Shi et al., 2021). The land-use data (forest, shrub, grassland and other) in the 1000 m radius for the focal oilseed rape field was collected using ground verification methods (Liu et al., 2016; Zou et al., 2017) in the spring of 2022. We selected a 1000 m radius for our landscape analysis as this is the foraging range for many pollinators (Zurbuchen et al., 2010; Chifflet et al., 2011) and the strongest impact of agricultural landscape on pollinator diversity has been shown at a scale of 1000 m radius (e.g., Zou et al., 2017). The proportion of semi-natural habitat in each site was calculated in Arcmap 10.8.
FITs and PTs were deployed in one focal oilseed rape field at each sampling site. Each FIT was composed of a transparent acrylic plate (55*50 cm and 3 mm in thickness) fixed on two wooden sticks using plastic cable ties. Under the acrylic plate, a white plastic tray (60*43 cm and 11 cm in depth) was fixed to the sticks using metal wires. A PT array was made of three plastic cups (450 ml with diameter of 8.3 cm) with UV white, blue and yellow color, fixed on a stick about 1.5 m in height using metal ring cupholders. For both interception trap and PTs, small holes were drilled near the top of the containers for rainwater drainage. Photos of the two trap types can be found in Figure 2. The cost of manufacturing 18 FITs was 1450 RMB, including acrylic plates (650 RMB), plastic tray (630 RMB), wooden sticks (90 RMB), and iron wire and plastic cable ties (80 RMB). The money cost of manufacturing 72 PTs was 1410 RMB, including plastic cups (500 RMB), paint (550 RMB), and wooden sticks (360 RMB). The money costs of manufacturing one FIT (80.6 RMB) and four PTs (78.3 RMB) were close. In addition, the human labor for manufacturing two trap types were close (e.g., labor hours for both traps were around 16 h in 2 days). Thus, in each focal oilseed rape field, four PT sets were placed ~1 m from the field edge and one FIT was deployed, along a randomly-selected field edge for intercepting pollinators visiting the focal oilseed rape field. As two passive traps, FIT and PT sampling does not involve in intensive labor, which is different sweeping net that involving intensive labor work from experienced personnel. Overall, monetary and labor costs for conducting this pollinator sampling project were both low and feasible.
Samples were collected and traps reset every seven days, resulting in 52 sampling days per site. The collected samples were stored in the refrigerator (-20°C) for further species identification. European honeybees (Apis mellifera), as managed non-native pollinators in China, were excluded from this study. All insect samples were sorted to morpho-species and then identified to species or morphospecies by taxonomists. Pollinators were classified as large (body length > 12 mm) and small (body length < 12 mm) insects according to Albrecht et al. (2007). Hence, butterflies, carpenter bees, bumblebees, wasps, one longhorn bee (Eucera floralia), two digger bees (Anthophora villosula and Anthophora plagiata), two scoliid wasp species (Scoliidae sp1 and Scoliidae sp2) and one large hoverfly (Phytomia zonata) were grouped as large-bodied pollinators and the rest pollinators were grouped as small-bodied pollinators (Supplementary Table S1).
Samples from each site caught using the same sampling method were pooled to have a robust dataset. Linear mixed models were used to compare the differences in wild pollinator abundance, diversity and species richness between two trap types, of which study site was the random factor. The abundance of the three most abundant species (Eucera floralia, Apis cerana and Gametis jucunda), as well as the abundance of large and small pollinators between the two trap types were also compared via linear mixed models. Species richness was rarefied to 31 individuals which is the lowest sample size using FITs and PTs among all sites. Abundance was calculated using the total amount of pollinator individuals divided by sampling days (e.g. number of individuals per sampling days). We firstly compared catches of one set of FITs with a set of PTs, then with single PTs.
In order to check whether or not samples from FITs and PTs had similar species compositions, we calculated the beta-diversity based on each site and different traps (i.e,. one FIT vs. 4 PTs from each site). To compare the pollinator community composition, we used Principal Coordinate Analysis (PCoA) based on Bray-Curtis distance. The Bray-Curtis distance matrix was calculated as an index for beta-diversity, and is relatively robust to sampling size (Ricotta and Podani, 2017). We then used Principal Coordinate Analysis (PCoA) to visualize our results. ANOSIM was conducted with 9999 permutations to analyze the dissimilarity between pollinator communities in PTs and FITs. We also conduct indicator species analysis to check the species that were more often collected by one trap type.
Multiple linear regression was used to investigate the effect of the proportion of semi-natural habitats in on wild pollinator abundance and rarefied species richness. The proportion of semi-natural habitats at 1000 m was used as our response variable. To check whether semi-natural habitats had consistent effects on samples from different traps, interactions between proportion of semi-natural habitats and trap types were added as an explanatory variable. In the case of no interaction effect (and this is our case, see “Results”), analysis between semi-natural habitats and pollinator variables were conducted separately for FITs and PTs. We checked the heteroscedasticity for all linear regression models. To check for spatial autocorrelation, we calculated Moran's I of model residuals (Gittleman and Kot, 1990) and we did not detect any significant spatial correlations in any of the analyses in this study (p > 0.05).
All statistical analyses were conducted in R 3.5.2 (R Core Team, 2016). To calculate rarefied species richness at each site (n = 31, which was the least number of sampled wild pollinator using FITs and PTs among all sites), we used the package “vegan” (Oksanen et al., 2019). To produce rarefaction extrapolation curves, we used the function iNEXT in the package “iNEXT” (Hsieh et al., 2016). Function “lme()” packages “nlme” (Pinheiro et al., 2017) was used to conduct the linear mixed model. Function “anosim ()” in the “vegan” package (Oksanen et al., 2019) was used to compare the differences in wild pollinator community composition in FITs and PTs. Function “multipatt” in the package “indicspecies” was used to conduct indicator species analysis (De Caceres et al., 2016). Breusch-Pagan test using “bptest ()” function in R package “lmtest” (Hothorn et al., 2015) was used to check the heteroscedasticity. Package “ape” (Paradis and Schliep, 2019) was used to check the Moran's I value.
In total, 2,970 (53 species) wild pollinator specimens were collected by FITs (1,230 individuals; 33 species) and PTs (1,740 individuals; 50 species) (species list see Supplementary Table S1 and rarefaction curve for PT and FIT see Supplementary Figure S1). The five most abundant wild pollinator species sampled by FITs were Eucera floralia (226), Apis cerana (203), Gametis jucunda (158), Pieris rapae (152) and Halictus aerarius (62). The five most abundant wild pollinators collected by PTs were E. floralia (254), G. jucunda (161), Xylocopa tranquabaroroum (146), A. cerana (140), and Lasioglossum sp (133) (Supplementary Table S1). Single FITs catch significantly more individuals per sampling day (1.31 ± 0.14) and rarefied species (9.22 ± 0.46) than single PTs (0.47 ± 0.04 per sampling day and 2.61 ± 0.12 species) (p < 0.05) while four PTs collected significantly higher individuals per sampling day (1.86 ± 0.14) and species (10.43 ± 0.46) than single FITs (p < 0.05) (Figures 3A, B). There was no significant difference in the abundance per sampling day of the three dominant pollinator species A. cerana, G. jucunda and E. floralia between PTs and FITs (p > 0.05). Lasioglossum sp.1, Ceratina japonica, Lasioglossum sp2, Chrysomya megacephala, Lasioglossum sp were five species caught significantly more often in the PTs (p < 0.0.05) while no species caught significantly more often in the FITs.
Figure 3. Number of species (A) and (B) pollinator individuals collected by a FIT, a single PT and four PTs combined (4PTs).
The proportion of each wild pollinator species (log-transformed) in the insect in PTs and FITs was significantly correlated (r2 = 0.70, p < 0.001) (Figure 4). Wild pollinator abundance measures collected using FITs and PTs were not significantly correlated (p > 0.05), but species richness and diversity in FITs and PTs were significantly positively correlated (p < 0.05). The analysis of similarities (ANOSIM) test recovered significant differences between the pollinator communities collected by FITs and PTs overall (R = 0.2081; p < 0.001; Figure 5). There was no significant difference in the abundance and relative abundance of large-sized pollinators in FITs and PTs (p > 0.05) (Supplementary Figure S2). The abundance of small pollinator insects in PTs was significantly higher than in FITs (p < 0.05) while there was no significant difference in relative abundance (p > 0.05) (Supplementary Figure S2).
Figure 5. Principal coordinate analysis (PCoA) ordination of wild pollinator communities using pan traps (PT) and flight interception traps (FIT). The ellipses indicate 95% confidence intervals.
Semi-natural habitat in both small-scale (500 m) and large-scale (1000 m) had positive effects on wild pollinator rarefied species richness and diversity (p < 0.05), while semi-natural habitat had no significant impact on wild pollinator abundance (p > 0.05) (Table 1). There was no significant effect of sampling type on wild pollinator abundance, rarefied species richness or diversity (p > 0.05) (Figure 6; Table 1). There was also no significant effect of interactions between sampling types and semi-natural habitats on wild pollinator abundance, rarefied species richness and diversity (p > 0.05) (Figure 6; Table 1). At the 1000 m radius scale, semi-natural habitat had no significant effect on wild pollinator abundance collected by FITs (slope = 0.09, intercept = 1.25, p > 0.05) or PTs (slope = 0.29, intercept = 1.65, p > 0.05) (Figure 6A). Semi-natural habitat had a significant positive impact on wild pollinator rarefied species richness collected by both FITs (slope = 10.43, intercept = 1.53, p < 0.001) and PTs (slope = 8.35, intercept = 4.28, p < 0.05) (Figure 6B). Semi-natural habitat in the agricultural landscape had significant positive impact on wild pollinator diversity collected by FITs (slope = 1.41, intercept = 0.95, p < 0.001) and had marginally significant effect on diversity collected by PTs (slope = 0.88, intercept = 1.52, p=0.07) (Figure 6C). At the 500 m radius scale, semi-natural habitat had no significant effect on wild pollinator abundance collected by FITs (slope = 0.72, intercept = 0.88, p > 0.05) or PTs (slope = 0.84, intercept = 1.36, p > 0.05) (Figure 6D). Semi-natural habitat had a significant positive impact on wild pollinator rarefied species richness collected by both FITs (slope = 7.31, intercept = 4.86, p < 0.001) and PTs (slope = 7.20, intercept = 6.13, p < 0.05) (Figure 6E). Semi-natural habitat in the agricultural landscape had significant positive impact on wild pollinator diversity collected by FITs (slope = 1.13, intercept = 1.31, p < 0.001) and PTs (slope = 0.82, intercept = 1.68, p < 0.05) (Figure 6F).
Table 1. The impact of sampling methods (FIT and PT; base: FIT), the proportion of semi-natural habitat and their interactions on wild pollinator abundance per sampling day, rarefied species richness and Shannon diversity in large scale (1000 m radius) and small scale (500 m radius).
Figure 6. The impact of sampling methods (FIT and PT) and the proportion of semi-natural habitat in on (A, D) wild pollinator abundance (per sampling day), (B, E) rarefied species richness and (C, F) Shannon diversity. (A–C) were in 1000 m radius and (D–F) were in 500 m radius scale. Solid lines occur where the impact of semi-natural habitat was significant (p < 0.05).
Despite much prior research on the topic, there remain many gaps in our knowledge of effective monitoring for pollinating insects, especially in subtropical environments of Asia and for the multitude of passive trap methods (Cane et al., 2000; Campbell and Hanula, 2007; González et al., 2020; Van Drunen et al., 2022). The sampling performance of FITs and PTs, as two common passive pollinator sampling methods, has been assessed at limited scales (one oilseed rape field) previously and found flight interception trap outperform pan trap (more individuals and species collected per trap; Shi et al., 2022b). Here, we expanded on prior efforts by expanding sampling coverage and incorporating trait and semi-natural area analyses to determine whether these factors introduce biases.
Our results suggested that both FITs and PTs are surprisingly similar in their per-cost effectiveness and coverage in trapping pollinators. Results showed that a single FIT caught more individuals and species than single PTs, agreeing with the prior study (Rubene et al., 2015; Shi et al., 2022b). It seems likely that the highly attractive oilseed rape reduces the catches of PTs, so non-attractant FITs are less impacted by this and collect more specimens per unit (Baum and Wallen, 2011; Vrdoljak and Samways, 2012; Prendergast et al., 2020).
To scale for large monitoring efforts, cost must also be considered. We found that PTs (four per site) outperformed FITs (one per site, similar in cost to four PT sets) in oilseed rape fields (more wild pollinator individuals and species), while there was also now a difference in pollinator community composition between the two trap types. Notably, FITs can still manage to catch wild pollinator species that PTs did not, such as Xylocopa nasalis (a common carpenter bee species widely distributed in southern China), and more individuals of Apis cerana. In addition, FITs can be used to track wild pollinator movement patterns in agricultural landscapes (Cunningham et al., 2013) while PTs cannot. Thus, depending on the budget and research questions, FITs might still be useful under some circumstances.
More small pollinators were collected by pan traps and more small species, such as Lasioglossum sp, were significantly caught by pan traps, while there were no significant differences in the collection of large-bodied species between PTs and FITs, an outcome previously unreported. Contrastingly, McCravy et al. (2019) found that Malaise traps, which are somewhat similar to FITs in that they physically block flight for capture, collected a higher proportion of small wild bees than PTs and vane traps. Krahner et al. (2021) also found that Malaise traps collected higher proportion of small wild bee than PTs, but their results were not consistent across 2 years. Consequently, it seems that these methods may both be better than malaise traps for sampling larger species, but this requires further study, and the unique collection of Xylocopa nasalis by only FITs suggests that perhaps some of the largest pollinators might still more easily avoid PTs.
Semi-natural habitat also had consistent positive impact on wild pollinator diversity and species richness across trap types. Semi-natural habitat inside agricultural landscapes can benefit wild pollinators through providing diverse floral resources as food, potential nesting sites and nesting materials (Tscharntke et al., 2005), and numerous studies have confirmed the important role of semi-natural habitats in maintaining wild pollinator diversity (Le Féon et al., 2010; Carvell et al., 2011; Zou et al., 2017; Wu et al., 2019; Shi et al., 2021). Prior studies have suggested that oilseed rape, as high-quality pollen and nectar source, can be extremely attractive for pollinators (Holzschuh et al., 2013) but both trapping methods recovered a positive effect of semi-natural areas nonetheless, possibly due to pesticides or other management regime factors specific to the area.
Many ecological studies integrate multiple trap types. The most commonly used combination is active sweeping net and passive PTs (Morandin et al., 2007; Kwaiser and Hendrix, 2008; Gill and O'Neal, 2015; Perrot et al., 2018; Van Drunen et al., 2022), and it is generally accepted that such a mix of active and passive methods is optimal (Prendergast et al., 2020). Many such studies have pooled samples across methods (Morandin and Winston, 2005; Tucker and Rehan, 2018), but some studies choose to analyze the results from different approaches separately (Perrot et al., 2018). FITs and PTs have also previously been analyzed together (Kehinde and Samways, 2012; Rader et al., 2014), but the consistency of these two traps across habitat types was previously unknown. With further testing in additional sites, it may be that FITs and PTs can be generally analyzed together, such that multiple questions can be addressed. To avoid overgeneralization, more sampling projects ought to be conducted at other sites, such as Jiangxi, Fujian and Hunan provinces, to comprehensively understanding the pollinator community in smallholder farmland in China. In addition, the potential competition between different methods used at the same sampling point (i.e., one trap may affect the other trap's catches) should be explored. Other potential concerns, for instance, causing damaging to crop when deploying traps and collecting samples, should be addressed in further studies.
In conclusion, PTs were more efficient than FITs in oilseed rape fields across various agricultural landscapes with a gradient of semi-natural habitat coverage in a cost-effectiveness framework. In terms of monitoring large and small-bodied pollinators, PTs collected more small individuals while there were no significant differences between FITs and PTs. Both also produced consistent results when investigating the impact of semi-natural habitat on wild pollinator diversity and species richness (semi-natural habitat had a significant positive impact on wild pollinator diversity and rarefied species richness). Consequently, PTs appear to be a viable method for monitoring pollinator diversity in subtropical agroecosystems, and these efforts could be enhanced by incorporating active sampling methods such as netting (Prendergast et al., 2020). Notably, although we recovered similar catches from these two methods, their sampling efficiency may vary in different habitats. Thus, further testing in additional habitats and climate would be useful for developing a generalizable guide for what methods should be used under different circumstances. Given the predominance of PTs in the literature, we suggest that for comparability purposes they should be preferred when limited resources for passive trapping are available, with resources devoted additionally to active methods that would better complement passive methods to provide a clearer view of the fauna.
The raw data supporting the conclusions of this article will be made available by the authors, without undue reservation.
X-YS, C-DZ, and YZ conceived the study. MO, HQ, AL, Q-SZ, PG, M-QW, and ZN were involved in the study design. X-YS collected the data and conducted the statistical analysis. All authors contributed to the article and approved the submitted version.
X-YS was funded as a postdoc researcher by both National Science and Technology Fundamental Resources Investigation Program to investigate pollinator insects in East China (2018FY100400) and the National Science Fund for Excellent Young Scholars (No. 32122016) to AL. C-DZ's lab was supported by grants from the Key Laboratory of the Zoological Systematics and Evolution of the Chinese Academy of Sciences (grant number 2008DP173354) and State Key Laboratory of Integrated Management of Pest Insects and Rodents.
We thank Feng Yuan from Institute of Zoology, Chinese Academy of Sciences, for identifying the pollinators. We thank all the oilseed rape farmers for their permission to sample in their fields as well as field work assistance. We thank Zhejiang Qianjiangyuan Forest Biodiversity National and Research Station for their support.
The authors declare that the research was conducted in the absence of any commercial or financial relationships that could be construed as a potential conflict of interest.
All claims expressed in this article are solely those of the authors and do not necessarily represent those of their affiliated organizations, or those of the publisher, the editors and the reviewers. Any product that may be evaluated in this article, or claim that may be made by its manufacturer, is not guaranteed or endorsed by the publisher.
The Supplementary Material for this article can be found online at: https://www.frontiersin.org/articles/10.3389/fsufs.2023.1155458/full#supplementary-material
Aizen, M. A., Garibaldi, L. A., Cunningham, S. A., and Klein, A. M. (2009). How much does agriculture depend on pollinators? Lessons from long-term trends in crop production. Ann Bot. 103, 1579–1588. doi: 10.1093/aob/mcp076
Albrecht, M., Duelli, P., Müller, C., Kleijn, D., and Schmid, B. (2007). The Swiss agri-environment scheme enhances pollinator diversity and plant reproductive success in nearby intensively managed farmland. J Appl Ecol 44, 813–822. doi: 10.1111/j.1365-2664.2007.01306.x
Baum, K. A., and Wallen, K. E. (2011). Potential bias in pan trapping as a function of floral abundance. J. Kans. Entomol. Soc. 84, 155–159. doi: 10.2317/JKES100629.1
Campbell, J. W., and Hanula, J. (2007). Efficiency of Malaise traps and colored pan traps for collecting flower visiting insects from three forested ecosystems. J Insect Conserv 11, 399–408. doi: 10.1007/s10841-006-9055-4
Cane, J. H., Minckley, R. L., and Kervin, L. J. (2000). Sampling bees (Hymenoptera : Apiformes) for pollinator community studies: Pitfalls of pan-trapping. J. Kans. Entomol. Soc. 73, 225–231.
Carvell, C., Osborne, J. L., Bourke, A. F., Freeman, S. N., Pywell, R. F., Heard, M. S., et al. (2011). Bumble bee species' responses to a targeted conservation measure depend on landscape context and habitat quality. Ecol. Appl. 21, 1760–1771. doi: 10.1890/10-0677.1
Chifflet, R., Klein, E. K., Lavigne, C., Le Féon, V., Ricroch, A. E., Lecomte, J., et al. (2011). Spatial scale of insect-mediated pollen dispersal in oilseed rape in an open agricultural landscape. J. Appl. Ecol. 48, 689–696. doi: 10.1111/j.1365-2664.2010.01904.x
Cunningham, S. A., Schellhorn, N. A., Marcora, A., and Batley, M. (2013). Movement and phenology of bees in a subtropical Australian agricultural landscape. Austral Ecol. 38, 456–464. doi: 10.1111/j.1442-9993.2012.02432.x
De Caceres, D., Jansen, M., and De Caceres, F. M. M. (2016). Indicator Species Analysis using ‘indicspecies’. Available online at: https://cran.r-project.org/web/packages/indicspecies/vignettes/IndicatorSpeciesAnalysis.html (accessed March 13, 2023).
Garibaldi, L., Oddi, F., Miguez, F., Bartomeus, I., Orr, M., Jobbágy, E., et al. (2020). Working landscapes need at least 20% native habitat. Conserv. Letters 14, 12773. doi: 10.1111/conl.12773
Gibbs, J., Joshi, N. K., Wilson, J. K., Rothwell, N. L., Powers, K., Haas, M., et al. (2017). Does passive sampling accurately reflect the bee (apoidea: anthophila) communities pollinating apple and sour cherry orchards? Environ. Entomol. 46, 579–588. doi: 10.1093/ee/nvx069
Gill, K., and O'Neal, M. (2015). Survey of soybean insect pollinators: community identification and sampling method analysis. Environ. Entomol. 44, 488–498. doi: 10.1093/ee/nvv001
Gittleman, J. L., and Kot, M. (1990). Adaptation: statistics and a null model for estimating phylogenetic effects. Syst. Zool. 39, 227–241. doi: 10.2307/2992183
González, E., Salvo, A., and Valladares, G. (2020). Insects moving through forest-crop edges: a comparison among sampling methods. J Insect Conserv 24, 249–258. doi: 10.1007/s10841-019-00201-6
Holland, J. M., Bianchi, F. J., Entling, M. H., Moonen, A. C., Smith, B. M., Jeanneret, P., et al. (2016). Structure, function and management of semi-natural habitats for conservation biological control: a review of European studies. Pest Manage. Sci. 72, 1638–1651. doi: 10.1002/ps.4318
Holzschuh, A., Dormann, C. F., Tscharntke, T., and Steffan-Dewenter, I. (2013). Mass-flowering crops enhance wild bee abundance. Oecologia 172, 477–484. doi: 10.1007/s00442-012-2515-5
Hothorn, T., Zeileis, A., Farebrother, R. W., Cummins, C., Millo, G., Mitchell, D., et al. (2015). Package ‘lmtest’. Testing Linear Regression Models. Available online at: https://cran.r-project.org/web/packages/lmtest/lmtest.~pdf (accessed February 15, 2023).
Howlett, B. G., Walker, M. K., Newstrom-Lloyd, L. E., Donovan, B. J., and Teulon, D. A. J. (2009). Window traps and direct observations record similar arthropod flower visitor assemblages in two mass flowering crops. J. Appl. Entomol. 133, 553–564. doi: 10.1111/j.1439-0418.2009.01395.x
Hsieh, T. C., Ma, K. H., and Chao, A. (2016). iNEXT: an R package for rarefaction and extrapolation of species diversity (Hill numbers). Methods. Ecol. E7, 1451–1456. doi: 10.1111/2041-210X.12613
Huda, A. N., Salmah, M., Hassan, A. A., Hamdan, A., and Razak, M. (2015). Pollination services of mango flower pollinators. J. Insect Sci. 15, 90. doi: 10.1093/jisesa/iev090
Jauker, F., Speckmann, M., and Wolters, V. (2016). Intra-specific body size determines pollination effectiveness. Basic Appl. Ecol. 17, 714–719. doi: 10.1016/j.baae.2016.07.004
Kehinde, T., and Samways, M. J. (2012). Endemic pollinator response to organic vs. conventional farming and landscape context in the Cape Floristic Region biodiversity hotspot. Agric. Ecosyst. Environ. 146, 162–167. doi: 10.1016/j.agee.2011.10.020
Klein, A. M., Vaissiere, B. E., Cane, J. H., Steffan-Dewenter, I., Cunningham, S. A., Kremen, C., et al. (2007). Importance of pollinators in changing landscapes for world crops. P Roy Soc. B-Biol. Sci. 274, 303–313. doi: 10.1098/rspb.2006.3721
Knuff, A. K., Winiger, N., Klein, A. M., Segelbacher, G., and Staab, M. (2019). Optimizing sampling of flying insects using a modified window trap. Methods Ecol. E10, 1820–1825. doi: 10.1111/2041-210X.13258
Krahner, A., Schmidt, J., Maixner, M., Porten, M., and Schmitt, T. (2021). Evaluation of four different methods for assessing bee diversity as ecological indicators of agro-ecosystems. Ecol. Indic. 125, 107573. doi: 10.1016/j.ecolind.2021.107573
Kwaiser, K. S., and Hendrix, S. D. (2008). Diversity and abundance of bees (Hymenoptera: Apiformes) in native and ruderal grasslands of agriculturally dominated landscapes. Agric. Ecosyst. Environ. 124, 200–204. doi: 10.1016/j.agee.2007.09.012
Lamarre, G. P. A., Molto, Q., Fine, P. V. A., and Baraloto, C. (2012). A comparison of two common flight interception traps to survey tropical arthropods. Zookeys 216, 43–55. doi: 10.3897/zookeys.216.3332
Larkin, M., and Stanley, D. A. (2021). Impacts of management at a local and landscape scale on pollinators in semi-natural grasslands. J. Appl. Ecol. 58, 2505–2514. doi: 10.1111/1365-2664.13990
Le Féon, V., Schermann-Legionnet, A., Delettre, Y., Aviron, S., Billeter, R., Bugter, R., et al. (2010). Intensification of agriculture, landscape composition and wild bee communities: a large scale study in four European countries. Agric. Ecosyst. Environ. 137, 143–150. doi: 10.1016/j.agee.2010.01.015
LeBuhn, G., and Vargas Luna, J. (2021). Pollinator decline: what do we know about the drivers of solitary bee declines? Curr. Opinion Insect Sci. 46, 106–111. doi: 10.1016/j.cois.2021.05.004
Liu, B., Yang, L., Yang, F., Wang, Q., Yang, Y., Lu, Y., et al. (2016). Landscape diversity enhances parasitism of cotton bollworm (Helicoverpa armigera) eggs by Trichogramma chilonis in cotton. Biol. Control 93, 15–23. doi: 10.1016/j.biocontrol.2015.11.004
Lou, W., Yao, Y., Sun, K., Deng, S., and Yang, M. (2019). Variability of heat waves and recurrence probability of the severe 2003 and 2013 heat waves in Zhejiang Province, southeast China. Clim. Res. 79, 63–75. doi: 10.3354/cr01578
Lowder, S. K., Skoet, J., and Raney, T. (2016). The number, size, and distribution of farms, smallholder farms, and family farms worldwide. World Dev. 87, 16–29. doi: 10.1016/j.worlddev.2015.10.041
McCravy, K. W., Geroff, R. K., and Gibbs, J. (2019). Bee (Hymenoptera: Apoidea: Anthophila) functional traits in relation to sampling methodology in a restored tallgrass prairie. Florida Entomol. 102, 134–140. doi: 10.1653/024.102.0122
Mesa, L. A., Howlett, B. G., Grant, J. E., and Didham, R. K. (2013). Changes in the relative abundance and movement of insect pollinators during the flowering cycle of Brassica rapa crops: implications for Gene Flow. J. Insect Sci. 13, 1–18. 18. doi: 10.1673/031.013.1301
Morandin, L. A., and Winston, M. L. (2005). Wild bee abundance and seed production in conventional, organic, and genetically modified canola. Ecol Appl 15, 871–881. doi: 10.1890/03-5271
Morandin, L. A., Winston, M. L., Abbott, V. A., and Franklin, M. T. (2007). Can pastureland increase wild bee abundance in agriculturally intense areas? Basic Appl Ecol 8, 117–124. doi: 10.1016/j.baae.2006.06.003
Oksanen, J., Blanchet, F. G., Friendly, M., Kindt, R., Legendre, P., McGlinn, D., et al. (2019). Vegan: Community ecology package. R Package Version. 2.0–10.
Ouvrard, P., and Jacquemart, A.-. L. (2019). Review of methods to investigate pollinator dependency in oilseed rape (Brassica napus). Field Crops Res. 231, 18–29. doi: 10.1016/j.fcr.2018.11.006
Papanikolaou, A. D., Kühn, I., Frenzel, M., and Schweiger, O. (2017). Semi-natural habitats mitigate the effects of temperature rise on wild bees. J Appl Ecol 54, 527–536. doi: 10.1111/1365-2664.12763
Paradis, E., and Schliep, K. (2019). ape 5.0: an environment for modern phylogenetics and evolutionary analyses in R. Bioinformatics 35, 526–528. doi: 10.1093/bioinformatics/bty633
Perrot, T., Gaba, S., Roncoroni, M., Gautier, J. L., and Bretagnolle, V. (2018). Bees increase oilseed rape yield under real field conditions. Agric. Ecosyst. Environ. 266, 39–48. doi: 10.1016/j.agee.2018.07.020
Pinheiro, J., Bates, D., DebRoy, S., Sarkar, D., Heisterkamp, S., Van Willigen, B., et al. (2017). Package ‘nlme’. Linear and Nonlinear Mixed Effects Models, Version 3.1–162. Available online at: https://cran.r-project.org/web/packages/nlme/nlme.pdf (accessed March 13, 2023).
Portman, Z. M., Bruninga-Socolar, B., and Cariveau, D. P. (2020). The state of bee monitoring in the United States: a call to refocus away from bowl traps and towards more effective methods. Annal. Entomol. Soc. Am. 113, 337–342. doi: 10.1093/aesa/saaa010
Potts, S. G., Biesmeijer, J. C., Kremen, C., Neumann, P., Schweiger, O., Kunin, W. E., et al. (2010). Global pollinator declines: trends, impacts and drivers. Trends Ecol. Evol. 25, 345–353. doi: 10.1016/j.tree.2010.01.007
Potts, S. G., Imperatriz-Fonseca, V., Ngo, H. T., Aizen, M. A., Biesmeijer, J. C., Breeze, T. D., et al. (2016). Safeguarding pollinators and their values to human well-being. Nature 540, 220–229. doi: 10.1038/nature20588
Prendergast, K. S., Menz, M. H. M., Dixon, K. W., and Bateman, P. W. (2020). The relative performance of sampling methods for native bees: an empirical test and review of the literature. Ecosphere 11, e03076. doi: 10.1002/ecs2.3076
R Core Team. (2016). R: A Language and Environment for Statistical Computing. Vienna: R Foundation for Statistical Computing.
Rader, R., Bartomeus, I., Tylianakis, J. M., and Lalibert,é, E. (2014). The winners and losers of land use intensification: pollinator community disassembly is non-random and alters functional diversity. Divers. Distrib. 20, 908–917. doi: 10.1111/ddi.12221
Raderschall, C. A., Bommarco, R., Lindström, S. A. M., and Lundin, O. (2021). Landscape crop diversity and semi-natural habitat affect crop pollinators, pollination benefit and yield. Agric. Ecosyst. Environ. 306, 107189. doi: 10.1016/j.agee.2020.107189
Rhodes, C. J. (2018). Pollinator decline - an ecological calamity in the making? Sci. Prog. 101, 121–160. doi: 10.3184/003685018X15202512854527
Ricotta, C., and Podani, J. (2017). On some properties of the Bray-Curtis dissimilarity and their ecological meaning. Ecol. Compl. 31, 201–205. doi: 10.1016/j.ecocom.2017.07.003
Roulston, T. H., Smith, S. A., and Brewster, A. L. (2007). A comparison of pan trap and intensive net sampling techniques for documenting a bee (Hymenoptera : Apiformes) fauna. J. Kansas Entomol. Soc. 80, 179–181. doi: 10.2317/0022-8567(2007)80[179:ACOPTA]2.0.CO;2
Rubene, D., Schroeder, M., and Ranius, T. (2015). Estimating bee and wasp (Hymenoptera: Aculeata) diversity on clear-cuts in forest landscapes - an evaluation of sampling methods. Insect Conserv. Div. 8, 261–271. doi: 10.1111/icad.12105
Shi, X., Axmacher, J. C., Chong, H., Xiao, H., Luo, S., Xu, H., et al. (2022a). Effects of farmland consolidation in southern China on wild bee species composition, nesting location and body size variations. Agric. Forest Entomol. 24, 371–376. doi: 10.1111/afe.12500
Shi, X., Fu, D., Xiao, H., Hodgson, J. A., Yan, D., Zou, Y., et al. (2022b). Comparison between window traps and pan traps in monitoring flower-visiting insects in agricultural fields. Bullet. Entomol. Res. 112, 1–6. doi: 10.1017/S0007485322000104
Shi, X., Xiao, H., Luo, S., Hodgson, J. A., Bianchi, F. J. J. A., He, H., et al. (2021). Can landscape level semi-natural habitat compensate for pollinator biodiversity loss due to farmland consolidation? Agric., Ecosyst. Environ. 319, 107519. doi: 10.1016/j.agee.2021.107519
Steffan-Dewenter, I., Potts, S. G., and Packer, L. (2005). Pollinator diversity and crop pollination services are at risk. Trends Ecol. Evol. 20, 651–652. doi: 10.1016/j.tree.2005.09.004
Steven, J. C., Rooney, T. P., Boyle, O. D., and Waller, D. M. (2003). Density-dependent pollinator visitation and self-incompatibility in upper great lakes populations of Trillium grandiflorum. J. Torrey Bot. Soc. 130, 23–29. doi: 10.2307/3557522
Templ, B., Mózes, E., Templ, M., Földesi, R., Szirák, Á., Báldi, A., et al. (2019). Habitat-dependency of transect walk and pan trap methods for bee sampling in farmlands. J. Apicult. Sci. 63, 93–115. doi: 10.2478/jas-2019-0014
Thompson, A., Frenzel, M., Schweiger, O., Musche, M., Groth, T., Roberts, S. P. M., et al. (2021). Pollinator sampling methods influence community patterns assessments by capturing species with different traits and at different abundances. Ecol. Indic. 132, 108284. doi: 10.1016/j.ecolind.2021.108284
Tscharntke, T., Klein, A. M., Kruess, A., Steffan-Dewenter, I., and Thies, C. (2005). Landscape perspectives on agricultural intensification and biodiversity - ecosystem service management. Ecol. Lett. 8, 857–874. doi: 10.1111/j.1461-0248.2005.00782.x
Tucker, E. M., and Rehan, S. M. (2018). Farming for bees: annual variation in pollinator populations across agricultural landscapes. Agric. Forest Entomol. 20, 541–548. doi: 10.1111/afe.12287
Van Drunen, S. G., Linton, J. E., Kuwahara, G., and Ryan Norris, D. (2022). Flower plantings promote insect pollinator abundance and wild bee richness in Canadian agricultural landscapes. J Insect Conserv. 26, 375–386. doi: 10.1007/s10841-022-00400-8
Vrdoljak, S. M., and Samways, M. J. (2012). Optimising coloured pan traps to survey flower visiting insects. J Insect Conserv 16, 345–354. doi: 10.1007/s10841-011-9420-9
Westerberg, L., Berglund, H.-.L., Jonason, D., and Milberg, P. (2021). Color pan traps often catch less when there are more flowers around. Ecol. Evol. 11, 3830–3840. doi: 10.1002/ece3.7252
Westphal, C., Bommarco, R., Carre, G., Lamborn, E., Morison, N., Petanidou, T., et al. (2008). Measuring bee diversity in different european habitats and biogeographical regions. Ecol. Monogr. 78, 653–671. doi: 10.1890/07-1292.1
Wu, P., Axmacher, J. C., Song, X., Zhang, X., Xu, H., Chen, C., et al. (2018). Effects of plant diversity, vegetation composition, and habitat type on different functional trait groups of wild bees in rural Beijing. J. Insect Sci. 18, 65. doi: 10.1093/jisesa/iey065
Wu, P. L., Axmacher, J. C., Li, X. D., Song, X., Yu, Z. R., Xu, H. L., et al. (2019). Contrasting effects of natural shrubland and plantation forests on bee assemblages at neighboring apple orchards in Beijing, China. Biol. Conserv. 237, 456–462. doi: 10.1016/j.biocon.2019.07.029
Zou, Y., Bianchi, F. J. J. A., Jauker, F., Xiao, H. J., Chen, J. H., Cresswell, J., et al. (2017). Landscape effects on pollinator communities and pollination services in small-holder agroecosystems. Agr. Ecosyst. Environ. 246, 109–116. doi: 10.1016/j.agee.2017.05.035
Keywords: pan trap, window trap, flight interception trap, mass-flowering crop, smallholder farmland, pollinator monitoring, pollinator diversity
Citation: Shi X-Y, Orr M, Luo A, Wang M-Q, Guo P, Zhou Q-S, Niu Z, Qiao H, Zou Y and Zhu C-D (2023) Optimizing low-cost sampling of pollinator insects in oilseed rape fields. Front. Sustain. Food Syst. 7:1155458. doi: 10.3389/fsufs.2023.1155458
Received: 31 January 2023; Accepted: 07 March 2023;
Published: 28 March 2023.
Edited by:
Xingyuan Men, Shandong Academy of Agricultural Sciences, ChinaReviewed by:
Wenhao Hu, Zhejiang A&F University, ChinaCopyright © 2023 Shi, Orr, Luo, Wang, Guo, Zhou, Niu, Qiao, Zou and Zhu. This is an open-access article distributed under the terms of the Creative Commons Attribution License (CC BY). The use, distribution or reproduction in other forums is permitted, provided the original author(s) and the copyright owner(s) are credited and that the original publication in this journal is cited, in accordance with accepted academic practice. No use, distribution or reproduction is permitted which does not comply with these terms.
*Correspondence: Chao-Dong Zhu, emh1Y2RAaW96LmFjLmNu
Disclaimer: All claims expressed in this article are solely those of the authors and do not necessarily represent those of their affiliated organizations, or those of the publisher, the editors and the reviewers. Any product that may be evaluated in this article or claim that may be made by its manufacturer is not guaranteed or endorsed by the publisher.
Research integrity at Frontiers
Learn more about the work of our research integrity team to safeguard the quality of each article we publish.